- 1ICBAS, Instituto de Ciências Biomédicas Abel Salazar, Universidade do Porto, Porto, Portugal
- 2CIIMAR/CIMAR, Centro Interdisciplinar de Investigação Marinha e Ambiental, Universidade do Porto, Matosinhos, Portugal
- 3IATS – CSIC, Instituto de Acuicultura Torre de la Sal, Castellón de la Plana, Spain
- 4GreenUPorto, DGAOT, Faculdade de Ciências, Universidade do Porto, Porto, Portugal
- 5SORGAL – Sociedade de Óleos e Rações, S.A., S. João de Ovar, Portugal
Objective: This study aims to determine the maximal inclusion level of defatted (d-) Tenebrio molitor larvae meal (TM) able to replace dietary fishmeal (FM) without compromising growth performance, general metabolism, and flesh quality traits in European sea bass, and to evaluate the major underlying physiological mechanisms.
Materials and Methods: Fish (55 ± 2 g) were fed with diets containing increasing levels of dTM: 0, 40, 80 and 100% (CTRL, TM40, TM80, and TM100, respectively) to replace FM. After 10 weeks of feeding, the growth performance, nutrient and energy balance, intestinal integrity, plasma metabolites and the expression of genes related to growth and nutrient metabolism, in liver and muscle were determined. The fatty acids (FA) profile, textural properties and color were also evaluated in muscle.
Results: Protein and lipids digestibility remained unaltered up to 80% dTM inclusion. Growth performance parameters were similar among dietary treatments. The dTM inclusion increased the hepatosomatic index in fish fed TM100. Muscle eicosapentaenoic acid, docosahexaenoic acid and n-3 long-chain polyunsaturated FA levels were maintained up to 80% dTM inclusion, but total cholesterol and non-esterified FA increased with dietary dTM inclusion. In liver, the expression of elongation of very long-chain FA protein 6 (elovl6) and FA desaturase 2 (fads2) did not change in fish fed TM40 and TM80, but elovl6 decreased whilst fads2 increased in fish fed TM100 when compared to those fed CTRL. The expression of cholesterol 7 alpha-monooxygenase (cyp7a1) decreased with dietary dTM inclusion. In muscle, the expression of myoblast determination protein-2 (myod2) decreased in fish fed TM80 and TM100.
Conclusion: It is feasible to substitute dietary FM by dTM up to 80% in European sea bass without detrimental effects on nutrient digestibility, growth performance and associated genetic pathways, whilst assuring fillet nutritional value for human consumption.
Introduction
The use of insect protein as sustainable alternative to animal and plant protein sources has been encouraged for direct human consumption and incorporation into animal feeds (Nogales-Mérida et al., 2018; Salter, 2019). Insects have several advantages when compared with the conventional protein sources, as they grow fast and reproduce easily, have low feed conversion ratio (FCR) and small need of arable land and water (Gasco et al., 2020). Additionally, insects are rich protein sources, with a well-balanced essential amino acid (EAA) profile; being also rich sources of fat and some vitamins and minerals (Koutsos et al., 2019). Insects can also be valuable sources of healthy compounds, such as chitin and antioxidant and antimicrobial peptides. Recent studies showed that insect-based diets modulate fish microbiota and improve the immune system, which may reduce the use of antibiotics in aquaculture (Bruni et al., 2018: Henry et al., 2018; Antonopoulou et al., 2019; Rimoldi et al., 2019; Stenberg et al., 2019; Li et al., 2021). In 2017, European Union (EU) authorized the use of insect meal (IM) from seven species, including yellow mealworm (Tenebrio molitor), in aquafeeds (European Commission, 2017). Basto et al. (2020) have recently demonstrated that defatted (d-) T. molitor larvae meal (TM) is a highly digestible protein source able to meet European sea bass (Dicentrarchus labrax) EAA requirements (Basto et al., 2020). The potential of TM to partially replace fishmeal (FM) in aquafeeds has been previously assessed in various marine fish species, such as European sea bass or sparids (Gasco et al., 2016; Piccolo et al., 2017; Henry et al., 2018; Antonopoulou et al., 2019; Iaconisi et al., 2019; Ido et al., 2019), whilst total replacement was only evaluated in red sea bream (Pargus major) (Ido et al., 2019). Results among authors are controversial, and the maximal replacement level of FM by TM are extremely variable and never exceeding 71% in diets for European sea bass (Gasco et al., 2016).
The main nutritional limitation of including insects in aquafeeds is their low content of long-chain polyunsaturated fatty acids (LC-PUFA), such as the eicosapentaenoic (EPA; 20:5n−3) and docosahexaenoic (DHA; 22:6n−3) acids. Fish muscle is the main dietary source of these two n-3 LC-PUFA for humans, which are associated with beneficial health effects (Lands, 2014). Thus, IM may compromise not only flesh quality, but also growth performance when included at high levels in diets for marine fish species. Fish oil (FO) and FM are the main dietary sources of n-3 LC-PUFA, such as EPA and DHA. Thus, high FM replacement levels, or the concomitant replacement of FM and FO, have to be carefully addressed to not compromise the recommended dietary levels of n-3 LC-PUFA for marine fish species (approximately 0.7% on dry matter (DM) basis) (Skalli and Robin, 2004). In blackspot sea bream (Pagellus bogaraveo), full-fat TM did not affect fillet EPA or DHA content, when included at 21% of feed, but decreased the EPA content when included at 40% (Iaconisi et al., 2017). This suggest that dietary IM may induce changes in lipid metabolism, depending on its dietary inclusion level. Besides, IM also has a limited content of phosphorus (P), which may affect not only lipid metabolism, but also fish growth performance (Sugiura et al., 2004; Prabhu et al., 2014).
The present study aimed to explore the impact of partial and total replacement of FM by dTM in a comprehensive approach focusing not only on growth performance and nutrient utilization, but also on the underlying mechanisms involved in nutrient metabolism, namely lipid metabolism, in European sea bass. This is one of the most important fish species in Mediterranean aquaculture, where production surpassed 194,000 tons in 2018 (Eurostat, 2020). Muscle quality traits and fillet nutritional value for human consumption were also evaluated.
Materials and Methods
Diets
Extruded practical diets were formulated and produced by SPAROS Lda. (Portugal) to meet all the known nutritional requirements of European sea bass (NRC, 2011). All diets contained FO (12–13% inclusion level) as the main dietary lipid source. In the control diet (CTRL), FM was added at 45% and was progressively replaced in experimental diets (40%, TM40; 80%, TM80; 100%, TM100) by dTM from Entomo Farm (France). The inclusion level of dTM increased up to 60% in TM100, and was due to the concomitant replacement of FM and plant proteins, resulting in increased protein and lipid content (Table 1). All experimental diets were properly supplemented with DL-methionine and mono-calcium phosphate. For determination of the apparent digestibility coefficients (ADC), 1% chromium oxide (Cr2O3) was added, as an inert marker, to each experimental diet. Details of dietary fatty acid (FA) and amino acid (AA) profile are shown in Table 2 and Supplementary Table 1, respectively.
Fish Husbandry
Juvenile fish of Atlantic origin were obtained from Acuinuga S. L. (Spain). Fish, for both the digestibility and the growth trials, were held in quarantine (2000 L tanks) for 2 weeks and hand-fed with a commercial diet (AQUASOJA, Portugal – 50% crude protein and 20% crude fat on DM basis). During quarantine period, fish were held in a recirculation aquaculture system (RAS) at 24 ± 1°C, 35 ± 0.5 ‰ and 6 L/min –1. Total ammonium (NH4+), nitrite (NO2–), nitrate (NO3–) and pH levels were maintained within the recommended ranges for marine species (NH4+ ≤ 0.05 mg L–1; NO2– ≤ 0.5 mg L–1; 5 ≤ mg L–1; 7.5 ≤ pH ≥ 8.5). Dissolved oxygen level was kept above 90% saturation and an artificial photoperiod of 12 h’ light/12 h’ dark cycle was fixed.
Digestibility Trial
Fish (58 ± 1 g) from the initial stock were randomly distributed in 8 tanks of 50 L (18 fish per tank) equipped with feces sedimentation columns (Cho and Slinger, 1979). Fish were fed the experimental diets (with 1% chromium oxide (Cr2O3) until apparent satiation, once a day, during 5 days for adaptation to the diets, before the feces collection began. Approximately 30 min after feeding, every tank was carefully cleaned to assure that no remains of uneaten feed were left in the bottom of the tank or in the sedimentation column. Feces were collected from the sedimentation column every morning, before feeding, and then centrifuged at 3000 g, to eliminate water excess, and kept at −20°C until chemical analysis. Daily collection of the feces was performed for 10 consecutive days. In order to test each diet in quadruplicate and since the RAS was only constituted by 12 tanks equipped with feces sedimentation columns, this procedure was repeated over two consecutive rounds. In each round, each diet was tested in duplicate. In the second round, diets were allocated to different tanks and fed to new groups of 18 fish per tank from the same initial stock and with the same size range (58 ± 1 g) used in the first round.
Growth Trial
Twelve homogeneous groups of 25 fish (55 ± 2 g) from the initial stock were randomly distributed in 160 L tanks. Each diet was distributed to triplicate groups of fish, by automatic feeders until visual satiation, three times daily, for 10 weeks. The amount of feed supplied to each tank was adjusted daily, and when some uneaten feed remained in the bottom of the tank, the total amount of feed distributed each day was reduced by 5%, until no feed losses were recorded. When no feed losses were observed, the amount of feed was maintained for 2 days, and then augmented by 5%.
Fish Sampling
After overnight fasting, ten fish from the initial stock, and five fish from each tank at the end of growth trial were sampled, sacrificed by anesthetic overdose (2-phenoxyethanol, 500 μL/L), and kept at −20°C until whole-body composition analysis. Also, at the end of the growth trial, five additional fish per tank were slightly anesthetized with 2-Phenoxyethanol (200 μL/L) for blood and tissue sampling. Blood was collected from the caudal vein with heparinized syringes, centrifuged at 10,000 g for 5 min at 4°C, and the collected plasma was stored at −80°C until metabolite analysis. Prior to sampling the remaining tissues, fish were sacrificed by a sharp blow on the head. Liver and white skeletal muscle (dorsal right side) portions (100 mg) were then collected, frozen in liquid nitrogen and stored at −80°C until RNA extraction. Sections of anterior intestine after the pyloric caeca (5 mm thick) were excised, washed, fixed in phosphate-buffered formalin 4% (pH 7) for 24 h, and posteriorly preserved in ethanol 70% until histological analysis. A cross-sectional slab with skin (5 mm thick) was taken just before the dorsal fin, and photographed (with a scale reference) for determination of the cross-sectional area (CSA). A portion from the left part of the fillet (1 × 1 cm) was frozen in isopentane, cooled by dry ice, and stored at −80°C for later histological analysis. Two other muscle samples, from the left part of the fillet, were taken, frozen in liquid nitrogen and stored at −80°C for DM, total lipid content and FA profile analyses. Additionally, the right dorsal fillet was collected with skin for instrumental color and texture analysis.
Proximate Composition Analysis
Freeze-dried fish, diets and feces were ground and homogenized prior to proximate composition analysis. Ash, DM, crude protein (N × 6.25), lipids, P and energy were determined according to AOAC methods (AOAC, 2006) as described by Basto et al. (2020). The Cr2O3 content in diets and feces was determined according to Bolin et al. (1952).
Total Lipids and Fatty Acids Analyses
Muscle total lipids were extracted and quantified gravimetrically according to Folch et al. (1957), using dichloromethane instead of chloroform. The muscle FA methyl esters (FAME) were obtained through transesterification of lipid extracts, whilst in diets FAME were obtained by direct transesterification. The identification and quantification of FAME was performed as described by Campos et al. (2017), using the tricosaenoic acid (23:0) as internal standard.
Histological Analysis
Samples of anterior intestine were embedded in paraffin and processed according to standard histological procedures. Intestinal cross-sections (3 μm) were stained with specific Alcian Blue/PAS staining (pH 2.5) and examined under a light microscope (Olympus BX51, GmbH, Germany) coupled with a camera (Olympus DP50, GmbH, Germany). An imaging software (Olympus cellSens Dimension Desktop) was used to determine the following parameters: intestine CSA (mm2); width of muscularis externa (μm), outer longitudinal and inner circular layers (OLL and ICL, respectively); submucosa and lamina propria width (μm); villus length and width (μm); number of neutral and acid goblet cells (NGC and AGC, respectively) per villus. The OLL, ICL, and submucosa width were measured in eight points of each transverse section analyzed, and the mean was considered. The lamina propria width (measured in the middle of the villus), and villus length (measured from the top to the bottom following their natural curves) and width (measured at the base) were measured in the eight highest villus. Goblet cells were counted on these selected highest villi. All measurements are exemplified in Figure 1.
