- 1School of Life Science, Jiangxi Science and Technology Normal University, Nanchang, China
- 2Experimental Animal Science and Technology Center, Jiangxi University of Traditional Chinese Medicine, Nanchang, China
- 3Model Animal Research Center, Nanjing University, Nanjing, China
The exocyst complex plays multiple roles via tethering secretory or recycling vesicles to the plasma membrane. Previous studies have demonstrated that the exocyst contains eight components, which possibly have some redundant but distinct functions. It is therefore interesting to investigate the biological function of each component. Here, we found that Sec3, one component of exocyst complex, is involved in Drosophila egg chamber development. Loss of sec3 results in egg chamber fusion through the abolishment of cell differentiation. In addition, loss of sec3 increases cell numbers but decreases cell size. These defects phenocopy Notch pathway inactivation. In line with this, loss of sec3 indeed leads to Notch protein accumulation, suggesting that the loss of Sec3 inhibits the delivery of Notch onto the plasma membrane and accumulates inactive Notch in the cytoplasm. Loss of sec3 also leads to the ectopic expression of two Notch pathway target genes, Cut and FasciclinIII, which should normally be downregulated by Notch. Altogether, our study revealed that Sec3 governs egg chamber development through the regulation of Notch, and provides fresh insights into the regulation of oogenesis.
Introduction
Notch signaling is an evolutionarily conserved pathway that controls various processes, including embryogenesis and cell differentiation (Lai, 2004; Kopan and Ilagan, 2009). Vesicle-mediated protein traffic is essential for the transduction of the Notch pathway. The newly synthesized Notch receptor and DSL (Delta/Serrate/LAG-2) ligands are transported through the endoplasmic reticulum (ER) and Golgi apparatus to reach the plasma membrane. After association with ligands, the receptors re-enter the cell via endocytosis. These endocytic vesicles from the cell membrane then fuse with early endosome. The early endosome works as a sorting center, from which the Notch can be recycled back to the plasma membrane, or to the late endosome for protein degradation (Sorkin and von Zastrow, 2009).
Increasing studies on Drosophila, have demonstrated that the Notch pathway is involved in multiple processes, including ovary development. The Drosophila ovary consists of about 16–20 ovarioles, each of which contains a series of egg chambers processed through 14 stages. Each egg chamber consists of 16 germ cells enveloped by a monolayer of follicular epithelium. The neighboring egg chamber is linked by the stock, which is a string of five to eight follicle cells. A pair of special follicle cells called polar cells differentiate during early stages at each pole of each egg chamber. Loss of polar cells induces fusion of neighboring egg chambers and results in a compound egg chamber containing two or more germline clusters. Reduction of Notch activity suppresses polar cell formation and results in fused egg chambers (Grammont and Irvine, 2001; Torres et al., 2003; Vachias et al., 2010). Moreover, Drosophila oogenesis is a complex but coordinated process. For example, follicle cells switch from the mitotic cycle to endocycle during mid stages. Notch signaling induces this cell-cycle switch (Sun and Deng, 2005).
Exocyst is an eight protein complex and was originally identified from mutants involved in the secretory pathway in yeast (Novick et al., 1980). Subsequent analysis of the exocyst has shown that it functions in intracellular vesicle transport and mediates the tethering of secretory or recycling vesicles to the plasma membrane. Defects in exocyst proteins result in the accumulation of secretory vesicles in cells (Novick et al., 1980; Guo et al., 1999; Zhang et al., 2005). The exocyst components, Sec3, Sec5, Sec6, Sec8, Sec10, Sec15, Exo70, and Exo84, are conserved from yeast to mammals. In yeast, the Sec3 component functions as a spatial landmark for polarized secretion, as it localizes to the exocytic site independently of other components (Finger et al., 1998; Boyd et al., 2004). The yeast Sec3 directly binds to phosphatidylinositol 4,5-bisphosphate (PIP2) and the small GTPases Rho1 and Cdc42 through its N-terminal domain, and these bindings are critical for the localization and function of Sec3 at the exocytic site in the plasma membrane (Zhang et al., 2008; Yamashita et al., 2010). The yeast Sec3 was also found to interact with the t-SNARE protein Sso2, which promotes membrane fusion between the vesicles and target membrane (Yue et al., 2017). In plants, Sec3 has also been widely investigated. Sec3 is required for root hair elongation, embryogenesis, and pollen germination (Wen et al., 2005; Zhang et al., 2013; Bloch et al., 2016). In Drosophila, Sec3 is involved in the polarized transport of guidance receptors during border cell migration (Wan et al., 2013). However, the function of Sec3 in animals has not been well elucidated.
In this study, we show that the loss of sec3 phenocopies loss-of-function Notch during Drosophila oogenesis. Further studies show that loss of sec3 indeed results in a Notch protein transport defect and a Notch pathway inactivation, indicating that Sec3 regulates Notch signaling during oogenesis. Altogether, our findings shed light on exocyst-mediated regulation of oogenesis through Notch.
Materials and Methods
Fly Stocks and Husbandry
All fly stocks except for RNAi experiments were cultured at 25 ± 1°C on a standard corn-yeast-sucrose medium under constant humidity and a 12:12 h light to dark cycle. The sec3GT and sec3PBac lines were described previously (Wan et al., 2013). The line hs-flp; ubi-GFP FRT80B was obtained from the Bloomington Drosophila Stock Center (BDSC). Females of the latter line were crossed to males of sec3GT and sec3PBac lines, respectively. Progeny were heat shocked for 2 h per day at 37°C for 2 days before eclosion and 1 day after eclosion, then dissected 2–3 days after the last heat shock. Progeny with genotypes of hs-flp; sec3GT FRT80B/ubi-GFP FRT80B or hs-flp; sec3PBac FRT80B/ubi-GFP FRT80B were analyzed. The sec3 mutant clones were marked by the absence of GFP. The sec3 RNAi line was obtained from the Vienna Drosophila RNAi Center (#108085) and c306-Gal4 from BDSC. The c306-Gal4>sec3-RNAi progeny were shifted to 29°C for 3–4 days before dissection.
Immunohistochemistry and Microscopy
Ovary dissection was carried out in phosphate buffered saline (PBS) and then fixed in PBS with 7% formaldehyde for 10 min. After being washed in PBS, ovaries were blocked in 10% goat serum in PBT (PBS containing 0.3% Triton X-100) for 30 min and then stained overnight at 4°C. Most primary antibodies were obtained from the Developmental Studies Hybridoma Bank (DSHB) and their dilutions were as follows: mouse anti-Orb (orb 4H8, 1:30), mouse anti-NICD (C17.9C6, 1:10), mouse anti-NECD (C458.2H, 1:100), mouse anti-FASIII (7G10, 1:100), mouse anti-Cut (2B10, 1:100), mouse anti-Delta (C594.9B, 1:100), mouse anti-Kel (Kel 1B, 1:5), mouse anti-β-Gal (40-1a, 1:25), mouse anti-CycA (A12, 1:15), rabbit anti-phosho-Histone H3 (1:1000, Cell Signaling #3377), and rabbit anti-Stau (1:1000, gift from St. Johnston D). After being washed in PBT, ovaries were incubated with secondary antibodies (Jackson ImmunoResearch) for 2 h at room temperature. Nuclei were labeled by DAPI (E607303, 1:50, Sangon Biotech) in the last half hour. Confocal images were performed on a Leica TCS SP8 confocal microscope.
