- 1State Key Laboratory of Veterinary Etiological Biology, Key Laboratory of Veterinary Parasitology of Gansu Province, Lanzhou Veterinary Research Institute, Chinese Academy of Agricultural Sciences, Lanzhou, China
- 2Department of Preventive Veterinary Medicine, College of Veterinary Medicine, Northwest A&F University, Yangling, China
- 3Jiangsu Co-innovation Center for the Prevention and Control of Important Animal Infectious Disease and Zoonoses, Yangzhou University, Yangzhou, China
Necrotic enteritis (NE), caused by Clostridium perfringens, is an economically important disease in the broiler. Among normal flora in the broiler intestinal region, Clostridium butyricum has been identified as a probiotic agent that reduces the susceptibility of broilers to C. perfringens. However, the effects of C. butyricum supplement on broiler intestinal integrity during NE are largely unknown. In this study, we investigated the effects of C. butyricum on the growth performance, intestinal morphology and barrier function, and the functions of immune-related cytokines under NE in broilers. Chickens were divided into five groups: control group (NC), supplement C. butyricum only group (CB), NE-infected group (PC), supplement C. butyricum from Day 14 (NECB1) to Day 22 NE-infected group, and supplement C. butyricum from Day 1 (NECB2) to Day 22 NE-infected group. The results showed that there were significantly decreased average daily weight gain and increased feed conversion rate in the infected group (PC) compared with the C. butyricum-supplemented groups (NECB1 and NECB2) through the diet. Histopathological observation on the Hematoxylin–Eosin staining avian small intestine sections revealed that supplementation of C. butyricum (NECB1 and NECB2) could increase the intestinal villus height/crypt depth and lessen the intestinal damage under NE. ELISA and Limulus test showed that broilers infected with NE (PC) had higher serum IgA and lipopolysaccharide content; however, after C. butyricum supplementation (NECB1 and NECB2), they returned to a normal level. Furthermore, real-time PCR and Western blot results indicated that compared with PC, supplementing C. butyricum (NECB1 and NECB2) could initialize the expressions of genes related to the intestinal barrier-associated molecules (such as CLDN-1, CLDN-3, OCLN, MUC2, ZO-1, and CLDN5), cytokines (such as IL-10, IL-6, and TGFB1), and C. perfringens plc gene expression. Moreover, the results detected by the Ussing chamber suggested that C. butyricum (NECB1 and NECB2) could amend the decrease in conductivity value and short-circuit current value caused by NE. In addition, NECB2 significantly reduced the upregulation of fluorescein isothiocyanate–dextran flux caused by the NE disease. In conclusion, these findings suggest that dietary supplementation of C. butyricum in broilers with NE improved chicken growth performance, intestinal integrity and barrier function, and immunological status. Notably, no statistical difference was observed with the addition of C. butyricum on day 1 or day 14.
Introduction
Necrotic enteritis (NE) in broilers is caused by the strains of Clostridium perfringens types A, C, and G (Opengart and Boulianne, 2019) and is sometimes accompanied by co-infection with Eimeria maxima (Opengart and Songer, 2013). Clinically, broiler NE caused by C. perfringens is mainly characterized by mucosal necrosis of the small intestine. After the onset of the disease, the intestinal wall becomes thin and brittle, resulting in intestinal bleeding. In some severe cases, the intestinal cavity is filled with blood. Acute cases of NE in broilers are marked with diarrhea, anorexia, messy feathers, depression, bloody stools, and coal tar feces, resulting in yolk pedicle contraction, intestinal fibrosis, and necrosis. Broilers with NE may also develop the subclinical disease, with mild or no symptoms of diarrhea, and gradually progress to chronic wasting (Gholamiandehkordi et al., 2007; Timbermont et al., 2011). Although the mortality caused by NE in broilers with subclinical symptoms is not high, the NE in broilers results in severe economic losses for the poultry industry. This disease not only increases the mortality of the flock but also reduces the feed conversion rate directly caused by the severe damage to the intestines of broilers, delays the time to slaughter, and drives over 6 billion dollar losses in the poultry industry worldwide (Moore, 2016).
The use of antibacterial growth agents and anticoccidial drugs in the feed is essential in controlling poultry diseases. However, the widespread use of antimicrobials has also led to bacterial resistance and subsequently to restrictions or prohibitions on antibiotics in several countries around the world. Since the European Union started to ban antibiotic growth promoters in 2006 completely (EU, 2003), the incidence and prevalence of NE have increased significantly (Van Immerseel et al., 2009). Moreover, coccidiosis/coccidiasis, one of the main predisposing factors of NE, caused the incidence of C. perfringens-associated enteritis to increase yearly (Williams, 2005). Hence, alternatives to antibiotics, such as probiotics, have received significant attention in improving the broiler industry.
Probiotics can be used as growth promoters and, in some cases, can be used to control intestinal pathogens (Zhou et al., 2020). Moreover, probiotics can maintain normal intestinal function and regulate innate and adaptive immunity, and the epithelial barrier function (Choct, 2009; Viswanathan et al., 2009; Gaggìa et al., 2010).
Some probiotics have beneficial effects in broilers with NE, such as Lactobacillus acidophilus (Li et al., 2018) and Bacillus coagulans (Wu et al., 2018). Among the available probiotics, C. butyricum, a gram-positive anaerobic rod isolated from the intestines of healthy humans and animals, has many functions in animal breeding, such as regulating animal intestinal health (Liao et al., 2015). C. butyricum promotes the growth performance and immune function of broilers, and it is beneficial to balance the intestinal microbiota in broilers (Liao et al., 2015). Zhang et al. (2016) showed that the dietary supplementation of C. butyricum is beneficial to the maintenance of the intestinal barrier. C. butyricum also has a beneficial effect on broilers infected by NE (Huang et al., 2019). Some studies have also demonstrated that C. butyricum can induce short-chain fatty acids such as lactic acid and butyric acid in the digestive tract (Nakanishi et al., 2003). Moreover, the butyric acid produced by C. butyricum can increase the growth of beneficial bacteria and inhibit pathogenic bacteria, such as C. perfringens (Kong et al., 2011). Notably, the butyric acid also plays a vital role in the nutritional properties of epithelial cells and the inhibitory effect on pathogens in the intestine (Meimandipour et al., 2010); furthermore, it contributes to epithelial cell development in the intestine (Leeson et al., 2005). Butyric acid can also affect tight junction (TJ) expression and epithelial cell proliferation to maintain the structure of villus and intestinal integrity (Timbermont et al., 2010; Eeckhaut et al., 2011).
C. butyricum is a potent antibiotic substitute and an effective probiotic in livestock and poultry breeding. However, there are few reports on the effect of C. butyricum in broilers with C. perfringens-induced NE. Therefore, we evaluated the biological effects of C. butyricum on C. perfringens-infected broilers in terms of growth performance, secretion of serum IgA and lipopolysaccharide (LPS), intestinal barrier function, immune-related gene expression, and Fluorescein isothiocyanate–dextran (FD4) flux.
Materials and Methods
Chickens, Diet, and Experimental Design
Coccidia- and C. perfringens-free 1-day-old Ling-nan yellow-feathered broilers (Xinxisheng Biotechnology, China) were raised in the animal house of the Lanzhou Institute of Veterinary Medicine, Chinese Academy of Agricultural Sciences (LVRI, CAAS). E. maxima (GD strain) and C. perfringens strain toxinotype G (Hu et al., 2015) were isolated and maintained by the laboratory for swine/poultry intestinal infections and mucosal immunity of the LVRI, CAAS. C. butyricum (GCMCC0313.1) was acquired from Baifude Biotechnology (Wuxi, China). The feed used in this experiment was customized from Xinxiwang Feedstuff Company (Lanzhou, China) without any anticoccidial and growth-promotion antibiotic additives (Table 1). The animal cages in one house were divided into five groups, each with 10 broilers, including the negative control group (NC), C. butyricum group (CB), NE infection (PC), and two groups of NE broilers supplemented with C. butyricum from day 14 (NECB1), and from day 1 (NECB2). In addition, two groups (E. max and ECB) were set up with or without C. butyricum from day 1 and were administered with 3 ×104 E. maxima sporulated oocysts to exclude the effect of C. butyricum on E. maxima infection (Table 2).
For predisposing the pathogenesis of NE caused by C. perfringens infection, the stored E. maxima oocysts at 4°C were passaged using 14 day-old coccidia-free broiler chickens. The progeny oocysts of E. maxima were purified, sporulated, and counted according to standard approaches (Conway and McKenzie, 2007). For inoculating E. maxima oocysts through the crop infusion of the broiler to predispose the infection of C. perfringens, these purified oocysts should be stored at 4°C for up to 20 days.
Type G C. perfringens strain stored at −80°C was resuscitated in fluid thioglycollate medium (with 0.5% D-cycloserine) under an anaerobic culture system (Whitley DG250, UK, gas conditions as 80% N2, 10% H2, 10% CO2) at 37°C for 18 h. Then, the concentration of C. perfringens was adjusted to 5 × 108 CFU/mL using McFarland nephelometer (HuanKai Biotechnology Company, Guangdong, China) (Bollela et al., 1999) and real-time recombinase polymerase amplification (Xu et al., 2019).
