- 1Faculty of Sports Science, Ningbo University, Ningbo, China
- 2Laboratory of Exercise Biochemistry in Health, Graduate Program in Health Sciences, School of Medicine, Pontifícia Universidade Católica do Paraná, Curitiba, Brazil
- 3School of Agricultural Sciences and Rural Development (SASRD), Nagaland University, Medziphema, India
- 4School of Health and Life Sciences, University of the West of Scotland, Blantyre, United Kingdom
Taurine is a naturally occurring amino acid involved in various functions, including regulating ion channels, cell volume, and membrane stabilization. However, how this molecule orchestrates such functions is unknown, particularly the dose response in exercised muscles. Therefore, this review aimed to systematically review the dose response of taurine on both aerobic and strength exercise performance. In accordance with the Preferred Reporting Items for Systematic Reviews and Meta-Analyses (PRISMA) statement, relevant articles were sought on PubMed, Medline, Web of Science, and Google Scholar using related terms, including taurine, exercise performance, exercise, muscle, physical training, running, strength, endurance exercise, resistance exercise, aerobic exercise, and swimming. Ten articles were retrieved, reviewed, and subjected to systematic analysis. The following parameters were used to assess exercise performance in the selected studies: creatine kinase (CK), lactic acid dehydrogenase, carbohydrate, fat, glycerol, malondialdehyde, enzymatic antioxidants, blood pH, taurine level, and muscular strength. From the selected literature, we observed that taurine supplementation (2 g three times daily) with exercise can decrease DNA damage. Furthermore, 1 g of acute taurine administration before or after exercise can decrease lactate levels. However, acute administration of taurine (6 g) at a high dose before the start of exercise had no effect on reducing lactate level, but increased glycerol levels, suggesting that taurine could be an effective agent for prolonged activities, particularly at higher intensities. However, further studies are warranted to establish the role of taurine in fat metabolism during exercise. Finally, we observed that a low dose of taurine (0.05 g) before performing strength enhancing exercises can decrease muscular fatigue and increase enzymatic antioxidants.
Systematic Review Registration: http://www.crd.york.ac.uk/PROSPERO, PROSPERO (CRD42021225243).
Introduction
Taurine is a prominent free amino acid with little or no role in protein synthesis. However, it has multiple physiological roles, including interacting with ion channels, membrane stabilization, and cellular osmoregulation (Shihabi et al., 1989; Schaffer and Kim, 2018). The intracellular concentration ranges of taurine range approximately between 5 and 20 μmol/g in many tissues, particularly in excitable tissues, including skeletal muscle, heart, and brain (De Luca et al., 2015). The main sources of dietary taurine are meat, shellfish, sea vegetables, and dairy products. The biosynthesis of taurine is as follows: First, cysteine is oxidized by cysteine dioxygenase to form cysteine sulfinic acid. Next, decarboxylation of cysteine sulfinic acid by cysteine sulfinic acid decarboxylase occurs, producing hypotaurine; finally, the oxidation of hypotaurine to taurine. This pattern may be affected by the person's nutritional intake and the availability of its precursor cysteine (Ripps and Shen, 2012; De Luca et al., 2015). Exercise is a crucial factor that can influence the pattern of biosynthesis of taurine by oxidizing the precursor cysteine. For example, high-intensity-induced redox modification of cysteine can undergo several post-translational modifications to produce either muscular adaptation or fatigue, which affects taurine biosynthesis (Thirupathi et al., 2020). This scenario can demand additional taurine requirements from external sources. Taurine a potential role in preventing oxidative damage and restoring muscle function in muscular disorders (Ripps and Shen, 2012; De Luca et al., 2015; Thirupathi et al., 2020). Furthermore, taurine-regulated calcium homeostasis can increase calcium-binding proteins during muscle contraction, resulting in greater muscle strength and endurance (El Idrissi and Trenkner, 1999; Galloway et al., 2008; Thirupathi et al., 2018; Wen et al., 2019).
A person's physical condition and fitness level may also influence the availability of taurine in skeletal muscle (Henriksson, 1991; Graham et al., 1995). This may be due to structural differences in an individual's physical exercise threshold. There are marked differences between trained and untrained individuals, that is, after prolonged exercise, induced amino acid changes show higher levels of taurine in trained individuals (Henriksson, 1991; Graham et al., 1995). Animal studies have shown that taurine can regulate excitation contraction by altering chloride conductance (De Luca et al., 1996), and taurine knockout mice exhibit myofibril derangements consistent with exercise capacity impairment (Ito et al., 2010). Nor can we omit the role of taurine in energy metabolism, as shown in other studies (Mozaffari et al., 1986; Wen et al., 2019). For example, rats fed with the taurine inhibitor beta-alanine can stimulate the rate of glycolysis and lactate production, but this effect was independent of taurine depletion (Mozaffari et al., 1986; Gladden, 2004). However, this study did not verify the role of taurine during exercise. Exercise with higher intensities can affect the redox status of cells, but taurine may catalyze the positive effect of exercise by replenishing the thiol pool in skeletal muscle, as previously reported (Silva et al., 2011). Exercise induced thiol-disulfide exchange activates various redox-sensitive signaling pathways, including PGC-1 alpha, which can greatly influence taurine biosynthesis. Although taurine improved the contractile properties of muscle by reducing muscle soreness and oxidative damage (Hamilton et al., 2006; De Carvalho et al., 2017), the concentration of taurine required to elicit a clinically relevant improvement in muscle function remains ambiguous. Since taurine is described in the literature as one of the safest amino acids to use, the exact dosage and duration of effectiveness in humans requires careful attention. Furthermore, gaps in understanding the role of supplements such as taurine in exercise performance, remain significant. Therefore, we hypothesize that establishing a proper taurine dose will not only increase exercise performance, but also improve muscle function by altering various biochemical functions.
