- 1Aquaculture Genetics and Breeding Laboratory, The Ohio State University South Centers, Piketon, OH, United States
- 2College of Fisheries, Huazhong Agricultural University, Wuhan, China
- 3Department of Immunology, College of Medicine, University of Manitoba, Winnipeg, MB, Canada
- 4College of Chemistry and Life Science, Zhejiang Normal University, Jinhua, China
Exposure to stress induces a series of responses and influences a wide range of biological processes including sex differentiation in fish. The present work investigated the molecular and physiological response to thermal stress throughout the early development stage covering the whole period of sex differentiation of bluegill, Lepomis macrochirus. Larvae were treated using three temperatures, 17, 24, and 32°C from 6 to 90 days posthatching (dph) in 30-L round tanks. There is no significant difference of the sex ratio and survival among the three temperature groups in the geographic population used in this study. Two ovarian differentiation-related genes foxl2 and cyp19a1a were detected at 7 dph suggesting that these genes have already played a role prior to sex differentiation. The expression of foxl2 reached the peak and was thermosensitive just prior to the onset of ovarian differentiation at 27 dph. Histological examination displayed that the proliferation of germ cells and ovarian differentiation were delayed at the low-temperature treatment (17°C) at 97 dph compared with higher temperatures. In conclusion, the water temperature regulates the sex differentiation of bluegill through modulation of the expression of foxl2 and cyp19a1a. A comparative study of the expression profile of sex differentiation-related genes in species will shed light on the evolution of sex-determination mechanisms and the impact of stress on sex differentiation.
Introduction
Sex could be initiated by genetic or environmental signals (e.g., temperature), and the sex-determining gene(s) vary among fishes (Kikuchi and Hamaguchi, 2013; Bachtrog et al., 2014; Shen and Wang, 2014, 2018a; Mei and Gui, 2015; Shen et al., 2016; Wang and Shen, 2018). The sex differentiation-related genes are relatively conserved (Siegfried, 2010; Kobayashi et al., 2013; Shen and Wang, 2014). Several studies explored the molecular aspects of sex differentiation, such as transcription factor genes (foxl2, dmrt1, and sox9) and steroidogenic-related genes (cyp19a1, amh, and sf-1). This line of research is important because comparative approaches across diverse taxa expand the understanding of the molecular function and interactions, cellular behaviors (e.g., germ cells), and evolution of signaling pathways in the realization of phenotypic sex.
A general schematic diagram represents the molecular players that are involved in sex differentiation of teleosts, which employ genetic sex determination (GSD) (Figure 1). Most of the interactions between ovarian differentiation-related genes (e.g., foxl2 and cyp19a1) and testicular differentiation-related genes (e.g., dmrt1, amh, and sox9) are elusive. However, the expression patterns are somewhat clear. Of these candidate genes, foxl2 and cyp19a1a, have received considerably more attention. The foxl2 is a forkhead domain transcription factor, which is required for granulosa cell differentiation and ovarian maintenance (Schmidt et al., 2004; Baron et al., 2005; Uhlenhaut and Treier, 2006; Corpuz et al., 2010; Kashimada et al., 2011; Georges et al., 2013). Sexual dimorphic expression of foxl2 during sex differentiation has been found in all species investigated except American Alligator Alligator mississippiensis, including species with either temperature-dependent sex determination (TSD) or GSD (Table 1). The expression of foxl2 also generally displays a parabola trend with a climax at the critical point of or right before sex differentiation. Regarding TSD, its expression displays a thermo-sensitive pattern, with female-producing (promoting) temperature increasing and male-producing temperature decreasing its expression (Table 1). The pivotal role of the foxl2 gene in fish ovarian differentiation has been confirmed by the evidence that foxl2 could upregulate aromatase gene transcription directly by binding to the promoter region of cyp19a1a, or indirectly through interacting with sf-1 (steroidgenic factor 1, also known as Ad4BP or Nr5a1) (Wang et al., 2007; Yamaguchi et al., 2007). Furthermore, foxl2 is regulated by water temperature and involved in temperature-induced sex reversal in Japanese flounder with TSD (Yamaguchi et al., 2007). Recently, a research study showed that XX female medaka with disrupted foxl3 (a paralog of foxl2) developed functional sperm (Nishimura et al., 2015), suggesting the crucial role of foxl3 in female fate.
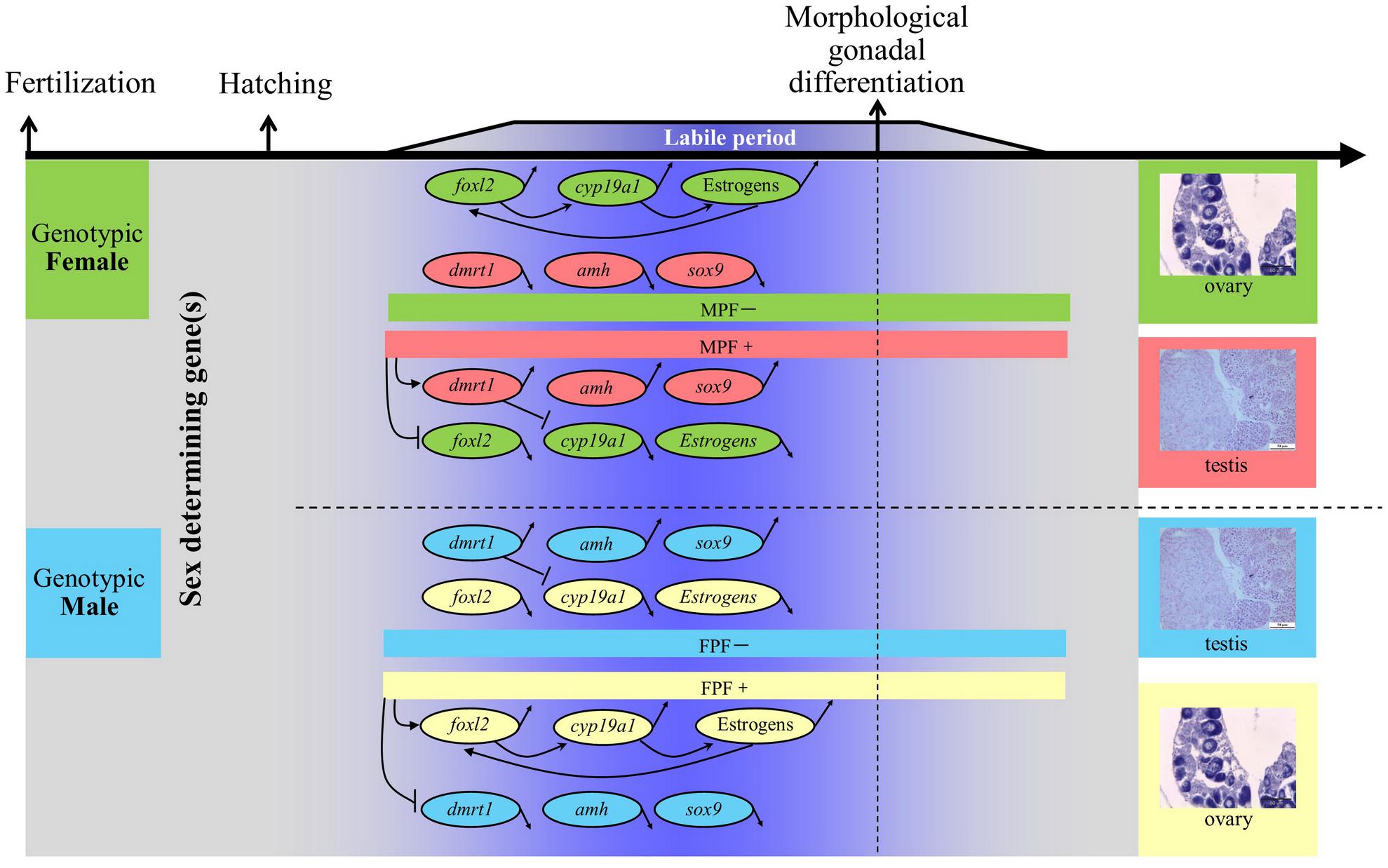
FIGURE 1. General schematic diagram of molecular players involved in sex differentiation of gonochoristic teleost with genetic sex determination. Note that this represents a “typical” sex-differentiation pathway, while large-scale variations exist with respect to timing and sexual dimorphism of expression, as well as regulatory mechanisms. MPF, male producing/promoting factors, including androgens, estrogen receptor antagonists, aromatase inhibitors, male producing temperature, etc. FPF, female producing/promoting factors, including estrogens, androgen receptor antagonists, female producing temperature, etc. Upward/downward arrows indicate the expression of specific gene is upregulated/downregulated, respectively. Arrows pointing to specific gene indicate that the expression is upregulated. Inhibiting symbols pointing to specific gene indicate that the expression is downregulated. Data refer to D’Cotta et al. (2007); Vizziano et al. (2007); Wang et al. (2007, 2010); Yamaguchi et al. (2007); Baron et al. (2008); Ijiri et al. (2008); Liu et al. (2010); Siegfried (2010); Poonlaphdecha et al. (2013); and Shen and Wang (2014).
