Gene and Cell Therapy for Epilepsy: A Mini Review
- 1Institute of Fundamental Medicine and Biology, Kazan Federal University, Kazan, Russia
- 2Center for Neuroscience, Ben Gurion University of the Negev, Be’er Sheva, Israel
Epilepsy is a chronic non-infectious disease of the brain, characterized primarily by recurrent unprovoked seizures, defined as an episode of disturbance of motor, sensory, autonomic, or mental functions resulting from excessive neuronal discharge. Despite the advances in the treatment achieved with the use of antiepileptic drugs and other non-pharmacological therapies, about 30% of patients suffer from uncontrolled seizures. This review summarizes the currently available methods of gene and cell therapy for epilepsy and discusses the development of these approaches. Currently, gene therapy for epilepsy is predominantly adeno-associated virus (AAV)-mediated delivery of genes encoding neuro-modulatory peptides, neurotrophic factors, enzymes, and potassium channels. Cell therapy for epilepsy is represented by the transplantation of several types of cells such as mesenchymal stem cells (MSCs), bone marrow mononuclear cells, neural stem cells, and MSC-derived exosomes. Another approach is encapsulated cell biodelivery, which is the transplantation of genetically modified cells placed in capsules and secreting various therapeutic agents. The use of gene and cell therapy approaches can significantly improve the condition of patient with epilepsy. Therefore, preclinical, and clinical studies have been actively conducted in recent years to prove the benefits and safety of these strategies.
Introduction
Epilepsy is one of the most common diseases of the nervous system (more than 50 million cases have been reported worldwide). This condition is characterized by recurrent unprovoked seizures that result from abnormally excessive firing of neurons due to an imbalance in levels of excitation and inhibition in the brain (Stafstrom and Carmant, 2015). Epilepsy can have a variety of etiologies: structural, infectious, metabolic, immune, genetic as well as unknown (Perucca et al., 2020). Despite the active research in this area, the causes of the disease are still unclear. Epilepsy is also a group of conditions that are heterogeneous in manifestations and causes, making it difficult to develop unambiguous diagnostic criteria (Thijs et al., 2019). Patients suffer from seizures that often worsen over time and is accompanied by cognitive function deterioration and mental health problems. Patients often become resistant to existing antiepileptic drugs (Sheng et al., 2018).
The production of effective methods of treatment is an urgent problem that requires an immediate solution since epilepsy is a serious medical and social problem. The risk of premature death in people with epilepsy is three times higher than in the general population (according to WHO1).
Currently, gene and cell therapy is being investigated as a way to reduce neuronal loss, inflammation, oxidative stress, and the frequency and duration of epileptic seizures. The new approaches being developed are capable of increasing the survival of neurons, improving neurogenesis, providing neuroprotection and preserving cognitive functions.
Thus, this paper discusses the promising results of gene and cell therapy for epilepsy. Table 1 and Figure 1 provide detailed information on existing in vivo studies and clinical trials.
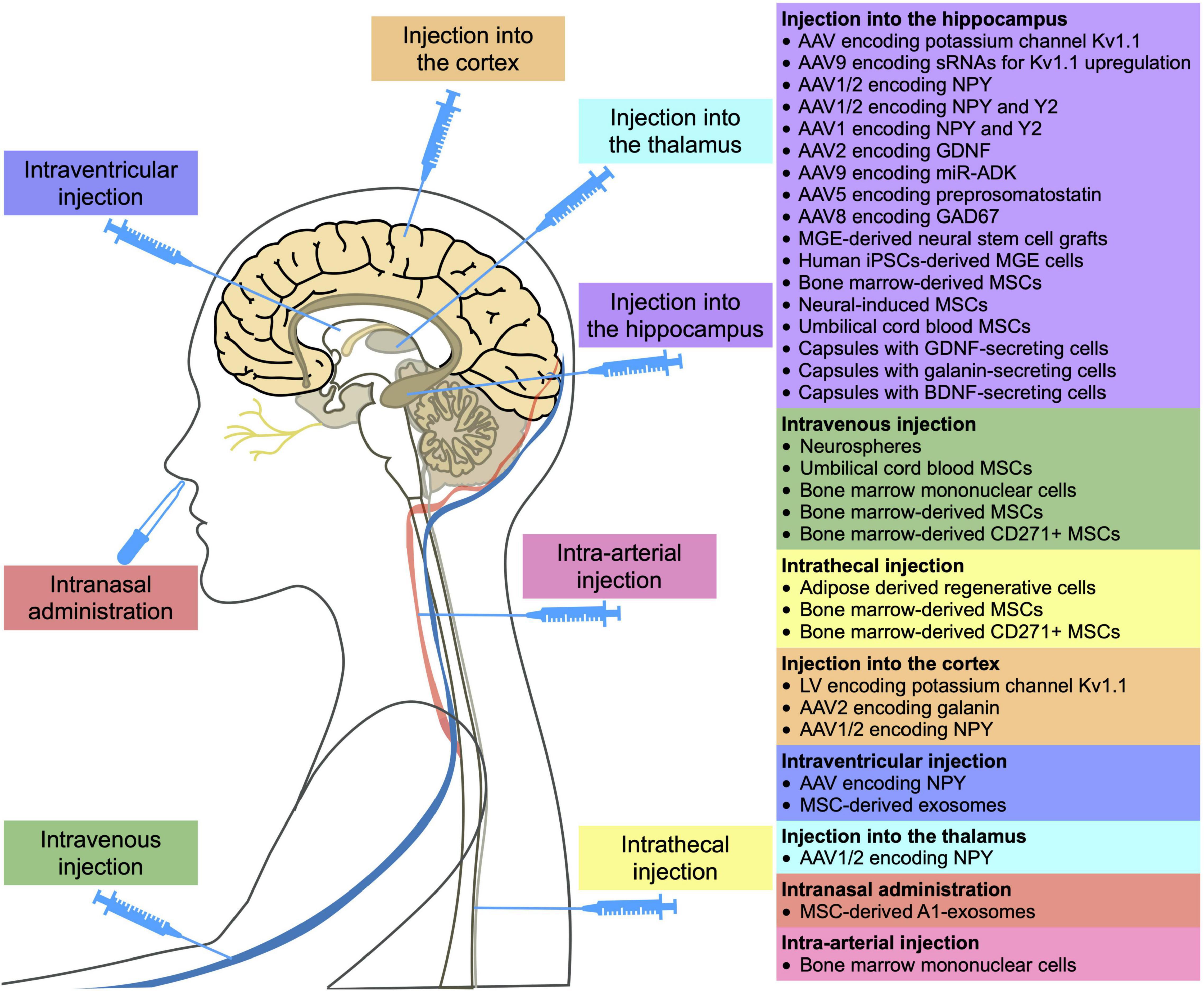
Figure 1. Methods of administration of gene and cell drugs for epilepsy reported in in vivo and clinical studies.
