- 1Department of Nutrition and Health Sciences, College of Education and Human Sciences, University of Nebraska-Lincoln, Lincoln, NE, United States
- 2Department of Physiology and Pharmacology, Karolinska Institutet, Stockholm, Sweden
- 3Department of Orthopaedics, Eksjö, Region Jönköping County and Department of Biomedical and Clinical Sciences, Linköping University, Linköping, Sweden
- 4Division of Pediatric Neurology, Department of Women’s and Children’s Health, Karolinska Institutet, Stockholm, Sweden
- 5Department of Pediatric Orthopedic Surgery, Karolinska University Hospital, Stockholm, Sweden
- 6Department of Neuroscience, University of Copenhagen, Copenhagen, Denmark
- 7Division of Clinical Physiology, Department of Laboratory Medicine, Karolinska Institutet, Stockholm, Sweden
- 8Unit of Clinical Physiology, Karolinska University Hospital, Stockholm, Sweden
In this study, the properties of circulating extracellular vesicles (EVs) were examined in cerebral palsy (CP) and typically developed (TD) individuals at rest and after aerobic exercise, focusing on the size, concentration, and microRNA cargo of EVs. Nine adult individuals with CP performed a single exercise bout consisting of 45 min of Frame Running, and TD participants completed either 45 min of cycling (n = 10; TD EX) or were enrolled as controls with no exercise (n = 10; TD CON). Blood was drawn before and 30 min after exercise and analyzed for EV concentration, size, and microRNA content. The size of EVs was similar in CP vs. TD, and exercise had no effect. Individuals with CP had an overall lower concentration (∼25%, p < 0.05) of EVs. At baseline, let-7a, let-7b and let-7e were downregulated in individuals with CP compared to TD (p < 0.05), while miR-100 expression was higher, and miR-877 and miR-4433 lower in CP compared to TD after exercise (p < 0.05). Interestingly, miR-486 was upregulated ∼2-fold in the EVs of CP vs. TD both at baseline and after exercise. We then performed an in silico analysis of miR-486 targets and identified the satellite cell stemness factor Pax7 as a target of miR-486. C2C12 myoblasts were cultured with a miR-486 mimetic and RNA-sequencing was performed. Gene enrichment analysis revealed that several genes involved in sarcomerogenesis and extracellular matrix (ECM) were downregulated. Our data suggest that circulating miR-486 transported by EVs is elevated in individuals with CP and that miR-486 alters the transcriptome of myoblasts affecting both ECM- and sarcomerogenesis-related genes, providing a link to the skeletal muscle alterations observed in individuals with CP.
Introduction
Extracellular vesicles (EVs) are a heterogeneous family of particles encompassed by a membrane that originates from the plasma membrane or endosome. Once believed to “just” serve as a disposal mechanism (Pan and Johnstone, 1983), EVs are now highlighted as an important form of intercellular communication with multiple roles in both physiological and pathophysiological processes (Abels and Breakefield, 2016). Indeed, EVs are capable of delivering molecular cargo such as mRNAs, microRNAs, proteins, and lipids to recipient cells (Février and Raposo, 2004; Raposo and Stoorvogel, 2013), with the potential to impact multiple cellular processes.
In response to exercise, skeletal muscle secretes molecules, including EVs, that mediate or even directly cause adaptations to non-skeletal muscle tissue, which in turn influences exercise adaptations (Estébanez et al., 2021). Skeletal muscle fibers secrete EVs with different functions affecting liver processes (Whitham et al., 2018) or promoting lipolysis in white adipose tissue (Vechetti et al., 2021a), to name a few examples. The actions triggered by exercise-induced EVs are suggested to be due to transient alterations in vesicular cargo and increased EV number, without modifications in vesicle size (Estébanez et al., 2021). For example, the concentration of miR-1 in circulating EVs increases in response to aerobic exercise and return to baseline concentrations 4 h after the exercise bout (D’Souza et al., 2018). Other skeletal muscle-enriched microRNAs in EVs that have been described to respond to acute exercise are miR-133a, miR-133b, miR-206, miR-208a, and miR-499 (Yin et al., 2019), highlighting exercise as an acute modifier of skeletal muscle EV content.
Cerebral palsy (CP) is a neurological disorder caused by a non-progressive brain injury. CP is the most common cause of motor impairment in children (Oskoui et al., 2013). After the initial insult, skeletal muscle function typically deteriorates over time (Hägglund and Wagner, 2011). Skeletal muscles of individuals with CP are smaller, weaker, and differ with respect to composition as compared with muscles from typically developed (TD) individuals. Specifically, increased collagen (Booth et al., 2001) and intramuscular adipose tissue content (Noble et al., 2014) is believed to contribute to poor muscle function. Individuals with CP respond to exercise, although differences in performance and adaptations exist when compared with TD individuals (Hjalmarsson et al., 2020; von Walden et al., 2020). Extracellular vesicle biology has never been investigated in individuals with CP, and it remains to be shown if the response of circulating EVs induced by exercise mimics that of TD individuals. In addition, the microRNA profile of individuals with CP has only been studied in neonates at risk for CP (Chapman et al., 2018). Data related to circulating microRNAs in CP adults, with the consequent phenotypic and molecular alterations induced by the long-term effects of CP, is non-existent.
With this background, we performed a study to (i) investigate potential differences in circulating EVs in terms of concentration and size between individuals with CP and controls (i.e., TD), and (ii) examine the response of microRNA cargo in EVs of CP and TD individuals after an acute bout of aerobic exercise. We hypothesized that there would be differences in the EV concentration at baseline between CP and TD individuals, and that specific microRNAs would be differentially expressed after exercise between these two groups.
Materials and methods
Study design
Nine individuals with CP performed a 45-min Frame Running (previously called RaceRunning) exercise bout (CP EX). Physically active TD individuals completed either 45 min of continuous cycling on a cycle ergometer (10 participants; TD EX) or were enrolled as controls with no exercise intervention (10 participants; TD CON). During the exercise bouts, heart rate and perceived level of exertion were registered. Blood samples were obtained before and 30 min post exercise. Plasma was obtained and used for analysis of EV concentration, size, and microRNA content. In-silico and in-vitro analyses were then performed to confirm the human data.