The recommended dosage of C. butyricum powder for broiler chickens is 200–400 g/t compound feed. Before inoculation, we counted the living organisms in this product with the reference method in Veterinary Pharmacopeia of the People's Republic of China, to ensure enough designed dosage. The C. butyricum powder was directly mixed with feed in the recommended dosage (2 × 108 CFU/g) by a mechanical mixer and then put into the manger for eating by chickens ad libitum (Table 2). The infected groups (PC, NECB1, and NECB2) were orally administrated with 3 × 104 E. maxima sporulated oocysts in 1 mL of suspension on day 14 and orally inoculated three times a day for 3 days with 5 × 108 CFU/mL of C. perfringens on days 18, 19, and 20 (Table 2) (Xu et al., 2018). The uninfected groups (NC and CB) were administrated with the same amount of sterile saline at the corresponding times.
Ethics Statement
All animal experiments and experimental procedures were approved by the State Key Laboratory of Veterinary Etiological Biology, Lanzhou Veterinary Research Institute, Chinese Academy of Agricultural Sciences, China.
Growth Performance and Sample Collection
The body weight and feed consumption of the broilers were recorded on days 1, 13, and 22 for evaluating the average daily weight gain (ADG), average daily feed intake (ADFI), and feed conversion ratio (FCR) on days 13 and 22. The chickens were sacrificed by cervical dislocation on day 22, and then, blood samples were taken from the heart at once. The collected blood samples were incubated at 37°C for 1 h and then at 4°C for 10 h. Serum was isolated by centrifugation at 8,000 rpm for 10 min and stored at −80°C. The abdominal cavity was opened, and the small intestine lesions were examined. Then, the isolated intestine was cut longitudinally and photographed, and the intestinal contents were taken (quickly frozen in liquid nitrogen and stored at −80°C) for C. perfringens enumeration. The small intestine tissues in front of Meckel's diverticulum were cut into 2 cm in length, quickly frozen in liquid nitrogen, and then stored at −80°C for RNA and protein extraction. Additional pieces in the length of 1 cm were stored in 4% paraformaldehyde at 4°C for pathohistological examination. Moreover, the 1 cm length of small intestine tissue post the Meckel's diverticulum was taken and immediately used for Ussing chamber testing.
Intestinal Histomorphology
The small intestine samples fixed with 4% paraformaldehyde were then dehydrated, paraffin-embedded, sectioned to 4 μM using Leica DM2016 microtome (Wuhan Junjie Electronics, China), and fixed on the glass slide. The slices were stained with hematoxylin–eosin (HE, Servicebio, China) and then scanned with a panoramic slice scanner (3DHistech, Hungary). The height of the villus and the depth of crypts were measured.
Serum Immunoglobulin a Assay
The serum immunoglobulin A (IgA) was determined using a broiler immunoassay A ELISA (Cusabio, China). In brief, the references for the calibration curve and samples were added to every microplate at the same time, and each reference and samples have by triplicate biological replicates. Then, the absorbance was measured at 450 nm wavelength with a microplate reader (SpectraMax M5, Molecular Devices, United States) within 5 min after the reaction was terminated. After obtaining the absorbance value, a standard curve was generated to calculate the IgA concentration fold-change.
Serum LPS Assay
The serum LPS in broilers was detected using the Limulus test (Iwanaga, 2007) and with the LPS detection Tachypleus Amebocyte Lysate kit (Xiamen Bioendo Technology, China) following the kit manual. The absorbance value was measured at 405 nm, and a standard curve was generated to calculate the LPS concentration.
E. maxima Oocyst Output
To determine the number of E. maxima oocysts per gram of excreta, 10 g of fresh feces was collected from the four corners and the middle of each cage of E. max and ECB groups on day 7 after E. maxima infection. Briefly, 10 g of feces was soaked in 10 mL of tap water for 24 h at 4°C in a 50 mL beaker that is tightly covered with a lid, and then, the oocysts were counted according to a standard procedure (Conway and McKenzie, 2007).
Intestinal C. perfringens Enumeration
The genomic DNA of broiler gut microbiota was extracted using the stool genome extraction kit (Mobio12888, Qiagen, Germany). The obtained DNA was used as the template, and bacterial 16s rDNA was used as the house-keeping gene for detecting C. perfringens alpha-toxin gene, plc, expression (Table 3) with the quantitative PCR method (Nagpal et al., 2015). For plc quantitation, 10 μL of ChamQ SYBR qPCR Master Mix (Q311-02, Vazyme, China), 1 μL of forward primer, 1 μL of reverse primer, 2 μL of DNA, and 6 μL of ddH2O were added into one PCR tube. The amplification reaction was run according to the program of pre-denaturation at 95°C for 30 s, denaturation at 95°C for 10 s, annealing at 59°C for 30 s, and melting curve after 40 cycles on CFX96TM Real-time fluorescence quantitative PCR instrument (Bio-Rad, United States). The Ct value 2−ΔΔCt was calculated to analyze the relative expression difference.
RNA Extraction, cDNA Synthesis, and Real-Time PCR
RNA extraction was performed using TRIzol (ThermoFisher, United States) following the instructions of the manufacturer. Total RNA concentration was measured using NanoPhotometer NP80 (Implen, Germany) and reverse transcribed to cDNA using the Prime Script RT reagent kit (RR047A, Takara, Japan) as described in the manual. The obtained cDNA was stored in a freezer at −20°C.
Primers for real-time PCR designed by Beacon Designer 8 are shown in Table 3. The fluorescence quantitative kit instructions were followed to do this experiment (RR820A, Takara, Japan). The real-time PCR reaction tube contained 1 μL of forward or reverse primers, 1 μL of template DNA, 10 μL of TB Green enzyme, and water, with a total volume of 20 μL, and the mixture and the samples were thoroughly mixed. The reaction program was pre-denaturation at 95°C for 5 min, denaturation at 95°C for 30 s, and annealing at 59°C for 30 s. Forty cycles were performed, and the melting curve was inserted. The Ct value obtained from 2−ΔΔCt was used to analyze the relative expression difference.
Western Blot
Total protein was extracted according to the procedure for RIPA lysis buffer (P0013B, Beyotime, China). The total protein concentration was measured using the BCA kit (T9300A, Taraka, Japan). Protein was resolved on 12% SDS–PAGE gel and transferred to a polyvinylidene difluoride membrane (Merck Millipore, Germany). Skimmed milk powder (5%) at room temperature was used to seal the membrane and then incubated with the primary antibody consisting of GAPDH 1:10,000 (Proteintech, United States), CLDN-1 1:125 (ThermoFisher, United States), OCLN 1:125 (ThermoFisher, United States), and C. perfringens plc 1:500 (Bioss, China) overnight at 4°C. This process was followed by incubation using the secondary antibody (Qiyan Biotech, China) for 1 h at room temperature. After exposure to the electrochemiluminescence kit (Merck Millipore, Germany), the film obtained was scanned for protein band and analyzed using the ImageJ software.
Ussing Chamber Testing
With the salt bridge in place, the sample holder was empty as required, and 5 mL of Krebs–Henselet (K–H) solution containing 117 mM NaCl, 4.7 mM KCl, 1.2 mM MgSO4·7H2O, 1.2 mM KH2PO4, 2.418 mM NaHCO3, 11.1 mM GluCose, and 2.56 mM CaCl2 was added to each chamber, with the passage of oxygen to set the resistance and voltage to zero and turned on the VCC MC6 (Physiologic Instruments, United States). After debugging the instrument, the K–H solution was eliminated, the sample holder was removed, and then ~1 cm of the intestinal tissue was immediately supplied with oxygen (95% O2 and 5% CO2) at 4°C and pre-cooled with K–H solution after the longitudinal cut. The tissue was fixed in a 0.3 cm3 sample holder and filled with 5 mL of K–H solution, and the sample holder was reinstalled. The alternative values of short-circuit current value (Isc) and conductivity value (Gt) were recorded for 20 min, and when the curve was in a stable state, 100 μL of FD4 (0.375 mg/mL) solution was added to the mucosal side, while 100 μL of liquid was aspirated from the serosal side after 30 min (Hu et al., 2013). And a microplate reader was used to record the value of the excitation wavelength at 490 nm and emission wavelength at 520 nm.
Statistical Analysis
Average daily weight gain, feed consumption, villus height, crypt depth, and Ussing chamber testing data used the AOV function in the R package to perform two-way ANOVA and calculated the p value. Tukey multiple comparisons and graph drawing were run on GraphPad Prism (v 7.0). One-way ANOVA was used to analyze the differences between different treatment groups and group NC in the remaining data, and graphs were generated using the GraphPad Prism.
Results
Growth Performance
The growth performance of broilers was evaluated by the analysis of ADG, ADFI, and FCR values. At 14–22 days, the decrease of ADG and ADFI caused by NE was lessened by the supplementation of C. butyricum (NECB1 and NECB2) (Figures 1A,B). The increase of FCR in NE broilers was significantly reduced (p < 0.01) at 14–22 days in groups NECB1 and NECB2 compared with the PC group (Figure 1C).