Methods
Search Strategy
In accordance with the guidelines for the Preferred Reporting Items for Systematic Reviews and Meta-Analyses (PRISMA) statement, a search for relevant articles was carried out on PubMed, Medline, ISI, Web of Science, and Google Scholar using a broad range of synonyms and related terms, namely taurine, exercise performance, exercise, muscle, physical training, running, strength, endurance exercise, resistance exercise, aerobic exercise, and swimming. To avoid the risk of missing relevant articles, additional papers were sought in the gray literature (i.e., generic Web search) and through the bibliography of previous reviews. One author ran the search and screened the initial titles after duplicates were removed. Other authors independently examined the search results in depth for potentially relevant articles. We included only papers published in peer-reviewed journals that reported findings from experimentally controlled human studies. We excluded articles such as unpublished papers, conference posters, or those reporting findings of non-experimental studies (e.g., pre-/post-intervention studies, case series, etc.). The first author's name, year of publication, sample of intervention, design and duration of the study, topic, type of intervention, outcome, assessment, and results were recorded using an electronic spreadsheet.
Inclusion and Exclusion Criteria
We performed the PICO acronym (participants, interventions, comparisons, outcomes) for inclusion, and the criteria are shown in Table 1. We included taurine studies involving exercise performance if they met the following eligibility criteria: (1) studies reporting participants on exercise programs, including running, swimming, and cycling with taurine supplementation; (2) search outputs that included only articles that were peer-reviewed and published in English language journals; (3) only parameters that were related to exercise performance were included as types of outcome measures, including oxygen uptake, oxidative stress, DNA damage, and energy metabolism parameters, including fat oxidation and glycerol levels.
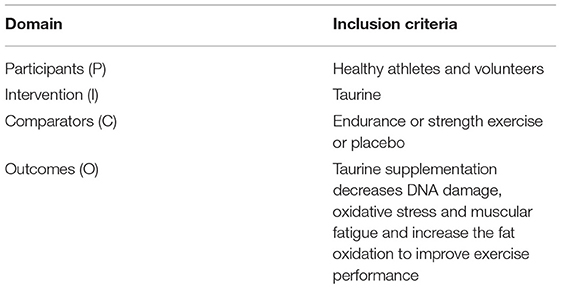
Table 1. Eligibility criteria in accordance with PICO (Participants, interventions, comparators, outcomes).
Full-Text Articles Selection
The abstracts of the articles were further refined using the following criteria: we included prospective cohort studies, cross-sectional studies, and randomized clinical trials. We excluded animal studies that used taurine supplementation to improve exercise performance.
Risk of Bias Assessment
The risk of bias assessment was independently performed by two authors based on the Cochrane risk of bias assessment tool. A third author was consulted if any disagreement arose.
Data Extraction and Analysis
For each study, the study characteristics (e.g., authors, nationality of the first author and published year), participant characteristics (e.g., number of participants, age, and gender), and intervention (taurine supplementation) were extracted and identified by an author and verified by another author based on taurine improving exercise performance. All parameters were evaluated based on blood and urine samples collected during or after the exercise program. Disagreements were resolved through discussion with other authors.
Results
Search Results
After a general search through PubMed, Scopus, and Google Scholar, 1,046 articles were identified from the initial database searches presented in Figure 1. After initial screening, the database was created based on the results of the selected articles' results (Table 2) and a total of 1,010 articles were excluded. The remaining 36 potential article abstracts were carefully evaluated, and a further 26 articles were excluded. The full text of the remaining 10 articles was retrieved, reviewed, and then included for systematic analysis.
Study Outcome
All the selected studies used taurine as supplements.
Risk of Bias in Included Studies
Of the 10studies included for systematic review, at least five had a risk of bias. Four studies had a high risk of blinding of the participants and outcome assessments (Table 3). Five studies had a high risk of randomization, and one had allocation concealment. All included studies had an unclear or low risk of incomplete outcome, selective reporting, and other forms of bias (Figure 2).
Exercise Performance Analysis With Taurine Administration
Although various roles of taurine have been reported in muscle, there is no specific mechanism reported, to-date, about taurine that can improve muscle performance and decrease muscle damage. Therefore, this review systematically analyzed the taurine dose response of improved exercise performance and decreasing muscle damage. For this reason, we selected 10studies that used taurine as a supplement to improve exercise performance (Table 4).