Participants
Individuals with CP from different Frame Running associations in Stockholm greater area volunteered for this study. TD participants were recreationally active individuals, i.e., involved in aerobic exercise (AE) one to three times per week and/or resistance exercise once or twice per week. Inclusion criteria were 18–50 years of age for the TD individuals and 16–50 years of age for the CP group. Exclusion criteria for the TD individuals were cardiovascular disease, neuromuscular disease, or severe knee problems, and for the CP group it was inability to participate in Frame Running due to motor difficulties and/or failure to understand and follow instructions. Thus, the CP group consisted of five male and four female participants [age; 27 years (min-max; 16-44), height; 163 cm (146–175 cm), weight; 53 kg (34–67 kg), BMI; 19.7 (14.7-24.6)], with a Gross Motor Function Classification System (GMFCS, scale from 1 to 5) between 2 and 4 (median GMFCS 3). Three of them had spastic CP, five had dyskinetic CP, and one had ataxic CP. The TD EX group consisted of six male and four female participants [age; 28 years (18-41), height; 176 cm (159–193 cm), weight; 79 kg (46–110 kg), BMI; 25.2 (18.4-34)]. The TD CON group consisted of six male and four female participants [age; 31 years (19-50), height; 178 cm (165–193 cm), weight; 81 kg (57–102 kg), BMI; 25.5 (20.7-32.0)]. All participants gave their informed written consent to participate, after receiving written and oral information about the study. In addition, a legal guardian provided written informed consent to participate for those individuals under 18. The study protocols were approved by the Regional Ethical Review boards in Linköping (#2017/183-31) and Stockholm (#2016/1139-31/2 with addendums 2016/1675-32, 2017/2237-32, 2019-01008).
Exercise bouts and blood sampling
All participants were asked to refrain from exercise 48 h before any activity included in this study. The exercise bout for TD EX consisted of 45 min of cycling (Monark 828 E, Monark Exercise AB, Vansbro, Sweden) at 70% of estimated VO2max, which was assessed a week prior to the acute bout using the Ekblom-Bak test (Björkman et al., 2016). Participants rated their own perceived level of exertion every 10 min using the Borg’s RPE scale and their heart rate was monitored continuously (Garmin Edge 25, Garmin, United States).
The exercise bout for CP EX consisted of 45 min of Frame Running (Hjalmarsson et al., 2020). Heart rate and perceived level of exertion was monitored following the same protocol as described for the TD EX group. Frame Running is a parasport adapted for people with movement disability. It consists of a custom-built tricycle with a saddle and a chest plate but no pedals, which provides the individual balance and stability for locomotion.
Blood was obtained at rest before the exercise bout and at 30 min post exercise via a peripheral venous catheter in individuals from TD EX and CP EX. For participants in TD CON, blood was sampled at rest at two occasions separated by 75 min. Plasma (1 ml) was prepared as previously described (von Walden et al., 2021) and kept at −80°C until further analysis.
Extracellular vesicle isolation from human plasma
Prior to EVs isolation, plasma was centrifuged twice at 3000 × g for 20 min. The resulting supernatant was then filtered through a 0.22 µm syringe-driven filter to remove large contaminating vesicles. Following the filtration, EVs were isolated using ExoEasy membrane affinity column (Enderle et al., 2015) according to the manufacturer’s directions (Qiagen, Carlsbad, CA, United States). Briefly, filtered plasma was mixed 1:1 with 2x binding buffer and then added to the membrane affinity column. After centrifugation, the column was washed, and extracellular vesicles eluted in 400 µL of elution buffer. As a quality control, EVs were visualized using transmission electron microscopy as previously described (Vechetti et al., 2021a) (Supplementary Figure S1).
Nanoparticle tracking analysis
Extracellular vesicle size and concentration were measured by nanoparticle tracking analysis (NTA) using a ZetaView® (Particle Metrix, Meerbusch, Germany) instrument. Isolated EV samples were appropriately diluted using 1X DPBS buffer (Life Technologies, Carlsbad, CA, United States) to measure the particle size and concentration. All NTA measurements were recorded and analyzed at 11 positions. The ZetaView system was calibrated using 100 nm polystyrene particles. Temperature was maintained around 25°C. Prior to each analysis, camera sensitivity and focus were adjusted to ensure the sensitivity remained constant throughout the analysis.
RNA isolation and small RNA sequencing
Total RNA was isolated from EVs using Qiazol Reagent (Qiagen, Carlsbad, CA, United States) and ExoRNAeasy kit (Qiagen) according to the manufacturer’s instructions. The RNA yield and size distribution were analyzed using an Agilent 2.100 Bioanalyzer with an RNA 6,000 Pico kit (Agilent Technologies, Foster City, CA, United States). Samples were shipped on dry ice for small RNA sequencing, performed by Norgen Biotek (Thorold, Ontario, Canada). For small RNA sequencing, pre- and post-exercise samples were pooled to generate an N = 3 per timepoint per group (TD EX; two groups of n = 3, i.e., 6 men, and one group of n = 4, i.e., 4 women, CP EX; three groups of n = 3 grouped by GMFCS levels–II, III, and IV). The complete data set is publicly available at Gene Expression Omnibus with accession ID (GSE219100).
In silico prediction of miR-486 target genes
To identify the possible targets of miR-486 we performed in silico prediction as described before (Valentino et al., 2021). Briefly, we used miRanda (Enright et al., 2003) and RNAhybrid (Krüger and Rehmsmeier, 2006) software for target prediction and utilized a custom Python script to select only shared miRNA:target gene between the two software programs with a minimal free energy set as ΔG° = −18 kJ.
C2C12 culture and miR-486 mimetic
The skeletal muscle C2C12 (ATCC, CRL-1772) myoblasts were cultured in Dulbecco’s Modified Eagles Medium (DMEM) supplemented with 10% Fetal Bovine Serum (FBS), 100 units/ml of Penicillin and 100 μg/ml of Streptomycin until reaching 60%–70% confluency. C2C12 myoblasts were transfected with either a mirVana miR-486 mimic or a scramble miRNA sequence (Life Technologies) used as a negative control. This control sequence does not target any known mature miRNA. Transfection was performed using lipofectamine 2,000 (Life Technologies), according to the manufacturer’s instructions. Shortly, lipofectamine complexes were stabilized in OptiMEM (Gibco) for 5 min and incubated with the miRNA probes, diluted to 25 nM in OptiMEM, for 20 min. Finally, the lipofectamine/probe complexes were added to the cells in a medium without antibiotics. After 6 h of incubation, fresh media was added, and cells were harvested following 24 h. Each transfection experiment was independently repeated at least in triplicate. RNA extraction was performed using TRI reagent (Sigma-Aldrich, St. Louis, MO, United States) coupled with further columns-based processing using the Direct-zol Kit (Zymo Research, Irvine, CA, United States). RNA sequencing was performed by Novogene on a Illumina HiSeq using 150 bp paired-end sequencing (Murach et al., 2022). The complete data set is publicly available at Gene Expression Omnibus with accession ID (GSE219100).
