- 1Applied Physiology Research Laboratory, School of Science and Sport, Institute of Clinical Exercise and Health Sciences, University of the West of Scotland, Hamilton, Scotland
- 2Kilgore Academy, Azle, TX, United States
- 3Department of Athletic Training and Exercise Physiology, Midwestern State University, Wichita Falls, TX, United States
- 4Université Clermont Auvergne, CNRS, LaPSCo, Physiological and Psychosocial Stress, University Hospital of Clermont–Ferrand, CHU Clermont–Ferrand, Preventive and Occupational Medicine, WittyFit, Clermont–Ferrand, France
- 5Faculty of Health, School of Exercise Science, Australian Catholic University, Melbourne, VIC, Australia
- 6Space Medicine Team, European Astronaut Centre (EAC), European Space Agency, Cologne, Germany
- 7KBR, Cologne, Germany
Background: Recommendations on resistance training (RT) set-volume protocols in preparation for spaceflight muscular strength conditioning remains equivocal. A meta-analysis was performed on the effects of single-set (S), or three-set (M3) RT on muscular strength per exercise for different body segments and joint types (multi-joint and single-joint).
Methods: Computerized searches were performed on PubMed, MEDLINE and SPORTDiscus™. Twelve studies were considered appropriate according to pre-set eligibility criteria. Outcomes analyzed were pre-to-post-muscular strength change on; multi-joint and single-joint combined; upper body only; lower body only; multi-joint exercises only; single-joint exercises only.
Results: Upper body exercise analysis on combined subjects and untrained subjects only reported greater but not significant strength gains with M3 (ES 0.37; 95% CI 0.09–0.82; P = 0.11 and ES 0.35; 95% CI−0.49 to 1.19; P = 0.42). Trained only subjects reported superior strength gains with M3 (ES 0.63; 95% CI 0.34–0.92; P = <0.0001). Lower body exercise on combined subjects and untrained subjects only reported superior strength gains with M3 (ES 0.35; 95% CI 0.10–0.60; P = 0.006 and ES 0.49; 95% CI 0.14–0.83; P = 0.005). Trained subjects only observed greater but not significant strength gains with M3 (ES 0.18; 95% CI −0.23 to 0.58; P = 0.39). Multi-joint exercise on combined subjects reported greater strength gains with M3 (ES 0.83; 95% CI 0.14–1.51; P = 0.02). Trained only subjects reported greater strength gains with M3 (ES 0.52; 95% CI 0.10–0.94; P = 0.02). Single-joint exercise on combined subjects and untrained only observed greater strength gains for M3 (ES 0.49; 95% CI 0.26–0.72; P = <0.0001 and ES 0.56; 95% CI 0.21–0.91; P = 0.002). Trained only subjects reported greater but not significant strength gains with M3 (ES 0.37; 95% CI −0.01 to 0.75; P = 0.06).
Conclusion: For astronauts in space-flight preparation, the findings suggest that M3 training appears to be preferable over S for developing muscular strength. Nevertheless, depending on the physical conditioning of the crew member or tight pre-flight scheduling, S is still able to provide a positive strength training stimulus.
Introduction
Recent advances in space technology, space medicine and collaboration among international space agencies, have contributed significantly toward sending humans deeper into interplanetary space. It is predicted that future crewed missions will focused on deeper space transit; however, at present, this has not transpired due to the significant demands placed on the human body. Governments and space agencies, however, are determined to achieve long duration space exploration and for this to be achieved the astronauts in-flight physical conditioning must be optimal for mission functionality. Astronauts, as part of pre-flight preparation, follow appropriate resistance training (RT) protocols that prepare them for microgravity (μG) environments. Currently, astronauts live and work in extreme environments but significant differences between low-earth orbit operations and exploring interplanetary space exist. Astronauts presently perform low-earth orbit operations in extreme environments including μG, confinement, radiation exposure, and social isolation. These extreme conditions significantly alter the physiological demands experienced by International Space Station (ISS) astronauts relative to terrestrial dwelling. Spaceflight poses unique physiological deconditioning and maladaptation due to prolonged exposure to μG, including significant muscle degradation and impaired skeletal functioning (Convertino, 1990; Stein, 2013; Bloomberg et al., 2016).
Exposure to a μG environment has been shown to have significant adverse effects on skeletal muscle tissue including changes in expression of structural, metabolic, and contractile proteins that adjust the function of tissue (LeBlanc et al., 1996; Fitts et al., 2001; Trappe et al., 2001; Adams et al., 2003; Carpenter et al., 2010). A reduction in muscle strength also leads to a reduction in applied mechanical forces to bones that may intensify the loss of bone mineral content that occurs due to the lack of ground reaction forces in a μG environment. As a result, astronauts will be physically weaker with bones more fragile when they land. These extreme effects of μG on muscle tissue in humans raised concerns by the National Aeronautics and Space Administration (NASA) about the structural and functional deconditioning in muscles that led to astronauts having; (1) loss of strength to perform emergency egress when landing in partial μG and; (2) the inability to perform and endure occupational activities in μG which vary in the magnitude of work-related loading and intensity (Adams et al., 2003). Widrick et al. (1999) suggested that exposure to two and a half weeks of μG led to an overall eight per cent reduction in fiber diameter or up to 15% in the cross-sectional area of slow twitch muscle fibers of the human soleus. LeBlanc et al. (2000) reported that during a 17 day mission significant post-flight changes occurred in muscle volume of between three-to-ten per cent in all muscle regions except hamstrings compared to baseline. LeBlanc and colleagues also observed significant decreases in muscle volume of between 5 and 17% in all muscle groups except the neck during Mir missions of 16–28 week durations. In addition, Trappe et al. (2009) reported losses of muscle strength and approximately two per cent muscle volume per month and five per cent in peak muscle power per month. Similarly, Gopalakrishnan et al. (2010) stated that up to four per cent loss of strength at the knee per month and a loss of approximately three per cent in elbow strength per month. This reduction in muscle activity during spaceflight compromises muscle mass and strength and could have significant consequences related to the success of long duration space exploration.
These decremental changes have driven the pursuit of adequate pre-spaceflight physical training protocols and suitable countermeasures, which has included electrical stimulation, artificial gravity, nutritional therapy, pharmacologic, and various forms of exercise interventions (Lang et al., 2017). Convertino and Sandler (1995) state that physical exercise is central to inhibit unloading-induced remodeling of the muscular and skeletal system. However, sustaining muscle and skeletal bone health remains a significant obstacle in human space exploration. Current pre-flight prescription of RT is primarily established from evidence-driven terrestrial RT and experience gained during previous missions. This has led to disparities in the physical conditioning of astronauts as no such established exercise prescription has been employed that would sustain in-flight muscle strength and functioning. Unfortunately, there does not appear to be a collectively accepted method regarding pre-flight RT prescription that all space agencies adhere too in preparation for space transit. With the daily set-volume, resistance loading, exercise type, and training frequency vary from the space agency to space agency.
The European Space Agency strategy for astronaut's pre-flight preparation focus on individualized training approaches that incorporate three stages (Kozlovskaya et al., 1995); (1) adaptation phase that acquaints individuals with ISS exercise hardware; (2) main phase that counteracts physiological adaptation to μG and; (3) preparation for re-entry and terrestrial landing. The RT prescription comprises of both multi-joint and single-joint exercises (squats, deadlifts, bench press, crunches, and heel raises) altering from training session to training session (Hackney et al., 2015). The Japan Aerospace Exploration Agency implements a pre-flight programme that consists of individualized programmes that are related to the anticipated mission tasks that crew members would perform (Loehr et al., 2015). The Canadian Space Agency uses a three-block approach with each stage lasting 4 weeks with set-volume for strength between two-to-five sets (Loehr et al., 2015). NASA implement increased set-volume (MS) with astronauts performing both concentric and eccentric actions that are prescribed by the American College of Sports Medicine (Garber et al., 2011).
Garber et al. (2011) constructed the position statement that provides direction on the prescription of exercise for apparently healthy adults. In the 2011 position statement, multiple-sets are cited for experienced trainees and competitive athletes that are comparable to astronauts' fitness status at the end of pre-flight conditioning. However, Stein (2013) argues that astronauts at their physical peak may have more to lose during in-flight unloading because of μG. Matsumoto et al. (2011) reported that astronauts who performed walking as part of the pre-flight protocol lost less body weight than those that performed intense exercise protocols. It could be debated that if astronaut's pre-flight physical conditioning is in an over-compensated state, they may experience more significant weight loss during spaceflight that may be detrimental. Consequentially, the set-volume training dose needed for astronauts to be in optimal condition requires further investigation. Daily RT set-volume has been an often-contested issue, established from different recommendations that support MS programming. However, in preparation for spaceflight with μG environments, it is perhaps more advantageous to implement S programming in which to develop functional strength that does not facilitate the same level of in-flight deconditioning and weight loss.
Published RT meta-analytical evidence is equivocal on what set-volume elicits superior strength improvements, with disparity existing in the recommendations (Table 1). Several meta-analytical studies have been performed that support the use of multiple-sets (MS) programmes compared to single-set (S) per exercise on untrained and trained subjects (Rhea et al., 2002b, 2003; Peterson et al., 2004; Wolfe et al., 2004; Krieger, 2009; Fröhlich et al., 2010). However, due to the absence of available studies, most meta-analytical evidence is drawn from S and MS (two-eight-sets per exercise) that does not fully quantify a dose-response relationship. Several meta-analyses that support increased set-volume (Rhea et al., 2002b, 2003; Peterson et al., 2004; Wolfe et al., 2004) include small ESs that potentially drifted toward greater set-volume. For example, Wolfe et al. (2004) inferred that athletes should perform eight-sets per muscle group to develop strength. This was established from only six effect sizes (ES) and data obtained came from one study and any conclusions derived concerning the direct impact of eight-sets compared to any other number would be unreliable. Besides, none have provided a specific set number for strength development and have pooled findings from studies that have combined different exercise types to generate ES. This, unfortunately, produces issues with daily RT set-volume recommendations, as most meta-analytical evidence have pooled data from studies that have combined exercise types (multi-joint and single-joint exercises) from different population groups (untrained and trained) utilizing a broad age ranges (18–65).
Although meta-analyses regarding the effects of S vs. MS have been published (Rhea et al., 2002b, 2003; Peterson et al., 2004; Wolfe et al., 2004; Krieger, 2009; Fröhlich et al., 2010), with support given for the application of MS to develop strength or muscular hypertrophy. Disagreement remains regarding the need to perform additional sets for increasing muscular strength. Published critical reviews (Smith and Bruce-Low, 2004; Winett, 2004; Otto and Carpinelli, 2006; Carpinelli, 2012; Fisher, 2012), have examined the validity of published meta-analyses on set-volume, concluding that reported data do not fully support a dose-response relationship between the additional number of sets and strength gains. These reviews identified confounding factors including the presence of low-quality studies, variations in subject characteristics and inconsistencies in experimental designs that generate spurious inferences regarding muscular strength increases.
Currently, no meta-analytical evidence is available that examines the effect of daily set-volume on body segmentations (upper or lower body) or specific joint types (MJ and SJ) on muscle strength change. In the context of pre-flight RT, it is critical that the magnitude of daily RT set-volume is examined to prepare astronauts for space transit. The purpose of this review and meta-analysis, therefore, was four-fold: (1) to re-examine the effects of RT volume (S or M3) of ST on muscular strength per exercise; (2) to determine if specific set-volume (S vs. M3) produce different strength gains when multi-joint exercises are compared with single-joint exercises; (3) to investigate if the magnitude of strength gain differs between multi-joint and single-joint exercises by population group (trained vs. untrained) and body segmentations (upper vs. lower body). The final objective; (4) is to provide a perspective on developing muscular strength that provides recommendations on daily RT set-volume for pre-flight strength development. Based on previous evidence (Rhea et al., 2002b, 2003; Peterson et al., 2004; Wolfe et al., 2004; Krieger, 2009), we hypothesized that there would be superior pre-to-post-training strength gains with M3 RT compared to S.
