- Department of Preventive Veterinary Medicine and Animal Health, Faculty of Veterinary Medicine, University of São Paulo, São Paulo, Brazil
The bacterium Rickettsia rickettsii is the etiological agent of Brazilian spotted fever (BSF), which is transmitted in Brazil mainly by the tick Amblyomma sculptum. Herein, larvae and nymphs of six populations of A. sculptum were exposed to R. rickettsii by feeding on needle-inoculated guinea pigs, and thereafter reared on uninfected guinea pigs or rabbits. Two tick populations were exposed to autochthone R. rickettsii strains, whereas four tick populations were exposed to non-autochthone strains. The six geographically different populations of A. sculptum showed different susceptibilities to R. rickettsii, higher among the two tick populations that were exposed to their autochthone R. rickettsii strain. In addition, higher rates of transovarial transmission of R. rickettsii and vector competence success also included the two tick populations that were exposed to autochthone R. rickettsii strains. These results indicate that the susceptibility of A. sculptum to R. rickettsii varies among different tick populations, with a clear bias for higher susceptibility to an autochthone R. rickettsii strain that has already coevolved with a tick population for some time. Our results demonstrated that the R. rickettsii infection induces higher mortality of engorged larvae and nymphs, and tend to reduce the reproductive fitness of engorged females. All together, these results might explain the low R. rickettsii-infection rates of A. sculptum under natural conditions (usually <1%), and indicate that an A. sculptum population should not be able to sustain a R. rickettsii infection for successive tick generations without the creation of new cohorts of infected ticks via horizontal transmission on vertebrate rickettsemic hosts (amplifying hosts). Finally, despite of the ubiquitous distribution of A. sculptum in southeastern and central-western Brazil, most of the populations of this tick species are devoid of R. rickettsii infection. This scenario might be related to two major factors: (i) insufficient numbers of susceptible amplifying hosts; and (ii) lower susceptibilities of many tick populations. While the first factor has been demonstrated by mathematical models in previous studies, the second is highlighted by the results observed in the present study.
Introduction
The bacterium Rickettsia rickettsii is the etiological agent of Brazilian spotted fever (BSF), the deadliest tick-borne disease of the New World. In southeastern Brazil, 776 cases of BSF were laboratory-confirmed in humans from 2007 to 2015, with an overall case-fatality rate of 55% (Oliveira et al., 2016). While several tick species of the genus Amblyomma have been implicated in the transmission of R. rickettsii to humans in South America (Labruna et al., 2014), Amblyomma sculptum (formerly called as Amblyomma cajennense in southeastern Brazil) is the most important vector, since most of the BSF-confirmed cases have been associated to this tick species (Pinter et al., 2011; Krawczak et al., 2014; Labruna et al., 2017).
Amblyomma sculptum, a member of the A. cajennense species complex, is by far the most frequent human-biting tick species in Brazil (Guglielmone et al., 2006; Ramos et al., 2014; Martins et al., 2016). While this may suggest a high exposure of humans to R. rickettsii infection, the incidence of BSF has been as low as 0.14/100,000 inhabitants (Oliveira et al., 2016), thanks to the very low R. rickettsii-infection rates among A. sculptum populations, reported to be between 0.05 and 1.28% (Guedes et al., 2005, 2011; Krawczak et al., 2014; Labruna et al., 2017). The very low R. rickettsii-infection rates of A. sculptum under natural conditions has been attributed to the lower susceptibility of this tick species to the bacterium when compared to other tick species that are found with much higher infection rates under natural conditions, such as Amblyomma aureolatum and Rhipicephalus sanguineus sensu lato (s.l.) (Labruna et al., 2008, 2011; Pacheco et al., 2011; Ogrzewalska et al., 2012; Soares et al., 2012).
Amblyomma sculptum has a ubiquitous distribution in southeastern and central-western Brazil (Martins et al., 2016); however, only a few populations of this tick species have been found infected by R. rickettsii (Sangioni et al., 2005; Pacheco et al., 2007, 2009; Soares et al., 2012). This scenario could be linked to different susceptibilities to R. rickettsii infection among different populations of A. sculptum. Previous studies that quantified the susceptibility of A. sculptum to R. rickettsii employed a rickettsial strain that was originally isolated from A. aureolatum ticks in an area without A. sculptum (Labruna et al., 2008; Soares et al., 2012). Therefore, more reliable data could be obtained with additional studies employing A. sculptum-derived R. rickettsii strains. Based on the above statements, the present study evaluated the comparative susceptibility of different populations of A. sculptum to the infection by R. rickettsii, using two rickettsial strains that were originally isolated from A. sculptum in Brazil.
Materials and Methods
Ethics Statements
This study has been approved by the Institutional Animal Care and Use Committee (IACUC) of the Faculty of Veterinary Medicine of the University of São Paulo (protocols 3104/2013 and 5948070314). Field capture of ticks was authorized by the Brazilian Ministry of the Environment (permit SISBIO Nos. 43259-1 and 11459-1).
Ticks
A total of six geographically distinct populations of A. sculptum were used in this study, as detailed in Table 1. From five populations, unfed adults were collected on the vegetation by dragging or dry ice traps following Terassini et al. (2010) or Szabó et al. (2009), and brought alive to the laboratory, where adult ticks were allowed to feed on tick-naïve rabbits, in order to establish five independent tick colonies. From a sixth tick population (E-ITU), engorged females were collected from capybaras (Hydrochoerus hydrochaeris) and brought to the laboratory to start a tick colony.
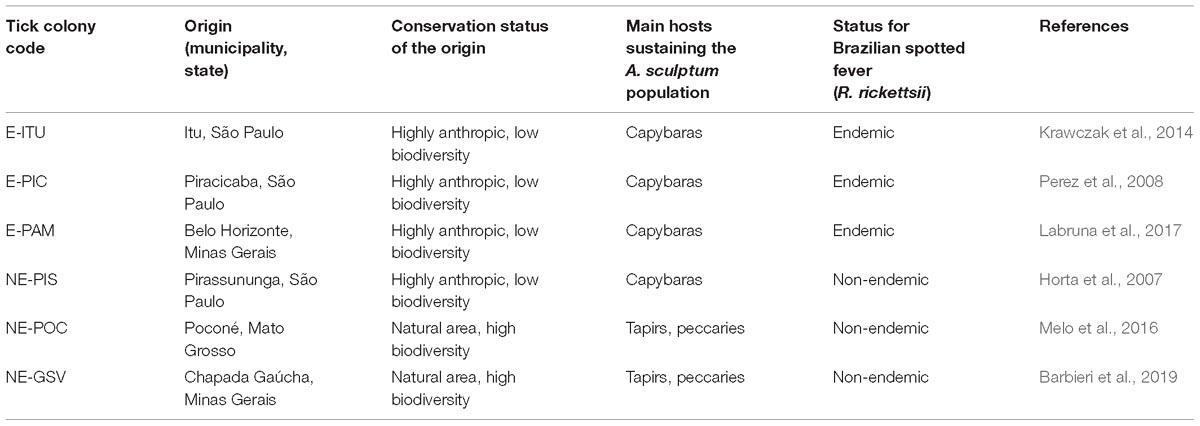
Table 1. General characteristics of the local origin of the Amblyomma sculptum populations that originated the six tick colonies used in the present study.
For all six tick colonies, off-host conditions were provided by an incubator set for a temperature of 27°C, 85% relative humidity and photoperiod 0:24 (light:dark). Our experimental infestations were started with the F1 larvae (first lab generation) of each of the six tick colonies. Before starting the experiments, we had the following evidence that these ticks were free of rickettsial infection: all engorged females that yielded the F1 larvae, as well as a small sample of their egg batches were negative by real-time PCR for the genus Rickettsia (protocol described below) at the end of oviposition; and all rabbits remained seronegative to R. rickettsii (protocol described below) 21 days after being infested with field-collected adult ticks.
Among the six colonies of A. sculptum, three were from BSF-endemic areas in which fatal cases of BSF have been reported recently, in association with the transmission by A. sculptum. Three other colonies were from areas where BSF was never reported (non-endemic areas), which include a highly anthropic area in the state of São Paulo, and two natural areas with a rich biodiversity (Table 1).