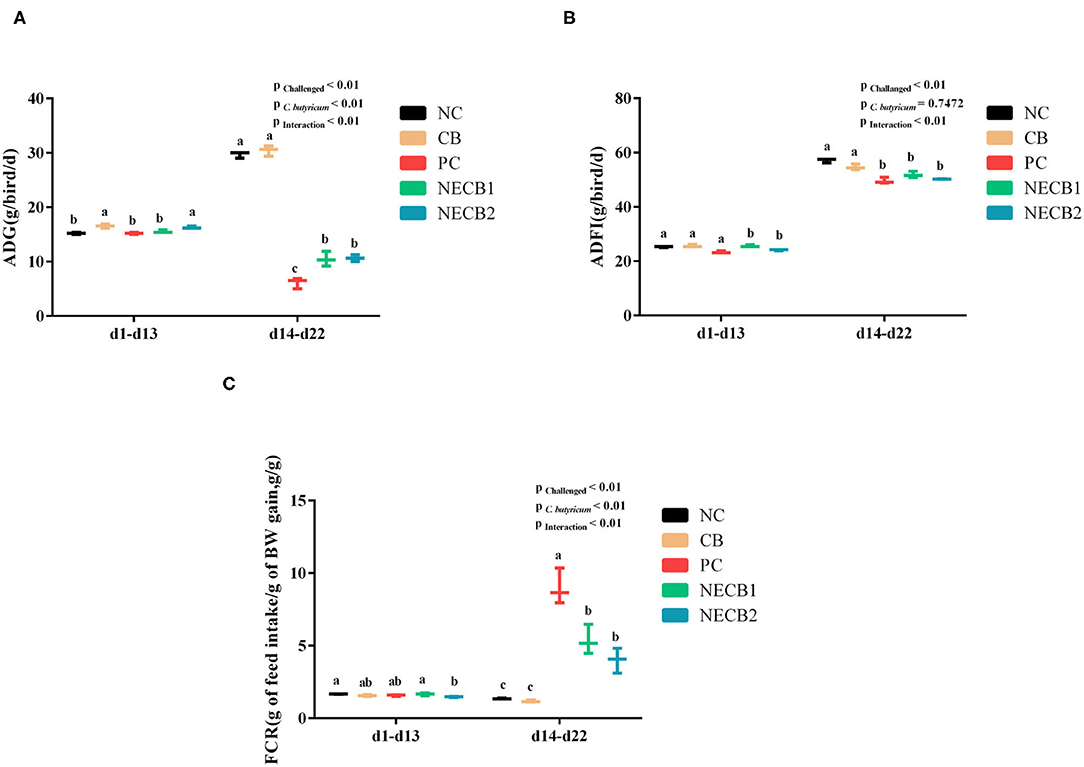
Figure 1. Effect of diet Clostridium butyricum supplementation on ADG, ADFI, and FCR of broilers infected with necrotic enteritis. (A) ADG: average daily weight gain. (B) ADFI: average daily feed intake. (C) FCR: feed conversion rate. Each result represents the mean ± SEM (n = 3). The different lowercase letters on the figure indicate significant differences (p < 0.05).
Pathohistological Examination
The intestinal macroscopic lesions are shown in Supplementary Figure 1. Compared with NC, the intestinal wall in the PC became thinner and brittle and had obvious bleeding points. The pathohistological visualizations are shown in Figure 2A. From that, we could find that the structure of the mucosal layer of the small intestine tissue was intact, and the intestinal cell or intestinal glands were abundant and arranged tightly in group NC. On the contrary, the villi in the intestinal epithelial cells fell off, and the lamina propria was exposed in PC. Furthermore, lamina propria capillaries (yellow arrow) were with mild congestion, and inflammatory cell infiltration was raised in the intestinal gland epithelium (red arrow) with increased diffuse lymphoid tissue (green arrow) and eosinophilic cytoplasm (blue arrow) in PC. However, in group NECB1, and similarly in group NECB2, although the intestinal villi shortened, more intestinal villi fused and a small amount of inflammatory cell infiltration in the intestinal gland epithelium was observed (red arrow).
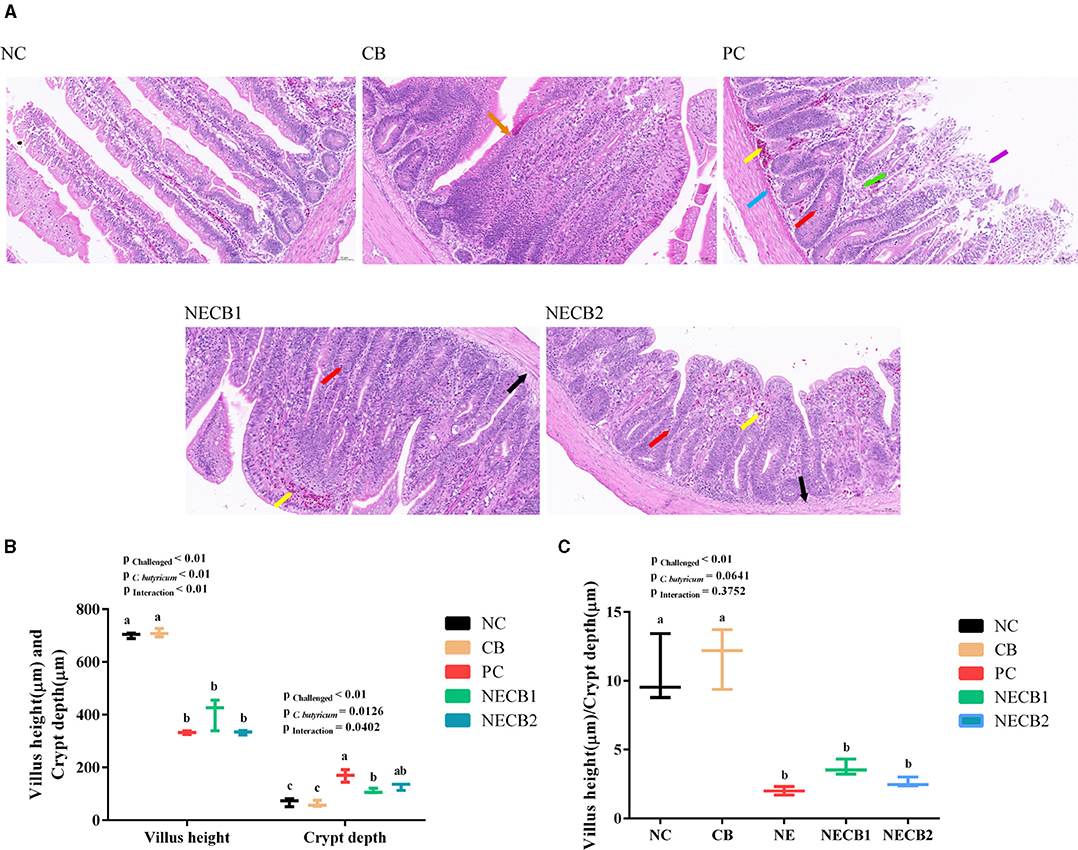
Figure 2. Effect of diet C. butyricum on the pathological section results, villus height, and crypt depth of necrotic enteritis-infected broilers. (A) Pathological section results. Yellow arrow: capillary congestion; red arrow: inflammatory cell infiltration; black arrow: swelling of mucosal muscularis cells, the cytoplasm is loose and lightly stained or vacuolated; blue arrow: eosinophils; orange arrow: intestinal villi merge; purple arrow: exposed lamina propria; and green arrow: increased diffuse lymphoid tissue. Scale bar=50 μm. (B) Villus height and crypt depth. (C) Villus height/crypt depth ratio. Each result represents the mean ± SEM (n = 3). The different lowercase letters on the figure indicate significant differences (p < 0.05).
The villus height, crypt depth, and their ratios were analyzed with Caseviewer software (Figures 2B,C), and it was observed that NE infection (PC) had a significant impact on the intestinal villus height and crypt depth (p < 0.01, p < 0.05). Compared with NC, NE infection (PC) could decrease significantly the villus height and increased significantly the crypt depth. However, C. butyricum supplement (NECB1 and NECB2) could reduce the decrease of villus height and increase of crypt depth caused by NE infection (PC). The villus height/crypt depth ratio (Figure 2C) revealed a significant interaction (p < 0.05) between the NE infection and the C. butyricum supplementation. However, it is notable that although supplementing C. butyricum (NECB1 and NECB2) could depress the declination of villus height/crypt depth ratio caused by NE infection (PC), no significant difference between NECB1 and/or NECB2 with PC was observed.
In the E. max group, a large number of shed mucosal epithelial cells in the intestinal lumen and eosinophils infiltrating (blue arrows) into the cavities of the intestinal villi were found. Moreover, a moderate inflammatory cell infiltration could also be detected in the mucosal epithelium and intestinal gland epithelium (red arrow). But in group ECB, there was only a moderate amount of inflammatory cell infiltration (red arrow) and little loss of mucosal epithelium at the top of the villi (Supplementary Figure 2B).