The cyp19a1a gene is expressed in the undifferentiated, differentiating, and differentiated gonads, as well as organs of adult fish and encodes aromatase, which is a key enzyme during the formation of estrogens from androgens (Piferrer and Blázquez, 2005; Guiguen et al., 2010; Shen and Wang, 2014). The expression of cyp19a1a is increased prior to morphological sex differentiation in the gonochoristic fish species and is tightly related with temperature-induced feminization (Siegfried, 2010; Shen and Wang, 2014). The cyp19a1a is also involved in natural sex reversal of hermaphroditic species (Huang et al., 2009).
Changes in the water temperature result in various physiological and molecular stress responses to maintain the essential biological functions in fish and other aquatic species (Eissa and Wang, 2013, 2016; Eissa et al., 2017, 2018). Bluegill sunfish (Lepomis macrochirus) belongs to Centrarchidae (Near and Koppelman, 2009) and receives much attention because of the extraordinary biological characteristics, such as sex-determining mechanism (Wang et al., 2014; Shen et al., 2016), hybridization (Shen and Wang, 2018b), and alternative mating tactics (Gross and Charnov, 1980; Dominey, 1981; Garner and Neff, 2013). These biological features influence population sex ratio solely or interactively and make bluegill to be an excellent organism for investigation of interactions, population dynamics, speciation, and sexual selection.
To our knowledge, molecular pathways involved in sex differentiation of bluegill have not been studied yet. Therefore, the present study aimed to investigate the molecular players that can regulate the sex differentiation of bluegill.
Materials and Methods
Fish, Experimental Design, and Sampling Points
The experiments were conducted in accordance with the ethical standards and according to the national and international guidelines. This study and all experimental procedures involving animals were performed according to the protocol that was approved by The Ohio State University Institutional Animal Care and Use Committee.
Larvae were produced according to the procedures of bluegill out-of-season spawning established in the Aquaculture Genetics and Breeding Lab at The Ohio State University South Centers (Gao et al., 2009; Wang et al., 2014). Newly hatched larvae were reared at 24 ± 1°C in 400-L round tanks (spawning tank). Five-days posthatching (dph) larvae were transferred to 30-L round tanks with aeration and flow-through water. The experiment consisted of three different temperature treatments: 17, 24, and 32°C. On the next day of the transfer on 6 dph, water temperatures were adjusted to targeted ones within a week period, using cold well water (17°C), heated water (24°C), or heated water plus heaters (32°C). Temperature treatments were carried out in triplicates, each having 500 larvae. After 90 dph, water temperatures for all groups were gradually adjusted to 24°C and then maintained at 24 ± 1°C until the end of the experiment. Photoperiod was adjusted at 16 h light and 8 h dark during the experiment.
Fish were periodically sacrificed with an overdose of MS-222 (300 mg kg-1) and whole fish samples were collected at 7, 17, 27, 37, and 57 dph from each treatment replicate. These sampling points cover the critical period of sex differentiation in bluegill according to Gao et al. (2009). The whole fish samples were stored in RNAlater® Stabilization Solution (Ambion®, Life Technologies, United States) at 4°C overnight and transferred to -80°C until further analysis. Moreover, six fish from each temperature group were overanesthetized and fixed in 10% formalin (Anatech Ltd., MI, United States) at 57 and 97 dph for histological examination.
RNA Extraction and cDNA Synthesis
Total RNA was extracted from the individual whole fish samples (except 7 dph, for which 30 samples taken from each treatment replicate were pooled) by homogenization in TRIzol® Reagent (Ambion®, Life Technologies, United States) following the manufacturer’s procedure. Concentrations were assessed by spectrophotometry (NanoDrop 1000, Thermo Fisher Scientific Inc., United States). The quality of the RNA was checked by electrophoresis on 1% agarose gel (SYBR® Green stain) and by A260 nm/A280 nm ratios. Isolated RNA samples were then treated with RQ1 RNase-Free DNase (Promega Corporation) according to the manufacturer’s procedure. Total RNA (1 μg) was reverse-transcribed to cDNA with high-capacity cDNA Reverse Transcription Kits (Applied Biosystems®, Life Technologies, United States) following the manufacturer’s instructions.
Quantitative and Qualitative Gene Expression Analysis
Real-time reverse transcription polymerase chain reaction (RT-PCR) reactions were performed with an ABI 7500 real-time PCR System (7500 Software v2.0.6, Applied Biosystems®) using the SYBR® Select Master Mix (Applied Biosystems). Primer sequences (5′–3′) used in the present study were: foxl2 forward CAGAGCATGGCGCTCCCCAGC, reverse AACGCCGAGTGTTTGGTCTCGTG (target length 227 bp); 18S rRNA forward AGGAATTGACGGAAGGGCAC, reverse GGTGAGGTTTCCCGTGTTGA (target length 73 bp); and cyp19a1a forward ACTCACTTAGACGGCTTGGACAG, reverse CACTCACAGGTACACCCAGGAAG (target length 109 bp). Primers were designed according to available sequences. The PCR conditions were optimized through gradient testing for the best annealing temperature, combining melt curve and electrophoresis for best primer concentration, etc. before quantitative analysis. Data analysis using the 2-ΔΔCt method was applied in the study (Livak and Schmittgen, 2001; Schmittgen and Livak, 2008). Therefore, primer PCR efficiencies (E) were evaluated with the standard curve method, and only primers for foxl2 (E = 104.048%) and 18S rRNA (E = 106.331%) were adopted for qRT-PCR. Primers for cyp19a1a were only used for qualitative analysis (express or not). The samples from 7 dph larvae were applied as a calibrator. Hence, exponential power for the fold change of gene expression deduced from 2-ΔΔCt formula can be expressed as follows:
Where Ct, foxl2 and Ct,18SrRNA denote the threshold cycles (Ct) for the target gene foxl2 and reference gene 18S rRNA, respectively; Time X denotes different sampling point; Time 0 denotes the calibrator sampling point, which was 7 dph in the study.
Triplicates were run in a MicroAmp® Optical 96-Well Reaction Plate (Applied Biosystems®) in a final volume of 20 μL, which consisted of 10 μL SYBR® Select Master Mix (2×), 300 nM of each primer (final concentration), 1 μL cDNA template (from 50 ng RNA), and double distilled water. Cycling parameters were 50°C for 2 min, 95°C for 2 min, followed by 40 cycles of amplification at 95°C for 15 s, annealing at 60°C for 15 s, and extension at 72°C for 1 min. Finally, the temperature melt curve step was performed at the end of the amplification phase to check non-specific amplification. In addition, electrophoresis of PCR products was performed to check early expression of cyp19a1a, foxl2, and 18S rRNA qualitatively, non-specific amplification, and the length of target genes. Raw data were exported from 7500 Software v2.0.6 in Excel format for further statistical analysis.