Gene Therapy
The current focus of gene therapy strategies for epilepsy is primarily aimed at reducing neuronal excitability by overexpressing neuro-modulatory peptides such as neuropeptide Y (Dong et al., 2013; Zhang et al., 2013), galanin etc. (McCown, 2006) or by the genetic modification of astrocytes, for example, to suppress adenosine kinase (ADK) expression (Young et al., 2014).
Overexpression of ion channels such as the Kv1 potassium channel, which reduces the intrinsic excitability of neurons, increases the threshold for action potential generation required for neuron firing. Thus, permanent inhibition of the increased internal excitability of neurons inside the epileptic focus may have a long-term antiepileptic effect (Snowball et al., 2019). In a rat model of chronic refractory focal neocortical epilepsy, a lentivirus encoding Kv1.1 was shown to suppress epileptic activity for several weeks upon injection into an epileptic focus (Wykes et al., 2012). In addition, the use of adeno-associated virus (AAV) overexpressing Kv1.1 has resulted in a decrease in both the frequency and duration of seizures in the temporal lobe epilepsy (TLE) model (Snowball et al., 2019). CRISPRa-mediated Kv1.1 upregulation decreased spontaneous generalized seizures in the mouse model of TLE (Colasante et al., 2020).
Another approach in antiepileptic treatment is the suppression of the activity of the excitatory mediator glutamate. Neuropeptide Y (NPY) preferentially binds to three G-protein coupled receptors (Y1, Y2, and Y5). Furthermore, the antiepileptic effect of NPY in the hippocampus is mediated by binding of NPY with presynaptic Y2 or Y5 receptors, which subsequently suppress glutamate release at excitatory synapses (Berglund et al., 2003). Binding of NPY to Y1 receptors leads to an increase in the epileptic activity (Benmaamar et al., 2003). Thus, genetic modification of the hippocampus by viruses with NPY can reduce the frequency of spontaneous seizures by 40% in both the TLE model (Noe et al., 2008) and kainate-induced seizures model (Dong et al., 2013; Zhang et al., 2013). Additionally, AAV-NPY was shown to have therapeutic effects when injected into the thalamus or somatosensory cortex (SC) of genetic epilepsy model rats (Powell et al., 2018). In addition to viruses encoding the NPY, viruses encoding the receptors for this protein have also been administered to suppress seizures, which significantly increased the antiepileptic effect. For example, the injection of AAV1/2 with the NPY and Y5 significantly reduced the number of kainate-induced seizures (Gotzsche et al., 2012). The use of AAV1/2 viral vector encoding the NPY in combination with Y2 resulted in the reduction in the number of kainate-induced seizures by 31–45% (Ledri et al., 2016; Melin et al., 2019). The mechanisms of the antiepileptic effect of NPY are still unclear. However, overexpression of NPY is known to result in the expression of the N-methyl-D-aspartate receptor subunits NR1, NR2A, and NR2B, which plays a critical role in the development of epilepsy (Dong et al., 2013). Moreover, the level of the synaptophysin protein, whose expression increased in the hippocampus of patients with epilepsy, was also decreased in the kainate-induced seizures model (Zhang et al., 2013). Overexpression of NPY has been studied in limited studies. Some studies have shown no effect on short- or long-term memory (Szczygiel et al., 2020), while others have illustrated that NPY decreases synaptic plasticity and negatively affects hippocampal-based spatial discrimination learning (Sorensen et al., 2008).
In addition to the treatment approaches described above, AAVs are actively being used to deliver proteins with therapeutic properties. Nevertheless, these are primarily in vivo studies that have not yet been further developed. For example, the introduction of AAV encoding the glial cell line-derived neurotrophic factor (GDNF) gene or the γ-aminobutyric acid decarboxylase 67 (GAD67) gene reduced the seizure frequency in the TLE model (Kanter-Schlifke et al., 2007; Shimazaki et al., 2019). Moreover, promising results were demonstrated for AVVs encoding preprosomatostatin injected into the hippocampus (Natarajan et al., 2017).
Astrocyte transduction using AAV9 differs from the approach based on genetic modification of neurons described above. For example, miR-mediated suppression of ADK expression in astrocytes allowed an increase in the concentration of adenosine, which plays a pivotal role in seizure termination, and led to a decrease in the duration of kainate-induced seizures (Young et al., 2014).
A potential limitation of the viral vectors for the treatment of epilepsy is that viruses can transduce not only the epileptic focus cells, but also surrounding brain areas, so it can be difficult to determine the optimal dosage to achieve a therapeutic effect without impairing normal brain function. These difficulties can be overcome by a new strategy for creating a group of G-protein coupled receptors called designer receptors exclusively activated by designer drugs (DREADDs). DREADD technology is based on mutated human muscarinic receptors, which, when expressed in cells, can only be specifically bound and activated by the pharmacologically inert compounds, such as clozapine-N-oxide (CNO). Systemic administration of CNO leads to the opening of Gi-protein gated inwardly rectifying potassium channels, resulting in membrane hyperpolarization and neuronal inhibition. The efficacy of DREADDs has already been confirmed in a number of therapeutically relevant animal models of epilepsy (Lieb et al., 2019). For example, injection of AAV5 expressing a synthetic receptor hM4Di into the dorsal hippocampus of rats with pilocarpine-induced epilepsy led to a significantly reduction of seizures (Katzel et al., 2014). Another limiting factor is the high invasiveness of virus injection methods. In addition, even the most non-immunogenic AAV-based vectors are capable of inducing both humoral and cellular immune responses, which can greatly influence the outcome of gene therapy, since neutralizing antibodies can bind the viral particles and significantly reduce the transduction efficiency (Ronzitti et al., 2020). Some viral vectors can also lead to insertional mutagenesis due to genome integration (Athanasopoulos et al., 2017). In addition, the capacity of the vector is of great importance. For example, AAVs can only package ∼4.7 kb of DNA (Weinberg and McCown, 2013).