Fertility Test
To check the fertility status of the c306>sec3.RNAi females, pair matings were set between individual c306>sec3.RNAi females and wild type males in a series of vials. All vials were raised in 29°C and changed to new vials every day, to retain a good oogenesis status. Egg laying/females/day of the fourth day were counted. The ratio of hatched vs. all laid eggs were then calculated after 24 h. Progeny of late stages were also observed to check the calculation. For the experimental control, wild type females were crossed with wild type males.
Quantification of Cell Number and Cell Size
Regions of mutant clones were chosen, while regions of their sister clones were chosen. Cell numbers and area were measured in ImageJ software (NIH) for each region. The mean number of cells was calculated as (nucleus number)/(clone area). The mean size of the nuclei was calculated as (nucleus area)/(nucleus number). The mean ratio is an average of ratios of each pair bar. Statistical analysis for the data set was done using paired Student’s t-test, using Prism 5 (GraphPad software, San Diego, CA, United States). Data comparisons were considered statistically significant if p < 0.05.
Results
Loss of sec3 Results in Egg Chamber Fusion
sec3GT and sec3PBac are two loss-of-function alleles of Drosophila sec3 which were identified previously (Wan et al., 2013). sec3 mutant follicle cell clones were generated with the Flp/FRT method and egg chambers were stained for the oocyte marker Orb. In the wild type, each egg chamber had only one Orb-positive oocyte and 15 nurse cells (Figures 1A–B’). In contrast, 12% (sec3GT) or 14% (sec3PBac) of the mutant mosaic egg chambers showed compound egg chambers, which had more than the normal complement of 15 nurse cells and an oocyte (n = 50) (Figures 1C–C” and Supplementary Figure S1). To confirm this, immunostaining of Kelch (Kel), a marker of ring canals, and Staufen (Stau), another marker of oocyte, was performed to count the number of germline cells in each egg chamber (Figures 1D,E). Ring canals are cytoplasmic bridges that intracellular materials can pass from nurse cells to oocyte. Every germline cell has one ring canal on average. Quantification results indicated that the germline cell number of these compound egg chambers was two copies of wild type (29.5 ± 0.2 vs. 14.8 ± 0.1, n = 12, p < 0.0001) (Figure 1F). Therefore, we hypothesized that loss of sec3 led to egg chamber fusion. Some “partially” fused egg chambers undergoing the process of fusion could be observed, in which the intervening follicle cells seemed to be in the process of breaking apart (Supplementary Figures S2A–A”). The earliest “partially” fused egg chamber was observed at stage 3, when stock cells formed (Supplementary Figures S2B–B”). Most fused egg chambers were completely fused before stage 8, without an intervening wall of follicle cells between the two cysts.
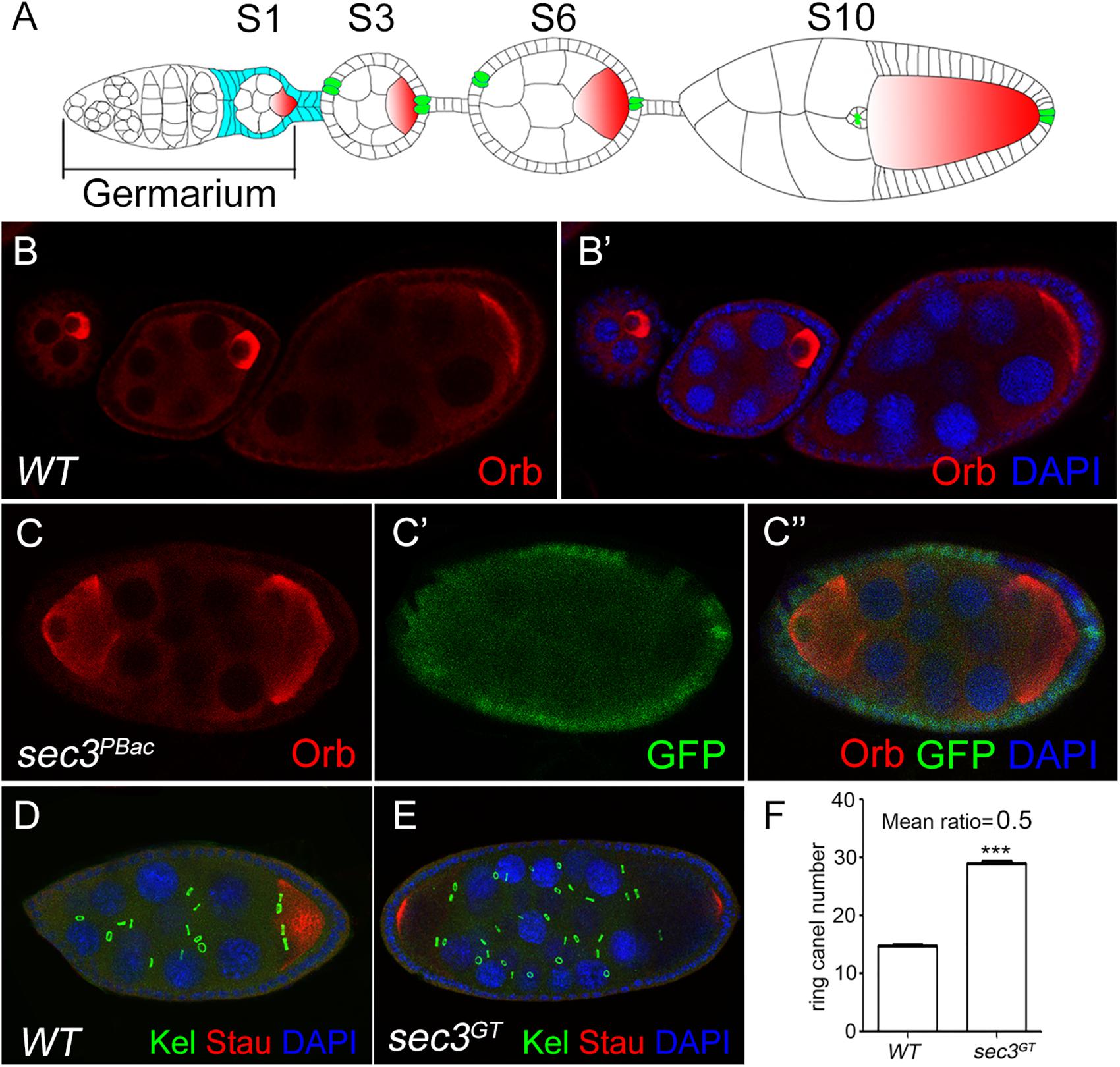
Figure 1. Loss of sec3 in follicle cells results in egg chamber fusion. (A) A model for Drosophila oogenesis showing egg chambers in different stages. Oocytes are in red and polar cells are in green from stage 3. Follicle cells cover all the germline cysts at the early stages and mainly cover the oocyte by stage 10. (B–B’) A wild type ovariole with three egg chambers, each of which has an oocyte. (C–C”) A compound egg chamber with sec3PBacclones showing two oocytes. (D) A wild type egg chamber showing 15 ring canals. (E) A compound egg chamber with two oocytes showing 30 ring canals. (F) Quantification of ring canal numbers in compound egg chambers and control egg chambers (29.5 ± 0.2 vs. 14.8 ± 0.1, n = 12, p < 0.0001). Images for Kel staining are overlays of the Z-section, as ring canals cannot be shown in a plane. In all the images in this report, the sec3 mutant clones are marked by an absence of GFP, and nuclei are stained by DAPI.