Serum IgA and LPS Content
Figures 3A,B presents the variations of serum IgA and LPS levels between the NC and the trial groups. In comparison with NC, although serum IgA was elevated in PC, this dynamic trend could be ameliorated by supplementing C. butyricum (NECB1 and NECB2), and there were no significant differences among them. Similarly, C. butyricum supplementation did not significantly lower the increase of serum LPS levels in PC.
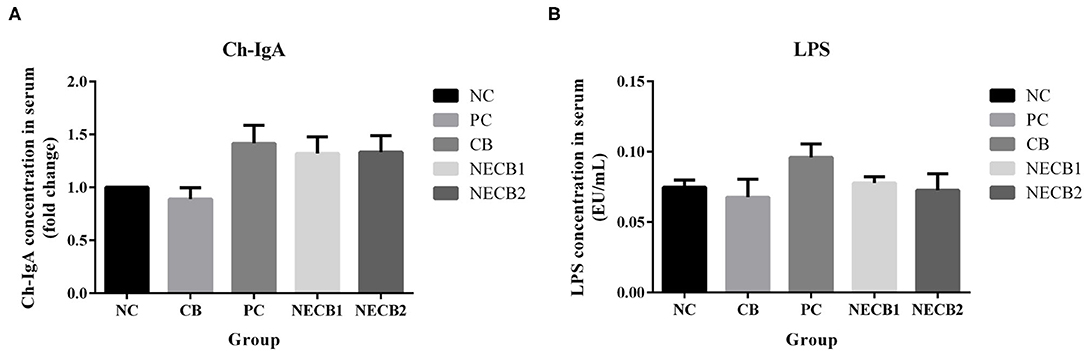
Figure 3. Effect of diet C. butyricum on serum immunoglobulin A (IgA) and lipopolysaccharide (LPS) in broilers infected with necrotic enteritis. (A) IgA. (B) LPS. Each result represents the mean ± SEM (n = 3).
E. maxima Oocyst Count
As compared with E. max, adding C. butyricum in the diet had no significant impacts on the E. maxima oocyst shedding in ECB (Supplementary Figure 3).
Intestinal Barrier Function-Related Genes, C. perfringens plc Gene, and Protein Expression
Necrotic enteritis infection resulted in the drop of TJ proteins, such as CLDN-1, CLDN-3, OCLN, and ZO-1, and mucosal barrier-associated protein MUC-2 mRNA expression; especially a significant fall in MUC-2, OCLN (p < 0.05), CLDN-1, and ZO-1 (p < 0.01) was observed (Figures 4A,B,D–F). However, elevatory CLDN-5 and significantly increased plc expression (p < 0.05) has been revealed in PC (Figures 4C,G). On the other hand, addition of C. butyricum (NECB1 and NECB2) retarded the decrease of CLDN-1, CLDN-3, MUC-2, OCLN, and ZO-1 caused by NE infection, although no significant differences were observed among them. Western blot results (Figure 5) showed that in PC, the expression of CLDN-1 and OCLN significantly decreased (p < 0.01) and increased the expression of plc. The addition of C. butyricum (CB) significantly increased the expression of CLDN-1 and OCLN contrasted with NC. Also, the addition of C. butyricum in NECB1 and NECB2 lightened the expression plc caused by NE.
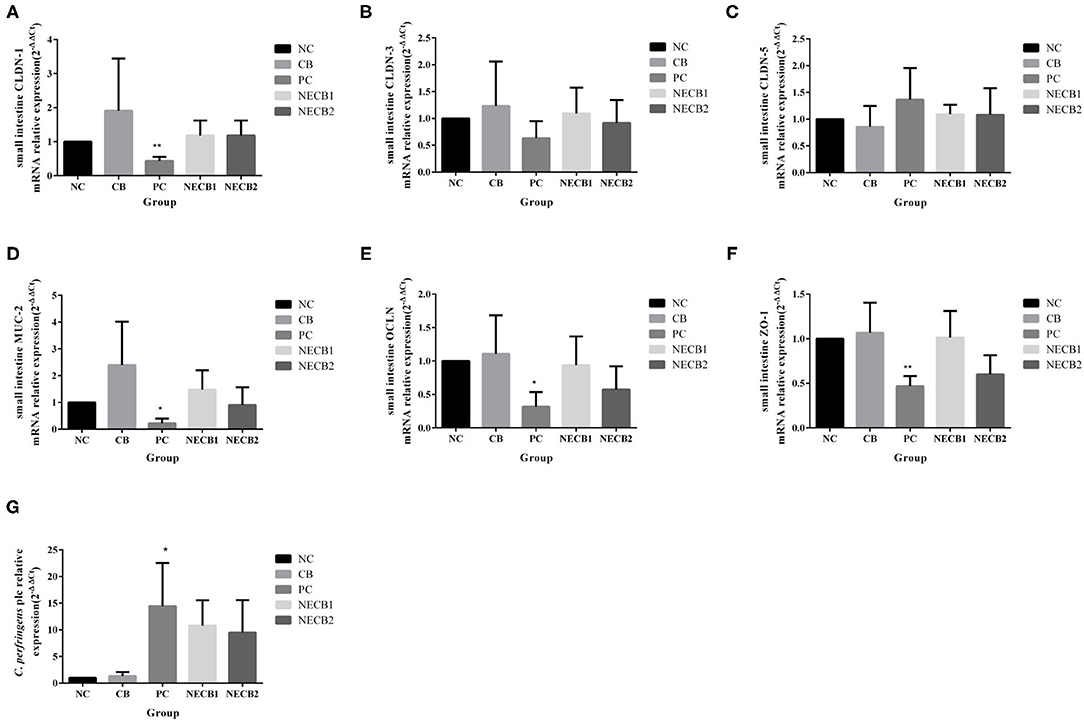
Figure 4. Relative mRNA expression intestinal barrier function-related in the small intestine and Clostridium perfringens plc gene in small intestine contents. (A) CLDN-1. (B) CLDN-3. (C) CLDN-5. (D) MUC-2. (E) OCLN. (F) ZO-1. (G) CPplc. Each result represents the mean ± SEM (n = 3). *Represent p < 0.05, **represent p < 0.01.
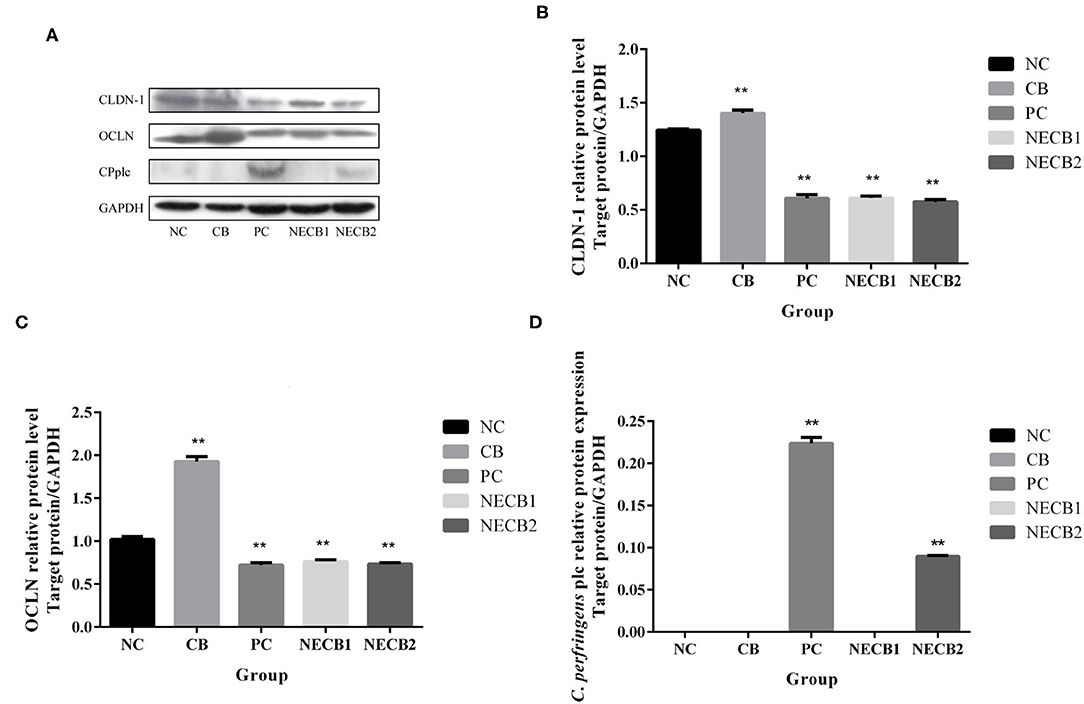
Figure 5. Protein expression in the small intestine. (A) Western blot analysis results of GAPDH, CLND-1, OCLN, CPplc. (B) CLDN-1. (C) OCLN. (D) CPplc. Each result represents the mean ± SEM (n = 3). **Represent p < 0.01.
Immune-Related Cytokines and Growth-Related Factor Gene Expression
Necrotic enteritis infection (PC) increased TGFB1 and immune-related factors, such as IL-10 and IL-6, compared with NC. However, supplementation of C. butyricum (NECB1 and NECB2) alleviated the upregulation of TGFB1, IL-10, and IL-6 in PC (Figure 6).