Rickettsia rickettsii Strains
Two strains of R. rickettsii were used in this study, strain Itu and strain Pampulha. The former was isolated from A. sculptum collected in a BSF-endemic area in Itu municipality (Krawczak et al., 2014), the same area where we collected A. sculptum adult ticks to form our E-ITU tick colony. The later was collected in the Pampulha Lake area, a BSF-endemic area in Belo Horizonte City (Labruna et al., 2017), where we collected A. sculptum adult ticks to form our E-PAM tick colony. Both R. rickettsii strains were originally isolated through the inoculation of guinea pigs with field-collected A. sculptum tick homogenate and subsequently adaptation of the strain to Vero cell culture (Krawczak et al., 2014; Labruna et al., 2017). However, only the guinea pig lineage of each strain (never in vitro cultured) was used for experimental infections in the present study. For this purpose, each rickettsial strain has been maintained through guinea pig passages in our laboratory, and in each passage we have cryopreserved fragments of infected spleen, lung, liver, and brain. In the present study, we used fragments of these four organs of the third guinea pig passage of each isolate to inoculate guinea pigs, as described below.
Tick Infestation Protocols
Throughout this study, larvae and nymphs were allowed to feed on tick-naïve adult male (or female in a few cases) guinea pigs, whereas adult ticks were allowed to feed on tick-naïve adult male domestic rabbits. In either case, tick infestations were performed inside cotton sleeves (10–20 cm diameter) that were glued to the shaved back of each animal, as previously described (Pinter et al., 2002; Horta et al., 2009). Ticks (larvae, nymphs or adults, 20–30 days old) were released into the sleeve. Larval infestations consisted of approximately 500–1500 larvae per guinea pig; nymphal infestations consisted of 80–100 nymphs per guinea pig; and adult infestations consisted of 7–20 couples per rabbit. The sleeves were opened daily, and the detached engorged ticks were removed, counted and immediately taken to the incubator where molting (for engorged larvae and nymphs) or oviposition (for engorged females) was observed daily. Engorged females were individually weighed in an electronic balance (precision of 0.0001 g) at the detachment day. Molting success (survivorship) was determined for engorged larvae and nymphs whereas the following biological parameters were determined for engorged females: feeding period (number of days from placement of the ticks on the host to the detachment), oviposition success (proportion of engorged females that successfully oviposited), preoviposition period (number of days from detachment to the beginning of oviposition) and egg mass incubation period (number of days from the beginning of oviposition to the hatching of the first larva). Additionally, the total egg mass laid by each female was weighed at the end of oviposition and a conversion efficiency index (CEI = mg egg mass/mg engorged female × 100), which measures the efficiency with which a tick species converts body weight into eggs (Drummond and Whetstone, 1970), was determined for each female that oviposited. Percentage of hatching for each female egg mass was visually estimated according to Labruna et al. (2000). During the experiment, animals were fed with commercial pellets and water ad libitum.
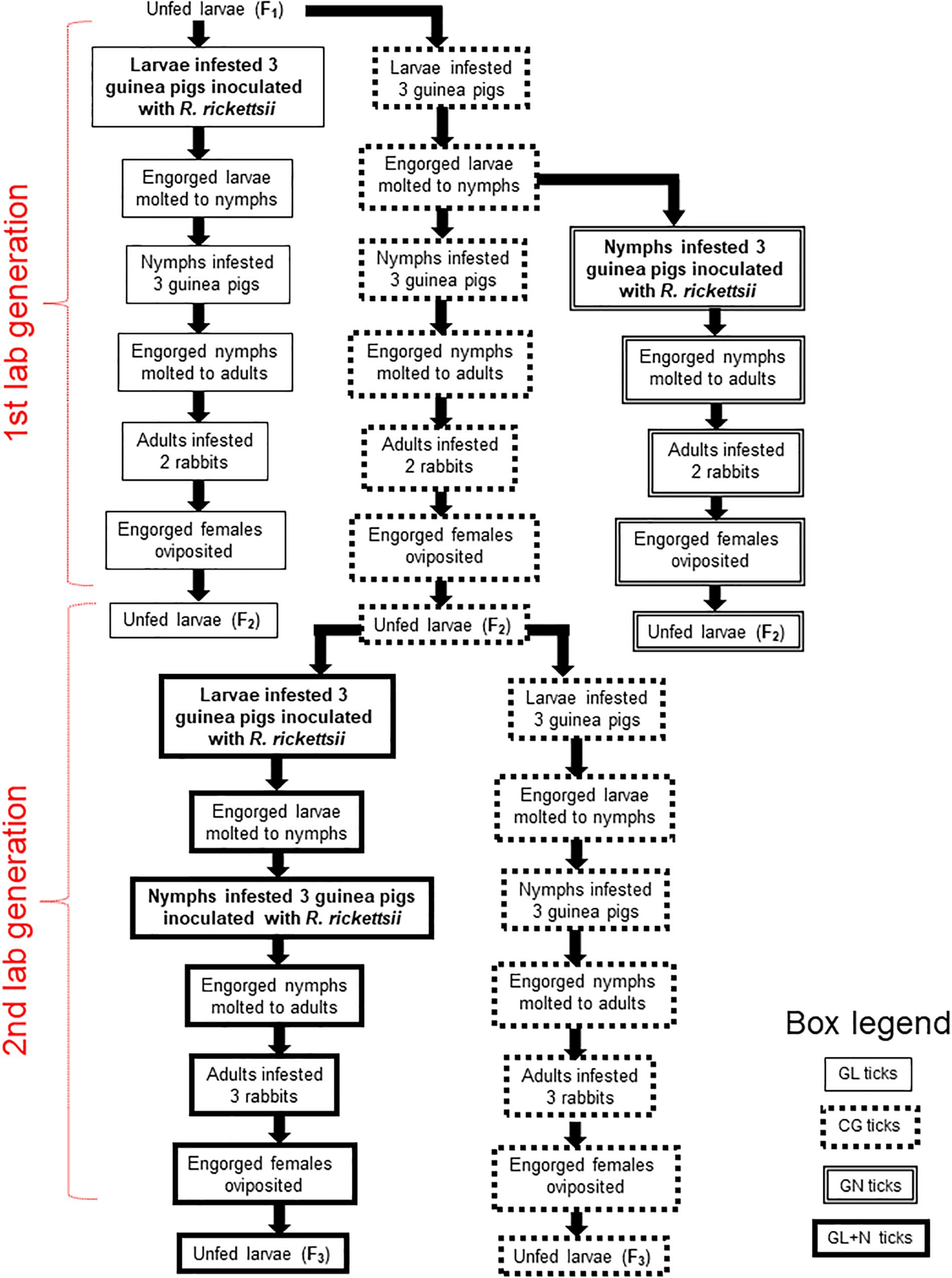
Figure 1. Diagram illustrating experimental procedures with each of the six colonies of Amblyomma sculptum ticks from F1 larvae to F3 larvae. GL and GN ticks were exposed to Rickettsia rickettsii-inoculated guinea pigs during the F1 larval and nymphal stages, respectively, and thereafter, they were reared through the F2 larval stage on susceptible animals (guinea pigs for larvae and nymphs; rabbits for adult ticks). GL + N ticks were dually exposed to R. rickettsii-inoculated guinea pigs, firstly during the F2 larval stage, secondly during the F2 nymphal stage, and then reared through the F3 larval stage on susceptible rabbits. CG ticks were the uninfected control group, always exposed to susceptible guinea pigs or rabbits in parallel to the infected groups.
Experimental Procedures
Most of the experimental procedures used in this study were exactly the same that were used in a previous study at our laboratory, in which an A. sculptum population from Pedreira, state of São Paulo, was experimentally infected with R. rickettsii strain Taiaçu, previously isolated from the tick A. aureolatum (Soares et al., 2012). These procedures were adopted, so most of our results can be compared to the results reported by Soares et al. (2012), as presented below in the Discussion section.
In the present study, the strain Itu of R. rickettsii was used for experimental infection of five tick colonies: E-ITU, E-PIC, NE-PIS, NE-POC, and NE-GSV. The strain Pampulha of R. rickettsii was used for experimental infection of only the E-PAM tick colony. With these procedures, we had two tick colonies exposed to autochthone strains of R. rickettsii (E-ITU to strain Itu; E-PAM to strain Pampulha), and four tick colonies exposed to a non-autochthone strain of R. rickettsii (E-PIC, NE-PIS, NE-POC, and NE-GSV to strain Itu); in the latter case, one tick colony (E-PIC) was from a BSF-endemic area, while the remaining three tick colonies were from BSF non-endemic areas. In addition, NE-POC and NE-GSV were from highly preserved areas with rich biodiversity, whereas NE-PIS was from a highly anthropic area (Table 1).