Aerobic Exercise Performance With Taurine
Among the 10 studies, nine used taurine supplementation to improve aerobic exercise performance. One study assessed the effects of taurine combined with low-fat chocolate milk supplementation, after exercise, on markers of muscle damage and inflammatory responses, including interleukin-6 (IL-6) and tumor necrosis factor-α (TNF-α) (Galan et al., 2018). Aerobic exercise-induced inflammatory markers have both positive and negative effects, including increased muscle damage. For example, aerobic exercise can release muscle-derived IL-6 into the circulation to maintain glucose homeostasis (Howlett et al., 1999; Pedersen et al., 2001). In contrast, elevated levels of TNF-α induce the formation of reactive oxygen species (ROS) and reduce muscle function, depending on exercise type and intensity (Mangner et al., 2013). In this study, taurine did not elevate IL-6 levels during endurance training, which implies that taurine has little or no role in IL-6-induced benefits (Mangner et al., 2013). However, endurance training showed that TNF-α levels did not significantly increase with taurine supplementation (Galan et al., 2018). This may be due to exercise type, intensity, duration, or the quantity of taurine administered. The blood lactate response to exercise is considered to be a normal variable to measure exercise performance. From the selected literature, six studies assessed blood lactate levels with taurine supplementation in endurance training (Balshaw et al., 2013; Milioni et al., 2016; Ward et al., 2016; De Carvalho et al., 2018; Kowsari et al., 2018). These studies demonstrated that acute taurine supplementation of 6 g/week before performing exercise had no effect on reducing blood lactate levels in endurance training, such as running or swimming (Milioni et al., 2016; De Carvalho et al., 2018), but it increased glycerol levels (De Carvalho et al., 2018). One g of acute taurine administration 5 times daily before and after exercise significantly decreased blood lactate levels (Kowsari et al., 2018). Different doses of taurine have different effects on increasing fat oxidation. For example, one study reported that 1.66 g of acute taurine administered before exercise increased fat oxidation (Rutherford et al., 2010), while a separate study reported that 6 g of taurine supplementation before a single bout of fasting aerobic exercise increased fat oxidation, but 3 g of taurine did not increase fat oxidation (Carvalho et al., 2020). After the first exercise, participants received 2 g of taurine three times daily during endurance training and exhibited decreased DNA damage (Zhang et al., 2004).
Strength Exercise Performance With Taurine
Strength exercise is more often characterized by a loading profile that combines high force and low fiber recruitment, which induces substantial mechanical stress, resulting in disruption of sarcomere contraction and excitation system failure. This scenario initiates injuries, including various biochemical and inflammatory alterations, and an increase in reactive oxygen species. One study used taurine supplementation (0.05 g) per day for 14 days and then performed eccentric strength exercise for 21 days with taurine supplementation. Taurine has been reported to improve enzymatic antioxidants and decrease muscular fatigue by reducing oxidative stress (da Silva et al., 2014). However, da Silva et al. (2014) reported that taurine did not have any effect on inflammation.
Discussion
This review systematically reported the dose response of taurine in improving exercise performance. We found from the selected literature that endurance training requires a higher dose of taurine, ~1 g five times daily, to prevent muscle-related damage whereas strength exercise requires a lower dose of taurine (0.05 g) to increase enzymatic antioxidants and decrease muscular fatigue.
Effects of Taurine Supplementation on Endurance Exercise Performance
Endurance performance is dependent not only on taurine dose, but also on age, gender, and duration of exercise (Balshaw et al., 2013; Ward et al., 2016; De Carvalho et al., 2018; Galan et al., 2018). Creatine kinase and blood lactate accumulation are important indicators for assessing muscle damage (Baird et al., 2012; Manojlović and Erčulj, 2019). From the selected literature, we observed that acute taurine supplementation (~1 g 5 times daily before and after exercise) can effectively reduce blood lactate levels during endurance training (Kowsari et al., 2018), while taurine supplementation before exercise (1 g) increased lactate levels during endurance training (Ward et al., 2016). However, acute administration of higher doses of taurine (6 g) during high-intensity endurance training had no effect on blood lactate levels (Milioni et al., 2016). This may be due to the blood buffering capacity of taurine (Nakada et al., 1992; Balshaw et al., 2013; Kowsari et al., 2018). Endurance training with taurine supplementation of 3 g daily immediately after exercise increased CK (Galan et al., 2018), which may be due to initial muscle damage caused by endurance exercise (Brancaccio et al., 2006). From these studies, we suggest that different exercise types require different doses of taurine to effectively improve exercise performance. Furthermore, 6 g of taurine per week can improve lipid metabolism by increasing glycerol in the plasma and muscle glycogen storage, indicating that high doses of taurine could be useful during high-intensity endurance exercises (De Carvalho et al., 2018). The intake of taurine at ~3,000–10,000 mg/day is safe for humans (Shao and Hathcock, 2008). The highest dose of taurine in humans in the pre-exercise condition was 3,000 mg (Eudy et al., 2013). Some studies have reported that taurine dose does not affect exercise performance (Zhang et al., 2004; Milioni et al., 2016; Ward et al., 2016; De Carvalho et al., 2018), suggesting that further studies regarding the taurine dose response of exercise, are warranted.
Effect of Taurine on Strength Exercise Performance
Strength exercise requires conflicting actions by which muscle length is increased while increasing mechanical stress. As a result, microstructural damage and further reduction in muscle contraction and excitation system occurs, and increased ROS production and activation of inflammatory cascades are also evident. Studies, including those undertaken by our group, have shown that taurine can increase muscle function, decrease oxidative damage, and increase antioxidants during strength exercise (Ra et al., 2016; Thirupathi et al., 2018). However, the mechanism by which taurine protects muscles from oxidative stress, remains unknown. Taurine decreases delayed oxidative stress and attenuates arterial stiffness after eccentric exercise through its antioxidant properties (Ra et al., 2016). This may be due to the role of taurine in nitric oxide production (Ra et al., 2016). Furthermore, taurine mitigates inflammation-induced secondary damage, which facilitates muscle recovery during strength exercise. We observed from the reviewed literature that taurine improves muscular strength and reduces muscle fatigue by reducing oxidative stress. However, there was no change in the inflammation status, which could be related to the intensity and duration of exercise. Other studies have also observed that taurine prevents oxidative stress without activating inflammation cascades during exercise (Khazaei, 2012; Shirvani et al., 2012).