Methods
Literature Search
This meta-analysis was performed using the recommendations and criteria defined in the Preferred Reporting Items for Systematic Reviews and Meta-Analyses (PRISMA) statement (Liberati et al., 2009). Computer-aided searches were conducted using the following databases: MEDLINE (PubMed), SWETSWISE, EMBASE, and SPORTDiscusTM. The period of search history assessed was inclusive to August 2018. An extensive manual search and cross-referencing of journals, reference lists, was also performed with citations and abstracts from studies published in foreign language journals and scientific conferences were excluded. Descriptive terms and keywords that were used to retrieve studies included: “resistance training and muscular strength,” “resistance training and training volume,” “single vs. multiple-sets,” and “one vs. multiple-sets and muscular strength.” Boolean operators, including AND, OR, and NOT, were used to focus literature searches with literature searches reduced to studies involving humans only.
As a result of systematic computerized database searches, journals were retrieved from 1960 to August 2014 in where S vs. M3 were examined, from different population demographics (trained, untrained, male, and female subjects). After preliminary literature searching, reference lists of articles were screened for additional studies of relevance on muscular strength development. During the first selection round, appropriate study titles were screened for relevance with the inclusion of either resistance training or training volume. In the second selection round, GR, LK, and DB read the abstracts and then selected the article if resistance training for muscle strength was evaluated before and after a minimum RT intervention period of 4-weeks. This minimum time course was chosen due to reports of muscular adaptations in response to RT (Stock et al., 2016). In the third selection round, full articles were read.
Eligibility Criteria
Studies were deemed eligible in this review if they met the following conditions; (a) human subjects free from chronic disease, muscular, or orthopedic injuries, or physical limitations; (b) trained and untrained adult male or female subjects between 18 and 45 years; (c) subject's descriptive characteristics included in the report (height, weight, training status, and training experience); (d) subjects training at least one primary muscle group-pectoralis major, deltoids (anterior, lateral, posterior); bicep brachii, or tricep brachii; latissimus dorsi; quadriceps (vastus medialis, vastus intermedius, vastus lateralis, rectus femoris); hamstrings (bicep femoris, semitendinosus, semimembranosus; (e) at least one performed pre-to-post measure of muscular strength; (f) studies that compared S vs. M3 performing resistance exercise only (active control group); (g) training protocols lasting a minimum of 4-weeks; (h) and appropriate information to calculate training ES. This meta-analysis included both randomized trials (RAN) and randomized control trials (RCTs) that observed the intervention treatments using stratified resistance exercises with S vs. M3. RAN allocation ensures no systematic variances between the intervention groups; however, no control group may influence the assessment of outcomes (Schünemann et al., 2013). RCTs are a more specific method for defining a cause-effect relationship between treatments and outcomes.
Search Strategy
Titles and abstracts of retrieved journal articles were independently evaluated for content relevance by three reviewers (GR, LK, and DB). Abstracts that contained the necessary information regarding the pre-set inclusion and exclusion criteria were retrieved and independently evaluated for full-text eligibility. Potential studies that did not have descriptive data tables but presented pre- to post-primary strength data in the form of figures resulted in extraction using WebPlot-Digitiser (Web Plot Digitiser V.3.11. Texas, USA: Ankit Rohatgi, 2017). Where differences between reviewers (GR, LK, and DB) occurred then additional dialogue and agreements were made by consensus. Ten randomly selected studies underwent post-hoc reassessment with the extracted results compared. For each reviewer coder drift was set at <10% in all cases, and inter-rater (GR and DB) reliability was >95%. Studies were read and individually coded for the following variables; (1) subject's descriptive characteristics, including age, training experience, and sample size; (2) programme characteristics including training frequency, number of sets performed per exercise, the number of reps performed per exercise; (3) measurement of pre-post-strength outcome(s) and; (4) treatment effects of mean (M) and SD values of changes in pre- and post-strength outcomes for RT intervention and control groups.
Assessment of Methodological Quality of Studies
Internal validity of retrieved studies was evaluated using the Physiotherapy Evidence Database (PEDro) scale. The PEDro scale (Verhagen, 1998; Maher et al., 2003) has 11 measures, with a maximum score of ten. However, a maximum score from the PEDro scale, in this case, was eight, as the therapists, assessors and technicians conducting the interventions cannot be blinded. Studies were included in this analysis if they had a PEDro score of ≥ four, as this was considered as having acceptable internal validity. Methodological quality was independently assessed by reviewers (GR, LK, and DB). Variances of judgement concerning the scoring of the journal articles were agreed between reviewers through consensus.
Calculation of Effect Size
Descriptive statistics were calculated to describe and summarize the results of the systematic review process. Data of individual study characteristics were entered into a spreadsheet (Microsoft, Redmond, WA, USA) to compare pre-post-strength outcomes of each study for coding, review and data reference. Descriptive statistics containing sample size (n), mean (M) and SD were extracted from each study. This provided data for the mean differences in pre- to post-intervention between groups (e.g., S and M3) on several strength outcomes. Muscular strength was deemed a continuous data variable; therefore, the standardized mean difference (SMD) with 95% confidence intervals (95% CI) were used to establish the ES measures. For each strength outcome variable, a SD score was calculated by using Cohen's d index of a single ES (di = [M1–M2]/SDpi) (Cohen, 1998), where d = ES, i = individual study, M1 = pre-intervention mean, M2 = post-intervention mean, and SDp = pooled standard deviation. The SD was calculated by summing the extracted pre-intervention and post-intervention SDs and dividing by two. If the standard error of measurement (SEM) of the mean was specified, the SD was calculated using the formula (SD = SEM*square root of N) (Howell, 2012). Separate ES was weighted to account for individual sample sizes. If a study reported, exact P-values for a change of strength, the SD of change was calculated. Studies that did not report, exact P-values, the SD of change was calculated using the pre- and post-intervention SDs. Due to diverse population demographics and methods with the included studies, a random-effects inverse variance (IV) using the DerSimonian-Laird method (DerSimonian and Laird, 1986) was applied with the effects measure of SMD. If a study had numerous time-periods, only the pre- to post-intervention strength outcomes were extracted and entered for analysis. The data was then used to compute ES estimates and CI. For each strength measure, an ES was calculated as the pre- to post-intervention change, divided by the pre-intervention SD (Morris and DeShon, 2002).
Meta-Essentials (Suurmond et al., 2017) was initially used to input pre-post-strength outcome data with each row denoted as an individual ES for a treatment group. If treatment groups had multiple ES, then each ES was coded in a separate row. This aided with the computation of ES, SEM, and study size to allocate appropriate weight to each study, and estimate a study effect. To determine the significance of the ES, the chi-square (Chi2) test was performed in each model used. For the statistical analyses, Review Manager (RevMan) version 5.3.5 was used to calculate the difference in SD of post-intervention strength outcomes and the generation of forest plots. Data needed were either; (1) means and SDs (pre- and post-strength change); (2) CI data for pre- to post-strength change for each treatment group (3) P-values for pre- to post-strength difference for each treatment group, or if only the level of significance was available, and; (4) default P-values (e.g., P ≤ 0.05 becomes P ≤ 0.49, P ≤ 0.01 becomes P ≤ 0.0099, and P ≥ not significant becomes P ≥ 0.05).
The random-effects model was implemented to allow for variability between the studies due to high heterogeneity. A random-effects model conceptualized the existing series of studies under investigation to be a random sample selected from a larger population of studies. In the random-effects model meta-analysis, there are two sources of variability; (1) variability of the effect parameters, and; (2) sampling variability of experimental units (i.e., subjects) into studies. If individual parameter estimates of each study lead to high levels of heterogeneity, the random-effects analysis considers the “true variance” (or the remaining unmeasured random-effects between studies) in addition to the modeled between-study variances and sampling error typically assumed in fixed-effects models. It should be highlighted that the random-effects model typically gives less specific estimates and larger CIs.
Heterogeneity and Risk of Bias
To evaluate heterogeneity between studies, the I-squared (I2) index test and Cochran Q (Q) heterogeneity statistic were applied. The I2 test was used to assess the degree of heterogeneity for each outcome, with an I2 > 50% applied to indicate heterogeneity. Non-significance signifies that the results of the different studies were similar (P ≥ 0.05) and P < 0.05 denotes a statistically significant effect. The Q statistic uses the sum of squared deviations of each estimate resulting from the pooled estimate and weights the contribution of each study. The Q heterogeneity test was applied to evaluate heterogeneity prior to estimating tau-square (Tau2), then I2 and Tau2 statistics were calculated. Comparing the Q statistic with an X2 distribution with k−1 degrees of freedom (where k denotes the number of included studies) allowed P-values to be attained. All analyses were conducted at the 95% confidence level. The ES of ≤ 0.2, ≤ 0.5, ≤ 0.8, and ≥0.8 were considered trivial, small, moderate and large, respectively (Cochran, 1954).
For the assessment and evaluation of publication bias, the use of funnel plot assessments with Duval and Tweedie's (2000) trim and fill correction was applied. The purpose of the “trim and fill” was to identify and correct for funnel plot asymmetry ascending from publication bias. This method is to; (1) remove the smaller studies causing funnel plot asymmetry; (2) apply the trimmed funnel plot to estimate the true “centre” of the funnel, then; (3) replace the removed or omitted studies around the center. Forest plots were produced to display the study-specific ES and the corresponding CI. All forest plots generated were visually examined against its standard error (SE) to account for publication bias also known as the “file drawer problem.” This refers to the influence of the results of a study that introduces bias into the scientific literature by selective publication, primarily by the propensity to publish positive results but not to publish negative results (Scargle, 2000).
Separate subgroup analysis on ES was performed with the resulting moderators, including; (1) single-joint or multi-joint resistance exercise on 1RM strength gains (trained only subjects); (2) single-joint or multi-joint resistance exercise on 1RM strength gains (untrained only subjects). In the subgroup analysis, mean differences in ES were computed for each study to produce a study-level ES for the difference between S and M3 allowing for the generation of forest plots. Sensitivity analysis was performed, by identifying any studies that were highly influential which may bias the analysis. This was achieved for each model by eliminating one study at a time and then inspecting the set-volume predictor. Influential studies were removed if they caused a significant change in the magnitude of the coefficient or change from significant (P ≤ 0.10) to non-significant (P ≥ 0.10) or vice versa.
Results
The procedure used for systematic literature search and retrieval is displayed in Figure 1 from “potentially relevant” to article inclusion. The specific stages of the selection procedure for the meta-analysis are described as a flow diagram (Figure 1).
Study Selection
The initial examination generated 8,418 related abstracts and citations. Thirty-seven full-text articles were initially deemed to meet the inclusion criteria. A total of 19 potentially relevant journal articles met the pre-set inclusion and exclusion criteria (Table 2) and were further assessed for content applicability. Six studies (Kraemer, 1997; Borst et al., 2001; McBride et al., 2003; Galvão and Taaffe, 2005; Munn et al., 2005; Rønnestad et al., 2007) were rejected prior to data extraction, with Galbraith plot identifying one further article (Starkey et al., 1996) as an outlier and was omitted. Descriptions for the seven studies that were excluded are detailed in Table 3.
Resistance Training Study Characteristics
Following appraisal and sensitivity measures 12 full-text articles (Reid et al., 1987; Kraemer, 1997; Hass et al., 2000; Schlumberger et al., 2001; Rhea et al., 2002a; Paulsen et al., 2003; Humburg et al., 2007; Kelly et al., 2007; Bottaro et al., 2009; Baker et al., 2013; Sooneste et al., 2013; Radaelli et al., 2014) met pre-set inclusion criteria (Table 2). Journal articles included in this analysis had dates ranging from 1987 to 2014. In total, 12 studies provided data on 393 subjects (Table 4) with the both randomized control groups (RCT [n = 4]) and random assignment of treatment conditions (RAN [n = 8]) experimental designs included.
The mean age of the subjects was 25.2 (± 5.4 years). The training status of subjects included in the 12 studies was untrained (n = 6) and trained (n = 6). Assigned cohorts consisted of male (n = 8 [67%]), female only groups (n = 1 [12%]), and mixed-sex studies (n = 3 [25%]) which were included in the analysis. The RT period ranged from 6 to 26 weeks (mean = 11.2 [±5.4] weeks), weekly training frequency ranged from 2 to 3 days per week (2.7 [±0.49] per week), and the repetitions used ranged from 3 to 18 repetitions (9.0 [±1.7]) per week. The total number of sets per week ranged from two-to-three-sets (2.7 [±1.7]) for S and six-to-nine-sets for M3 (7.5 [±2.1]) per exercise. Also, training loads ranged from 63 to 90% 1RM (76.7 [±4.1]) with the subject's resistance training characteristics and weekly training volume specified in Table 5.