Procedures of experimental infection of ticks with R. rickettsii and the follow up infestations, from F1 larvae to F3 larvae (or F3 nymphs for a single tick colony) were the same for each tick colony, and are summarized in Figure 1. In all cases, the initial exposition of ticks to R. rickettsii infection consisted of ticks (larvae or nymphs) feeding on R. rickettsii-infected guinea pigs. For this purpose, samples from our stock of cryopreserved guinea pig-infected organs were thawed at room temperature, crushed in a mortar with brain–heart infusion (BHI) and the resultant homogenate was used to inoculate guinea pigs intraperitoneally, as previously described (Soares et al., 2012). At the same day of guinea pig inoculation, these animals were infested with ticks. During the F1 generation, three experimental groups of ticks (GL, GN, and CG) were formed in each colony. GL and GN consisted of ticks that were exposed to R. rickettsii-inoculated guinea pigs during the F1 larval or nymphal stages, respectively, and thereafter they were reared through the F2 larval stage on susceptible hosts (guinea pigs or rabbits). CG was the uninfected control group, always exposed to susceptible, uninfected hosts (Figure 1).
In the second lab generation of the study, F2 larvae of the uninfected control group (CG) of each tick colony were exposed to R. rickettsii-inoculated guinea pigs, and the resultant nymphs were also exposed to R. rickettsii-inoculated guinea pigs, forming the GL + N group (ticks with dual exposure to R. rickettsii-inoculated guinea pigs). These ticks were reared through the F3 larval or nymphal stage, with ticks feeding on susceptible, uninfected hosts. In parallel, CG ticks were reared through the F3 larval stage, always exposed to susceptible, uninfected hosts (Figure 1).
Guinea Pig and Rabbit Evaluation
All infested animals had their rectal temperature measured from 0 to 21 days post-inoculation or infestation (dpi). Guinea pigs were considered febrile when rectal temperature was >39.5°C (Monteiro, 1931); rabbits were considered febrile when rectal temperature was >40.0°C (Monteiro, 1933). The occurrence of scrotal reactions (edema, congestion, necrosis), typical of R. rickettsii acute infection in guinea pigs and rabbits (Monteiro, 1933), were annotated. In all infestations, approximately 0.5–1.0 mL of blood was obtained from each guinea pig or rabbit at days 0 and 21 dpi. Guinea pig blood samples were collected intra-cardiacally under anesthesia (20 mg/Kg xylazine + 20 mg/kg cetamin + 1 mg/kg acepromazin), while rabbit samples were collected through the central ear vein. Samples were centrifuged to obtain sera, which were tested for seroconversion to R. rickettsii, as described below. If any guinea pig or rabbit died before 21 dpi, it was subjected to necropsy, when we tried to obtain a blood sample through cardiac puncture. In addition, a lung sample was collected and evaluated by PCR for rickettsial infection, using the protocol described below.
Molecular Analysis
Throughout the study, samples of unfed larvae, nymphs and adults, eggs, and engorged females at the end of oviposition, were tested by PCR for detection of rickettsial DNA. For this purpose, DNA was extracted from eggs (in pools of ≈50 eggs or individually), unfed larvae (in pools of ≈50 larvae or individually), and unfed nymphs by the boiling method, as previously described (Horta et al., 2005), and from unfed adult ticks or engorged females at the end of oviposition by the guanidine isothiocyanate-phenol technique (Sangioni et al., 2005). Guinea pig or rabbit lung fragments were submitted to DNA extraction using the DNeasy tissue Kit (Qiagen, Chatsworth, CA, United States). Blank tubes were always included in the DNA extraction procedures, as negative controls of this step.
Extracted DNA samples were tested by a Taqman real-time PCR assay targeting the rickettsial gltA gene, as described (Soares et al., 2012). The sensitivity of this PCR assay was determined to be one DNA copy of R. rickettsii (Labruna et al., 2004). Tick DNA samples that were shown to contain no rickettsial DNA by the real-time PCR described above were tested by a conventional PCR protocol targeting the tick mitochondrial 16S rRNA gene, as previously described (Mangold et al., 1998). If the tick sample yielded no product by this PCR, it was considered that DNA extraction was not successful, and the sample was discarded from the study. Random samples of F1 adult ticks, positive by the real-time PCR assay, were further tested using a conventional PCR assay targeting a 532-bp fragment of the rickettsial ompA gene, as described (Regnery et al., 1991); PCR products were DNA-sequenced and the resultant sequences were submitted to BLAST analysis1 in order to confirm the identity of the Rickettsia species.
Serological Analysis
Guinea pig and rabbit blood serum samples were individually tested by the indirect immunofluorescence assay (IFA) using crude antigens derived from R. rickettsii strain Taiaçu, as previously described (Labruna et al., 2007). Each serum was diluted in two-fold increments with PBS from 1:64 to the endpoint titer (Labruna et al., 2007). A commercial fluorescein isothiocyanate-labeled goat anti-rabbit IgG (Sigma Diagnostics, St. Louis, MO, United States) or rabbit anti-guinea pig IgG (Sigma Diagnostics) was used. In each slide, a serum previously shown to be non-reactive (negative control) and a known reactive serum (positive control) were tested at the 1:64 dilution. These serum samples were from the study of Soares et al. (2012).
Statistical Analyses
The proportions of ticks that successfully molted or oviposited fertile eggs from different groups, the proportion of R. rickettsii-infected ticks from different colonies, and fatality rates of inoculated guinea pigs were compared by using the chi-square test. Reproductive parameters of engorged females were compared between colonies/groups by using the t-test, after the Kolmogorov–Smirnov normality test. Analyses were performed using the program Epi InfoTM version 7 (chi-square) and Minitab® 18.1 (t-Student). Significant differences were considered for P < 0.05.
Phylogenetic Analyses of Ticks
In order to perform a comparative genetic analysis of R. rickettsii-infected and uninfected ticks of each colony, we generated partial sequences of the tick mitochondrial 16S rRNA gene and the tick second internal transcribed spacer (ITS2). Noteworthy, the uninfected tick samples were composed by adult ticks that were exposed to R. rickettsii infection (through feeding as larvae and/or nymphs on rickettsemic guinea pigs), but were shown to contain no rickettsial DNA when tested as unfed adults by the real-time PCR protocol described above.
From each tick colony, DNA of R. rickettsii-infected and uninfected ticks were submitted to two PCR protocols, one targeting a ≈460-bp fragment of the tick mitochondrial 16S rRNA gene (Mangold et al., 1998) and one targeting a ≈1,100-bp of the tick nuclear ribosomal region, which includes the entire ITS2, as previously described (Marrelli et al., 2007). All PCR products were treated with ExoSap (USB, Cleveland, OH, United States) and sequenced in an ABI automated sequencer (Applied Biosystems/Thermo Fisher Scientific, model ABI 3500 Genetic Analyzer, Foster City, CA, United States) with the same primers used for PCR. The consensus 16S rRNA sequences were aligned by using Clustal/W v.1.8.1 (Thompson et al., 1994), including the 16S rRNA sequence of Amblyomma tonelliae from Paraguay retrieved from GenBank (KF179349), which was used as the outgroup. A nucleotide identity matrix was calculated by using the Bioedit software v.7.0.5.3 (Hall, 1999). A phylogenetic tree was generated with the Maximum Likelihood criteria (Tamura 3-parameter model) for the 16S rRNA mitochondrial gene partial nucleotide sequences (410 sites on the dataset) and 1,000 bootstrap replicates using Mega X software (Kumar et al., 2018).
Results
Inoculated Guinea Pigs
In order to form the R. rickettsii-infected tick cohorts, larvae and nymphs of the six colonies were exposed to R. rickettsii by feeding on guinea pigs that had been inoculated with homogenates of R. rickettsii-infected guinea pig organs. A total of 72 guinea pigs were inoculated, which all but one developed fever that started 3–8 dpi (median: 4; mean: 3.9 ± 0.9), and lasted for 3–11 days (median: 5; mean: 5.4 ± 2.0) (Supplementary Tables S1–S12). Ticks that had fed on the only non-febrile-inoculated guinea pig (guinea pig G.P.78) were discarded from the study (Supplementary Table S6). Rickettsial infection was confirmed in all febrile guinea pigs by seroconversion to R. rickettsii at 21 dpi (endpoint titers ranging from 16,384 to 65,536; median: 32,768) or by detection of rickettsial DNA in a lung sample collected from those guinea pigs that died during the febrile period. For instance, 53 out of 71 guinea pigs died during the febrile period (Supplementary Tables S1–S12), resulting in an overall fatality rate of 74%. Considering the two R. rickettsii strains separately, fatality rates were 75% (45/60) for strain Itu, and 72% (8/11) for strain Pampulha (P > 0.05). Larval or nymphal feeding periods lasted from 3 to 10 days, with most of the engorged ticks detaching between 4 and 7 dpi. Since larval and nymphal infestations were performed at the same day of guinea pig inoculation, tick feeding period overlapped with the febrile period, a condition that certifies that these ticks fed during the rickettsemic period; hence, they were exposed to R. rickettsii.