Knocking Down of sec3 Suppresses Polar Cell Differentiation
RNA interference was used to knock down sec3 expression with the UAS/Gal4 method, using c306-Gal4 which was expressed in most follicle cells. In sec3 knocked down egg chambers, fused egg chambers were observed (Figures 2A–A”). Quantification results indicated that the germline cell number of the sec3 knocked down compound egg chambers was two copies of the wild type (28.0 ± 0.7 vs. 14.8 ± 0.2, n = 8, p < 0.0001) (Figure 2B). The knock down experiment confirmed that reduced Sec3 activity induced egg chamber fusion. Signals from the polar cells are essential for stalk formation, and egg chamber fusion can be induced by suppressing polar cell differentiation (Baksa et al., 2002; McGregor et al., 2002). Polar cells in sec3 knockdown egg chambers were examined, using an enhancer trap line A101 (neutralized-lacZ), in which polar cells were marked with lacZ (Lopez-Schier and St Johnston, 2001; Figures 2C–C”). In sec3 knocked down egg chambers, expression of A101-lacZ was reduced in fused egg chambers (Figures 2D–D” and Supplementary Figure S3). Therefore, reduced sec3 activity resulted in the attenuation of polar cells, which contributed to the fusion of adjacent egg chambers.
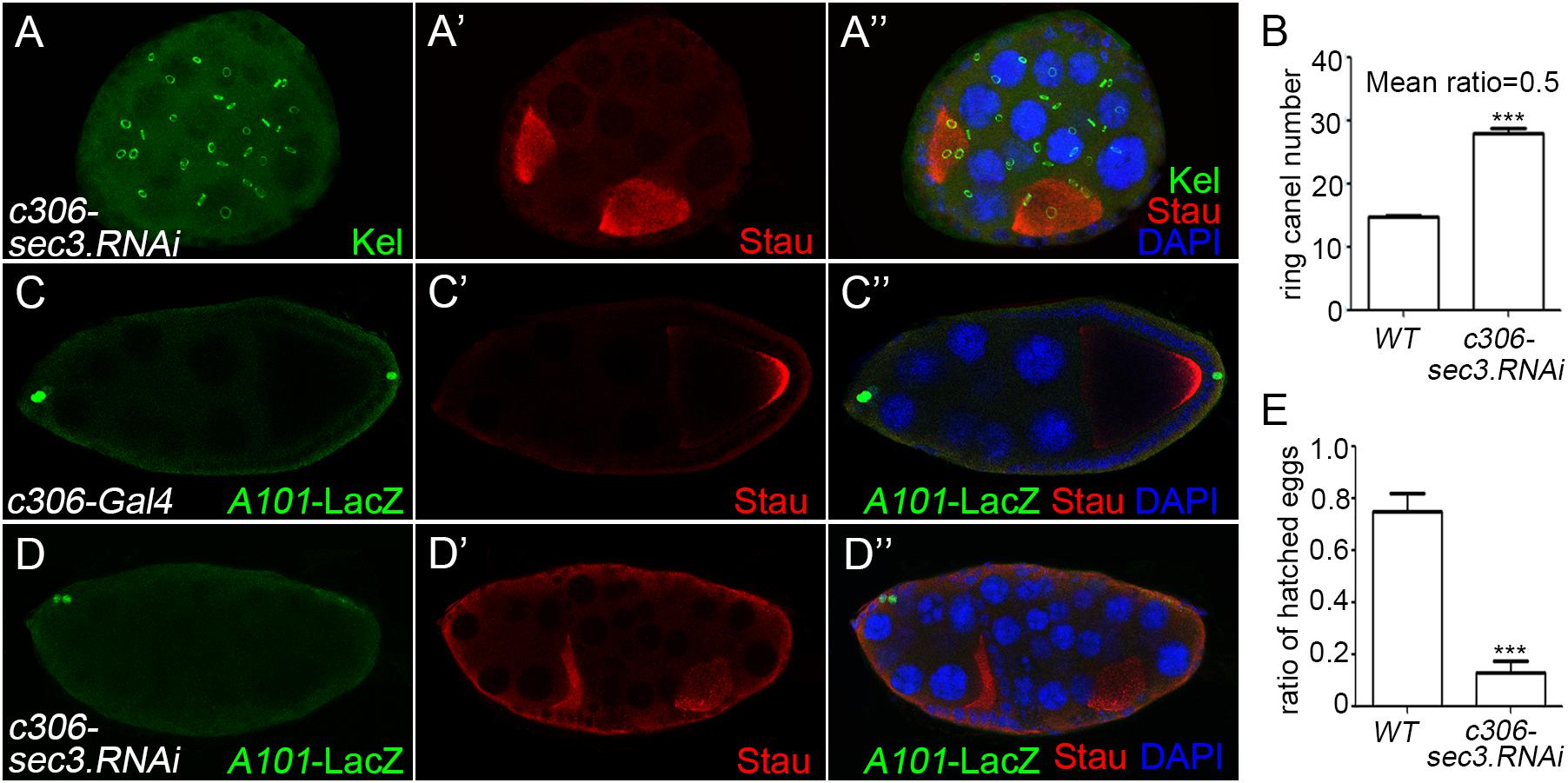
Figure 2. Knocking down of sec3 induces a fused egg chamber and suppresses polar cell differentiation. (A–A”) In c306>sec3-RNAi background, a compound egg chamber with two oocytes shows 29 ring canals. Images for Kel staining are overlays of the Z-section, as ring canals cannot be shown in a plane. (B) Quantification of ring canal numbers in sec3 knockdown compound egg chambers and control egg chambers (28.0 ± 0.7 vs. 14.8 ± 0.2, n = 8, p < 0.0001). (C–C”) In c306-Gal4 background, A101-LacZ shows normal expression at the two poles of egg chamber. (D–D”) In c306>sec3-RNAi background, a partial fused egg chamber, which has no stock cells with only a single layer of follicle cells between the two cysts, contains only a pair of cells expressing A101-LacZ at only one pole of the egg chamber. Images for A101-LacZ staining are overlays of the Z-section, showing all the staining. (E) Fertility test of c306>sec3-RNAi females; see Methods for details. c306>sec3-RNAi females show a reduced ratio of hatched vs. all laid eggs (0.75 ± 0.07 vs. 0.13 ± 0.04, n = 14, p < 0.0001).