Role of Taurine on Metabolism
Taurine improves metabolism, and taurine deficiency may reduce metabolic activity (Wen et al., 2019). Therefore, taurine could be a useful candidate for improving exercise performance because it may increase fat oxidation by altering intracellular and extracellular metabolic environments (Wolfe, 1998; de Bari et al., 2002; Zhang et al., 2004; Galloway et al., 2008) (Figure 3). Rutherford and colleagues have shown that taurine supplementation (1.66 g ingestion) increased fat oxidation by up to 16% in athletes during exercise, compared to placebo, and this may be due to the activation of adenylate cyclase and cyclic adenosine monophosphate (AMPc) (Shepherd et al., 1981; Rutherford et al., 2010; Pistor et al., 2014). Exercise with taurine improves energy expenditure and white adipose tissue metabolism, which supports the regulation of both body weight and exercise performance time. For example, De Carvalho et al., reported that exercise with taurine supplementation increased resting energy expenditure and released blood irisin in obese women (De Carvalho et al., 2021). De Carvalho et al. (2018) reported that taurine increases glycerol levels in plasma and improves lipolysis in healthy individuals (De Carvalho et al., 2018). Taurine supplementation with exercise may also increase mitochondrial activity and fatty acid oxidation by altering the gene expression of various enzymes and proteins, including peroxisome proliferator-activated receptor gamma coactivator 1-alpha (PGC-1α), lipoprotein lipase, acyl-CoA synthase, and acyl-CoA oxidase (ACOX), which are linked to lipid substrate oxidation (Murakami, 2015; Jia et al., 2016). De Carvalho et al. (2018) showed that taurine supplementation with exercise improved lipid metabolism by increasing mitochondrial respiratory capacity, uncoupling protein 1 (UCP1), and uncoupling protein 2 (UCP2), and increased the expression of fat oxidation-related genes, including aconitase 2 (ACO2) and ACOX1 in obese women (De Carvalho et al., 2021). These studies suggest a potential role for taurine in the regulation of energy metabolism in both active and obese individuals.
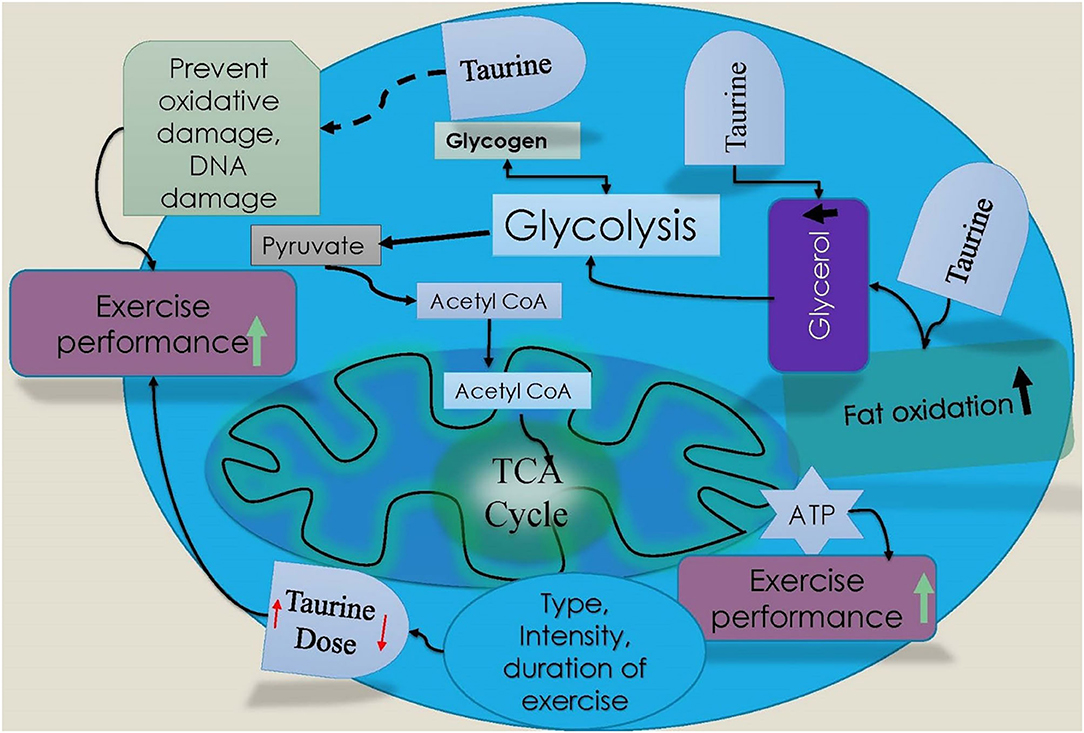
Figure 3. Schematic diagram of taurine effect on exercise performance. Taurine is involved increase in fatty acid oxidation and glycerol during exercise, which could increase performance during high intensity exercise. Taurine‘s (Red arrow marks indicate taurine dose increase or decrease for exercise performance) role in increasing exercise performance depends on type, intensity, and duration of exercise.
