- Department of Neuroscience, Medical University of South Carolina, Charleston, SC, United States
Substance use induces long-lasting behavioral changes and drug craving. Increasing evidence suggests that epigenetic gene regulation contributes to the development and expression of these long-lasting behavioral alterations. Here we systematically review extensive evidence from rodent models of drug-induced changes in epigenetic regulation and epigenetic regulator proteins. We focus on histone acetylation and histone methylation in a brain region important for drug-related behaviors: the nucleus accumbens. We also discuss how experimentally altering these epigenetic regulators via systemically administered compounds or nucleus accumbens-specific manipulations demonstrate the importance of these proteins in the behavioral effects of drugs and suggest potential therapeutic value to treat people with substance use disorder. Finally, we discuss limitations and future directions for the field of epigenetic studies in the behavioral effects of addictive drugs and suggest how to use these insights to develop efficacious treatments.
Introduction
Substance use disorder (SUD) is defined by the DSM-5 as problematic patterns of “using alcohol or another substance that results in impairment in daily life or noticeable distress” (American Psychiatric Association, 2013). SUD contributes to major health problems in society, like the current opioid crisis in the United States (Seth et al., 2018; Volkow and Blanco, 2021). People suffering from SUD can severely impact their own personal health and negatively impact society around them, but we still only have a limited understanding of how a SUD is formed and maintained in the brain. Of particular note, our knowledge of how substance use-promoting mechanisms in the brain are maintained for years or even decades after the last use of a substance is incomplete. One possible mechanism for these long-lasting changes in the brain that promote SUD involves epigenetic changes. Epigenetic mechanisms provide a molecular basis for long-term gene regulation following interactions with the environment like using addictive substances repeatedly over time. Understanding these mechanisms is a major goal of epigenetic research on SUD. Below we will discuss how epigenetic regulation occurs, some of the evidence for epigenetic regulation in SUD in humans and in rodent models, and some challenges facing the field going forward.
Introduction to Drug-Related Behaviors
Most of the references below discuss findings from rodent models of SUD. Broadly speaking, rodent models can be separated into two classes.
Experimenter administered (non-contingent) models. These include conditioned place preference (CPP), locomotor sensitization, and alcohol vapor exposure where the rodents have no choice in drug exposure.
Self-administration (contingent) models. These models allow the rodents more choice over when to take drugs. These assays include alcohol drinking (2-bottle choice, drinking-in-the-dark) and drug self-administration (heroin, cocaine, methamphetamine, nicotine, etc.).
As we will detail below, these contingent and non-contingent experimental models sometimes indicate a similar role of epigenetic regulators in the development or maintenance of drug reward and/or conditioned behaviors. However, in other cases, similar manipulations produce different effects depending on the behavior. In this text, we refer to one or more of these behaviors (regardless of contingent or non-contingent) as “drug-related” behaviors.
Genetic Versus Epigenetic Mechanisms of Substance Use Disorder
Drug-related behaviors can be influenced by both genetic and epigenetic mechanisms.
Genetic Mechanisms of Substance Use Disorder
Genetic mechanisms involve inheritable DNA base pair differences, and a consensus of the field is that about 50% of the vulnerability to develop a SUD is genetic (Wang et al., 2012; Reilly et al., 2017). For instance, clear genetic effects exist for certain alleles of alcohol dehydrogenase (ALD) to reduce excessive alcohol use (Wang et al., 2012), and adoption studies have shown that genetic inheritance plays a stronger role than an individual’s familial environment in predicting who will develop alcohol use disorders (Schuckit et al., 1972; Goodwin et al., 1973, 1974, 1977; Reilly et al., 2017).
Epigenetic Mechanisms of Substance Use Disorder
In contrast to genetics, epigenetics in its simplest definition means “above” or “on top of” (“epi” – Greek) genetics and broadly refers to the ability to induce long-lasting changes based on environmental influences instead of DNA base pair differences. The term “epigenetics” has many definitions though (Deans and Maggert, 2015; Allis and Jenuwein, 2016), and here we will discuss the two most common in the literature. The first definition refers strictly to transgenerational, inherited changes depending on the environment of the offspring’s parents. The second definition involves the regulation of gene expression through changes in DNA methylation, histone post-translational modifications (PTMs), and chromatin structure. We discuss evidence for each of these below.
Transgenerational Epigenetic Mechanisms
Epigenetic mechanisms can influence drug-related behaviors of offspring. For instance, if rats self-administer cocaine before they reproduce, their male offspring will - surprisingly - have reductions in cocaine self-administration behavior (Vassoler et al., 2013) and cocaine locomotor sensitization in the 1st generation (F1), but not the 2nd (F2) generation (Wimmer et al., 2019). A similar finding was reported for morphine exposed fathers and their F1 and F2 offspring (Vassoler et al., 2017). In addition, nicotine exposed males sire F1 generation offspring with increased spontaneous locomotor activity and learning deficits. Furthermore, males in the F2 generation also display deficits in learning (McCarthy et al., 2018). In addition, similar findings have been shown in alcohol models, where males that had chronic alcohol exposure sire F1 offspring that later display reductions in alcohol self-administration (Nieto et al., 2022). Somewhat in contrast to these studies, however, other studies have suggested that the offspring of rats exposed to cocaine have increases in the motivation for cocaine in the F1 and F2 generations (Le et al., 2017). Together, this indicates that while most reports do show transgenerational effects, their results are not always similar. Despite the evidence for transgenerational epigenetic effects, most studies of epigenetic mechanisms in rodent models focus on a different aspect of substance-induced epigenetic regulation.
Environmental Epigenetic Mechanisms
A second definition of epigenetics is the regulation of gene expression caused by environmental changes. This definition is similar to our previous review (Anderson et al., 2018b) and other reviews in the field (Jaenisch and Bird, 2003; Nestler, 2013; Kenny, 2014; Allis and Jenuwein, 2016; Werner et al., 2021). These gene expression changes can occur through alterations in DNA methylation, histone post-translational modifications (PTMs), and chromatin structure as detailed below.
DNA Methylation. DNA can be methylated on cytosine residues when they are immediately followed by a guanine residue (CpG sites). These methylation marks can inhibit or promote transcription depending on their location on DNA (Christman et al., 1977; Bird and Southern, 1978; Desrosiers et al., 1979; Jones and Taylor, 1980) by reducing the binding of transcription initiators or by recruiting repressor proteins like methyl-CpG binding protein 2 (MeCp2) (Meehan et al., 1989, 1992; Lewis et al., 1992; Deng et al., 2010, 2014).
Histone Post-translational Modifications. DNA in the nucleus is wrapped around sets of 8 proteins called histones to form a nucleosome, the basic structural unit of the chromosome. There are several types of histones including H2A, H2B, H3, and H4 (Luger et al., 1997) and they can undergo a variety of PTMs on their N-terminal tails that can influence transcription and form the basis of the “histone code” (Allis and Jenuwein, 2016).
Histone Acetylation
The first such regulation discovered was that increased acetylation of histones results in increased transcription (Allfrey et al., 1964). This effect may be caused by increased physical accessibility for transcriptional machinery due to an electrostatic repulsion of the negatively charged phosphates of DNA by negatively charged acetyl groups on histones (Sterner and Berger, 2000; Eberharter and Becker, 2002). In addition, acetylation also recruits regulatory factors like the bromodomain-containing protein Creb-binding protein (CBP) that has histone acetyltransferase activity (HAT) and can increase transcription (Hong et al., 1993; Grunstein, 1997; Yang, 2004; Bannister and Kouzarides, 2011).
Histone Methylation
Histone methylation is another common histone PTM, where a methyl group is attached to a lysine or arginine (Di Lorenzo and Bedford, 2011; Benevento et al., 2015; Zhang et al., 2015). Like acetylation however, methylation also recruits regulatory factors like heterochromatin protein 1 (HP1) to alter transcription (Lachner et al., 2001; Bannister and Kouzarides, 2011; Benevento et al., 2015).
Other Post-translational Modifications
Many more PTMs exist like phosphorylation, sumoylation, ubiquitination, and ADP-ribosylation; however, we will not discuss these in this review.
Chromatin Structure. Histone- and DNA-containing nucleosomes are grouped together into chromatin. Chromatin can consist of dense, compact regions that are transcriptionally repressed and not actively transcribed known as heterochromatin. Heterochromatin can also be subcategorized into constitutive (condensed/not transcribed) and facultative (loose/transcribable under certain conditions). Chromatin can also exist in forms that are easily transcribed known as euchromatin (Huisinga et al., 2006; Delcuve et al., 2009). Drug exposure has been shown to alter chromatin accessibility status through epigenetic mechanisms through DNA methylation and histone PTMs and these mechanisms likely act in concert with one another as we previously described (Anderson et al., 2018b).
Importantly, in this review, we will focus on histone acetylation and histone methylation (Figure 1), but DNA methylation and chromatin structure are also altered by drug exposure in rodent models (Deng et al., 2010, 2014; Massart et al., 2015; Werner et al., 2021).
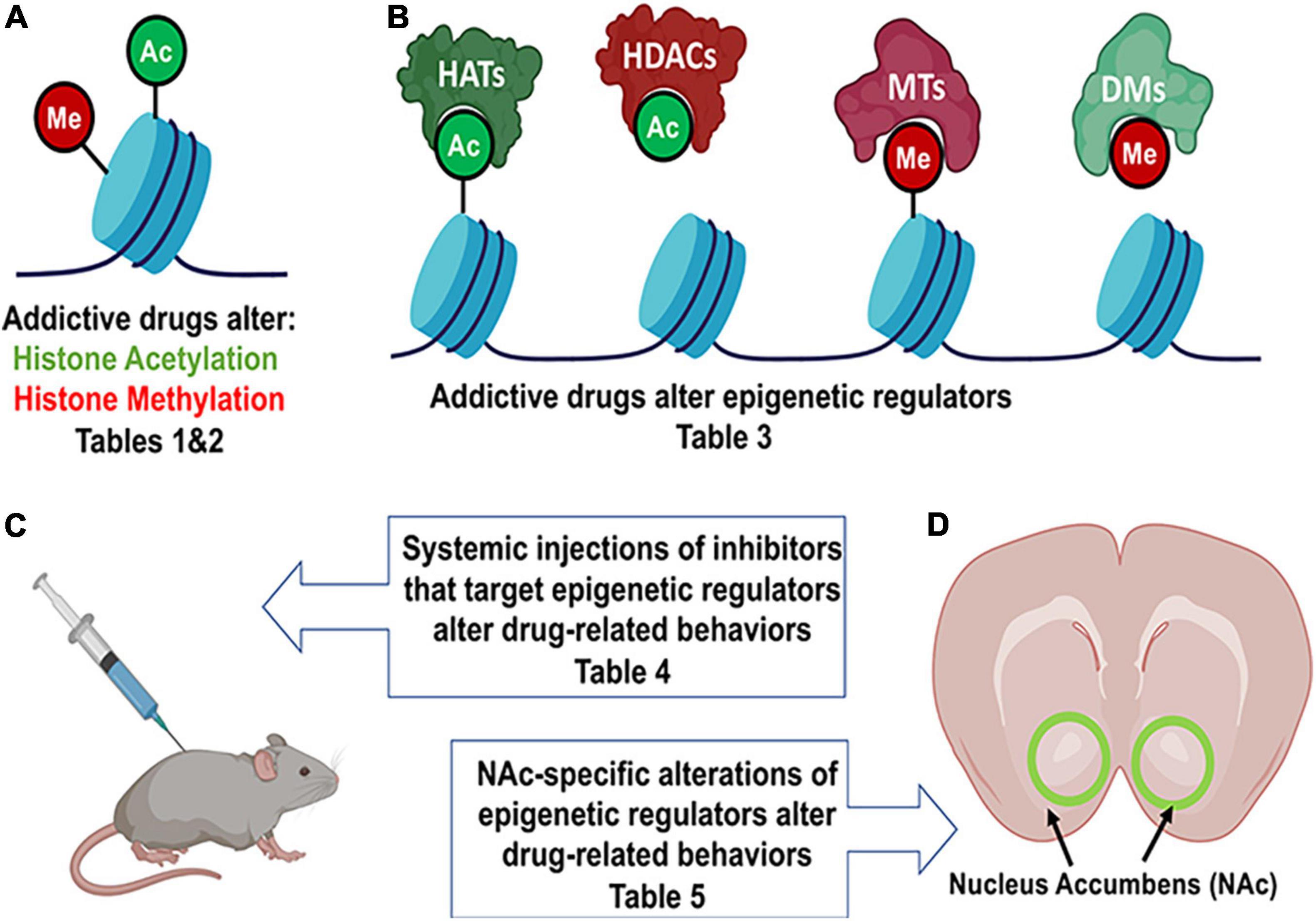
Figure 1. Drug exposure induces changes in epigenetic regulation in the nucleus accumbens and alters behavior. (A) Histone modifications are a form of epigenetic regulation that can alter DNA transcription. Two well studied modifications are acetylation (Ac) and methylation (Me). Increases in acetylation typically increase transcription and are thought to be “go” signals (green). In contrast, increases in methylation typically decrease transcription and are considered “stop” signals (red). Drug exposure has been shown to cause changes in histone acetylation (Table 1) and histone methylation (Table 2). (B) Histone acetylation levels of histones can be increased by histone acetyltransferases (HATs) and decreased by histone deacetylases (HDACs). Histone methylation levels can be increased by methyltransferases (MTs) and reduced by demethylases (DMs). Epigenetic proteins that modify histone acetylation and histone methylation are altered by drug exposure (Table 3). (C) Altering the activity of epigenetic proteins via systemic injection of inhibitor or activator compounds can alter drug-related behaviors in rodent models (Table 4). (D) Finally, accumbens-specific manipulations of epigenetic proteins can also alter drug-related behaviors (Table 5). This figure was created in part with biorender.com.