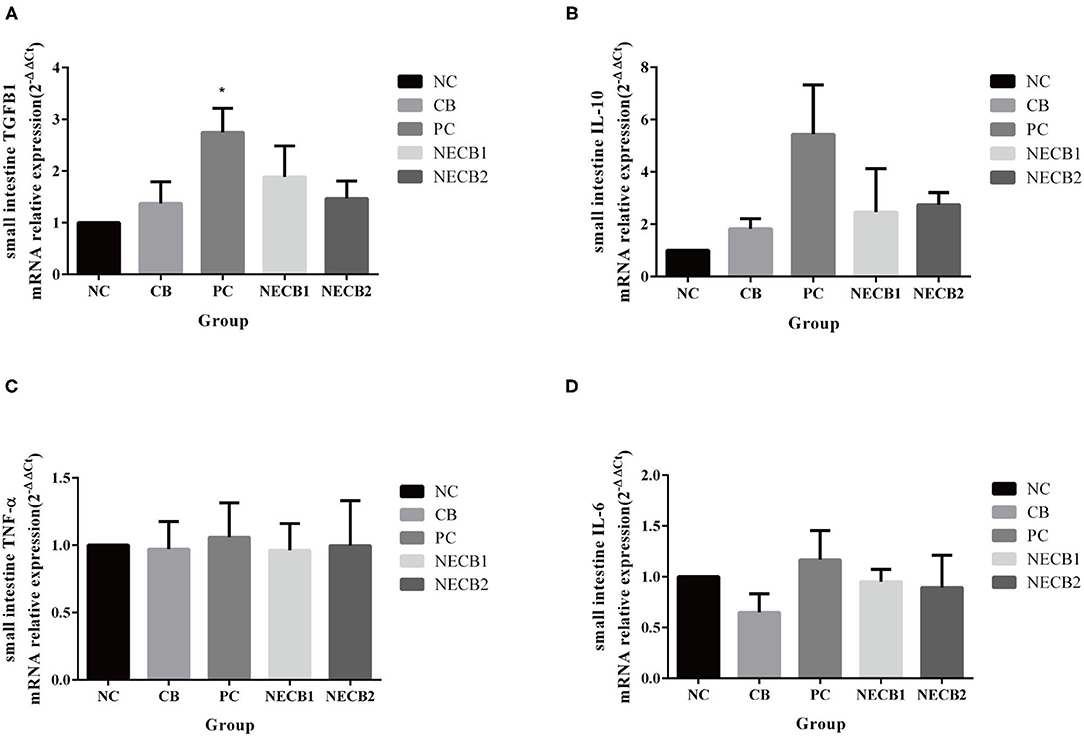
Figure 6. Effect of diet C. butyricum on the expression of cytokines related-genes in the small intestine of broilers infected with NE. (A) TGFB1. (B) IL-10. (C) TNF-α. (D) IL-6. Each result represents the mean ± SEM (n = 3). *Represent p < 0.05.
Data From Ussing Chamber Testing
In comparison with the NC group, there was no significant decrease of Gt in the PC group. The addition of C. butyricum prevented the decrease in Gt caused by NE, especially in NECB2, where Gt significantly increased (p < 0.01). The Isc of PC also decreased, and supplement C. butyricum (NECB1 and NECB2) could relieve the decrease of Isc caused by NE. In this case, no statistical difference was observed (NECB1 and NECB2, Figure 7A). Furthermore, the FD4 flux in PC increased, while C. butyricum addition in NECB2 could significantly inhibit the increase of FD4 flux caused by NE infection (p < 0.01). The fluorescence intensity of FD4 and the expression level of OCLN were inversely proportional in the NC, CB, and PC groups, but no such trend was observed in the NECB1 and NECB2 groups. Two-way analysis revealed that C. butyricum addition and NE infection at the same time affect the expression level of the protein OCLN, and the effect of interaction between C. butyricum and NE on OCLN expression was found to be significant (p < 0.01) (Figure 7B).
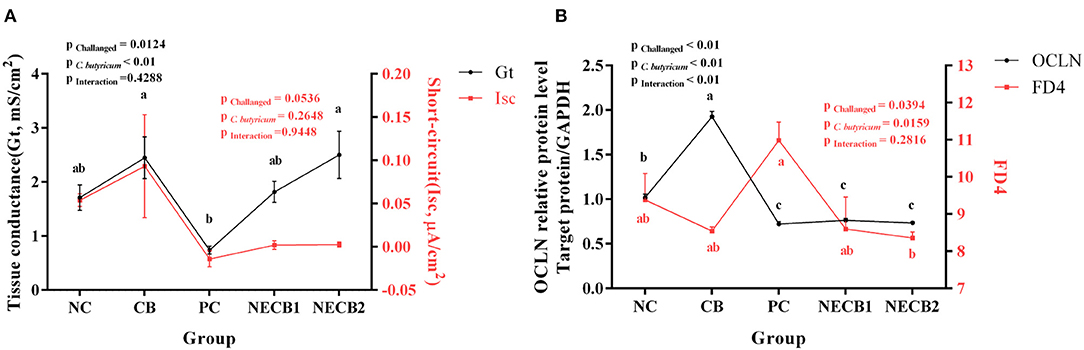
Figure 7. Ussing chamber experiment results. (A) Changes in short-circuit current (Isc) and conductance (Gt). (B) OCLN expression level and FD4 flux. Each result represents the mean ± SEM (n = 3). The different lowercase letters on the lines indicate significant differences (p < 0.05).
Discussion
Necrotic enteritis in broilers is caused by C. perfringens types A, C, and G, which shows necrotic lesions in the intestinal mucosa of the broilers, thus reducing the FCR and causing severe economic losses in the broiler industry. C. butyricum, as a probiotic, can maintain the growth performance of broilers (Leeson et al., 2005). The supplement of C. butyricum in feed can promote growth performance and increase feed utilization (Yang et al., 2012; Zhang et al., 2014), improve intestinal barrier functions, and play an antagonistic effect against C. perfringens (Yang et al., 2012), which will mitigate the lesion score of NE and ameliorate the decreased ADG and increased FCR in NE (Takahashi et al., 2018). Similarly, our results also suggested that C. butyricum supplementation could improve the ADG and FCR in NE models caused by C. perfringens under the predisposing E. maxima.
The intestinal tissue morphology, villus height, and crypt depth are important indicators for the measurement of intestinal health, injury, and recovery (Golder et al., 2011). Furthermore, the nutrient absorption in the intestine potentized with the increase of intestinal epithelial transport and the height of the villus (Ruhnke et al., 2015). Dietary supplementation with probiotics such as Lactobacillus acidophilus and C. butyricum enhanced the restoration of intestinal morphology due to the increase of villus height, and the decrease of crypt depth (Zhang et al., 2016; Chen et al., 2018; Li et al., 2018). In this study, the NE broilers showed severe hemorrhage in the small intestinal tissue, the proliferation of inflammatory cells, shortening of the villus, and an increase of crypt depth. By comparison, the selected indices such as small intestinal lesion and crypt depth were improved in the broilers fed with C. butyricum, suggesting that C. butyricum was beneficial to NE broilers to maintain intestinal function.
The concentration of LPS in serum was used to evaluate the intestinal permeability (Shini et al., 2008; Gilani et al., 2016), and it is an indicator for evaluating the integrity and function of the intestinal barrier (Li et al., 2018). Our results showed that the serum LPS was increased in NE broilers but was not increased in NE broilers fed with C. butyricum-added diet, suggesting that C. butyricum aided in the maintenance of the intestinal function in NE broilers. These results are consistent with previous reports (Zhang et al., 2016; Li et al., 2018).
The E. maxima oocyst shedding result was as described in other studies, which showed that the addition of acid preparations or probiotics can improve coccidiosis, but the inhibition of oocysts of Eimeria spp. was not completely (Giannenas et al., 2012; Tonda et al., 2018). C. butyricum maybe improve intestinal microbial and pH to decrease oocyst shedding, without directly affect E. maxima. Therefore, in this experiment, we thought there was still have an amount of E. maxima that can be used as an inducement to cause chicken NE.
Tight junction proteins are vital for the integrity and function of the epithelial barrier. They maintain the diffusion barrier and seal the intercellular space. OCLN is a TJ protein that acts as an adhesion molecule between cells to maintain and regulate the barrier function of TJs. CLDNs also play an essential role in regulating cellular signal transduction and paracellular transport in the intestinal epithelium (Krause et al., 2008). ZO proteins are located on the surface of the inner cytoplasmic membrane. ZO-1 is crucial because it connects TJ proteins and the actin backbone (Furuse et al., 1994). Alpha-toxin of C. perfringens can damage the intestinal mucosal barrier (Rehman et al., 2006), and the expression of TJ proteins such as CLDN-1, CLDN-3, OCLN, and ZO-1 after NE decreased to varying degrees (Song et al., 2017; Zhang et al., 2017; Wu et al., 2019). NE leads to the increase in mRNA expression in the pore-forming TJ protein CLDN-2 (Zhang et al., 2017). Similar to CLDN-2, CLDN-5 is a pore-forming protein. In the present study, we observed that CLDN-5 increased with C. perfringens-induced NE but with supplementation of C. butyricum, the mRNA expression of CLDN-5 decreased. This showed that the addition of C. butyricum removed the decreasing tendencies of CLDN-1, CLDN-3, OCLN, and ZO-1 expression because of NE, thus confirming the results of previous studies (Song et al., 2017; Huang et al., 2019). MUCs interact with IgA and various growth factors to maintain a relatively stable intestinal environment (Fukuda, 2002). MUCs have potential binding sites for pathogenic microorganisms, and colonization of some bacteria could induce change in the expression of MUCs (Van Klinken et al., 1995). Furthermore, MUCs could provide nutrition for the proliferation of C. perfringens (Deplancke et al., 2002). After broilers develop NE, the expression of MUCs such as MUC2 and MUC5AC changed (Forder et al., 2012; Wu et al., 2018). Butyric acid could increase the mRNA expression of MUC2 inhibited by NE (Song et al., 2017). Our data are in agreement with these results.