Sensitivity Analysis
The PEDro scale was based on the Delphi list (Verhagen, 1998) with column 1a not used in the calculation of the scores. Only criterion 2–11 are scored giving a total out of ten. Each column number corresponds to the following criteria on the PEDro scale (Table 6): 1a = eligibility criteria (1a = eligibility criteria specified [1 = yes/0 = no]); 2 = subjects randomly allocated; 3 = allocation was concealed; 4 = groups similar at baseline; 5 = blinded subjects; 6 = therapists blinded; 7 = assessors blinded; 8 = follow-up measures obtained for >85% of subjects; 9 = intention to treat analysis; 10 = between groups statistical comparison; 11 = point measures and measures of variability. The included studies had PEDro scores that ranged from five through to six (Table 6). Though the maximum PEDro score is 11, it is problematic and unrealistic to achieve this total. Realistically the maximum score on PEDro was eight as it is problematic to blind both participants and researchers to an exercise intervention. Consequently, included journal articles had a common area of bias as subjects, therapists or researchers were not blinded. Galbraith plots were used to investigate for study heterogeneity and identification of potential outliers. Examination of Galbraith plots exposed no outliers (Figure 2). To assess for publication bias trim and fill funnel plot were also performed in all comparison models. This was to safeguard for overestimations of the ES of set-volume and strength outcomes in the included studies. The shape of the funnel plot did not expose any evidence of apparent asymmetry.
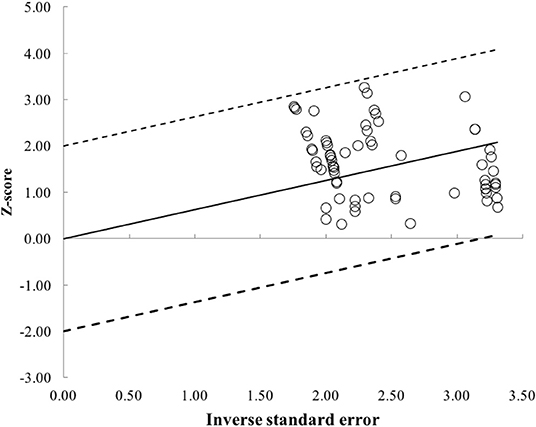
Figure 2. Galbraith plot used to examine study heterogeneity (pre- vs. post-strength change). Each open circle represents one pre- vs. post-study data.
Effects of 1- vs. 3- Sets on Multi-Joint and Single-Joint Exercise
Pre- to post-strength outcomes were assessed via a meta-analytic procedure for all included studies. Subgroup analysis was then performed with multi-joint and single-joint exercises combined into separate subdivision analysis. A random-effects model was incorporated into each strength measure due to the potential of pooled study data generating significant heterogeneity with I2 used to evaluate heterogeneity.
The pooled mean ES estimates (untrained and trained) of multi-joint and single-joint data (Tables 7, 8) comprised of 70 treatment groups from 12 studies (Reid et al., 1987; Kramer et al., 1997; Hass et al., 2000; Schlumberger et al., 2001; Rhea et al., 2002a; Paulsen et al., 2003; Humburg et al., 2007; Kelly et al., 2007; Bottaro et al., 2009; Baker et al., 2013; Sooneste et al., 2013; Radaelli et al., 2014). The random-effects model exposed a considerable amount of variability between studies. Heterogeneity prior to taking Tau2 into consideration (Q heterogeneity test) was: Chi2 = 131.56, d.f. = 11, P < 0.00001. The heterogeneity statistic I2 (%) = 92 [interpreted as high, (Higgins et al., 2003)], and the tau2 test (between-trials variance) = 0.74. When a random effect analysis was implemented, a large effect was detected for combined multi-joint and single-joint exercises on RT set training volume [mean effect size (ES) 0.93; 95% CI 0.41–1.45]. Pre- to post-intervention strength change was greater with M3 compared to S (ES difference 0.25) with the effect statistically significant (P = 0.0005). The mean for S was 0.64 (95% CI 0.44–0.84). The mean ES for M3 was 0.89 (95% CI 0.66–1.12).
Untrained Subjects' Effects of Single -vs. Three-Sets on Combined Exercises
Examination of the effects of pre- vs. post-training strength (untrained subjects only) categorized as either S or M3 from six studies (Reid et al., 1987; Paulsen et al., 2003; Humburg et al., 2007; Bottaro et al., 2009; Sooneste et al., 2013; Radaelli et al., 2014). The random-effects model exposed a considerable amount of variability between studies. Q heterogeneity test was: Chi2 = 78.30, d.f. = 5, P < 0.00001. The heterogeneity statistic I2 (%) = 94 [interpreted as high, (Higgins et al., 2003)], and the tau2 test (between-trials variance) = 0.94. A large effect was observed on untrained only subjects for multi-joint and single-joint exercises combined (ES 1.20; 95% CI 0.39–2.01). Pre- to post-intervention strength change was greater when M3 was compared to S (ES difference 0.22) with statistical significance (P = 0.004). The mean for S was 0.60 (95% CI 0.34–0.85). The mean ES for M3 was 0.82 (95% CI 0.52–1.11).
Trained Subjects' Effects of Single -vs. Three-Sets on Combined Exercises
Separate subgroup examination on the effects of pre- vs. post-training strength (trained subjects only) categorized as either S or M3 from six studies (Kramer et al., 1997; Hass et al., 2000; Schlumberger et al., 2001; Rhea et al., 2002a; Kelly et al., 2007; Baker et al., 2013). The random-effects model exposed a considerable amount of variability between studies. Q heterogeneity test was: Chi2 = 29.21, d.f. = 5, P < 0.0001. The heterogeneity statistic I2 (%) = 83 (interpreted as high; Higgins et al., 2003), and the tau2 test (between-trials variance) = 0.42. A moderate effect was observed on trained only subjects for multi-joint and single-joint exercises combined (ES 0.63; 95% CI 0.05–1.22). Pre- to post-intervention strength gain was greater when M3 was compared to S (ES difference 0.28) with statistical significance (P = 0.03). The mean for S was 0.68 (95% CI 0.35–1.01). The mean ES for M3 was 0.96 (95% CI 0.61–1.31).
Effects of 1-vs. 3-Sets on Upper Body Exercise
Examination of upper body exercises (multi-joint and single-joint exercises combined) comprised 10 studies (Reid et al., 1987; Hass et al., 2000; Schlumberger et al., 2001; Rhea et al., 2002a; Paulsen et al., 2003; Humburg et al., 2007; Bottaro et al., 2009; Baker et al., 2013; Sooneste et al., 2013; Radaelli et al., 2014) are displayed in the forest plot (Figure 3). The random-effects model exposed a large amount of variability between studies (I2 = 93%). Removal of Humburg et al. (2007) and Radaelli et al. (2014) data, resulted in moderate heterogeneity (Q heterogeneity test was: Chi2 = 21.71, d.f. = 7, P = 0.003). The heterogeneity statistic I2 (%) = 68 (interpreted as moderate heterogeneity, Higgins et al., 2003), and the tau2 test (between-trials variance) = 0.27, and a small effect was observed (ES 0.37; 95% CI −0.09 to 0.82). Pre- to post-intervention strength change was greater when M3 was compared with S (ES difference 0.19) with no statistical significance (P = 0.11). The mean ES for S was 0.68 (95% CI 0.42–0.94). The mean ES for M3 was 0.87 (95% CI 0.61–1.14).
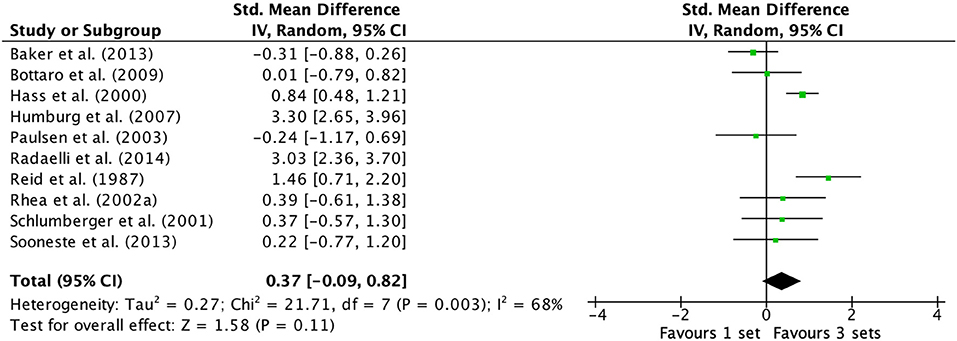
Figure 3. Forest plot of 1 vs. 3-sets (trained and untrained subjects [upper body exercise] combined) with Humburg et al. (2007) and Radaelli et al. (2014) datum excluded.
Untrained Subjects' Effects of 1- vs. 3-Sets on Upper Body Exercise
Examination of upper body exercises (multi-joint and single-joint exercises combined) on untrained subjects comprised of six studies (Reid et al., 1987; Paulsen et al., 2003; Humburg et al., 2007; Bottaro et al., 2009; Sooneste et al., 2013; Radaelli et al., 2014). The random-effects model exposed a large amount of variability between studies (I2 = 94%). Removal of Humburg et al. (2007) and Radaelli et al. (2014) data, resulted in large heterogeneity (Q heterogeneity test was: Chi2 = 11.45, d.f. = 3, P = 0.010). The heterogeneity statistic I2 (%) = 74 (interpreted as high heterogeneity, Higgins et al., 2003), and the tau2 test (between-trials variance) = 0.54, and a small effect was observed (ES 0.35; 95% CI −0.49 to 1.19). Pre- to post-intervention strength change was greater when M3 was compared with S (ES difference 0.23) with no statistical significance (P = 0.42). The mean ES for S was 0.81 (95% CI 0.67–0.95). The mean ES for M3 was 1.04 (95% CI 0.66–1.41). Subgroup examination of S vs. M3 pre- to post-intervention strength differences on trained only and untrained only subjects was not viable due to inadequate study data.
Trained Subjects' Effects of 1- vs. 3-Sets on Upper Body Exercise
Examination of upper body exercises (multi-joint and single-joint exercises combined) on trained subjects comprised of four studies (Hass et al., 2000; Schlumberger et al., 2001; Rhea et al., 2002a; Baker et al., 2013). Q heterogeneity test was: Chi2 = 3.09, d.f. = 3, P = 0.38. The random-effects model exposed a low amount of variability between studies. The heterogeneity statistic I2 (%) = 3 (interpreted as low, Higgins et al., 2003), and the tau2 test (between-trials variance) = 0.00. When a random effect analysis was implemented, a moderate effect was detected on trained only subjects for upper body exercise resistance exercises (ES 0.63; 95% CI 0.34–0.92). Pre- to post-intervention strength change was greater when M3 was compared to S (ES difference 0.33) with statistical significance (P < 0.0001). The mean for S was 0.59 (95% CI 0.16–1.02). The mean ES for M3 was 0.92 (95% CI 0.53–1.31).
Effects of 1- vs. 3-Sets on Lower Body Exercise
Examination of lower body exercises (multi-joint and single-joint exercises combined) on untrained and trained subjects comprised of 10 studies (Reid et al., 1987; Hass et al., 2000; Schlumberger et al., 2001; Rhea et al., 2002a; Paulsen et al., 2003; Humburg et al., 2007; Kelly et al., 2007; Bottaro et al., 2009; Radaelli et al., 2014) are displayed in the forest plot (Figure 4). Q heterogeneity test was: Chi2 = 54.00, d.f. = 9, P < 0.00001. The random-effects model exposed a high amount of variability between studies I2 (%) = 83. Removal of Hass et al. (2000) and Humburg et al. (2007) data, resulted in no heterogeneity (Q heterogeneity test was: Chi2 = 4.05, d.f. = 7, P = 0.77). The heterogeneity statistic I2 (%) = 0 (interpreted as no heterogeneity, Higgins et al., 2003), and the tau2 test (between-trials variance) = 0.00, and a small effect was observed (ES 0.35; 95% CI 0.10–0.60). Pre- to post-intervention strength change was greater when M3 was compared with S (ES difference 0.27) with statistical significance (P = 0.006). The mean ES for S was 0.60 (95% CI 0.36–0.84). The mean ES for M3 was 0.87 (95% CI 0.57–1.17).