Epigenetic Regulation in the Nucleus Accumbens
The NAc is part of the endogenous reward system and is critically involved in behavioral effects of addictive drugs. Drug exposure activates this system acutely but overtime are hypothesized to “hijack” this circuitry to increase drug-seeking (Nesse and Berridge, 1997). Many studies have examined epigenetic regulation in the NAc and found that many changes in histone acetylation and histone methylation occur after exposure to drugs (Anderson et al., 2018b; Werner et al., 2021). Though many areas in the brain show drug-induced epigenetic regulation like the dorsal striatum (Li et al., 2018), central nucleus of the amygdala, and orbitofrontal cortex (Cates et al., 2018), this review will only focus on the nucleus accumbens (NAc) (Figure 1). Here we summarize the literature in several tables that can be sorted by drug, type of administration (acute, chronic, or self-administered) or by the various drug-induced change in histone acetylation (Table 1) and histone methylation (Table 2) marks reported.
Effects of Drug Exposure on Histone Acetylation
Many addictive drugs cause changes in histone acetylation, including cocaine, methamphetamine, ethanol, opioids, MDMA, THC, toluene, and nicotine.
Cocaine
Cocaine exposure alters many histone acetylation marks in the NAc (Table 1). Cocaine exposure typically increases global acetylation of the histones H3 and H4 in the NAc, likely by increasing individual sites like H2K12, H3K9, H3K14, H3K27, H4K5, H4K8, and H4K16 (Kumar et al., 2005; Cleck et al., 2008; Renthal et al., 2009; Wang et al., 2010; Levine et al., 2011; Malvaez et al., 2011; Kennedy et al., 2013; Rogge et al., 2013; Ferguson et al., 2015; Li Y. et al., 2015; Wimmer et al., 2019; Carpenter et al., 2020; Campbell et al., 2021). Some of these changes in acetylation reflect global changes from whole NAc tissue, but others reflect specific changes at certain promoters (see Table 1 for details). Cocaine-induced decreases in acetylation have also been reported for H3ac, H3K18, H4ac, and H4K12 (Renthal et al., 2009; Malvaez et al., 2011; Wimmer et al., 2019). Cocaine can alter histone acetylation very quickly, but can also produce long-lasting changes as the findings reflect a range of timepoints following the last exposure to cocaine from 20 min to 28 days (Levine et al., 2011; Carpenter et al., 2020). Importantly, while most of these studies used experimenter (non-contingent) exposure, self-administered (contingent) cocaine similarly increases acetylation of histone H3 and H4 at certain promoters 3-24 h after the last self-administration (Kumar et al., 2005; Wang et al., 2010). These data show that exposure to cocaine rapidly changes histone acetylation in many gene promoters, and at least some of these changes can last up to 28 days later.
Methamphetamine
Non-contingent methamphetamine exposure increases pan-H3 acetylation, H4ac at a specific promoter, H4K5, and H4K8 (Shibasaki et al., 2011; Martin et al., 2012). Non-contingent methamphetamine also decreases H3K9 and H3K18 up to 24 hrs later (Martin et al., 2012).
Ethanol
Non-contingent ethanol exposure increases pan-H4 acetylation, and the specific marks H3K14, H3K9, and H4K12 (Pascual et al., 2009; Botia et al., 2012; Sprow et al., 2014; Finegersh et al., 2015). In contrast, self-administered ethanol reduces pan-H4 acetylation (Warnault et al., 2013) and increases H3K27 acetylation (Griffin et al., 2017). These findings suggest that at least some differences (pan-H4 acetylation) are found between contingent and non-contingent rodent models of SUD.
Opioids
Heroin primed reinstatement of drug-seeking behavior following heroin self-administration increases acetylation of H3K18, H4K5, and H4K8 (Chen et al., 2016). Naloxone-precipitated withdrawal administration after chronic non-contingent morphine exposure reduces H3K14 acetylation in the NAc shell (Ciccarelli et al., 2013).
MDMA
Non-contingent MDMA changes H3K9 acetylation at specific promoters (Caputi et al., 2016).
THC
Non-contingent THC increases H3K14ac at 24hrs after the last exposure but then decreases by 48 hrs after the last exposure (Prini et al., 2017).
Toluene
Chronic non-contingent exposure to toluene increases pan-H3 acetylation in the NAc (Sanchez-Serrano et al., 2011).
Nicotine
Chronic nicotine exposure for 7 days through drinking water increases the acetylation level of Histone H3K9 and H4K5-K16 (Levine et al., 2011).
Combined, these studies suggest that most psychoactive, addictive drugs alter histone acetylation in the NAc and highlight that some of these changes may be short-lived and very dynamic (Prini et al., 2017).
Effects of Drugs Exposure on Histone Methylation
The nucleus accumbens also undergoes changes in methylated histone marks following exposure to addictive drugs like cocaine, methamphetamine, ethanol, opioids, MDMA, and THC (Table 2).
Cocaine
Non-contingent cocaine exposure alters many histone methylation sites including H3K27me3, H3K36me3, H3K4me1, H3K4me2, H3K4me3, H3K9/K27me2, H3K9me2, H3K9me3, H3R2me2a, H4K9me3, and H4R3me2a (Adams and Bushell, 1989; Renthal et al., 2009; Maze et al., 2010, 2011; Covington et al., 2011; Malvaez et al., 2011; Damez-Werno et al., 2012, 2016; Kennedy et al., 2013; Caputi et al., 2014; Feng et al., 2014; Li Y. et al., 2015; Chandra et al., 2017; Engmann et al., 2017; Carpenter et al., 2020). These changes include both increases and decreases of methylation at these histone sites (see Table 2 for details on each study). In addition to non-contingent rodent models of SUD, contingent cocaine decreases methylation of H3K9me2 at specific promoters and pan-H3R2me2a as well (Damez-Werno et al., 2016; Wimmer et al., 2019).
Methamphetamine and Amphetamine
Non-contingent methamphetamine exposure decreases H3K27me3 methylation, but increases H3K4me2 and H3K4me3 methylation. Some promoter specific changes remain for at least 24 h (Ikegami et al., 2010; Aguilar-Valles et al., 2014). Amphetamine increases H3K9 methylation on the fos promoter 5 days after the last exposure (Renthal et al., 2008).
Ethanol
Non-contingent alcohol exposure by the chronic intermittent ethanol vapor exposure model alters H3K27me3 and decreases H3K9me2 (Anderson et al., 2021; Johnstone et al., 2021).
Opioids
Non-contingent morphine exposure causes both increases and decreases in H3K9me2 and H3K4me3 that are promoter specific (Sun et al., 2012; Ferguson et al., 2013).
MDMA
Non-contingent MDMA increases H3K27me3 and H3K4me3 at specific promoters and decreases H3K9me2 at others (Caputi et al., 2016).
THC
Non-contingent THC alters H3K9me2 and H3K4me3 levels, some at specific promoters (Tomasiewicz et al., 2012; Prini et al., 2017). Of note, THC causes bidirectional changes in H3K9me2 over 1 vs 2 days after the last exposure (Prini et al., 2017) suggesting some of these histone changes may be very short-lived and highly dynamic. Also, some of these THC-induced changes last up to 30 days later at specific promoters (Tomasiewicz et al., 2012).
General Conclusions on Drug-Altered Histone Post-translational Modifications
Some similar general conclusions can be drawn when examining both histone acetylation and histone methylation following exposure to drugs exposure (Tables 1, 2).
First, different drugs cause different changes in the histone marks. This suggests no clear common “histone code” for drug exposure in the NAc. For instance, cocaine, ethanol, and THC lead to increases in H3K14ac cocaine (Malvaez et al., 2011; Kennedy et al., 2013; Finegersh et al., 2015; Prini et al., 2017), but morphine leads to a decrease (Ciccarelli et al., 2013). Differences in study design and timepoints could affect these findings, for instance, H3K14ac is increased 24 h after THC, but reduced 48 h later (Prini et al., 2017).
Second, most of these histone PTM changes are present at very early timepoints following the last exposure to an addictive drug. In addition, these histone acetylation and histone methylation changes appear to occur very rapidly - even after an acute dose (Martin et al., 2012; Godino et al., 2015) - and are likely highly dynamic or short-lived. In other words, there are large signaling changes in epigenetic marks shortly after the last drug exposure, but most of these changes appear to return to baseline levels following longer timescales.
Third, a small subset of changes at certain gene promoters appear to persist for longer periods of time after the last drug exposure. For instance, increased H3 acetylation at the BDNF promoter (an important mediator of drug-related behaviors (Graham et al., 2007; Bahi et al., 2008; Lobo et al., 2010; Li et al., 2013; Anderson et al., 2017) is observed after 7d withdrawal (Kumar et al., 2005) and increased methylation of H4R3me2a is observed at both 1d and 7d (but not 14d) withdrawal from cocaine (Li Y. et al., 2015). Cocaine also leads to a stable decrease in H3K9me2 at the D2 promoter after a month of withdrawal in rats bred for high responding (Flagel et al., 2016). In addition, THC causes lasting changes at H3K9, as a decrease in methylation is observed at the proenkephalin gene promoter at both 1d and 30d withdrawal (Tomasiewicz et al., 2012). Also, chronic intermittent alcohol vapor exposure decreases H3K27me3 after 3 weeks of withdrawal (Johnstone et al., 2021). Finally, cocaine causes an increase in H3K27ac and H3K4me3 at the cartpt promoter that was found at both 1d and 28d of abstinence. This same study also found that H3K27me3 was increased after 1d of abstinence, but was reduced after 28d of abstinence (Carpenter et al., 2020). So, while all classes of drugs exposure led to short term changes in histone marks, at least some of these changes may remain for longer periods of time and could possibly cause long-lasting behavioral changes.
Effects of Drugs on Epigenetic Regulators in the Nucleus Accumbens
Addictive drugs also cause changes to the proteins that regulate histone marks in the NAc, and this suggests that we can alter these drug-induced histone marks by targeting their epigenetic regulators. As shown in Table 3 there are many known candidates that are regulated by drug exposure in the NAc.
Effects of Drugs on Epigenetic Regulators in the Nucleus Accumbens in Humans
Notably, though we mainly only review rodent studies, several important pieces of evidence in post-mortem human NAc studies demonstrate that drug exposure induces alteration of many epigenetic regulators for histone acetylation and histone methylation. HDAC5 mRNA is downregulated in the NAc of people that use heroin (Egervari et al., 2017). In addition, the methyltransferases G9a and PRMT6 are downregulated in post-mortem NAc tissue from people that take cocaine (Maze et al., 2014; Damez-Werno et al., 2016). Finally, the histone lysine-specific demethylase KDM6B is upregulated in people diagnosed with alcohol use disorder (Johnstone et al., 2021). This down-regulation of methyltransferases and upregulation of demethylases may produce some similar changes in histone marks in the NAc of humans with SUD as compared to rodent models of SUD (Tables 1, 2), but these have not been closely examined yet.
Effects of Addictive Drugs on Epigenetic Regulators in the Nucleus Accumbens in Rodent Models
As shown in Table 3 there are many known candidates that are regulated by drug exposure in the NAc.
Histone Deacetylase Proteins
Many histone deacetylase proteins (HDACs), including Class I (HDAC1, 2, 3, and 8), Class IIa (HDAC4, 5, 7, and 9), Class IIb (HDAC6 and 10), Class III (SirtI and II), and Class IV (HDAC11), are regulated by drug exposure as detailed below.
Class I Histone Deacetylase Proteins (HDAC1, 2, 3, and 8)
HDAC1 protein expression decreases after acute non-contingent methamphetamine exposure for at least 1-16 h (Martin et al., 2012). In contrast, chronic non-contingent cocaine exposure increases the enrichment of HDAC1 on G9a and GLP promoters at 4 hrs after the last drug exposure (Kennedy et al., 2013). Chronic non-contingent amphetamine exposure for consecutive 7 days increases HDAC1 enrichment on the cfos promoter at 5 days after the last exposure (Renthal et al., 2008). HDAC2 expression increases after acute non-contingent exposure to nicotine and methamphetamine, chronic non-contingent exposure to ethanol, and contingent self-administered cocaine (Host et al., 2011; Martin et al., 2012; Faillace et al., 2015; Torres et al., 2015; Sharma et al., 2021). HDAC3 expression and binding to some promoter regions decreases after acute non-contingent exposure to cocaine and methamphetamine (Rogge et al., 2013; Torres et al., 2016). Like HDAC2, chronic exposure to non-contingent cocaine increases HDAC3 expression and binding to some promoters (Campbell et al., 2021). Finally, HDAC8 expression decreases after acute non-contingent methamphetamine exposure (Torres et al., 2016). These data demonstrate that there are distinct effects of different drugs on the expression of class I HDACs, and that both contingent and non-contingent administration can alter HDACs.