A fertility test was carried out to test whether the dysfunction of the ovary was caused by the encapsulated multiple egg chambers. This was demonstrated by setting up pair matings between individual c306>sec3.RNAi females and wild type males. After being raised in 29°C for 4 days, egg laying/females/day and the ratio of hatched vs. all laid eggs were quantified. Most of the laid eggs from these pair matings remained unhatched and in some of the vials one to two eggs had hatched. Therefore, reduced sec3 activity resulted in reduced fertility (Figure 2E).
Loss of sec3 Shows Dysregulated Cell Cycle
During Drosophila oogenesis, follicle cells cease mitosis and duplicate chromosomes without cell division to generate polyploidy (endocycle) after stage 6 (Sun and Deng, 2005). The mitotic cycle/endocycle switch was then detected in sec3 mosaic egg chambers. Follicle cells in stage 10 egg chambers were examined. Follicle cells in sec3 mutant clones had obvious smaller nuclei, and were more densely distributed, compared with cells in the neighboring sister clone (Figures 3A–A”). The quantification results of mean cell number supported that mutant clones had more cell numbers than their sister clones (mutant/wt = 1.4, n = 6, p = 10-2) (Figure 3B). It suggested that sec3 mutant cells failed to cease mitosis and processed extra rounds of cell division. The quantification results of mean nuclei size confirmed that mutant clones had smaller nuclei than their sister clones (mutant/wt = 0.65, n = 6, p = 3 × 10-2) (Figure 3C), which suggested that sec3 mutant cells did not reach the endocycle.
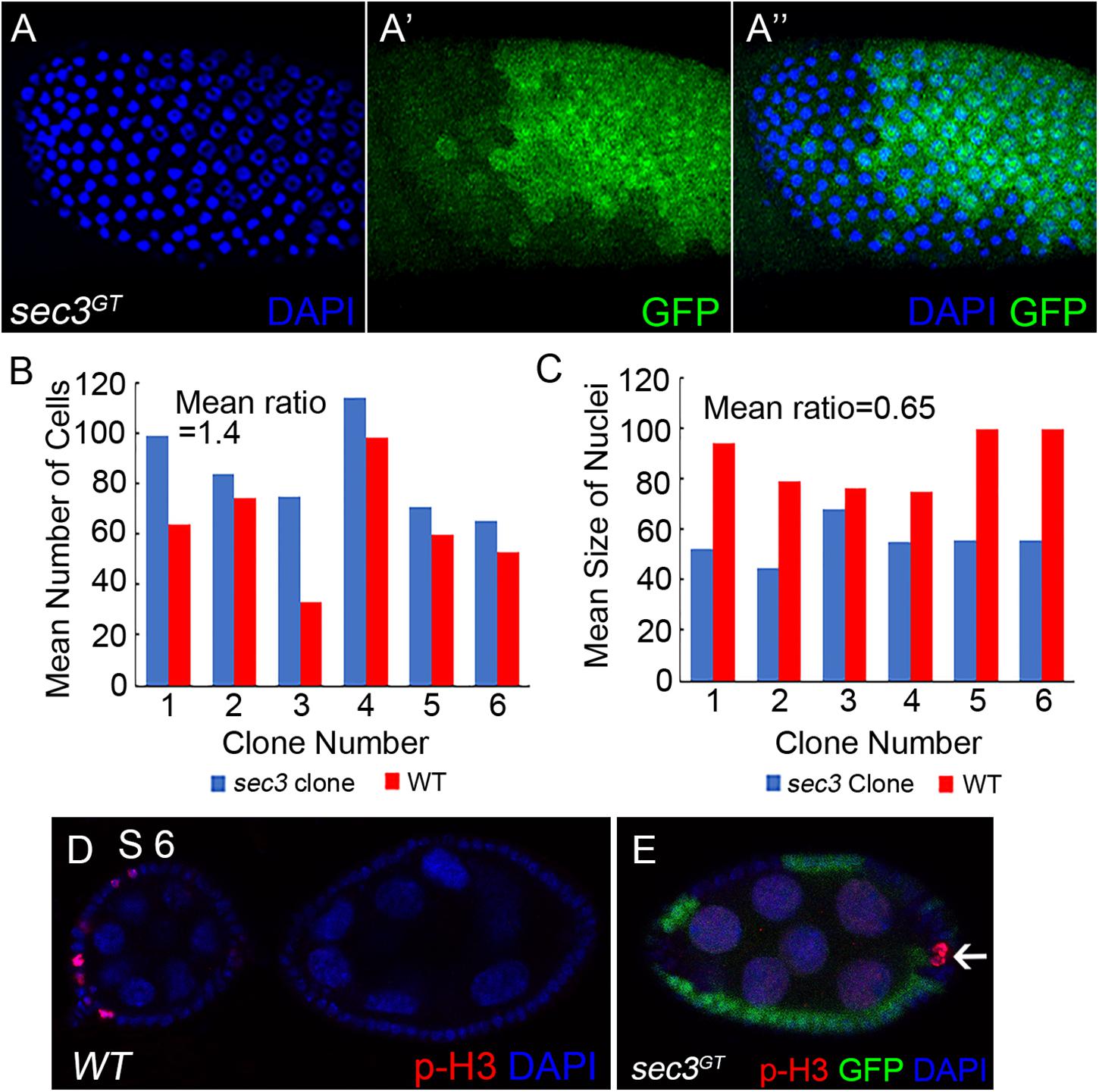
Figure 3. Loss of sec3 disrupts the mitotic cycle/endocycle switch. (A–A”) In a stage 10 egg chamber, sec3 mutant follicle clones show more cell numbers and smaller nuclei. Images are overlays of Z-section, as nuclei cannot be shown in a plane. (B) Number of nuclei in sec3 mutant clones (blue bars) compared with that in their associated sister clones (red bars) in stage 10 egg chambers. The x-axis represents the clone number, and the y-axis represents the number of cells per clone or corresponding sister clone. Mean ratio of mutant/wt = 1.4, n = 6, p = 10-2. See Methods for details. (C) Quantification of mean nucleus size in sec3 mutant clones and their sister clones. Mean ratio of mutant/wt = 0.65, n = 6, p = 3 × 10-2. See Methods for details. (D) In wild type egg chambers, p-H3 positive follicle cells are detected only up to stage 6. Note that while mitosis of follicle cells is not synchronized, p-H3 are detected only in some cells. (E) In sec3 mutant follicle cells, p-H3 staining can be detected after stage 6.