Rickettsial Infection in GL Ticks
The GL ticks consisted of F1 larvae initially exposed to R. rickettsii by feeding on rickettsemic guinea pigs, and thereafter, reared until F2 unfed larvae by feeding on susceptible hosts. After F1 acquisition larval feeding, rickettsial infection rates of F1 nymphs (transstadial perpetuation of R. rickettsii from larvae to nymphs) varied from 0 to 16% among the six tick colonies, with higher rates for the two tick colonies that were exposed to autochthone R. rickettsii strains, namely E-ITU and E-PAM (Table 2). Infestation of these F1 nymphs on susceptible guinea pigs resulted in successful rickettsial transmission (fever followed by death or seroconversion with high endpoint titers) in all three guinea pigs exposed to E-ITU and E-PAM nymphs, only one guinea pig exposed to NE-PIS or NE-GSV, and none of the guinea pigs exposed to E-PIC and NE-POC (Table 3). Transstadial perpetuation of R. rickettsii from F1 nymphs to adults were observed by DNA detection only in E-ITU and E-PAM ticks (33 and 47%, respectively), which were infected with autochthone R. rickettsii strains (Table 2). Infestation of F1 adults on susceptible rabbits resulted in successful rickettsial transmission (fever followed by death or seroconversion with high endpoint titers) in all two rabbits exposed to E-ITU ticks, and one out of two rabbits exposed to E-PAM, NE-PIS, and NE-GSV adults, and none of the two rabbits exposed to either E-PIC or NE-POC (Table 3). Transovarial transmission of R. rickettsii was observed by DNA detection in engorged females of only the E-ITU, E-PAM, and NE-PIS tick colonies. In these cases, the transovarial transmission rate (no. females with PCR-positive eggs or larvae/no. PCR-positive females at the end of oviposition × 100) varied from 12 to 18% (Table 2). For these infected females with rickettsial transovarial transmission, we calculated the filial infection rate (No. infected eggs or larvae/No. tested eggs or larvae × 100), which was 100% for either E-ITU or E-PAM ticks (infected with autochthone R. rickettsii strains), and 0–10% for NE-PIS ticks (infected with non-autochthone R. rickettsii strain) (Supplementary Table S13). The transovarial acquisition infection of E-PAM F2-infected larvae was confirmed by allowing part of these larvae to feed on a guinea pig, which became ill and seroconverted to R. rickettsii (guinea pig G.P. 73 in Supplementary Table S5).
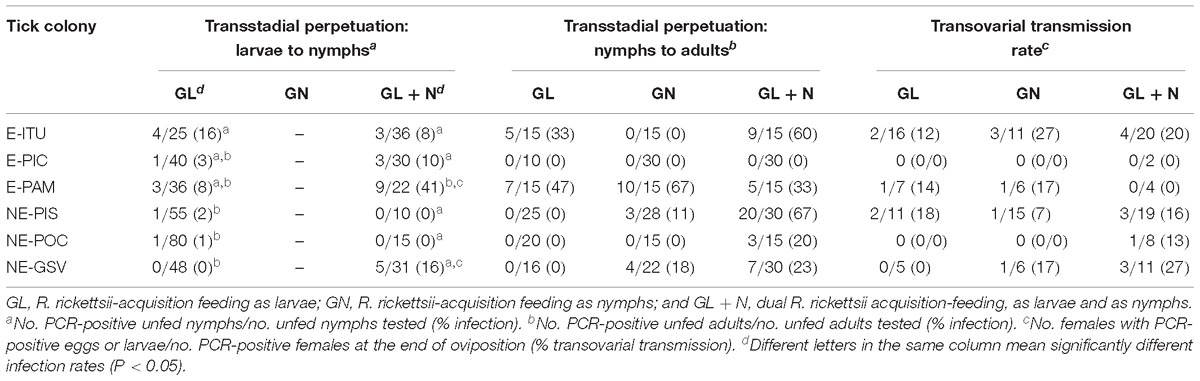
Table 2. Tick-Rickettsia rickettsii acquisition (feeding on rickettsemic guinea pig) and maintenance (transstadial perpetuation and transovarial transmission).
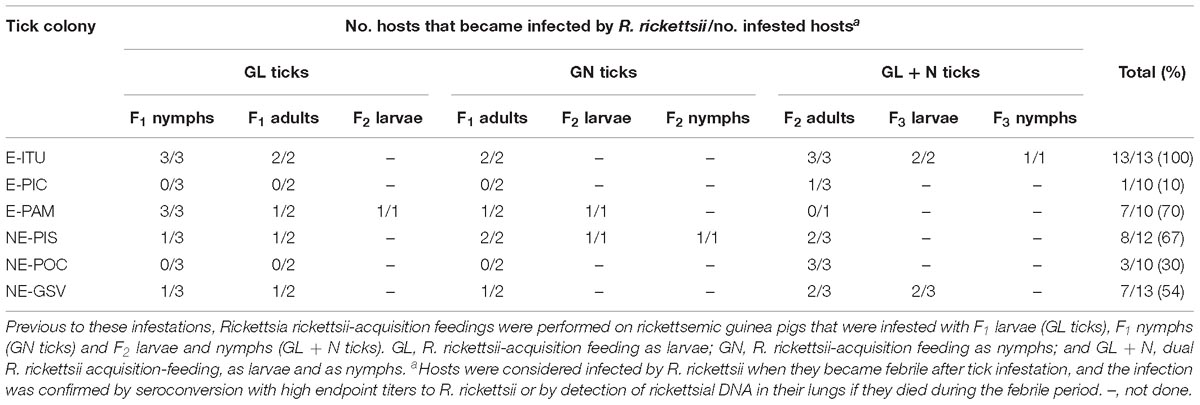
Table 3. Results of rickettsial transmission by ticks (vector competence) of six colonies of Amblyomma sculptum that were allowed to feed on susceptible hosts (guinea pigs for larvae and nymphs; rabbits for adults).
Rickettsial Infection in GN Ticks
The GN ticks consisted of F1 nymphs initially exposed to R. rickettsii by feeding on rickettsemic guinea pigs, and thereafter, reared until F2 unfed larvae or nymphs by feeding on susceptible hosts. After F1 acquisition nymphal feeding, rickettsial infection rates of F1 adults (transstadial perpetuation of R. rickettsii from nymphs to adults) were observed by DNA detection in ticks of only three tick colonies, 67% for E-PAM, 11% for NE-PIS, and 18% for NE-GSV (Table 2). Infestation of these F1 adults on susceptible rabbits resulted in successful rickettsial transmission (fever followed by death or seroconversion with high endpoint titers) in all two rabbits exposed to either E-ITU, E-PAM or NE-PIS ticks, and one out of two rabbits exposed to NE-GSV adults, and none of the two rabbits exposed to either E-PIC or NE-POC (Table 3). Transovarial transmission of R. rickettsii was observed by DNA detection in engorged females of the E-ITU, E-PAM, NE-PIS, and NE-GSV tick colonies. In these cases, the transovarial transmission rate varied from 7 to 27% (Table 2). For these infected females with rickettsial transovarial transmission, the filial infection rates were 90–100% for E-ITU, 60% for E-PAM, 10–50% for NE-PIS, and 70% for NE-GSV (Supplementary Table S13). The transovarial acquisition infection of either E-PAM or NE-PIS F2-infected larvae was confirmed by allowing part of these larvae to feed on guinea pigs, which became ill and seroconverted to R. rickettsii (guinea pigs G.P.74 in Supplementary Table S5, and G.P.102 in Supplementary Table S7). Moreover, NE-PIS F2 nymphs (molted from the engorged larvae that had fed on G.P.102) were also able to transmit R. rickettsii to guinea pig G.P.103 (Tables 3 and Supplementary Table S7).