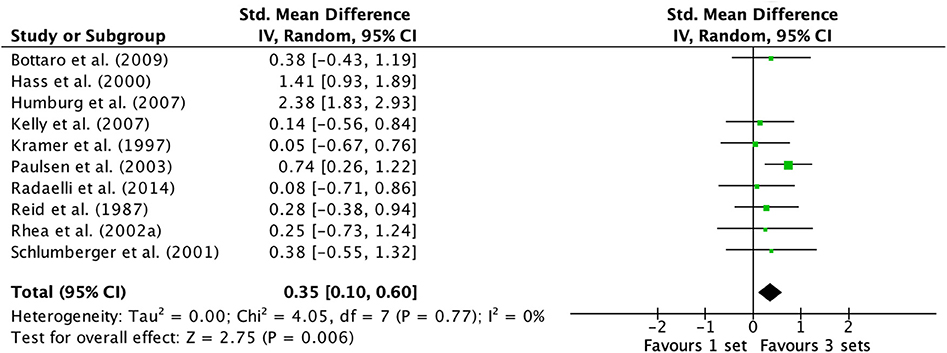
Figure 4. Forest plot of 1 vs. 3-sets (untrained and trained subjects [lower body exercise] combined) with Hass et al. (2000) and Humburg et al. (2007) datum excluded.
Untrained Subjects' Effects of 1- vs. 3-Sets on Lower Body Exercise
Examination of lower body exercises (multi-joint and single-joint exercises combined) on untrained comprised of five studies (Reid et al., 1987; Paulsen et al., 2003; Humburg et al., 2007; Bottaro et al., 2009; Radaelli et al., 2014). Q heterogeneity test was: Chi2 = 34.99, d.f. = 4, P = < 0.00001). The random-effects model exposed a high amount of variability between studies I2 (%) = 89. Removal of Humburg et al. (2007) data, resulted in no heterogeneity (Q heterogeneity test was: Chi2 = 2.36, d.f. = 3, P = 0.50). The heterogeneity statistic I2 (%) = 0 (interpreted as no heterogeneity, Higgins et al., 2003), and the tau2 test (between-trials variance) = 0.00, and a moderate effect was observed (ES 0.49; 95% CI 0.14–0.83). Pre- to post-intervention strength change was greater when M3 was compared with S (ES difference 0.28) with statistical significance (P = 0.005). The mean ES for S was 0.75 (95% CI 0.30–1.2). The mean ES for M3 was 1.03 (95% CI 0.51–1.55).
Trained Subjects' Effects of 1- vs. 3-Sets on Lower Body Exercise
Examination of lower body exercises (multi-joint and single-joint exercises combined) on trained subjects comprised of five studies (Kramer et al., 1997; Hass et al., 2000; Schlumberger et al., 2001; Rhea et al., 2002a; Kelly et al., 2007). Q heterogeneity test was: Chi2 = 15.24, d.f. = 4, P = < 0.004. The random-effects model exposed a high amount of variability between studies I2 (%) = 74. Removal of Hass et al. (2000) data, resulted in no heterogeneity (Q heterogeneity test was: Chi2 = 0.34, d.f. = 3, P-value = 0.95). The heterogeneity statistic I2 (%) = 0 (interpreted as no heterogeneity, Higgins et al., 2003), and the tau2 test (between-trials variance) = 0.00, and a trivial effect was observed (ES 0.18; 95% CI −0.23 to 0.58). Pre- to post-intervention strength change was greater when M3 was compared with S (ES difference 0.32) with no statistical significance (P = 0.39). The mean ES for S was 0.63 (95% CI 0.18–1.08). The mean ES for M3 was 0.95 (95% CI 0.43–1.47).
Effects of 1- vs. 3-Sets on Multi-Joint Only Exercise
Outcomes for S vs. M3 on multi-joint exercise (combined trained and untrained subjects) classified as S or M3 are displayed in the forest plot (Figure 5). The forest plot includes the mean ES and CIs for strength change separated for interventions featuring S and M3 and the overall effect test and heterogeneity analysis. The pooled mean ES estimates of S vs. M3 on multi-joint exercise data comprised of 34 treatment groups from eight studies (Kramer et al., 1997; Hass et al., 2000; Schlumberger et al., 2001; Rhea et al., 2002a; Paulsen et al., 2003; Humburg et al., 2007; Baker et al., 2013; Radaelli et al., 2014). The random-effects model exposed a considerable amount of variability between studies. Q heterogeneity test was: Chi2 = 69.07, d.f. = 7, P c< 0.00001. The heterogeneity statistic I2 (%) = 90 (interpreted as high, Higgins et al., 2003), and the tau2 test (between-trials variance) = 0.86. A moderate effect was observed for multi-joint exercise and M3 (ES 0.83; 95% CI 0.14–1.51). Pre- to post-intervention strength change was greater when M3 was compared to S (ES difference 0.22) with statistical significance (P = 0.02). The mean for S was 0.61 (95% CI 0.34–0.88). The mean ES for M3 was 0.83 (95% CI 0.53–1.13).
Trained Subjects' Effects of 1- vs. 3-Sets on Multi-Joint Only Exercise
Separate subgroup examination on the effects of pre- vs. post-training strength (trained subjects only) categorized as either S or M3 from five studies (Kramer et al., 1997; Hass et al., 2000; Schlumberger et al., 2001; Rhea et al., 2002a; Baker et al., 2013). The random-effects model exposed a low degree of variability between studies. Q heterogeneity test was: Chi2 = 7.73, d.f. = 4, P = 0.10. The heterogeneity statistic I2 (%) = 48 (interpreted as low-moderate, Higgins et al., 2003), and the tau2 test (between-trials variance) = 0.07. A moderate effect was observed on trained only subjects for multi-joint exercises combined (ES 0.52; 95% CI 0.10–0.94). Pre- to post-intervention strength change was greater when M3 was compared to S (ES difference 0.25) with statistical significance (P = 0.02). The mean for S was 0.67 (95% CI 0.30 to 1.04). The mean ES for M3 was 0.92 (95% CI 0.56–1.28). Subgroup analysis of S vs. M3 pre-to post-intervention strength differences on untrained subjects was not feasible due to inadequate study data.
Effects of 1- vs. 3-Sets on Multi-Joint Upper Body Exercise
Subgroup analysis on the effects of pre- vs. post-training strength multi-joint upper body exercises (trained and untrained subjects) categorized as either S or M3 from seven studies (Hass et al., 2000; Schlumberger et al., 2001; Rhea et al., 2002a; Paulsen et al., 2003; Humburg et al., 2007; Baker et al., 2013; Radaelli et al., 2014). The random-effects model exposed a significant amount of variability between studies. Q heterogeneity test was: Chi2 = 70.88, d.f. = 6, P < 0.00001. The heterogeneity statistic I2 (%) = 92 [interpreted as high, (Higgins et al., 2003)] and the tau2 test (between-trials variance) = 1.57. A large effect was observed (ES 1.15; 95% CI 0.17–2.12). Pre- to post-intervention strength change was greater when M3 was compared to S (ES difference 0.24) with statistical significance between RT set volumes (P = 0.02). The mean for S was 0.55 (95% CI 0.23–0.86). The mean ES for M3 was 0.79 (95% CI 0.44–1.14).
Trained Subjects' Effects of 1– vs. 3-Sets on Multi-Joint Upper Body Exercise
Subgroup examination of S vs. M3 pre- vs. post-training strength differences on trained subjects multi-joint upper body exercises comprised of four studies (Hass et al., 2000; Schlumberger et al., 2001; Rhea et al., 2002a; Baker et al., 2013). The random-effects model exposed a low to moderate amount of variability between studies. Q heterogeneity test was: Chi2 = 4.56, d.f. = 3, P = 0.21. The heterogeneity statistic I2 (%) = 34 (interpreted as low-moderate, Higgins et al., 2003), and the tau2 test (between-trials variance) = 0.07. A moderate effect was observed (ES 0.52; 95% CI 0.08–0.96). Pre- to post-intervention strength gain was greater when M3 was compared to S (ES difference 0.32) with statistical significance (P = 0.02). The mean for S was 0.59 (95% CI 0.09–1.08). The mean ES for M3 was 0.91 (95% CI 0.49–1.32). Subgroup examination of S vs. M3 pre- to post-intervention strength differences on untrained subjects was not feasible due to limited study data.
Effects of 1- vs. 3-Sets on Multi-Joint Lower Body Exercise
Analysis of S vs. M3 pre- to post-intervention strength differences on subject's multi-joint lower body exercises comprised of five studies (Kramer et al., 1997; Rhea et al., 2002a; Paulsen et al., 2003; Humburg et al., 2007; Radaelli et al., 2014). The random-effects model showed a significant amount of variability between studies. Removal of (Humburg et al., 2007) data resulted in no heterogeneity (Q heterogeneity test was: Chi2 = 0.13, d.f. = 3, P = 0.99). The heterogeneity statistic I2 (%) = 0 (interpreted as none, Higgins et al., 2003), and the tau2 test (between-trials variance) = 0.00 and trivial effect observed (ES 0.09; 95% CI −0.32 to 0.51). Pre- to post-intervention strength change was greater with M3 compared with S (ES difference 0.17) with no statistical significance (P = 0.66). The mean ES for S was 0.81 (95% CI 0.41–1.21). The mean ES for M3 was 0.98 (95% CI 0.45–1.51). Subgroup examination of S vs. M3 pre- to post-intervention strength differences on trained only and untrained only subjects was not feasible due to inadequate study data.
Effects of 1- vs. 3-Sets on Single-Joint Exercise
Outcomes for 1-vs.-3 sets categorized as S or M3 for single-joint resistance exercises are displayed in the forest plot (Figure 6). The pooled mean ES estimates of single-joint resistance exercises comprised of 36 treatment groups from nine studies (Reid et al., 1987; Hass et al., 2000; Schlumberger et al., 2001; Paulsen et al., 2003; Humburg et al., 2007; Kelly et al., 2007; Bottaro et al., 2009; Baker et al., 2013; Sooneste et al., 2013). The random-effects model exposed a low to moderate amount of variability between studies. Q heterogeneity test was: Chi2 = 10.61, d.f. = 8, P = 0.22. The heterogeneity statistic I2 (%) = 25 (interpreted as low-moderate, Higgins et al., 2003), and the tau2 test (between-trials variance) = 0.03 and a small effect was observed (ES 0.49; 95% CI 0.26–0.72). Pre- to post-intervention strength change was marginally greater with M3 compared with S (ES difference 0.19) with statistical significance (P < 0.0001). The mean ES for S was 0.57 (95% CI 0.26–0.87). The mean ES for M3 was 0.76 (95% CI 0.54–0.98).
Trained Subjects' Effects of 1- vs. 3-Sets on Single-Joint Exercise
Subgroup examination on the effects of pre- vs. post-training strength (trained subjects only) categorized as either S or M3 from four studies (Hass et al., 2000; Schlumberger et al., 2001; Kelly et al., 2007; Baker et al., 2013). Q heterogeneity test was: Chi2 = 3.91, d.f. = 3, P = 0.27. The random-effects model exposed a low amount of variability between studies. The heterogeneity statistic I2 (%) = 23 (interpreted as low, Higgins et al., 2003), and the tau2 test (between-trials variance) = 0.04. When a random effect analysis was implemented, a small effect was detected on trained only subjects for single-joint resistance exercises (ES 0.37; 95% CI −0.01 to 0.75). Pre- to post-intervention strength change was greater when M3 was compared to S (ES difference 0.09) with no statistical significance (P-value = 0.06). The mean for S was 0.75 (95% CI 0.12–1.28). The mean ES for M3 was 0.84 (95% CI 0.39–1.28).