Class IIa Histone Deacetylase Proteins (HDAC4, 5, 7, and 9)
Class IIa HDACs are also regulated by drug exposure. HDAC4 expression decreases after acute non-contingent methamphetamine exposure (Torres et al., 2016). Contingent ethanol drinking in rodents increases HDAC4 mRNA expression and decreases protein expression (Griffin et al., 2017; Pozhidayeva et al., 2020). HDAC5 decreases after non-contingent cocaine conditioned place preference conditioning (Rogge et al., 2013) and after contingent chronic ethanol exposure (Pozhidayeva et al., 2020). As noted above, HDAC5 mRNA is similarly downregulated in the NAc of people that use heroin (Egervari et al., 2017). HDAC7 expression decreases for at least 1-8 h following an acute methamphetamine exposure, like HDAC4 (Torres et al., 2016). HDAC9 expression decreases in rodents subjected to chronic non-contingent ethanol exposure after a withdrawal of 3 weeks (Johnstone et al., 2021).
Class IIb Histone Deacetylase Proteins (HDAC6 and 10)
HDAC6 mRNA increases after acute, non-contingent methamphetamine from 1-8 h after the exposure (Torres et al., 2016).
Class III (SirtI and II)
Increased Sirt1 expression and activity is observed at both 4-24 h and 5 days after chronic non-contingent cocaine exposure. Similarly, Sirt2 expression and activity increases after chronic non-contingent cocaine exposure (Renthal et al., 2009; Ferguson et al., 2015). Also, ChIP-seq with Sirt1 analysis identified changes in Sirt1 enrichment on some promoter regions after chronic non-contingent cocaine exposure (Ferguson et al., 2015).
Class IV Histone Deacetylase Proteins
HDAC11 mRNA expression changes after exposure to contingent cocaine self-administration (Host et al., 2011), non-contingent methamphetamine (Torres et al., 2016), and non-contingent ethanol exposure (Botia et al., 2012). In these studies, both acute non-contingent ethanol and chronic ethanol exposure decreases HDAC11 mRNA. Similarly, acute methamphetamine decreases mRNA expression from 1 to 8 hrs. In contrast, contingent cocaine self-administration increases HDAC11 expression at 2 hrs after the last drug exposure.
Histone Acetyltransferases
Histone acetyltransferases (HATs) are also regulated by drug exposure. Both acute and chronic non-contingent cocaine exposure increases the enrichment of Creb-binding protein (CBP) on a specific promoter (Malvaez et al., 2011) (see Table 3 for details). Also, chronic contingent ethanol exposure decreases CBP mRNA expression (Sharma et al., 2021). Finally, lysine acetyltransferase 6A, KAT6A (also known as Myst3) mRNA increases after contingent chronic exposure to ethanol (Wolstenholme et al., 2011) and Atf-2 increases following a non-contingent methamphetamine exposure (Martin et al., 2012).
Histone Lysine Methyltransferases
The histone methyltransferase G9a regulates several histone marks including H3K9me2 and G9a expression in the NAc is reduced by chronic exposure to non-contingent cocaine (Maze et al., 2014), non-contingent morphine (Sun et al., 2012), and both contingent/non-contingent alcohol models (Wolstenholme et al., 2011; Anderson et al., 2021). Consistent with decreasing G9a protein expression after chronic cocaine exposure, G9a enrichment on several gene promoters increase at 1 h after acute non-contingent cocaine exposure and decrease at 24 h after chronic cocaine exposure (Maze et al., 2010). As mentioned above, G9a is also downregulated in humans that use cocaine (Maze et al., 2014). Of note, another histone methyltransferase called G9a-like protein (GLP, also called EHMT1) also decreases following non-contingent cocaine exposure (Maze et al., 2010, 2014). Also, the lysine methyltransferase (KMT) KMT1A (also known as Suv39h1) increases after 7 days of non-contingent amphetamine exposure (Renthal et al., 2008). In addition, KMT2a (also known as Mll1) increases after non-contingent methamphetamine conditioned place preference conditioning (Aguilar-Valles et al., 2014), and the KMTs Setd6 and Smyd3 decrease following both acute and chronic exposure to non-contingent ethanol (Botia et al., 2012).
Protein Arginine Methyltransferases
Protein arginine methyltransferases (PRMTs) like PRMT1 to PRMT6 and PRMT8 to PRMT10 are also altered after drug exposure. PRMT1 was initially reported to increase expression and activity after acute and chronic non-contingent exposure to cocaine, and following contingent cocaine self-administration (Li Y. et al., 2015). In contrast, a later paper reported that PRMT1 decreases after acute and chronic non-contingent cocaine exposure (Damez-Werno et al., 2016), so there is some disagreement in this area. PRMT2, PRMT5, PRMT6, PRMT8, and PRMT9 decrease following chronic non-contingent cocaine exposure (Li Y. et al., 2015; Damez-Werno et al., 2016). PRMT6 also decreases 7 days after the last contingent cocaine self-administration and 28 days after the last non-contingent cocaine exposure (Damez-Werno et al., 2016), suggesting this may be a long-lasting change in the NAc. As noted above, PRMT6 mRNA is similarly downregulated in post-mortem samples from people that take cocaine. PRMT5, PRMT6, and PRMT7 mRNA expression decreases 30 min after a non-contingent ethanol challenge at 17 days after 10 days of chronic non-contingent ethanol exposure (Botia et al., 2012). Finally, PRMT4 decreases at least 3 weeks after chronic intermittent alcohol vapor exposure (Johnstone et al., 2021).
Other Epigenetic Regulators
In addition, other epigenetic regulators are also altered by drugs exposure like the lysine demethylase (KDM) KDM6B. KDM6B mRNA and protein expression were increase and decrease, respectively, at 3 weeks after chronic exposure to non-contingent ethanol (Johnstone et al., 2021). As noted above, KDM6B is similarly upregulated in humans with AUD.
Activity, Localization, Phosphorylation, and Binding Changes of Epigenetic Regulators
Most of the previously mentioned studies measure RNA or protein levels, however some have shown that drug exposure can alter other aspects of protein regulation like nuclear versus cytoplasmic localization of HDAC4 and HDAC5 (Renthal et al., 2009; Taniguchi et al., 2012; Penrod et al., 2018), phosphorylation (Renthal et al., 2009; Taniguchi et al., 2012; Penrod et al., 2018), or their binding activity to genes (Renthal et al., 2009; Maze et al., 2010; Levine et al., 2011; Malvaez et al., 2011; Kennedy et al., 2013; Rogge et al., 2013; Ferguson et al., 2015; Li Y. et al., 2015; Torres et al., 2015; Campbell et al., 2021). These changes suggest that simply examining the up- or -down regulation of mRNA and/or protein levels may be insufficient to understand how epigenetic regulators are altered by addictive drugs.
Conclusion
Many epigenetic regulator proteins are altered by drug exposure. These changes have been observed in rodent studies and human post-mortem studies as well. These findings suggest that at least some preclinical findings translate to the clinic. Finally, this suggests that treatments that can alter drug-related behaviors in preclinical studies may be beneficial clinically to treat SUD.
Effects of Systemically Injected Inhibitors of Epigenetic Regulators on Drug-Related Behaviors
Many studies have altered drug-related behaviors in preclinical rodent studies by systemic or i.c.v. administration of inhibitors/activators of epigenetic proteins. As shown in Table 4, these studies have produced mixed results. The same or similar inhibitor compounds sometimes increase or decrease drug-related behaviors depending on the drug used or the behavioral procedure. We have organized Table 4 based on the type of inhibitor used and described the epigenetic target, drugs, behavioral model, and whether it was reported to increase or decrease drug-taking or drug-seeking behavior.
Histone Deacetylase Protein Activators/inhibitors
Many different compounds that alter HDAC activity have been injected systemically to study their effects on drug-related behaviors.
Histone Deacetylase Protein Inhibitor: Sodium Butyrate
The non-selective HDAC inhibitor sodium butyrate (NaBut) has been shown to alter many drug-related behaviors, but can produce mixed results. For instance, NaBut increases non-contingent psychostimulant- and morphine-induced locomotor sensitization, but in contrast decreases ethanol-induced locomotor sensitization (Kumar et al., 2005; Kalda et al., 2007; Sanchis-Segura et al., 2009; Legastelois et al., 2013). In addition, NaBut increases cocaine and morphine conditioned place preference (CPP) (Sanchis-Segura et al., 2009; Itzhak et al., 2013) and decreases cocaine CPP extinction (Itzhak et al., 2013) in some papers. However, there is some disagreement as other studies show that NaBut increases cocaine CPP extinction (Malvaez et al., 2010), reduces primed reinstatement in cocaine CPP (Malvaez et al., 2010), and decreased nicotine CPP (Pastor et al., 2011). The effects of NaBut administration are thus inconsistent between these studies on non-contingent drug-related behaviors.
The effects of NaBut on contingent self-administration behaviors are also inconsistent as some papers report that NaBut increases cocaine self-administration and increases heroin prime-induced reinstatement (Sun et al., 2008; Chen et al., 2016), but others report it decreases reinstatement to cocaine-seeking and decreases alcohol drinking (Romieu et al., 2011; Simon-O’Brien et al., 2015). These discrepancies could be due to the non-selective nature of NaBut or differences in experimental design.
Histone Deacetylase Protein Inhibitor: Trichostatin A
The non-selective HDAC inhibitor trichostatin A (TSA) also alters drug-related behaviors, but not all studies are consistent. TSA increases cocaine CPP (Kumar et al., 2005) and also increases contingent ethanol intake (Wolstenholme et al., 2011), however, other reports suggest that TSA reduces ethanol drinking (Sakharkar et al., 2014), reduces ethanol withdrawal-induced anxiety (Pandey et al., 2008), and reduces psychostimulant self-administration, sensitization, and reinstatement (Romieu et al., 2008; Host et al., 2010; Arndt et al., 2019). Again, these discrepancies could be due to the non-selective nature of TSA or differences in experimental design.
Other Histone Deacetylase Protein Inhibitors
Less well studied non-selective HDAC inhibitors like phenylbutyrate and depudecin have been shown to reduce contingent cocaine self-administration (Romieu et al., 2008).
Selective Class I and II Histone Deacetylase Protein Inhibitors
Given the many differences in studies following the use of non-selective HDAC inhibitors, more selective inhibitors that act only on a subset of HDACs have also been studied (Table 4). As described above, HDACs can be divided into several classes and Class I includes HDAC1, HDAC2, HDAC3, and HDAC8. Class II includes HDAC4-HDAC7 and HDAC9-HDAC10. Both valproic acid (VPA) and suberoylanilide hydroxamic acid (SAHA) are selective for only these classes of HDACs and not other class III and IV HDACs like SIRT1-7 and HDAC11. These two inhibitors more consistently reduce drug-related behaviors. SAHA reduces contingent ethanol intake, ethanol self-administration, ethanol-seeking (Warnault et al., 2013), and SAHA also increases extinction and reduces non-contingent CPP reinstatement to morphine (Saberian et al., 2021). Like SAHA, VPA also reduces ethanol drinking and ethanol CPP (Al Ameri et al., 2014). However, other studies report that SAHA increases cocaine CPP (Renthal et al., 2007) and VPT increases amphetamine locomotor sensitization (Kalda et al., 2007). These divergent effects could be due to these drugs acting on many different targets.
Class Selective Histone Deacetylase Protein Inhibitors
Inhibitors selective for Class I HDACs (MS275) or Class II HDACs (MC1568) have also been used to alter drug-related behaviors. Interestingly, the Class 1 inhibitor reduces ethanol drinking (Warnault et al., 2013), ethanol self-administration, and reinstatement to ethanol-seeking (Jeanblanc et al., 2015), whereas the Class II inhibitor increases the motivation for cocaine self-administration (Griffin et al., 2017) suggesting different roles for these HDAC classes.
More Selective HDAC Inhibitors
Moving from classes to specific proteins, Compound 60 is a selective inhibitor of HDAC1 and HDAC2 and reduces acute non-contingent amphetamine locomotor behavior (Schroeder et al., 2013). Also, RGFP966 is a selective inhibitor of HDAC3 and increases CPP extinction, blocks reinstatement (Malvaez et al., 2013), and also reduces reinstatement to cocaine seeking (Hitchcock et al., 2019). Finally, LMK235 is an HDAC4/HDAC5 inhibitor that reduces contingent ethanol intake (Pozhidayeva et al., 2020). In general, these reports suggest that more selective HDAC inhibitors may be more consistent in reducing cocaine-seeking and ethanol intake behaviors, though they are still understudied at this point.
Histone Deacetylase Protein Activators
Activators of HDACs have also been studied, but rarely. The HDAC activator theophylline decreases extinction to non-contingent morphine CPP and (Saberian et al., 2021) the SIRT agonist resveratrol increases non-contingent cocaine CPP (Renthal et al., 2009). Since these studies suggest that HDAC activation increases drug-related behaviors, they complement some of the HDAC inhibitor studies that show decreases in drug-related behaviors.
Histone Deacetylase Protein Inhibitor/Activator Conclusions
Examining all these HDAC inhibitor/activator studies together, it is not possible to draw a strong conclusion on their effects on behavior. This could be due to these systemically administered compounds affecting many different brain and/or body regions, but could also be due to differences in experimental design and timing of exposure.
G9a Inhibitors
The systemic G9a inhibitor UNC0642 has recently been shown to reduce stress-induced alcohol drinking (Anderson et al., 2021), suggesting other epigenetic regulators can be targeted with systemic injections as well.
Effects of Nucleus Accumbens-Specific Epigenetic Regulator Manipulations on Drug-Related Behaviors
Since systemic inhibitors likely alter many brain regions, NAc-specific manipulations are more helpful to determine the specific effect of epigenetic regulator proteins in this brain region. Many epigenetic modifiers have been targeted in a NAc-specific manner as thoroughly described in Table 5.