In gene therapy for epilepsy, the use of AAVs encoding potassium channel or NPY genes are the most investigated. However, the evaluation of the effectiveness of these therapeutic agents has not gone beyond in vivo animal systems. To date, there are no registered clinical trials aimed at investigating gene drugs for the treatment of epilepsy. In this regard, we can say that gene therapy is at an early stage of development and obviously requires more attention from researchers.
Cell Therapy
Cell therapy for epilepsy includes a variety of approaches, including the use of MSCs (Abdanipour et al., 2011; Mohammed et al., 2014; Park et al., 2015; Hlebokazov et al., 2017, 2021; Fukumura et al., 2018; Salem et al., 2018; Melin et al., 2019; Szczepanik et al., 2020; Wang et al., 2021), mononuclear cells (Costa-Ferro et al., 2010; Leal et al., 2014; DaCosta et al., 2018), cells of the nervous system (Baraban et al., 2009; Waldau et al., 2010; Cunningham et al., 2014; de Gois da Silva et al., 2018; Upadhya et al., 2019), and encapsulated cells expressing various therapeutic factors (Kanter-Schlifke et al., 2009; Kuramoto et al., 2011; Nikitidou et al., 2014; Falcicchia et al., 2018; Paolone et al., 2019), as well as exosomes (Long et al., 2017; Xian et al., 2019). Cell therapy is an alternative treatment that can be used to reduce the incidence rate of seizures in epilepsy, including drug-resistant epilepsy, as shown in several clinical studies (Hlebokazov et al., 2017, 2021; DaCosta et al., 2018; Milczarek et al., 2018; Szczepanik et al., 2020).
Transplantation of Neural Cells
Promising approaches for epilepsy treatment are aimed at replenishing the neuronal loss in the hippocampus by transplanting cells of the nervous system. The neural stem cell is capable of self-renewal and differentiation into neurons, glial cells, and oligodendrocytes as well as expression of neurotrophic factors. Transplantation of neural stem cells into the hippocampus of epileptic rats led to an increase in the number of GABAergic neurons and cells expressing GDNF, which resulted in the suppression of seizures (Waldau et al., 2010).
The protective role of neurospheres, which are spherical clusters of neural stem cells grown in vitro, have also been studied. Intravenous administration of neurospheres into epileptic rats has been shown to reduce oxidative damage by significantly increasing the level of antioxidant enzymes such as glutathione, superoxide dismutase and catalase (de Gois da Silva et al., 2018).
It has been shown that progenitor cells from embryonic medial ganglionic eminence (MGE) can differentiate into functional GABAergic interneurons. Transplantation of MGE precursors into the cortex of model mice with a deletion of potassium channels led to a significant reduction in the duration and frequency of spontaneous electrographic seizures, which is achieved due to GABA-mediated synaptic inhibition (GABA is an inhibitory neurotransmitter) (Baraban et al., 2009).
GABAergic interneurons can be derived from induced pluripotent stem cells (iPSCs). It has been shown that transplantation of pluripotent stem cell-derived GABAergic interneurons into the hippocampus of model animals led to a decrease in seizures and other behavioral abnormalities (Cunningham et al., 2014; Upadhya et al., 2019).
Mesenchymal Stem Cell Transplantation
Mesenchymal stem cells (MSCs) have many therapeutic properties useful in epilepsy, including neuroprotection, immunomodulation, neurogenesis support, inflammation, and oxidative stress damage suppression. These effects are achieved due to the fact that MSCs secrete various neurotrophic factors, anti-inflammatory cytokines, growth factors and other biologically active molecules (Vizoso et al., 2017; Milczarek et al., 2018). It is also known that MSCs can cross the blood-brain barrier and migrate to the affected area. Even after intravenous administration, MSCs migrate into the hippocampus of model animals with epilepsy and have a therapeutic effect (Abdanipour et al., 2011).
Numerous studies have shown that the administration of undifferentiated MSCs can significantly decrease the frequency of seizures (Hlebokazov et al., 2017, 2021; Milczarek et al., 2018; Szczepanik et al., 2020), improve cognitive (Fukumura et al., 2018; Milczarek et al., 2018; Wang et al., 2021) and motor functions (Mohammed et al., 2014), increase the number of neurons (Abdanipour et al., 2011), reduce oxidative stress (Salem et al., 2018). The introduction of MSCs promotes the survival of GABAergic interneurons (Mohammed et al., 2014; Fukumura et al., 2018).
It has been shown that MSCs begin to secrete BDNF, NT3, and NT4 after neuronal differentiation. Their transplantation into the hippocampus of rats with kainic acid-induced epilepsy led to an increase in the level of BCL-2 and BCL-XL anti-apoptotic proteins and a decrease in the level of BAX pro-apoptotic protein in the hippocampus. Suppression of seizure activity and restoration of learning ability have also been noted (Wang et al., 2021).
The results of clinical studies also confirm benefits of MSC transplantation. For example, phase I/II clinical trials have demonstrated that the administration of antiepileptic drugs along with one or two intravenous and intrathecal administrations of MSCs has been safe and effective in treating patients with drug-resistant epilepsy. Combined use of levetiracetam (an antiepileptic drug) and MSCs led to a decrease in the frequency of seizures, and a repeated course of MSC administration contributed to a further improvement in the patients’ condition (NCT02497443) (Hlebokazov et al., 2017, 2021).