Data analysis
Heart rate and Borg’s scale data was compared between TD EX and CP EX employing a two-tailed unpaired t-test. Potential differences in EV concentration and size across groups and in response to exercise were investigated using a mixed model analysis with group (CP EX, TD EX, TD CON) and time (Pre, 30 min Post) as factors. If a significant interaction was seen, Bonferroni post hoc corrections were performed. The significance level was set at 5% (p < 0.05). Statistical analyses were performed using Prism 7 for Mac OS X (GraphPad Software, San Diego, CA) and Jamovi (v1.6.23.0).
For the microRNA-seq analysis, library preparation and sequencing were performed by Norgen Biotek using the Small RNA Library Prep Kit (Cat. 63600). Sequencing was performed using the NextSeq 500/550 High Output Kit v2 (51 Cycles using a 75-Cycle Kit) on the Illumina NextSeq 500 platform. Single-end reads for miRNAs were mapped to miRbase version 22 using BowTie2. The alignment was performed by Norgen Biotek using sRNAbench and sRNAtoolbox. Raw counts were acquired with featurecounts and they were used as input for the statistical analysis, using DESeq2 (Love et al., 2015) and other Bioconductor packages in R3.6.2. MicroRNAs with less than 10 counts were removed from the analysis. A model taking into account the genotype (TD or CP) and activity (EX or CON) was built and the results were considered statistically significant at an adjusted p < 0.05 (FDR: Benjamini–Hochberg). DESeq2’s regularized-logarithm transformation (rlog) of the count data was used to generate a matrix of regularized counts for sample visualizations.
For target prediction analysis, we utilized the common targets from two different prediction software as previously described by us (Valentino et al., 2021). Briefly, target genes were detected using miRanda (Enright et al., 2003) and RNAhybrid (Krüger and Rehmsmeier, 2006) software, followed by a custom Python script to select only shared miRNA:target gene between the two software programs with a minimal free energy set as ΔG° = −18 kJ. To determine the strength of the predicted miR-486 to pax7 target pairing, we evaluated seed region match quality using the RNAhybrid program (Krüger and Rehmsmeier, 2006) to calculate the minimal free energy of the hybridization.
Gene ontology (GO, sources; Reactome, Wikipathways, BioCarta) and Kyoto Encyclopedia of Genes and Genomes (KEGG) pathway enrichment analyses were carried out in cluster profiler (Yu et al., 2012) on the predicted target genes. The GO terms and KEGG pathways with FDR <0.05 were considered to be significantly enriched.
Results
Data originating from the same participants (TD) has been previously used to investigate the effects of acute exercise on mitochondria-derived peptides in healthy participants (von Walden et al., 2021).
Heart rate and rate of perceived exertion
Average heart rate during the exercise bout was similar (p > 0.05) between CP EX (150 ± 32 bpm) and TD EX (157 ± 18 bpm). Likewise, the rate of perceived exertion averaged over the exercise bout was not different (p > 0.05) between CP EX (13.9 ± 1.6) and TD EX (14.6 ± 0.9).
EV concentration and size
There was a significant main effect of group (p = 0.003, F = 7.1) in EV concentration in plasma (Figure 1A). This effect was mainly driven by a lower concentration of EVs in individuals with CP compared with TD participants. Exercise did not affect EV concentration in any of the groups (Figure 1A). No differences were found in EV size across groups (Figure 1B). Also, exercise did not have any effect on EV size (Figure 1B).
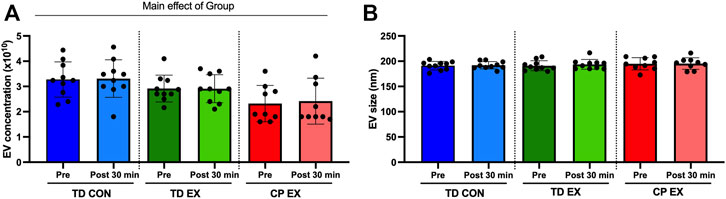
FIGURE 1. Extracellular vesicle (EV) characterization in typically developed (TD) and cerebral palsy (CP) individuals and its response to exercise. (A); EV concentration (particles per mL of plasma) before (Pre) and after (Post) 30 min of aerobic exercise (EX) or control (CON). (B); EV size before (Pre) and after (Post) 30 min of EX or CON condition.
microRNA cargo in EVs
At baseline (rest), miR-486 was higher, and let-7a, let-7b, and let-7e lower in individuals with CP when compared with TD participants (Figures 2A, B; Supplementary Table S1). Thirty minutes after exercise miR-486 remained upregulated in CP vs. TD. In addition, miR-100 expression was higher, and miR-877 and miR-4433 lower in CP EX than in TD EX (Figures 2C, D; Supplementary Table S1). Within-group analysis of sequencing data is shown in Supp. Table 1. Exercise did not affect EV cargo in any of the groups (Supplementary Figure S2).