Nucleus Accumbens-Specific Injections of Histone Deacetylase Protein Inhibitors
Several of the epigenetic inhibitors discussed above have also been injected into the NAc specifically including TSA, VPA, SAHA, and MS275. Some data suggest that injecting the non-selective HDAC inhibitors TSA and VPA into the NAc reduces drug-related behaviors like cocaine reinstatement (Romieu et al., 2011), ethanol drinking (Warnault et al., 2013), and amphetamine locomotor sensitization (Kim et al., 2008). In contrast, others suggest the opposite as TSA increases heroin CPP (Sheng et al., 2011), increases ethanol-induced locomotor behavior (Sprow et al., 2014), and increases the motivation for cocaine and cocaine sensitivity using self-administration assays (Wang et al., 2010).
The more selective HDAC inhibitors SAHA and MS275 have also been injected in the NAc. The Class I and Class II HDAC inhibitor SAHA increases CPP (Renthal et al., 2007) and increases the motivation for cocaine and cocaine sensitivity (using dose-response testing) as measured with contingent cocaine self-administration assays (Wang et al., 2010). Finally, the selective Class I HDAC inhibitor MS275 reduces locomotor sensitization when injected into the NAc (Kennedy et al., 2013). These studies demonstrate a NAc-specific effect of epigenetic regulation on drug-related behaviors, however, cannot determine which epigenetic proteins (or combination of proteins) are responsible for these effects.
Nucleus Accumbens-Specific Overexpression and/or Knockdown of Histone Deacetylase Proteins
To understand the role of individual epigenetic regulators in the NAc, many investigators have site-specifically altered the expression of a target protein and examined its effects on drug-related behaviors.
HDAC4
The first evidence of a specific functional epigenetic protein acting in the NAc was that overexpressing HDAC4 decreases cocaine CPP (Kumar et al., 2005). Later studies suggested that overexpressing HDAC4 - but not a catalytic HDAC-domain deletion mutant - reduces the motivation for cocaine as well (Wang et al., 2010), suggesting HDAC4 reduces cocaine reward-seeking behaviors. However, there are still inconsistencies as in contrast, other studies in Hdac4 NAc conditional knockout mice show HDAC4 increases drug-related behaviors like CPP and sensitization (Penrod et al., 2018).
HDAC5
Other class IIb HDAC studies show that HDAC5 blocks cocaine CPP (Renthal et al., 2007; Taniguchi et al., 2012) and reduces the reinstatement of drug-seeking behavior following cocaine self-administration (Taniguchi et al., 2017). As described above, cocaine exposure induces the dephosphorylation and nuclear accumulation of HDAC5 in a cAMP-dependent manner in D1-containing medium spiny neurons (D1-MSNs). The dephosphorylated nuclear-accumulated form of HDAC5, but not wild-type HDAC5, limits drug-related behaviors. These data suggest a MSN-cell-type dependent HDAC5 function on drug-related behaviors and in response to drug exposure.
HDAC3
The Class I HDAC, HDAC3 also alters drug-related behavior. A NAc-specific conditional knockout of HDAC3 in mice increases cocaine CPP (Rogge et al., 2013). A follow up study demonstrated a D1-MSN cell-type-specific role of HDAC3 in cocaine CPP as a mutated deacetylase activity-dead HDAC3 overexpressed only in NAc increases cocaine CPP. In the same study, the mutated deacetylase activity-dead HDAC3 also attenuates the reinstatement of drug-seeking behavior following cocaine self-administration (Campbell et al., 2021) suggesting discrepancies in the effects of epigenetic regulators on contingent vs non-contingent drug-related behaviors depending on the model used.
SIRTs
Class III HDACs (SIRTs) also regulate drug-related behavior as SIRT1 or SIRT2 overexpression increases cocaine and morphine CPP (Ferguson et al., 2013). Also, a Sirt1 conditional knockout in mice reduces cocaine and morphine CPP (Ferguson et al., 2013). Finally, a NAc-specific injection of the SIRT antagonist sirtinol reduces cocaine CPP and cocaine self-administration (Renthal et al., 2009).
Nucleus Accumbens-Specific Overexpression and/or Knockdown of HATs
Histone acetyltransferases in the NAc also function in cocaine-related behaviors as a NAc conditional knockout of CBP in mice decreases cocaine locomotor activity and cocaine CPP (Malvaez et al., 2011).
Nucleus Accumbens-Specific Overexpression and/or Knockdown of Histone Methyltransferases
Moving from acetylation to methylation, initial studies suggested that the methyltransferase G9a reduces drug-induced locomotor sensitization and drug-conditioned place preference since overexpressing G9a blocks cocaine CPP (Maze et al., 2010, 2014), morphine CPP, and morphine locomotor sensitization (Sun et al., 2012). In addition, intra-NAc administration of the G9a inhibitor BIX01294 and G9a NAc conditional knockout increases cocaine CPP (Maze et al., 2010). Also, a NAc conditional knockout of G9a increases morphine CPP and morphine locomotor sensitization (Sun et al., 2012). However, later studies using contingent cocaine self-administration as a model demonstrated that overexpressing G9a in the NAc increases cocaine sensitivity (using dose-response testing), motivation (using progressive ratio testing), and stress-induced reinstatement (Anderson et al., 2018a). A subsequent study showed that reducing NAc G9a levels via shRNA reduces the sensitivity to cocaine self-administration, motivation, and stress-induced reinstatement (Anderson et al., 2019). Together, these studies showed that G9a levels in the NAc have bi-directional effects on cocaine self-administration and cocaine-seeking behaviors (Anderson et al., 2018a,2019). In addition, reducing G9a in the NAc also blocks stress-induced ethanol drinking and this effect is recapitulated by systemic administration of UNC0642 - a selective G9a inhibitor – as mentioned above (Anderson et al., 2021). These studies again suggest that at least some differences in preclinical studies could be explained by differences in contingent vs non-contingent models.
Other Epigenetic Modifiers
Other methyltransferases like PRMT1 alter cocaine CPP as shown by PRMT1 knockdown and pharmacological inhibition studies (Li Y. et al., 2015). Also, PRMT6 overexpression in the NAc increases cocaine CPP, and miRNA-mediated knockdown reduces cocaine CPP (Damez-Werno et al., 2016). Finally, another study shows that KDM5C or Mll1 knockdown reduces methamphetamine CPP (Aguilar-Valles et al., 2014).
Conclusion
These studies and others in Table 5 demonstrate the powerful effects that epigenetic regulators can have on drug-related behaviors in pre-clinical models. However, these reports often conflict concerning the function of these NAc-specific manipulations, suggesting that differences in experimental design (like the use of contingent vs non-contingent models) can produce different behavioral effects. Still, these reports suggest that translating some of these methods into the clinic could potentially help to reduce the negative effects of substance use.
Limitations and Challenges in the Field of Epigenetics and Substance Use Disorder
Diversity of Epigenetic Modifications and Their Substrates
Despite the large amount of data on epigenetic regulation in rodent models of SUD, and the ability to alter drug-related behaviors through either systemically administered or NAc-specific manipulations, there are still many limitations and challenges for the field. Beginning with examining changes in drug-induced epigenetic modifications, we think that there is a great need for more unbiased approaches. For instance, after initial studies found changes in H3 and H4 PTMs, many subsequent studies only examined these sites with specific antibodies. While these studies often found changes, the focus on these known sites could have prevented the discovery of other important sites of regulation, especially considering the wide array of time-dependent and substance-dependent changes shown in Tables 1, 2. These biases extended to a concentrated study of just a handful of histone PTMs when there are over 100 histone PTMs, most of which have not been examined following exposure to addictive drugs. For example, there are reports on other epigenetic markers like histone phosphorylation that can be altered following cocaine (Bertran-Gonzalez et al., 2008), morphine (Ciccarelli et al., 2013), and methamphetamine use (Rotllant and Armario, 2012). In addition, poly-ADP-ribosylation of histones is altered by drug exposure (Scobie et al., 2014). Notably, two novel histone modifications, serotonylation (Farrelly et al., 2019) and dopaminylation of H3Q5 (Lepack et al., 2020), have been recently reported and could play a role in NAc-mediated drug-related behaviors. Dopaminylation of H3Q5 in the VTA is dysregulated by cocaine exposure and may alter cocaine self-administration behavior (Lepack et al., 2020) and could play a role in the NAc as well. Given the recent reports of these novel histone PTMs, it is possible that we are still missing other important PTMs too.
Some of these issues are due to technical challenges like the need for better antibodies to other PTMs. ChIP assays are limited by the available antibodies so even large “unbiased” approaches have an inherent bias based on these tools (Renthal et al., 2009; Feng et al., 2014) and truly unbiased genome wide PTMs analyses are not yet possible. In addition to examining the epigenetic modifications that influence the transcriptome, chromatin accessibility could be examined by unbiased genome-wide approaches using an Assay for Transposase-Accessible Chromatin (ATAC)-seq or DNase-seq (Fullard et al., 2018; Carullo et al., 2020; Scherma et al., 2020). These assays could be coupled with other unbiased approaches to examine the downstream effects of these targets on transcriptional changes like RNA-seq and/or microarrays to get closer to a complete picture of the effects of these epigenetic changes produced by drugs exposure. Indeed, many of the studies examined in this review did not report on transcriptomic changes and only examined histone PTMs or select gene expression changes. Finally, very little is known of the actual protein changes (and not just mRNA changes) that occur following these epigenetic manipulations and future studies should examine the functional outcomes of these epigenetic effects.
Other forms of epigenetic regulation not involving direct histone PTM regulation can also influence drug-related behaviors. Though out of the scope of this review, DNA methylation is another form of epigenetic regulation that can be altered by drug exposure for weeks after the last drug exposure. In addition, manipulating proteins associated with DNA methylation can also alter drug-related behaviors (see (Werner et al., 2021) and (Anderson et al., 2018b) for reviews). Also, other types of cellular regulation, including non-coding RNAs (ncRNA), are beginning to be understood extensively (Gu et al., 2021). For example, long ncRNAs can be regulated by cocaine at least 24 hrs after the last injection (Bu et al., 2012). Also, microRNAs are regulated by cocaine, heroin, and methamphetamine in the NAc (Eipper-Mains et al., 2011; Su et al., 2019; Dash et al., 2020; Yang et al., 2020; Li et al., 2021; Xu et al., 2021) and the dorsal striatum (Hollander et al., 2010; Im et al., 2010). Finally, small nucleolar RNAs (snoRNAs) are also regulated by cocaine and knockdown of MBII-52 attenuates cocaine CPP (Chen et al., 2014). Taking a broader look at epigenetic regulation of these RNA subtypes could help us determine which epigenetic mechanisms should be targeted to reduce the negative effects of SUD in humans.
Cell Type Specific Epigenetic Regulations
Another major limitation of almost all studies to date is that they do not separate different cellular populations like neurons vs glia. Drug-induced molecular and synaptic plasticity alterations occur in specific cell types to drive behavioral changes (Lobo et al., 2010; Pascoli et al., 2011; Maze et al., 2014; Campbell et al., 2021), but these are often overlooked in whole NAc tissue punches. Not only are glia often included in these epigenetic assays, but neurons that may not be involved in drug-related behaviors are also included. Only small populations of neurons that have been activated during drug-related learning, called engrams, seem to be important for various drug-related behaviors (Koya et al., 2009; Cruz et al., 2014; Hsiang et al., 2014). In contrast, most epigenetic and molecular studies examine whole tissue in the NAc following drug exposure. This means that all cells are being studied in these analyses including dopamine receptor D1 or D2-containing medium spiny neurons (D1- or D2-MSNs), interneurons, glial cells, microglia, and even some amount of blood vessel and blood cells. This hodgepodge of cells may be limiting our ability to detect the specific changes relating to SUD. Perhaps the subset of important cells that drive addictive behavior do retain a distinct histone methylation or acetylation signature, but this is diluted by other cells that return to baseline thus limiting our ability to detect lasting changes that are still present.
Reducing this signal to noise ratio is possible thanks to technologies like cre-driven gene expression or single cell RNA-seq (Macaulay et al., 2017). Several studies have examined differences in D1- and D2-MSN cell type specific regulation of epigenetic mechanisms. These studies suggest distinct epigenetic regulation in specific cell types. For instance, HDAC3, G9a, and PRMT6 have unique roles in D1 vs D2-MSN cell type specific manner (Maze et al., 2014; Damez-Werno et al., 2016; Campbell et al., 2021). Also, cocaine increased H3 phosphorylation only in D1 cells according to one report (Bertran-Gonzalez et al., 2008). Using a combination of cell-type specific transcriptomic analyses and cre-dependent cell lines will be able to further elucidate the role of epigenetic regulation in D1- and D2-MSNs in SUD. Technologies like Fos-Targeted Recombination in Active Population (TRAP) and ArcTRAP allow for examining groups of cells that are regulated together through activity. This technique was recently used and found that despite no overall changes in dorsal striatum HDAC4 and HDAC5 mRNA levels, these transcripts were altered in FOS-positive neurons following prolonged methamphetamine self-administration withdrawal (Li X. et al., 2015). These current advanced technologies enable us to examine transcription and open chromatin status at the single-cell or single-nucleus level. Combining these techniques with RNA-seq, ATAC-seq, or ChIP-seq could also provide data on epigenetic changes at the single-cell level (Rotem et al., 2015). These powerful techniques could help to determine more specific roles of epigenetic regulation caused by exposure to addictive drugs.