A combination therapy has also been used for pediatric patients with drug-resistant epilepsy. The intrathecal and intravenous administration of autologous bone marrow nucleated cells, followed by four intrathecal injections of MSCs every 3 months, resulted in neurological and cognitive improvement in all patients, including a decrease in the number of seizures (Milczarek et al., 2018).
Intrathecal administration of adipose-derived regenerative cells (a heterogeneous population of cells, including multipotent stem cells, fibroblasts, regulatory T-cells, and macrophages) into patients with autoimmune refractory epilepsy (3 times every 3 months) has also been reported. Only 1 out of 6 patients achieved complete remission (there were no seizures for more than 3 years), in 3 out of 6 there was a slight and short-term decrease in the frequency of seizures, and in two out of six no effect was found (Szczepanik et al., 2020). In all these clinical studies, some improvement after the introduction of cells without serious side effects was observed.
Exosome Injection
Not only MSCs, but also exosomes derived from them, exhibit immunoregulatory, anti-inflammatory and trophic effects (Harrell et al., 2019; Ha et al., 2020; Xunian and Kalluri, 2020), which means they also have great potential for the treatment of nervous systems diseases (Gorabi et al., 2019; Guy and Offen, 2020). As a result of intraventricular injection of exosomes, hippocampal astrocytes are able to take up the exosomes and attenuate astrogliosis and inflammation in model mice (Xian et al., 2019). After intranasal administration, exosomes also were able to reach the hippocampus, reduce the loss of glutamatergic and GABAergic neurons, and significantly reduce inflammation in the hippocampus of model animals (Long et al., 2017).
Mononuclear Cell Transplantation
Bone marrow mononuclear cells (BMCs) also possess immunomodulatory and neuroprotective properties (Yoshihara et al., 2007; Shrestha et al., 2014; do Prado-Lima et al., 2019; Wei et al., 2020) due to the secretion of various trophic factors and cytokines (Liu et al., 2004; Wei et al., 2020).
It has been shown that after intravenous administration to animals with status epilepticus, BMCs can migrate to the hippocampus, reduce the frequency and duration of seizures, maintain neuronal density (Costa-Ferro et al., 2010), increase proliferation of neurons, reduce the level of inflammatory cytokines and increase the level of anti-inflammatory cytokines (Leal et al., 2014).
Phase I/II clinical trials have investigated the safety and efficacy of intra-arterial injection of BMCs in TLE patients. Researchers demonstrated safety, improved memory, a decrease in theta activity, and a decrease in spike density (DaCosta et al., 2018).
Encapsulated Cell Biodelivery
Despite the proven efficacy, gene and cell therapy methods have a number of known disadvantages and side effects. It should be noted that these methods of therapy are irreversible and unregulated, and some of them can lead to an immune response and malignant transformation.
In the case of cell therapy, as mentioned above for gene therapy, the major limiting factor is safety, specifically the possibility of a significant immune response induction and oncogenic transformation. In some cases, long-term immunosuppressive therapy and monitoring of the cells after the administration are required (Aly, 2020). It is important to select a suitable donor in the case of allogeneic transplantation, not only in terms of HLA compatibility, but also in the heterogeneity of effector molecules secreted by the cells, which vary considerably between different donors and can lead to a significant variation in the treatment efficacy (Montzka et al., 2009; Mukhamedshina et al., 2019). The migration ability of cells also imposes restrictions on the use of cell-based therapy in clinical practice since highly invasive cell delivery is sometimes required to reach the target area and cross the blood-brain barrier (Issa et al., 2020).
One of the approaches to overcome some of the difficulties of cell-based therapy is encapsulated cell biodelivery (ECB). ECB usually involves the implantation of a capsule with a semipermeable membrane containing genetically modified cells that secrete therapeutic substances. In this case, the cells do not leave the capsule, but the therapeutically active molecules leave and spread in the area of transplantation. This method allows (1) preventing an immune response and transplant rejection, since the cells are physically isolated, (2) locally delivering therapeutic substances, and (3) stopping treatment by removing the capsule from the brain. To suppress epileptic activity, such capsules were designed to contain cells expressing GDNF (Kanter-Schlifke et al., 2009; Paolone et al., 2019), BDNF (Kuramoto et al., 2011; Falcicchia et al., 2018), or galanin (Nikitidou et al., 2014). ECB has shown positive results in epilepsy therapy since these neurotrophic factors and neuropeptide exhibit antiepileptic activity.
Conclusion
This review discussed recent developments in gene and cell therapy for epilepsy. To date, in vivo models have shown the potential benefit of viral vectors (mainly AAVs, rarely LVs) encoding genes of therapeutic agents. Thus, the effectiveness of viral delivery of (1) neuro-modulatory peptides, such as NPY or galanin, (2) potassium channels to inhibit increased internal excitability of neurons, (3) GDNF, (4) GAD67, (5) preprosomatostatin, as well as (6) miR to suppress ADK expression has been shown.
Cell-based therapy has made more progress with documented clinical trials showing the benefits and safety of MSC or BMC transplantation. The therapeutic effect can be achieved due to the neuroprotective, anti-inflammatory, immunomodulatory properties of these cells, which is also shown for MSC-derived exosomes. Researchers are also focusing on the transplantation of neural stem cells into the hippocampus to reduce neuronal loss in animal models. ECB, which is the injection of capsules with cells expressing BDNF, GDNF or galanin in the hippocampus, has shown an antiepileptic effect.
The majority of gene and cell therapies have not yet reached clinical practice, but they have made significant progress. To date, preclinical studies in animal model are ongoing and new clinical trials are being registered to confirm both the effectiveness and safety of these approaches. Considering the heterogeneous nature of the onset and manifestation of epilepsy, the development of methods of gene and cell therapy can make a significant contribution to progress in the treatment of this disease.