Limitation
A few factors that could limit the complete benefit of taurine in improving exercise performance include type of exercise, taurine dose, ingestion strategy, time of administration, biosynthesis, and antioxidant properties (Schaffer and Kim, 2018). For example, different doses elicit different effects during exercise. A high dose of taurine before exercise only had an effect on increasing glycerol, whereas taurine administration before strength exercises or during and after endurance exercise effectively reduced muscle damage by reducing DNA damage and oxidative stress. However, these studies did not evaluate direct DNA damage and oxidative damage markers, and this could be a limitation of those studies. Furthermore, a lower dose of taurine administered before undertaking strength exercise decreases muscle damage by attenuating oxidative stress, and this may be due to strength exercise-induced ROS formation, thereby preconditioning the exercise to adaptation. However, this must be established with studies involving a larger number of participants. In addition, excess availability of taurine as an antioxidant may interfere with the activity of mitochondrial respiration and adaptation induced by exercise. This scenario could limit the exercise-induced benefits, and this must be studied by fixing the taurine dose as low as possible, otherwise, it could limit the redox signaling benefits. Focusing on these aspects may effectively warrant the use of taurine to improve exercise performance.
Future Directions
For the past two decades, researchers have shown that exercise-induced benefits are linked to a person's nutritional uptake and health status. Taurine is a sulfur-containing free amino acid present in almost all excitable tissues, with concentration ranging from 5 to 20 μm/g. Since taurine was discovered in 1827, there has been much research investigating the mechanistic role of taurine in skeletal muscle and other systems, including antioxidation, Ca2+ homeostasis, and osmoregulation (Cozzoli et al., 2011; Terrill et al., 2016; Seidel et al., 2019). However, excess availability of taurine may blunt the ROS-induced benefits, as mentioned earlier. Conversely, increased contractile properties of muscles, particularly during high-intensity exercises, may affect the release of taurine into muscle fibers, especially when taurine depletion occurs among fast-twitch fibers, which ultimately compromises exercise performance (Galler et al., 1990; Tallon et al., 2007). In this scenario, taurine supplementation could be useful to compensate for the large loss of taurine. However, taurine administration as a supplement before, after, or during exercise, has a different effect, particularly on reducing muscle damage or muscle fatigue, which is a hallmark of limiting muscle performance and this correct dosage must be established by further study. However, designing and prescribing doses of taurine for effective and convincing outcomes remains difficult. Amino acids require several other supplemental conditions because of their first-pass effects and solubility constraints in crossing cell membranes. However, taurine is now regarded as an essential amino acid and perhaps a pharmaconutrient. In the future, further investigation may result in supportive evidence leading to taurine as a novel agent for such activities. Furthermore, taurine supplementation affects its concentration in blood plasma, and increased levels of taurine may be an indicator of muscle damage, muscle fatigue, or osmolarity adaptation in the whole blood, which can be misinterpreted during or after exercise. Therefore, establishing a method to determine specific taurine threshold values in the plasma that can either indicate muscle damage or are sensitive enough to increase its uptake to retain its cellular level, is a worthwhile study for the future. It is also important to explore methods that can increase muscle taurine concentrations following taurine supplementation. This may be achieved through the manipulation of dietary content or by establishing a method that could increase the cellular uptake of taurine by the specific transporter molecule taurine transporter (TauT) during exercise. One study has reported that exercise suppresses the expression of TauT (Han et al., 2006), future work focusing on increasing the availability of TauT in order to retain an optimal taurine concentration within cells is warranted.
Conclusions
This review systematically reviewed the effects of taurine on exercise performance. From the selected literature, different doses of taurine can increase lipid metabolism and decrease DNA damage during aerobic exercise. For example, acute administration of taurine (1.66 g) before performing exercise can increase lipid metabolism, and even higher doses of taurine (6 g) supplementation before a single bout of exercise can increase lipid metabolism. However, 3 g of taurine had no impact on lipid metabolism, which may be due to differences in the taurine kinetics of various tissues, which may fluctuate the release of taurine into the blood and further increase in blood taurine. Furthermore, after the first exercise, 2 g of taurine, 3 times a day, can decrease DNA damage. Regarding strength exercise, a low dose of taurine supplementation (0.05 g) with strength exercise improves enzymatic antioxidants and muscular fatigue by reducing exercise-induced oxidative stress.
Data Availability Statement
The original contributions presented in the study are included in the article/Supplementary Material, further inquiries can be directed to the corresponding authors.
Author Contributions
AT, QC, ZL, and YG conceived the original idea, developed the framework, and wrote the manuscript. AT, RP, UU, RG, and YG provided critical feedback and contributed to the final version. All authors were involved in the final direction of the paper and contributed to the final version of the manuscript. All authors have read and agreed to the published version of the manuscript.
Conflict of Interest
The authors declare that the research was conducted in the absence of any commercial or financial relationships that could be construed as a potential conflict of interest.
Publisher's Note
All claims expressed in this article are solely those of the authors and do not necessarily represent those of their affiliated organizations, or those of the publisher, the editors and the reviewers. Any product that may be evaluated in this article, or claim that may be made by its manufacturer, is not guaranteed or endorsed by the publisher.
Acknowledgments
The authors would like to acknowledge Kishore Kumar and Patric Francis Murphy, who helped with editing the manuscript.