Effects of Contingent and Non-contingent Drugs Exposure
Some differences discussed in this review may be due to issues in the rodent models used in these studies. As mentioned above, rodent models can be broadly separated into two classes: (1) experimenter administered (non-contingent) models, including CPP, locomotor sensitization, and alcohol vapor exposure where the rodents have no choice in drug exposure or (2) self-administration (contingent) models that allow the rodents more choice over when to take drugs. These assays include alcohol drinking (2-bottle choice, drinking-in-the-dark) and drug self-administration. Sometimes, these contingent and non-contingent experimental models indicate a similar role of epigenetic regulators in the development of drug reward-conditioned behaviors. For example, HDAC5 has similar roles on drug-related behavior following both non-contingent cocaine CPP and contingent reinstatement of cocaine-seeking behaviors after cocaine SA (Taniguchi et al., 2017). In addition, HDAC4 overexpression in the NAc decreases cocaine intake and reduces motivation in the progressive ratio schedule in a contingent self-administration model (Wang et al., 2010) and also decreases non-contingent cocaine CPP (Kumar et al., 2005). In contrast, in a later study, Hdac4 NAc conditional knockout mice exhibited decreases in cocaine-induced locomotor activity, sensitization, and cocaine CPP in non-contingent experiments (Penrod et al., 2018). Disagreements in the literature like these examples are often found and can lead to very different interpretations. For example, G9a NAc conditional knockout mice exhibited increases in cocaine CPP and overexpressing G9a decreases cocaine CPP suggesting G9a reduces the cocaine-induced behavioral plasticity using non-contingent models (Maze et al., 2010). However, in a contingent cocaine self-administration model, G9a overexpression increases sensitivity in dose-response test, motivation in progressive ratio testing, and stress-induced reinstatement, suggesting that G9a increases cocaine-related behaviors (Anderson et al., 2018a). Since behavioral differences are observed using similar manipulations of epigenetic regulators, it is important to examine the effects of epigenetic manipulations in a variety of behavioral tasks to find those that may be more likely to translate to humans.
Future Directions and Conclusion
As shown above, we now know drug exposure regulates histone marks and epigenetic regulators. Most of these changes appear to be very short-lived, but some can be long-lasting (at least a month) especially when examining changes at specific promoters (Damez-Werno et al., 2012, 2016; Tomasiewicz et al., 2012; Flagel et al., 2016; Carpenter et al., 2020; Johnstone et al., 2021). However, we still do not understand the mechanisms that promote some changes and not others, and it is often difficult to tell if these changes are functional and whether they are addiction-promoting or counter adaptive protective mechanisms (Anderson et al., 2018a). Fortunately, new technologies are being developed that allow for the selective targeting of specific genomic loci (Heller et al., 2014, 2016; Hamilton et al., 2018), these cutting-edge tools allow for epigenetic regulation of a single gene and allow very precise control of gene expression in neurons. Targeting epigenetic mechanisms, possibly through systemic administration of protein inhibitors (Anderson et al., 2021), viral vectors like AAV in select groups of neurons like cell-type or engram-specific circuits - using the methods described in Guenthner et al. (2013), Maze et al. (2014), Damez-Werno et al. (2016), Campbell et al. (2021) - could lead to breakthrough future translational therapeutics in SUD.
Author Contributions
EA and MT wrote sections of the manuscript and organized the database. Both authors contributed to manuscript revision, read, and approved the submitted version.
Funding
This work was supported by grants from NIH (K01 DA046513, P50DA046373, and DA032708).
Conflict of Interest
EA is a co-founder of NeuroEpigenix, LLC.
The author declares that the research was conducted in the absence of any commercial or financial relationships that could be construed as a potential conflict of interest.
Publisher’s Note
All claims expressed in this article are solely those of the authors and do not necessarily represent those of their affiliated organizations, or those of the publisher, the editors and the reviewers. Any product that may be evaluated in this article, or claim that may be made by its manufacturer, is not guaranteed or endorsed by the publisher.
Acknowledgments
We thank Christopher W. Cowan for the many discussions on the topics covered in this review.
References
Adams, J. H., and Bushell, G. R. (1989). Changes in the cytoplasmic elements of cultured cells infected with Eimeria vermiformis sporozoites. J Protozool 36, 133–138. doi: 10.1111/j.1550-7408.1989.tb01059.x
Aguilar-Valles, A., Vaissiere, T., Griggs, E. M., Mikaelsson, M. A., Takacs, I. F., Young, E. J., et al. (2014). Methamphetamine-associated memory is regulated by a writer and an eraser of permissive histone methylation. Biol. Psychiatry 76, 57–65. doi: 10.1016/j.biopsych.2013.09.014
Al Ameri, M., Al Mansouri, S., Al Maamari, A., and Bahi, A. (2014). The histone deacetylase (HDAC) inhibitor valproic acid reduces ethanol consumption and ethanol-conditioned place preference in rats. Brain Res. 1583, 122–131. doi: 10.1016/j.brainres.2014.07.051
Allfrey, V. G., Faulkner, R., and Mirsky, A. E. (1964). Acetylation and methylation of histones and their possible role in the regulation of rna synthesis. Proc. Natl. Acad. Sci. U. S. A. 51, 786–794. doi: 10.1073/pnas.51.5.786
Allis, C. D., and Jenuwein, T. (2016). The molecular hallmarks of epigenetic control. Nat. Rev. Genet. 17, 487–500. doi: 10.1038/nrg.2016.59
American Psychiatric Association (2013). Diagnostic and statistical manual of mental disorders : DSM-5. Washington, DC: American Psychiatric Association.
Anderson, E. M., Larson, E. B., Guzman, D., Wissman, A. M., Neve, R. L., Nestler, E. J., et al. (2018a). Overexpression of the histone dimethyltransferase g9a in nucleus accumbens shell increases cocaine self-administration, stress-induced reinstatement, and anxiety. J. Neurosci. 38, 803–813. doi: 10.1523/JNEUROSCI.1657-17.2017
Anderson, E. M., Penrod, R. D., Barry, S. M., Hughes, B. W., Taniguchi, M., and Cowan, C. W. (2018b). It’s a complex issue: emerging connections between epigenetic regulators in drug addiction. Eur. J. Neurosci. 50, 2477–2491.
Anderson, E. M., Lopez, M. F., Kastner, A., Mulholland, P. J., Becker, H. C., and Cowan, C. W. (2021). The histone methyltransferase G9a mediates stress-regulated alcohol drinking. Addict. Biol. 27:e13060. doi: 10.1111/adb.13060
Anderson, E. M., Sun, H., Guzman, D., Taniguchi, M., Cowan, C. W., Maze, I., et al. (2019). Knockdown of the histone di-methyltransferase G9a in nucleus accumbens shell decreases cocaine self-administration, stress-induced reinstatement, and anxiety. Neuropsychopharmacology 44, 1370–1376. doi: 10.1038/s41386-018-0305-4
Anderson, E. M., Wissman, A. M., Chemplanikal, J., Buzin, N., Guzman, D., Larson, E. B., et al. (2017). BDNF-TrkB controls cocaine-induced dendritic spines in rodent nucleus accumbens dissociated from increases in addictive behaviors. Proc. Natl. Acad. Sci. U. S. A. 114, 9469–9474. doi: 10.1073/pnas.1702441114
Arndt, D. L., Wukitsch, T. J., Garcia, E. J., and Cain, M. (2019). Histone deacetylase inhibition differentially attenuates cue-induced reinstatement: an interaction of environment and acH3K9 expression in the dorsal striatum. Behav. Neurosci. 133, 478–488. doi: 10.1037/bne0000333
Bahi, A., Boyer, F., Chandrasekar, V., and Dreyer, J. L. (2008). Role of accumbens BDNF and TrkB in cocaine-induced psychomotor sensitization, conditioned-place preference, and reinstatement in rats. Psychopharmacology 199, 169–182. doi: 10.1007/s00213-008-1164-1
Bannister, A. J., and Kouzarides, T. (2011). Regulation of chromatin by histone modifications. Cell Res. 21, 381–395. doi: 10.1038/cr.2011.22
Benevento, M., van de Molengraft, M., van Westen, R., van Bokhoven, H., and Kasri, N. N. (2015). The role of chromatin repressive marks in cognition and disease: a focus on the repressive complex GLP/G9a. Neurobiol. Learn. Mem. 124, 88–96. doi: 10.1016/j.nlm.2015.06.013
Bertran-Gonzalez, J., Bosch, C., Maroteaux, M., Matamales, M., Herve, D., Valjent, E., et al. (2008). Opposing patterns of signaling activation in dopamine D1 and D2 receptor-expressing striatal neurons in response to cocaine and haloperidol. J. Neurosci. 28, 5671–5685. doi: 10.1523/JNEUROSCI.1039-08.2008
Bird, A. P., and Southern, E. M. (1978). Use of restriction enzymes to study eukaryotic DNA methylation: I. The methylation pattern in ribosomal DNA from Xenopus laevis. J. Mol. Biol. 118, 27–47. doi: 10.1016/0022-2836(78)90242-5
Botia, B., Legastelois, R., Alaux-Cantin, S., and Naassila, M. (2012). Expression of ethanol-induced behavioral sensitization is associated with alteration of chromatin remodeling in mice. PLoS One 7:e47527. doi: 10.1371/journal.pone.0047527
Bu, Q., Hu, Z., Chen, F., Zhu, R., Deng, Y., Shao, X., et al. (2012). Transcriptome analysis of long non-coding RNAs of the nucleus accumbens in cocaine-conditioned mice. J. Neurochem. 123, 790–799. doi: 10.1111/jnc.12006
Campbell, R. R., Kramar, E. A., Pham, L., Beardwood, J. H., Augustynski, A. S., Lopez, A. J., et al. (2021). HDAC3 activity within the nucleus accumbens regulates cocaine-induced plasticity and behavior in a cell-type-specific manner. J. Neurosci. 41, 2814–2827. doi: 10.1523/JNEUROSCI.2829-20.2021
Caputi, F. F., Di Benedetto, M., Carretta, D., Bastias del Carmen, Candia, S., D’Addario, C., et al. (2014). Dynorphin/KOP and nociceptin/NOP gene expression and epigenetic changes by cocaine in rat striatum and nucleus accumbens. Prog. Neuropsychopharmacol. Biol. Psychiatry 49, 36–46. doi: 10.1016/j.pnpbp.2013.10.016
Caputi, F. F., Palmisano, M., Carboni, L., Candeletti, S., and Romualdi, P. (2016). Opioid gene expression changes and post-translational histone modifications at promoter regions in the rat nucleus accumbens after acute and repeated 3,4-methylenedioxy-methamphetamine (MDMA) exposure. Pharmacol. Res. 114, 209–218. doi: 10.1016/j.phrs.2016.10.023
Carpenter, M. D., Hu, Q., Bond, A. M., Lombroso, S. I., Czarnecki, K. S., Lim, C. J., et al. (2020). Nr4a1 suppresses cocaine-induced behavior via epigenetic regulation of homeostatic target genes. Nat. Commun. 11:504. doi: 10.1038/s41467-020-14331-y
Carullo, N. V. N., Phillips Iii, R. A., Simon, R. C., Soto, S. A. R., Hinds, J. E., Salisbury, A. J., et al. (2020). Enhancer RNAs predict enhancer-gene regulatory links and are critical for enhancer function in neuronal systems. Nucleic Acids Res. 48, 9550–9570. doi: 10.1093/nar/gkaa671
Cates, H. M., Li, X., Purushothaman, I., Kennedy, P. J., Shen, L., Shaham, Y., et al. (2018). Genome-wide transcriptional profiling of central amygdala and orbitofrontal cortex during incubation of methamphetamine craving. Neuropsychopharmacology 43, 2426–2434. doi: 10.1038/s41386-018-0158-x
Chandra, R., Engeln, M., Francis, T. C., Konkalmatt, P., Patel, D., and Lobo, M. K. (2017). A role for peroxisome proliferator-activated receptor gamma coactivator-1alpha in nucleus accumbens neuron subtypes in cocaine action. Biol. Psychiatry 81, 564–572. doi: 10.1016/j.biopsych.2016.10.024
Chen, H., Qiang, H., Fan, K., Wang, S., and Zheng, Z. (2014). The snoRNA MBII-52 regulates cocaine-induced conditioned place preference and locomotion in mice. PLoS One 9:e99986. doi: 10.1371/journal.pone.0099986
Chen, W. S., Xu, W. J., Zhu, H. Q., Gao, L., Lai, M. J., Zhang, F. Q., et al. (2016). Effects of histone deacetylase inhibitor sodium butyrate on heroin seeking behavior in the nucleus accumbens in rats. Brain Res. 1652, 151–157. doi: 10.1016/j.brainres.2016.10.007
Christman, J. K., Price, P., Pedrinan, L., and Acs, G. (1977). Correlation between hypomethylation of DNA and expression of globin genes in Friend erythroleukemia cells. Eur. J. Biochem. 81, 53–61. doi: 10.1111/j.1432-1033.1977.tb11926.x
Ciccarelli, A., Calza, A., Santoru, F., Grasso, F., Concas, A., Sassoe-Pognetto, M., et al. (2013). Morphine withdrawal produces ERK-dependent and ERK-independent epigenetic marks in neurons of the nucleus accumbens and lateral septum. Neuropharmacology 70, 168–179. doi: 10.1016/j.neuropharm.2012.12.010
Cleck, J. N., Ecke, L. E., and Blendy, J. A. (2008). Endocrine and gene expression changes following forced swim stress exposure during cocaine abstinence in mice. Psychopharmacology 201, 15–28. doi: 10.1007/s00213-008-1243-3
Covington, H. E. III, Maze, I., Sun, H., Bomze, H. M., DeMaio, K. D., Wu, E. Y., et al. (2011). A role for repressive histone methylation in cocaine-induced vulnerability to stress. Neuron 71, 656–670. doi: 10.1016/j.neuron.2011.06.007
Cruz, F. C., Babin, K. R., Leao, R. M., Goldart, E. M., Bossert, J. M., Shaham, Y., et al. (2014). Role of nucleus accumbens shell neuronal ensembles in context-induced reinstatement of cocaine-seeking. J. Neurosci. 34, 7437–7446. doi: 10.1523/JNEUROSCI.0238-14.2014
Damez-Werno, D., LaPlant, Q., Sun, H., Scobie, K. N., Dietz, D. M., Walker, I. M., et al. (2012). Drug experience epigenetically primes Fosb gene inducibility in rat nucleus accumbens. J. Neurosci. 32, 10267–10272. doi: 10.1523/JNEUROSCI.1290-12.2012
Damez-Werno, D. M., Sun, H., Scobie, K. N., Shao, N., Rabkin, J., Dias, C., et al. (2016). Histone arginine methylation in cocaine action in the nucleus accumbens. Proc. Natl. Acad. Sci. U. S. A. 113, 9623–9628. doi: 10.1073/pnas.1605045113
Dash, S., Balasubramaniam, M., Martinez-Rivera, F. J., Godino, A., Peck, E. G., Patnaik, S., et al. (2020). Cocaine-regulated microRNA miR-124 controls poly (ADP-ribose) polymerase-1 expression in neuronal cells. Sci. Rep. 10:11197. doi: 10.1038/s41598-020-68144-6
Deans, C., and Maggert, K. A. (2015). What do you mean, “epigenetic”? Genetics 199, 887–896. doi: 10.1534/genetics.114.173492
Delcuve, G. P., Rastegar, M., and Davie, J. R. (2009). Epigenetic control. J. Cell Physiol. 219, 243–250.