Untrained Subjects' Effects of 1- vs. 3-Sets on Single-Joint Exercise
Examination of the effects of pre- vs. post-training strength (untrained subjects only) categorized as either S or M3 from five studies (Reid et al., 1987; Paulsen et al., 2003; Humburg et al., 2007; Bottaro et al., 2009; Sooneste et al., 2013). The random-effects model showed a low to moderate amount of variability between studies. Q heterogeneity test was: Chi2 = 6.76, d.f. = 4, P = 0.15. The heterogeneity statistic I2 (%) = 41 (interpreted as low-moderate, Higgins et al., 2003), and the tau2 test (between-trials variance) = 0.06, and a moderate effect was observed on untrained only subjects for single-joint exercises (ES 0.56; 95% CI 0.21–0.91). Pre- to post-intervention strength change was marginally greater when M3 was compared to S (ES difference 0.23) with statistical significance (P = 0.002). The mean for S was 0.51 (95% CI 0.24–0.77). The mean ES for M3 was 0.74 (95% CI 0.47–1.02).
Effects of 1- vs. 3-Sets on Upper Body Single-Joint Exercise
Subgroup examination of upper body single-joint exercises are presented in the forest plot. The pooled mean ES estimates comprised of six studies (Reid et al., 1987; Hass et al., 2000; Humburg et al., 2007; Bottaro et al., 2009; Baker et al., 2013; Sooneste et al., 2013). The random-effects model exposed a low to moderate amount of variability between studies. Q heterogeneity test was: Chi2 = 2.74, d.f. = 5, P = 0.74. The heterogeneity statistic I2 (%) = 0 (interpreted as no heterogeneity, Higgins et al., 2003), and the tau2 test (between-trials variance) = 0.00, and a trivial effect was detected (ES 0.20; 95% CI −0.07 to 0.47). Pre- to post-intervention strength change was comparable when M3 was compared with S (ES difference 0.07) with no statistically significance (P = 0.16). The mean ES for S was 0.69 (95% CI 0.28–1.11). The mean ES for M3 was 0.76 (95% CI 0.42–1.09). Subgroup examination of S vs. M3 pre- to post-intervention strength differences on trained only and untrained only subjects was not possible due to inadequate study data.
Effects of One –vs. Three-Sets on Lower Body Single-Joint Exercise
Examination on lower body single-joint exercises comprised of six studies (Reid et al., 1987; Hass et al., 2000; Schlumberger et al., 2001; Paulsen et al., 2003; Kelly et al., 2007; Bottaro et al., 2009). The random-effects model exposed a moderate amount of variability between studies (I2 = 63%). Removal of Hass et al. (2000) data, resulted in no heterogeneity (Q heterogeneity test was: Chi2 = 2.24, d.f. = 4, P = 0.69. The heterogeneity statistic I2 (%) = 0 (interpreted as no heterogeneity, Higgins et al., 2003), and the tau2 test (between-trials variance) = 0.00, and a small effect was observed (ES 0.41; 95% CI 0.08–0.74). Pre- to post-intervention strength change was greater when M3 was compared with S (ES difference 0.32) with statistical significance (P = 0.02). The mean ES for S was 0.40 (95% CI 0.17–0.63). The mean ES for M3 was 0.72 (95% CI 0.51–0.93). Subgroup examination of S vs. M3 pre- to post-intervention strength differences on trained only and untrained only subjects was not viable due to inadequate study data.
Discussion
This paper is the first meta-analytical review that compares terrestrial RT set-volume evidence and provides recommendations for pre-spaceflight preparation. This meta-analysis sought to determine whether M3 is associated with more significant strength gains than S per resistance exercise in RT programmes (Table 9). Additionally, results from this meta-analysis indicate the future need for research that explores appropriate physical RT implemented during spaceflight preparation. When trained and untrained subjects were combined, M3 was associated with greater ES in both combined multi-joint and single-joint exercises compared to S (Table 9). However, significant heterogeneity existed when combining different population groups (trained and untrained) and exercise type (multi-joint and single-joint). When body segmentation was analyzed (upper and lower body) M3 reported greater ES compared to S. Subgroup analysis on upper body only (trained and untrained combined and untrained studies only) reported marginally greater gains in strength when M3 was compared to S but were not significant. Trained subjects only reported statistically significant pre-post-strength changes (P < 0.0001) in upper body RT exercise. The data for lower body exercise reported that M3 was associated with greater ES in both combined population group (P = 0.02) and untrained subjects only (P = 0.02), but not trained subjects (P = 0.39).
Analysis of combined population groups on pooled multi-joint exercise and upper body multi-joint exercise reported greater strength gains with M3 compared to S. However, multi-joint lower body exercise reported marginally greater gains in strength with M3 compared to S, but these were not statistically significant. Subgroup analysis on untrained subjects only reported greater ESs on single-joint only exercises with M3 compared to S, with moderate heterogeneity detected between studies. When subgroup analysis was performed on trained only subjects on combined multi-joint exercises, upper body multi-joint only, and lower body multi-joint only the ES for M3 was statistically greater compared to S for strength gains. However, analysis of single-joint only exercise on trained subjects cannot fully support the contention that M3 produced greater ESs than S, as findings were not statistically significant. The results of this analysis provide support for the use of additional sets (up to three-sets) per exercise for increasing muscle strength. However, both S and M3 demonstrated significant pre-post-strength differences in multi-joint and single-joint exercises.
Ground-Based Meta-Analytical Recommendations for Untrained Individuals
The scientific literature on daily set-volume has been heavily contested with some suggesting that S produce similar adaptations to MS (Silvester et al., 1982; Messier and Dill, 1985; Reid et al., 1987; Pollock et al., 1993; Starkey et al., 1996; Hass et al., 2000). However, others indicate that MS produces greater strength, hypertrophy, and power adaptations (Kraemer, 1997; Hass et al., 2000; Borst et al., 2001; Marx et al., 2001; McBride et al., 2003; Paulsen et al., 2003). In the context of pre-flight conditioning, the initial physical training status of each crew member must be fully considered. Specifically, as the inclusion of smaller doses of daily RT (e.g., S per exercise) may be appropriate to develop muscular strength in less conditioned (untrained) crew members, whereas more substantial doses of RT (e.g., ≥M3 per exercise) may be essential to attain further strength.
Currently, the prescription of RT for pre-flight conditioning is recommended from ground-based models and experience gained during previous missions. Present recommendations for ST consist of one-to-three-sets per exercises for untrained individuals and MS used with variations in RT volume and intensity over a period for trained individuals [American College of Sports Medicine Position Stand (ACSM), 2009]. These recommendations on terrestrial RT set-volume have been subject to strong criticism due to reported methodological constraints within the included meta-analyses (Smith and Bruce-Low, 2004; Winett, 2004; Otto and Carpinelli, 2006; Carpinelli, 2012; Fisher, 2012). For example, the meta-analysis by Rhea et al. (2003) presented data that may have nullified the mean ES and spuriously affected the results. This is due to increasing the heterogeneity of the meta-analysis, therefore, erroneously favoring MS programming. Rhea and colleagues reported that untrained subjects should perform four-sets for strength increases (ES = 2.28 ± 1.96 SD), compared with S (ES = 1.16 ± 1.59 SD), two-sets (ES = 1.75 ± 3.23 SD), and M3 (ES = 1.94 ± 3.23 SD). However, the ES standard deviation for M3 was significantly larger in comparison to the other treatment groups, with no explanation provided. Otto and Carpinelli (2006) stated that several critical errors could invalidate the results including bias in the selection process of studies, incorrect classification of subjects training status, and variances of RT loading.
A meta-analysis by Wolfe et al. (2004) suggested that untrained subjects in the initial stages of training (6–15 weeks) perform with an S programme. Wolfe et al. (2004) also reported that untrained subjects had comparable pre-post-strength gains as those of trained individuals when performing MS. When subjects performed, RT exercises to physical failure vs. subjects' perceived end, multiple-set programmes generated more substantial increases in strength compared to S (P ≤ 0.002). A meta-analysis by Krieger (2009) examined the effects of S vs. multiple-sets of exercises on strength and suggested that two-to-three-sets were associated with a more significant ES than S. Krieger (2009) concluded that two-to-three-sets per resistance exercise were associated with 46% greater strength gains than S in both trained and untrained subjects. Finally, a meta-analysis by Fröhlich et al. (2010) of 72 studies found S training regimes to be the equivalent of multiple-set training for short intervention phases but multi-set training to be superior overextended RT intervention periods.
This current meta-analysis provides evidence that supports the contention that M3 RT leads to greater strength gains than S programming for untrained individuals or those astronauts that may be on the early phase of pre-flight conditioning. However, this analysis examined the differences in pre-post-strength gain between body segments and joint types rather than only pooling RT exercises together to generate ES. For upper body exercise, untrained subjects demonstrated a larger pooled mean ES estimate for M3 0.82 (95% CI 0.52–1.11) compared with S (0.60; 95% CI 0.34–0.85), however these results were not statistically significant (P = 0.42). Also, a significant degree of heterogeneity was present when exercises were “pooled” together. This intriguingly may explain why other previous meta-analyses reported varying results with regards to specific set-volume and encountered criticism from others as the included studies combined RT exercises to aggregate ES. Subgroup examination on untrained subjects was only feasible for upper and lower body and single-joint only exercises due to limited available data for multi-joint exercise. Untrained subjects pre- to post-strength gains on upper body exercise was greater when M3 was compared with S (ES difference 0.23) but was not statistically significant (P = 0.42). In contrast, lower body exercises reported significantly greater strength changes (P = 0.005) with M3 compared with S (ES difference 0.28). Strength gains on single-joint exercises exposed a larger pooled mean ES estimate for M3 (0.74; 95% CI 0.47–1.02) compared with S (0.51; 95% CI 0.24–0.77). When S was used as a control group and M3 used as the experimental set-volume, a moderate ES of 0.56 (95% CI 0.21–0.91; P = 0.002) suggested that M3 was effective in generating larger strength increases.
Even though evidence from this analysis supports increase set-volume for strength gain, caution is warranted concerning the interpretation of the findings as exercises performed in the included studies may not transfer toward improved operational task functioning. Furthermore, even though superior increases in strength may be produced with M3, the necessary time and physical effort required to attain such strength developments may not be essential for preparation toward spaceflight. The time to perform this additional conditioning may be better served in completing other necessary operational tasks.
Ground-Based Meta-Analytical Recommendations for Trained Individuals
Current recommendations from NASA for pre-flight conditioning suggest that trained astronauts who are in the final stages of pre-spaceflight preparation perform MS with variations in RT volume and intensity (American College of Sports Medicine Position Stand (ACSM), 2009; Garber et al., 2011). Several ground-based meta-analyses (Rhea et al., 2003; Peterson et al., 2004; Wolfe et al., 2004; Krieger, 2009; Fröhlich et al., 2010) that are included within the American College of Sports Medicine Position Stand (ACSM) (2009) recommendations have reported superior results when performing MS for trained subjects. Rhea et al. (2003) reported strength increases in the bench press of 20% for S, compared with a 33% increase with M3. Pre- to post leg strength increased by 25.4% for S compared with an increase of 52.1% with M3. However, the inclusion of the Rhea et al. (2002a) study may have generated errors or bias when comparing the means of the one-set and three-set groups (bench press ES = 2.3 and leg press ES 6.5). Also, the post-training standard deviation bench press findings were two-to-three times greater to the pre-training standard deviation in both groups, and the authors did not provide confidence intervals for ES. The meta-analysis by Peterson et al. (2004) stated that experienced athletes should complete eight-sets per muscle group to increase muscular strength. However, due to the low number of ES in the eight-set group, these conclusions may be unreliable when evaluating the specific percentage of 1RM training. An additional meta-analysis performed by Wolfe et al. (2004) reported that MS generated more significant increases in strength (P ≤ 0.001) for trained subjects.