Although C. perfringens may be present in the normal flora of healthy broilers, it is generally believed that a rapid proliferation of C. perfringens caused by some predisposing factors such as coccidiasis/coccidiosis (Opengart and Boulianne, 2019) will lead to NE in chickens. In healthy birds, C. perfringens is present in the range of 102-104 CFU/g intestinal contents, but in NE, the concentration of C. perfringens increased to 107-109 CFU/g (Shojadoost et al., 2012). In our results, the C. perfringens plc increased due to NE, but the supplementation of probiotics could affect the increase in plc level. The possible reason is that C. butyricum exerts a competitive exclusion effect on C. perfringens, thus reducing the growth of C. perfringens (Takahashi et al., 2018). Otherwise, bacteriocin (Nakanishi and Tanaka, 2010) and short-chain fatty acids (Antonissen et al., 2016; Xu et al., 2018; Huang et al., 2019) produced by C. butyricum may also help to reduce the risk of C. perfringens overgrowth. This finding indicates that C. butyricum can reduce the proliferation rate of C. perfringens and the intestinal barrier damage caused by NE.
Cytokines regulate cell growth and immune response and participate in the inflammatory response. Among these cytokines, TGFB1 is related to mucosal immune tolerance. IL-10 is mainly secreted by T cells and is an important anti-inflammatory cytokine, and IL-10 has a barrier protective effect (Scumpia and Moldawer, 2005). IL-6 is an effective pro-inflammatory cytokine of Th1 cells, mainly secreted by the intestinal epithelial cells (Al-Sadi, 2009). At the instance of NE, we observed that the expression of anti-inflammatory factors TGFB1, IL-10, and pro-inflammatory cytokines IL-6 increased. However, supplementation of C. butyricum lowered the upregulation of TGFB1, IL-10, and IL-6 observed in NE infection. Our findings support the increase in cytokine secretion after adding probiotics or food additives that can reduce inflammation after pathogen infection in broilers and pigs (Chen et al., 2018; Cao et al., 2019; Fasina and Lillehoj, 2019; Zhang et al., 2019). This result shows that an increase of immune factors caused by NE owing to C. perfringens stimulates the inflammatory immune response in the intestine. Notably, although no significant differences in serum IgA levels between NC and testing groups were observed, a similar downtrend of serum IgA in C. butyricum addition for the groups infected with NE (NECB1 and NECB2) has been shown, which was also reported in Zhang et al.'s (Zhang et al., 2014) results and the case of C. butyricum for Escherichia coli K88 infection.
The Using chamber is a helpful tool for detecting the changes in the current and resistance of epithelial tissue by using electrodes to assess the integrity of the intestinal epithelial barrier. Evidence has been presented that after supplementing probiotic Yeast Saccharomyces boulardii in pig for 8 days, Gt remained unchanged, while Isc decreased, but Isc recovered after 16 days (Schroeder et al., 2004). Gt (tissue conductance) is a sign of tissue integrity, while Isc (short-circuit current) is a sign of net ion migration activity in the intestine, and the decrease of Gt and Isc indicates the closure of ion channels (Ruhnke et al., 2015). Previous work had reported that after broilers were infected with Salmonella or Campylobacter, Gt decreased because of the closure of ion channels, and the decrease of Gt was related to the decrease of net charge transfer of epithelial cells and the decrease in Isc (Awad et al., 2012, 2014). In the present study, Ussing chamber analysis showed that Gt was decreased in NE broilers, suggesting that the integrity of the intestinal epithelial barrier declined. Interestingly, the supplementation of C. butyricum could reverse the decrease in the values of Gt, signifying that C. butyricum can potentially maintain the integrity of the intestinal epithelial barrier in NE broilers. These results were in accordance with the expression patterns of CLDN-1, MUC-2, OCLN, and ZO-1 as indicated by the real-time PCR assay. However, some argue that the addition of organic acids did not affect the intestinal barrier (Ruhnke et al., 2015). The differential values of Gt and Isc in this study may be caused by the different dosages of C. butyricum. The FD4 flux between the intestinal epithelia mainly occurred through the cell bypass pathway. The increased flux of FD4 reflects the increased paracellular permeability and impaired intestinal barrier, which is inversely proportional to the OCLN expression level (Cani et al., 2009; Hu et al., 2013; Zhang et al., 2017). In the present study, when the C. perfringens infection-induced NE, the flux of FD4 increased. In contrast, the expression of OCLN decreased, indicating that the permeability of the intestine was increased, that is, the intestinal barrier was damaged. The addition of food additives such as L-arginine can inhibit the increase of FD4 flux caused by NE (Zhang et al., 2017). In the present study, the addition of C. butyricum showed the same result, indicating that C. butyricum can promote and maintain intestinal permeability of broilers.
Conclusions
Our results showed that supplementing C. butyricum in the broiler chicken diet would be beneficial to improve the production performance of broilers under the NE caused by C. perfringens, in which C. butyricum reduced the colonization of C. perfringens and ameliorated the intestinal barrier function and integrity damaged by C. perfringens. In conclusion, C. butyricum can enhance broiler intestinal health and serve as an effective probiotic in poultry farming.
Data Availability Statement
The raw data supporting the conclusions of this article will be made available by the authors, without undue reservation.
Ethics Statement
The animal study was reviewed and approved by State Key Laboratory of Veterinary Etiological Biology, Lanzhou Veterinary Research Institute, Chinese Academy of Agricultural Sciences, China.
Author Contributions
JC and XX designed the experiments. XX performed the experiments. SY provided the Ussing chamber method, and ZG and ZQ provided E. maxima oocyst (Guangdong strain). JW, YZ, HW, LX, and KZ participated in the animal experiments. XX wrote the manuscript. SY and JO helped in draft revision, and JC and EZ revised and finalized the manuscript. All authors approved the final version of the manuscript.
Funding
This work was supported by Key Technologies Research and Development Program (Key Technologies R&D Program) 2017YFD050040320 and the Innovative Special Project of Agricultural Science and Technology (Grant No. CAAS-ASTIP-2014-LVRI-09) for JC.
Conflict of Interest
The authors declare that the research was conducted in the absence of any commercial or financial relationships that could be construed as a potential conflict of interest.
Publisher's Note
All claims expressed in this article are solely those of the authors and do not necessarily represent those of their affiliated organizations, or those of the publisher, the editors and the reviewers. Any product that may be evaluated in this article, or claim that may be made by its manufacturer, is not guaranteed or endorsed by the publisher.
Supplementary Material
The Supplementary Material for this article can be found online at: https://www.frontiersin.org/articles/10.3389/fphys.2021.737481/full#supplementary-material
Supplementary Figure 1. Effect of diet C. butyricum on the macroscopic and pathological section results. (A) NC. (B) PC. (C) NECB2.
Supplementary Figure 2. Effect of diet C. butyricum on the macroscopic and pathological section results. (A) E. max. (B) ECB. Red arrow: inflammatory cell infiltration; Blue arrow: eosinophils. Scale bar = 50 μm.
Supplementary Figure 3. Effect of diet C. butyricum on E. maxima oocyst output. Each result represents the mean ± SEM (n = 3).