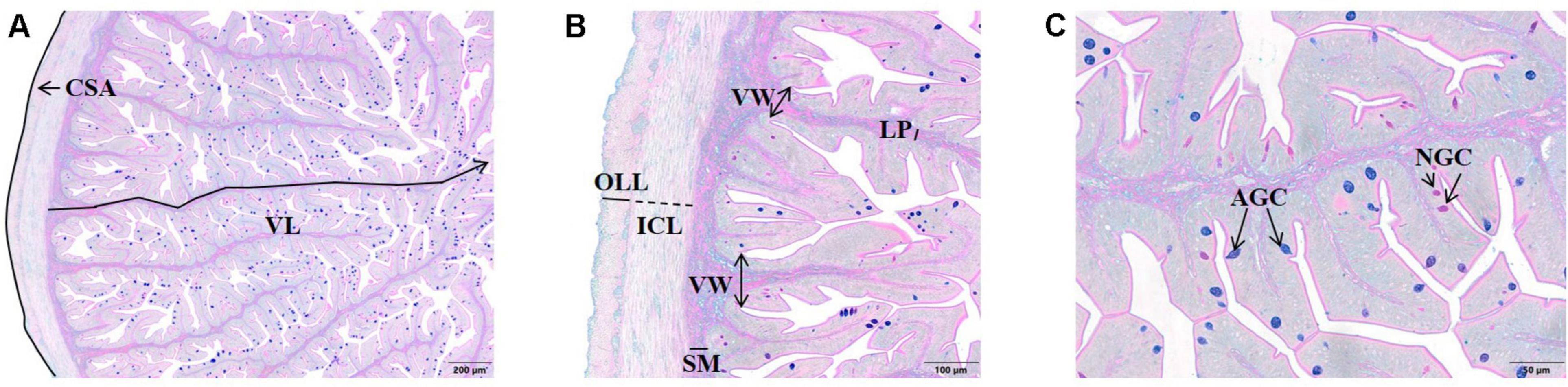
Figure 1. Histological sections (Alcian blue/PAS staining, pH = 2.5) of the anterior intestine of European sea bass. (A) CSA, cross-sectional area, VL, villus length (40×). (B) ICL, inner circular layer, LP, lamina propria, OLL, outer longitudinal layer, SM, submucosa VL, villus width (100×). (C) AGC, acid goblet cell, NGC, neutral goblet cell (200×).
Frozen cross-sections of muscle (12 μm) were stained with hematoxylin-eosin and examined using the previously mentioned imaging software. The following parameters were calculated: muscle CSA (mm2); total number of fibers per CSA; fiber number per unit of area (density; n°/mm2); and frequency of fibers classes according to their diameter (≤20 μm and >140 μm) as reported in Lopes et al. (2017).
Instrumental Texture and Color Analysis
Muscle color measurements were done with a CR-400 chromameter (Konica Minolta Inc., Japan) with an aperture of 8 mm, with respect to CIE standard illuminant D65. The chromameter was applied onto each sample and three replicates of lightness, redness and yellowness (L∗, a∗, and b∗, respectively) values were recorded. From a∗ and b∗ values the hue angle [H° = tan–1 (b∗/a∗)] and the chroma [C∗ = (a*2 + b*2)1/2] were calculated (Choubert et al., 1997). Muscle texture was analyzed using a TA.XT.plus Texture Analyser with a 5 kg load cell and a 2.0 mm diameter probe (Stable Micro systems Inc., United Kingdom). Texture profile parameters [hardness (N), adhesiveness (J), springiness (-), cohesiveness (-), chewiness (J), and resilience (-)] were obtained by double penetration (probe speed of 1 mm/s; probe penetration depth of 4 mm; wait time between penetrations of 5 s) on the thickest part of each raw fillet according to Batista et al. (2020).
Plasma Biochemistry
Glucose, total protein, triglycerides, total cholesterol and non-esterified FA (NEFA) levels were determined enzymatically using commercial kits (1001190, 1001291, 1001313, and 1001090, Spinreact, Spain; 434-91795 NEFA-HR (2) R1 and 436-91995 NEFA-HR (2) R2, Wako Chemicals, Germany, respectively), adapting manufacturer’s instructions to microplate format.
Gene Expression
Total RNA from liver and skeletal muscle was extracted using the MagMAX-96 total RNA isolation kit (Life Technologies, United States) after tissue homogenization in TRI reagent following manufacturers’ instructions. The RNA quantity and purity were determined by Nanodrop (Thermo Fisher Scientific, United States) with absorbance ratios (A260/A280) of 1.9–2.1. Reverse transcription (RT) of 500 ng of total RNA was performed with random decamers using the High-Capacity cDNA Archive Kit (Applied Biosystems, United States) following manufacturer’s instructions. The RT reactions were incubated for 10 min at 25°C and 2 h at 37°C. Negative control reactions were run without reverse transcriptase. The synthetized cDNA was used for PCR quantification with a SYBR Green Master Mix (Bio-Rad, Hercules, CA, United States), and specific primers at a final concentration of 0.9 μM (Supplementary Table 2). Two customized PCR-array layouts were designed for the simultaneous gene expression profiling of 49 genes covering a number of markers of growth-hormone/insulin-growth-factors (GH/IGF) system (10), lipid metabolism (12), energy metabolism (11), muscle cell proliferation and differentiation (12), and protein turnover (4) (Table 3). The program used for qPCR reactions included an initial denaturation step at 95°C for 3 min, followed by 40 cycles of denaturation for 15 s at 95°C and annealing/extension for 60 s at 60°C. All the pipetting operations were made by means of an EpMotion 5070 Liquid Handling Robot (Eppendorf, Germany) to improve data reproducibility. The efficiency of qPCRs was checked, and the specificity of reactions was verified by analysis of melting and linearity of serial dilutions of RT reactions. Fluorescence data acquired during the extension phase were normalized by the delta-delta CT method (Livak and Schmittgen, 2001), using β-actin as housekeeping gene due to its stability among different experimental conditions (average CT among experimental groups varied less than 0.2).
Calculations
The ADC of the experimental diets were calculated according to Maynard et al. (1979): dry matter ADC (%) = 100 × [1 - (dietary Cr2O3 level/feces Cr2O3 level)] and nutrients or energy ADC (%) = 100 × [1 - (dietary Cr2O3 level/feces Cr2O3 level) × (feces nutrient or energy level/dietary nutrient or energy)]; Average body weight (ABW) = (final body weight + initial body weight)/2; Digestible nitrogen (N), lipids (L), P or energy (E) intake = (dry feed consumption × N, L, P (%) or E (kJ/g) in the diet × ADC N, L, P, or E)/ABW/days; N, L, P, or E gain = (final carcass N, L, P or E content - initial carcass N, L, P, or E content)/ABW/days; N, L, P, or E retention efficiency (NRE, LRE, PRE, or ERE) = (N, L, P, or E gain/digestible N, L, P or E intake) × 100; Fecal N, L, P, or E losses = crude N, L, P, or E intake × [1 - (ADC N, L, P, or E/100)]. Metabolic N, L, or P losses = digestible N, L, or P intake−N, L or P gain; Branchial and urinary E losses = non-fecal N losses × 25 kJ/N; Total N, L, P, or L losses = crude N, L, or P intake−N, L, or P gain; Metabolizable energy (ME) = digestible E intake - branchial and urinary E losses; Total heat loss = E intake – E gain; Total heat production = ME - energy gain. Condition factor (K) = [final body weight/(final body length)3] × 100; Daily growth index (DGI) = 100 × [(final body weight)1/3 - (initial body weight)1/3]/days; Voluntary feed intake (VFI) = 100 × dry feed intake/average body weight/day; Feed conversion ratio (FCR) = dry feed intake/weight gain; Protein efficiency ratio (PER) = weight gain/crude protein intake; Hepatosomatic index (HSI) = 100 × liver weight/body weight; Viscerosomatic index (VSI) = 100 × weight of viscera/body weight.
Statistical Analysis
Data were tested for normality and homogeneity of variances by Kolmogorov-Smirnov and Levene’s tests, respectively, and log-transformed whenever required before submitted to a one-way ANOVA using IBM SPSS Statistics 26.0 (IBM corporation, United States). When one-way ANOVA showed significance (p < 0.05), individual means were compared using Student-Newman-Keuls post-test. Fold-changes of genes were submitted to a student’s t-test with significance set at p < 0.05. A bivariate spearmen’s rank correlation coefficient (rs) test was applied to all variables. Significant correlations were considered at the bilateral level of 0.05.
Results
Digestibility and Nutrient Balance
Overall, all diets were well digested (DM ADC > 76%; protein ADC ≥ 89%; lipids ADC ≥ 97%; energy ADC > 86%; P ADC > 62%), but differences on nutrient digestibility were found among groups in the digestibility trial (Table 4). Fish fed TM100 had the lowest protein apparent digestibility, and the highest lipid and P digestibility. As a result, N intake and metabolic N losses decreased with total FM replacement, and the opposite pattern was found for N retention efficiency. Fecal N losses increased both in fish fed TM100 and in those fed TM80. Digestible P intake decreased with FM replacement, being significantly lower in fish fed TM80 and TM100 compared to fish fed CTRL. Fecal P losses decreased significantly with the concomitant increase of dietary dTM. Total P losses of fish fed dTM decreased significantly compared to fish fed CTRL, regardless of the dietary dTM level. Conversely, in comparison to fish fed CTRL and other experimental groups, both lipid and energy gain and retention efficiency were consistently higher in fish fed TM100, whilst lower branchial and urinary E losses, total E losses and total heat production were observed. Fecal E losses increased significantly in fish fed TM40 and TM80. The net balance (total gain, fecal and metabolic losses) of N, P, and L is summarized in Figure 2.
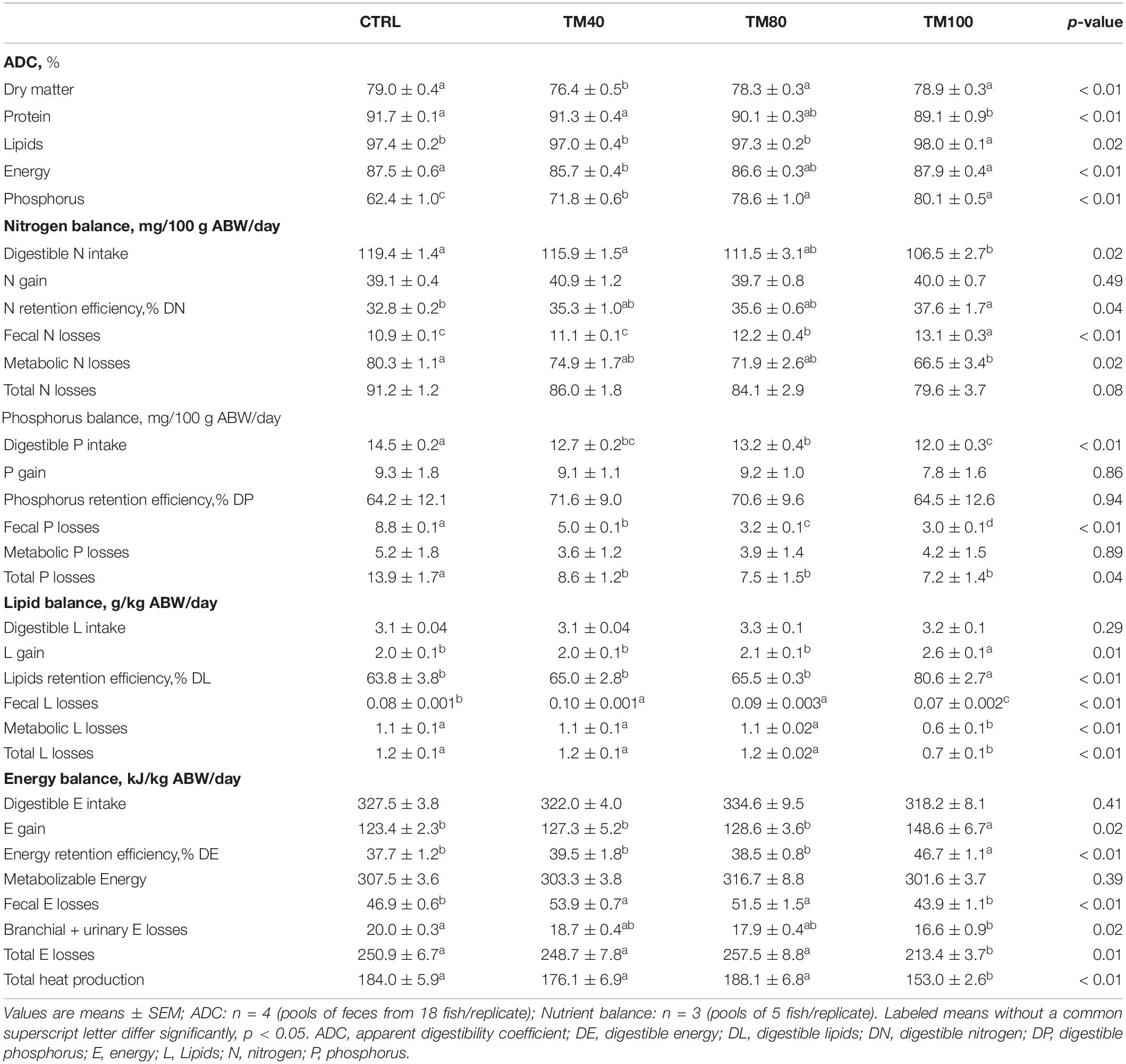
Table 4. Apparent digestibility coefficients and nutrient balances of European sea bass fed experimental diets.