Deng, J. V., Rodriguiz, R. M., Hutchinson, A. N., Kim, I. H., Wetsel, W. C., and West, A. E. (2010). MeCP2 in the nucleus accumbens contributes to neural and behavioral responses to psychostimulants. Nat. Neurosci. 13, 1128–1136. doi: 10.1038/nn.2614
Deng, J. V., Wan, Y., Wang, X., Cohen, S., Wetsel, W. C., Greenberg, M. E., et al. (2014). MeCP2 phosphorylation limits psychostimulant-induced behavioral and neuronal plasticity. J. Neurosci. 34, 4519–4527. doi: 10.1523/JNEUROSCI.2821-13.2014
Desrosiers, R. C., Mulder, C., and Fleckenstein, B. (1979). Methylation of Herpesvirus saimiri DNA in lymphoid tumor cell lines. Proc. Natl. Acad. Sci. U. S. A. 76, 3839–3843. doi: 10.1073/pnas.76.8.3839
Eberharter, A., and Becker, P. B. (2002). Histone acetylation: a switch between repressive and permissive chromatin. Second in review series on chromatin dynamics. EMBO Rep. 3, 224–229. doi: 10.1093/embo-reports/kvf053
Egervari, G., Landry, J., Callens, J., Fullard, J. F., Roussos, P., Keller, E., et al. (2017). Striatal H3K27 acetylation linked to glutamatergic gene dysregulation in human heroin abusers holds promise as therapeutic target. Biol. Psychiatry 81, 585–594. doi: 10.1016/j.biopsych.2016.09.015
Eipper-Mains, J. E., Kiraly, D. D., Palakodeti, D., Mains, R. E., Eipper, B. A., and Graveley, B. R. (2011). microRNA-Seq reveals cocaine-regulated expression of striatal microRNAs. RNA 17, 1529–1543. doi: 10.1261/rna.2775511
Engmann, O., Labonte, B., Mitchell, A., Bashtrykov, P., Calipari, E. S., Rosenbluh, C., et al. (2017). Cocaine-induced chromatin modifications associate with increased expression and three-dimensional looping of Auts2. Biol. Psychiatry 82, 794–805. doi: 10.1016/j.biopsych.2017.04.013
Faillace, M. P., Zwiller, J., and Bernabeu, R. O. (2015). Effects of combined nicotine and fluoxetine treatment on adult hippocampal neurogenesis and conditioned place preference. Neuroscience 300, 104–115. doi: 10.1016/j.neuroscience.2015.05.017
Farrelly, L. A., Thompson, R. E., Zhao, S., Lepack, A. E., Lyu, Y., Bhanu, N. V., et al. (2019). Histone serotonylation is a permissive modification that enhances TFIID binding to H3K4me3. Nature 567, 535–539. doi: 10.1038/s41586-019-1024-7
Feng, J., Wilkinson, M., Liu, X., Purushothaman, I., Ferguson, D., Vialou, V., et al. (2014). Chronic cocaine-regulated epigenomic changes in mouse nucleus accumbens. Genome Biol. 15:R65.
Ferguson, D., Koo, J. W., Feng, J., Heller, E., Rabkin, J., Heshmati, M., et al. (2013). Essential role of SIRT1 signaling in the nucleus accumbens in cocaine and morphine action. J. Neurosci. 33, 16088–16098. doi: 10.1523/JNEUROSCI.1284-13.2013
Ferguson, D., Shao, N., Heller, E., Feng, J., Neve, R., Kim, H. D., et al. (2015). SIRT1-FOXO3a regulate cocaine actions in the nucleus accumbens. J. Neurosci. 35, 3100–3111. doi: 10.1523/JNEUROSCI.4012-14.2015
Finegersh, A., Ferguson, C., Maxwell, S., Mazariegos, D., Farrell, D., and Homanics, G. E. (2015). Repeated vapor ethanol exposure induces transient histone modifications in the brain that are modified by genotype and brain region. Front. Mol. Neurosci. 8:39. doi: 10.3389/fnmol.2015.00039
Flagel, S. B., Chaudhury, S., Waselus, M., Kelly, R., Sewani, S., Clinton, S. M., et al. (2016). Genetic background and epigenetic modifications in the core of the nucleus accumbens predict addiction-like behavior in a rat model. Proc. Natl. Acad. Sci. U. S. A. 113, E2861–E2870. doi: 10.1073/pnas.1520491113
Fullard, J. F., Hauberg, M. E., Bendl, J., Egervari, G., Cirnaru, M. D., Reach, S. M., et al. (2018). An atlas of chromatin accessibility in the adult human brain. Genome Res. 28, 1243–1252. doi: 10.1101/gr.232488.117
Godino, A., Jayanthi, S., and Cadet, J. L. (2015). Epigenetic landscape of amphetamine and methamphetamine addiction in rodents. Epigenetics 10, 574–580. doi: 10.1080/15592294.2015.1055441
Goodwin, D. W., Schulsinger, F., Hermansen, L., Guze, S. B., and Winokur, G. (1973). Alcohol problems in adoptees raised apart from alcoholic biological parents. Arch. Gen. Psychiatry 28, 238–243. doi: 10.1001/archpsyc.1973.01750320068011
Goodwin, D. W., Schulsinger, F., Knop, J., Mednick, S., and Guze, S. B. (1977). Psychopathology in adopted and nonadopted daughters of alcoholics. Arch. Gen. Psychiatry 34, 1005–1009. doi: 10.1001/archpsyc.1977.01770210019001
Goodwin, D. W., Schulsinger, F., Moller, N., Hermansen, L., Winokur, G., and Guze, S. B. (1974). Drinking problems in adopted and nonadopted sons of alcoholics. Arch. Gen. Psychiatry 31, 164–169. doi: 10.1001/archpsyc.1974.01760140022003
Graham, D. L., Edwards, S., Bachtell, R. K., DiLeone, R. J., Rios, M., and Self, D. W. (2007). Dynamic BDNF activity in nucleus accumbens with cocaine use increases self-administration and relapse. Nat. Neurosci. 10, 1029–1037. doi: 10.1038/nn1929
Griffin, E. A. Jr., Melas, P. A., Zhou, R., Li, Y., Mercado, P., Kempadoo, K. A., et al. (2017). Prior alcohol use enhances vulnerability to compulsive cocaine self-administration by promoting degradation of HDAC4 and HDAC5. Sci. Adv. 3:e1701682. doi: 10.1126/sciadv.1701682
Grunstein, M. (1997). Histone acetylation in chromatin structure and transcription. Nature 389, 349–352.
Gu, Y. J., Chen, L., Cheng, L., Zhou, M. Y., and Wang, Y. (2021). Non-coding RNA: insights into the mechanism of methamphetamine neurotoxicity. Mol. Cell Biochem. 476, 3319–3328. doi: 10.1007/s11010-021-04160-y
Guenthner, C. J., Miyamichi, K., Yang, H. H., Heller, H. C., and Luo, L. (2013). Permanent genetic access to transiently active neurons via TRAP: targeted recombination in active populations. Neuron 78, 773–784. doi: 10.1016/j.neuron.2013.03.025
Hamilton, P. J., Burek, D. J., Lombroso, S. I., Neve, R. L., Robison, A. J., Nestler, E. J., et al. (2018). Cell-type-specific epigenetic editing at the fosb gene controls susceptibility to social defeat stress. Neuropsychopharmacology 43, 272–284. doi: 10.1038/npp.2017.88
Heller, E. A., Cates, H. M., Pena, C. J., Sun, H., Shao, N., Feng, J., et al. (2014). Locus-specific epigenetic remodeling controls addiction- and depression-related behaviors. Nat. Neurosci. 17, 1720–1727. doi: 10.1038/nn.3871
Heller, E. A., Hamilton, P. J., Burek, D. D., Lombroso, S. I., Pena, C. J., Neve, R. L., et al. (2016). Targeted epigenetic remodeling of the Cdk5 gene in nucleus accumbens regulates cocaine- and stress-evoked behavior. J. Neurosci. 36, 4690–4697. doi: 10.1523/JNEUROSCI.0013-16.2016
Hitchcock, L. N., Raybuck, J. D., Wood, M. A., and Lattal, K. M. (2019). Effects of a histone deacetylase 3 inhibitor on extinction and reinstatement of cocaine self-administration in rats. Psychopharmacology 236, 517–529. doi: 10.1007/s00213-018-5122-2
Hollander, J. A., Im, H. I., Amelio, A. L., Kocerha, J., Bali, P., Lu, Q., et al. (2010). Striatal microRNA controls cocaine intake through CREB signalling. Nature 466, 197–202. doi: 10.1038/nature09202
Hong, L., Schroth, G. P., Matthews, H. R., Yau, P., and Bradbury, E. M. (1993). Studies of the DNA binding properties of histone H4 amino terminus. Thermal denaturation studies reveal that acetylation markedly reduces the binding constant of the H4 “tail” to DNA. J. Biol. Chem. 268, 305–314.
Host, L., Anglard, P., Romieu, P., Thibault, C., Dembele, D., Aunis, D., et al. (2010). Inhibition of histone deacetylases in rats self-administering cocaine regulates lissencephaly gene-1 and reelin gene expression, as revealed by microarray technique. J. Neurochem. 113, 236–247.