Histological Analysis and Sex Identification
Histological sectioning was carried out to investigate the effects of temperature on sex differentiation. The fish heads were removed prior to the branchiostegal membrane, and the tails were removed after the anal opening. The remaining middle portion samples were transferred into 70% ethanol, dehydrated, and embedded in paraffin. Cross- or longitudinal sections of 6–7 μm were cut, and slices were stained with H&E, and counterstained with eosin. Tissue slices were examined and photographed under a light microscope with an imaging system (Olympus MicroSuite FIVE, FL, United States). Development stages and cellular identification were based on descriptions and photographs from Gao et al. (2009). All fish were overanesthetized and subjected to sex identification under microscope using the gonadal squash method at the age of 170–190 dph.
Statistical Analysis
Differences in mean gene expression among three different temperature treatments and at different days posthatching were analyzed by two-way analysis of variance (Two-Way ANOVA, General Linear Model). Before the analysis, data were tested for normality (Shapiro-Wilk test) and gene expression levels ln (natural log) transformed to ensure the homogeneity of variances. Differences of sex ratio among the three temperature groups or deviation from the balanced sex ratio of 1:1 were analyzed by Chi-squared test. Statistical analyses were performed with IBM SPSS Statistics Version 19. Differences were considered statistically significant when P < 0.05.
Results
Early Expression of foxl2 and cyp19a1a
Both genes related to sex differentiation, foxl2 and cyp19a1a, were expressed as early as 7 dph (at 5.5 mm total length, Figure 2).
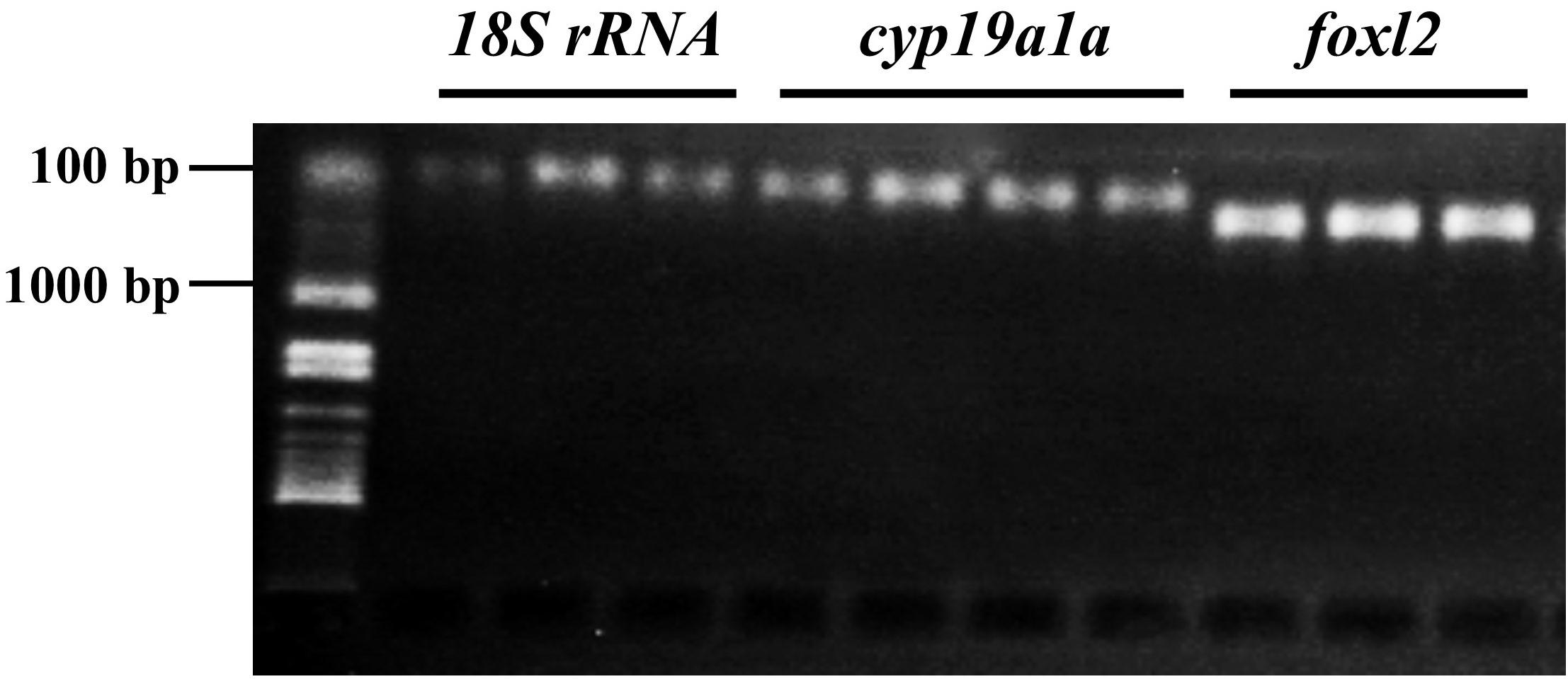
FIGURE 2. Expression of sex differentiation-related gene foxl2 and cyp19a1a at 7 and 17 days posthatching (dph) in bluegill L. macrochirus. The leftmost sample in sample group of each gene was from 7 dph individual, and the rest two or three were from 17 dph individuals. The leftmost column was molecular size marker.
Temporal Expression of foxl2 and Temperature Effects
The RT-qPCR standard curves exhibited a significant linear relationship between the values of threshold cycle (CT) and the gene copy number in both foxl2 and 18S rRNA genes (Supplementary Figure S1). The PCR efficiencies of both genes were high (104.048 and 106.331%), indicating the reliability of primers for quantification of these genes.
Expression of foxl2 increased dramatically from 7 to 17 dph, stabilized from 17 to 27 dph, and then started to decrease significantly at 37 dph (Figure 3). Remarkable effects of temperature on foxl2 expression were observed at 27 dph, where foxl2 expression was the highest in 17°C treatment and the lowest in 24°C treatment. No dph–temperature interaction on foxl2 expression was observed. Furthermore, the expression of foxl2 of four individuals at 37 dph in 32°C treatment was dramatically lower than other groups, even significantly lower than the samples at 7 dph.