This current meta-analysis cautiously provides evidence concerning increased sets and strength gain with support toward the use of additional sets (up to M3) compared to S for trained astronauts pre-flight conditioning programme. In this analysis, we reveal that multi-joint only exercises on trained subjects demonstrated a larger pooled mean ES estimate for M3 (0.99; 95% CI 0.60–1.38) compared with S (0.68; 95% CI 0.27–1.10) suggesting that M3 was more effective in producing larger strength gains. Further examination of upper body multi-joint only exercises further supports the use of M3. The pooled mean ES estimate for S was 0.59 (95% CI 0.09–1.08) compared with M3 (0.91; 95% CI 0.49–1.32) suggesting that M3 was more effective in producing strength gains. For single-joint exercises on trained subjects, there was a larger pooled mean ES estimate for M3 (0.84; 95% CI 0.39–1.28) compared with S (0.75; 95% CI 0.12–1.28). However, this was not statistically significant in producing more substantial strength gains.
Exercise prescription currently implemented by space agencies include the prescription of greater RT set-volume, but crew members do not perform the volume in a linear manner. It could be hypothesized, therefore, that by increasing set-volume prior to the commencement of spaceflight may develop astronaut's pre-flight levels of upper and lower body strength. Similarly, Krieger (2010) found that this range of sets and reps was superior in producing muscular hypertrophy. This suggests that the atrophy effects of long duration spaceflight could be mitigated, but not prevented, by a more rigorous deployment of multi-set RT methods. Astronauts that are appropriately conditioned and have progressed in their individualized RT toward a trained state should perform MS per exercise to maximize muscular strength, as it could safeguard against decreases in strength. However, caution should be given with the prescription of daily RT set-volume as exposing astronaut's to chronically high training volume may expose crew members to overtraining syndrome before spaceflight. Also, the inclusion of increased set-volume may also come at the determinant of the application toward training intensity and loading (e.g., 85% 1RM) and may feasibly inhibit strength development (Stone et al., 2006). Collectively, when accounting for other programme variables (RT loading and training frequency), M3 appears to a certain degree to be more advantageous for strength development in individuals that are trained. However, careful consideration must be given toward the astronauts initial pre-training status because of variance between individuals. Furthermore, comprehensive monitoring should be considered to prevent the potential of excess fatigue and to overtrain (Day et al., 2004; Halson, 2014).
Future Considerations Toward Pre-spaceflight Muscle Conditioning
Historically, exercise has been prescribed as a method to counteract the undesired physiological effects of long duration exposure to weightlessness and to safeguard astronaut's health (both acute and chronic). Space agencies have recently acknowledged that the pre-flight physical fitness of astronauts must be higher than age centered normative measurements before spaceflight. The preparation of crew members has advanced from methods that traditionally only improved physiological tolerance toward spaceflight to practices that prepare individuals to live and work in space (Garshnek, 1989). These physical training protocols are employed as a method of countering detrimental physiological adaptations that result from μG. The physical conditioning and preparation of astronauts for spaceflight are essential; however, space agencies approach mission preparation differently. It is evident that most agencies foster a graded dose-response continuum with the initial phase of training emphasizing lower set-volume progressing to MS at the concluding stages of flight preparation.
Based on evidence generated from this analysis, it would be appropriate to suggest that astronauts perform RT via a graded dose-response continuum in preparation for in space transit. However, published research in this area is limited with evidence centered on ground-based studies from older adult populations and bed rest studies that may not fully replicate the physiological pre-spaceflight muscle conditioning status of astronauts. More evidence is required concerning the effects of RT bouts and the dose-response relationship on the training status, age and sex differences for protecting the muscular systems. A greater understanding of the mechanisms for physiological conditioning would, therefore, help to develop appropriate individual centered countermeasures that mitigate deconditioning and must be an essential area of focus for future space physiology research. Ensuring each astronaut's pre-spaceflight strength is at the desired level may help to counteract in-flight and post-flight physiological stress which is paramount for the development of a viable human space exploration programme that goes beyond Earth's orbit.
Strengths and Limitations
There are numerous strengths of this meta-analysis that separate it from previous investigations that compare S vs. M3 configurations. Considerations toward evidence derived from previous critical reviews that identify limitations regarding the quality and selection of included studies that may bias outcomes have been applied [in part]. These review articles debated the findings from previous meta-analytical studies, identifying confounding factors and imprecisions that may have influenced outcome reliability. Previous review articles in this area, have reported issues concerning robustness and rigor that control confounding variables when comparing strength outcomes. Subsequently, this meta-analysis has been founded on critical consensus, current studies, and previous evidence gathered from meta-analyses presenting a re-examination of the evidence. Greater emphasis was placed on strict inclusion and exclusion criteria, specifying why each study was excluded to allow for greater transparency. The identification and the inclusion criteria of studies were restricted to reduce heterogeneity between studies making it easier to apply the results to specific population groups (trained and untrained). This enabled direct assessments to be drawn from studies that compared S and M3 while endeavoring to control for other variables that influence strength outcomes.
This meta-analysis endeavored to limit where possible publication bias which is the most significant source of type I error (the increase of false positive results). Graphical representation via funnel plots evaluated the presence of publication bias which was not present in other meta-analytical studies (Rhea et al., 2002b, 2003; Peterson et al., 2004; Wolfe et al., 2004; Krieger, 2010). Where no publication bias was present, the ES of each included study is distributed symmetrically around the underlying true ES, with a more random variation of this value in smaller studies. Asymmetry in the plot is suggestive of bias, most often due to smaller studies which are non-significant or have an effect in the opposite direction from that assumed (Greco et al., 2013). Within our design, we were conscious of potential heterogeneity and implemented a multi-level model as a method for assessing heterogeneity across studies. Also, visual inspection of forests plots further verified this and indicated the need for further subgroupings of studies which reduced the degree of heterogeneity.
Moreover, subgroup analysis was performed to allow for the assessment of training status (trained and untrained) separately and also the analysis of multi-joint and single-joint exercises. This helped to reduce heterogeneity between groups and allowed for more consistent comparisons to be made. Lastly, efforts were made to restrict the subject pool to population groups (trained and untrained) that were physically active and within a comparable age range that mirrored that of an astronaut's pre-flight fitness status. This is due to the disparity existing with astronaut's fundamental physiological characteristics, with physical fitness levels ranging from sedentary individuals who performed little or no physical activity to athletic standard.
Limitations of this meta-analysis include the use of small sections of the published information that is often derived from an insufficient range of methodological designs that has been determined by the inadequacy of available primary data. As with all meta-analyses, limitations and restrictions exist that include the assessment of aggregate outcomes that inevitably do not assess the same construct (i.e., “comparing apples and oranges”). This is the process of search and retrieval of available journal articles, and the potential effect of publication bias (Rosenthal, 1991). Cooper and Hedges (1994) stated that “retrieval bias” may exist even when robust inclusion criteria for gathering journals articles is applied. For example, in this analysis, retrieval bias may have occurred due to the selection and retrieval of journal articles published only in English. Although we strived to include journal articles from high-quality sources, the number of relevant studies was restricted, and there remained variances in experimental design and control among included studies. For example, four of the 12 included journal articles used RCTs. The other eight used a RAN that did not include a control group; instead, a repeated measures design was used with a baseline measure implemented as the control. However, baseline measures were not uniformly applied across those studies.
In this meta-analysis, the strength increases may be due to other physiological factors that have been acknowledged to affect strength changes including sex, age, physical activity levels, prior training history, genetics and endocrine status. This analysis was unable to subcategories subjects into either male or female population groups; instead, it combined population groups. Several studies have reported that sex influences muscle morphology and functioning (Chorney and Bourgeois, 1999; Sale, 1999). Häkkinen et al. (1996) reported that males have greater muscle strength and size than women due to higher levels of anabolic hormones and greater body mass. Also, it has been inferred that due to lower blood androgen levels in women that the response to RT would induce less muscle hypertrophy compared to men. Equally, some studies have reported no differences between sexes with similar improvements in strength adaptations (Colliander and Tesch, 1991; O'Hagan et al., 1995; Roth et al., 2001).
For this analysis, every effort was made to assess strength outcomes of comparable training designs and methodological construct. However, attempting to include studies that had a standardized RT programme was problematic. Even when attempting to control for variance within studies, confounding issues that may have influenced strength development were present. The RT loading had various ranges (63–93% 1RM) that could not be controlled for which could affect strength gains. As training loading is one of the most critical parameters of ST and the volume of RT training makes it challenging to provide a clear dose-response relationship that supports M3 compared to S programming for strength gain. The previous analyses found that loading of 60% of subjects 1RM (Rhea et al., 2003; Peterson et al., 2004) was sufficient to increase strength for untrained subjects, with trained subjects recommended to perform between 80 and 85% of their 1RM [American College of Sports Medicine Position Stand (ACSM), 2009]. The disparity in the training programme type and the order of resistance exercise between groups were not comparable in all included studies which feasibly could impact upon the set number and muscular strength. Scientific literature is currently equivocal regarding the implementation of heavy or light RT loads for muscular adaptations (Fisher et al., 2017). It must be acknowledged that the different repetition ranges included within this meta-analysis may also be a possible limitation. Several studies have reported comparable strength gains when training at different repetition ranges (Morton et al., 2011; Fisher et al., 2017) whereas others have shown improved strength when individuals trained with higher RT load and a low number of repetitions (Campos et al., 2002). This could be explained by the specificity of the strength test, as training with a low number of repetitions is closer to the 1RM test (Buckner et al., 2017; Gentil et al., 2017).
Finally, strength adaptations may have resulted from the performance of repeated 1RM testing as the resistance exercise loading specificity of the 1RM-tested exercises may have indirectly affected the subject's strength. For example, a subject's pre-to-post leg extension results may have increased, due to the performance of the leg press but not to the same amount as a leg press itself. This is supported by Dankel et al. (2016) who performed 1RM and maximum voluntary contraction testing on elbow flexion. The results intimated that the increase in the 1RM result might not be solely correlated to set-volume, but due to the “learning effect” of the specific resistance exercise. This could explain the variation in untrained subjects' strength due to the principle of training specificity, as neurological learning effects could, therefore, explain the broad range of heterogeneity between the inexperienced group.
Conclusion
The prescription for increasing maximal strength is a multifaceted process that involves careful manipulation of RT programme variables. A known association between sets (training volume) and strength improvement would be valuable to researchers, clinicians and astronauts whether they are pre-or in-flight. Recommendations on the appropriate number of sets per session per RE that elicit strength improvements is a contentious issue. The current academic literature does little to resolve this debate with the sets to strength gain correlation remaining unquantified. It is difficult to fully draw accurate conclusions from previous meta-analyses because of confounding training variables including programme duration, training frequency, muscle groups trained and measured, strength testing procedures, repetition velocity, and training status of the subjects. Independent of training status, M3 protocols should be included when maximal strength development is the primary objective of the training routine. However, single-set programmes also produce considerable increases in muscular strength, albeit not to the same degree as that of M3, and are advocated when training time is restricted or at the start of pre-flight conditioning programme.
For astronauts at the end of pre-flight conditioning and considered to be trained individuals, the use of M3 may be at the minimum necessary if not mandatory for trained individuals to further produce strength gains. These physically trained individuals may benefit from additional time and training volume to develop minor increases in performance customarily observed at this level of training progression. However, attention should be given with these advanced trainees due to the interaction of additional RT volume and time with the other fitness and mission orientated goals. However, it is essential to clarify that these modestly greater strength improvements occur at the expense of additional training effort. Ultimately, M3 entails 200–400% greater training volume than S training modes. For astronauts lacking in time, a reduced number of sets (S) per exercise may be what is needed to achieve their desired training goals. It is essential to consider this issue as a lack of time can be a barrier to exercise adherence, and one should be cautious before recommending M3 per exercise to deconditioned astronauts as it can hinder training progression. Also, astronauts in spaceflight preparation are subject to tight scheduling and most often experience time constraints. In such phases of limited time-availability, S can still provide some (yet less) training benefit and should be favored as an alternative exercise mode rather than nothing at all. More research is necessary to define the dose-response relationship for terrestrial RT. However, due to the unique environmental conditions experienced during spaceflight, it may be difficult to fully translate evidence generated from conventional terrestrial RT intervention studies to μG.
Author Contributions
All authors listed have made a substantial, direct and intellectual contribution to the work, and approved it for publication.
Conflict of Interest Statement
PJ is employed by KBR.
The remaining authors declare that the research was conducted in the absence of any commercial or financial relationships that could be construed as a potential conflict of interest.
