- 1School of Life Sciences, Northwestern Polytechnical University, Xi’an, China
- 2Shaanxi Engineering Laboratory for Food Green Processing and Safety Control, College of Food Engineering and Nutritional Science, Shaanxi Normal University, Xi’an, China
- 3Research Center for Prevention and Treatment of Respiratory Disease, School of Clinical Medicine, Xi’an Medical University, Xi’an, China
- 4Department of Cardiology, 986th Hospital, Fourth Military Medical University, Xi’an, China
- 5School of Aerospace Medicine, Fourth Military Medical University, Xi’an, China
Gluconolactone (D-glucono-1,5-lactone or GDL) is a food additive which presents in dietary products such as tofu, yogurt, cheese, bread, wine, etc. GDL has long been considered as a free radical scavenger; however, its role in cardioprotection remains elusive. In this study, using a mouse model of myocardial ischemia/reperfusion (I/R) injury and a model of hypoxia/reoxygenation (H/R) in neonatal rat cardiomyocytes (NRCM), we explored the role of GDL in I/R injury. We found that GDL (5 mg/kg, i.p.) attenuated myocardial I/R injury as evidenced by decreased infarct size, release of cardiac injury markers and apoptosis. Additionally, GDL decreased reperfusion-induced arrhythmias and oxidative stress. These effects were also observed in parallel in vitro studies. Mechanistically, we found that GDL treatment was strongly associated with activation of pro-survival extracellular signal-regulated kinase (ERK) signaling both in vivo and in vitro, and pharmacological inhibition of ERK signaling via U0126 attenuated GDL-induced cardioprotection against H/R injury in NRCM cells. To reveal how GDL regulates ERK signaling, we predicted the putative targets of GDL by Swiss Target Prediction, and protein kinase C (PKC) emerged as the most promising target for GDL. By pharmacological intervention and immunofluorescence, we found that PKCε, an important member of the PKC family, was activated after GDL treatment in heart, thereby leading to ERK activation and cardioprotection against I/R injury. Taken together, our results demonstrated that GDL acts as a potent activator of PKCε and, thus, provides cardioprotection against I/R injury via activation of ERK signaling.
Introduction
Gluconolactone (D-glucono-1,5-lactone or GDL) is a polyhydroxy acid (PHA), a lactone or oxidized derivative of glucose, and is widely distributed in nature ranging from bacteria to humans. GDL can be naturally found in honey, grapes and fruit juices, and widely used in dietary products such as yogurt, cheese, bread, wine and tofu, etc., as a food additive (European food additive number E575). Recently, GDL as a human natural metabolite has attracted more and more attention, and its levels were shown to change significantly under different pathophysiological conditions. Zhao et al. (2018) demonstrated that fecal GDL increased about twofold in normal human subjects after half-marathon race compared with those before race. Makowski et al. (2014) showed that threefold decrease of GDL were observed in tumors from obese mice compared with lean counterparts. However, the role of GDL in the development of cardiovascular diseases remains elusive.
Ischemia/reperfusion (I/R) injury is a common pathological process in the clinic, and is associated with adverse postoperative outcomes. Reactive oxygen species (ROS) burst (superoxide radicals or hydrogen peroxide) at the onset of reperfusion is considered as one of the key factors contributing to reperfusion injury. In the presence of metals such as iron and copper, superoxide radicals or hydrogen peroxide could produce the most reactive ROS, the hydroxyl radical (•OH), thus aggravating the oxidative stress (Wu and Cederbaum, 2003). GDL, a natural PHA, is capable of chelating metals as an antioxidant chelating agent, and provides protection up to 50% against UV radiation (Bernstein et al., 2004; Bowes, 2013; Audina, 2021). Moreover, GDL could maintain the redox balance via increasing NADPH generation through entering the gluconate pathway and subsequent pentose-phosphate pathway (PPP; Wushensky et al., 2018), and was thus used as a probe to detect PPP flux (Moreno et al., 2017). These results indicated that GDL might exert cardioprotection against I/R injury via decreasing oxidative stress.
Evidence has shown that lactone exerts protective effects against various pathological conditions, including myocardial infarction and I/R injury. Wang et al. (2018) have shown that lactone component from Ligusticum chuanxiong significantly reduced infarct size in myocardial ischemia injured rats and H9c2 cardiomyocytes, probably due to the restoration of autophagic flux through activation of PI3K/Akt/mTOR signaling pathway. Moreover, 10 kinds of diterpene lactone compounds including Ginkgolide B (GB), Ginkgolide A (GA), and Ginkgolide K (GK) have been isolated from Ginkgo biloba leaves, and some of them are reported to provide the cardiocerebral protection (reviewed by Geng et al., 2018; Liu et al., 2019 in rat brain). Regarding I/R injury, lactones, such as ginkgo diterpene lactones, parthenolide, etc., have been reported to attenuate reperfusion injury in brain (Li et al., 2020), heart (Zingarelli et al., 2002; Liu et al., 2011) and PC12 cells (Zhang et al., 2017). Thus, it is easily to postulate that GDL, a δ-lactone formed by an inner ester of gluconic acid, might have the same effect like other lactones. GDL is a special lactone, as it is a human natural metabolite showing anti-oxidant properties, and revealing of its role in cardioprotection will help to discover new disease pathogenesis and find possible therapeutic targets.