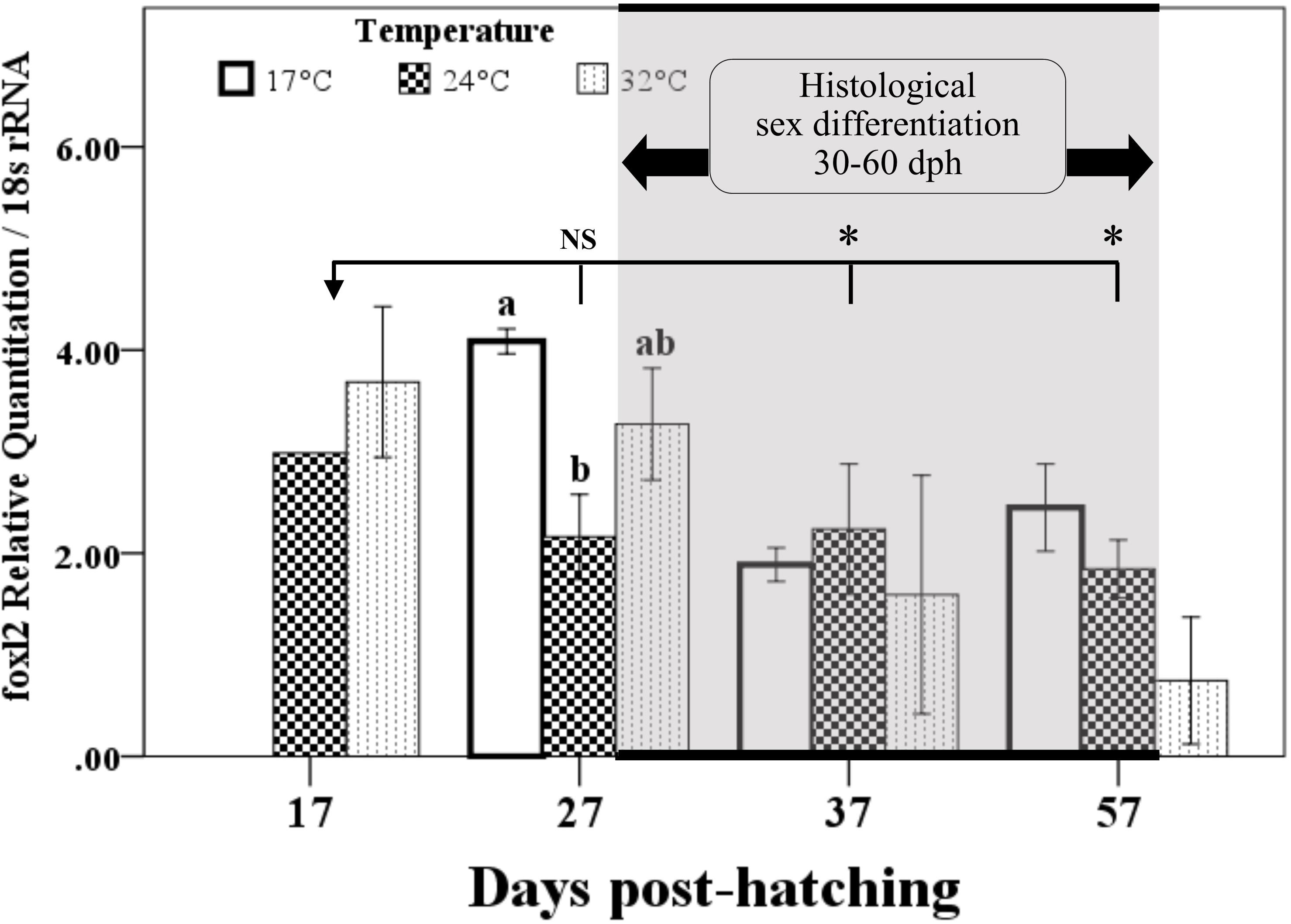
FIGURE 3. Temporal expression of foxl2 and temperature effects in the early life stage of bluegill L. macrochirus normalized against 18S rRNA measured by real-time RT-PCR. Results are mean ± SE. Different letters in 27 days posthatching (dph) sampling point indicate significant difference among temperature treatments. Asterisks denote significant difference between 17 and 37 dph groups or 17 and 57 dph groups; NS, no significance between 17 and 27 dph group when pooling different temperature treatments together. Gray shadow area indicates that histological sex differentiation occurs between 30 to 60 dph according to our previous study (Gao et al., 2009).
Gonadal Histology
Gonadal histology of bluegill larvae at different rearing temperatures was investigated from 57 to 97 dph in the present work (Figure 4). Sex could be distinguished in the 24°C (Figures 4D,E) and 32°C treatment groups (Figures 4H,I), but not in the 17°C group (Figure 4A) at 57 dph. Ovaries differentiated earlier, in which primordial germ cells overnumbered on 57 dph in comparison with presumptive testis of larvae reared at 24 and 32°C. In addition, ovaries developed dramatically from 57 to 97 dph in light of which the perinucleolus stage oocytes had been observed at 97 dph both in the 24 and 32°C groups (Figures 4F,J). In contrast, testes did not display obvious changes from 57 to 97 dph (Figures 4G,K) in any treatment group, other than size increase. Ovarian development was inhibited by low temperature because sex could not be identified up to 97 dph in the 17°C group (Figures 4B,C).
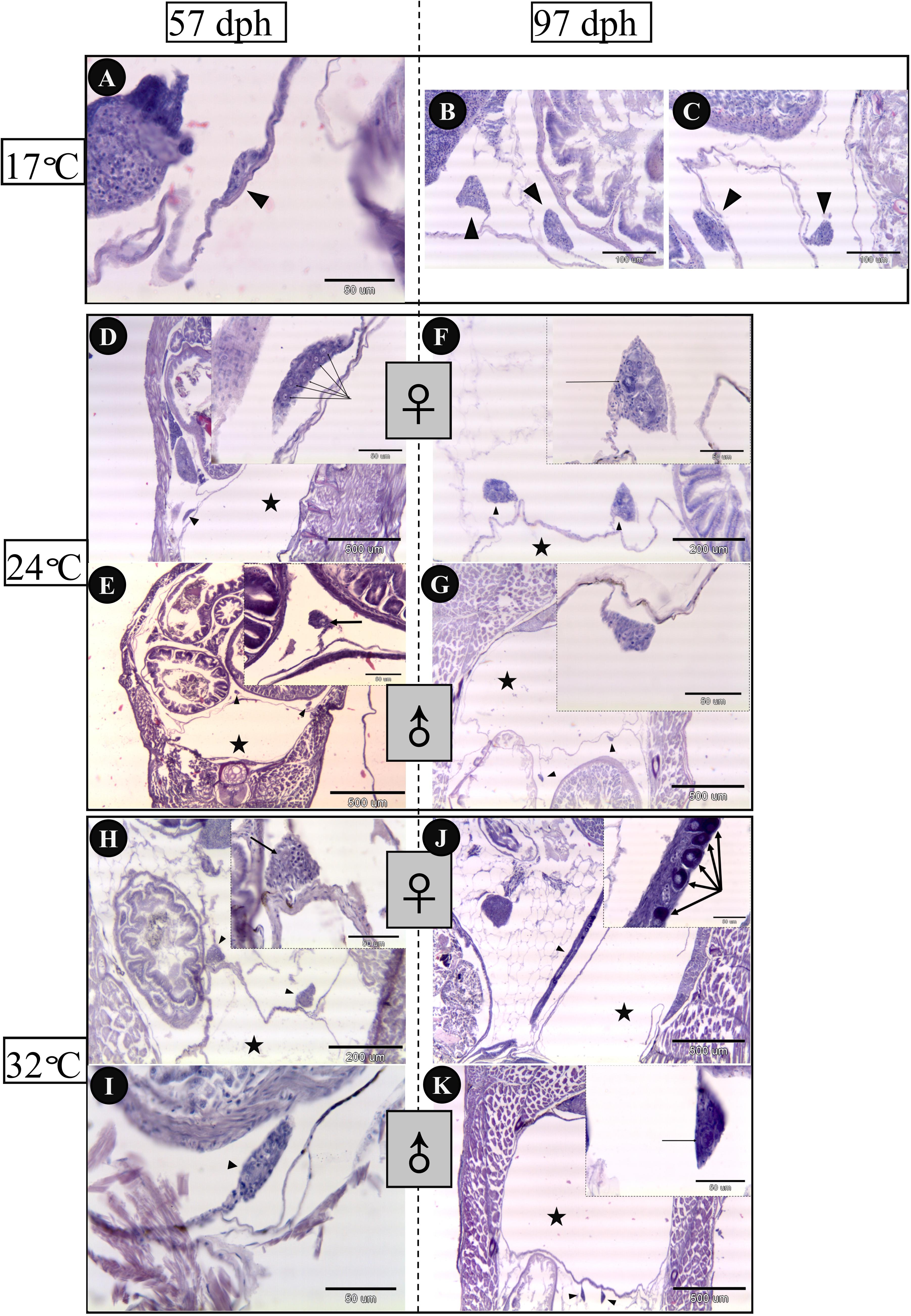
FIGURE 4. Gonadal histology of bluegill L. macrochirus in three temperature-treatment groups (17, 24, and 32°C) at 57 and 97 days posthatching (dph). Solid triangles indicate gonads. Five-pointed stars denote the place of swim bladder. 17°C group: (A) undistinguishable gonad at 57 dph. (B,C) undistinguishable gonad at 97 dph. 24°C group: (D) presumptive ovary at 57 dph, arrows indicate several germ cells. (E) presumptive testes at 57 dph, arrow indicates germ cell. (F) ovary at 97 dph, arrow points to peri-nucleolus oocyte. (G) testes at 97 dph. 32°C group: (H) presumptive ovaries at 57 dph, arrow indicates germ cell. (I) presumptive testis at 57 dph. (J) ovary at 97 dph, arrows point to peri-nucleolus oocytes. (K) testes at 97 dph, arrow indicates germ cell.