Supplementary Material
The Supplementary Material for this article can be found online at: https://www.frontiersin.org/articles/10.3389/fphys.2021.700352/full#supplementary-material
References
Baird, M. F., Graham, S. M., Baker, J. S., and Bickerstaff, G. F. (2012). Creatine-kinase- and exercise-related muscle damage implications for muscle performance and recovery. J. Nutr. Metab. 2012:960363. doi: 10.1155/2012/960363
Balshaw, T. G., Bampouras, T. M., Barry, T. J., and Sparks, S. A. (2013). The effect of acute taurine ingestion on 3-km running performance in trained middle-distance runners. Amino Acids 44, 555–561. doi: 10.1007/s00726-012-1372-1
Brancaccio, P., Limongelli, F. M., and Maffulli, N. (2006). Monitoring of serum enzymes in sport. Br. J. Sport Med. 40, 96–97. doi: 10.1136/bjsm.2005.020719
Carvalho, M. B., Brandao, C. F. C., Fassini, P. G., Bianco, T. M., Batitucci, G., Galan, B. S. M., et al. (2020). Taurine supplementation increases post-exercise lipid oxidation at moderate intensity in fasted healthy males. Nutrients 12:1540. doi: 10.3390/nu12051540
Cozzoli, A., Rolland, J. F., Capogrosso, R. F., Sblendorio, V. T., Longo, V., Simonetti, S., et al. (2011). Evaluation of potential synergistic action of a combined treatment with alpha-methyl-prednisolone and taurine on the mdx mouse model of Duchenne muscular dystrophy. Neuropathol. Appl. Neurobiol. 37, 243–256. doi: 10.1111/j.1365-2990.2010.01106.x
da Silva, L. A., Tromm, C. B., Bom, K. F., Mariano, I., Pozzi, B., da Rosa, G. L., et al. (2014). Effects of taurine supplementation following eccentric exercise in young adults. Appl. Physiol. Nutri. Metab. 39, 101–104. doi: 10.1139/apnm-2012-0229
de Bari, L., Atlante, A., Guaragnella, N., Principato, G., and Passarella, S. (2002). D-Lactate transport and metabolism in rat liver mitochondria. Biochem. J. 365, 391–403. doi: 10.1042/bj20020139
De Carvalho, F. G., Barbieri, R. A., Carvalho, M. B., Dato, C. C., Campos, E. Z., Gobbi, R. B., et al. (2018). Taurine supplementation can increase lipolysis and affect the contribution of energy systems during front crawl maximal effort. Amino Acids 50, 189–198. doi: 10.1007/s00726-017-2505-3
De Carvalho, F. G., Brandao, C. F. C., Batitucci, G., Souza, A. O., Ferrari, G. D., Alberici, L. C., et al. (2021). Taurine supplementation associated with exercise increases mitochondrial activity and fatty acid oxidation gene expression in the subcutaneous white adipose tissue of obese women. Clin. Nutr. 40, 2180–2187. doi: 10.1016/j.clnu.2020.09.044
De Carvalho, F. G., Galan, B. S. M., Santos, P. C., Pritchett, K., Pfrimer, K., Ferriolli, E., et al. (2017). Taurine: a potential ergogenic aid for preventing muscle damage and protein catabolism and decreasing oxidative stress produced by endurance exercise. Front. Physiol. 20:710. doi: 10.3389/fphys.2017.00710
De Luca, A., Pierno, S., and Camerino, D. C. (1996). Effect of taurine depletion on excitation-contraction coupling and Cl- conductance of rat skeletal muscle. Eur. J. Pharmacol. 296, 215–222. doi: 10.1016/0014-2999(95)00702-4
De Luca, A., Pierno, S., and Camerino, D. C. (2015). Taurine: the appeal of a safe amino acid for skeletal muscle disorders. J. Transl. Med. 13, 243. doi: 10.1186/s12967-015-0610-1
El Idrissi, A., and Trenkner, E. (1999). Growth factors and taurine protect against excitotoxicity by stabilizing calcium homeostasis and energy metabolism. J. Neurosci. 19, 9459–9468. doi: 10.1523/JNEUROSCI.19-21-09459.1999
Eudy, A. E., Gordon, L. L., Hockaday, B. C., Lee, D. A., Lee, V., Luu, D., et al. (2013). Efficacy and safety of ingredients found in preworkout supplements. Am. J. Health Sys. Pharm. 70, 577–588. doi: 10.2146/ajhp120118
Galan, B. S., Carvalho, F. G., Santos, P. C., Pritchett, K., Pfrimer, K., Ferriolli, E., et al. (2018). Effects of taurine on markers of muscle damage, inflammatory response and physical performance in triathletes. J. Sport Med. Phys. Fit. 58, 1318–1324. doi: 10.23736/S0022-4707.17.07497-7
Galler, S., Hutzler, C., and Haller, T. (1990). Effects of taurine on Ca2(+)-dependent force development of skinned muscle fibre preparations. J. Exp. Biol. 152, 255–264. doi: 10.1242/jeb.152.1.255
Galloway, S. D., Talanian, J. L., Shoveller, A. K., Heigenhauser, G. J., and Spriet, L. L. (2008). Seven days of oral taurine supplementation does not increase muscle taurine content or alter substrate metabolism during prolonged exercise in humans. J. Appl. Physiol. (1985) 105, 643–651. doi: 10.1152/japplphysiol.90525.2008