Abbreviations
ACSM, American College of Sports Medicine; Chi2, Chi-square; CI, 95% confidence intervals; d.f., Degrees of freedom; ES, Effect size; I2, I-squared index test; ISS, International Space Station; IV, Inverse variance; M, Mean; M3, Three-set; MVC, Maximum voluntary contraction; NASA, National Aeronautics and Space Administration; n, Sample size number; P, Probability; PEDro, Physiotherapy evidence database; PRISMA, Preferred reporting items for systematic reviews and meta-analyses; Q, Cochran Q; RAN, Randomized trials; RCTs, Randomized control trials; RevMan, Review manager; RT, Resistance training; S, One-set; SD, Standard deviation; SE, Standard error; SEM, Standard error of measurement; SMD, Standardized mean difference; Tau2, Tau-square; μG, Microgravity; 1RM, One repetition maximum.
References
Adams, G. R., Caiozzo, V. J., and Baldwin, K. M. (2003). Skeletal muscle unweighting: spaceflight and ground-based models. J. Appl. Physiol. 95, 2185–2201. doi: 10.1152/japplphysiol.00346.2003
American College of Sports Medicine Position Stand (ACSM) (2009). Progression models in resistance training for healthy adults. Med. Sci. Sports Exerc. 41, 687–708. doi: 10.1249/MSS.0b013e3181915670
Baker, J. S., Davies, B., Cooper, S. M., Wong, D. P., Buchan, D. S., and Kilgore, L. (2013). Strength and body composition changes in recreationally strength-trained individuals: comparison of one versus three sets resistance training programmes. Biomed. Res. Int. 2013:615901. doi: 10.1155/2013/615901
Bloomberg, J. J., Reschke, M. F., Clement, G. R., Mulavara, A. P., and Taylor, L.C. (2016). Risk of Impaired Control of Spacecraft/Associated Systems and Decreased Mobility Due to Vestibular/Sensorimotor Alterations Associated with Space Flight. Houston, TX: Johnson Space Center. National Aeronautics and Space Administration report TM JSC-CN-36487.
Borst, S. E., De Hoyos, D. V., Garzarella, L., Vincent, K., Pollock, B. H., and Lowenthal, D. T., et al. (2001). Effects of resistance training on insulin-like growth factor-I and IGF binding proteins. Med. Sci. Sports Exerc. 33, 648–653. doi: 10.1097/00005768-200104000-00021
Bottaro, M., Veloso, J., de Salle, B. F., Simão, R., Celes, R., and Brown, L. E. (2009). Early phase adaptations of single vs. multiple sets of strength training on upper and lower body strength gains. Isokinet. Exerc. Sci. 17, 207–212. doi: 10.3233/IES-2009-0355
Buckner, S. L., Jessee, M. B., Mattocks, K. T., Mouser, J. G., Mouser, J. G., Dankel, S. J., et al. (2017). Determining strength: a case for multiple methods of measurement. Sport Med. 47, 193–195. doi: 10.1007/s40279-016-0580-3
Campos, G. E., Luecke, T. J., Wendeln, H. K., Toma, K., Hagerman, F. C., Murray, T. F., et al. (2002). Muscular adaptations in response to three different resistance-training regimens: specificity of repetition maximum training zones. Eur. J. Appl. Physiol. 88, 50–60. doi: 10.1007/s00421-002-0681-6
Carpenter, R. D., LeBlanc, A. D., Evans, H., Sibonga, J. D., and Lang, T. F. (2010). Long-term changes in the density and structure of the human hip and spine after long duration spaceflight. Acta Astronaut. 67, 71–81. doi: 10.1016/j.actaastro.2010.01.022
Carpinelli, R. N. (2012). Critical review of a meta-analysis for the effect of single and multiple sets of resistance training on strength gains. Med. Sport 16, 122–130. doi: 10.5604/17342260.1011393
Chorney, K., and Bourgeois, J. (1999). “Gender differences in skeletal muscle histology and ultrastructure,” in Gender Differences in Metabolism, ed M. A. Tarnopolsky (New York, NY: CRC Press), 37–59.
Cochran, W. (1954). The combination of estimates from different experiments. Biometrics 10, 101–129. doi: 10.2307/3001666
Cohen, J. (1998). Statistical Power Analysis for the Behavioral Sciences. 2nd Edn. Hillsdale, NJ: Erlbaum Associates.
Colliander, E. B., and Tesch, P. A. (1991). Responses to eccentric and concentric resistance training in females and males. Acta Physiol. Scand. 141, 149–156. doi: 10.1111/j.1748-1716.1991.tb09063.x
Convertino, V. A. (1990). Physiological adaptations to weightlessness: effects on exercise and work performance. Exerc. Sport Sci. Rev. 18, 119–166. doi: 10.1249/00003677-199001000-00007
Convertino, V. A., and Sandler, H. (1995). Exercise countermeasures for spaceflight. Acta Astronaut. 35, 253–270. doi: 10.1016/0094-5765(95)98731-N
Cooper, H., and Hedges, L. (1994). The Handbook of Research Synthesis. New York, NY: Russell Sage Foundation, 573.
Dankel, S. J., Counts, B. R., Barnett, B. E., Buckner, S. L., Abe, T., and Loenneke, J. P. (2016). Muscle adaptations following 21 consecutive days of strength test familiarization compared with traditional training. Muscle Nerve 53, 438–445. doi: 10.1002/mus.25488
Day, M. L., McGuigan, M. R., Brice, G., and Foster C. (2004). Monitoring exercise intensity during resistance training using the session-RPE scale. J. Strength Cond. Res. 18, 353–358. doi: 10.1519/R-13113.1
DerSimonian, R., and Laird, N. (1986). Meta-analysis in clinical trials. Control. Clin. Trials 7, 177–188. doi: 10.1016/0197-2456(86)90046-2
Duval, S., and Tweedie, R. (2000). Trim and fill: a simple funnel-plot-based method of testing and adjusting for publication bias in meta-analysis. Biometrics 56, 455–463. doi: 10.1111/j.0006-341X.2000.00455.x
Fisher, J. (2012). Beware the meta-analysis: is multiple set training really better than single set training for muscle hypertrophy? J. Exerc. Physiol. 15, 23–30.
Fisher, J., Steele, J., and Smith, D. (2017). High- and low-load resistance training: interpretation and practical application of current research findings. Sport Med. 47, 393–400. doi: 10.1007/s40279-016-0602-1
Fitts, R. H., Riley, D. R., and Widrick, J. J. (2001). Functional and structural adaptations of skeletal muscle to microgravity. J. Exp. Biol. 204, 3201–3208.
Fröhlich, M., Emrich, E., and Schmidtbleicher, D. (2010). Outcome effects of single-set versus multiple-set training-an advanced replication study. Res. Sports Med. 18, 157–175. doi: 10.1080/15438620903321045
Galvão, D. A., and Taaffe, D. R. (2005). Resistance exercise dosage in older adults: single-versus multiset effects on physical performance and body composition. J. Am. Geriatr. Soc. 53, 2090–2097. doi: 10.1111/j.1532-5415.2005.00494.x
Garber, C. E., Blissmer, B., Deschenes, M. R., Franklin, B. A., Lamonte, M. J., and Lee, I. M., et al. (2011). Quantity and quality of exercise for developing and maintaining cardiorespiratory, musculoskeletal, and neuromotor fitness in apparently healthy adults: guidance for prescribing exercise. Med. Sci. Sports Exerc. 43, 1334–1359. doi: 10.1249/MSS.0b013e318213fefb
Garshnek, V. (1989). Soviet space flight: the human element. Aviation Space Environ. Med. 60, 695–705.
Gentil, P., Del Vecchio, F. B., Paoli, A., Schoenfeld, B. J., and Bottaro, M. (2017). Isokinetic dynamometry and 1RM tests produce conflicting results for assessing alterations in muscle strength. J. Hum. Kinet. 56, 19–27. doi: 10.1515/hukin-2017-0019
Gopalakrishnan, R., Genc, K. O., Rice, A. J., Lee, S. M., Evans, H. J., and Maender, C. C. (2010). Muscle volume, strength, endurance, and exercise loads during 6-month missions in space. Aviat. Space Environ. Med. 81, 91–102. doi: 10.3357/ASEM.2583.2010
Greco, T., Zangrillo, A., Biondi-Zoccai, G., and Landoni, G. (2013). Meta-analysis: pitfalls and hints. Heart Lung Vessel. 5, 219–225.
Hackney, K. J., Scott, J. M., Hanson, A. M., English, K. L., Downs, M. E., and Ploutz-Snyder, L. L. (2015). The astronaut-athlete: optimizing human performance in space. J. Strength Cond. Res. 29, 3531–3545. doi: 10.1519/JSC.0000000000001191
Häkkinen, K., Kallinen, M., Linnamo, V., Pastinen, U. M., Newton, R. U., and Kraemer, W. J. (1996). Neuromuscular adaptations during bilateral versus unilateral strength training in middle-aged and elderly man and women. Acta Physiol. Scand. 158, 77–88. doi: 10.1046/j.1365-201X.1996.523293000.x
Halson, S. L. (2014). Monitoring training load to understand fatigue in athletes. Sports Med. 44, S139–S147. doi: 10.1007/s40279-014-0253-z
Hass, C. J., Garzarella, L., de Hoyos, D., and Pollock, M. L. (2000). Single versus multiple sets in long-term recreational weightlifters. Med. Sci. Sports Exerc. 32, 235–242. doi: 10.1097/00005768-200001000-00035
Higgins, J. P., Thompson, S. G., Deeks, J. J., Altman, D. G., Higgins, J. P., Thompson, S. G., et al. (2003). Measuring inconsistency in meta-analyses. BMJ 327, 557–560. doi: 10.1136/bmj.327.7414.557
Howell, D. C. (2012). Statistical Methods for Psychology. 8th Edn. Belmont, CA: Wadsworth Publishing.
Humburg, H., Baars, H., Schröder, J., Reer, R., and Braumann, K. M. (2007). 1-set vs. 3-set resistance training: a crossover study. J. Strength Cond. Res. 21, 578–582. doi: 10.1519/R-21596.1
Kelly, S. B., Brown, L. E., Coburn, J. W., Zinder, S. M., Gardner, L. M., and Nguyen, D. (2007). The effect of single versus multiple sets on strength. J. Strength Cond. Res. 21, 1003–1006. doi: 10.1519/00124278-200711000-00003
Kozlovskaya, I. B., Grogoriev, A. I., and Stepanstov, V. I. (1995). Countermeasure of the negative effects of weightlessness on physical systems in long-term space flights. Acta Astronaut. 36, 661–668. doi: 10.1016/0094-5765(95)00156-5
Kraemer, W. J. (1997). A series of studies: the physiological basis for strength training in American football: fact over philosophy. J. Strength Cond. Res. 11, 131–142. doi: 10.1519/00124278-199708000-00001
Kramer, J. B., Stone, M. H., O'Bryant, H. S., Conley, M. S., Johnson, R. L., Nieman, D. C., et al. (1997). Effects of single vs. multiple sets of weight training: impact of volume intensity, and variation. J. Strength Cond. Res. 11, 143–147. doi: 10.1519/00124278-199708000-00002
Krieger, J. W. (2009). Single vs multiple-sets of resistance exercise: a meta regression. J. Strength Cond. Res. 23, 1890–1901. doi: 10.1519/JSC.0b013e3181b370be
Krieger, J. W. (2010). Single vs. multiple sets of resistance exercise for muscle hypertrophy: a meta-analysis. J. Strength Cond. Res. 24, 1150–1159. doi: 10.1519/JSC.0b013e3181d4d436
Lang, T., Van Loon, J. J. W. A., Bloomfield, S., Vico, L., Chopard, A., and Rittweger, J. (2017). Towards human exploration of space: the THESEUS review series on muscle and bone research priorities. NPJ Microgravity 3:8. doi: 10.1038/s41526-017-0013-0
LeBlanc, A., Lin, C., Shackelford, L., Sinitsyn, V., Evans, H., and Belichenko, O. (2000). Muscle volume, MRI relaxation times(T2), and body composition after spaceflight. J. Appl. Physiol. 89, 2158–2164. doi: 10.1152/jappl.2000.89.6.2158
LeBlanc, A., Schneider, V., Shackelford, L., West, S., Oganov, V., and Bakulin, A. (1996). Bone mineral and lean tissue loss after long duration space flight. J. Bone Miner. Res. 11:S323.