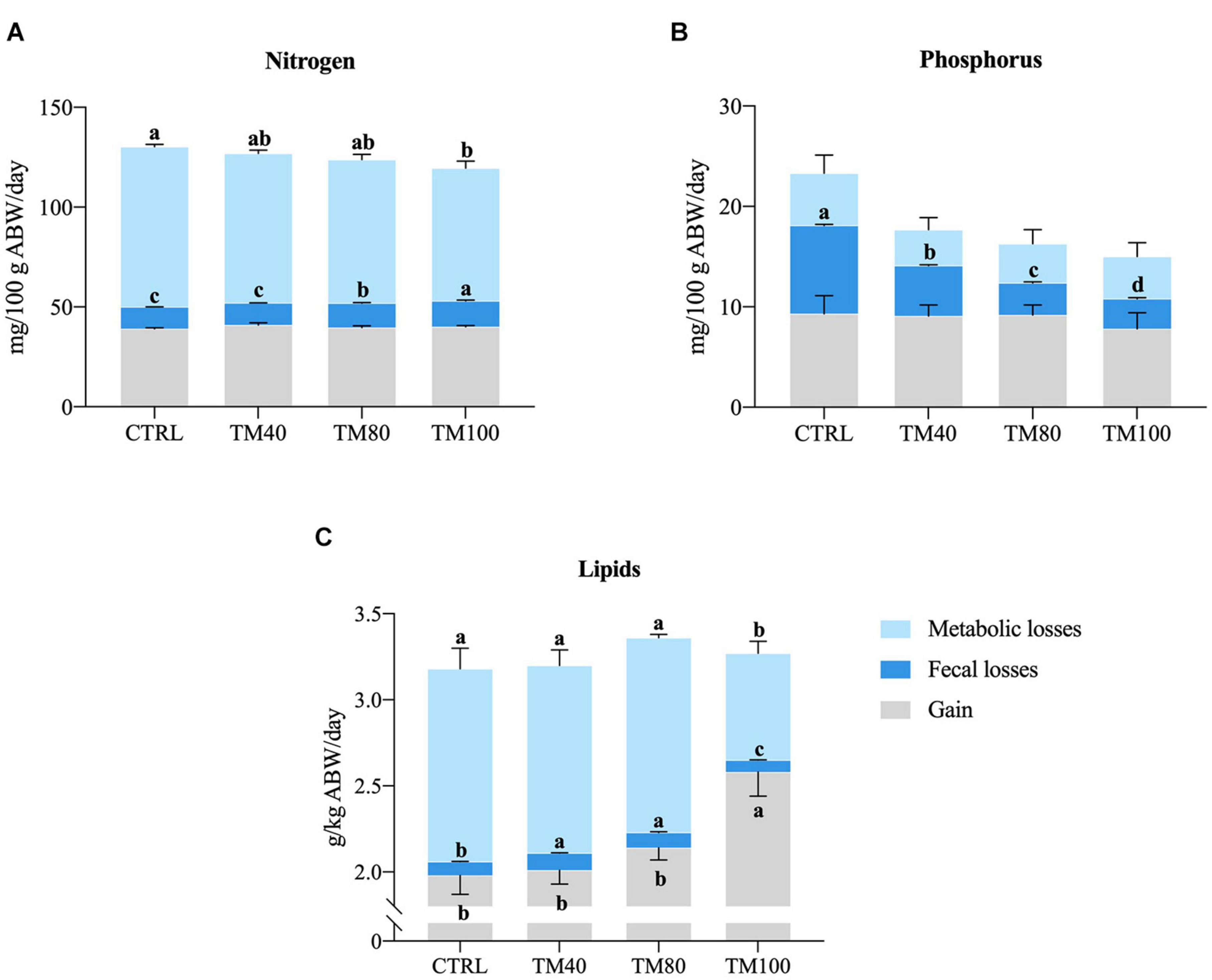
Figure 2. Daily nitrogen (A), phosphorous (B), and lipids (C) balance of European sea bass fed experimental diets. Bars represent means ± SD; n = 3 (pools of 5 fish/replicate). Labeled means without a common superscript letter differ significantly, p < 0.05.
Growth Performance and Blood Biochemistry
As shown in Table 5, fish almost tripled their initial weight, and no mortality was registered during the entire growth trial. All groups grew at the same rate and a significant decrease of FCR from 1.2 in fish fed CTRL to 1.0 in those fed TM100 was observed. Final K remained similar among dietary treatments. The whole-body lipids and energy content increased in fish fed TM100 with a concomitant decrease of moisture. This group also showed a statistically significant increase of HSI that was positively correlated with whole-body lipid content (0.59; p = 0.04) and lipid gain (0.59; p = 0.04). The VSI also displayed a tendency to increase in fish fed TM100 fish, being this biometric index negatively correlated with metabolic (−0.64; p = 0.03) and total lipid (−0.64; p = 0.03) losses (Supplementary Table 3). Regarding blood analysis, plasma levels of total cholesterol and NEFA increased with the replacement of FM by dTM, regardless of the inclusion level. Plasma triglycerides were only significantly affected by TM100, resulting in the highest values (Figure 3). Cholesterol and/or triglycerides showed positive correlations with HSI and VSI (Supplementary Table 3). Concerning the fish-in:fish-out ratio (Fi:Fo), the calculated values were reduced by 20% in fish fed TM100 in comparison to those fed CTRL or the two other experimental groups (Figure 4).
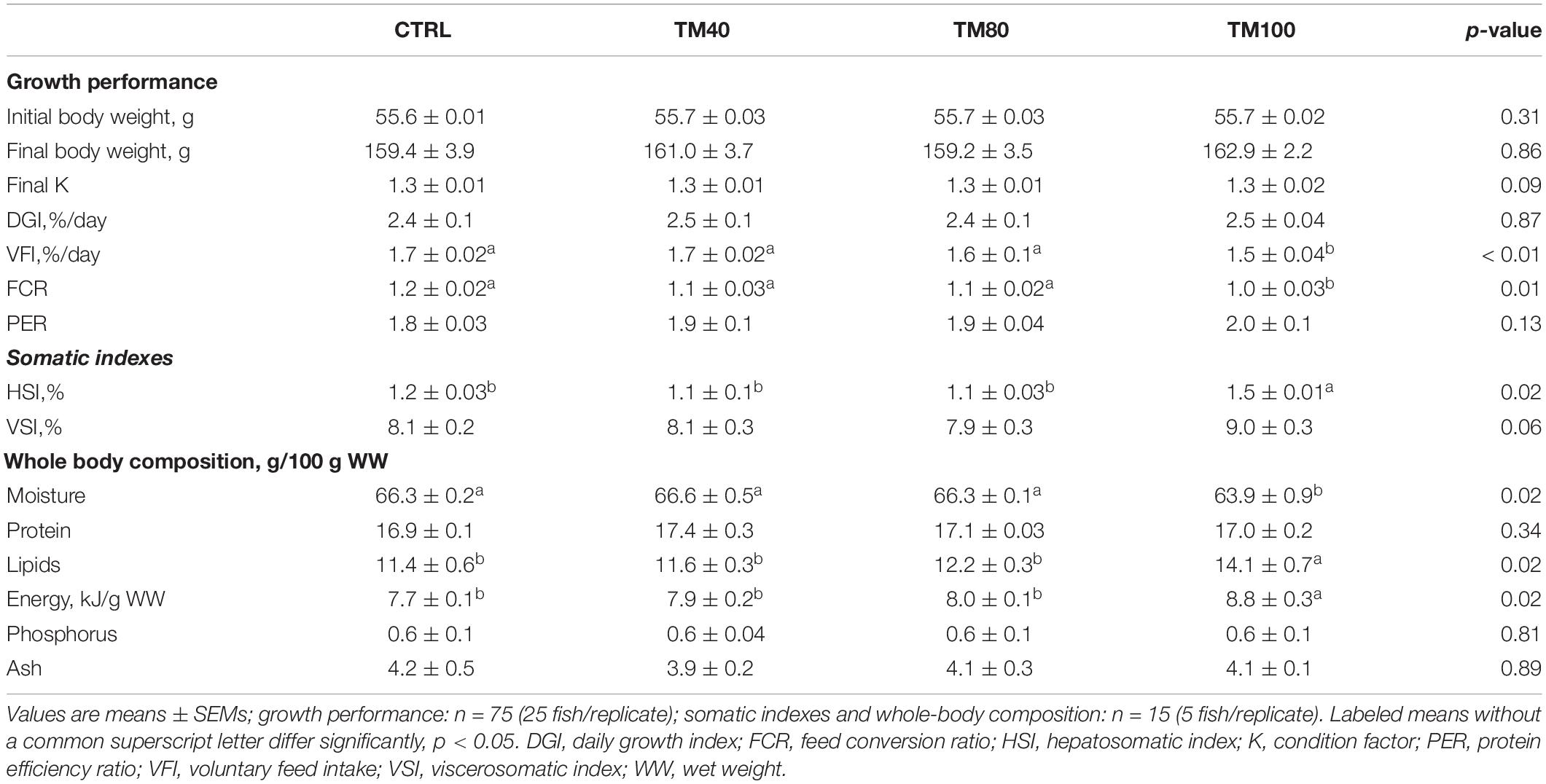
Table 5. Growth performance, somatic indexes and whole-body composition of European sea bass fed experimental diets.
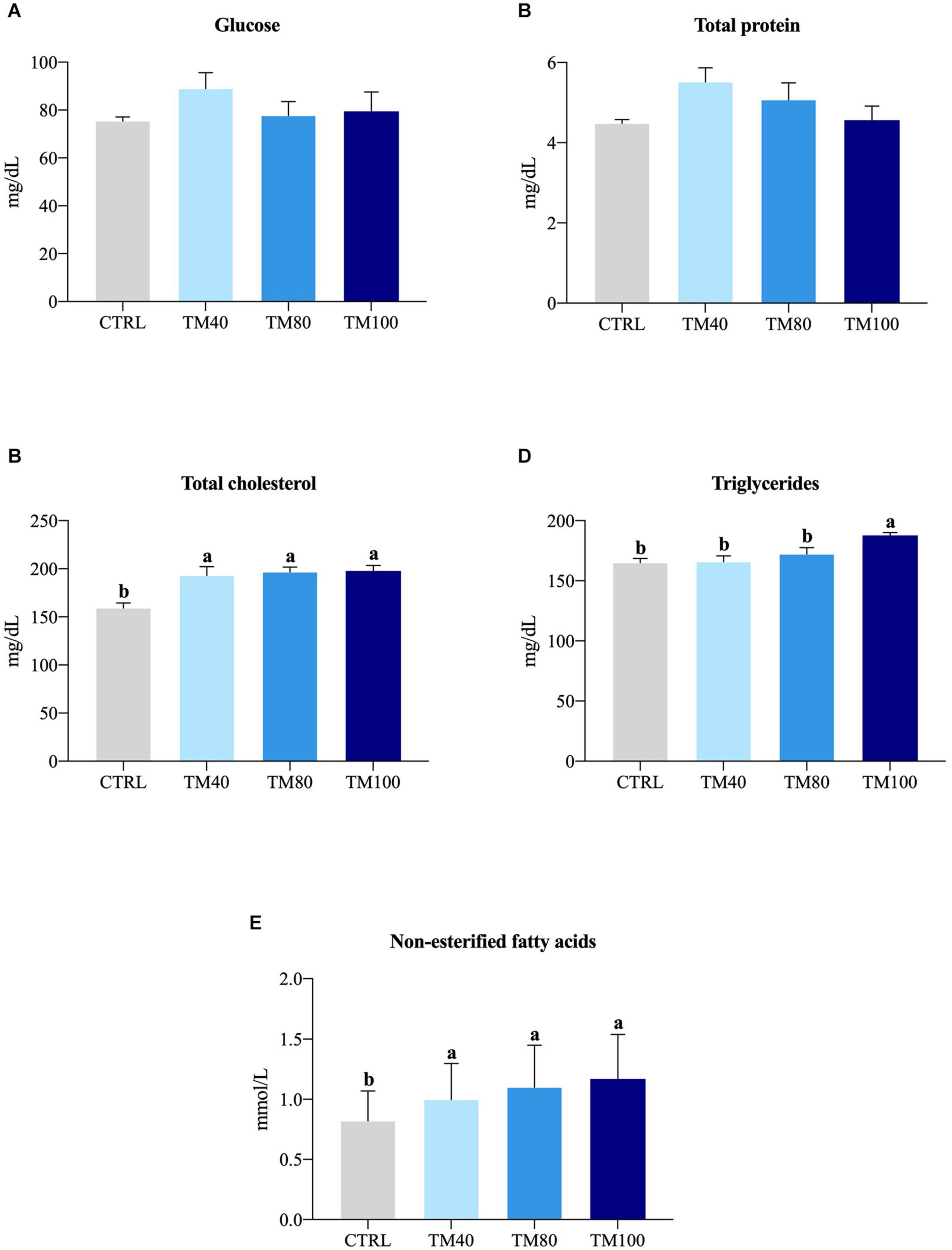
Figure 3. Plasma glucose (A), total protein (B), total cholesterol (C), triglycerides (D) and non-esterified fatty acids (E) of European sea bass fed experimental diets. Bars represent means ± SEM; n = 15 (5 fish/replicate). Labeled means without a common superscript letter differ significantly, p < 0.05.