Immunostaining results of the mitotic marker phospho-histone H3 (p-H3) also demonstrated that sec3 mutant follicle cells continued to divide after stage 6. In wild type egg chambers, p-H3 positive follicle cells were detected only up to stage 6 during their mitotic cycle (Figure 3D). Note that while mitosis of follicle cells was not synchronized, p-H3 was detected only in some cells. In sec3 mutant follicle cells p-H3 staining was observed after stage 6 (Figure 3E and Supplementary Figure S4).
Notch Protein Traffic Is Destroyed in sec3 Mutant Follicle Cells
Both the fused eggs chamber and dysregulated cell proliferation phenocopied that of the Notch mutant clone (Deng et al., 2001; Grammont and Irvine, 2001; Vachias et al., 2010). There was therefore a need to examine whether Sec3 was involved in the traffic of Notch protein, as expected for a functional component of the exocyst complex. Without the exocyst, vesicles could not tether to the cell membrane which resulted in accumulation of vesicles and their cargo protein in the cytoplasm. The Notch receptor is a heterodimer composed of a Notch Intracellular Domain (NICD) and a Notch Extracellular Domain (NECD). In mosaic egg chambers, accumulation of NICD and NECD were specifically shown in the sec3 mutant follicle cells, compared with the neighboring wild type follicle cells (Figure 4). Therefore, loss of Sec3 function might inhibit the delivery of Notch onto the plasma membrane, causing an accumulation of Notch protein in the cytoplasm, suggesting that Sec3 is essential for the delivery of Notch onto he plasma membrane.
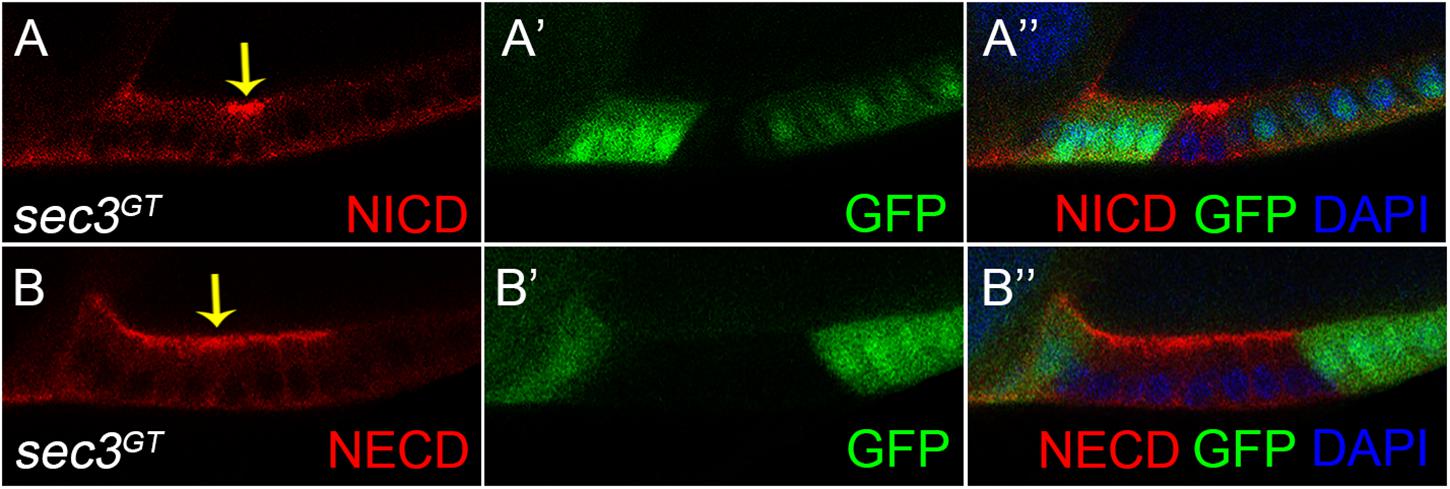
Figure 4. Accumulated Notch protein in sec3 mutant follicle cells. (A–A”) NICD obviously accumulates in sec3 mutant follicle cells in a stage 10 egg chamber (indicated by arrow). (B–B”) NECD obviously accumulates in sec3 mutant follicle cells in a stage 10 egg chamber (indicated by arrow).
Notch Signaling Target Genes Are Falsely Expressed in sec3 Mutant Follicle Cells
Cut, a DNA binding protein containing homeodomain, links Notch signaling and cell-cycle regulators. Cut is expressed in follicle cells during the early stages and is downregulated by the Notch signaling during the mid stages, which results in the cessation of mitosis and entry into the endocycle (Sun and Deng, 2005). We found that, in contrast to the wild type follicle cells, sec3 mutant follicle cells showed continued expression of Cut beyond stage 6 (Figures 5A–A”). Consistent with Cut expression, CycA, the mitotic cyclin, continued to be expressed in mutant clones after stage 6, whereas wild type follicle cells demonstrated no CycA expression during these stages. Note that while CycA was detected in 50% of follicle cells in wild type egg chambers during stages 4–6, CycA was not uniformly expressed in mutant clones (Figures 5B–B”; Shcherbata et al., 2004).