Liberati, A., Altman, D. G., Tetzlaff, J., Mulrow, C., Gøtzsche, P. C., Ioannidis, J. P. A., et al. (2009). The PRISMA statement for reporting systematic reviews and meta-analyses of studies that evaluate health care interventions: explanation and elaboration. J. Clin. Epidemiol. 62, e1–e34. doi: 10.1016/j.jclinepi.2009.06.006
Loehr, J. A., Guilliams, M. E., Petersen, N., Hirsch, N., Kawashima, S., and Ohshima, H. (2015). Physical training for long-duration spaceflight. Aerosp. Med. Hum. Perform. 86, A14–A23. doi: 10.3357/AMHP.EC03.2015
Maher, C., Sherrington, C., Herbert, R., Moseley, A. M., and Elkins, M. (2003). Reliability of the PEDro scale for rating quality of randomised controlled trials. Phys. Ther. 83, 713–721.
Marx, J. O., Ratamess, N. A., Nindl, B. C., Gotshalk, L. A., Volek, J. S., Dohi, K., et al. (2001). The effects of singleset vs periodized multiple-set resistance training on muscular performance and hormonal concentrations in women. Med. Sci. Sports Exerc. 33, 635–643. doi: 10.1097/00005768-200104000-00019
Matsumoto, A., Storch, K. J., Stolfi, A., Mohler, S. R., Frey, M. A., and Stein, T. P. (2011). Weight loss in humans in space. Aviat. Space Environ. Med. 82, 615–621. doi: 10.3357/ASEM.2792.2011
McBride, J. M., Blaak, J. B., and Triplett-McBride, T. (2003). Effect of resistance exercise volume and complexity on EMG, strength, and regional body composition. Eur. J. Appl. Physiol. 90, 626–632. doi: 10.1007/s00421-003-0930-3
Messier, S. P., and Dill, M. E. (1985). Alterations in strength and maximal oxygen uptake consequence to Nautilus circuit weight training. Res. Q. Exerc. Sport. 56, 345–351. doi: 10.1080/02701367.1985.10605339
Morris, S. B., and DeShon, R. P. (2002). Combining effect size estimates in meta-analysis with repeated measures and independent-groups designs. Psychol. Methods. 7, 105–125. doi: 10.1037/1082-989X.7.1.105
Morton, S. K., Whitehead, J. R., Brinkert, R. H., and Caine, D. J. (2011). Resistance training vs. static stretching: effects on flexibility and strength. J. Strength Cond. Res. 25, 3391–3398. doi: 10.1519/JSC.0b013e31821624aa
Munn, J., Herbert, R. D., Hancock, M. J., and Gandevia, S. C. (2005). Resistance training for strength: effect of number of sets and contraction speed. Med. Sci. Sports Exerc. 37, 1622–1626. doi: 10.1249/01.mss.0000177583.41245.f8
O'Hagan, F. T., Sale, D. G., MacDougall, J. D., and Garner, S. H. (1995). Comparative effectiveness of accommodating and weight resistance training modes. Med. Sci. Sports Exerc. 27, 1210–1219.
Otto, R. M., and Carpinelli, R. N. (2006). The critical analysis of the single versus multiple set debate. J. Exerc. Physiol. 9, 32–57.
Paulsen, G., Myklestad, D., and Raastad, T. (2003). The influence of volume of exercise on early adaptations to strength training. J. Strength Cond. Res. 17, 115–120. doi: 10.1519/00124278-200302000-00019
Peterson, M. D., Rhea, M. R., and Alvar, B. A. (2004). Maximizing strength development in athletes: a meta-analysis to determine the dose- response relationship. J. Strength Cond. Res. 18, 377–382. doi: 10.1519/00124278-200405000-00031
Peterson, M. D., Rhea, M. R., and Alvar, B. A. (2005). Applications of the dose-response for muscular strength development: a review of meta-analytic efficacy and reliability for designing training prescription. J. Strength Cond. Res. 19, 950–958. doi: 10.1519/R-16874.1
Pollock, M. L., Graves, J. E., Bamman, M. M., Pollock, M. L., Graves, J. E., Bamman, M. M., et al. (1993). Frequency and volume of resistance training: effect of cervical extension strength. Arch. Phys. Med. Rehabil. 74, 1080–1086.
Radaelli, R., Fleck, S. J., Leite, T., Leite, R. D., Pinto, R. S., Fernandes L., et al. (2014). Dose-response of 1, 3 and 5 sets of resistance exercise on strength, local muscular endurance and hypertrophy. J. Strength Cond. Res. 29:134951348. doi: 10.1519/JSC.0000000000000758
Reid, C. M., Yeater, R. A., and Ulrich, I. H. (1987). Weight training and strength, cardiorespiratory functioning and body composition of men. Br. J. Sports Med. 21, 40–44. doi: 10.1136/bjsm.21.1.40
Rhea, M. R., Alvar, B. A., Ball, S. D., and Burkett, L. N. (2002a). Three sets of weight training superior to 1 set with equal intensity for eliciting strength. J. Strength Cond. Res. 16, 525–529. doi: 10.1519/00124278-200211000-00006
Rhea, M. R., Alvar, B. A., and Burkett, L. N. (2002b). Single versus multiple sets for strength: a meta-analysis to address the controversy. Res. Q. Exerc. Sport 73, 485–488. doi: 10.1080/02701367.2002.10609050
Rhea, M. R., Alvar, B. A., Burkett, L. N., and Ball, S. D. (2003). A meta-analysis to determine the dose-response for strength development. Med. Sci. Sports Exerc. 35, 456–464. doi: 10.1249/01.MSS.0000053727.63505.D4
Rohatgi, A. (2017). WebPlotDigitizer: Web Based Tool to Extract Data From Plots, Images, and Map. Austin, TX. Available online at: http://arohatgi.info/WebPlotDigitizer
Rønnestad, B. R., Egeland, W., Kvamme, N. H., Refsnes, P. E., Kadi, F., and Raastad, T. (2007). Dissimilar effects of one- and three-set strength training on strength and muscle mass gains in upper and lower body in untrained subjects. J. Strength Cond. Res. 21, 157–163. doi: 10.1519/00124278-200702000-00028
Rosenthal, R. (1991). Meta-Analytic Procedures for Social Research. Newbury Park, CA: Sage Publications, 168.
Roth, S. M., Ivey, F. M., and Martel, G. F. (2001). Muscle size responses to strength training in young and older men and women. J. Am. Geriatr. Soc. 49, 1428–1433. doi: 10.1046/j.1532-5415.2001.4911233.x
Sale, D. G. (1999). “Neuromuscular function,” in Gender Differences in Metabolism, ed M. A. Tarnopolsky (New York, NY: CRC Press), 61–85.
Scargle, J. (2000). Publication bias: The “file-drawer” problem in scientific inference. J. Sci. Explor. 14, 91–106.
Schlumberger, A., Stec, J., and Schmidtbleicher, D. (2001). Single- vs. multiple-set strength training in women. J. Strength Cond. Res. 15, 284–289. doi: 10.1519/00124278-200108000-00004
Schünemann, H. J., Tugwell, P., Reeves, B. C., Akl, E. A., Santesso, N., Spencer, F. A., et al. (2013). Non-randomized studies as a source of complementary, sequential or replacement evidence for randomized controlled trials in systematic reviews on the effects of interventions. Res. Synth. Methods 4, 49–62. doi: 10.1002/jrsm.1078
Silvester, L. J., Stiggins, C., McGown, C., and Bryce, G. (1982). The effect of variable resistance and free weight training programs on strength and vertical jump. Natl. Strength Cond. J. 3, 30–33.
Smith, D., and Bruce-Low, S. (2004). Strength training methods and the work of Arthur Jones. J. Exerc. Physiol. 7, 52–68.
Sooneste, H., Tanimoto, M., Kakigi, R., Saga, N., and Katamoto, S. (2013). Effects of training volume on strength and hypertrophy in young men. J. Strength Cond. Res. 27, 8–13. doi: 10.1519/JSC.0b013e3182679215
Starkey, D. B., Pollock, M. L., Ishida, Y., Welsch, M. A., Brechue, W. F., Graves, J. E., et al. (1996). Effect of resistance training volume on strength and muscle thickness. Med. Sci. Sports Exerc. 28, 1311–1320. doi: 10.1097/00005768-199610000-00016
Stein, T. P. (2013). Weight, muscle and bone loss during space flight: another perspective. Eur. J. Appl. Physiol. 113, 2171–2181. doi: 10.1007/s00421-012-2548-9
Stock, M. S., Olinghouse, K. D., Drusch, A. S., Mota, J. A., Hernandez, J. M., Akalonu, C. C., et al. (2016). Evidence of muscular adaptations within four weeks of barbell training in women. Hum. Mov. Sci. 45, 7–22. doi: 10.1016/j.humov.2015.11.004
Stone, H., Stone, M., Sands, W. A., Pierce, K., Newton, R. U., Haff, G. G., et al. (2006). Maximum strength and Strength training. A relationship to endurance? Strength Cond. J. 28, 44–53.
Suurmond, R., van Rhee, H., and Hak, T. (2017). Introduction, comparison and validation of Meta-Essentials: a free and simple tool for meta-analysis. Res. Synth. Methods 8, 537–553. doi: 10.1002/jrsm.1260
Trappe, S., Costill, D., Gallagher, P., Creer, A., Peters, J. R., Evans, H., et al. (2009). Exercise in space: human skeletal muscle after 6 months aboard the International Space Station. J. Appl. Physiol. 106, 1159–1168. doi: 10.1152/japplphysiol.91578.2008
Trappe, S. W., Trappe, T. A., Lee, G. A., Widrick, J. J., Costill, D. L., and Fitts, R. H. (2001). Comparison of a space shuttle flight (STS-78) and bed rest on human muscle function. J. Appl. Physiol. 91, 57–64. doi: 10.1152/jappl.2001.91.1.57
Verhagen, A. P. (1998). The Delphi list. A criteria list for quality assessment of randomized clinical trials for conducting systematic reviews developed by Delphi consensus. J. Clin. Epidemiol. 51, 1235–1241. doi: 10.1016/S0895-4356(98)00131-0
Widrick, J. J., Knuth, S. T., Norenberg, K. M., Romatowski, J. G., Bain, J. L., Riley, D. A., et al. (1999). Effect of a 17-day spaceflight on contractile properties of human soleus muscle fibres. J. Physiol. 516, 915–930. doi: 10.1111/j.1469-7793.1999.0915u.x
Winett, R. A. (2004). Meta-analyses do not support performance of multiple sets of high volumes resistance training. J. Exerc. Physiol. 7, 10–20.
Keywords: resistance training and muscular strength, resistance training and training volume, single vs.multiple-sets, one vs.multiple-sets and muscular strength, one vs.three-sets and muscular strength
Citation: Ralston GW, Kilgore L, Wyatt FB, Dutheil F, Jaekel P, Buchan DS and Baker JS (2019) Re-examination of 1- vs. 3-Sets of Resistance Exercise for Pre-spaceflight Muscle Conditioning: A Systematic Review and Meta-Analysis. Front. Physiol. 10:864. doi: 10.3389/fphys.2019.00864
Received: 30 January 2019; Accepted: 20 June 2019;
Published: 24 July 2019.
Edited by:
Richard D. Boyle, National Aeronautics and Space Administration (NASA), United StatesReviewed by:
Satoshi Iwase, Aichi Medical University, JapanSonia Julia-Sanchez, Ministerio de Educación Cultura y Deporte, Spain
Copyright © 2019 Ralston, Kilgore, Wyatt, Dutheil, Jaekel, Buchan and Baker. This is an open-access article distributed under the terms of the Creative Commons Attribution License (CC BY). The use, distribution or reproduction in other forums is permitted, provided the original author(s) and the copyright owner(s) are credited and that the original publication in this journal is cited, in accordance with accepted academic practice. No use, distribution or reproduction is permitted which does not comply with these terms.
*Correspondence: Grant W. Ralston, Z3JhbnQucmFsc3RvbkBmb3J0aHZhbGxleS5hYy51aw==