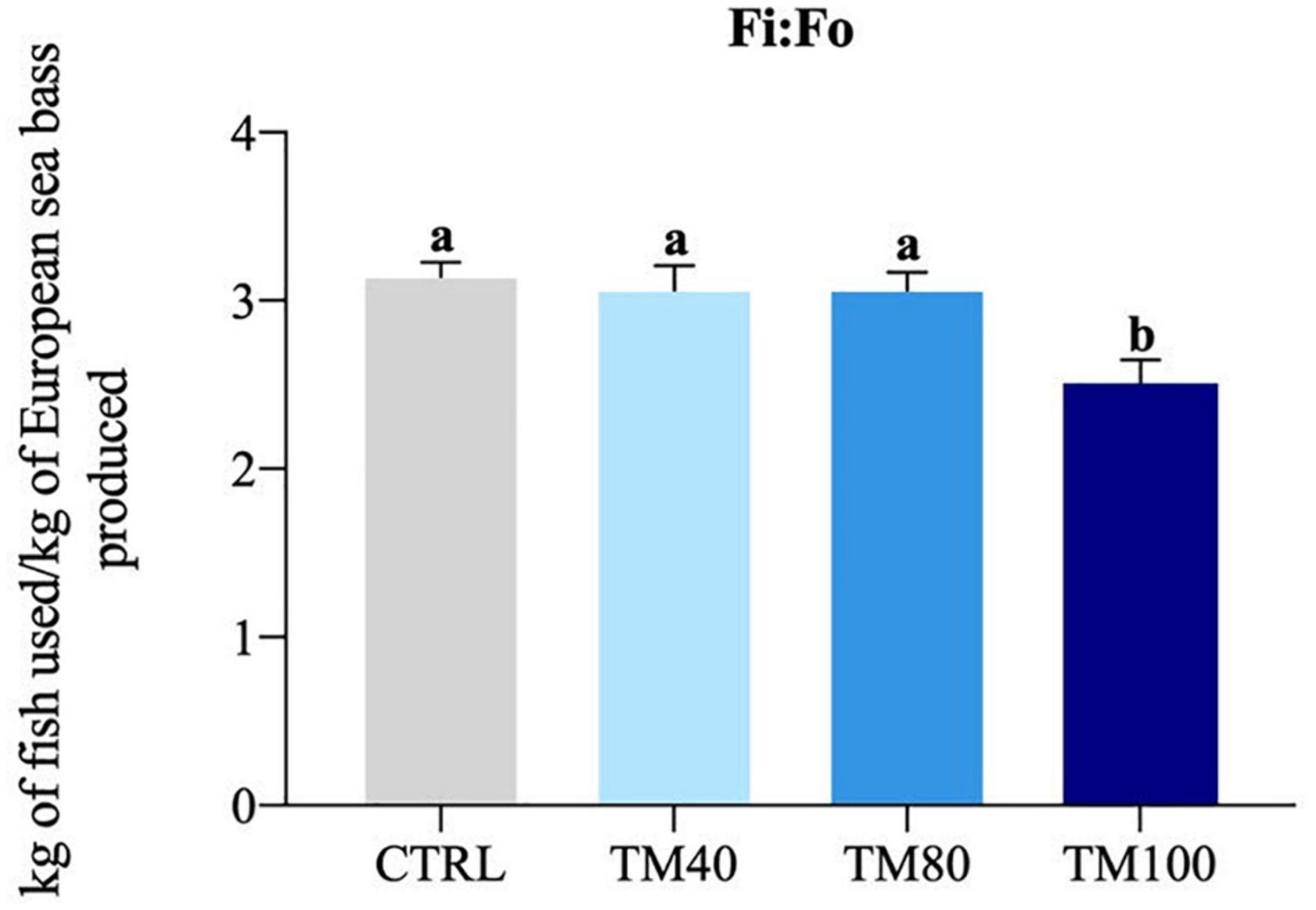
Figure 4. Fi:Fo ratio. Bars represent means ± SD; n = 3 (pools of 5 fish/replicate). Labeled means without a common superscript letter differ significantly, p < 0.05. Fi:Fo was calculated according to Tacon and Metian (2008), assuming yield from forage fish of 24% for FM and 4.8% for FO.
Muscle Total Lipids and Fatty Acids Analyses
Despite the increase of lipids in TM100 diet, the muscle total lipids content was similar among groups (Table 6). The muscle saturated (SFA) and monounsaturated FA (MUFA) profile reflected the dietary FA profile (Table 2). Up to 80% of FM replacement by dTM, the muscle PUFA profile also reflected the dietary FA profile. On the other hand, and despite the highest percentage of PUFA in TM100 diet, muscle PUFA levels in fish fed TM100 were comparable to those of all other treatments. The relative percentage of muscle EPA, DHA, and n-3 LC-PUFA of fish fed TM40 and TM80 was similar to those fed CTRL, despite their dietary percentage being decreased with the dTM inclusion. Although fish fed TM100 had the lowest relative percentage of EPA and DHA, when expressed in wet weight (g/100 g WW), both muscle EPA and EPA + DHA final contents were similar among all fish. As observed in the experimental diets, the percentage of muscle n-6 LC-PUFA increased with dTM inclusion and consequently the n-3/n-6 ratio decreased.
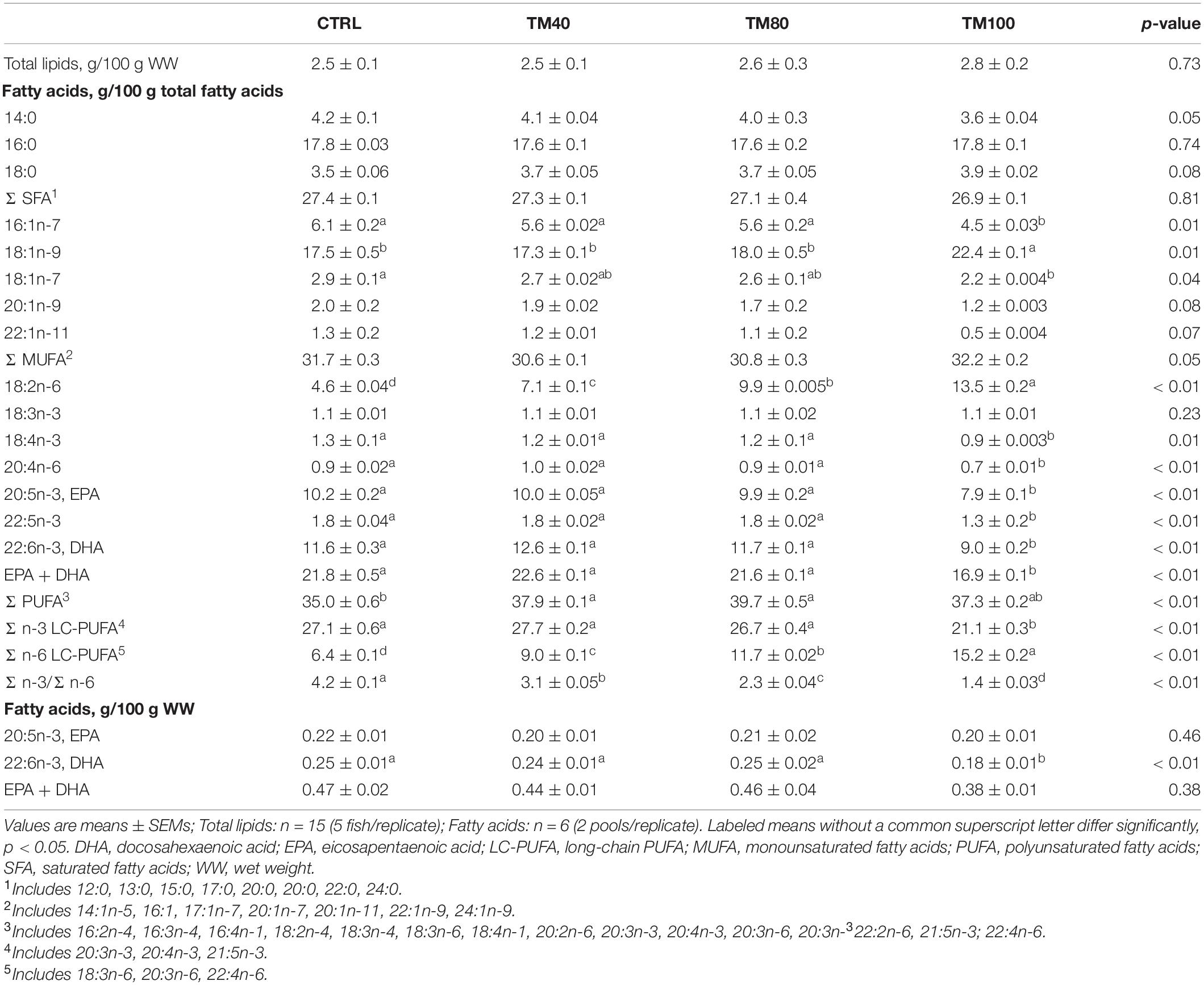
Table 6. Muscle total lipid content and fatty acid composition of the European sea bass fed experimental diets.
Intestine and Muscle Histological Analysis
The morphology of anterior intestine was well-preserved in all fish, with no signs of villus fusion or enterocyte vacuolization. Intestine CSA varied between 11 and 15 mm2. The OLL and ICL varied between 32 and 41 μm, and 58 and 69 μm, respectively. The lamina propria width varied between 27 and 30 μm. The villus length and width ranged from 1749 to 2001 μm and 161 to 180 μm, respectively. The number of AGC and NGC per villus ranged from 147 to 192, and 70 to 113, respectively. The morphometric parameters analyzed in the anterior intestine did not vary significantly among dietary treatments, with the exception of submucosa thickness that increased concomitantly with dietary dTM inclusion level: the submucosa thickness increased from 27 μm in fish fed CTRL to 29, 30, and 33 μm in those fed TM40, TM80 and TM100, respectively (Supplementary Table 4). White muscle CSA varied between 459 and 496 mm2, total number of fibers ranged from 104 to 108 thousand, and fiber density from 217 to 228 (Supplementary Table 5). Fiber diameter varied between 65 and 67 μm, with 5–6% of the fibers having below 20 μm and 2–3% above 140 μm. None of the morphometric parameters analyzed in muscle had significant differences among dietary treatments.
Muscle Instrumental Color and Texture Analysis
Texture and color parameters were similar among dietary treatments (Supplementary Table 6). Hardness ranged from 0.9 to 1 N, adhesiveness from −0.01 to −0.004 J, springiness from 1.2 to 1.6, cohesiveness from 0.4 to 0.5, chewiness from 0.5 to 0.8 J, and resilience was 0.4 irrespectively of the dietary treatment. In the muscle, L∗ varied from 41 to 42, a∗ from −0.5 to −0.2, b∗ from −0.7 to 0.1, H° from 183 to 250 and C° from 1 to 1.2.