Discussion
The foxl2 and cyp19a1a Play a Role Before the Onset of Morphological Ovarian Differentiation
In this study, we showed that the expression of the two sex differentiation-related genes, foxl2 and cyp19a1a, were detected in bluegill larvae at 7 dph (Figure 2), which is much earlier than morphological gonadal differentiation (Gao et al., 2009). The foxl2 gene is a regulator of the aromatase gene (Pannetier et al., 2006; Wang et al., 2007). In tilapia, alteration of the expression of foxl2 could cause sex reversal of XX females to males, while overexpression of foxl2 leads to degeneration of the testicular structure and a stimulation of estrogen production (Wang et al., 2007). Estrogen or aromatase inhibitor treatment during the labile period of sex differentiation suggested that foxl2 is regulated through a positive feedback mechanism (Baron et al., 2004; Liu et al., 2007): foxl2–aromatase gene–aromatase–estrogen–foxl2 (Figure 1). The expression of foxl2 was detected before morphological gonadal differentiation in all investigated species except medaka, indicating its role prior to sex differentiation. Furthermore, expression of foxl2 is significantly increased just at the onset of or during sex differentiation, strongly supporting the notion that foxl2 plays an essential role in ovarian differentiation.
The cyp19a1a and estrogens are key players during the ovarian differentiation in fish (Piferrer and Blázquez, 2005; Guiguen et al., 2010; Shen and Wang, 2014). Expression of cyp19a1a has been detected prior to sex differentiation (Shen and Wang, 2014). The present work showed that cyp19a1a is expressed prior to sex differentiation, suggesting its essential role in ovarian differentiation. However, future studies are warranted to focus on the underlying mechanism connecting environmental factors (e.g., water temperature) and cyp19a1a expression.
Expression of foxl2 Was Thermo-Sensitive
The expression of foxl2 was thermo-sensitive and significant differences were found between temperature treatments at 27 dph prior to the onset of morphological sex differentiation (Figure 3). However, no significant differences have been detected in sex ratio among the three temperature treatments. The present study shows that the low temperature treatment (17°C) suppressed the growth and inhibited gonad development as well (Figures 4A–C). The progress of sex differentiation is more dependent on length than on age (Piferrer et al., 2005; Gao et al., 2009). Furthermore, the growth is related to phenotypic sex in European sea bass (Saillant et al., 2001) and assigned as growth-dependent sex differentiation (Ospina-Álvarez and Piferrer, 2008). The so-called growth-dependent sex differentiation was observed in southern brook lamprey (Ichthyomyzon gagei), American eel (Anguilla rostrata), and olive flounder (Kraak and De Looze, 1992; Ospina-Álvarez and Piferrer, 2008). This study suggests that bluegill from different geographic locations might have different genotypes and sensitivity to environmental temperatures. Therefore, sex differentiation of bluegill could be more complicated and mysterious because of growth-dependent sex differentiation or TSD.
Thermosensitivity of foxl2 has been reported in Japanese flounder (Paralichthys olivaceus) in which temperature treatment (18 or 27°C) could result in 100% sex reversal. The expression of foxl2 also varied with rearing temperatures in Pacific oyster (Santerre et al., 2013). Therefore, foxl2 is the key regulator for the sex differentiation. In species with TSD, no consistent genetic differences exist between female and male (Valenzuela et al., 2003; Conover, 2004; Ospina-Álvarez and Piferrer, 2008; Shen and Wang, 2014). Moreover, in TSD species, the ambient temperatures trigger the gonadal differentiation (Shoemaker and Crews, 2009). In species with GSD (or with GSD+TE), the initiation of sex differentiation is activated by sex-determining gene(s). Therefore, the comparative analysis of the expression profile of foxl2 and other sex differentiation-related genes will further enhance the understanding of the evolution of sex-determination mechanisms.
Thermal Stress on foxl2 Expression and Ovarian Development
Temperature has an extensive influence on an organism, including a series of physiological and biochemical processes, which results in a specific phenotype (Mccue, 2004). The water temperature influences developmental, behavioral, physiological, and morphological traits in many kinds of animals, especially in reptiles and fish (Mccue, 2004; Rhen and Lang, 2004; Booth, 2006). However, there is poor understanding of how temperature transduces molecular signals into the body and affects sex differentiation-related genes (Shen and Wang, 2014, 2018a). The present study exhibits that low temperature increased the expression of foxl2 at 27 dph, while low-temperature treatment suppressed ovarian development as revealed by histological examination (Figure 3). In Pacific oyster, the best growth performance was observed between 27 and 32°C (Rico-Villa et al., 2009). However, low temperature (18°C) suppressed gonadal differentiation and increased expression of foxl2, while high temperature promoted gonadal differentiation and decreased expression of foxl2 (Santerre et al., 2013). Bluegill has better growth performance at water temperature of 28 and 32°C (Beitinger and Magnuson, 1979). The inconsistency in the current findings can be explained by that foxl2 is only thermo-sensitive during the critical period of sex differentiation (around 27 dph). Moreover, foxl2 is not involved in TSD in bluegill because its elevated expression did not affect sex ratio in the low-temperature group. Therefore, the expression changes of foxl2 might be a result of physiological development. Temperature effects on foxl2 expression during critical period of sex differentiation or its effects on germ cells have been investigated (Lee et al., 2009; Pandit et al., 2015). However, the effects of foxl2 on both of them have not been reported yet. Further studies are required to address the expression difference of sex differentiation-related genes, e.g., foxl2, cyp19a1a, dmrt1, and amh, in species with both GSD and TSD and the roles of these genes in the transition of sex-determining mechanisms.
Thermal Stress on Primordial Germ Cells and Sex Differentiation
Mitotic proliferation of primordial germ cells and formation of the ovarian cavity are acceptable indictors of the initialization of ovarian differentiation in teleost (Nakamura et al., 1998). This study shows that the germ cells of bluegill in putative ovaries outnumbered those in putative testes (Figures 4D,E,H,I). This study shows delayed proliferation and reduced numbers of germ cells in the low-temperature treatment (17°C) and morphological sex differentiation had not been detected up to 97 dph (Figure 4). In pufferfish (Takifugu rubripes), high-temperature treatment during early gonadal development induced germ cell degeneration and masculinization of ovarian somatic cells (Lee et al., 2009). In Nile tilapia, high temperature treatment (37°C) also resulted in a permanent depletion of germ cells indicating the involvement of germ cells in temperature-induced sex reversal (Pandit et al., 2015). Furthermore, germ cell ablations have confirmed the involvement of germ cells in sex differentiation in zebrafish (Danio rerio) and medaka (Slanchev et al., 2005; Kurokawa et al., 2007; Siegfried and Nüsslein-Volhard, 2008) and no involvement in loach (Misgurnus anguillicaudatus) and goldfish (Carassius auratus) (Fujimoto et al., 2010; Goto et al., 2012). Germ-cell-deficient genotypic female in zebrafish developed as sterile males, which are able to mate with normal females and induce them to lay eggs (Slanchev et al., 2005). These findings may be of practical importance for fish population control or fisheries management through production of genetically modified male (even YY super-male) and/or disturbing the germ cells, which may cause rapid decrease in population.
Conclusion
The present work investigated the molecular and physiological response to thermal stress throughout the early development stage of sex differentiation in bluegill. Our results suggest that the two ovarian differentiation-related genes, foxl2 and cyp19a1a, play a role prior to sex differentiation of bluegill. The foxl2 may be involved in TSD in some species, but not in others. Further studies will focus on the comparative analysis of molecular network of sex differentiation.