Rickettsial Infection in GL + N Ticks
The GL + N ticks consisted of F2 uninfected ticks that were dually exposed to R. rickettsii by feeding on rickettsemic guinea pigs during both larval and nymphal feeding, and thereafter, reared until F3 larvae or nymphs by feeding on susceptible hosts. After F2 acquisition nymphal feeding, rickettsial infection rates of F2 nymphs (transstadial perpetuation of R. rickettsii from larvae to nymphs) were observed by DNA detection in ticks of four tick colonies, 41% for E-PAM, 16% for NE-GSV, 10% for E-PIC, and 8% for E-ITU ticks (Table 2). After the second acquisition feeding (F2 nymphs on rickettsemic guinea pigs), rickettsial infection rates of F2 adults (transstadial perpetuation of R. rickettsii from nymphs to adults) were observed in ticks of five out of the six tick colonies, 67% for NE-PIS, 60% for E-ITU, 33% for E-PAM, 23% for NE-GSV, and 20% for NE-POC ticks; rickettsial infection was not detected in any of the E-PIC adult ticks (Table 2). Infestation of these F2 adults on susceptible rabbits resulted in successful rickettsial transmission (fever followed by death or seroconversion with high endpoint titers) in all three rabbits exposed to either E-ITU or NE-POC ticks, two out of three rabbits exposed to either NE-PIS or NE-GSV ticks, and one out of three rabbits exposed to E-PIC adults. Rickettsial transmission was not observed in any of the two rabbits exposed to either E-PIC or NE-POC, and in the one exposed to E-PAM ticks (Table 3). Transovarial transmission of R. rickettsii was observed in engorged females of four colonies, with transovarial transmission rates of 27% for NE-GSV, 20% for E-ITU, 16% for NE-PIS, and 13% for NE-POC. There was no transovarial transmission in E-PIC and E-PAM ticks (Table 2). For the infected females with rickettsial transovarial transmission, the filial infection rates were 30–100% for NE-GSV, 0–100% for E-ITU, 50–100% for NE-PIS, and 100% for NE-POC (Supplementary Table S13). The transovarial acquisition infection of either E-ITU or NE-GSV F2-infected larvae was confirmed by allowing part of these larvae to feed on guinea pigs, which became ill and seroconverted to R. rickettsii (guinea pigs G.P.28 and G.P.29 in Table S2, and G.P.171 and G.P.172 in Table S12). Moreover, E-ITU F2 nymphs (molted from the engorged larvae that had fed on G.P.29) were also able to transmit R. rickettsii to guinea pig G.P.30 (Tables 3 and Supplementary Table S2).
CG Ticks and Additional Molecular Tests
Each of the six tick colonies had its own CG group, which consisted of uninfected ticks that were reared in parallel to each of the infected groups, always exposed to susceptible guinea pigs or rabbits. During all infestations from F1 larvae to F2 adults, all animals remained afebrile and did not seroconvert to R. rickettsii. Moreover, larvae, nymphs and adult ticks were always negative by PCR, indicating absence of rickettsiae in this tick control group (Supplementary Tables S1–S12).
All Rickettsia-negative samples from the six experimental groups yielded amplicons by the PCR assay targeting the tick 16S rRNA gene, indicating that DNA extraction was successful. The only few exceptions, five tick samples that failed to amplify the tick 16S rRNA gene, were discarded from the study. Random samples of two R. rickettsii-infected F1 adult ticks per colony yielded ompA amplicons that generated DNA sequences 100% identical to the sequence of R. rickettsii strain Taiaçu from GenBank (KU321853).
Tick Molting Success and Reproductive Performance
Molting success rates of engorged larvae to nymphs were higher for CG (uninfected ticks) than for GL or GL + N (exposed to R. rickettsii) ticks in all six tick colonies, with statistically significant differences (P < 0.05) in most of the cases (Table 4). A similar trend was observed for engorged nymphs, which displayed significantly higher (P < 0.05) molting success for CG ticks in most of the times when compared to GL, GN or GL + N ticks (Table 4). Hence, exposure to R. rickettsii infection caused higher mortality rates of engorged larvae and nymphs of all six tick colonies.
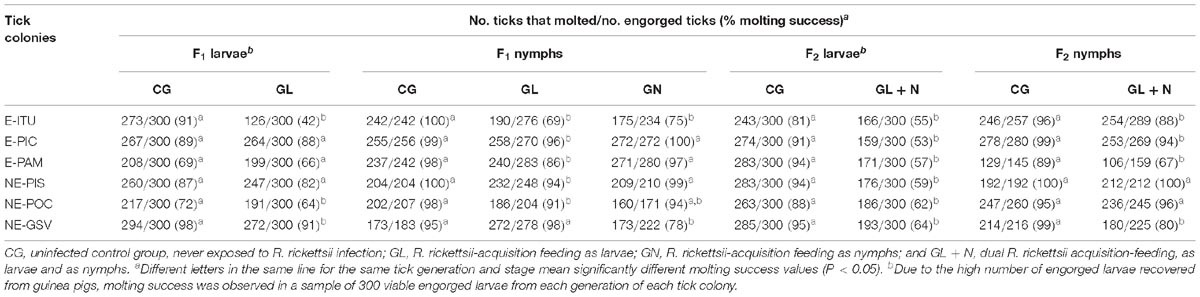
Table 4. Molting success according to Amblyomma sculptum tick generations (F1 or F2), tick stages (larvae or nymph) and experimental groups (CG, GL, GN, and GL + N).
Overall, the reproductive performance of engorged females of the uninfected ticks tended to be higher than those of the R. rickettsii-infected ticks, as demonstrated by higher engorged female weight, egg mass weight, CEI, and % egg hatching values of the uninfected females (Supplementary Tables S14–S19). However, only in a few cases these values were significantly different (P < 0.05) between uninfected and infected females of the same tick group, as for example, the engorged female weight and % egg hatching values in the GL group of E-ITU ticks (Supplementary Table S14), engorged female weight and CEI values in the GL + N group of E-PIC ticks (Supplementary Table S15), the CEI values in the GL group of E-PAM ticks (Supplementary Table S16), and the % egg hatching values in the GL group of NE-PIS ticks (Supplementary Table S17) and the GL + N group of NE-POC ticks (Supplementary Table S18).
Tick Molecular and Phylogenetic Analyses
Regarding the mitochondrial 16S rRNA gene, we generated partial sequences from a total of 98 R. rickettsii-infected ticks (4–43 sequences from each of the six colonies) and from 138 uninfected ticks (15–43 ticks from each of the six colonies) (Supplementary Table S20). Ticks of each colony yielded a single haplotype, except for the E-PAM ticks, which yielded two distinct haplotypes (A and B). E-PIC and NE-PIS ticks shared the same haplotype, as also did NE-GSV and NE-POC ticks, and also E-ITU and part of the E-PAM ticks; the other part of E-PAM yielded a unique haplotype (Supplementary Table S21). Despite these inter-colony differences, R. rickettsii-infected and uninfected ticks of the same colony shared a single haplotype. Even among E-PAM ticks (the only colony that generated more than one haplotype), each of the two haplotypes were shared by both infected and uninfected ticks. Differences among the seven haplotypes of A. sculptum ranged from 0% (between the above identical haplotypes) to 3.3% [between E-PAM (haplotype B) and either NE-PIS or E-PIC]. E-PAM haplotypes A and B differed by 3% (Supplementary Table S21). The phylogenetic tree containing these seven 16S rRNA haplotypes shows two major clusters, one containing E-ITU, E-PAM (haplotype A), E-PIC and NE-PIS ticks, which segregated under high bootstrap support (96%) from the other clade that contains NE-GSV, NE-POC, and E-PAM (haplotype B) (Figure 2).