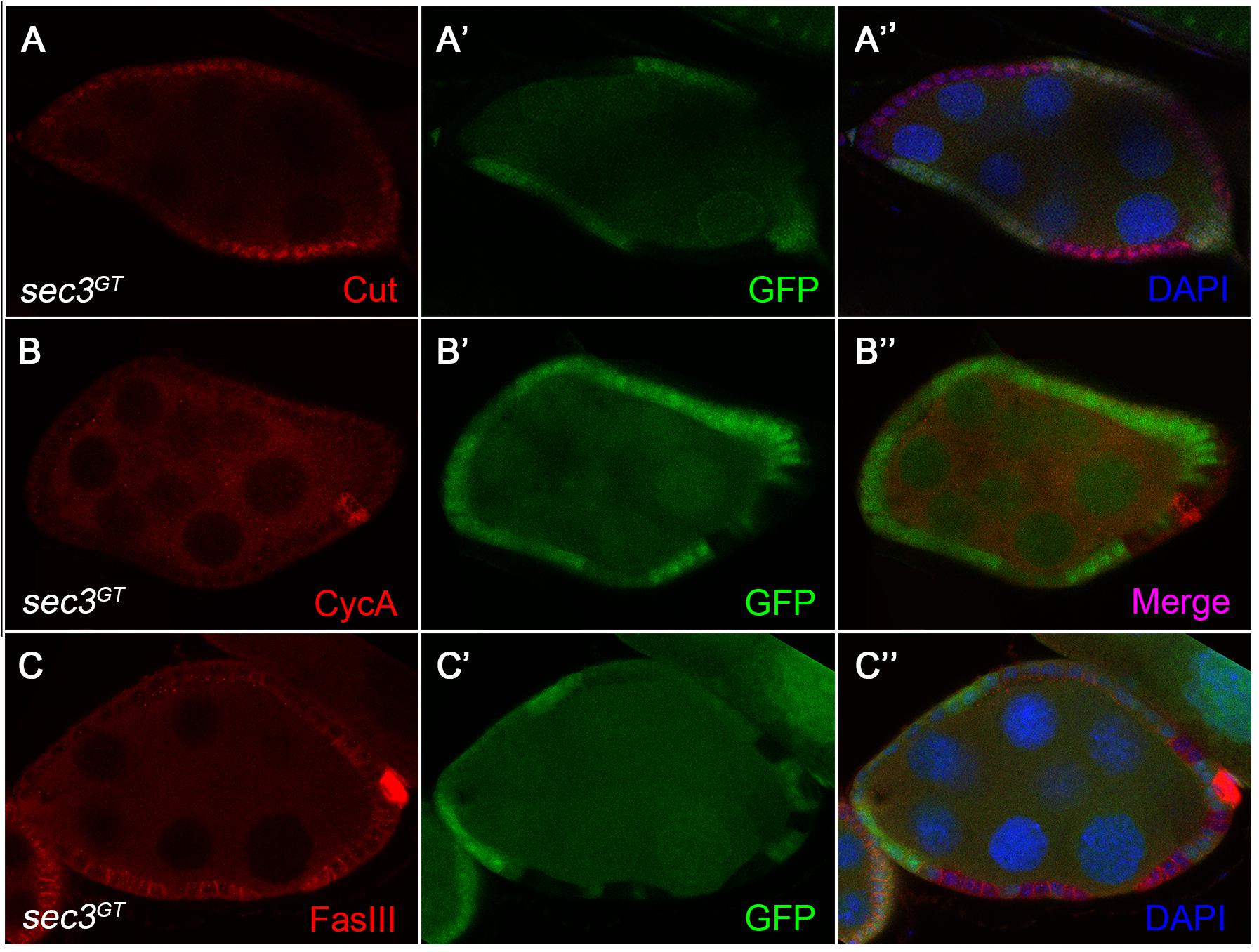
Figure 5. Altered expression of Notch signaling target genes in sec3 mutant follicle cells. (A–A”) sec3 mutant clones show continued expression of Cut, in contrast to the wild type follicle cells. (B–B”) sec3 mutant clones show continued expression of CycA, in contrast to the wild type follicle cells. Note that while CycA was detected in 50% of follicle cells in the wild type egg chambers during stages 4–6, CycA was not uniformly expressed in mutant clones. (C–C”) sec3 mutant clones show continued expression of FasIII, in contrast to the wild type follicle cells.
Notch signaling also regulates the expressions of immature cell-fate markers in follicle cells. For example, in wild type egg chambers, FasciclinIII (FasIII) is expressed in all follicle cells during the early stages and then only expressed in two pairs of polar follicle cells at each end of the egg chamber by stage 4. This downregulation of FasIII marks the differentiation of the follicle cell. Reduced Notch activity catches follicle cells in an undifferentiated state and induces ectopic expression of FasIII at the late stages (Lopez-Schier and St Johnston, 2001). We observed strong expression of FasIII in cells mutant for sec3 even after stage 6, suggesting that they were defective in Notch signaling (Figures 5C–C”). FasIII is also used as a marker of polar cells. However, mutant follicle cells failed to down regulate FasIII and should not be polar cells. Eyes absent (Eya) is normally detected in main-body follicle cells and absent from both polar cells and stalk cells during stage 1 to stage 8 (Bai and Montell, 2002). Immunostaining of Eya was performed and lack of Eya was not detected in mutant main-body follicle clones during stage 1 to stage 8 (Supplementary Figure S5).
Discussion
This report demonstrates a role of sec3 in regulating egg chamber development in Drosophila through Notch. This conclusion is based on results that show that: (1) a loss of sec3 results in abnormal egg chamber development, which phenocopies Notch pathway inactivation; (2) notch accumulates in sec3 clones; (3) target genes of Notch signaling are mis-expressed in sec3 clones. The exocyst has been reported to have functions in the traffic of several membrane proteins, including the adhesion molecule, receptor, and the glucose transporter. However, in this study, the defect in the traffic of Notch protein does not seem to be a general one, as the exocyst is not required for all secretory events. For instance, the exocyst promotes recycling and tethering of E-Cadherin (E-Cad) containing vesicles in the Drosophila ovary or wing, but does not affect the distribution pattern and amount of another two polarity molecules, Baz/Par-3, and Dlg1 (Langevin et al., 2005; Wan et al., 2013). The exocyst is not required for synaptic vesicle release at mature synapses (Murthy et al., 2003). Fibrocystin, Polycystin-2, and Smoothened are transmembrane receptors localized in the ciliary membrane. The exocyst complex regulates the delivery of Fibrocystin and Polycystin-2 to the cilium, but not Smoothened (Monis et al., 2017). Moreover, Delta, the ligand of Notch, is not accumulated in sec3 mutant cells (Supplementary Figure S6).
The positioning of the oocyte requires the upregulation of the E-cad in both the oocyte and the posterior follicle cells, which causes them to adhere to each other (Godt and Tepass, 1998; Gonzalez-Reyes and St Johnston, 1998). Although, it has been reported that E-cad accumulates in sec3 mutant follicle cells (Wan et al., 2013), E-cad is not required for oocyte determination (Godt and Tepass, 1998; Gonzalez-Reyes and St Johnston, 1998), the change of protein levels of E-cad in follicle cells can change the position of the oocyte, but could not generate more than one oocyte in an egg chamber. Therefore, the compound egg chamber phenotype is not a consequence of abnormal E-cad intracellular traffic.
Recent studies have revealed a sophisticated regulation of the Notch receptor by vesicle trafficking. Endocytosis is important in Notch signaling as genetic interactions have been found between Notch and shibire, the Drosophila homolog of dynamin, a key regulator of endocytosis. shibire mutant cells may fail to internalize Notch and show Notch accumulation on the cell surface (Lu and Bilder, 2005). The Notch accumulation phenotype is also found in mutants in avl (avalanche) and rab5, two genes required for maturation of early endosomes (Yan et al., 2009). Active receptors can be internalized to the lysosome and degraded, which is a common mechanism of desensitization. Both NICD and NECD are detected in late endosomal compartments in Drosophila (Kooh et al., 1993). Rab11, a GTPase on the recycling endosomes and Sec15, another component of the exocyst complex, have functions in Delta recycling in the development of the Drosophila sensory organ precursor (SOP) cell lineage (Emery et al., 2005; Jafar-Nejad et al., 2005). In this study, we suppose that the accumulated Notch protein may be in recycling vesicles or in vesicles that transport from the post-Golgi to the plasma membrane. A shortcoming of this study is that we were not able to identify what kind of vesicle the Notch protein accumulated in.