Author Contributions
Z-GS and H-PW conceived and designed the experiments. Z-GS, NE, HY, and Z-GX performed the experiments. Z-GS analyzed the data and wrote the paper. H-PW and HY contributed reagents, materials, and analysis tools. H-PW, NE, and Z-GX revised the draft version. All authors have read and approved the manuscript.
Funding
This study was supported by the National Institute of Food and Agriculture (NIFA), U.S. Department of Agriculture (USDA, Agreement No. 2010-38879-20946), the USDA-North Central Region Aquaculture Center (2014-3850022138), and by the Fundamental Research Funds for the Central Universities (52902-0900201669) and Natural Science Foundation of Hubei Province (2018CFB215). The funders had no role in study design, data collection and analysis, decision to publish, or preparation of the manuscript.
Conflict of Interest Statement
The authors declare that the research was conducted in the absence of any commercial or financial relationships that could be construed as a potential conflict of interest.
Acknowledgments
Salaries and research support were provided by state and federal funds appropriated to The Ohio State University, Ohio Agricultural Research and Development Center. We thank Paul O’Bryant and Dean Rapp for their assistance throughout the experiment, and Joy Bauman for her comments on the manuscript. Great thanks to the reviewers for their efforts to improve the quality of this paper.
Supplementary Material
The Supplementary Material for this article can be found online at: https://www.frontiersin.org/articles/10.3389/fphys.2018.01208/full#supplementary-material
FIGURE S1 | Standard curves of foxl2 and 18S rRNA gene indicating a linear relationship between threshold cycle (CT) and gene copy number (concentration, copies μL-1). Eff %, PCR efficiency.
References
Ashida, H., Ueyama, N., Kinoshita, M., and Kobayashi, T. (2013). Molecular identification and expression of FOXL2 and DMRT1 genes from willow minnow Gnathopogon caerulescens. Reprod. Biol. 13, 317–324. doi: 10.1016/j.repbio.2013.10.002
Bachtrog, D., Mank, J. E., Peichel, C. L., Kirkpatrick, M., Otto, S. P., Ashman, T.-L., et al. (2014). Sex determination: why so many ways of doing it? PLoS Biol. 12:e1001899. doi: 10.1371/journal.pbio.1001899
Baron, D., Batista, F., Chaffaux, S., Cocquet, J., Cotinot, C., Cribiu, E., et al. (2005). Foxl2 gene and the development of the ovary: a story about goat, mouse, fish and woman. Reprod. Nutr. Dev. 45, 377–382. doi: 10.1051/rnd:2005028
Baron, D., Cocquet, J., Xia, X., Fellous, M., Guiguen, Y., and Veitia, R. A. (2004). An evolutionary and functional analysis of FoxL2 in rainbow trout gonad differentiation. J. Mol. Endocrinol. 33, 705–715. doi: 10.1677/jme.1.01566
Baron, D., Houlgatte, R., Fostier, A., and Guiguen, Y. (2008). Expression profiling of candidate genes during ovary-to-testis trans-differentiation in rainbow trout masculinized by androgens. Gen. Comp. Endocrinol. 156, 369–378. doi: 10.1016/j.ygcen.2008.01.016
Beitinger, T. L., and Magnuson, J. J. (1979). Growth rates and temperature selection of bluegill, Lepomis macrochirus. Trans. Am. Fish. Soc. 108, 378–382.
Booth, D. T. (2006). Influence of incubation temperature on hatchling phenotype in reptiles. Physiol. Biochem. Zool. PBZ 79, 274–281. doi: 10.1086/499988
Conover, D. O. (2004). “Temperature-dependent sex determination in fishes,” in Temperature-dependent Sex Determination in Vertebrates, eds V. Nicole and V. Lance (Washington DC: Smithsonian Books), 11–20.
Corpuz, P. S., Lindaman, L. L., Mellon, P. L., and Coss, D. (2010). FoxL2 Is required for activin induction of the mouse and human follicle-stimulating hormone beta-subunit genes. Mol. Endocrinol. 24, 1037–1051. doi: 10.1210/me.2009-0425
D’Cotta, H., Pepey, E., Pfennig, F., Bienvenu, D., Gutzeit, H., and Volff, J. (2007). “Sox9a, Sox9b and Amh are up-regulated in the gonads during natural and temperature-induced tilapia male differentiation,” in Proceedings of the 8th International Symposium on Reproductive Physiology of Fish, (Saint-Malo: University of Rennes).
Dominey, W. J. (1981). Maintenance of female mimicry as a reproductive strategy in bluegill sunfish (Lepomis macrochirus). Environ. Biol. Fishes 6, 59–64. doi: 10.1007/BF00001800
Eissa, N., and Wang, H.-P. (2013). Physiological stress response of yellow perch subjected to repeated handlings and salt treatments at different temperatures. North Am. J. Aquac. 75, 449–454. doi: 10.1080/15222055.2013.799622
Eissa, N., and Wang, H.-P. (2016). Transcriptional stress responses to environmental and husbandry stressors in aquaculture species. Rev. Aquac. 8, 61–88.
Eissa, N., Wang, H.-P., Yao, H., and Abou-ElGheit, E. (2018). Mixed Bacillus species enhance the innate immune response and stress tolerance in yellow perch subjected to hypoxia and air-exposure stress. Sci. Rep. 8:6891. doi: 10.1038/s41598-018-25269-z
Eissa, N., Wang, H. P., Yao, H., Shen, Z. G., Shaheen, A. A., and Abouelgheit, E. N. (2017). Expression of Hsp70, Igf1, and three oxidative stress biomarkers in response to handling and salt treatment at different water temperatures in yellow perch, Perca flavescens. Front. Physiol 8:683. doi: 10.3389/fphys.2017.00683
Fujimoto, T., Nishimura, T., Goto-Kazeto, R., Kawakami, Y., Yamaha, E., and Arai, K. (2010). Sexual dimorphism of gonadal structure and gene expression in germ cell-deficient loach, a teleost fish. Proc. Natl. Acad. Sci. U.S.A. 107, 17211–17216. doi: 10.1073/pnas.1007032107
Gao, Z., Wang, H.-P., Rapp, D., O’Bryant, P., Wallat, G., Wang, W., et al. (2009). Gonadal sex differentiation in the bluegill sunfish Lepomis macrochirus and its relation to fish size and age. Aquaculture 294, 138–146. doi: 10.1016/j.aquaculture.2009.05.024
Garner, S. R., and Neff, B. D. (2013). Alternative male reproductive tactics drive asymmetrical hybridization between sunfishes (Lepomis spp.). Biol. Lett. 9:20130658. doi: 10.1098/rsbl.2013.0658
Georges, A., Auguste, A., Bessière, L., Vanet, A., Todeschini, A.-L., and Veitia, R. A. (2013). FOXL2: a central transcription factor of the ovary. J. Mol. Endocrinol. 52, R17–R33. doi: 10.1530/JME-13-0159
Goto, R., Saito, T., Takeda, T., Fujimoto, T., Takagi, M., Arai, K., et al. (2012). Germ cells are not the primary factor for sexual fate determination in goldfish. Dev. Biol. 370, 98–109. doi: 10.1016/j.ydbio.2012.07.010
Gross, M. R., and Charnov, E. L. (1980). Alternative male life histories in bluegill sunfish. Proc. Natl. Acad. Sci. U.S.A. 77, 6937–6940. doi: 10.1073/pnas.77.11.6937
Guiguen, Y., Fostier, A., Piferrer, F., and Chang, C.-F. (2010). Ovarian aromatase and estrogens: a pivotal role for gonadal sex differentiation and sex change in fish. Gen. Comp. Endocrinol. 165, 352–366. doi: 10.1016/j.ygcen.2009.03.002
Hossain, M. S. (2010). Molecular Analyses of Gonad Differentiation and Function in Zebrafish. PhD Thesis, National University of Singapore, Singapore.