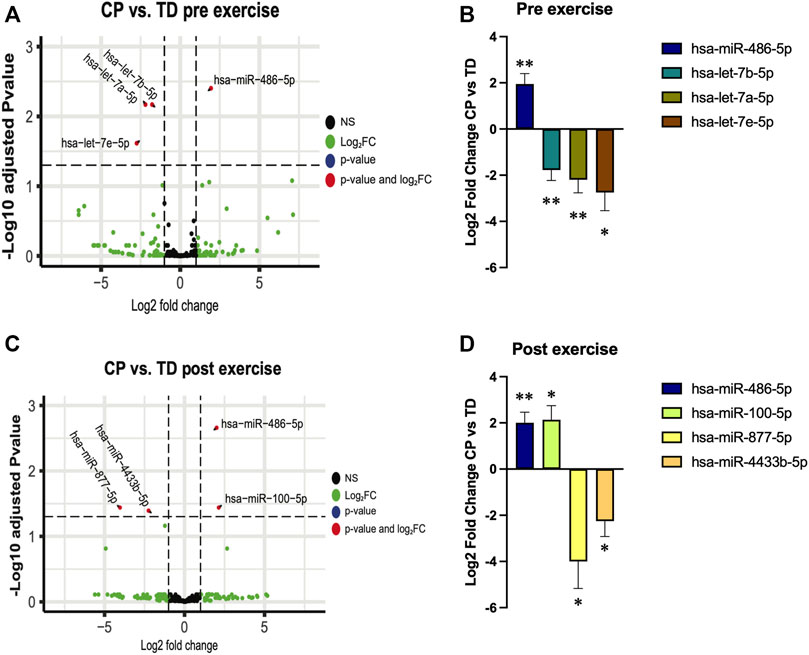
FIGURE 2. Extracellular vesicles microRNA cargo differences between cerebral palsy (CP) and typically developed (TD) individuals before (pre exercise) and 30 min after (post exercise) a bout of aerobic exercise. (A,C); volcano plots illustrating changes in EV cargo between TD and CP before (A) and after (C) exercise. Each point on the scatter plot represents a microRNA where its position on the x-axis corresponds to the average log2-fold change after exercise and the position on the y-axis corresponds to log10 p-value of its differential expression after adjustment for false discovery rate. (B,D); bar graphs illustrating the expression of selected microRNAs in CP individuals relative to TD participants before (B) and after (D) exercise; **; p < 0.01, *; p < 0.05.
With these results at hand, we decided to focus our analysis on the muscle-specific miR-486, which was ∼2-fold upregulated in TD at both baseline and after exercise. Our prediction model (Supplementary Figure S3) indicated that, among other genes, miR-486 targets Pax7, an important gene for skeletal muscle progenitor cell (SMPC) stemness, supporting previous reports (Dey et al., 2011). To further characterize the impact of miR-486 on the SMPC transcriptome, we cultured C2C12 cells with a miR-486 mimetic. Our results showed that genes related to sarcomere, contractile fiber, and myofibril, as well as genes related to extracellular matrix (ECM), were downregulated by the miR-486 mimetic (Figures 3A, C). In contrast, genes involved in protein translation (ribosome) seemed to be upregulated (Figures 3B, D). The complete list of differentially expressed genes and gene ontologies is presented in Supplementary Table S2. To deepen our understanding and interpretation of the changes induced by the miR-486 mimetic, we compared the differentially expressed gene list obtained from the current C2C12 cell data set with DEG lists originated from hamstrings (Smith et al., 2012) and wrist (Smith et al., 2009) skeletal muscle of CP individuals, as well as muscle from miR-486-KO mice (Samani et al., 2022) (Supplementary Figure S4 and Supplementary Table S2). Among the genes differentially expressed in our C2C12 experiments and in CP skeletal muscle, we identified mediators of insulin growth factors (IGFBP5), regulators of the organization of the actin filament system (RHOBTB1), several collagen related genes (e.g., COL1A1, COL3A1, COL4A1, COL5A1), and myosin heavy chain (MYH1, MYH3) related genes. Four common genes were identified when cross-referencing the CP hamstring, miR-486-KO and C2C12 data sets related either to the ECM (COL1A1, COL1A2) or the cytoskeleton (MAP1A, PLXNA2).
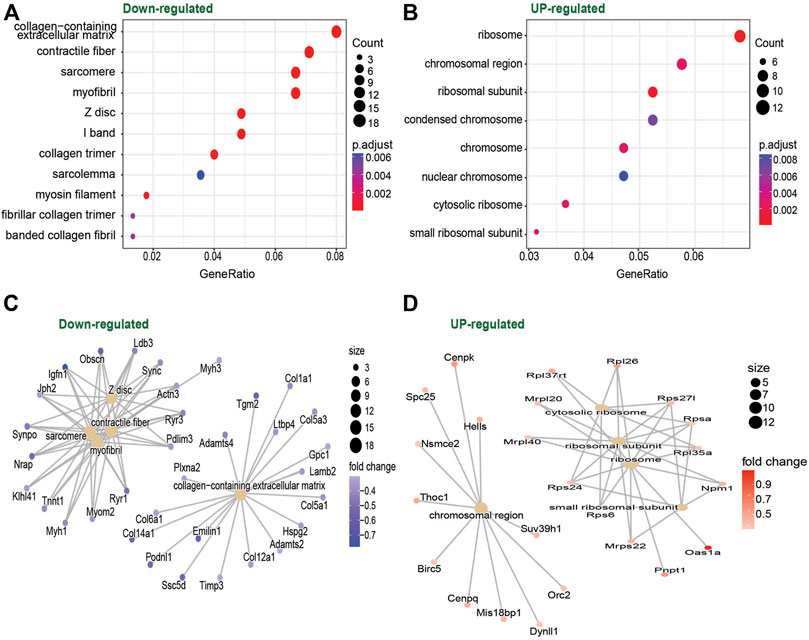
FIGURE 3. Dot plot of gene ontology enrichment of Down-regulated (A) and Upregulated (B) genes in C2C12 cells culture with vs. without miR-486. Red indicates higher enrichment; blue indicates lower enrichment. The sizes of the dots represent the gene counts of each row (GO category). Enrichment map of the inter-relation of the top 5 enriched biological processes on the Downregulated (C) and Upregulated (D) genes.
Discussion
In this study, the characteristics of circulating EVs were compared between CP and TD individuals at rest and after acute aerobic exercise. Our results indicate that the size of plasma EVs is similar between CP and TD and that exercise does not affect this morphological characteristic. However, CP individuals presented an overall lower concentration of EVs both at baseline and after exercise. The microRNA cargo transported by circulating EVs was different between the CP and TD groups, and exercise influenced these differences. Interestingly, the skeletal muscle specific miRNA miR-486 was upregulated in EVs from CP individuals both at baseline and after exercise. Our in silico and in-vitro follow-up experiments showed that miR-486 targets Pax7, an important regulator of satellite cells, and that it affects the transcriptome of skeletal muscle progenitor cells related to sarcomerogenesis and ECM.