Host, L., Dietrich, J. B., Carouge, D., Aunis, D., and Zwiller, J. (2011). Cocaine self-administration alters the expression of chromatin-remodelling proteins; modulation by histone deacetylase inhibition. J. Psychopharmacol. 25, 222–229. doi: 10.1177/0269881109348173
Hsiang, H. L., Epp, J. R., van den Oever, M. C., Yan, C., Rashid, A. J., Insel, N., et al. (2014). Manipulating a “cocaine engram” in mice. J. Neurosci. 34, 14115–14127. doi: 10.1523/JNEUROSCI.3327-14.2014
Huisinga, K. L., Brower-Toland, B., and Elgin, S. C. (2006). The contradictory definitions of heterochromatin: transcription and silencing. Chromosoma 115, 110–122. doi: 10.1007/s00412-006-0052-x
Ikegami, D., Narita, M., Imai, S., Miyashita, K., Tamura, R., Narita, M., et al. (2010). Epigenetic modulation at the CCR2 gene correlates with the maintenance of behavioral sensitization to methamphetamine. Addict. Biol. 15, 358–361. doi: 10.1111/j.1369-1600.2010.00219.x
Im, H. I., Hollander, J. A., Bali, P., and Kenny, P. J. (2010). MeCP2 controls BDNF expression and cocaine intake through homeostatic interactions with microRNA-212. Nat. Neurosci. 13, 1120–1127. doi: 10.1038/nn.2615
Itzhak, Y., Liddie, S., and Anderson, K. L. (2013). Sodium butyrate-induced histone acetylation strengthens the expression of cocaine-associated contextual memory. Neurobiol. Learn. Mem. 102, 34–42. doi: 10.1016/j.nlm.2013.03.007
Jaenisch, R., and Bird, A. (2003). Epigenetic regulation of gene expression: how the genome integrates intrinsic and environmental signals. Nat. Genet. 33(Suppl.), 245–254. doi: 10.1038/ng1089
Jeanblanc, J., Lemoine, S., Jeanblanc, V., Alaux-Cantin, S., and Naassila, M. (2015). The class I-specific HDAC inhibitor MS-275 decreases motivation to consume alcohol and relapse in heavy drinking rats. Int. J. Neuropsychopharmacol. 18:pyv029. doi: 10.1093/ijnp/pyv029
Johnstone, A. L., Andrade, N. S., Barbier, E., Khomtchouk, B. B., Rienas, C. A., Lowe, K., et al. (2021). Dysregulation of the histone demethylase KDM6B in alcohol dependence is associated with epigenetic regulation of inflammatory signaling pathways. Addict. Biol. 26:e12816. doi: 10.1111/adb.12816
Jones, P. A., and Taylor, S. M. (1980). Cellular differentiation, cytidine analogs and DNA methylation. Cell 20, 85–93. doi: 10.1016/0092-8674(80)90237-8
Kalda, A., Heidmets, L. T., Shen, H. Y., Zharkovsky, A., and Chen, J. F. (2007). Histone deacetylase inhibitors modulates the induction and expression of amphetamine-induced behavioral sensitization partially through an associated learning of the environment in mice. Behav. Brain Res. 181, 76–84. doi: 10.1016/j.bbr.2007.03.027
Kennedy, P. J., Feng, J., Robison, A. J., Maze, I., Badimon, A., Mouzon, E., et al. (2013). Class I HDAC inhibition blocks cocaine-induced plasticity by targeted changes in histone methylation. Nat. Neurosci. 16, 434–440. doi: 10.1038/nn.3354
Kenny, P. J. (2014). Epigenetics, microRNA, and addiction. Dialogues Clin. Neurosci. 16, 335–344. doi: 10.31887/DCNS.2014.16.3/pkenny
Kim, W. Y., Kim, S., and Kim, J. H. (2008). Chronic microinjection of valproic acid into the nucleus accumbens attenuates amphetamine-induced locomotor activity. Neurosci. Lett. 432, 54–57. doi: 10.1016/j.neulet.2007.12.005
Koya, E., Golden, S. A., Harvey, B. K., Guez-Barber, D. H., Berkow, A., Simmons, D. E., et al. (2009). Targeted disruption of cocaine-activated nucleus accumbens neurons prevents context-specific sensitization. Nat. Neurosci. 12, 1069–1073. doi: 10.1038/nn.2364
Kumar, A., Choi, K. H., Renthal, W., Tsankova, N. M., Theobald, D. E., Truong, H. T., et al. (2005). Chromatin remodeling is a key mechanism underlying cocaine-induced plasticity in striatum. Neuron 48, 303–314. doi: 10.1016/j.neuron.2005.09.023
Lachner, M., O’Carroll, D., Rea, S., Mechtler, K., and Jenuwein, T. (2001). Methylation of histone H3 lysine 9 creates a binding site for HP1 proteins. Nature 410, 116–120. doi: 10.1038/35065132
Le, Q., Yan, B., Yu, X., Li, Y., Song, H., Zhu, H., et al. (2017). Drug-seeking motivation level in male rats determines offspring susceptibility or resistance to cocaine-seeking behaviour. Nat. Commun. 8:15527. doi: 10.1038/ncomms15527
Legastelois, R., Botia, B., and Naassila, M. (2013). Blockade of ethanol-induced behavioral sensitization by sodium butyrate: descriptive analysis of gene regulations in the striatum. Alcohol. Clin. Exp. Res. 37, 1143–1153. doi: 10.1111/acer.12088
Lepack, A. E., Werner, C. T., Stewart, A. F., Fulton, S. L., Zhong, P., Farrelly, L. A., et al. (2020). Dopaminylation of histone H3 in ventral tegmental area regulates cocaine seeking. Science 368, 197–201. doi: 10.1126/science.aaw8806
Levine, A., Huang, Y., Drisaldi, B., Griffin, E. A. Jr., Pollak, D. D., Xu, S., et al. (2011). Molecular mechanism for a gateway drug: epigenetic changes initiated by nicotine prime gene expression by cocaine. Sci. Transl. Med. 3:107ra109. doi: 10.1126/scitranslmed.3003062
Lewis, J. D., Meehan, R. R., Henzel, W. J., Maurer-Fogy, I., Jeppesen, P., Klein, F., et al. (1992). Purification, sequence, and cellular localization of a novel chromosomal protein that binds to methylated DNA. Cell 69, 905–914. doi: 10.1016/0092-8674(92)90610-o
Li, J., Zhu, L., Su, H., Liu, D., Yan, Z., Ni, T., et al. (2021). Regulation of miR-128 in the nucleus accumbens affects methamphetamine-induced behavioral sensitization by modulating proteins involved in neuroplasticity. Addict. Biol. 26:e12881. doi: 10.1111/adb.12881
Li, X., Carreria, M. B., Witonsky, K. R., Zeric, T., Lofaro, O. M., Bossert, J. M., et al. (2018). Role of dorsal striatum histone deacetylase 5 in incubation of methamphetamine craving. Biol. Psychiatry 84, 213–222. doi: 10.1016/j.biopsych.2017.12.008
Li, X., DeJoseph, M. R., Urban, J. H., Bahi, A., Dreyer, J. L., Meredith, G. E., et al. (2013). Different roles of BDNF in nucleus accumbens core versus shell during the incubation of cue-induced cocaine craving and its long-term maintenance. J. Neurosci. 33, 1130–1142. doi: 10.1523/JNEUROSCI.3082-12.2013
Li, X., Rubio, F. J., Zeric, T., Bossert, J. M., Kambhampati, S., Cates, H. M., et al. (2015). Incubation of methamphetamine craving is associated with selective increases in expression of Bdnf and trkb, glutamate receptors, and epigenetic enzymes in cue-activated fos-expressing dorsal striatal neurons. J. Neurosci. 35, 8232–8244. doi: 10.1523/JNEUROSCI.1022-15.2015
Li, Y., Zhu, R., Wang, W., Fu, D., Hou, J., Ji, S., et al. (2015). Arginine methyltransferase 1 in the nucleus accumbens regulates behavioral effects of cocaine. J. Neurosci. 35, 12890–12902. doi: 10.1523/JNEUROSCI.0246-15.2015
Lobo, M. K., Covington, H. E. III, Chaudhury, D., Friedman, A. K., Sun, H., Damez-Werno, D., et al. (2010). Cell type-specific loss of BDNF signaling mimics optogenetic control of cocaine reward. Science 330, 385–390. doi: 10.1126/science.1188472
Luger, K., Mader, A. W., Richmond, R. K., Sargent, D. F., and Richmond, T. J. (1997). Crystal structure of the nucleosome core particle at 2.8 A resolution. Nature 389, 251–260.
Macaulay, I. C., Ponting, C. P., and Voet, T. (2017). Single-cell multiomics: multiple measurements from single cells. Trends Genet. 33, 155–168. doi: 10.1016/j.tig.2016.12.003
Malvaez, M., McQuown, S. C., Rogge, G. A., Astarabadi, M., Jacques, V., Carreiro, S., et al. (2013). HDAC3-selective inhibitor enhances extinction of cocaine-seeking behavior in a persistent manner. Proc. Natl. Acad. Sci. U. S. A. 110, 2647–2652. doi: 10.1073/pnas.1213364110
Malvaez, M., Mhillaj, E., Matheos, D. P., Palmery, M., and Wood, M. A. (2011). CBP in the nucleus accumbens regulates cocaine-induced histone acetylation and is critical for cocaine-associated behaviors. J. Neurosci. 31, 16941–16948. doi: 10.1523/JNEUROSCI.2747-11.2011
Malvaez, M., Sanchis-Segura, C., Vo, D., Lattal, K. M., and Wood, M. A. (2010). Modulation of chromatin modification facilitates extinction of cocaine-induced conditioned place preference. Biol. Psychiatry 67, 36–43. doi: 10.1016/j.biopsych.2009.07.032
Martin, T. A., Jayanthi, S., McCoy, M. T., Brannock, C., Ladenheim, B., Garrett, T., et al. (2012). Methamphetamine causes differential alterations in gene expression and patterns of histone acetylation/hypoacetylation in the rat nucleus accumbens. PLoS One 7:e34236. doi: 10.1371/journal.pone.0034236
Massart, R., Barnea, R., Dikshtein, Y., Suderman, M., Meir, O., Hallett, M., et al. (2015). Role of DNA methylation in the nucleus accumbens in incubation of cocaine craving. J. Neurosci. 35, 8042–8058. doi: 10.1523/JNEUROSCI.3053-14.2015
Maze, I., Chaudhury, D., Dietz, D. M., Von Schimmelmann, M., Kennedy, P. J., Lobo, M. K., et al. (2014). G9a influences neuronal subtype specification in striatum. Nat. Neurosci. 17, 533–539. doi: 10.1038/nn.3670
Maze, I., Covington, H. E. III, Dietz, D. M., LaPlant, Q., Renthal, W., Russo, S. J., et al. (2010). Essential role of the histone methyltransferase G9a in cocaine-induced plasticity. Science 327, 213–216. doi: 10.1126/science.1179438
Maze, I., Feng, J., Wilkinson, M. B., Sun, H., Shen, L., and Nestler, E. J. (2011). Cocaine dynamically regulates heterochromatin and repetitive element unsilencing in nucleus accumbens. Proc. Natl. Acad. Sci. U.S.A. 108, 3035–3040. doi: 10.1073/pnas.1015483108
McCarthy, D. M., Morgan, T. J. Jr., Lowe, S. E., Williamson, M. J., Spencer, T. J., Biederman, J., et al. (2018). Nicotine exposure of male mice produces behavioral impairment in multiple generations of descendants. PLoS Biol. 16:e2006497. doi: 10.1371/journal.pbio.2006497
Meehan, R. R., Lewis, J. D., and Bird, A. P. (1992). Characterization of MeCP2, a vertebrate DNA binding protein with affinity for methylated DNA. Nucleic Acids Res. 20, 5085–5092. doi: 10.1093/nar/20.19.5085
Meehan, R. R., Lewis, J. D., McKay, S., Kleiner, E. L., and Bird, A. P. (1989). Identification of a mammalian protein that binds specifically to DNA containing methylated CpGs. Cell 58, 499–507. doi: 10.1016/0092-8674(89)90430-3
Nesse, R. M., and Berridge, K. C. (1997). Psychoactive drug use in evolutionary perspective. Science 278, 63–66.