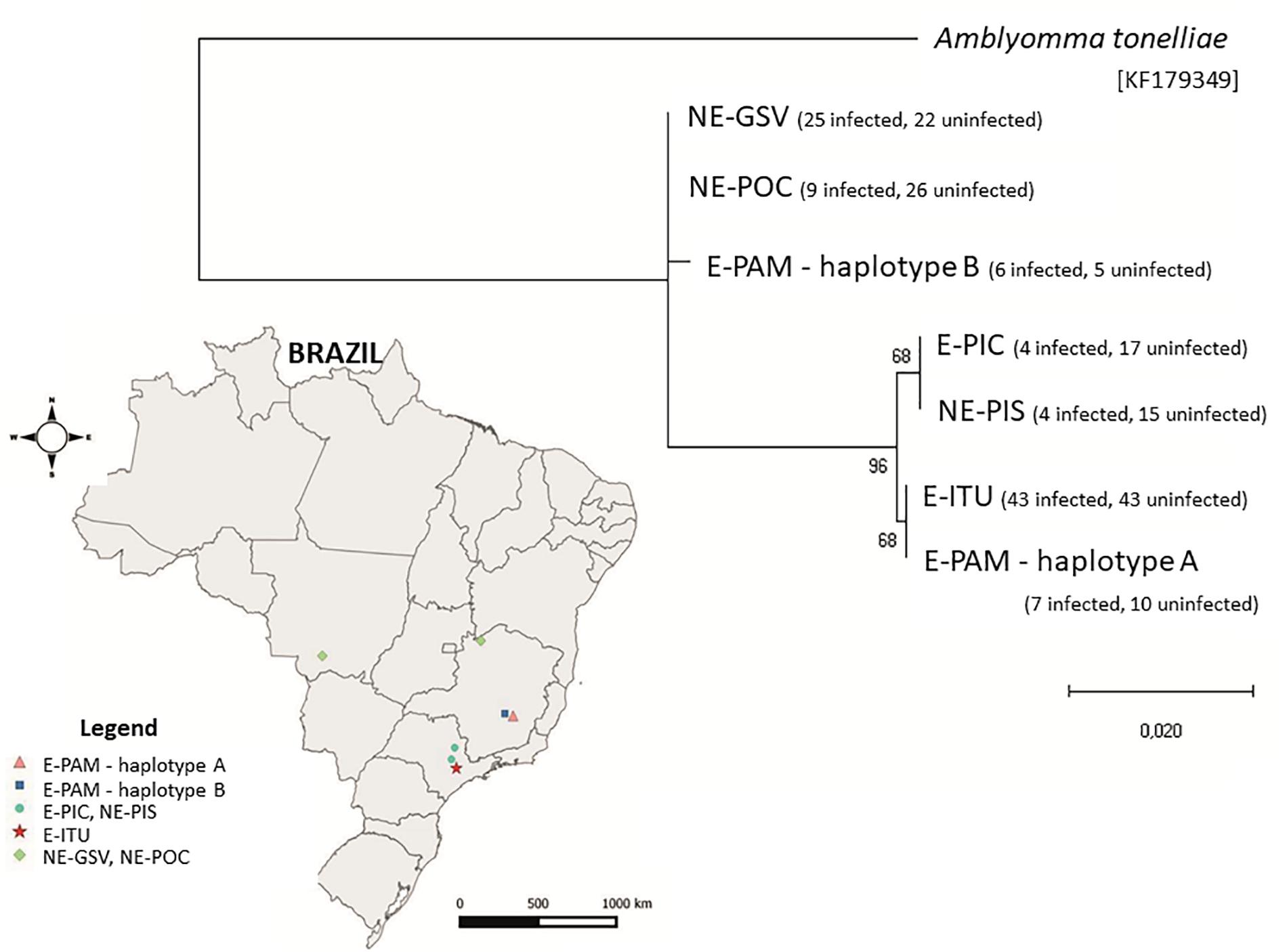
Figure 2. Maximum likelihood tree based on the Tamura 3-parameter model for the 16S rRNA mitochondrial gene partial nucleotide sequences of six tick colonies (E-ITU, E-PIC, E-PAM, NE-PIS, NE-POC, and NE-GSV) of Amblyomma sculptum (410 sites on the dataset). The sequence of Amblyomma tonelliae from Paraguay (KF179349) was used as outgroup. The numbers at each node are bootstrap values greater than 60% from 1,000 replicates. The tree is drawn to scale, with branch lengths measured in the number of substitutions per site. The map of Brazil indicates the geographical location of each of the seven A. sculptum haplotypes from the tree. The numbers of sequences obtained for infected and uninfected ticks for each haplotype are shown within parentheses.
Regarding the nuclear ITS2 nuclear gene, we generated partial sequences of 557-bp from a total of 77 R. rickettsii-infected ticks (3–40 sequences from each of the six colonies) and from 112 uninfected ticks (8–40 ticks from each of the six colonies). All these sequences were identical to each other, and when submitted to BLAST analysis, they were 100% identical to several A. sculptum sequences from Brazil (KU169885-KU169888).
Discussion
Exposure of ticks of six geographically different populations of A. sculptum to R. rickettsii acquisition feeding and transmission revealed different susceptibilities among the six tick populations. After larval acquisition feeding (GL ticks), these differences were clearly in favor of higher infection rates for the two tick colonies that were exposed to their autochthone R. rickettsii strain, namely strain Itu for E-ITU ticks, and strain Pampulha for E-PAM ticks. Rickettsial infection rates in F1 unfed nymphs of these two colonies were significantly higher than those observed in the other four tick colonies, which were exposed to R. rickettsii strain Itu. These differences among GL ticks were even more remarkable for the F1 adult stage, as shown by 33 and 47% rickettsial detection in unfed adults of E-ITU and E-PAM ticks, in contrast to 0% for the remaining tick colonies (Table 2). The same trend was somewhat observed when R. rickettsii acquisition feeding occurred during the nymphal stage (GN ticks) or during both larval and nymphal feeding (GL + GN ticks), as shown by generally higher rickettsial infection rates among E-PAM and E-ITU ticks. While comparable infection rates were sometimes observed in ticks of the other colonies, it is noteworthy that 0% infection rates were observed several times for E-PIC, NE-PIS, NE-POC and NE-GSV ticks. In addition, highest rates of transovarial transmission of R. rickettsii also included E-PAM and E-ITU ticks, while 0% rates were more frequent on the other tick colonies (Table 2). These results were corroborated by the vector competence infestations, which resulted in more events of successful transmission of R. rickettsii when susceptible hosts were infested by E-PAM and E-ITU ticks; i.e., 70–100% successful transmission versus 10–67% (Table 3). All together, these results indicate that the susceptibility of A. sculptum to R. rickettsii varies among different tick populations, with a clear bias for higher susceptibility to an autochthone R. rickettsii strain that has already coevolved with a tick population for some time.
Soares et al. (2012) evaluated the susceptibility of an A. sculptum population by an A. aureolatum-derived strain of R. rickettsii, using the same type of protocols of the present study. Their results for the GL group (acquisition feeding during the larva stage) were comparable with our results for the tick colonies that were exposed to a non-autochthone R. rickettsii strain, although their GN group (acquisition feeding during the nymphal stage) displayed infection rates similar or superior to all GN groups of the present study. On the other hand, most of the transovarial acquisition infection-larvae of the study of Soares et al. (2012) failed to transmit R. rickettsii to guinea pigs, contrasting to the high vector competence of the transovarial acquisition infection-larvae of the present study. These results reinforce our above statement that suggests the existence of some adaptive interaction of R. rickettsii strains with its natural tick populations. Interestingly, our molecular analyses of two tick genes (16S rRNA and ITS2) showed that ticks of the two most R. rickettsii-susceptible populations of the present study (E-PAM and E-ITU) shared the same 16S rRNA haplotype (Figure 2), suggesting a genetic role in the tick-R. rickettsii interaction. However, further studies are needed to investigate this interaction more deeply, which could also be affected by tick endosymbionts, as for example, bacteria of the genera Coxiella, which have been reported to infect some A. sculptum (reported as A. cajennense) populations (Machado-Ferreira et al., 2011; Duron et al., 2015).
Regardless of the different susceptibilities of A. sculptum populations to R. rickettsii, Soares et al. (2012) and the present study clearly demonstrated that this tick species is partially refractory to R. rickettsii infection, in conjunction with relatively low transovarial transmission rates, always <50%. In addition, our results demonstrated that the R. rickettsii infection induces higher mortality of engorged larvae and nymphs, and tend to reduce the reproductive fitness of engorged females; this later impairment was also demonstrated by Soares et al. (2012). While these findings have been used to explain the low R. rickettsii-infection rates of A. sculptum under natural conditions, usually <1% (Krawczak et al., 2014; Labruna et al., 2017), they indicate that an A. sculptum population should not be able to sustain a R. rickettsii infection for successive tick generations without the creation of new cohorts of infected ticks via horizontal transmission on vertebrate rickettsemic hosts (amplifying hosts). In this case, capybaras have been incriminated as the main amplifying host of R. rickettsii for A. sculptum ticks in most of the BSF-endemic areas of Brazil (Souza et al., 2009; Polo et al., 2017). Finally, despite the ubiquitous distribution of A. sculptum in southeastern and central-western Brazil (Martins et al., 2016), most of the populations of this tick species are devoid of R. rickettsii infection (Sangioni et al., 2005; Pacheco et al., 2007, 2009). This scenario might be related to two major factors: (i) insufficient numbers of susceptible amplifying hosts; and (ii) lower susceptibilities of many tick populations. While the first factor has been demonstrated by mathematical models (Polo et al., 2017), the second is highlighted by the results observed in the present study.