Gene Expression
The relative gene expression profile of key selected markers in liver and muscle is shown in Supplementary Tables 7, 8, with arbitrarily assigned values of 1 for hormone sensitive lipase (hsl) in liver of fish fed CTRL, and calpain 2 (capn2) in muscle of fish fed CTRL. Among all the analyzed genes, up to three markers of hepatic lipid metabolism and four markers of muscle growth were differentially expressed with the FM replacement, and the corresponding log2 fold-changes (dTM/CTRL) were graphically represented. In liver, the expression of elongation of very long-chain FA protein 6 (elovl6) was significantly down-regulated in fish fed TM100, but not in those fed TM40 and TM80 (Figure 5A). Conversely, FA desaturase 2 (fads2) was markedly up-regulated in fish fed TM100, remaining almost unaltered in those fed TM40 and TM80 (Figure 5B). A strong nutritional regulation was also found for cholesterol 7-alpha-monooxygenase (cyp7a1) with a significant down-regulation in all dTM groups (Figure 5C). A similar trend was observed for the expression of stearoyl-CoA desaturase 1b (scd1b), although this down-regulation was not statistically significant (Figure 5D). The expression of lipolytic peroxisome proliferator-activated receptor α (pparα) also evidenced a non-significant down-regulation trend for fish fed TM100 (Figure 5E). The expression of elovl6, cyp7a1 and pparα was negatively correlated with plasma cholesterol levels (−0.64 (p < 0.01), −0.46 (p < 0.01), and −0.37 (p = 0.02), respectively) (Supplementary Table 9). Myoblast determination protein 2 (myod2) was down-regulated in TM80 and TM100 (Figure 6A), whereas a significant down-regulation of muscle atrophy F-box (mafbx/atrogin-1) was only found in fish fed TM100 (Figure 6B). Contrarily, the expression of myostatin (mstn) (Figure 6C) and myoblast fusion factor (mymk) (Figure 6D) tended to increase with FM replacement level, but this increase was only statistically significant in TM100.
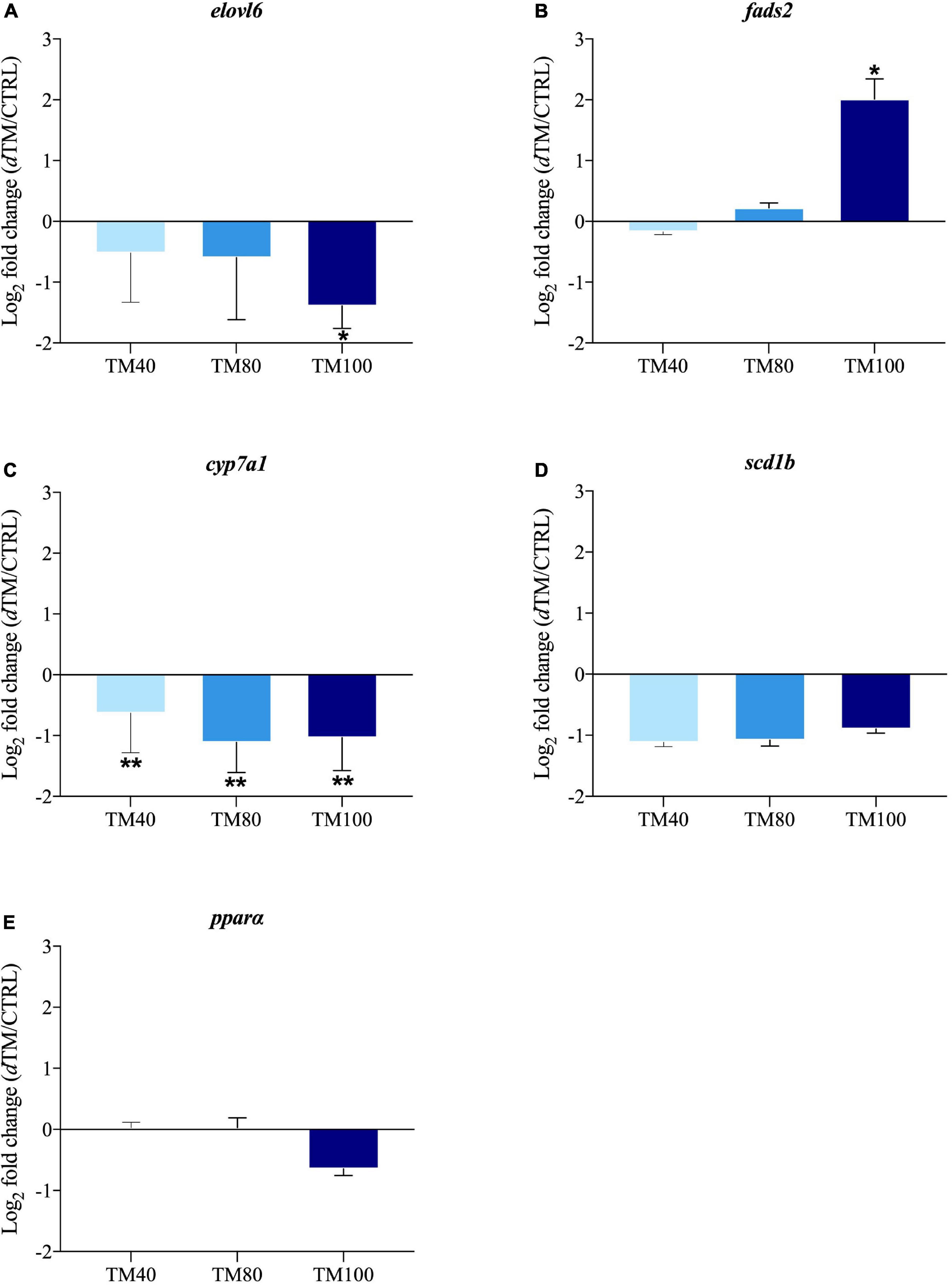
Figure 5. Fold-changes (dTM/CTRL) of differentially expressed genes in liver tissue: elovl6 (A), fads (B), cyp7a1 (C), scd1b (D) and pparα (E). The asterisks indicate statistically significant differences (*p < 0.05; **p < 0.01; t-student) between European sea bass fed dTM and CTRL diets. Values >1 indicate up-regulated genes in dTM fish; values <1 indicate down-regulated genes in dTM fish; n = 9 (3 fish/replicate).
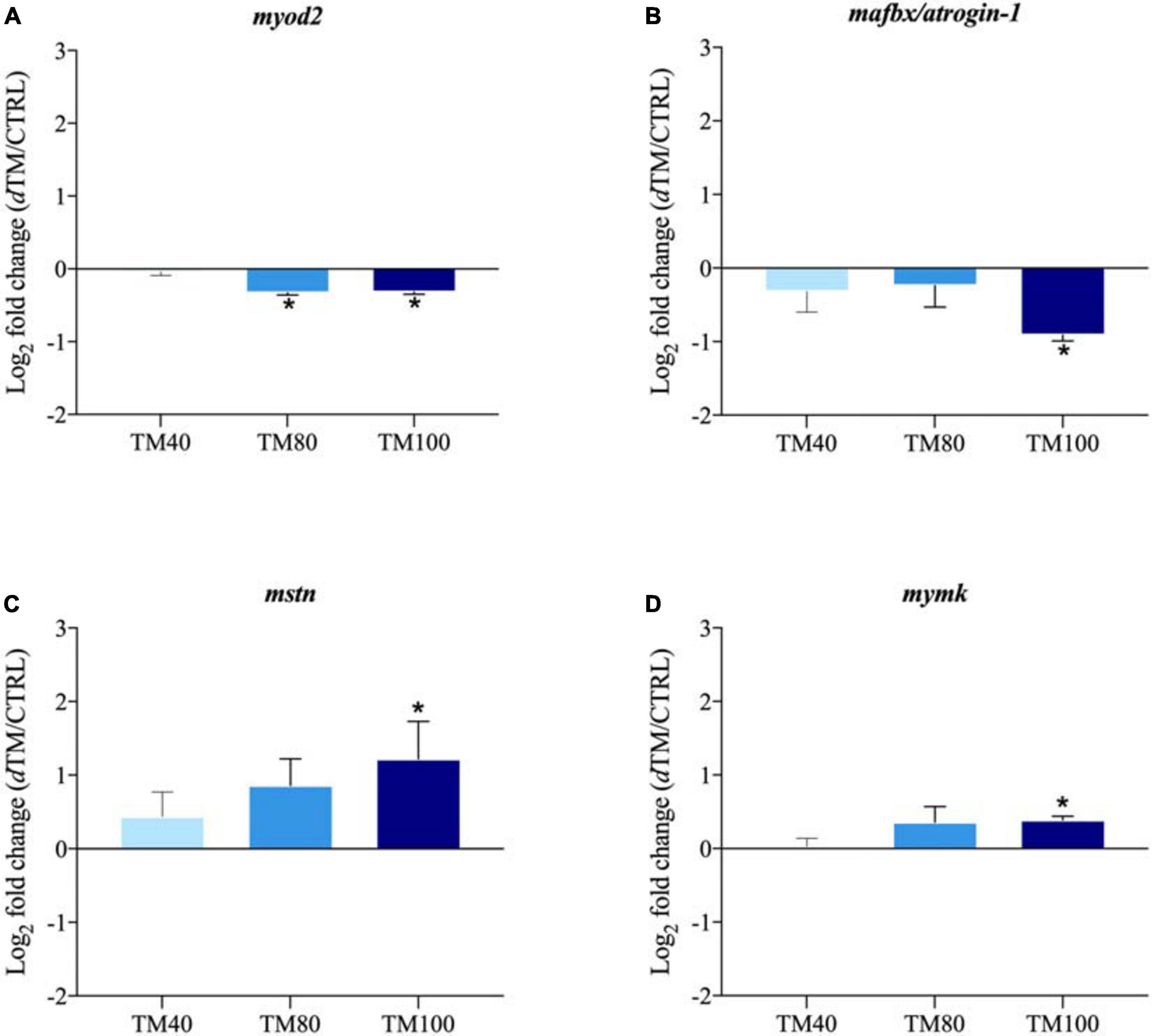
Figure 6. Fold-changes (dTM/CTRL) of differentially expressed genes in muscle tissue: myod2 (A), mstn (B), mafbx/atrogin 1 (C), and mymk (D). The asterisks indicate statistically significant differences (*p < 0.05; t-student) between European sea bass fed dTM and CTRL diets. Values >1 indicate up-regulated genes in dTM fish; values <1 indicate down-regulated genes in dTM fish. n = 9 (3 fish/replicate).
Discussion
Since the use of IM in aquafeeds was authorized by EU in 2017, increasing efforts have been made to identify the best insect species to substitute FM, and its highest dietary inclusion level (Gasco et al., 2020). Despite the current high market prices of insect meal compared to conventional protein sources (Arru et al., 2019), the insect production is a fast-growing sector, and several companies or start-ups have been recently founded in Europe. This may lead to increased price competitiveness in the near future. The present study clearly shows that the total substitution of FM by dTM can be achieved in European sea bass without impairing growth performance or condition factor and even improving FCR after 10 weeks of feeding. To the best of our knowledge, the maximum replacement of FM by full-fat IM, successfully tested in European sea bass, varied between 36% and 50%, in trials of similar duration (Gasco et al., 2016; Abdel-Tawwab et al., 2020). Skalli and Robin (2004) pointed out that growth impairment at higher FM replacement levels is primarily due to nutrient deficiencies in n-3 LC-PUFA, which are fixed for marine fish close to 0.7% on DM basis (Skalli and Robin, 2004). In fact, a previous study in sea bass with 71% FM replacement by TM did not fulfil this requirement, impairing growth (Gasco et al., 2016), whilst all experimental diets tested in the present study contained 3.8–4.3% DM n-3 LC-PUFA, probably due to the high quality of FO, and did not affect growth performance. In blackspot sea bream and gilthead sea bream (Sparus aurata), it was possible to replace FM by full-fat TM up to 49% and 74%, respectively, without affecting growth performance or FCR, after 18–23 weeks of feeding (Iaconisi et al., 2017; Piccolo et al., 2017). In red sea bream, the total replacement of FM by dTM was successfully achieved without affecting FCR and even increasing growth after 4 weeks of feeding (Ido et al., 2019). Contrarily, the use of defatted black soldier fly (Hermetia illucens) to replace FM at levels above 18% in turbot (Psetta maxima) and 35% in meagre (Argyrosomus regius), for 7–9 weeks, did not affect FCR, but impaired growth performance (Kroeckel et al., 2012; Guerreiro et al., 2020). Thus, optimal FM substitution levels could vary among fish, insect species, rearing conditions, processing methods of IM, and duration of the experimental trials making comparisons difficult (Osimani et al., 2016; Iaconisi et al., 2019). Therefore, standardization of insect protein production is needed to assure the correct formulation of diets not only for fish, but also for other animal species.