Author Contributions
AS, DC, VS, and AR: conceptualization. AS and DC: writing-original draft preparation. VS, AM, RG, ZA, and AR: writing-review and editing. AS: visualization. VS and AR: supervision. All authors have read and agreed to the published version of the manuscript.
Funding
This work was funded by the subsidy allocated to KFU for the state assignment 0671-2020-0058 in the sphere of scientific activities.
Conflict of Interest
The authors declare that the research was conducted in the absence of any commercial or financial relationships that could be construed as a potential conflict of interest.
Publisher’s Note
All claims expressed in this article are solely those of the authors and do not necessarily represent those of their affiliated organizations, or those of the publisher, the editors and the reviewers. Any product that may be evaluated in this article, or claim that may be made by its manufacturer, is not guaranteed or endorsed by the publisher.
Acknowledgments
We thank the Center for Precision Genome Editing and Genetic Technologies for Biomedicine, IGB RAS for scientific advice on development of gene therapy drugs. This article has been supported by the Kazan Federal University Strategic Academic Leadership Program (PRIORITY-2030).
Footnotes
References
Abdanipour, A., Tiraihi, T., and Mirnajafi-Zadeh, J. (2011). Improvement of the pilocarpine epilepsy model in rat using bone marrow stromal cell therapy. Neurol. Res. 33, 625–632. doi: 10.1179/1743132810Y.0000000018
Aly, R. M. (2020). Current state of stem cell-based therapies: an overview. Stem Cell Investig. 7:8. doi: 10.21037/sci-2020-001
Athanasopoulos, T., Munye, M. M., and Yanez-Munoz, R. J. (2017). Nonintegrating Gene Therapy Vectors. Hematol. Oncol. Clin. North Am. 31, 753–770. doi: 10.1016/j.hoc.2017.06.007
Baraban, S. C., Southwell, D. G., Estrada, R. C., Jones, D. L., Sebe, J. Y., Alfaro-Cervello, C., et al. (2009). Reduction of seizures by transplantation of cortical GABAergic interneuron precursors into Kv1.1 mutant mice. Proc. Natl. Acad. Sci. U S A 106, 15472–15477. doi: 10.1073/pnas.0900141106
Benmaamar, R., Pham-Le, B. T., Marescaux, C., Pedrazzini, T., and Depaulis, A. (2003). Induced down-regulation of neuropeptide Y-Y1 receptors delays initiation of kindling. Eur. J. Neurosci. 18, 768–774. doi: 10.1046/j.1460-9568.2003.02810.x
Berglund, M. M., Hipskind, P. A., and Gehlert, D. R. (2003). Recent developments in our understanding of the physiological role of PP-fold peptide receptor subtypes. Exp. Biol. Med. (Maywood) 228, 217–244. doi: 10.1177/153537020322800301
Colasante, G., Qiu, Y., Massimino, L., Di Berardino, C., Cornford, J. H., Snowball, A., et al. (2020). In vivo CRISPRa decreases seizures and rescues cognitive deficits in a rodent model of epilepsy. Brain 143, 891–905. doi: 10.1093/brain/awaa045
Costa-Ferro, Z. S., Vitola, A. S., Pedroso, M. F., Cunha, F. B., Xavier, L. L., Machado, D. C., et al. (2010). Prevention of seizures and reorganization of hippocampal functions by transplantation of bone marrow cells in the acute phase of experimental epilepsy. Seizure 19, 84–92. doi: 10.1016/j.seizure.2009.12.003
Cunningham, M., Cho, J. H., Leung, A., Savvidis, G., Ahn, S., Moon, M., et al. (2014). hPSC-derived maturing GABAergic interneurons ameliorate seizures and abnormal behavior in epileptic mice. Cell Stem Cell 15, 559–573. doi: 10.1016/j.stem.2014.10.006
DaCosta, J. C., Portuguez, M. W., Marinowic, D. R., Schilling, L. P., Torres, C. M., DaCosta, D. I., et al. (2018). Safety and seizure control in patients with mesial temporal lobe epilepsy treated with regional superselective intra-arterial injection of autologous bone marrow mononuclear cells. J. Tiss. Eng. Regen. Med. 12, e648–e656. doi: 10.1002/term.2334
de Gois da Silva, M. L., da Silva Oliveira, G. L., de Oliveira Bezerra, D., da Rocha, et al. (2018). Neurochemical properties of neurospheres infusion in experimental-induced seizures. Tissue Cell 54, 47–54. doi: 10.1016/j.tice.2018.08.002
do Prado-Lima, P. A. S., Onsten, G. A., de Oliveira, G. N., Brito, G. C., Ghilardi, I. M., de Souza, E. V., et al. (2019). The antidepressant effect of bone marrow mononuclear cell transplantation in chronic stress. J. Psychopharmacol. 33, 632–639. doi: 10.1177/0269881119841562
Dong, C., Zhao, W., Li, W., Lv, P., and Dong, X. (2013). Anti-epileptic effects of neuropeptide Y gene transfection into the rat brain. Neural Regen. Res. 8, 1307–1315. doi: 10.3969/j.issn.1673-5374.2013.14.007
Falcicchia, C., Paolone, G., Emerich, D. F., Lovisari, F., Bell, W. J., Fradet, T., et al. (2018). Seizure-Suppressant and Neuroprotective Effects of Encapsulated BDNF-Producing Cells in a Rat Model of Temporal Lobe Epilepsy. Mol. Ther. Methods Clin. Dev. 9, 211–224. doi: 10.1016/j.omtm.2018.03.001