Huang, W., Zhou, L., Li, Z., and Gui, J.-F. (2009). Expression pattern, cellular localization and promoter activity analysis of ovarian aromatase (Cyp19a1a) in protogynous hermaphrodite red-spotted grouper. Mol. Cell. Endocrinol. 307, 224–236. doi: 10.1016/j.mce.2009.04.003
Ijiri, S., Kaneko, H., Kobayashi, T., Wang, D.-S., Sakai, F., Paul-Prasanth, B., et al. (2008). Sexual dimorphic expression of genes in gonads during early differentiation of a teleost fish, the Nile tilapia Oreochromis niloticus. Biol. Reprod. 78, 333–341. doi: 10.1095/biolreprod.107.064246
Janes, D. E., Elsey, R. M., Langan, E. M., Valenzuela, N., and Edwards, S. V. (2013). Sex-biased expression of sex-differentiating genes FOXL2 and FGF9 in American alligators, alligator Mississippiensis. Sex. Dev. 7, 253–260. doi: 10.1159/000350787
Kashimada, K., Pelosi, E., Chen, H., Schlessinger, D., Wilhelm, D., and Koopman, P. (2011). FOXL2 and BMP2 act cooperatively to regulate follistatin gene expression during ovarian development. Endocrinology 152, 272–280. doi: 10.1210/en.2010-0636
Kikuchi, K., and Hamaguchi, S. (2013). Novel sex-determining genes in fish and sex chromosome evolution. Dev. Dyn. 242, 339–353. doi: 10.1002/dvdy.23927
Kobayashi, Y., Nagahama, Y., and Nakamura, M. (2013). Diversity and plasticity of sex determination and differentiation in fishes. Sex. Dev. 7, 115–125. doi: 10.1159/000342009
Kraak, S. B. M., and De Looze, E. M. A. (1992). A new hypothesis on the evolution of sex determination in vertebrates; big females Zw, big males Xy. Netharlands J. Zool. 43, 260–273. doi: 10.1163/156854293X00034
Kurokawa, H., Saito, D., Nakamura, S., Katoh-Fukui, Y., Ohta, K., Baba, T., et al. (2007). Germ cells are essential for sexual dimorphism in the medaka gonad. Proc. Natl. Acad. Sci. U.S.A. 104, 16958–16963. doi: 10.1073/pnas.0609932104
Lee, K. H., Yamaguchi, A., Rashid, H., Kadomura, K., Yasumoto, S., and Matsuyama, M. (2009). Germ cell degeneration in high-temperature treated pufferfish, Takifugu rubripes. Sex. Dev. 3, 225–232. doi: 10.1159/000228723
Liu, Z., Wu, F., Jiao, B., Zhang, X., Hu, C., Huang, B., et al. (2007). Molecular cloning of doublesex and mab-3-related transcription factor 1, forkhead transcription factor gene 2, and two types of cytochrome P450 aromatase in Southern catfish and their possible roles in sex differentiation. J. Endocrinol. 194, 223–241.
Liu, Z. H., Zhang, Y. G., and Wang, D. S. (2010). Studies on feminization, sex determination, and differentiation of the Southern catfish, Silurus meridionalis—a review. Fish Physiol. Biochem. 36, 223–235. doi: 10.1007/s10695-008-9281-7
Livak, K. J., and Schmittgen, T. D. (2001). Analysis of relative gene expression data using real-time quantitative PCR and the 2(-Delta Delta C(T)) method. Methods 25, 402–408. doi: 10.1006/meth.2001.1262
Loffler, K. A., Zarkower, D., and Koopman, P. (2003). Etiology of ovarian failure in blepharophimosis ptosis epicanthus inversus syndrome: FOXL2 is a conserved, early-acting gene in vertebrate ovarian development. Endocrinology 144, 3237–3243. doi: 10.1210/en.2002-0095
Mccue, M. D. (2004). “General effects of temperature on animal biology,” in Temperature-dependent sex determination in Vertebrates, eds N. Valenzuela and V. A. Lance (Washington DC: Smithsonian Books), 71–78.
Mei, J., and Gui, J.-F. (2015). Genetic basis and biotechnological manipulation of sexual dimorphism and sex determination in fish. Sci. China Life Sci. 58, 124–136. doi: 10.1007/s11427-014-4797-9
Nakamoto, M., Matsuda, M., Wang, D.-S., Nagahama, Y., and Shibata, N. (2006). Molecular cloning and analysis of gonadal expression of Foxl2 in the medaka, Oryzias latipes. Biochem. Biophys. Res. Commun. 344, 353–361. doi: 10.1016/j.bbrc.2006.03.137
Nakamoto, M., Muramatsu, S., Yoshida, S., Matsuda, M., Nagahama, Y., and Shibata, N. (2009). Gonadal sex differentiation and expression of Sox9a2, Dmrt1, and Foxl2 in Oryzias luzonensis. Genesis 47, 289–299. doi: 10.1002/dvg.20498
Nakamura, M., Kobayashi, T., Chang, X.-T., and Nagahama, Y. (1998). Gonadal sex differentiation in teleost fish. J. Exp. Zool. 281, 362–372. doi: 10.1002/(SICI)1097-010X(19980801)281:5<362::AID-JEZ3>3.0.CO;2-M
Near, T. J., and Koppelman, J. (2009). “Species diversity, phylogeny and phylogeography of Centrarchidae,” in Centrarchid Fishes: Diversity, Biology and Conservation, eds S. Cooke and D. P. Philipp (Hoboken, NJ: Wiley-Blackwell).
Nishimura, T., Sato, T., Yamamoto, Y., Watakabe, I., Ohkawa, Y., Suyama, M., et al. (2015). foxl3 is a germ cell–intrinsic factor involved in sperm-egg fate decision in medaka. Science 349, 328–331. doi: 10.1126/science.aaa2657
Ospina-Álvarez, N., and Piferrer, F. (2008). Temperature-dependent sex determination in fish revisited: prevalence, a single sex ratio response pattern, and possible effects of climate change. PLoS One 3:e2837. doi: 10.1371/journal.pone.0002837
Pandit, N. P., Bhandari, R. K., Kobayashi, Y., and Nakamura, M. (2015). High temperature-induced sterility in the female Nile tilapia, Oreochromis niloticus. Gen. Comp. Endocrinol. 213, 110–117. doi: 10.1016/j.ygcen.2015.01.028
Pannetier, M., Fabre, S., Batista, F., Kocer, A., Renault, L., Jolivet, G., et al. (2006). FOXL2 activates P450 aromatase gene transcription: towards a better characterization of the early steps of mammalian ovarian development. J. Mol. Endocrinol. 36, 399–413.
Piferrer, F., and Blázquez, M. (2005). Aromatase distribution and regulation in fish. Fish Physiol. Biochem. 31, 215–226. doi: 10.1007/s10695-006-0027-0
Piferrer, F., Blázquez, M., Navarro, L., and González, A. (2005). Genetic, endocrine, and environmental components of sex determination and differentiation in the European sea bass (Dicentrarchus labrax L.). Gen. Comp. Endocrinol. 142, 102–110. doi: 10.1016/j.ygcen.2005.02.011
Poonlaphdecha, S., Pepey, E., Canonne, M., de Verdal, H., Baroiller, J.-F., and D’Cotta, H. (2013). Temperature induced-masculinisation in the Nile tilapia causes rapid up-regulation of both dmrt1 and amh expressions. Gen. Comp. Endocrinol. 193, 234–242. doi: 10.1016/j.ygcen.2013.06.007
Rhen, T., and Lang, J. W. (2004). “Phenotypic Effects of Incubation Temperature in Reptiles,” in Temperature-dependent sex determination in Vertebrates, eds N. Valenzuela and V. A. Lance (Washington DC: Smithsonian Books), 90–98.