In the present study, up to 80% of FM replacement did not alter N intake, N retention or metabolic N losses. However, this trade-off was apparently altered in fish fed TM100; these fish conserved a higher N retention compared to control by reducing metabolic N losses. Digestibility disturbances with the use of IM are attributed to the chitin, which has been negatively correlated with protein digestibility in in vitro digestibility studies (Marono et al., 2015). Although chitinolytic activity was reported in some fish species (Gutowska et al., 2004; Krogdahl et al., 2005; Kawashima et al., 2016), there are evidences that high dietary chitin levels have negative impacts on fish growth and nutrient utilization (Alegbeleye et al., 2012; Kroeckel et al., 2012). In the present study, this negative impact seems to have apparently been mitigated by the low chitin content (4.8% DM) in dTM. Moreover, the integrity of anterior intestine was well preserved in all fish, without major morphological changes, with the exception of submucosa thickening in fish fed dTM. Li et al. (2020) have observed a higher degree of submucosa cellularity in the anterior intestine of seawater-phase Atlantic salmon (Salmo salar) fed a H. illucens based diet, but the submucosa of mid and posterior intestine remained unaltered after 8 weeks of feeding such diets to freshwater-phase fish (Li et al., 2019). According to Sitjà-Bobadilla et al. (2005), the increase in thickness of submucosa is usually due to infiltrations of granular eosinophil cells, but this could not be confirmed in the present study. In contrast to the present results, in rainbow trout (Oncorhynchus mykiss), the replacement of FM by 25–50% full-fat or 100% defatted H. illucens reduced villus length in the anterior intestine after 12–14 weeks of feeding (Dumas et al., 2018; Cardinaletti et al., 2019). These controversial results may be associated to different species, feeds and processing methods used for IM production, leading to different nutritional profiles.
It was also observed that P ADC increased from 62% in fish fed CTRL to 72–80% in those fed the dTM diets. This higher P digestibility resulted in a pronounced reduction of total P losses, which suggests that the use of dTM as a FM replacer in European sea bass could help reducing P output into the environment. Likewise, Wang et al. (2017) demonstrated in Nile tilapia that FM replacement by increasing levels of M. domestica from 18% to 100% resulted in lower concentrations of total phosphate in the water. This metabolic feature might be part of the adaptive response of fish facing a reduced dietary P supply. Indeed, P deficiencies are a common nutritional disturbance of FM replacement by alternative proteins in aquafeeds (Sugiura et al., 2004; Prabhu et al., 2014). In the case of IM, this is a relevant issue because the mineral content of this feedstuff is markedly lower than FM or other conventionally FM substitutes, such as plant proteins. Indeed, ash content was reduced herein from 11.7% in CTRL diet to 6.5% in TM100 diet.
With total FM replacement, the increase of dietary lipids and energy content was concomitant with an improved digestibility of lipids that would favor increased whole-body lipid content by the end of the growth trial. This trend was not found in previous European sea bass studies where FM was replaced by IM from 25% to 71% (Gasco et al., 2016; Magalhães et al., 2017; Abdel-Tawwab et al., 2020). Likewise, in rainbow trout, Rema et al. (2019) did not observe any impact of dTM on lipid digestibility and retention. Regardless of this, our data of tissue body fat depots suggest an enhanced flux of lipids toward liver and secondly mesenteric fat when FM is totally replaced by dTM. This assumption is supported by data on muscle lipid content, and circulating lipid levels that were positively correlated with HSI and VSI. This is not surprising since European sea bass is a fish with low to moderate lipid deposition rates in the fillet (Ballester-Lozano et al., 2016). Although lipid content of liver has not been assessed, the strong and positive correlation between circulating triglycerides and HSI, and the moderate and positive correlation between final whole-body lipids content, lipid gain and HSI may indicate liver steatosis which is a sign of deficiencies in minerals and n-3 LC-PUFA (Ballester-Lozano et al., 2015). In contrast, enhanced deposition rate in mesenteric fat depots is highly informative of P deficiencies (Sugiura et al., 2004). In any case, both mineral and lipid metabolism seem to be highly sensitive to FM replacement by IM, which might be exacerbated at long-term through the production cycle, especially in the case of lipid-enriched diets (see below).
Liver plays a major role in fish lipid metabolism, and its gene expression profile is greatly influenced by dietary composition and feeding levels (Monroig et al., 2018). In the present study, all diets were formulated with 12–13% of FO and the n-3 LC-PUFA levels (3.8–4.3% DM) were above recommended level for marine fish species (approximately 0.7% DM) (Skalli and Robin, 2004). When revisiting the gene expression profile of gilthead sea bream fed isolipidic diets with a maximal replacement of FO by vegetable oils or semisynthetic diets formulated to be deficient in n-3 LC-PUFA, the most responsive hepatic enzymes of lipid metabolism are scd1 and elovl6, and secondly fads2 (Benedito-Palos et al., 2016). This will contribute to mitigate the signs of deficiencies in n-3 LC-PUFA, though it is very important to limit hepatic lipogenesis to avoid the lipotoxic effects of excessive fat accumulation (Perera et al., 2019). Indeed, scd1 is the rate limiting enzyme in the synthesis of MUFA, especially oleic acid (OA, 18:1n-9) and palmitoleic acid (16:1n-7) from stearoyl-CoA and palmitoyl-CoA, respectively. Likewise, elovl6 is responsible for the elongation of SFA and MUFA of 12, 14 and 16 carbons to form 18-carbon FA (Weiss-Hersh et al., 2020). Therefore, the up-regulation of scd1b and elovl6 enhances the biosynthesis of MUFA, which in turn increases the unsaturation index of FA membrane phospholipids. This is reinforced by the recent observation of Perera et al. (2019) that scd1a is epigenetically regulated in gilthead sea bream by broodstock nutrition with alpha-linolenic acid (ALA, 18:3n-3) enriched diets. In the present study, no changes in ALA were found among diets, whilst both OA and linoleic acid (LA, 18:2n-6) were 3–7 times higher in TM100 than in the CTRL diet. The observed down-regulation of elovl6 in fish fed TM100, accompanied by a global decreasing trend of scd1b expression with FM replacement, support a reduced de novo lipogenic activity. In this scenario, the simultaneous up-regulated expression of fads2 in fish fed TM100 will serve to further desaturate PUFA, but the capacity of de novo synthesis of n-3 LC-PUFA is limited in marine fish and European sea bass in particular, due to the lack of elovl2 and reduced delta 5 desaturase activity (Monroig et al., 2018). Thus, although all dietary treatments provided European sea bass recommended n-3 LC-PUFA levels, TM100 diet may compromise the muscle n-3 LC-PUFA content at long term.
Cholesterol metabolism is also affected by FM replacement, and the hypocholesterolemic effect of plant proteins as FM replacers has been observed in a wide range of fish species, including gilthead sea bream (Gómez-Requeni et al., 2004; Benedito-Palos et al., 2016), European sea bass (Messina et al., 2013), rainbow trout (Romarheim et al., 2008) and salmon (Hartviksen et al., 2014). Likewise, feeding trials conducted with semisynthetic diets highlighted that FO replacement with vegetal oils acts lowering triglycerides and cholesterol in gilthead sea bream (Ballester-Lozano et al., 2015). In the same study, diets formulated for deficiencies in phospholipids and vitamins also showed a hypocholesterolemic effect, whereas P deficiency acted as a hypercholesterolemic factor. Since this same trend was found herein with FM replacement by dTM, the availability of bioactive P becomes again a limiting factor for the successful replacement of FM, though signs of growth and health impairment were mostly masked at short-term. The precise mechanism remains unknown, but it would be favored by the down-regulated expression of hepatic cyp7a1, the rate limiting enzyme of the bile acid synthesis pathway (Jelinek et al., 1990). This assumption was supported by the negative correlation between circulating cholesterol and the expression level of hepatic cyp7a1, as cyp7a1 deficiency has been reported to lead to hypercholesterolemia (Pullinger et al., 2002; Erickson et al., 2003; Qayyum et al., 2018). The ultimate physiological consequences of these findings remain elusive, but intriguingly the suppression of the hepatic cyp7a1 expression in mice fed cholesterolemic diets mainly involves the activation of inflammatory cytokines (Henkel et al., 2011).
Growth of fish is intrinsically related to protein deposition in muscle, which is regulated by systemic and local signaling pathways involving the GH/IGF system (Mommsen, 2001). In this study, no differences in the expression of gh receptors or igfs transcripts were observed among diets, which is in line with growth performance results. Nonetheless, myod2 plays a pivotal role in myoblast proliferation and differentiation (Valente et al., 2013), and its down-regulated expression in fish fed TM80 and TM100 should be indicative of some muscle growth derangement. Moreover, mstn is a negative regulator of muscle growth through inhibition of proliferation and differentiation of myogenic progenitor cells (Bonnieu et al., 2007), and its up-regulation coupled to the down-regulated expression of myod2 highly supports muscle growth inhibition at the transcriptional level. The extent to which this negative feature is counter-regulated by compensatory growth mechanisms remains uncertain, but the expression of key transcripts of the ubiquitin proteasome pathway, with a main role in the protein turnover of skeletal muscle (Foletta et al., 2011), remained unaltered (muscle RING-finger protein-1; murf1) or were even down-regulated (mafbx/atrogin-1) with the total FM replacement. Furthermore, a recent study in zebrafish demonstrated that mymk plays a pivotal role in myoblast fusion and consequently in muscle growth (Shi et al., 2018), and interestingly the expression of this growth-promoting factor was consistently up-regulated with the total FM replacement. The net result at short-term would be, thereby, the preservation of muscle growth with the use of IM as a main protein source in European sea bass. This was supported by the lack of changes in skeletal muscle cellularity and instrumental texture properties. To our best knowledge, the muscle cellularity of fish fed IM diets was never assessed before, but previous studies in different farmed fish species have evidenced the preservation of textural properties with high levels of FM replacement by IM (Lock et al., 2016; Borgogno et al., 2017; Iaconisi et al., 2017; Wang et al., 2017; Secci et al., 2019; Bruni et al., 2020).
In conclusion, the results of the present study demonstrate that FM replacement by dTM is largely feasible in European sea bass without detrimental effects on growth performance, nutrient utilization, intestinal integrity, and flesh nutritional and textural quality. Indeed, FM replacement by dTM resulted in fair levels of EPA and DHA in muscle of European sea bass (0.38–0.46 g/100 g WW), which are above those recommended by EFSA (2010) for human consumption to decrease the risk of cardiovascular diseases (0.25 g of EPA plus DHA per 100 g of fish). Also, most of the possible negative effects on P and lipid metabolism are largely mitigated at this high replacement level. Nonetheless, further research is needed to fully validate such nutritional approach at farm level throughout the production cycle, giving special attention to the total energy content of the diet since lipid-energized diets can exacerbate the disturbance of lipid metabolism. It is also important to mention that the reduction of P emissions to the environment, coupled with the reduction in the Fi:Fo ratio, prove that dTM is an environmentally sustainable alternative to FM.
Data Availability Statement
The raw data supporting the conclusions of this article will be made available by the authors, without undue reservation.
Ethics Statement
The animal study was reviewed and approved by IATS-CSIC and CSIC Review Boards, European (2010/63/EU) Animal Directives, and Spanish Laws (Royal Decree RD53/2013) on the handling of experimental animals.
Author Contributions
LV, JP-S, JC-G, and EM conceived and designed the study. AB, BO, LP, TS, MM, and SF conducted research, analyzed data, and performed statistical analysis. AB, JP-S, and LV wrote the article and had primary responsibility for final content. All authors read and approved the final manuscript.
Funding
This work was funded by the EU H2020 Research Innovation Program under the TNA Program (project AE090027) at IATS-CSIC Research Infrastructure within AQUAEXCEL2020 Project (652831). Additional financial support was received by the structured program of R&D&I ATLANTIDA - Platform for the monitoring of the North Atlantic Ocean and tools for the sustainable exploitation of the marine resources (reference NORTE-01-0145-FEDER-000040), supported by the North Portugal Regional Operational Programme (NORTE2020), through the European Regional Development Fund (ERDF) and by ANIMAL4AQUA Project, funded by Portugal 2020, financed by ERFD through COMPETE – POCI-01-0247-FEDER – 017610. This output reflects only the author’s view and the European Union cannot be held responsible for any use that may be made of the information contained herein. AB and MM were financially supported by FCT, Portugal (SFRH/BD/138593/2018 and DL57 – Norma transitória, respectively). Financial support from FCT – Foundation for Science and Technology within the scope of UIDB/04423/2020 and UIDP/04423/2020 is also acknowledged.
Conflict of Interest
EM was employed by the company SORGAL – Sociedade de Óleos e Rações, S.A., S. João de Ovar, Portugal.
The remaining authors declare that the research was conducted in the absence of any commercial or financial relationships that could be construed as a potential conflict of interest.