Fukumura, S., Sasaki, M., Kataoka-Sasaki, Y., Oka, S., Nakazaki, M., Nagahama, H., et al. (2018). Intravenous infusion of mesenchymal stem cells reduces epileptogenesis in a rat model of status epilepticus. Epilep. Res. 141, 56–63. doi: 10.1016/j.eplepsyres.2018.02.008
Gorabi, A. M., Kiaie, N., Barreto, G. E., Read, M. I., Tafti, H. A., and Sahebkar, A. (2019). The Therapeutic Potential of Mesenchymal Stem Cell-Derived Exosomes in Treatment of Neurodegenerative Diseases. Mol. Neurobiol. 56, 8157–8167. doi: 10.1007/s12035-019-01663-0
Gotzsche, C. R., Nikitidou, L., Sorensen, A. T., Olesen, M. V., Sorensen, G., Christiansen, S. H., et al. (2012). Combined gene overexpression of neuropeptide Y and its receptor Y5 in the hippocampus suppresses seizures. Neurobiol. Dis. 45, 288–296. doi: 10.1016/j.nbd.2011.08.012
Guy, R., and Offen, D. (2020). Promising Opportunities for Treating Neurodegenerative Diseases with Mesenchymal Stem Cell-Derived Exosomes. Biomolecules 10:1320. doi: 10.3390/biom10091320
Ha, D. H., Kim, H. K., Lee, J., Kwon, H. H., Park, G. H., Yang, S. H., et al. (2020). Mesenchymal Stem/Stromal Cell-Derived Exosomes for Immunomodulatory Therapeutics and Skin Regeneration. Cells 9:1157. doi: 10.3390/cells9051157
Harrell, C. R., Jovicic, N., Djonov, V., Arsenijevic, N., and Volarevic, V. (2019). Mesenchymal Stem Cell-Derived Exosomes and Other Extracellular Vesicles as New Remedies in the Therapy of Inflammatory Diseases. Cells 8:1605. doi: 10.3390/cells8121605
Hlebokazov, F., Dakukina, T., Ihnatsenko, S., Kosmacheva, S., Potapnev, M., Shakhbazau, A., et al. (2017). Treatment of refractory epilepsy patients with autologous mesenchymal stem cells reduces seizure frequency: An open label study. Adv. Med. Sci. 62, 273–279. doi: 10.1016/j.advms.2016.12.004
Hlebokazov, F., Dakukina, T., Potapnev, M., Kosmacheva, S., Moroz, L., Misiuk, N., et al. (2021). Clinical benefits of single vs repeated courses of mesenchymal stem cell therapy in epilepsy patients. Clin. Neurol. Neurosurg. 207:106736. doi: 10.1016/j.clineuro.2021.106736
Issa, S., Shaimardanova, A., Valiullin, V., Rizvanov, A., and Solovyeva, V. (2020). Mesenchymal Stem Cell-Based Therapy for Lysosomal Storage Diseases and Other Neurodegenerative Disorders. Front. Pharmacol. 13:859516. doi: 10.3389/fphar.2022.859516
Kanter-Schlifke, I., Fjord-Larsen, L., Kusk, P., Angehagen, M., Wahlberg, L., and Kokaia, M. (2009). GDNF released from encapsulated cells suppresses seizure activity in the epileptic hippocampus. Exp. Neurol. 216, 413–419. doi: 10.1016/j.expneurol.2008.12.021
Kanter-Schlifke, I., Georgievska, B., Kirik, D., and Kokaia, M. (2007). Seizure suppression by GDNF gene therapy in animal models of epilepsy. Mol. Ther. 15, 1106–1113. doi: 10.1038/sj.mt.6300148
Katzel, D., Nicholson, E., Schorge, S., Walker, M. C., and Kullmann, D. M. (2014). Chemical-genetic attenuation of focal neocortical seizures. Nat. Commun. 5:3847. doi: 10.1038/ncomms4847
Kuramoto, S., Yasuhara, T., Agari, T., Kondo, A., Jing, M., Kikuchi, Y., et al. (2011). BDNF-secreting capsule exerts neuroprotective effects on epilepsy model of rats. Brain Res. 1368, 281–289. doi: 10.1016/j.brainres.2010.10.054
Leal, M. M., Costa-Ferro, Z. S., Souza, B. S., Azevedo, C. M., Carvalho, T. M., Kaneto, C. M., et al. (2014). Early transplantation of bone marrow mononuclear cells promotes neuroprotection and modulation of inflammation after status epilepticus in mice by paracrine mechanisms. Neurochem. Res. 39, 259–268. doi: 10.1007/s11064-013-1217-7
Ledri, L. N., Melin, E., Christiansen, S. H., Gotzsche, C. R., Cifra, A., Woldbye, D. P., et al. (2016). Translational approach for gene therapy in epilepsy: Model system and unilateral overexpression of neuropeptide Y and Y2 receptors. Neurobiol. Dis. 86, 52–61. doi: 10.1016/j.nbd.2015.11.014
Lieb, A., Weston, M., and Kullmann, D. M. (2019). Designer receptor technology for the treatment of epilepsy. EBioMedicine 43, 641–649. doi: 10.1016/j.ebiom.2019.04.059
Liu, Y., Guo, J., Zhang, P., Zhang, S., Chen, P., Ma, K., et al. (2004). Bone marrow mononuclear cell transplantation into heart elevates the expression of angiogenic factors. Microvasc. Res. 68, 156–160. doi: 10.1016/j.mvr.2004.06.008
Long, Q., Upadhya, D., Hattiangady, B., Kim, D. K., An, S. Y., Shuai, B., et al. (2017). Intranasal MSC-derived A1-exosomes ease inflammation, and prevent abnormal neurogenesis and memory dysfunction after status epilepticus. Proc. Natl. Acad. Sci. U S A 114, E3536–E3545. doi: 10.1073/pnas.1703920114
McCown, T. J. (2006). Adeno-associated virus-mediated expression and constitutive secretion of galanin suppresses limbic seizure activity in vivo. Mol. Ther. 14, 63–68. doi: 10.1016/j.ymthe.2006.04.004