Multiple intercellular signaling pathways involved in the steps of egg chamber development, and a group of mutations have shown an egg chamber fusion phenotype. In particular, reduction in Notch or Delta activity suppresses polar cell formation and results in fused egg chambers (Xu et al., 1992; Bender et al., 1993; Grammont and Irvine, 2001; Lopez-Schier and St Johnston, 2001). The polar cells signal through the JAK/STAT pathway to induce the formation of the stalk which separates adjacent cysts. Reduced JAK/STAT pathway activity results in fused egg chambers (Baksa et al., 2002; McGregor et al., 2002). The Drosophila gene brainiac (brn) and egghead (egh) show an identical egg chamber fusion phenotype. But they are required in the germline and not essential for differentiation of polar and stalk cells (Goode et al., 1996). The activity of the hedgehog (hh) gene stimulates the proliferation of pre-follicle somatic cells in the germarium. Reduced activity of hh produces compound egg chambers which result from a failure in the package of the germline cysts by somatic cells (Forbes et al., 1996). Egg chamber fusion in the sec3 mosaic is not due to abnormal hh signaling, since sec3 mutant clones can encapsulate germline cysts normally at stage 1 (Supplementary Figure S7). Data in this report support that the egg chamber fusion phenotype of sec3 is due to the failure in polar cell formation for abnormal Notch activity.
In summary, this report has identified the developmental function of Sec3 and its link with Notch during Drosophila oogenesis. To our knowledge, this is the first report to identify a link between Sec3 and Notch. Components of the exocyst are conserved from yeast to a human, so it is conceivable that Sec3, as well as other components of the exocyst, are involved in regulating Notch in tissues of other species.
Author Contributions
XW and PW designed the experiments. PW, SZ, LC, DW, and TL performed the data collection and analysis. PW and XY provided the fund. PW and XW wrote the manuscript.
Funding
This work was supported by the Natural Science Foundation of Jiangxi Province (20143ACB21006 and 20142BCB23028), the Science and Technology Program of the Education Department of Jiangxi Province (GJJ150802), and the National Natural Science Foundation of China (31660330 and 31460591).
Conflict of Interest Statement
The authors declare that the research was conducted in the absence of any commercial or financial relationships that could be construed as a potential conflict of interest.
Acknowledgments
We thank the Bloomington Drosophila Stock Center, the Vienna Drosophila RNAi Center, and the Developmental Studies Hybridoma Bank for their Drosophila stocks and antibodies. We also thank St. Johnston D for the antibody reagent.
Supplementary Material
The Supplementary Material for this article can be found online at: https://www.frontiersin.org/articles/10.3389/fphys.2019.00345/full#supplementary-material
References
Bai, J., and Montell, D. (2002). Eyes absent, a key repressor of polar cell fate during Drosophila oogenesis. Development 129, 5377–5388. doi: 10.1242/dev.00115
Baksa, K., Parke, T., Dobens, L. L., and Dearolf, C. R. (2002). The Drosophila STAT protein, stat92E, regulates follicle cell differentiation during oogenesis. Dev. Biol. 243, 166–175. doi: 10.1006/dbio.2001.0539
Bender, L. B., Kooh, P. J., and Muskavitch, M. A. (1993). Complex function and expression of delta during Drosophila oogenesis. Genetics 133, 967–978.
Bloch, D., Pleskot, R., Pejchar, P., Potocky, M., Trpkosova, P., Cwiklik, L., et al. (2016). Exocyst SEC3 and phosphoinositides define sites of exocytosis in pollen tube initiation and growth. Plant Physiol. 172, 980–1002. doi: 10.1104/pp.16.006901
Boyd, C., Hughes, T., Pypaert, M., and Novick, P. (2004). Vesicles carry most exocyst subunits to exocytic sites marked by the remaining two subunits. Sec3p and Exo70p. J. Cell Biol. 167, 889–901. doi: 10.1083/jcb.200408124
Deng, W. M., Althauser, C., and Ruohola-Baker, H. (2001). Notch-delta signaling induces a transition from mitotic cell cycle to endocycle in Drosophila follicle cells. Development 128, 4737–4746.
Emery, G., Hutterer, A., and Berdnik, D. (2005). Asymmetric Rab 11 endosomes regulate delta recycling and specify cell fate in the Drosophila nervous system. Cell 122, 763–773. doi: 10.1016/j.cell.2005.08.017
Finger, F. P., Hughes, T. E., and Novick, P. (1998). Sec3p is a spatial landmark for polarized secretion in budding yeast. Cell 92, 559–571. doi: 10.1016/S0092-8674(00)80948-4
Forbes, A. J., Lin, H., Ingham, P. W., and Spradling, A. C. (1996). hedgehog is required for the proliferation and specification of ovarian somatic cells prior to egg chamber formation in Drosophila. Development 122, 1125–1135.
Godt, D., and Tepass, U. (1998). Drosophila oocyte localization is mediated by differential cadherin-based adhesion. Nature 395, 387–391. doi: 10.1038/26493
Gonzalez-Reyes, A., and St Johnston, D. (1998). The Drosophila AP axis is polarised by the cadherin-mediated positioning of the oocyte. Development 125, 3635–3644.
Goode, S., Melnick, M., Chou, T. B., and Perrimon, N. (1996). The neurogenic genes egghead and brainiac define a novel signaling pathway essential for epithelial morphogenesis during Drosophila oogenesis. Development 122, 3863–3879.
Grammont, M., and Irvine, K. D. (2001). fringe and notch specify polar cell fate during Drosophila oogenesis. Development 128, 2243–2253.
Guo, W., Roth, D., Walch-Solimena, C., and Novick, P. (1999). The exocyst is an effector for Sec4p, targeting secretory vesicles to sites of exocytosis. EMBO J. 18, 1071–1080. doi: 10.1093/emboj/18.4.1071
Jafar-Nejad, H., Andrews, H. K., and Acar, M. (2005). Sec15, a component of the exocyst, promotes notch signaling during the asymmetric division of Drosophila sensory organ precursors. Dev. Cell 9, 351–363. doi: 10.1016/j.devcel.2005.06.010
Kooh, P. J., Fehon, R. G., and Muskavitch, M. A. (1993). Implications of dynamic patterns of delta and notch expression for cellular interactions during Drosophila development. Development 117, 493–507.