Supplementary Material
The Supplementary Material for this article can be found online at: https://www.frontiersin.org/articles/10.3389/fphys.2021.659567/full#supplementary-material
References
Abdel-Tawwab, M., Khalil, R. H., Metwally, A. A., Shakweer, M. S., Khallaf, M. A., and Abdel-Latif, H. M. R. (2020). Effects of black soldier fly (Hermetia illucens L.) larvae meal on growth performance, organs-somatic indices, body composition, and hemato-biochemical variables of European sea bass, Dicentrarchus labrax. Aquaculture 522, 735136. doi: 10.1016/j.aquaculture.2020.735136
Alegbeleye, W. O., Obasa, S. O., Olude, O. O., Otubu, K., and Jimoh, W. (2012). Preliminary evaluation of the nutritive value of the variegated grasshopper (Zonocerus variegatus L.) for African catfish Clarias gariepinus (Burchell. 1822) fingerlings. Aquacult. Res. 43, 412–420. doi: 10.1111/j.1365-2109.2011.02844.x
Antonopoulou, E., Nikouli, E., Piccolo, G., Gasco, L., Gai, F., Chatzifotis, S., et al. (2019). Reshaping gut bacterial communities after dietary Tenebrio molitor larvae meal supplementation in three fish species. Aquaculture 503, 628–635. doi: 10.1016/j.aquaculture.2018.12.013
AOAC, (2006). Official Methods of Analysis. Gaithersburg, MD: Association of Official Analytical Chemists.
Arru, B., Furesi, R., Gasco, L., Madau, F., and Pulina, P. (2019). The introduction of insect meal into fish diet: the first economic analysis on European sea bass farming. Sustainability 11:1697.
Ballester-Lozano, G. F., Benedito-Palos, L., Estensoro, I., Sitja-Bobadilla, A., Kaushik, S., and Perez-Sanchez, J. (2015). Comprehensive biometric, biochemical and histopathological assessment of nutrient deficiencies in gilthead sea bream fed semi-purified diets. Br. J. Nutr. 114, 713–726. doi: 10.1017/S0007114515002354
Ballester-Lozano, G. F., Benedito-Palos, L., Mingarro, M., Navarro, J. C., and Pérez-Sánchez, J. (2016). Up-scaling validation of a dummy regression approach for predictive modelling the fillet fatty acid composition of cultured European sea bass (Dicentrarchus labrax). Aquacult. Res. 47, 1067–1074. doi: 10.1111/are.12563
Basto, A., Matos, E., and Valente, L. M. P. (2020). Nutritional value of different insect larvae meals as protein sources for European sea bass (Dicentrarchus labrax) juveniles. Aquaculture 521:735085. doi: 10.1016/j.aquaculture.2020.735085
Batista, S., Pereira, R., Oliveira, B., Baião, L. F., Jessen, F., Tulli, F., et al. (2020). Exploring the potential of seaweed Gracilaria gracilis and microalga Nannochloropsis oceanica, single or blended, as natural dietary ingredients for European seabass Dicentrarchus labrax. J. Appl. Phycol. 32, 2041–2059. doi: 10.1007/s10811-020-02118-z
Benedito-Palos, L., Ballester-Lozano, G. F., Simó, P., Karalazos, V., Ortiz, Á, Calduch-Giner, J., et al. (2016). Lasting effects of butyrate and low FM/FO diets on growth performance, blood haematology/biochemistry and molecular growth-related markers in gilthead sea bream (Sparus aurata). Aquaculture 454, 8–18. doi: 10.1016/j.aquaculture.2015.12.008
Bolin, D. W., King, R. P., and Klosterman, E. W. (1952). A simplified method for the determination of chromic oxide (Cr2O3) when used as an index substance. Science 116, 634–635. doi: 10.1126/science.116.3023.634
Bonnieu, A., Carnac, G., and Vernus, B. (2007). Myostatin in the pathophysiology of skeletal muscle. Curr. Genomics 8, 415–422. doi: 10.2174/138920207783591672
Borgogno, M., Dinnella, C., Iaconisi, V., Fusi, R., Scarpaleggia, C., Schiavone, A., et al. (2017). Inclusion of Hermetia illucens larvae meal on rainbow trout (Oncorhynchus mykiss) feed: effect on sensory profile according to static and dynamic evaluations. J. Sci. Food Agric. 97, 3402–3411. doi: 10.1002/jsfa.8191
Bruni, L., Belghit, I., Lock, E. J., Secci, G., Taiti, C., and Parisi, G. (2020). Total replacement of dietary fish meal with black soldier fly (Hermetia illucens) larvae does not impair physical, chemical or volatile composition of farmed Atlantic salmon (Salmo salar L.). J. Sci. Food Agric. 100, 1038–1047. doi: 10.1002/jsfa.10108
Bruni, L., Pastorelli, R., Viti, C., Gasco, L., and Parisi, G. (2018). Characterisation of the intestinal microbial communities of rainbow trout (Oncorhynchus mykiss) fed with Hermetia illucens (black soldier fly) partially defatted larva meal as partial dietary protein source. Aquaculture 487, 56–63. doi: 10.1016/j.aquaculture.2018.01.006
Campos, I., Matos, E., Marques, A., and Valente, L. M. P. (2017). Hydrolyzed feather meal as a partial fishmeal replacement in diets for European seabass (Dicentrarchus labrax) juveniles. Aquaculture 476, 152–159. doi: 10.1016/j.aquaculture.2017.04.024
Cardinaletti, G., Randazzo, B., Messina, M., Zarantoniello, M., Giorgini, E., Zimbelli, A., et al. (2019). Effects of graded dietary inclusion level of full-fat Hermetia illucens prepupae meal in practical diets for rainbow trout (Oncorhynchus mykiss). Animals (Basel) 9:5. doi: 10.3390/ani9050251
Cho, C. Y., and Slinger, S. J. (1979). “Apparent digestibility measurement in feedstuffs for rainbow trout,” in Finfish Nutrition and Fishfeed Technology, eds J. E. Halver and K. Tiews, (Berlin: Heenemann), 239–247.
Choubert, G., Blanc, J. M., and Vallée, F. (1997). Colour measurement, using the CIELCH colour space, of muscle of rainbow trout, Oncorhynchus mykiss (Walbaum), fed astaxanthin: effects of family, ploidy, sex, and location of reading. Aquacult. Res. 28, 12–22.
Dumas, A., Raggi, T., Barkhouse, J., Lewis, E., and Weltzien, E. (2018). The oil fraction and partially defatted meal of black soldier fly larvae (Hermetia illucens) affect differently growth performance, feed efficiency, nutrient deposition, blood glucose and lipid digestibility of rainbow trout (Oncorhynchus mykiss). Aquaculture 492, 24–34. doi: 10.1016/j.aquaculture.2018.03.038
EFSA, (2010). Scientific opinion on dietary reference values for fats, including saturated fatty acids, polyunsaturated fatty acids, monounsaturated fatty acids, trans fatty acids, and cholesterol. EFSA J. 8:1461. doi: 10.2903/j.efsa.2010.1461.European
Erickson, S. K., Lear, S. R., Deane, S., Dubrac, S., Huling, S. L., Nguyen, L., et al. (2003). Hypercholesterolemia and changes in lipid and bile acid metabolism in mabolism in male and female cyp7A1-deficient mice. J. Lipid Res. 44, 1001–1009. doi: 10.1194/jlr.M200489-JLR200
European Commission, (2017). Commission Regulation (EU) 2017/893 of 24 May 2017 amending Annexes I and IV to Regulation (EC) No 999/2001 of the European Parliament and of the Council and Annexes X, XIV and XV to Commission Regulation (EU) No 142/2011 as regards the provisions on processed animal protein. Off. J. Eur. Union. 60, 92–116.
Eurostat, (2020). Production from Aquaculture Excluding Hatcheries and Nurseries (from 2008 Onwards). Available online at: https://appsso.eurostat.ec.europa.eu/nui/submitViewTableAction.do (acessed January 5, 2020).
Folch, J., Lees, M., and Sloane-Stanley, G. H. (1957). A simple method for the isolation and purification of total lipides from animal tissues. J. Biol. Chem. 226, 497–509.
Foletta, V. C., White, L. J., Larsen, A. E., Leger, B., and Russell, A. P. (2011). The role and regulation of MAFbx/atrogin-1 and MuRF1 in skeletal muscle atrophy. Pflugers Arch. Eur. J. Physiol. 461, 325–335. doi: 10.1007/s00424-010-0919-9
Gasco, L., Acuti, G., Bani, P., Dalle Zotte, A., Danieli, P. P., De Angelis, A., et al. (2020). Insect and fish by-products as sustainable alternatives to conventional animal proteins in animal nutrition. Ital. J. Anim. Sci. 19, 360–372. doi: 10.1080/1828051x.2020.1743209
Gasco, L., Henry, M., Piccolo, G., Marono, S., Gai, F., Renna, M., et al. (2016). Tenebrio molitor meal in diets for European sea bass (Dicentrarchus labrax L.) juveniles: growth performance, whole body composition and in vivo apparent digestibility. Anim. Feed Sci. Technol. 220, 34–45. doi: 10.1016/j.anifeedsci.2016.07.003
Gómez-Requeni, P., Mingarro, M., Calduch-Giner, J. A., Médale, F., Martin, S. A. M., Houlihan, D. F., et al. (2004). Protein growth performance, amino acid utilisation and somatotropic axis responsiveness to fish meal replacement by plant protein sources in gilthead sea bream (Sparus aurata). Aquaculture 232, 493–510. doi: 10.1016/s0044-8486(03)00532-5
Guerreiro, I., Castro, C., Antunes, B., Coutinho, F., Rangel, F., Couto, A., et al. (2020). Catching black soldier fly for meagre: growth, whole-body fatty acid profile and metabolic responses. Aquaculture 2:516. doi: 10.1016/j.aquaculture.2019.734613
Gutowska, M. A., Drazen, J. C., and Robison, B. H. (2004). Digestive chitinolytic activity in marine fishes of Monterey Bay, California. Comp. Biochem. Physiol. A Mol. Integr. Physiol. 139, 351–358. doi: 10.1016/j.cbpb.2004.09.020
Hartviksen, M., Bakke, A. M., Vecino, J. G., Ringo, E., and Krogdahl, A. (2014). Evaluation of the effect of commercially available plant and animal protein sources in diets for Atlantic salmon (Salmo salar L.): digestive and metabolic investigations. Fish Physiol. Biochem. 40, 1621–1637. doi: 10.1007/s10695-014-9953-4
Henkel, A. S., Anderson, K. A., Dewey, A. M., Kavesh, M. H., and Green, R. M. (2011). A chronic high-cholesterol diet paradoxically suppresses hepatic CYP7A1 expression in FVB/NJ mice. J. Lipid Res. 52, 289–298. doi: 10.1194/jlr.M012781
Henry, M. A., Gasco, L., Chatzifotis, S., and Piccolo, G. (2018). Does dietary insect meal affect the fish immune system? The case of mealworm, Tenebrio molitor on European sea bass, Dicentrarchus labrax. Dev. Comp. Immunol. 81, 204–209. doi: 10.1016/j.dci.2017.12.002
Iaconisi, V., Marono, S., Parisi, G., Gasco, L., Genovese, L., Maricchiolo, G., et al. (2017). Dietary inclusion of Tenebrio molitor larvae meal: effects on growth performance and final quality treats of blackspot sea bream (Pagellus bogaraveo). Aquaculture 476, 49–58. doi: 10.1016/j.aquaculture.2017.04.007
Iaconisi, V., Secci, G., Sabatino, G., Piccolo, G., Gasco, L., Papini, A. M., et al. (2019). Effect of mealworm (Tenebrio molitor L.) larvae meal on amino acid composition of gilthead sea bream (Sparus aurata L.) and rainbow trout (Oncorhynchus mykiss W.) fillets. Aquaculture 513:734403. doi: 10.1016/j.aquaculture.2019.734403
Ido, A., Hashizume, A., Ohta, T., Takahashi, T., Miura, C., and Miura, T. (2019). Replacement of fish meal by defatted yellow mealworm (Tenebrio molitor) larvae in diet improves growth performance and disease resistance in red seabream (Pargus major). Animals 9:3. doi: 10.3390/ani9030100
Jelinek, D. F., Andersson, S., Slaughter, C. A., and Russell, D. W. (1990). Cloning and regulation of cholesterol 7 alpha-hydroxylase, the rate-limiting enzyme in bile acid biosynthesis. J. Biol. Chem. 265, 8190–8197.