Gladden, L. B. (2004). Lactate metabolism: a new paradigm for the third millennium. J. Physiol. 558, 5–30. doi: 10.1113/jphysiol.2003.058701
Graham, T. E., Turcotte, L. P., Kiens, B., and Richter, E. A. (1995). Training and muscle ammonia and amino acid metabolism in humans during prolonged exercise. J. Appl. Physiol. (1985) 78, 725–735. doi: 10.1152/jappl.1995.78.2.725
Hamilton, E. J., Berg, H. M., Easton, C. J., and Bakker, A. J. (2006). The effect of taurine depletion on the contractile properties and fatigue in fast-twitch skeletal muscle of the mouse. Amino Acids 31, 273–278. doi: 10.1007/s00726-006-0291-4
Han, X., Patters, A. B., Jones, D. P., Zelikovic, I., and Chesney, R. W. (2006). The taurine transporter: mechanisms of regulation. Acta Physiol. (Oxf) 187, 61–73. doi: 10.1111/j.1748-1716.2006.01573.x
Henriksson, J. (1991). Effect of exercise on amino acid concentrations in skeletal muscle and plasma. J. Exper. Biol. 160, 149–165. doi: 10.1242/jeb.160.1.149
Howlett, K., Febbraio, M., and Hargreaves, M. (1999). Glucose production during strenuous exercise in humans: role of epinephrine. Am. J. Physiol. 276, E1130–E1135. doi: 10.1152/ajpendo.1999.276.6.E1130
Ito, T., Oishi, S., Takai, M., Kimura, Y., Uozumi, Y., Fujio, Y., et al. (2010). Cardiac and skeletal muscle abnormality in taurine transporter-knockout mice. J. Biomed. Sci. 17:S20. doi: 10.1186/1423-0127-17-S1-S20
Jia, N., Sun, Q., Su, Q., Dang, S., and Chen, G. (2016). Taurine promotes cognitive function in prenatally stressed juvenile rats via activating the Akt-CREB-PGC1α pathway. Redox Biol. 10, 179–190. doi: 10.1016/j.redox.2016.10.004
Khazaei, M. (2012). Chronic low-grade inflammation after exercise: controversies. Iran. J. Basic. Med. Sci. 15, 1008–1009.
Kowsari, E., Moosavi, Z. A., Rahimi, A., Faramarzi, M., and Haghighi, M. M. (2018). The effect of short-term taurine amino acid supplement on neuromuscular fatigue, serum lactate level and choice reaction time after maximal athletic performance. J. Res. Med. Dent. Sci. 6, 358–364. doi: 10.24896/jrmds.20186158
Mangner, N., Linke, A., Oberbach, A., Kullnick, Y., Gielen, S., Sandri, M., et al. (2013). Exercise training prevents TNF-α induced loss of force in the diaphragm of mice. PLoS ONE. 8:e52274. doi: 10.1371/journal.pone.0052274
Manojlović, V., and Erčulj, F. (2019). Using blood lactate concentration to predict muscle damage and jump performance response to maximal stretch-shortening cycle exercise. J. Sports Med. Phys. Fitness 59, 581–586. doi: 10.23736/S0022-4707.18.08346-9
Milioni, F., Malta Ede, S., Rocha, L. G., Mesquita, C. A., de Freitas, E. C., and Zagatto, A. M. (2016). Acute administration of high doses of taurine does not substantially improve high-intensity running performance and the effect on maximal accumulated oxygen deficit is unclear. Appl. Physiol. Nutr. Metab. 41, 498–503. doi: 10.1139/apnm-2015-0435
Mozaffari, M. S., Tan, B. H., Lucia, M. A., and Schaffer, S. W. (1986). Effect of drug-induced taurine depletion on cardiac contractility and metabolism. Biochem. Pharmacol. 35, 985–989. doi: 10.1016/0006-2952(86)90087-0
Murakami, S. (2015). Role of taurine in the pathogenesis of obesity. Mol. Nutr. Food Res. 59, 1353–1363. doi: 10.1002/mnfr.201500067
Nakada, T., Hida, K., and Kwee, I. L. (1992). Brain pH and lactic acidosis: quantitative analysis of taurine effect. Neurosci. Res. 15, 115–123. doi: 10.1016/0168-0102(92)90024-7
Pedersen, B. K., Steensberg, A., and Schjerling, P. (2001). Muscle-derived interleukin-6: possible biological effects. J. Physiol. 536, 329–337. doi: 10.1111/j.1469-7793.2001.0329c.xd
Pistor, K. E., Sepa-Kishi, D. M., Hung, S., and Ceddia, R. B. (2014). Lipolysis, lipogenesis, and adiposity are reduced while fatty acid oxidation is increased in visceral and subcutaneous adipocytes of endurance-trained rats. Adipocyte 4, 22–31. doi: 10.4161/21623945.2014.955423
Ra, S. G., Choi, Y., Akazawa, N., Ohmori, H., and Maeda, S. (2016). Taurine supplementation attenuates delayed increase in exercise-induced arterial stiffness. Appl. Physiol. Nutr. Metab. 41, 618–623. doi: 10.1139/apnm-2015-0560
Ripps, H., and Shen, W. (2012). Review: taurine: a “very essential” amino acid. Mol. Vis. 18, 2673–2686.