Kopan, R., and Ilagan, M. X. (2009). The canonical notch signaling pathway: unfolding the activation mechanism. Cell 137, 216–233. doi: 10.1016/j.cell.2009.03.045
Lai, E. C. (2004). Notch signaling: control of cell communication and cell fate. Development 131, 965–973. doi: 10.1242/dev.01074
Langevin, J., Morgan, M. J., Sibarita, J. B., Aresta, S., Murthy, M., Schwarz, T., et al. (2005). Drosophila exocyst components Sec5, Sec6, and Sec15 regulate DE-cadherin trafficking from recycling endosomes to the plasma membrane. Dev. Cell 9, 365–376. doi: 10.1016/j.devcel.2005.07.013
Lopez-Schier, H., and St Johnston, D. (2001). Delta signaling from the germ line controls the proliferation and differentiation of the somatic follicle cells during Drosophila oogenesis. Genes Dev. 15, 1393–1405. doi: 10.1101/gad.200901
Lu, H., and Bilder, D. (2005). Endocytic control of epithelial polarity and proliferation in Drosophila. Nat. Cell Biol. 7, 1232–1239. doi: 10.1038/ncb1324
McGregor, J. R., Xi, R., and Harrison, D. A. (2002). JAK signaling is somatically required for follicle cell differentiation in Drosophila. Development 129, 705–717.
Monis, W. J., Faundez, V., and Pazour, G. J. (2017). BLOC-1 is required for selective membrane protein trafficking from ensosomes to primary cilia. J. Cell Biol. 216, 2131–2150. doi: 10.1083/jcb.201611138
Murthy, M., Garza, D., Scheller, R. H., and Schwarz, T. L. (2003). Mutations in the exocyst component Sec5 disrupt neuronal membrane traffic, but neurotransmitter release persists. Neuron 37, 433–447. doi: 10.1016/S0896-6273(03)00031-X
Novick, P., Field, C., and Schekman, R. (1980). Identification of 23 complementation groups required for post-translational events in the yeast secretory pathway. Cell 21, 205–215. doi: 10.1016/0092-8674(80)90128-2
Shcherbata, H. R., Althauser, C., Findley, S. D., and Ruohola-Baker, H. (2004). The mitotic-to-endocycle switch in Drosophila follicle cells is executed by notch-dependent regulation of G1/S. G2/M and M/G1 cell-cycle transitions. Development 131, 3169–3181. doi: 10.1242/dev.01172
Sorkin, A., and von Zastrow, M. (2009). Endocytosis and signalling: intertwining molecular networks. Nat. Rev. Mol. Cell Biol. 10, 609–622. doi: 10.1038/nrm2748
Sun, J., and Deng, W. M. (2005). Notch-dependent downregulation of the homeodomain gene cut is required for the mitotic cycle/endocycle switch and cell differentiation in Drosophila follicle cells. Development 132, 4299–4308. doi: 10.1242/dev.02015
Torres, I. L., Lopez-Schier, H., and St Johnston, D. (2003). A notch/delta-dependent relay mechanism establishes anterior-posterior polarity in Drosophila. Dev. Cell 5, 547–558. doi: 10.1016/S1534-5807(03)00272-7
Vachias, C., Couderc, J. L., and Grammont, M. (2010). A two-step notch-dependant mechanism controls the selection of the polar cell pair in Drosophila oogenesis. Development 137, 2703–2711. doi: 10.1242/dev.052183
Wan, P., Wang, D., Luo, J., Chu, D., Wang, H., Zhang, L., et al. (2013). Guidance receptor promotes the asymmetric distribution of exocyst and recycling endosome during collective cell migration. Development 140, 4797–4806. doi: 10.1242/dev.094979
Wen, T. J., Hochholdinger, F., Sauer, M., Bruce, W., and Schnable, P. S. (2005). The roothairless1 gene of maize encodes a homolog of sec3, which is involved in polar exocytosis. Plant Physiol. 138, 1637–1643. doi: 10.1104/pp.105.062174
Xu, T., Caron, L. A., Fehon, R. G., and Artavanis-Tsakonas, S. (1992). The involvement of the notch locus in Drosophila oogenesis. Development 115, 913–922.
Yamashita, M., Kurokawa, K., Sato, Y., Yamagata, A., Mimura, H., Yoshikawa, A., et al. (2010). Structural basis for the Rho- and phosphoinositide-dependent localization of the exocyst subunit Sec3. Nat. Struct. Mol. Biol. 17, 180–186. doi: 10.1038/nsmb.1722
Yan, Y., Denef, N., and Schupbach, T. (2009). The vacuolar proton pump, V-ATPase, is required for notch signaling and endosomal trafficking in Drosophila. Dev. Cell 17, 387–402. doi: 10.1016/j.devcel.2009.07.001
Yue, P., Zhang, Y., Mei, K., Wang, S., Lesigang, J., Zhu, Y., et al. (2017). Sec3 promotes the initial binary t-SNARE complex assembly and membrane fusion. Nat. Commun. 8:14236. doi: 10.1038/ncomms14236
Zhang, X., Orlando, K., He, B., Xi, F., Zhang, J., Zajac, A., et al. (2008). Membrane association and functional regulation of Sec3 by phospholipids and Cdc42. J. Cell Biol. 180, 145–158. doi: 10.1083/jcb.200704128
Zhang, X., Wang, P., Gangar, A., Zhang, J., Brennwald, P., Terbush, D., et al. (2005). Lethal giant larvae proteins interact with the exocyst complex and are involved in polarized exocytosis. J. Cell. Biol. 170, 273–283. doi: 10.1083/jcb.200502055
Keywords: Sec3, exocyst, Notch, Drosophila, ovary
Citation: Wan P, Zheng S, Chen L, Wang D, Liao T, Yan X and Wang X (2019) The Exocyst Component Sec3 Controls Egg Chamber Development Through Notch During Drosophila Oogenesis. Front. Physiol. 10:345. doi: 10.3389/fphys.2019.00345
Received: 01 October 2018; Accepted: 14 March 2019;
Published: 29 March 2019.
Edited by:
Giorgio F. Gilestro, Imperial College London, United KingdomReviewed by:
Gábor Juhász, Eötvös Loránd University, HungaryMarko Brankatschk, Dresden University of Technology, Germany
Copyright © 2019 Wan, Zheng, Chen, Wang, Liao, Yan and Wang. This is an open-access article distributed under the terms of the Creative Commons Attribution License (CC BY). The use, distribution or reproduction in other forums is permitted, provided the original author(s) and the copyright owner(s) are credited and that the original publication in this journal is cited, in accordance with accepted academic practice. No use, distribution or reproduction is permitted which does not comply with these terms.
*Correspondence: Xiaoji Wang, V2FuZ3hqNzdAaG90bWFpbC5jb20=