Data Availability
The raw data supporting the conclusions of this manuscript will be made available by the authors, without undue reservation, to any qualified researcher.
Ethics Statement
This study has been approved by the Institutional Animal Care and Use Committee (IACUC) of the Faculty of Veterinary Medicine of the University of São Paulo (protocols 3104/2013 and 5948070314). Field capture of ticks was authorized by the Brazilian Ministry of the Environment (permit SISBIO Nos. 43259-1 and 11459-1).
Author Contributions
MG and ML designed the experiments. MG, AR-H, LB, and FK performed the experiments. MG, FG, and ML analyzed the data and wrote the manuscript. All authors read and approved the final manuscript.
Funding
This research was supported by the Fundação de Amparo a Pesquisa do Estado de São Paulo (FAPESP Grants 2013/14222-5 and 2013/18046-7).
Conflict of Interest Statement
The authors declare that the research was conducted in the absence of any commercial or financial relationships that could be construed as a potential conflict of interest.
Acknowledgments
We thank João Fábio Soares, Francisco Borges Costa, and Jonas Moraes-Filho for their valuable help during experiments. Special acknowledgment to all animals that involuntarily gave their lives for this study to be concluded.
Supplementary Material
The Supplementary Material for this article can be found online at: https://www.frontiersin.org/articles/10.3389/fphys.2019.00653/full#supplementary-material
Footnotes
References
Barbieri, A. R. M., Szabó, M. P. J., Costa, F. B., Martins, T. F., Soares, H. S., Pascoli, G., et al. (2019). Species richness and seasonal dynamics of ticks with notes on rickettsial infection in a natural park of the cerrado biome in Brazil. Ticks Tick Borne Dis. 10, 442–453. doi: 10.1016/j.ttbdis.2018.12.010
Drummond, R. O., and Whetstone, T. M. (1970). Oviposition of the gulf coast tick. J. Econ. Entomol. 66, 130–133.
Duron, O., Noël, V., McCoy, K. D., Bonazzi, M., Sidi-Boumedine, K., Morel, O., et al. (2015). The recent evolution of a maternally-inherited endosymbiont of ticks led to the emergence of the Q fever pathogen, Coxiella burnetii. PLoS Pathog. 11:e1004892. doi: 10.1371/journal.ppat.1004892
Guedes, E., Leite, R. C., Prata, M. C. A., Pacheco, R. C., Walker, D. H., and Labruna, M. B. (2005). Detection of Rickettsia rickettsii in the tick Amblyomma cajennense in a new Brazilian spotted fever-endemic area in the state of minas gerais. Mem. Inst. Oswaldo Cruz 100, 841–845. doi: 10.1590/s0074-02762005000800004
Guedes, E., Leite, R. C., Pacheco, R. C., Silveira, I., and Labruna, M. B. (2011). Rickettsia species infecting Amblyomma ticks from an area endemic for Brazilian spotted fever in Brazil. Rev. Bras. Parasitol. Vet. 20, 308–311. doi: 10.1590/s1984-29612011000400009
Guglielmone, A. A., Beati, L., Barros-Battesti, D. M., Labruna, M. B., Nava, S., Venzal, J. M., et al. (2006). Ticks (Ixodidae) on humans in South America. Exp. Appl. Acarol. 40, 83–100. doi: 10.1007/s10493-006-9027-0
Hall, T. A. (1999). BioEdit: a user-friendly biological sequence alignment editor and analysis program for Windows 95/98/NT. Nucleic Acids Symp. Ser. 41, 95–98.
Horta, M. C., Pinter, A., Cortez, A., Soares, R. M., Gennari, S. M., Schumaker, T. T. S., et al. (2005). Rickettsia felis (Rickettsiales: Rickettsiaceae) in Ctenocephalides felis felis (Siphonaptera: Pulicidae) in the state of São Paulo, Brazil. Arq. Bras. Med. Vet. Zootec. 57, 321–325. doi: 10.1590/s0102-09352005000300008
Horta, M. C., Labruna, M. B., Pinter, A., Linardi, P. M., and Schumaker, T. T. (2007). Rickettsia infection in five areas of the state of São Paulo, Brazil. Mem. Inst. Oswaldo Cruz 102, 793–801. doi: 10.1590/s0074-02762007000700003
Horta, M. C., Moraes-Filho, J., Casagrande, R. A., Saito, T. B., Rosal, S. C., Martins, T. F., et al. (2009). Experimental infection of opossums Didelphis aurita by Rickettsia rickettsii and evaluation of the transmission of the infection to ticks Amblyomma cajennense. Vector Borne Zoonotic Dis. 9, 109–118. doi: 10.1089/vbz.2008.0114
Krawczak, F. S., Nieri-Bastos, F. A., Nunes, F. P., Soares, J. F., Moraes-Filho, J., and Labruna, M. B. (2014). Rickettsial infection in Amblyomma cajennense ticks and capybaras (Hydrochoerus hydrochaeris) in a Brazilian spotted fever-endemic area. Parasit. Vectors 7:7. doi: 10.1186/1756-3305-7-7
Kumar, S., Stecher, G., Li, M., Knyaz, C., and Tamura, K. (2018). MEGA X. Molecular evolutionary genetics analysis across computing platforms. Mol. Biol. Evol. 35, 1547–1549. doi: 10.1093/molbev/msy096
Labruna, M. B., Leite, R. C., Faccini, J. L. H., and Ferreira, F. (2000). Life-cycle of the tick Haemaphysalis leporis-palustris (Acari: Ixodidae) under laboratory conditions. Exp. Appl. Acarol. 24, 683–694.
Labruna, M. B., Whitworth, T., Horta, M. C., Bouyer, D. H., McBride, J. W., Pinter, A., et al. (2004). Rickettsia species infecting Amblyomma cooperi ticks from an area in the state of São Paulo, Brazil, where Brazilian spotted fever is endemic. J. Clin. Microbiol. 42, 90–98. doi: 10.1128/jcm.42.1.90-98.2004
Labruna, M. B., Horta, M. C., Aguiar, D. M., Cavalcante, G. T., Pinter, A., Gennari, S. M., et al. (2007). Prevalence of Rickettsia infection in dogs from the urban and rural areas of monte negro municipality, western Amazon, Brazil. Vector Borne Zoonotic Dis. 7, 249–255. doi: 10.1089/vbz.2006.0621
Labruna, M. B., Ogrzewalska, M., Martins, T. F., Pinter, A., and Horta, M. C. (2008). Comparative susceptibility of larval stages of Amblyomma aureolatum, Amblyomma cajennense, and Rhipicephalus sanguineus to infection by Rickettsia rickettsii. J. Med. Entomol. 45, 1156–1159. doi: 10.1093/jmedent/45.6.1156
Labruna, M. B., Ogrzewalska, M., Soares, J. F., Martins, T. F., Soares, H. S., Moraes-Filho, J., et al. (2011). Experimental infection of Amblyomma aureolatum ticks with Rickettsia rickettsii. Emerg. Infect. Dis. 17, 829–834. doi: 10.3201/eid1705.101524
Labruna, M. B., Santos, F. C., Ogrzewalska, M., Nascimento, E. M., Colombo, S., Marcili, A., et al. (2014). Genetic identification of rickettsial isolates from fatal cases of Brazilian spotted fever and comparison with Rickettsia rickettsii isolates from the American continents. J. Clin. Microbiol. 52, 3788–3791. doi: 10.1128/JCM.01914-14
Labruna, M. B., Krawczak, F. S., Gerardi, M., Binder, L. C., Barbieri, A. R. M., Paz, G. F., et al. (2017). Isolation of Rickettsia rickettsii from the tick Amblyomma sculptum from a Brazilian spotted fever–endemic area in the Pampulha Lake region, southeastern Brazil. Vet. Parasitol. Reg. Stud. Rep. 8, 82–85. doi: 10.1016/j.vprsr.2017.02.007
Machado-Ferreira, E., Dietrich, G., Hojgaard, A., Levin, M., Piesman, J., Zeidner, N. S., et al. (2011). Coxiella symbionts in the Cayenne tick Amblyomma cajennense. Microb. Ecol. 62, 134–142. doi: 10.1007/s00248-011-9868-x
Mangold, A. J., Bargues, M. D., and Mas-Coma, S. (1998). Mitochondrial 16SrDNA sequences and phylogenetic relationships of species of Rhipicephalus and other tick genera among Metastriata (Acari: Ixodidae). Parasitol. Res. 84, 478–484. doi: 10.1007/s004360050433
Marrelli, M. T., Souza, L. F., Marques, R. C., Labruna, M. B., Matioli, S. R., Tonon, A. P., et al. (2007). Taxonomic and phylogenetic relationships between neotropical species of ticks from genus Amblyomma (Acari: Ixodidae) inferred from second internal transcribed spacer sequences of rDNA. J. Med. Entomol. 44, 222–228. doi: 10.1603/0022-2585(2007)44
Martins, T. F., Barbieri, A. R., Costa, F. B., Terassini, F. A., Camargo, L. M., Peterka, C. R., et al. (2016). Geographical distribution of Amblyomma cajennense (sensu lato) ticks (Parasitiformes: Ixodidae) in Brazil, with description of the nymph of A. cajennense (sensu stricto). Parasit. Vectors 9:186. doi: 10.1186/s13071-016-1460-2
Melo, A. L., Witter, R., Martins, T. F., Pacheco, T. A., Alves, A. S., Chitarra, C. S., et al. (2016). A survey of tick-borne pathogens in dogs and their ticks in the Pantanal biome, Brazil. Med. Vet. Entomol. 30, 112–116. doi: 10.1111/mve.12139
Monteiro, J. L. (1931). Estudos sobre o typho exanthematico de S. Paulo. Mem. Inst. Butantan 6, 5–135.