The effect of exercise on EV concentration is a matter of current debate (Estébanez et al., 2021). Our results support the studies indicating that acute exercise does not affect EV concentration (Lovett et al., 2018; Brahmer et al., 2019; Rigamonti et al., 2020). On the other hand, there seems to be agreement that EV size remains constant after exercise (Estébanez et al., 2021), a notion supported by the current results. An interesting finding of this study was that individuals with CP appeared to have a lower concentration of EVs compared to TD individuals. Lower EV concentration has been previously described in elderly versus young individuals (Eitan et al., 2017) and could indicate suboptimal intercellular and consequently interorgan communication. It remains to be shown what cell type(s) are responsible for this lower EV release in individuals with CP.
The molecular cargo transported and delivered by EVs has a major impact on cell-to-cell communication, as well as on the crosstalk between different organs. A novel finding of our analysis was that EVs from individuals with CP had significantly lower expression of three microRNAs, namely let-7a, let-7b, and let-7e, at baseline compared with TD individuals. Reduced levels of let-7 family microRNAs in circulating EVs have been linked to increased fibrosis in the liver (Matsuura et al., 2016). One could speculate that the low let-7 content in EVs reported in the current study could be associated with the well-known skeletal muscle phenotype of CP individuals showing a marked increased collagen content (Booth et al., 2001; Bruin et al., 2014; Von Walden et al., 2018). Another important function of let-7 microRNAs is the regulation of skeletal development, with let-7 deficiencies leading to an impairment in skeletal growth (Papaioannou et al., 2013). With this background, it could be that the lower let-7 levels found in participants with CP in this investigation could explain, at least partly, the generally shorter stature compared to TD individuals (Ruiz Brunner et al., 2022). Even though the two hypotheses linking reduced levels of circulating let-7 with muscle ECM changes and reduced height in individuals with CP need to be specifically tested in future studies, we show that aerobic exercise normalized (i.e., similar to TD individuals) circulating let-7 levels, which could be interpreted as an exercise-induced adaptation towards a healthier EV-content profile.
Exercise is a powerful stimulus to influence the content of EVs present in the bloodstream (Vechetti et al., 2021b). After an aerobic exercise bout, miR-877 and miR-4433b were downregulated and miR-100 was upregulated in CP compared with TD individuals. To our knowledge, this is the first report indicating effects of exercise on miR-877 and miR-4433b expression in EVs. However, circulating miR-100 has been shown to decrease after exercise in trained individuals (Sansoni et al., 2018). Thus, it appears that individuals with CP responded to the exercise performed in a more naïve or untrained fashion compared to TD participants. This is not surprising given that individuals with CP generally engage in lower levels of high-intensity physical activity than their TD peers (Nooijen et al., 2014).
An important and novel finding of the current study was the ∼2-fold elevated levels of miR-486 in EVs from CP individuals compared with TD subjects, both at baseline and after exercise. A possible explanation for such differences may be the lower physical fitness status of CP individuals compared with TD, as previously mentioned, since there are reports indicating that chronic exercise decreases circulating miR-486 levels (Aoi et al., 2013; Barber et al., 2019). The host gene of miR-486 is ankyrin-1, and this gene has been shown to be upregulated in skeletal muscle from CP individuals (Smith et al., 2012). Therefore, it is possible that the differences in circulating levels of miR-486 in the current study had an origin and/or important effect on skeletal muscle tissue. To test this hypothesis, we performed a target prediction model for miR-486, which showed that one of the target genes of this microRNA is Pax-7. Previous reports have indicated that Pax-7 is downregulated by miR-486 (Dey et al., 2011). Pax-7 is an important transcription factor for satellite cell (skeletal muscle progenitor cell) function in adult skeletal muscle (von Maltzahn et al., 2013) and consequently plays a critical role in myogenesis. Pax-7 is expressed in proliferating satellite cells and undergoes rapid downregulation during satellite cell differentiation. The results presented in this study showing higher expression of miR-486 in individuals with CP may therefore indicate a miR-486-induced mismatch between proliferation and differentiation of satellite cells. This mismatch could lead to altered numbers of viable and normally functioning myofibers in skeletal muscle from CP individuals, as previously suggested (Domenighetti et al., 2018). On the other hand, contrasting reports indicate a beneficial effect of miR-486 on muscle health, reversing skeletal muscle defects in an animal model of Duchenne muscular dystrophy (Wang et al., 2022). Thus, an alternative interpretation of the greater miR-486 abundance in EVs from CP individuals could be an attempt, by the muscle itself, to counteract the negative effects of CP on skeletal muscle.
To further investigate the role of miR-486 in skeletal muscle cells, we cultured C2C12 cells (immortalized mouse myoblast cell line) with a miR-486 mimetic. The results showed that several genes involved in sarcomerogenesis and ECM were downregulated. Downregulation of these groups of genes has been previously reported in skeletal muscle disuse atrophy (Urso et al., 2006; Chen et al., 2007). The similarities between acute (days or weeks) disuse atrophy and a chronic condition affecting the skeletal muscle, i.e., CP, cannot be overlooked. Indeed, individuals with CP exhibit weak and thin muscles (von Walden et al., 2017) with altered ECM properties (Von Walden et al., 2018; Smith et al., 2021). Sarcomerogenesis and ECM changes driven at least in part by miR-486 thus appear to be a common signature of muscle deconditioning. When we compared the current C2C12 dataset with other CP- and miR-486 related datasets, we found common genes related to cellular structure (genes coding for myosin and actin), as well as a set of genes coding for different types of collagens. Overall, these data seem to indicate overlapping transcriptional regulation between skeletal muscle in CP individuals and the direct effects of miR-486. However, such a relationship should be investigated in further detail.
In our study, exercise did not alter the expression of any EV-transported microRNA. This is in contrast with other studies showing that acute endurance exercise alters circulating microRNAs, e.g., miR-20a and miR-21 (Zhou et al., 2020). Differences across studies in relation to the source of the microRNAs [i.e., plasma/serum vs. EVs (D’Souza et al., 2018)], the type of exercise (Sieland et al., 2021), the timepoints selected, and the specific population investigated may explain, at least partly, these contrasting findings.
In summary, this study provides novel and valuable information on the properties and cargo of EVs in CP individuals at rest and after aerobic exercise. The size of EVs was similar in all groups and independent of the exercise stimulus. Conversely, CP individuals had a lower concentration of EVs both at baseline and after exercise. TD and CP participants presented a different EV-transported microRNA profile at rest, and exercise affected these differences. However, the upregulation of miR-486 in EVs from CP individuals was stable and evident both at baseline and after exercise. Our in silico and in vitro follow-up experiments showed that miR-486 targets Pax7, an important regulator of satellite cells, and that miR-486 affects the expression of genes responsible for sarcomerogenesis and ECM in skeletal muscle cells. Overall, our data suggest that miR-486 is stably overrepresented in CP EVs, plausibly providing a link to skeletal muscle alterations seen in individuals with CP.