Kawashima, S., Ikehata, H., Tada, C., Ogino, T., Kakizaki, H., Ikeda, M., et al. (2016). Stomach chitinase from Japanese sardine Sardinops melanostictus: purification, characterization, and molecular cloning of chitinase Isozymes with a long linker. Mar. Drugs 14:22. doi: 10.3390/md14010022
Koutsos, L., McComb, A., Finke, M., and Stull, V. (2019). Insect composition and uses in animal feeding applications: a brief review. Ann. Entomol. Soc. Am. 112, 544–551. doi: 10.1093/aesa/saz033
Kroeckel, S., Harjes, A. G. E., Roth, I., Katz, H., Wuertz, S., Susenbeth, A., et al. (2012). When a turbot catches a fly: evaluation of a pre-pupae meal of the black soldier fly (Hermetia illucens) as fish meal substitute - growth performance and chitin degradation in juvenile turbot (Psetta maxima). Aquaculture 36, 345–352. doi: 10.1016/j.aquaculture.2012.08.041
Krogdahl, Å, Hemre, G. I, and Mommsen, T. P. (2005). Carbohydrates in fish nutrition: digestion and absorption in postlarval stages. Aquacult. Nutr. 11, 103–122. doi: 10.1111/j.1365-2095.2004.00327.x
Lands, B. (2014). Historical perspectives on the impact of n-3 and n-6 nutrients on health. Prog. Lipid. Res. 55, 17–29. doi: 10.1016/j.plipres.2014.04.002
Li, Y., Bruni, L., Jaramillo-Torres, A., Gajardo, K., Kortner, T. M., and Krogdahl, A. (2021). Differential response of digesta- and mucosa-associated intestinal microbiota to dietary insect meal during the seawater phase of Atlantic salmon. Anim. Microbiome 3:8. doi: 10.1186/s42523-020-00071-3
Li, Y., Kortner, T. M., Chikwati, E. M., Belghit, I., Lock, E. J., and Krogdahl, A. (2020). Total replacement of fish meal with black soldier fly (Hermetia illucens) larvae meal does not compromise the gut health of Atlantic salmon (Salmo salar). Aquaculture 520:734967. doi: 10.1016/j.aquaculture.2020.734967
Li, Y., Kortner, T. M., Chikwati, E. M., Munang’andu, H. M., Lock, E. J., and Krogdahl, A. (2019). Gut health and vaccination response in pre-smolt Atlantic salmon (Salmo salar) fed black soldier fly (Hermetia illucens) larvae meal. Fish Shellfish Immunol. 86, 1106–1113. doi: 10.1016/j.fsi.2018.12.057
Livak, K. J., and Schmittgen, T. D. (2001). Analysis of relative gene expression data using real-time quantitative PCR and the 2−ΔΔCT Method. Methods 25, 402–408. doi: 10.1006/meth.2001.1262
Lock, E. R., Arsiwalla, T., and Waagbø, R. (2016). Insect larvae meal as an alternative source of nutrients in the diet of Atlantic salmon (Salmo salar) postsmolt. Aquacult. Nutr. 22, 1202–1213. doi: 10.1111/anu.12343
Lopes, G., Castro, L. F. C., and Valente, L. M. P. (2017). Total substitution of dietary fish oil by vegetable oils stimulates muscle hypertrophic growth in Senegalese sole and the upregulation of fgf6. Food Func. 8, 1869–1879. doi: 10.1039/c7fo00340d
Magalhães, R., Sánchez-López, A., Leal, R. S., Martínez-Llorens, S., Oliva-Teles, A., and Peres, H. (2017). Black soldier fly (Hermetia illucens) pre-pupae meal as a fish meal replacement in diets for European seabass (Dicentrarchus labrax). Aquaculture 476, 79–85. doi: 10.1016/j.aquaculture.2017.04.021
Marono, S., Piccolo, G., Loponte, R., Di Meo, C., Attia, Y. A., Nizza, A., et al. (2015). In vitro crude protein digestibility of Tenebrio molitor and Hermetia illucens insect meals and its correlation with chemical composition traits. Ital. J. Anim. Sci. 14:3889. doi: 10.4081/ijas.2015.3889
Maynard, L. A., Loosli, J. K., Hintz, H. F., and Warner, R. G. (1979). Animal Nutrition. New York, USA.
Messina, M., Piccolo, G., Tulli, F., Messina, C. M., Cardinaletti, G., and Tibaldi, E. (2013). Lipid composition and metabolism of European sea bass (Dicentrarchus labrax L.) fed diets containing wheat gluten and legume meals as substitutes for fish meal. Aquaculture 37, 6–14. doi: 10.1016/j.aquaculture.2012.11.005
Mommsen, T. P. (2001). Paradigms of growth in fish. Comp. Biochem. Physiol. B Biochem. Mol. Biol. 129, 207–219. doi: 10.1016/S1096-4959(01)00312-8
Monroig, O., Tocher, D. R., and Castro, L. F. C. (2018). “Polyunsaturated fatty acid biosynthesis and metabolism in fish,” in Polyunsaturated Fatty Acid Metabolism, ed. G. C. Burdge, (Urbana, IL: AOCS Press), 31–60.
Nogales-Mérida, S., Gobbi, P., Józefiak, D., Mazurkiewicz, J., Dudek, K., Rawski, M., et al. (2018). Insect meals in fish nutrition. Rev. Aquac. 2018, 1–14. doi: 10.1111/raq.12281
Osimani, A., Garofalo, C., Milanović, V., Taccari, M., Cardinali, F., Aquilanti, L., et al. (2016). Insight into the proximate composition and microbial diversity of edible insects marketed in the European Union. Eur. Food Res. Technol. 243, 1157–1171. doi: 10.1007/s00217-016-2828-4
Perera, E. A.-O., Turkmen, S. A.-O., Simó-Mirabet, P. A.-O., Zamorano, M. A.-O., Xu, H. A.-O., Naya-Català, F. A.-O. X., et al. (2019). Stearoyl-CoA desaturase (scd1a) is epigenetically regulated by broodstock nutrition in gilthead sea bream (Sparus aurata). Epigenetics 19, 1559–2308. doi: 10.1080/15592294.2019.1699982
Piccolo, G., Iaconisi, V., Marono, S., Gasco, L., Loponte, R., Nizza, S., et al. (2017). Effect of Tenebrio molitor larvae meal on growth performance, in vivo nutrients digestibility, somatic and marketable indexes of gilthead sea bream (Sparus aurata). Anim. Feed Sci. Technol. 226, 12–20. doi: 10.1016/j.anifeedsci.2017.02.007
Prabhu, P. A. J., Schrama, J. W., and Kaushik, S. J. (2014). Mineral requirements of fish: a systematic review. Rev. Aquac. 8, 172–219. doi: 10.1111/raq.12090
Pullinger, C. R., Eng, C., Salen, G., Shefer, S., Batta, A. K., Erickson, S. K., et al. (2002). Human cholesterol 7alpha-hydroxylase (CYP7A1) deficiency has a hypercholesterolemic phenotype. J. Clin. Invest. 110, 109–117. doi: 10.1172/JCI15387
Qayyum, F., Lauridsen, B. K., Frikke-Schmidt, R., Kofoed, K. F., Nordestgaard, B. G., and Tybjćrg-Hansen, A. (2018). Genetic variants in CYP7A1 and risk of myocardial infarction and symptomatic gallstone disease. Eur. Heart J. 39, 2106–2116. doi: 10.1093/eurheartj/ehy068
Rema, P., Saravanan, S., Armenjon, B., Motte, C., and Dias, J. (2019). Graded incorporation of defatted yellow mealworm (Tenebrio molitor) in rainbow trout (Oncorhynchus mykiss) diet improves growth performance and nutrient retention. Animals (Basel) 9:4. doi: 10.3390/ani9040187
Rimoldi, S., Gini, E., Iannini, F., Gasco, L., and Terova, G. (2019). The effects of dietary insect meal from Hermetia illucens prepupae on autochthonous gut microbiota of rainbow trout (Oncorhynchus mykiss). Animals (Basel) 9:4. doi: 10.3390/ani9040143
Romarheim, O. H., Skrede, A., Penn, M., Mydland, L. T., and Krogdahl, Å, and Storebakken, T. (2008). Lipid digestibility, bile drainage and development of morphological intestinal changes in rainbow trout (Oncorhynchus mykiss) fed diets containing defatted soybean meal. Aquaculture 274, 329–338. doi: 10.1016/j.aquaculture.2007.11.035
Salter, A. M. (2019). Insect protein: a sustainable and healthy alternative to animal protein? J. Nutr. 149, 545–546. doi: 10.1093/jn/nxy315
Secci, G., Mancini, S., Iaconisi, V., Gasco, L., Basto, A., and Parisi, G. (2019). Can the inclusion of black soldier fly (Hermetia illucens) in diet affect the flesh quality/nutritional traits of rainbow trout (Oncorhynchus mykiss) after freezing and cooking? Int. J. Food Sci. Nutr. 70, 161–171. doi: 10.1080/09637486.2018.1489529
Shi, J., Cai, M., Si, Y., Zhang, J., and Du, S. (2018). Knockout of myomaker results in defective myoblast fusion, reduced muscle growth and increased adipocyte infiltration in zebrafish skeletal muscle. Hum. Mol. Genet. 27, 3542–3554. doi: 10.1093/hmg/ddy268
Sitjà-Bobadilla, A., Peña-Llopis, S., Gómez-Requeni, P., Médale, F., Kaushik, S., and Pérez-Sánchez, J. (2005). Effect of fish meal replacement by plant protein sources on non-specific defence mechanisms and oxidative stress in gilthead sea bream (Sparus aurata). Aquaculture 249, 387–400. doi: 10.1016/j.aquaculture.2005.03.031
Skalli, A., and Robin, J. H. (2004). Requirement of n-3 long chain polyunsaturated fatty acids for European sea bass (Dicentrarchus labrax) juveniles: growth and fatty acid composition. Aquaculture 240, 399–415. doi: 10.1016/j.aquaculture.2004.06.036
Stenberg, O. K., Holen, E., Piemontese, L., Liland, N. S., Lock, E. J., Espe, M., et al. (2019). Effect of dietary replacement of fish meal with insect meal on in vitro bacterial and viral induced gene response in Atlantic salmon (Salmo salar) head kidney leukocytes. Fish Shellfish Immunol. 91, 223–232. doi: 10.1016/j.fsi.2019.05.042
Sugiura, S. H., Hardy, R. W., and Roberts, R. J. (2004). The pathology of phosphorus deficiency in fish – a review. J. Fish Dis. 27, 255–265. doi: 10.1111/j.1365-2761.2004.00527.x
Tacon, A. G. J., and Metian, M. (2008). Global overview on the use of fish meal and fish oil in industrially compounded aquafeeds: trends and future prospects. Aquaculture 285, 146–158. doi: 10.1016/j.aquaculture.2008.08.015
Valente, L. M. P., Moutou, K. A., Conceição, L. E. C., Engrola, S., Fernandes, J. M. O., and Johnston, I. A. (2013). What determines growth potential and juvenile quality of farmed fish species? Rev. Aquac. 5, S168–S193. doi: 10.1111/raq.12020
Wang, L., Li, J., Jin, J. N., Zhu, F., Roffeis, M., and Zhang, X. Z. (2017). A comprehensive evaluation of replacing fishmeal with housefly (Musca domestica) maggot meal in the diet of Nile tilapia (Oreochromis niloticus): growth performance, flesh quality, innate immunity and water environment. Aquacult. Nutr. 23, 983–993. doi: 10.1111/anu.12466
Weiss-Hersh, K., Garcia, A. L., Marosvolgyi, T., Szklenar, M., Decsi, T., and Ruhl, R. (2020). Saturated and monounsaturated fatty acids in membranes are determined by the gene expression of their metabolizing enzymes SCD1 and ELOVL6 regulated by the intake of dietary fat. Eur. J. Nutr. 59, 2759–2769. doi: 10.1007/s00394-019-02121-2
Keywords: alternative protein sources, fatty acids profile, feedstuffs, insect meal, muscle cell proliferation and differentiation, nutrient utilization
Citation: Basto A, Calduch-Giner J, Oliveira B, Petit L, Sá T, Maia MRG, Fonseca SC, Matos E, Pérez-Sánchez J and Valente LMP (2021) The Use of Defatted Tenebrio molitor Larvae Meal as a Main Protein Source Is Supported in European Sea Bass (Dicentrarchus labrax) by Data on Growth Performance, Lipid Metabolism, and Flesh Quality. Front. Physiol. 12:659567. doi: 10.3389/fphys.2021.659567
Received: 27 January 2021; Accepted: 17 March 2021;
Published: 15 April 2021.
Edited by:
Enric Gisbert, Institute of Agrifood Research and Technology (IRTA), SpainReviewed by:
Silvia Torrecillas, University of Las Palmas de Gran Canaria, SpainMansour Torfi Mozanzadeh, South Iran Aquaculture Research Center, Iran
Copyright © 2021 Basto, Calduch-Giner, Oliveira, Petit, Sá, Maia, Fonseca, Matos, Pérez-Sánchez and Valente. This is an open-access article distributed under the terms of the Creative Commons Attribution License (CC BY). The use, distribution or reproduction in other forums is permitted, provided the original author(s) and the copyright owner(s) are credited and that the original publication in this journal is cited, in accordance with accepted academic practice. No use, distribution or reproduction is permitted which does not comply with these terms.
*Correspondence: Luisa M. P. Valente, bHZhbGVudGVAaWNiYXMudXAucHQ=