Monteiro, J. L. (1933). Comportamento experimental do Coelho aos virus do typho Exanthemático de São Paulo e da febre maculosa das Montanhas Rochosas. Mem. Inst. Butantan 8, 3–80.
Ogrzewalska, M., Saraiva, D. G., Moraes-Filho, J., Martins, T. F., Costa, F. B., Pinter, A., et al. (2012). Epidemiology of brazilian spotted fever in the atlantic forest, state of São Paulo, Brazil. Parasitology 139, 1283–1300. doi: 10.1017/s0031182012000546
Oliveira, S., Guimarães, J., Reckziegel, G., Costa, B., Araújo-Vilges, K., Fonseca, L., et al. (2016). An update on the epidemiological situation of spotted fever in Brazil. J. Venom. Anim. Toxins Incl. Trop. Dis. 22:22. doi: 10.1186/s40409-016-0077-4
Pacheco, R. C., Horta, M. C., Moraes-Filho, J., Ataliba, A. C., Pinter, A., and Labruna, M. B. (2007). Rickettsial infection in capybaras (Hydrochoerus hydrochaeris) from São Paulo, Brazil: serological evidence for infection by Rickettsia bellii and Rickettsia parkeri. Biomedica 27, 364–371.
Pacheco, R. C., Horta, M. C., Pinter, A., Moraes-Filho, J., Martins, T. F., Nardi, M. S., et al. (2009). Pesquisa de Rickettsia spp. em carrapatos Amblyomma cajennense e Amblyomma dubitatum no Estado de São Paulo. Rev. Soc. Bras. Med. Trop. 42, 351–353. doi: 10.1590/s0037-86822009000300023
Pacheco, R. C., Moraes-Filho, J., Guedes, E., Silveira, I., Richtzenhain, L. J., Leite, R. C., et al. (2011). Rickettsial infections of dogs, horses and ticks in Juiz de Fora, southeastern Brazil, and isolation of Rickettsia rickettsii from Rhipicephalus sanguineus ticks. Med. Vet. Entomol. 25, 148–155. doi: 10.1111/j.1365-2915.2010.00915.x
Perez, C. A., Almeida, A. F., Almeida, A., Carvalho, V. H., Balestrin, D. C., Guimarães, M. S., et al. (2008). Ticks of genus Amblyomma (Acari: Ixodidae) and their relationship with hosts in endemic area for spotted fever in the State of São Paulo. Rev. Bras. Parasitol. Vet. 17, 210–217.
Pinter, A., Franca, A., Souza, C., Sabbo, C., Mendes, E., Pereira, F., et al. (2011). Febre maculosa brasileira. BEPA Suplemento 8, 19–24.
Pinter, A., Labruna, M. B., and Faccini, J. L. (2002). The sex ratio of Amblyomma cajennense (Acari: Ixodidae) with notes on the male feeding period in the laboratory. Vet. Parasitol. 105, 79–88. doi: 10.1016/S0304-4017(01)00650-1
Polo, G., Mera Acosta, C., Labruna, M. B., and Ferreira, F. (2017). Transmission dynamics and control of Rickettsia rickettsii in populations of Hydrochoerus hydrochaeris and Amblyomma sculptum. PLoS Negl. Trop. Dis. 11:e0005613. doi: 10.1371/journal.pntd.0005613
Ramos, V. N., Osava, C. F., Piovezan, U., and Szabó, M. P. (2014). Ticks on humans in the pantanal wetlands, Brazil. Ticks Tick Borne Dis. 5, 497–499. doi: 10.1016/j.ttbdis.2014.03.004
Regnery, R. L., Spruill, C. L., and Plikaytis, B. D. (1991). Genotypic identification of rickettsiae and estimation of intraspecies sequence divergence for portions of two rickettsial genes. J. Bacteriol. 173, 1576–1589. doi: 10.1128/jb.173.5.1576-1589.1991
Sangioni, L. A., Horta, M. C., Vianna, M. C. B., Gennari, S. M., Soares, R. S., Galvão, M. A. M., et al. (2005). Rickettsial infection in animals and Brazilian spotted fever endemicity. Emerg. Infect. Dis. 11, 265–270. doi: 10.3201/eid1102.040656
Soares, J. F., Soares, H. S., Barbieri, A. M., and Labruna, M. B. (2012). Experimental infection of the tick Amblyomma cajennense, Cayenne tick, with Rickettsia rickettsii, the agent of Rocky Mountain spotted fever. Med. Vet. Entomol. 26, 139–151. doi: 10.1111/j.1365-2915.2011.00982.x
Souza, C. E., Moraes-Filho, J., Ogrzewalska, M., Uchoa, F. C., Horta, M. C., Souza, S. S., et al. (2009). Experimental infection of capybaras Hydrochoerus hydrochaeris by Rickettsia rickettsii and evaluation of the transmission of the infection to ticks Amblyomma cajennense. Vet. Parasitol. 161, 116–121. doi: 10.1016/j.vetpar.2008.12.010
Szabó, M. P., Labruna, M. B., Garcia, M. V., Pinter, A., Castagnolli, K. C., Pacheco, R. C., et al. (2009). Ecological aspects of the free-living ticks (Acari: Ixodidae) on animal trails within Atlantic rainforest in south-eastern Brazil. Ann. Trop. Med. Parasitol. 103, 57–72. doi: 10.1179/136485909X384956
Terassini, F. A., Barbieri, F. S., Albuquerque, S., Szabó, M. P., Camargo, L. M., and Labruna, M. B. (2010). Comparison of two methods for collecting free-living ticks in the Amazonian forest. Ticks Tick Borne Dis. 1, 194–196. doi: 10.1016/j.ttbdis.2010.08.002
Keywords: spotted fever group Rickettsia, experimental infection, vector competence, transovarial transmission, transstadial maintenance
Citation: Gerardi M, Ramírez-Hernández A, Binder LC, Krawczak FS, Gregori F and Labruna MB (2019) Comparative Susceptibility of Different Populations of Amblyomma sculptum to Rickettsia rickettsii. Front. Physiol. 10:653. doi: 10.3389/fphys.2019.00653
Received: 02 April 2019; Accepted: 09 May 2019;
Published: 28 May 2019.
Edited by:
Itabajara Da Silva Vaz Jr., Federal University of Rio Grande do Sul, BrazilReviewed by:
Ben J. Mans, Agricultural Research Council, South AfricaJosé M. Venzal, Universidad de la República, Uruguay
Copyright © 2019 Gerardi, Ramírez-Hernández, Binder, Krawczak, Gregori and Labruna. This is an open-access article distributed under the terms of the Creative Commons Attribution License (CC BY). The use, distribution or reproduction in other forums is permitted, provided the original author(s) and the copyright owner(s) are credited and that the original publication in this journal is cited, in accordance with accepted academic practice. No use, distribution or reproduction is permitted which does not comply with these terms.
*Correspondence: Marcelo B. Labruna, bGFicnVuYUB1c3AuYnI=
†Present address: Felipe S. Krawczak, Setor de Medicina Veterinária Preventiva, Escola de Veterinária e Zootecnia, Universidade Federal de Goiás, Goiânia, Brazil