Data availability statement
The transcriptomic datasets presented in this study can be found in online repositories. The names of the repository/repositories and accession number(s) can be found below: https://www.ncbi.nlm.nih.gov/, GSE219100. Other data generated during the current study are available from the corresponding author on reasonable request.
Ethics statement
The studies involving human participants were reviewed and approved by Regional Ethical Review boards in Linköping and Stockholm. The patients/participants provided their written informed consent to participate in this study.
Author contributions
JN, BA, EH, AP, JP, FW, and RF-G performed the exercise interventions. FW and IJV prepared the samples for RNA-sequencing. IJV completed the in silico analysis. FW and IJV performed the cell experiments. IJV, FW, and RF-G analyzed and interpreted the results. RF-G, FW, and IJV drafted the manuscript. JN, EH, EP, and JP reviewed the manuscript. All authors approved the final version of the paper.
Funding
This work was supported by Futurum–the Academy for Health and Care, Region Jönköping County, Sweden (Grant Nos FUTURUM-937508 and 870471) to BA and the Swedish Kidney Foundation (F2019-0048), NIH P20GM104320-07 (IJV), H.K.H. Kronprinsessan Lovisas förening för barnasjukvård (FW), the Swedish Society of Medical Research (FW), Linnea and Josef Carlsson (RF-G, FW), Sunnerdahls handikappstiftelse (FW and EP) and Norrbacka Eugenia Stiftelsen (FW and EP). RF-G is supported by a career grant from the Swedish National Space Agency (2021-00159).
Acknowledgments
The authors would like to thank Kevin Murach for proofreading the manuscript.
Conflict of interest
The authors declare that the research was conducted in the absence of any commercial or financial relationships that could be construed as a potential conflict of interest.
Publisher’s note
All claims expressed in this article are solely those of the authors and do not necessarily represent those of their affiliated organizations, or those of the publisher, the editors and the reviewers. Any product that may be evaluated in this article, or claim that may be made by its manufacturer, is not guaranteed or endorsed by the publisher.
Supplementary material
The Supplementary Material for this article can be found online at: https://www.frontiersin.org/articles/10.3389/fphys.2022.1072040/full#supplementary-material
References
Abels E. R., Breakefield X. O. (2016). Introduction to extracellular vesicles: Biogenesis, RNA cargo selection, content, release, and uptake. Cell. Mol. Neurobiol. 36, 301–312. doi:10.1007/s10571-016-0366-z
Aoi W., Ichikawa H., Mune K., Tanimura Y., Mizushima K., Naito Y., et al. (2013). Muscle-enriched microRNA miR-486 decreases in circulation in response to exercise in young men. Front. Physiol. 4, 80. doi:10.3389/fphys.2013.00080
Barber J. L., Zellars K. N., Barringhaus K. G., Bouchard C., Spinale F. G., Sarzynski M. A. (2019). The effects of regular exercise on circulating cardiovascular-related MicroRNAs. Sci. Rep. 9, 7527. doi:10.1038/s41598-019-43978-x
Björkman F., Ekblom-Bak E., Ekblom Ö., Ekblom B. (2016). Validity of the revised Ekblom Bak cycle ergometer test in adults. Eur. J. Appl. Physiol. 116, 1627–1638. doi:10.1007/s00421-016-3412-0
Booth C. M., Cortina-Borja M. J. F., Theologis T. N. (2001). Collagen accumulation in muscles of children with cerebral palsy and correlation with severity of spasticity. Dev. Med. Child. Neurol. 43, 314–320. doi:10.1017/s0012162201000597
Brahmer A., Neuberger E., Esch-Heisser L., Haller N., Jorgensen M. M., Baek R., et al. (2019). Platelets, endothelial cells and leukocytes contribute to the exercise-triggered release of extracellular vesicles into the circulation. J. Extracell. Vesicles 8, 1615820. doi:10.1080/20013078.2019.1615820
Bruin M. de, Smeulders M. J., Kreulen M., Huijing P. A., Jaspers R. T. (2014). Intramuscular connective tissue differences in spastic and control muscle: A mechanical and histological study. PLOS ONE 9, e101038. doi:10.1371/journal.pone.0101038
Chapman S., Farina L., Kronforst K., Dizon M. (2018). MicroRNA profile differences in neonates at risk for cerebral palsy. Phys. Med. Rehabil. Int. 5, 1148.