Melin, E., Nanobashvili, A., Avdic, U., Gotzsche, C. R., Andersson, M., Woldbye, D. P. D., et al. (2019). Disease Modification by Combinatorial Single Vector Gene Therapy: A Preclinical Translational Study in Epilepsy. Mol. Ther. Methods Clin. Dev. 15, 179–193. doi: 10.1016/j.omtm.2019.09.004
Milczarek, O., Jarocha, D., Starowicz-Filip, A., Kwiatkowski, S., Badyra, B., and Majka, M. (2018). Multiple Autologous Bone Marrow-Derived CD271(+) Mesenchymal Stem Cell Transplantation Overcomes Drug-Resistant Epilepsy in Children. Stem Cells Transl. Med. 7, 20–33. doi: 10.1002/sctm.17-0041
Mohammed, A. S., Ewais, M. M., Tawfik, M. K., and Essawy, S. S. (2014). Effects of intravenous human umbilical cord blood mesenchymal stem cell therapy versus gabapentin in pentylenetetrazole-induced chronic epilepsy in rats. Pharmacology 94, 41–50. doi: 10.1159/000365219
Montzka, K., Lassonczyk, N., Tschoke, B., Neuss, S., Fuhrmann, T., Franzen, R., et al. (2009). Neural differentiation potential of human bone marrow-derived mesenchymal stromal cells: misleading marker gene expression. BMC Neurosci. 10:16. doi: 10.1186/1471-2202-10-16
Mukhamedshina, Y., Shulman, I., Ogurcov, S., Kostennikov, A., Zakirova, E., Akhmetzyanova, E., et al. (2019). Mesenchymal Stem Cell Therapy for Spinal Cord Contusion: A Comparative Study on Small and Large Animal Models. Biomolecules 9:811. doi: 10.3390/biom9120811
Natarajan, G., Leibowitz, J. A., Zhou, J., Zhao, Y., McElroy, J. A., King, M. A., et al. (2017). Adeno-associated viral vector-mediated preprosomatostatin expression suppresses induced seizures in kindled rats. Epilepsy Res 130, 81–92. doi: 10.1016/j.eplepsyres.2017.01.002
Nikitidou, L., Torp, M., Fjord-Larsen, L., Kusk, P., Wahlberg, L. U., and Kokaia, M. (2014). Encapsulated galanin-producing cells attenuate focal epileptic seizures in the hippocampus. Epilepsia 55, 167–174. doi: 10.1111/epi.12470
Noe, F., Pool, A. H., Nissinen, J., Gobbi, M., Bland, R., Rizzi, M., et al. (2008). Neuropeptide Y gene therapy decreases chronic spontaneous seizures in a rat model of temporal lobe epilepsy. Brain 131(Pt 6), 1506–1515. doi: 10.1093/brain/awn079
Paolone, G., Falcicchia, C., Lovisari, F., Kokaia, M., Bell, W. J., Fradet, T., et al. (2019). Long-Term, Targeted Delivery of GDNF from Encapsulated Cells Is Neuroprotective and Reduces Seizures in the Pilocarpine Model of Epilepsy. J. Neurosci. 39, 2144–2156. doi: 10.1523/JNEUROSCI.0435-18.2018
Park, G. Y., Lee, E. M., Seo, M. S., Seo, Y. J., Oh, J. S., Son, W. C., et al. (2015). Preserved Hippocampal Glucose Metabolism on 18F-FDG PET after Transplantation of Human Umbilical Cord Blood-derived Mesenchymal Stem Cells in Chronic Epileptic Rats. J. Kor. Med. Sci. 30, 1232–1240. doi: 10.3346/jkms.2015.30.9.1232
Perucca, P., Bahlo, M., and Berkovic, S. F. (2020). The Genetics of Epilepsy. Annu. Rev. Genom. Hum. Genet. 21, 205–230. doi: 10.1146/annurev-genom-120219-074937
Powell, K. L., Fitzgerald, X., Shallue, C., Jovanovska, V., Klugmann, M., Von Jonquieres, G., et al. (2018). Gene therapy mediated seizure suppression in Genetic Generalised Epilepsy: Neuropeptide Y overexpression in a rat model. Neurobiol. Dis. 113, 23–32. doi: 10.1016/j.nbd.2018.01.016
Ronzitti, G., Gross, D. A., and Mingozzi, F. (2020). Human Immune Responses to Adeno-Associated Virus (AAV) Vectors. Front. Immunol. 11:670. doi: 10.3389/fimmu.2020.00670
Salem, N. A., El-Shamarka, M., Khadrawy, Y., and El-Shebiney, S. (2018). New prospects of mesenchymal stem cells for ameliorating temporal lobe epilepsy. Inflammopharmacology 26, 963–972. doi: 10.1007/s10787-018-0456-2
Sheng, J., Liu, S., Qin, H., Li, B., and Zhang, X. (2018). Drug-Resistant Epilepsy and Surgery. Curr. Neuropharmacol. 16, 17–28. doi: 10.2174/1570159X15666170504123316
Shimazaki, K., Kobari, T., Oguro, K., Yokota, H., Kasahara, Y., Murashima, Y., et al. (2019). Hippocampal GAD67 Transduction Using rAAV8 Regulates Epileptogenesis in EL Mice. Mol. Ther. Methods Clin. Dev. 13, 180–186. doi: 10.1016/j.omtm.2018.12.012
Shrestha, R. P., Qiao, J. M., Shen, F. G., Bista, K. B., Zhao, Z. N., and Yang, J. (2014). Intra-Spinal Bone Marrow Mononuclear Cells Transplantation Inhibits the Expression of Nuclear Factor-kappaB in Acute Transection Spinal Cord Injury in Rats. J. Kor. Neurosurg. Soc. 56, 375–382. doi: 10.3340/jkns.2014.56.5.375
Snowball, A., Chabrol, E., Wykes, R. C., Shekh-Ahmad, T., Cornford, J. H., Lieb, A., et al. (2019). Epilepsy Gene Therapy Using an Engineered Potassium Channel. J. Neurosci. 39, 3159–3169. doi: 10.1523/JNEUROSCI.1143-18.2019
Sorensen, A. T., Kanter-Schlifke, I., Carli, M., Balducci, C., Noe, F., During, M. J., et al. (2008). NPY gene transfer in the hippocampus attenuates synaptic plasticity and learning. Hippocampus 18, 564–574. doi: 10.1002/hipo.20415
Stafstrom, C. E., and Carmant, L. (2015). Seizures and epilepsy: an overview for neuroscientists. Cold Spring Harb. Perspect. Med. 5:a022426. doi: 10.1101/cshperspect.a022426