References
Al-Sadi, R. (2009). Mechanism of cytokine modulation of epithelial tight junction barrier. Front. Biosci. 14:2765. doi: 10.2741/3413
Antonissen, G., Eeckhaut, V., Van Driessche, K., Onrust, L., Haesebrouck, F., Ducatelle, R., et al. (2016). Microbial shifts associated with necrotic enteritis. Avian. Pathol. 45, 308–312. doi: 10.1080/03079457.2016.1152625
Awad, W. A., Aschenbach, J. R., Khayal, B., Hess, C., and Hess, M. (2012). Intestinal epithelial responses to Salmonella enterica serovar enteritidis: effects on intestinal permeability and ion transport. Poult. Sci. 91, 2949–2957. doi: 10.3382/ps.2012-02448
Awad, W. A., Molnár, A., Aschenbach, J. R., Ghareeb, K., Khayal, B., Hess, C., et al. (2014). Campylobacter infection in chickens modulates the intestinal epithelial barrier function. Innate. Immun. 21, 151–160. doi: 10.1177/1753425914521648
Bollela, V. R., Sato, D. N., and Fonseca, B. A. (1999). McFarland nephelometer as a simple method to estimate the sensitivity of the polymerase chain reaction using Mycobacterium tuberculosis as a research tool. Braz. J. Med. Biol. Res 32, 1073–1076. doi: 10.1590/S0100-879X1999000900003
Cani, P. D., Possemiers, S., Van de Wiele, T., Guiot, Y., Everard, A., Rottier, O., et al. (2009). Changes in gut microbiota control inflammation in obese mice through a mechanism involving GLP-2-driven improvement of gut permeability. Gut 58, 1091–1103. doi: 10.1136/gut.2008.165886
Cao, G., Tao, F., Hu, Y., Li, Z., Zhang, Y., Deng, B., et al. (2019). Positive effects of a Clostridium butyricum-based compound probiotic on growth performance, immune responses, intestinal morphology, hypothalamic neurotransmitters, and colonic microbiota in weaned piglets. Food. Funct. 10, 2926–2934. doi: 10.1039/C8FO02370K
Chen, L., Li, S., Zheng, J., Li, W., Jiang, X., Zhao, X., et al. (2018). Effects of dietary Clostridium butyricum supplementation on growth performance, intestinal development, and immune response of weaned piglets challenged with lipopolysaccharide. J. Anim. Sci. Biotechnol. 9:62. doi: 10.1186/s40104-018-0275-8
Choct, M. (2009). Managing gut health through nutrition. Br. Poultry Sci. 50, 9–15. doi: 10.1080/00071660802538632
Conway, D., and McKenzie, M. (2007). Poultry Coccidiosis: Diagnostic and Testing Procedures, 3rd Edn. Ames, IA: Blackwell. doi: 10.1002/9780470344620
Deplancke, B., Vidal, O., Ganessunker, D., Donovan, S. M., Mackie, R. I., and Gaskins, H. R. (2002). Selective growth of mucolytic bacteria including Clostridium perfringens in a neonatal piglet model of total parenteral nutrition. Am. J. Clin. Nutr. 76, 1117–1125. doi: 10.1093/ajcn/76.5.1117
Eeckhaut, V., Van Immerseel, F., Croubels, S., De Baere, S., Haesebrouck, F., Ducatelle, R., et al. (2011). Butyrate production in phylogenetically diverse Firmicutes isolated from the chicken caecum. Microb. Biotechnol. 4, 503–512. doi: 10.1111/j.1751-7915.2010.00244.x
EU (2003). Commission of the european of european communities, commission regulation (EU) no.1831/2003. Off. J. Eur. Union L. 268, 29–43. Available online at: https://ec.europa.eu/jrc/sites/default/files/EC-1831-2003.pdf
Fasina, Y. O., and Lillehoj, H. S. (2019). Characterization of intestinal immune response to Clostridium perfringens infection in broiler chickens. Poult. Sci. 98, 188–198. doi: 10.3382/ps/pey390
Forder, R. E., Nattrass, G. S., Geier, M. S., Hughes, R. J., and Hynd, P. I. (2012). Quantitative analyses of genes associated with mucin synthesis of broiler chickens with induced necrotic enteritis. Poult. Sci. 91, 1335–1341. doi: 10.3382/ps.2011-02062
Fukuda, M. (2002). Roles of mucin-type O-glycans in cell adhesion. BBA 1573, 394–405. doi: 10.1016/S0304-4165(02)00409-9
Furuse, M., Itoh, M., Hirase, T., Nagafuchi, A., Yonemura, S., Tsukita, S., et al. (1994). Direct association of occludin with ZO-1 and its possible involvement in the localization of occludin at tight junctions. J. Cell. Biol. 127, 1617–1626. doi: 10.1083/jcb.127.6.1617
Gaggìa, F., Mattarelli, P., and Biavati, B. (2010). Probiotics and prebiotics in animal feeding for safe food production. Int. J. Food. Microbiol. 141, S15–S28. doi: 10.1016/j.ijfoodmicro.2010.02.031
Gholamiandehkordi, A. R., Timbermont, L., Lanckriet, A., Van Den Broeck, W., Pedersen, K., Dewulf, J., et al. (2007). Quantification of gut lesions in a subclinical necrotic enteritis model. Avian. Pathol. 36, 375–382. doi: 10.1080/03079450701589118
Giannenas, I., Papadopoulos, E., Tsalie, E., Triantafillou, E., Henikl, S., Teichmann, K., et al. (2012). Assessment of dietary supplementation with probiotics on performance, intestinal morphology and microflora of chickens infected with Eimeria tenella. Vet Parasitol 188, 31–40. doi: 10.1016/j.vetpar.2012.02.017
Gilani, S., Howarth, G. S., Kitessa, S. M., Forder, R. E. A., Tran, C. D., and Hughes, R. J. (2016). New biomarkers for intestinal permeability induced by lipopolysaccharide in chickens. Anim. Prod. Sci. 56:1984. doi: 10.1071/AN15725
Golder, H. M., Geier, M. S., Forder, R. E. A., Hynd, P. I., and Hughes, R. J. (2011). Effects of necrotic enteritis challenge on intestinal micro-architecture and mucin profile. Br. Poultry Sci. 52, 500–506. doi: 10.1080/00071668.2011.587183
Hu, C. H., Xiao, K., Luan, Z. S., and Song, J. (2013). Early weaning increases intestinal permeability, alters expression of cytokine and tight junction proteins, and activates mitogen-activated protein kinases in pigs1. J. Anim. Sci. 91, 1094–1101. doi: 10.2527/jas.2012-5796
Hu, H., Qin, Z. H., Wang, Y. H., Gong, Z. X., Liu, B. H., Yin, H., et al. (2015). Regional variations of toxinotypes and drug-resistance profiles in Clostridium perfringens strains isolated from broiler chickens in China. Anim. Husbandry Vet. Med. 47, 28–34. (in Chinese).
Huang, T., Peng, X. Y., Gao, B., Wei, Q. L., Xiang, R., Yuan, M. G., et al. (2019). The effect of clostridium butyricum on gut microbiota, immune response and intestinal barrier function during the development of necrotic enteritis in chickens. Front. Microbiol. 10:2309. doi: 10.3389/fmicb.2019.02309
Iwanaga, S. (2007). Biochemical principle of Limulus test for detecting bacterial endotoxins. Proc. Jpn. Acad. Ser. B. Phys. Biol. Sci. 83, 110–119. doi: 10.2183/pjab.83.110
Kong, Q., He, G. Q., Jia, J. L., Zhu, Q. L., and Ruan, H. (2011). Oral administration of Clostridium butyricum for modulating gastrointestinal microflora in mice. Curr. Microbiol. 62, 512–517. doi: 10.1007/s00284-010-9737-8
Krause, G., Winkler, L., Mueller, S. L., Haseloff, R. F., Piontek, J., and Blasig, I. E. (2008). Structure and function of claudins. BBA. 1778, 631–645. doi: 10.1016/j.bbamem.2007.10.018
Leeson, S., Namkung, H., Antongiovanni, M., and Lee, E. H. (2005). Effect of butyric acid on the performance and carcass yield of broiler chickens. Poult. Sci. 84, 1418–1422. doi: 10.1093/ps/84.9.1418
Li, Z., Wang, W., Liu, D., and Guo, Y. (2018). Effects of Lactobacillus acidophilus on the growth performance and intestinal health of broilers challenged with Clostridium perfringens. J. Anim. Sci. Biotechno. 9, 197–206. doi: 10.1186/s40104-018-0243-3
Liao, X. D., Ma, G., Cai, J., Fu, Y., Yan, X. Y., Wei, X. B., et al. (2015). Effects of Clostridium butyricum on growth performance, antioxidation, and immune function of broilers. Poult. Sci. 94, 662–667. doi: 10.3382/ps/pev038
Meimandipour, A., Shuhaimi, M., Soleimani, A. F., Azhar, K., Hair-Bejo, M., Kabeir, B. M., et al. (2010). Selected microbial groups and short-chain fatty acids profile in a simulated chicken cecum supplemented with two strains of Lactobacillus. Poult. Sci. 89, 470–476. doi: 10.3382/ps.2009-00495
Moore, R. J. (2016). Necrotic enteritis predisposing factors in broiler chickens. Avian. Pathol. 45, 275–281. doi: 10.1080/03079457.2016.1150587
Nagpal, R., Ogata, K., Tsuji, H., Matsuda, K., Takahashi, T., Nomoto, K., et al. (2015). Sensitive quantification of Clostridium perfringens in human feces by quantitative real-time PCR targeting alpha-toxin and enterotoxin genes. BMC Microbiol. 15:219. doi: 10.1186/s12866-015-0561-y