Chen Y.-W., Gregory C. M., Scarborough M. T., Shi R., Walter G. A., Vandenborne K. (2007). Transcriptional pathways associated with skeletal muscle disuse atrophy in humans. Physiol. Genomics 31, 510–520. doi:10.1152/physiolgenomics.00115.2006
Dey B. K., Gagan J., Dutta A. (2011). miR-206 and -486 induce myoblast differentiation by downregulating Pax7. Mol. Cell. Biol. 31, 203–214. doi:10.1128/MCB.01009-10
Domenighetti A. A., Mathewson M. A., Pichika R., Sibley L. A., Zhao L., Chambers H. G., et al. (2018). Loss of myogenic potential and fusion capacity of muscle stem cells isolated from contractured muscle in children with cerebral palsy. Am. J. Physiol. Cell Physiol. 315, C247–C257. doi:10.1152/ajpcell.00351.2017
D’Souza R. F., Woodhead J. S. T., Zeng N., Blenkiron C., Merry T. L., Cameron-Smith D., et al. (2018). Circulatory exosomal miRNA following intense exercise is unrelated to muscle and plasma miRNA abundances. Am. J. Physiol. Endocrinol. Metab. 315, E723–E733. doi:10.1152/ajpendo.00138.2018
Eitan E., Green J., Bodogai M., Mode N. A., Bæk R., Jørgensen M. M., et al. (2017). Age-related changes in plasma extracellular vesicle characteristics and internalization by leukocytes. Sci. Rep. 7, 1342. doi:10.1038/s41598-017-01386-z
Enderle D., Spiel A., Coticchia C. M., Berghoff E., Mueller R., Schlumpberger M., et al. (2015). Characterization of RNA from exosomes and other extracellular vesicles isolated by a novel spin column-based method. PloS One 10, e0136133. doi:10.1371/journal.pone.0136133
Enright A. J., John B., Gaul U., Tuschl T., Sander C., Marks D. S. (2003). MicroRNA targets in Drosophila. Genome Biol. 5, R1. doi:10.1186/gb-2003-5-1-r1
Estébanez B., Jiménez-Pavón D., Huang C.-J., Cuevas M. J., González-Gallego J. (2021). Effects of exercise on exosome release and cargo in in vivo and ex vivo models: A systematic review. J. Cell. Physiol. 236, 3336–3353. doi:10.1002/jcp.30094
Février B., Raposo G. (2004). Exosomes: Endosomal-derived vesicles shipping extracellular messages. Curr. Opin. Cell Biol. 16, 415–421. doi:10.1016/j.ceb.2004.06.003
Hägglund G., Wagner P. (2011). Spasticity of the gastrosoleus muscle is related to the development of reduced passive dorsiflexion of the ankle in children with cerebral palsy: A registry analysis of 2, 796 examinations in 355 children. Acta Orthop. 82, 744–748. doi:10.3109/17453674.2011.618917
Hjalmarsson E., Fernandez-Gonzalo R., Lidbeck C., Palmcrantz A., Jia A., Kvist O., et al. (2020). RaceRunning training improves stamina and promotes skeletal muscle hypertrophy in young individuals with cerebral palsy. BMC Musculoskelet. Disord. 21, 193. doi:10.1186/s12891-020-03202-8
Krüger J., Rehmsmeier M. (2006). RNAhybrid: microRNA target prediction easy, fast and flexible. Nucleic Acids Res. 34, W451–W454. doi:10.1093/nar/gkl243
Lex A., Gehlenborg N., Strobelt H., Vuillemot R., Pfister H. (2014). UpSet: Visualization of intersecting sets. IEEE Trans. Vis. Comput. Graph. 20, 1983–1992. doi:10.1109/TVCG.2014.2346248
Love M. I., Anders S., Kim V., Huber W. (2015). RNA-seq workflow: Gene-level exploratory analysis and differential expression. F1000Res. 4, 1070. doi:10.12688/f1000research.7035.1
Lovett J. A. C., Durcan P. J., Myburgh K. H. (2018). Investigation of circulating extracellular vesicle MicroRNA following two consecutive bouts of muscle-damaging exercise. Front. Physiol. 9, 1149. doi:10.3389/fphys.2018.01149
Matsuura K., De Giorgi V., Schechterly C., Wang R. Y., Farci P., Tanaka Y., et al. (2016). Circulating let-7 levels in plasma and extracellular vesicles correlate with hepatic fibrosis progression in chronic hepatitis C. Hepatology 64, 732–745. doi:10.1002/hep.28660
Murach K. A., Liu Z., Jude B., Figueiredo V. C., Wen Y., Khadgi S., et al. (2022). Multi-transcriptome analysis following an acute skeletal muscle growth stimulus yields tools for discerning global and MYC regulatory networks. J. Biol. Chem. 298, 102515. doi:10.1016/j.jbc.2022.102515
Noble J. J., Charles-Edwards G. D., Keevil S. F., Lewis A. P., Gough M., Shortland A. P. (2014). Intramuscular fat in ambulant young adults with bilateral spastic cerebral palsy. BMC Musculoskelet. Disord. 15, 236. doi:10.1186/1471-2474-15-236
Nooijen C. F., Slaman J., Stam H. J., Roebroeck M. E., Berg-Emons R. J. V. D. (2014). Inactive and sedentary lifestyles amongst ambulatory adolescents and young adults with cerebral palsy. J. Neuroeng. Rehabil. 11, 49. doi:10.1186/1743-0003-11-49
Oskoui M., Coutinho F., Dykeman J., Jetté N., Pringsheim T. (2013). An update on the prevalence of cerebral palsy: A systematic review and meta-analysis. Dev. Med. Child. Neurol. 55, 509–519. doi:10.1111/dmcn.12080
Pan B.-T., Johnstone R. M. (1983). Fate of the transferrin receptor during maturation of sheep reticulocytes in vitro: Selective externalization of the receptor. Cell 33, 967–978. doi:10.1016/0092-8674(83)90040-5
Papaioannou G., Inloes J. B., Nakamura Y., Paltrinieri E., Kobayashi T. (2013). let-7 and miR-140 microRNAs coordinately regulate skeletal development. Proc. Natl. Acad. Sci. U. S. A. 110, E3291–E3300. doi:10.1073/pnas.1302797110
Raposo G., Stoorvogel W. (2013). Extracellular vesicles: Exosomes, microvesicles, and friends. J. Cell Biol. 200, 373–383. doi:10.1083/jcb.201211138
Rigamonti A. E., Bollati V., Pergoli L., Iodice S., De Col A., Tamini S., et al. (2020). Effects of an acute bout of exercise on circulating extracellular vesicles: Tissue-sex-and BMI-related differences. Int. J. Obes. 44, 1108–1118. doi:10.1038/s41366-019-0460-7
Ruiz Brunner M. D. L. M., Cuestas E., Heinen F., Schroeder A. S. (2022). Growth in infants, children and adolescents with unilateral and bilateral cerebral palsy. Sci. Rep. 12, 1879. doi:10.1038/s41598-022-05267-y
Samani A., Hightower R. M., Reid A. L., English K. G., Lopez M. A., Doyle J. S., et al. (2022). miR-486 is essential for muscle function and suppresses a dystrophic transcriptome. Life Sci. Alliance 5, e202101215. doi:10.26508/lsa.202101215