Nestler, E. J. (2013). Cellular basis of memory for addiction. Dialogues Clin. Neurosci. 15, 431–443. doi: 10.31887/DCNS.2013.15.4/enestler
Nieto, S. J., Haile, C. N., Quave, C. B., Harding, M. J., Nielsen, D. A., Meisch, R. A., et al. (2022). Paternal alcohol exposure reduces acquisition of operant alcohol self-administration and affects Bdnf DNA methylation in male and female offspring. Addict. Biol. 27:e13078. doi: 10.1111/adb.13078
Pandey, S. C., Ugale, R., Zhang, H., Tang, L., and Prakash, A. (2008). Brain chromatin remodeling: a novel mechanism of alcoholism. J. Neurosci. 28, 3729–3737. doi: 10.1523/JNEUROSCI.5731-07.2008
Pascoli, V., Turiault, M., and Luscher, C. (2011). Reversal of cocaine-evoked synaptic potentiation resets drug-induced adaptive behaviour. Nature 481, 71–75. doi: 10.1038/nature10709
Pascual, M., Boix, J., Felipo, V., and Guerri, C. (2009). Repeated alcohol administration during adolescence causes changes in the mesolimbic dopaminergic and glutamatergic systems and promotes alcohol intake in the adult rat. J. Neurochem. 108, 920–931. doi: 10.1111/j.1471-4159.2008.05835.x
Pastor, V., Host, L., Zwiller, J., and Bernabeu, R. (2011). Histone deacetylase inhibition decreases preference without affecting aversion for nicotine. J. Neurochem. 116, 636–645. doi: 10.1111/j.1471-4159.2010.07149.x
Penrod, R. D., Carreira, M. B., Taniguchi, M., Kumar, J., Maddox, S. A., and Cowan, C. W. (2018). Novel role and regulation of HDAC4 in cocaine-related behaviors. Addict. Biol. 23, 653–664. doi: 10.1111/adb.12522
Pozhidayeva, D. Y., Farris, S. P., Goeke, C. M., Firsick, E. J., Townsley, K. G., Guizzetti, M., et al. (2020). Chronic chemogenetic stimulation of the nucleus accumbens produces lasting reductions in binge drinking and ameliorates alcohol-related morphological and transcriptional changes. Brain Sci 10:109. doi: 10.3390/brainsci10020109
Prini, P., Penna, F., Sciuccati, E., Alberio, T., and Rubino, T. (2017). Chronic delta(8)-thc exposure differently affects histone modifications in the adolescent and adult rat brain. Int. J. Mol. Sci. 18:2094. doi: 10.3390/ijms18102094
Reilly, M. T., Noronha, A., Goldman, D., and Koob, G. F. (2017). Genetic studies of alcohol dependence in the context of the addiction cycle. Neuropharmacology 122, 3–21. doi: 10.1016/j.neuropharm.2017.01.017
Renthal, W., Carle, T. L., Maze, I., Covington, H. E. III, Truong, H. T., Alibhai, I., et al. (2008). Delta FosB mediates epigenetic desensitization of the c-fos gene after chronic amphetamine exposure. J. Neurosci. 28, 7344–7349. doi: 10.1523/JNEUROSCI.1043-08.2008
Renthal, W., Kumar, A., Xiao, G., Wilkinson, M., Covington, H. E. III, Maze, I., et al. (2009). Genome-wide analysis of chromatin regulation by cocaine reveals a role for sirtuins. Neuron 62, 335–348. doi: 10.1016/j.neuron.2009.03.026
Renthal, W., Maze, I., Krishnan, V., Covington, H. E. III, Xiao, G., Kumar, A., et al. (2007). Histone deacetylase 5 epigenetically controls behavioral adaptations to chronic emotional stimuli. Neuron 56, 517–529. doi: 10.1016/j.neuron.2007.09.032
Rogge, G. A., Singh, H., Dang, R., and Wood, M. A. (2013). HDAC3 is a negative regulator of cocaine-context-associated memory formation. J. Neurosci. 33, 6623–6632. doi: 10.1523/JNEUROSCI.4472-12.2013
Romieu, P., Deschatrettes, E., Host, L., Gobaille, S., Sandner, G., and Zwiller, J. (2011). The inhibition of histone deacetylases reduces the reinstatement of cocaine-seeking behavior in rats. Curr. Neuropharmacol. 9, 21–25. doi: 10.2174/157015911795017317
Romieu, P., Host, L., Gobaille, S., Sandner, G., Aunis, D., and Zwiller, J. (2008). Histone deacetylase inhibitors decrease cocaine but not sucrose self-administration in rats. J. Neurosci. 28, 9342–9348. doi: 10.1523/JNEUROSCI.0379-08.2008
Rotem, A., Ram, O., Shoresh, N., Sperling, R. A., Goren, A., Weitz, D. A., et al. (2015). Single-cell ChIP-seq reveals cell subpopulations defined by chromatin state. Nat. Biotechnol. 33, 1165–1172. doi: 10.1038/nbt.3383
Rotllant, D., and Armario, A. (2012). Brain pattern of histone H3 phosphorylation after acute amphetamine administration: its relationship to brain c-fos induction is strongly dependent on the particular brain area. Neuropharmacology 62, 1073–1081. doi: 10.1016/j.neuropharm.2011.10.019
Saberian, H., Asgari Taei, A., Torkaman-Boutorabi, A., Riahi, E., Aminyavari, S., Naghizadeh, A., et al. (2021). Effect of histone acetylation on maintenance and reinstatement of morphine-induced conditioned place preference and DeltaFosB expression in the nucleus accumbens and prefrontal cortex of male rats. Behav. Brain Res. 414:113477. doi: 10.1016/j.bbr.2021.113477
Sakharkar, A. J., Zhang, H., Tang, L., Baxstrom, K., Shi, G., Moonat, S., et al. (2014). Effects of histone deacetylase inhibitors on amygdaloid histone acetylation and neuropeptide Y expression: a role in anxiety-like and alcohol-drinking behaviours. Int. J. Neuropsychopharmacol. 17, 1207–1220. doi: 10.1017/S1461145714000054
Sanchez-Serrano, S. L., Cruz, S. L., and Lamas, M. (2011). Repeated toluene exposure modifies the acetylation pattern of histones H3 and H4 in the rat brain. Neurosci. Lett. 489, 142–147. doi: 10.1016/j.neulet.2010.12.004
Sanchis-Segura, C., Lopez-Atalaya, J. P., and Barco, A. (2009). Selective boosting of transcriptional and behavioral responses to drugs of abuse by histone deacetylase inhibition. Neuropsychopharmacology 34, 2642–2654. doi: 10.1038/npp.2009.125
Scherma, M., Qvist, J. S., Asok, A., Huang, S. C., Masia, P., Deidda, M., et al. (2020). Cannabinoid exposure in rat adolescence reprograms the initial behavioral, molecular, and epigenetic response to cocaine. Proc. Natl. Acad. Sci. U. S. A. 117, 9991–10002. doi: 10.1073/pnas.1920866117
Schroeder, F. A., Lewis, M. C., Fass, D. M., Wagner, F. F., Zhang, Y. L., Hennig, K. M., et al. (2013). A selective HDAC 1/2 inhibitor modulates chromatin and gene expression in brain and alters mouse behavior in two mood-related tests. PLoS One 8:e71323. doi: 10.1371/journal.pone.0071323
Schuckit, M. A., Goodwin, D. A., and Winokur, G. (1972). A study of alcoholism in half siblings. Am. J. Psychiatry 128, 1132–1136. doi: 10.1176/ajp.128.9.1132
Scobie, K. N., Damez-Werno, D., Sun, H., Shao, N., Gancarz, A., Panganiban, C. H., et al. (2014). Essential role of poly(ADP-ribosyl)ation in cocaine action. Proc. Natl Acad. Sci. U. S. A. 111, 2005–2010. doi: 10.1073/pnas.1319703111
Seth, P., Scholl, L., Rudd, R. A., and Bacon, S. (2018). Overdose deaths involving opioids, cocaine, and psychostimulants - United States, 2015-2016. MMWR Morb. Mortal Wkly. Rep. 67, 349–358. doi: 10.15585/mmwr.mm6712a1
Sharma, R., Parikh, M., Mishra, V., Soni, A., Rubi, S., Sahota, P., et al. (2021). Antisense-induced downregulation of major circadian genes modulates the expression of histone deacetylase-2 (HDAC-2) and CREB-binding protein (CBP) in the medial shell region of nucleus accumbens of mice exposed to chronic excessive alcohol consumption. J. Neurochem. 161, 8–19. doi: 10.1111/jnc.15547
Sheng, J., Lv, Z., Wang, L., Zhou, Y., and Hui, B. (2011). Histone H3 phosphoacetylation is critical for heroin-induced place preference. Neuroreport 22, 575–580. doi: 10.1097/WNR.0b013e328348e6aa
Shibasaki, M., Mizuno, K., Kurokawa, K., and Ohkuma, S. (2011). L-type voltage-dependent calcium channels facilitate acetylation of histone H3 through PKCgamma phosphorylation in mice with methamphetamine-induced place preference. J. Neurochem. 118, 1056–1066. doi: 10.1111/j.1471-4159.2011.07387.x
Simon-O’Brien, E., Alaux-Cantin, S., Warnault, V., Buttolo, R., Naassila, M., and Vilpoux, C. (2015). The histone deacetylase inhibitor sodium butyrate decreases excessive ethanol intake in dependent animals. Addict. Biol. 20, 676–689. doi: 10.1111/adb.12161
Sprow, G. M., Rinker, J. A., and Thiele, T. E. (2014). Histone acetylation in the nucleus accumbens shell modulates ethanol-induced locomotor activity in DBA/2J mice. Alcohol. Clin. Exp. Res. 38, 2377–2386. doi: 10.1111/acer.12502
Sterner, D. E., and Berger, S. L. (2000). Acetylation of histones and transcription-related factors. Microbiol. Mol. Biol. Rev. 64, 435–459.
Su, H., Zhu, L., Li, J., Wang, R., Liu, D., Han, W., et al. (2019). Regulation of microRNA-29c in the nucleus accumbens modulates methamphetamine -induced locomotor sensitization in mice. Neuropharmacology 148, 160–168. doi: 10.1016/j.neuropharm.2019.01.007
Sun, H., Maze, I., Dietz, D. M., Scobie, K. N., Kennedy, P. J., Damez-Werno, D., et al. (2012). Morphine epigenomically regulates behavior through alterations in histone H3 lysine 9 dimethylation in the nucleus accumbens. J. Neurosci. 32, 17454–17464. doi: 10.1523/JNEUROSCI.1357-12.2012
Sun, J., Wang, L., Jiang, B., Hui, B., Lv, Z., and Ma, L. (2008). The effects of sodium butyrate, an inhibitor of histone deacetylase, on the cocaine- and sucrose-maintained self-administration in rats. Neurosci. Lett. 441, 72–76. doi: 10.1016/j.neulet.2008.05.010
Taniguchi, M., Carreira, M. B., Cooper, Y. A., Bobadilla, A. C., Heinsbroek, J. A., Koike, N., et al. (2017). HDAC5 and its target gene, npas4, function in the nucleus accumbens to regulate cocaine-conditioned behaviors. Neuron 96:e136. doi: 10.1016/j.neuron.2017.09.015
Taniguchi, M., Carreira, M. B., Smith, L. N., Zirlin, B. C., Neve, R. L., and Cowan, C. W. (2012). Histone deacetylase 5 limits cocaine reward through cAMP-induced nuclear import. Neuron 73, 108–120. doi: 10.1016/j.neuron.2011.10.032
Tomasiewicz, H. C., Jacobs, M. M., Wilkinson, M. B., Wilson, S. P., Nestler, E. J., and Hurd, Y. L. (2012). Proenkephalin mediates the enduring effects of adolescent cannabis exposure associated with adult opiate vulnerability. Biol. Psychiatry 72, 803–810. doi: 10.1016/j.biopsych.2012.04.026
Torres, O. V., Ladenheim, B., Jayanthi, S., McCoy, M. T., Krasnova, I. N., Vautier, F. A., et al. (2016). An acute methamphetamine injection downregulates the expression of several histone deacetylases (HDACs) in the mouse nucleus accumbens: potential regulatory role of HDAC2 expression. Neurotox. Res. 30, 32–40. doi: 10.1007/s12640-015-9591-3
Torres, O. V., McCoy, M. T., Ladenheim, B., Jayanthi, S., Brannock, C., Tulloch, I., et al. (2015). CAMKII-conditional deletion of histone deacetylase 2 potentiates acute methamphetamine-induced expression of immediate early genes in the mouse nucleus accumbens. Sci. Rep. 5:13396. doi: 10.1038/srep13396
Vassoler, F. M., Oliver, D. J., Wyse, C., Blau, A., Shtutman, M., Turner, J. R., et al. (2017). Transgenerational attenuation of opioid self-administration as a consequence of adolescent morphine exposure. Neuropharmacology 113, 271–280. doi: 10.1016/j.neuropharm.2016.10.006
Vassoler, F. M., White, S. L., Schmidt, H. D., Sadri-Vakili, G., and Pierce, R. C. (2013). Epigenetic inheritance of a cocaine-resistance phenotype. Nat. Neurosci. 16, 42–47. doi: 10.1038/nn.3280
Volkow, N. D., and Blanco, C. (2021). The changing opioid crisis: development, challenges and opportunities. Mol. Psychiatry 26, 218–233. doi: 10.1038/s41380-020-0661-4
Wang, J. C., Kapoor, M., and Goate, A. M. (2012). The genetics of substance dependence. Annu. Rev. Genomics Hum. Genet. 13, 241–261.
Wang, L., Lv, Z., Hu, Z., Sheng, J., Hui, B., Sun, J., et al. (2010). Chronic cocaine-induced H3 acetylation and transcriptional activation of CaMKIIalpha in the nucleus accumbens is critical for motivation for drug reinforcement. Neuropsychopharmacology 35, 913–928. doi: 10.1038/npp.2009.193
Warnault, V., Darcq, E., Levine, A., Barak, S., and Ron, D. (2013). Chromatin remodeling–a novel strategy to control excessive alcohol drinking. Transl. Psychiatry 3:e231. doi: 10.1038/tp.2013.4
Werner, C. T., Altshuler, R. D., Shaham, Y., and Li, X. (2021). Epigenetic mechanisms in drug relapse. Biol. Psychiatry 89, 331–338. doi: 10.1016/j.biopsych.2020.08.005
Wimmer, M. E., Vassoler, F. M., White, S. L., Schmidt, H. D., Sidoli, S., Han, Y., et al. (2019). Impaired cocaine-induced behavioral plasticity in the male offspring of cocaine-experienced sires. Eur. J. Neurosci. 49, 1115–1126. doi: 10.1111/ejn.14310
Wolstenholme, J. T., Warner, J. A., Capparuccini, M. I., Archer, K. J., Shelton, K. L., and Miles, M. F. (2011). Genomic analysis of individual differences in ethanol drinking: evidence for non-genetic factors in C57BL/6 mice. PLoS One 6:e21100. doi: 10.1371/journal.pone.0021100
Xu, W., Hong, Q., Lin, Z., Ma, H., Chen, W., Zhuang, D., et al. (2021). Role of nucleus accumbens microRNA-181a and MeCP2 in incubation of heroin craving in male rats. Psychopharmacology 238, 2313–2324. doi: 10.1007/s00213-021-05854-3
Yang, J., Li, L., Hong, S., Zhang, D., and Zhou, Y. (2020). Methamphetamine leads to the alterations of microRNA profiles in the nucleus accumbens of rats. Pharm. Biol. 58, 797–805. doi: 10.1080/13880209.2020.1803366
Yang, X. J. (2004). Lysine acetylation and the bromodomain: a new partnership for signaling. Bioessays 26, 1076–1087. doi: 10.1002/bies.20104
Keywords: epigenetic, substance use disorder, histone (de)acetylation, histone methlyation, nucleus accumbens, alcohol use disorder (AUD)
Citation: Anderson EM and Taniguchi M (2022) Epigenetic Effects of Addictive Drugs in the Nucleus Accumbens. Front. Mol. Neurosci. 15:828055. doi: 10.3389/fnmol.2022.828055
Received: 02 December 2021; Accepted: 30 May 2022;
Published: 23 June 2022.
Edited by:
Shusaku Uchida, Kyoto University, JapanReviewed by:
Yavin Shaham, National Institute on Drug Abuse (NIH), United StatesHiromasa Funato, Toho University, Japan
Copyright © 2022 Anderson and Taniguchi. This is an open-access article distributed under the terms of the Creative Commons Attribution License (CC BY). The use, distribution or reproduction in other forums is permitted, provided the original author(s) and the copyright owner(s) are credited and that the original publication in this journal is cited, in accordance with accepted academic practice. No use, distribution or reproduction is permitted which does not comply with these terms.
*Correspondence: Ethan M. Anderson, ZXRoYW4uYW5kZXJzb24ucGhkQGdtYWlsLmNvbQ==; Makoto Taniguchi, dGFuaWd1Y2hAbXVzYy5lZHU=