Nakanishi, S., Kataoka, K., Kuwahara, T., and Ohnishi, Y. (2003). Effects of high amylose maize starch and Clostridium butyricum on metabolism in colonic microbiota and formation of azoxymethane-induced aberrant crypt foci in the rat colon. Microbiol. Immunol. 47, 951–958. doi: 10.1111/j.1348-0421.2003.tb03469.x
Nakanishi, S., and Tanaka, M. (2010). Sequence analysis of a bacteriocinogenic plasmid of Clostridium butyricum and expression of the bacteriocin gene in Escherichia coli. Anaerobe 16, 253–257. doi: 10.1016/j.anaerobe.2009.10.002
Rehman, H., Awad, W. A., Lindner, I., Hess, M., and Zentek, J. (2006). Clostridium perfringens alpha toxin affects electrophysiological properties of isolated jejunal mucosa of laying hens. Poult. Sci. 85, 1298–1302. doi: 10.1093/ps/85.7.1298
Ruhnke, I., Röhe, I., Goodarzi Boroojeni, F., Knorr, F., Mader, A., Hafeez, A., et al. (2015). Feed supplemented with organic acids does not affect starch digestibility, nor intestinal absorptive or secretory function in broiler chickens. J. Anim. Physiol. Anim. Nutr. 99, 29–35. doi: 10.1111/jpn.12313
Schroeder, B., Winckler, C., Failing, K., and Breves, G. (2004). Studies on the time course of the effects of the probiotic yeast Saccharomyces boulardii on electrolyte transport in pig jejunum. Dig. Dis. Sci. 49, 1311–1317. doi: 10.1023/B:DDAS.0000037828.05100.52
Scumpia, P. O., and Moldawer, L. L. (2005). Biology of interleukin-10 and its regulatory roles in sepsis syndromes. Crit. Care. Med. 33, S468–S471. doi: 10.1097/01.CCM.0000186268.53799.67
Shini, S., Kaiser, P., Shini, A., and Bryden, W. L. (2008). Differential alterations in ultrastructural morphology of chicken heterophils and lymphocytes induced by corticosterone and lipopolysaccharide. Vet. Immunol. Immunop. 122, 83–93. doi: 10.1016/j.vetimm.2007.10.009
Shojadoost, B., Vince, A. R., and Prescott, J. F. (2012). The successful experimental induction of necrotic enteritis in chickens by Clostridium perfringens: a critical review. Vet. Res. 43:74. doi: 10.1186/1297-9716-43-74
Song, B., Li, H., Wu, Y., Zhen, W., and Guo, Y. (2017). Effect of microencapsulated sodium butyrate dietary supplementation on growth performance and intestinal barrier function of broiler chickens infected with necrotic enteritis. Anim. Feed. Sci. Tech. 232, 6–15. doi: 10.1016/j.anifeedsci.2017.07.009
Takahashi, M., McCartney, E., Knox, A., Francesch, M., Oka, K., Wada, K., et al. (2018). Effects of the butyric acid-producing strain Clostridium butyricum MIYAIRI 588 on broiler and piglet zootechnical performance and prevention of necrotic enteritis. Anim. Sci. J. 89, 895–905. doi: 10.1111/asj.13006
Timbermont, L., Haesebrouck, F., Ducatelle, R., and Van Immerseel, F. (2011). Necrotic enteritis in broilers: an updated review on the pathogenesis. Avian. Pathol. 40, 341–347. doi: 10.1080/03079457.2011.590967
Timbermont, L., Lanckriet, A., Dewulf, J., Nollet, N., Schwarzer, K., Haesebrouck, F., et al. (2010). Control of Clostridium perfringens- induced necrotic enteritis in broilers by target-released butyric acid, fatty acids and essential oils. Avian. Pathol. 39, 117–121. doi: 10.1080/03079451003610586
Tonda, R. M., Rubach, J. K., Lumpkins, B. S., Mathis, G. F., and Poss, M. J. (2018). Effects of tannic acid extract on performance and intestinal health of broiler chickens following coccidiosis vaccination and/or a mixed-species Eimeria challenge. Poult. Sci. 97, 3031–3042. doi: 10.3382/ps/pey158
Van Immerseel, F., Rood, J. I., Moore, R. J., and Titball, R. W. (2009). Rethinking our understanding of the pathogenesis of necrotic enteritis in chickens. Trends Microbiol. 17, 32–36. doi: 10.1016/j.tim.2008.09.005
Van Klinken, B. J., Dekker, J., Buller, H. A., and Einerhand, A. W. (1995). Mucin gene structure and expression: protection vs. adhesion. Am. J. Physiol. Gastr. L. 269, G613–G627. doi: 10.1152/ajpgi.1995.269.5.G613
Viswanathan, V. K., Hodges, K., and Hecht, G. (2009). Enteric infection meets intestinal function: how bacterial pathogens cause diarrhoea. Nat. Rev. Microbiol. 7, 110–119. doi: 10.1038/nrmicro2053
Williams, R. B. (2005). Intercurrent coccidiosis and necrotic enteritis of chickens: rational, integrated disease management by maintenance of gut integrity. Avian. Pathol. 34, 159–180. doi: 10.1080/03079450500112195
Wu, Y., Shao, Y., Song, B., Zhen, W., Wang, Z., Guo, Y., et al. (2018). Effects of Bacillus coagulans supplementation on the growth performance and gut health of broiler chickens with Clostridium perfringens-induced necrotic enteritis. J. Anim. Sci. Biotechno. 9:9. doi: 10.1186/s40104-017-0220-2
Wu, Y., Zhen, W., Geng, Y., Wang, Z., and Guo, Y. (2019). Pretreatment with probiotic Enterococcus faecium NCIMB 11181 ameliorates necrotic enteritis-induced intestinal barrier injury in broiler chickens. Sci. Rep. 9:10256. doi: 10.1038/s41598-019-46578-x
Xu, S., Lin, Y., Zeng, D., Zhou, M., Zeng, Y., Wang, H., et al. (2018). Bacillus licheniformis normalize the ileum microbiota of chickens infected with necrotic enteritis. Sci. Rep. 8:1744. doi: 10.1038/s41598-018-20059-z
Xu, X., Wang, Y. H., Muhammad, U. Z. K., Wang, H., and Cai, J. P. (2019). Recombinase polymerase amplification: a detection method for Clostridium perfringens from chicken. Chin. Vet. Sci. 49, 1475–1483. (in Chinese). doi: 10.16656/j.issn.1673-4696.2019.0194
Yang, C. M., Cao, G. T., Ferket, P. R., Liu, T. T., Zhou, L., Zhang, L., et al. (2012). Effects of probiotic, Clostridium butyricum, on growth performance, immune function, and cecal microflora in broiler chickens. Poult. Sci. 91, 2121–2129. doi: 10.3382/ps.2011-02131
Zhang, B., Gan, L., Shahid, M. S., Lv, Z., Fan, H., Liu, D., et al. (2019). In vivo and in vitro protective effect of arginine against intestinal inflammatory response induced by Clostridium perfringens in broiler chickens. J. Anim. Sci. Biotechno. 10:73. doi: 10.1186/s40104-019-0371-4
Zhang, B., Lv, Z., Li, H., Guo, S., Liu, D., and Guo, Y. (2017). Dietary l-arginine inhibits intestinal Clostridium perfringens colonisation and attenuates intestinal mucosal injury in broiler chickens. Br. J. Nutr. 118, 321–332. doi: 10.1017/S0007114517002094
Zhang, L., Cao, G. T., Zeng, X. F., Zhou, L., Ferket, P. R., Xiao, Y. P., et al. (2014). Effects of Clostridium butyricum on growth performance, immune function, and cecal microflora in broiler chickens challenged with Escherichia coli K88. Poult. Sci. 93, 46–53. doi: 10.3382/ps.2013-03412
Zhang, L., Zhang, L., Zhan, X. A., Zeng, X., Zhou, L., Cao, G., et al. (2016). Effects of dietary supplementation of probiotic, Clostridium butyricum, on growth performance, immune response, intestinal barrier function, and digestive enzyme activity in broiler chickens challenged with Escherichia coli K88. J. Anim. Sci. Biotechno. 7, 1–9. doi: 10.1186/s40104-016-0061-4
Keywords: Clostridium butyricum, Clostridium perfringens, necrotic enteritis, gut health, intestinal barrier
Citation: Xu X, Yang S, Olajide JS, Qu Z, Gong Z, Wang J, Zhang Y, Wang H, Xiong L, Zhang K, Zhou E and Cai J (2021) Clostridium butyricum Supplement Can Ameliorate the Intestinal Barrier Roles in Broiler Chickens Experimentally Infected With Clostridium perfringens. Front. Physiol. 12:737481. doi: 10.3389/fphys.2021.737481
Received: 07 July 2021; Accepted: 26 August 2021;
Published: 24 September 2021.
Edited by:
Daniele Maria-Ferreira, Pelé Pequeno Príncipe Research Institute, BrazilReviewed by:
Daniel Hernandez-Patlan, Universidad Nacional Autonóma de México, MexicoAbdul Khalique, Sichuan Agricultural University, China
Copyright © 2021 Xu, Yang, Olajide, Qu, Gong, Wang, Zhang, Wang, Xiong, Zhang, Zhou and Cai. This is an open-access article distributed under the terms of the Creative Commons Attribution License (CC BY). The use, distribution or reproduction in other forums is permitted, provided the original author(s) and the copyright owner(s) are credited and that the original publication in this journal is cited, in accordance with accepted academic practice. No use, distribution or reproduction is permitted which does not comply with these terms.
*Correspondence: Enmin Zhou, emhvdWVtQG53c3VhZi5lZHUuY24=; Jianping Cai, Y2FpamlhbnBpbmdAY2Fhcy5jbg==