Rutherford, J. A., Spriet, L. L., and Stellingwerff, T. (2010). The effect of acute taurine ingestion on endurance performance and metabolism in well-trained cyclists. Int. J. Sport Nutr. Exerc. Metab. 20, 322–329. doi: 10.1123/ijsnem.20.4.322
Schaffer, S., and Kim, H. W. (2018). Effects and mechanisms of taurine as a therapeutic agent. Biomol. Ther. (Seoul) 26, 225–241. doi: 10.4062/biomolther.2017.251
Seidel, U., Huebbe, P., and Rimbach, G. (2019). Taurine: a regulator of cellular redox homeostasis and skeletal muscle function. Mol. Nutr. Food Res. 63:e1800569. doi: 10.1002/mnfr.201800569
Shao, A., and Hathcock, J. N. (2008). Risk assessment for the amino acids taurine, L-glutamine and L-arginine. Regul. Toxicol. Pharmacol. 50, 376–399. doi: 10.1016/j.yrtph.2008.01.004
Shepherd, R. E., Noble, E. G., Klug, G. A., and Gollnick, P. D. (1981). Lipolysis and cAMP accumulation in adipocytes in response to physical training. J. Appl. Physiol. Respir. Environ. Exerc. Physiol. 50, 143–148. doi: 10.1152/jappl.1981.50.1.143
Shihabi, Z. K., Goodman, H. O., Holmes, R. P., and O'connor, M. L. (1989). The taurine content of avian erythrocytes and its role in osmoregulation. Comp. Biochem. Physiol. Part A: Physiol. 92, 545–549. doi: 10.1016/0300-9629(89)90363-0
Shirvani, H., Nikbakht, H., and Ebrahim Kh, G. A. (2012). The effects of soccer specific exercise and Taurine supplementation on serum cytokine response in male elite soccer players. Ann. Biol. Res. 3, 4420–4426.
Silva, L. A., Silveira, P. C., Ronsani, M. M., Souza, P. S., Scheffer, D., Vieira, L. C., et al. (2011). Taurine supplementation decreases oxidative stress in skeletal muscle after eccentric exercise. Cell. Biochem. Funct. 29, 43–49. doi: 10.1002/cbf.1716
Tallon, M. J., Harris, R. C., Maffulli, N., and Tarnopolsky, M. A. (2007). Carnosine, taurine and enzyme activities of human skeletal muscle fibres from elderly subjects with osteoarthritis and young moderately active subjects. Biogerontology 8, 129–137. doi: 10.1007/s10522-006-9038-6
Terrill, J. R., Grounds, M. D., and Arthur, P. G. (2016). Increased taurine in pre-weaned juvenile mdx mice greatly reduces the acute onset of myofibre necrosis and dystropathology and prevents inflammation. PLoS Curr. 29:8. doi: 10.1371/currents.md.77be6ec30e8caf19529a00417614a072
Thirupathi, A., Freitas, S., Sorato, H. R., Pedroso, G. S., Effting, P. S., Damiani, A. P., et al. (2018). Modulatory effects of taurine on metabolic and oxidative stress parameters in a mice model of muscle overuse. Nutrition 54, 158–164. doi: 10.1016/j.nut.2018.03.058
Thirupathi, A., Pinho, R. A., Baker, J. S., István, B., and Gu, Y. (2020). Taurine reverses oxidative damages and restores the muscle function in overuse of exercised muscle. Front. Physiol. 11:582449. doi: 10.3389/fphys.2020.582449
Ward, R., Bridge, C. A., McNaughton, L. R., and Sparks, S. A. (2016). The effect of acute taurine ingestion on 4-km time trial performance in trained cyclists. Amino Acids 48, 2581–2587. doi: 10.1007/s00726-016-2282-4
Wen, C., Li, F., Zhang, L., Duan, Y., Guo, Q., Wang, W., et al. (2019). Taurine is involved in energy metabolism in muscles, adipose tissue, and the liver. Mol. Nutr. Food Res. 63:e1800536. doi: 10.1002/mnfr.201800536
Wolfe, R. R. (1998). Fat metabolism in exercise. Adv. Exp. Med. Biol. 441, 147–156. doi: 10.1007/978-1-4899-1928-1_14
Keywords: taurine, exercise, muscle, oxidative stress, antioxidants
Citation: Chen Q, Li Z, Pinho RA, Gupta RC, Ugbolue UC, Thirupathi A and Gu Y (2021) The Dose Response of Taurine on Aerobic and Strength Exercises: A Systematic Review. Front. Physiol. 12:700352. doi: 10.3389/fphys.2021.700352
Received: 28 April 2021; Accepted: 28 July 2021;
Published: 18 August 2021.
Edited by:
Katsuhiko Suzuki, Waseda University, JapanReviewed by:
Shideh Narouei, Waseda University, JapanFlávia Giolo De Carvalho, University of São Paulo, Brazil
Copyright © 2021 Chen, Li, Pinho, Gupta, Ugbolue, Thirupathi and Gu. This is an open-access article distributed under the terms of the Creative Commons Attribution License (CC BY). The use, distribution or reproduction in other forums is permitted, provided the original author(s) and the copyright owner(s) are credited and that the original publication in this journal is cited, in accordance with accepted academic practice. No use, distribution or reproduction is permitted which does not comply with these terms.
*Correspondence: Anand Thirupathi, YW5hbnRoemVhbEBnbWFpbC5jb20=; Yaodong Gu, Z3V5YW9kb25nQG5idS5lZHUuY24=