Sansoni V., Perego S., Vernillo G., Barbuti A., Merati G., Torre A. L., et al. (2018). Effects of repeated sprints training on fracture risk-associated miRNA. Oncotarget 9, 18029–18040. doi:10.18632/oncotarget.24707
Sieland J., Niederer D., Engeroff T., Vogt L., Troidl C., Schmitz-Rixen T., et al. (2021). Effects of single bouts of different endurance exercises with different intensities on microRNA biomarkers with and without blood flow restriction: A three-arm, randomized crossover trial. Eur. J. Appl. Physiol. 121, 3243–3255. doi:10.1007/s00421-021-04786-2
Smith L. R., Chambers H. G., Subramaniam S., Lieber R. L. (2012). Transcriptional abnormalities of hamstring muscle contractures in children with cerebral palsy. PloS One 7, e40686. doi:10.1371/journal.pone.0040686
Smith L. R., Pichika R., Meza R. C., Gillies A. R., Baliki M. N., Chambers H. G., et al. (2021). Contribution of extracellular matrix components to the stiffness of skeletal muscle contractures in patients with cerebral palsy. Connect. Tissue Res. 62, 287–298. doi:10.1080/03008207.2019.1694011
Smith L. R., Pontén E., Hedström Y., Ward S. R., Chambers H. G., Subramaniam S., et al. (2009). Novel transcriptional profile in wrist muscles from cerebral palsy patients. BMC Med. Genomics 2, 44. doi:10.1186/1755-8794-2-44
Urso M. L., Scrimgeour A. G., Chen Y.-W., Thompson P. D., Clarkson P. M. (2006). Analysis of human skeletal muscle after 48 h immobilization reveals alterations in mRNA and protein for extracellular matrix components. J. Appl. Physiol. 101, 1136–1148. doi:10.1152/japplphysiol.00180.2006
Valentino T., Figueiredo V. C., Mobley C. B., McCarthy J. J., Vechetti I. J. (2021). Evidence of myomiR regulation of the pentose phosphate pathway during mechanical load-induced hypertrophy. Physiol. Rep. 9, e15137. doi:10.14814/phy2.15137
Vechetti I. J., Peck B. D., Wen Y., Walton R. G., Valentino T. R., Alimov A. P., et al. (2021a). Mechanical overload-induced muscle-derived extracellular vesicles promote adipose tissue lipolysis. FASEB J. 35, e21644. doi:10.1096/fj.202100242R
Vechetti I. J., Valentino T., Mobley C. B., McCarthy J. J. (2021b). The role of extracellular vesicles in skeletal muscle and systematic adaptation to exercise. J. Physiol. 599, 845–861. doi:10.1113/JP278929
von Maltzahn J., Jones A. E., Parks R. J., Rudnicki M. A. (2013). Pax7 is critical for the normal function of satellite cells in adult skeletal muscle. Proc. Natl. Acad. Sci. U. S. A. 110, 16474–16479. doi:10.1073/pnas.1307680110
von Walden F., Fernandez-Gonzalo R., Norrbom J., Emanuelsson E. B., Figueiredo V. C., Gidlund E.-K., et al. (2021). Acute endurance exercise stimulates circulating levels of mitochondrial-derived peptides in humans. J. Appl. Physiol. 131, 1035–1042. doi:10.1152/japplphysiol.00706.2019
Von Walden F., Gantelius S., Liu C., Borgström H., Björk L., Gremark O., et al. (2018). Muscle contractures in patients with cerebral palsy and acquired brain injury are associated with extracellular matrix expansion, pro-inflammatory gene expression, and reduced rRNA synthesis. Muscle Nerve 58, 277–285. doi:10.1002/mus.26130
von Walden F., Hjalmarsson E., Reimeringer M., Kvist O., Raffalt P. C., Pontén E., et al. (2020). Comparative analysis of power, work and muscle activation during weight-stack and iso-inertial flywheel resistance exercise in young adults with cerebral palsy. J. Rehabil. Med. 52, jrm00060. doi:10.2340/16501977-2682
von Walden F., Jalaleddini K., Evertsson B., Friberg J., Valero-Cuevas F. J., Pontén E. (2017). Forearm flexor muscles in children with cerebral palsy are weak, thin and stiff. Front. Comput. Neurosci. 11, 30. doi:10.3389/fncom.2017.00030
Wang R., Kumar B., Doud E. H., Mosley A. L., Alexander M. S., Kunkel L. M., et al. (2022). Skeletal muscle-specific overexpression of miR-486 limits mammary tumor-induced skeletal muscle functional limitations. Mol. Ther. Nucleic Acids 28, 231–248. doi:10.1016/j.omtn.2022.03.009
Whitham M., Parker B. L., Friedrichsen M., Hingst J. R., Hjorth M., Hughes W. E., et al. (2018). Extracellular vesicles provide a means for tissue crosstalk during exercise. Cell Metab. 27, 237–251.e4. doi:10.1016/j.cmet.2017.12.001
Yin X., Zhao Y., Zheng Y. L., Wang J. Z., Li W., Lu Q. J., et al. (2019). Time-course responses of muscle-specific MicroRNAs following acute uphill or downhill exercise in sprague-dawley rats. Front. Physiol. 10, 1275. doi:10.3389/fphys.2019.01275
Yu G., Wang L.-G., Han Y., He Q.-Y. (2012). clusterProfiler: an R package for comparing biological themes among gene clusters. Omics J. Integr. Biol. 16, 284–287. doi:10.1089/omi.2011.0118
Keywords: exosomes, endurance exercise, miR-486, skeletal muscle, frame running
Citation: Vechetti IJ, Norrbom J, Alkner B, Hjalmarsson E, Palmcrantz A, Pontén E, Pingel J, von Walden F and Fernandez-Gonzalo R (2022) Extracellular vesicle characteristics and microRNA content in cerebral palsy and typically developed individuals at rest and in response to aerobic exercise. Front. Physiol. 13:1072040. doi: 10.3389/fphys.2022.1072040
Received: 17 October 2022; Accepted: 06 December 2022;
Published: 21 December 2022.
Edited by:
Katsuhiko Suzuki, Waseda University, JapanReviewed by:
Ajai Tripathi, Merck, United StatesMarie-Claude Sincennes, Université du Québec, Canada
Liz Simon, Louisiana State University, United States
Copyright © 2022 Vechetti, Norrbom, Alkner, Hjalmarsson, Palmcrantz, Pontén, Pingel, von Walden and Fernandez-Gonzalo. This is an open-access article distributed under the terms of the Creative Commons Attribution License (CC BY). The use, distribution or reproduction in other forums is permitted, provided the original author(s) and the copyright owner(s) are credited and that the original publication in this journal is cited, in accordance with accepted academic practice. No use, distribution or reproduction is permitted which does not comply with these terms.
*Correspondence: Rodrigo Fernandez-Gonzalo, cm9kcmlnby5mZXJuYW5kZXotZ29uemFsb0BraS5zZQ==
†These authors have contributed equally to this work