Szczepanik, E., Mierzewska, H., Antczak-Marach, D., Figiel-Dabrowska, A., Terczynska, I., Tryfon, J., et al. (2020). Intrathecal Infusion of Autologous Adipose-Derived Regenerative Cells in Autoimmune Refractory Epilepsy: Evaluation of Safety and Efficacy. Stem Cells Int. 2020:7104243. doi: 10.1155/2020/7104243
Szczygiel, J. A., Danielsen, K. I., Melin, E., Rosenkranz, S. H., Pankratova, S., Ericsson, A., et al. (2020). Gene Therapy Vector Encoding Neuropeptide Y and Its Receptor Y2 for Future Treatment of Epilepsy: Preclinical Data in Rats. Front. Mol. Neurosci. 13:232. doi: 10.3389/fnmol.2020.603409
Thijs, R. D., Surges, R., O’Brien, T. J., and Sander, J. W. (2019). Epilepsy in adults. Lancet 393, 689–701. doi: 10.1016/S0140-6736(18)32596-0
Upadhya, D., Hattiangady, B., Castro, O. W., Shuai, B., Kodali, M., Attaluri, S., et al. (2019). Human induced pluripotent stem cell-derived MGE cell grafting after status epilepticus attenuates chronic epilepsy and comorbidities via synaptic integration. Proc. Natl. Acad. Sci. U S A 116, 287–296. doi: 10.1073/pnas.1814185115
Vizoso, F. J., Eiro, N., Cid, S., Schneider, J., and Perez-Fernandez, R. (2017). Mesenchymal Stem Cell Secretome: Toward Cell-Free Therapeutic Strategies in Regenerative Medicine. Int. J. Mol. Sci. 18, 1852. doi: 10.3390/ijms18091852
Waldau, B., Hattiangady, B., Kuruba, R., and Shetty, A. K. (2010). Medial ganglionic eminence-derived neural stem cell grafts ease spontaneous seizures and restore GDNF expression in a rat model of chronic temporal lobe epilepsy. Stem Cells 28, 1153–1164. doi: 10.1002/stem.446
Wang, L., Zhao, Y., Pan, X., Zhang, Y., Lin, L., Wu, Y., et al. (2021). Adipose-derived stem cell transplantation improves learning and memory via releasing neurotrophins in rat model of temporal lobe epilepsy. Brain Res. 1750:147121. doi: 10.1016/j.brainres.2020.147121
Wei, W., Li, L., Deng, L., Wang, Z. J., Dong, J. J., Lyu, X. Y., et al. (2020). Autologous Bone Marrow Mononuclear Cell Transplantation Therapy Improved Symptoms in Patients with Refractory Diabetic Sensorimotor Polyneuropathy via the Mechanisms of Paracrine and Immunomodulation: A Controlled Study. Cell Transplant. 29:963689720949258. doi: 10.1177/0963689720949258
Weinberg, M. S., and McCown, T. J. (2013). Current prospects and challenges for epilepsy gene therapy. Exp. Neurol. 244, 27–35. doi: 10.1016/j.expneurol.2011.10.003
Wykes, R. C., Heeroma, J. H., Mantoan, L., Zheng, K., MacDonald, D. C., Deisseroth, K., et al. (2012). Optogenetic and potassium channel gene therapy in a rodent model of focal neocortical epilepsy. Sci. Transl. Med. 4:161ra152. doi: 10.1126/scitranslmed.3004190
Xian, P., Hei, Y., Wang, R., Wang, T., Yang, J., Li, J., et al. (2019). Mesenchymal stem cell-derived exosomes as a nanotherapeutic agent for amelioration of inflammation-induced astrocyte alterations in mice. Theranostics 9, 5956–5975. doi: 10.7150/thno.33872
Xunian, Z., and Kalluri, R. (2020). Biology and therapeutic potential of mesenchymal stem cell-derived exosomes. Cancer Sci. 111, 3100–3110. doi: 10.1111/cas.14563
Yoshihara, T., Ohta, M., Itokazu, Y., Matsumoto, N., Dezawa, M., Suzuki, Y., et al. (2007). Neuroprotective effect of bone marrow-derived mononuclear cells promoting functional recovery from spinal cord injury. J. Neurotrauma 24, 1026–1036. doi: 10.1089/neu.2007.132R
Young, D., Fong, D. M., Lawlor, P. A., Wu, A., Mouravlev, A., McRae, M., et al. (2014). Adenosine kinase, glutamine synthetase and EAAT2 as gene therapy targets for temporal lobe epilepsy. Gene Ther. 21, 1029–1040. doi: 10.1038/gt.2014.82
Keywords: epilepsy, gene therapy, cell therapy, adeno-associated virus, mesenchymal stem cells, neural stem cells, mononuclear cells, encapsulated cell biodelivery
Citation: Shaimardanova AA, Chulpanova DS, Mullagulova AI, Afawi Z, Gamirova RG, Solovyeva VV and Rizvanov AA (2022) Gene and Cell Therapy for Epilepsy: A Mini Review. Front. Mol. Neurosci. 15:868531. doi: 10.3389/fnmol.2022.868531
Received: 02 February 2022; Accepted: 30 March 2022;
Published: 11 May 2022.
Edited by:
Tobias Engel, Royal College of Surgeons in Ireland, IrelandReviewed by:
Dinesh Upadhya, Manipal Academy of Higher Education, IndiaCopyright © 2022 Shaimardanova, Chulpanova, Mullagulova, Afawi, Gamirova, Solovyeva and Rizvanov. This is an open-access article distributed under the terms of the Creative Commons Attribution License (CC BY). The use, distribution or reproduction in other forums is permitted, provided the original author(s) and the copyright owner(s) are credited and that the original publication in this journal is cited, in accordance with accepted academic practice. No use, distribution or reproduction is permitted which does not comply with these terms.
*Correspondence: Albert A. Rizvanov, rizvanov@gmail.com