Rico-Villa, B., Pouvreau, S., and Robert, R. (2009). Influence of food density and temperature on ingestion, growth and settlement of Pacific oyster larvae, Crassostrea gigas. Aquaculture 287, 395–401. doi: 10.1016/j.aquaculture.2008.10.054
Saillant, E., Fostier, A., Menu, B., Haffray, P., and Chatain, B. (2001). Sexual growth dimorphism in sea bass Dicentrarchus labrax. Aquaculture 202, 371–387. doi: 10.1016/S0044-8486(01)00786-4
Santerre, C., Sourdaine, P., Marc, N., Mingant, C., Robert, R., and Martinez, A.-S. (2013). Oyster sex determination is influenced by temperature — First clues in spat during first gonadic differentiation and gametogenesis. Comp. Biochem. Physiol. A Mol. Integr. Physiol. 165, 61–69. doi: 10.1016/j.cbpa.2013.02.007
Schmidt, D., Ovitt, C. E., Anlag, K., Fehsenfeld, S., Gredsted, L., Treier, A.-C., et al. (2004). The murine winged-helix transcription factor Foxl2 is required for granulosa cell differentiation and ovary maintenance. Development 131, 933–942. doi: 10.1242/dev.00969
Schmittgen, T. D., and Livak, K. J. (2008). Analyzing real-time PCR data by the comparative CT method. Nat. Protoc. 3, 1101–1108. doi: 10.1038/nprot.2008.73
Shen, Z.-G., and Wang, H.-P. (2018a). “Environmental sex determination and sex differentiation in teleost–how sex is established,” in Sex Control in Aquaculture: Theory and Practice, eds H. P. Wang, F. Piferrer, and S. L. Chen (Hoboken, NJ: Wiley-Blackwell).
Shen, Z., and Wang, H.-P. (2018b). “Hybridization and its application in Centrarchids (Perciformes),” in Sex Control in Aquaculture: Theory and Practice, eds H. P. Wang, F. Piferrer, and S. L. Chen (Hoboken, NJ: Wiley-Blackwell).
Shen, Z.-G., and Wang, H.-P. (2014). Molecular players involved in temperature-dependent sex determination and sex differentiation in Teleost fish. Genet. Sel. Evol. 46:26. doi: 10.1186/1297-9686-46-26
Shen, Z.-G., Wang, H.-P., Yao, H., O’Bryant, P., Rapp, D., and Zhu, K.-Q. (2016). Sex determination in bluegill Sunfish Lepomis macrochirus: effect of temperature on sex ratio of four geographic strains. Biol. Bull. 230, 197–208. doi: 10.1086/BBLv230n3p197
Shoemaker, C. M., and Crews, D. (2009). Analyzing the coordinated gene network underlying temperature-dependent sex determination in reptiles. Semin. Cell Dev. Biol. 20, 293–303. doi: 10.1016/j.semcdb.2008.10.010
Shoemaker, C. M., Queen, J., and Crews, D. (2007). Response of candidate sex-determining genes to changes in temperature reveals their involvement in the molecular network underlying temperature-dependent sex determination. Mol. Endocrinol. 21, 2750–2763. doi: 10.1210/me.2007-0263
Shoemaker-Daly, C. M., Jackson, K., Yatsu, R., Matsumoto, Y., and Crews, D. (2010). Genetic network underlying temperature-dependent sex determination is endogenously regulated by temperature in isolated cultured Trachemys scripta Gonads. Dev. Dyn. 239, 1061–1075. doi: 10.1002/dvdy.22266
Siegfried, K. R. (2010). In search of determinants: gene expression during gonadal sex differentiation. J. Fish Biol. 76, 1879–1902. doi: 10.1111/j.1095-8649.2010.02594.x
Siegfried, K. R., and Nüsslein-Volhard, C. (2008). Germ line control of female sex determination in zebrafish. Dev. Biol. 324, 277–287. doi: 10.1016/j.ydbio.2008.09.025
Slanchev, K., Stebler, J., de la Cueva-Méndez, G., and Raz, E. (2005). Development without germ cells: the role of the germ line in zebrafish sex differentiation. Proc. Natl. Acad. Sci. U.S.A 102, 4074–4079. doi: 10.1073/pnas.0407475102
Sridevi, P., and Senthilkumaran, B. (2011). Cloning and differential expression of FOXL2 during ovarian development and recrudescence of the catfish, Clarias gariepinus. Gen. Comp. Endocrinol. 174, 259–268. doi: 10.1016/j.ygcen.2011.08.015
Uhlenhaut, N. H., and Treier, M. (2006). Foxl2 function in ovarian development. Mol. Genet. Metab. 88, 225–234. doi: 10.1016/j.ymgme.2006.03.005
Valenzuela, N., Adams, D. C., and Janzen, F. J. (2003). Pattern does not equal process: exactly when is sex environmentally determined? Am. Nat. 161, 676–683. doi: 10.1086/368292
Vizziano, D., Randuineau, G., Baron, D., Cauty, C., and Guiguen, Y. (2007). Characterization of early molecular sex differentiation in rainbow trout, Oncorhynchus mykiss. Dev. Dyn. 236, 2198–2206. doi: 10.1002/dvdy.21212
Wang, D.-S., Kobayashi, T., Zhou, L.-Y., Paul-Prasanth, B., Ijiri, S., Sakai, F., et al. (2007). Foxl2 up-regulates aromatase gene transcription in a female-specific manner by binding to the promoter as well as interacting with ad4 binding protein/steroidogenic factor 1. Mol. Endocrinol. 21, 712–725. doi: 10.1210/me.2006-0248
Wang, D.-S., Zhou, L.-Y., Kobayashi, T., Matsuda, M., Shibata, Y., Sakai, F., et al. (2010). Doublesex- and Mab-3-related transcription factor-1 repression of aromatase transcription, a possible mechanism favoring the male pathway in tilapia. Endocrinology 151, 1331–1340. doi: 10.1210/en.2009-0999
Wang, H.-P., Gao, Z.-X., Rapp, D., O’Bryant, P., Yao, H., and Cao, X.-J. (2014). Effects of temperature and genotype on sex determination and sexual size dimorphism of bluegill sunfish Lepomis macrochirus. Aquaculture420–421(Suppl. 1), S64–S71. doi: 10.1016/j.aquaculture.2013.09.010
Wang, H.-P., and Shen, Z. (2018). “Sex Control in Aquaculture: New Theory to Novel Practice,” in Sex Control in Aquaculture: Theory and Practice, eds H. P. Wang, F. Piferrer, and S. L. Chen (Hoboken, NJ: Wiley-Blackwell).
Yamaguchi, T., Yamaguchi, S., Hirai, T., and Kitano, T. (2007). Follicle-stimulating hormone signaling and Foxl2 are involved in transcriptional regulation of aromatase gene during gonadal sex differentiation in Japanese flounder, Paralichthys olivaceus. Biochem. Biophys. Res. Commun. 359, 935–940. doi: 10.1016/j.bbrc.2007.05.208
Keywords: foxl2, cyp19a, germ cells, stress, sex differentiation, TSD, GSD
Citation: Shen Z-G, Eissa N, Yao H, Xie Z-G and Wang H-P (2018) Effects of Temperature on the Expression of Two Ovarian Differentiation-Related Genes foxl2 and cyp19a1a. Front. Physiol. 9:1208. doi: 10.3389/fphys.2018.01208
Received: 18 October 2017; Accepted: 13 August 2018;
Published: 25 September 2018.
Edited by:
Menghong Hu, Shanghai Ocean University, ChinaReviewed by:
Tulin Arslan, Muğla University, TurkeyHossam A. Abdelhamed, Mississippi State University, United States
Copyright © 2018 Shen, Eissa, Yao, Xie and Wang. This is an open-access article distributed under the terms of the Creative Commons Attribution License (CC BY). The use, distribution or reproduction in other forums is permitted, provided the original author(s) and the copyright owner(s) are credited and that the original publication in this journal is cited, in accordance with accepted academic practice. No use, distribution or reproduction is permitted which does not comply with these terms.
*Correspondence: Han-Ping Wang, d2FuZy45MDBAb3N1LmVkdQ==
†Present address: Zhi-Gang Shen, College of Fisheries, Huazhong Agricultural University, Wuhan, China