In this study, using a mouse model of I/R injury and a cellular model of hypoxia/reoxygenation (H/R) mimicking I/R injury, we try to explore the role of GDL in I/R injury and the underlying mechanism. Our results demonstrated that GDL exerted cardioprotection against I/R injury, partly due to the activation of its potential target PKC and downstream pro-survival extracellular signal-regulated kinase (ERK) signaling.
Materials and Methods
Materials and Chemicals
D-glucono-1,5-lactone was obtained from MedChem Express (Princeton, NJ, United States). Triphenyltetrazolium chloride (TTC), PI3K inhibitor Wortmannin (Wm; inhibition of PI3K/Akt signaling) and mitogen-activated protein kinase kinase (MEK) inhibitor U0126 (inhibition of MEK/ERK signaling) were purchased from Sigma (St. Louis, MO, United States). PKC inhibitors Chelerythrine and Rottlerin were purchased from Topscience (Shanghai, China). PKC inhibitor Gö6976 was purchased from Beyotime (Haimen, China). PKCεV1-2 was obtained from Medbio (Shanghai, China). Dihydroethidium (DHE) was purchased from Beyotime.
Cell Counting Kit-8 (CCK-8) was obtained from Topscience. Lipid Peroxidation Malondialdehyde (MDA) Kit and Total Superoxide Dismutase (SOD) Assay Kit were purchased from Beyotime. In Situ Cell Death Detection Kit was purchased from Roche Co. (Mannheim, Germany). Lactate Dehydrogenase (LDH) Assay Kit, Creatine Kinase MB (CK-MB) isoenzyme Assay Kit and Coenzyme II (NADPH/NADP+) Content Test Kit were purchased from Nanjing Jiancheng Bioengineering Institute (Nanjing, China).
Animals
Age-matched male C57BL/6J mice (8- to 10-week-old) were provided by the Experimental Animal Center of the Fourth Military Medical University (Xi’an, China). All animals were kept at controlled environmental conditions (22 ± 2°C with a 12 h light/dark cycle), and allowed free access to food and water. All experiments were performed in strict accordance with the recommendations in the Guide for the National Institutes of Health Guidelines for the Use of Laboratory Animals (Publication No. 85–23, revised 1996), and approved by the Animal Care Committee of Northwestern Polytechnical University (Xi’an, China). The mice were allowed to adapt to the new conditions for 1 week before being used in this experiment.
Myocardial Ischemia/Reperfusion and Electrophysiological Examinations
The mouse model of myocardial I/R injury was established by occluding the left anterior descending coronary artery (LAD) for 30 min followed by reperfusion for 120 min. Briefly, mice were anesthetized by pentobarbital sodium (60 mg/kg) after fasted overnight. After endotracheal intubation, mouse heart was exposed between the third and fourth ribs, and LAD was ligated with a 7-0 silk suture. After 30 min of ischemia, the slipknot was released, and myocardium was reperfused for 120 min. Mice in the sham group underwent the same surgical procedures, but without ligation. GDL (5 mg/kg) or its control vehicle (saline) was intraperitoneally administrated at 10 min pre-reperfusion. Surface lead II electrocardiogram (ECG) was continuously recorded during I/R by a physiologic signal-acquisition system (RM6240; Chengdu instrument factory, Chengdu, China), and the arrhythmia incidence and score were assessed according to the Lambeth Conventions (Curtis et al., 2013).
Measurement of Infarct Size
The myocardial infarct size was evaluated by TTC-Evans blue double staining. After 2 h of reperfusion, the LAD was re-tied to stop the blood flow, and 1 mL of 2% Evans blue dye (Beijing Solarbio Science & Technology Co., Ltd., Beijing, China) was infused into the hearts via the ascending aorta. After that, the heart was sliced into 1 mm sections, and incubated with 1% TTC at 37°C for 20 min. Finally, the slices were store in 4% paraformaldehyde solution before digitally photographed. The area of left ventricle (LV), the area at risk (AAR), and infarct size (INF) were assessed with FIJI (ImageJ) software (National Institutes of Health, United States).
Evaluation of Apoptosis
At the end of reperfusion, cardiac apoptosis was evaluated by the Terminal deoxynucleotidyl transferase dUTP nick end labeling (TUNEL) assay using the In Situ Cell Death Detection Kit (Roche) according to the manufacturer’s instructions.
Isolation of Neonatal Rat Cardiomyocytes
Neonatal rat cardiomyocytes (NRCM) were isolated from the hearts of 1- to 3-day-old Sprague–Dawley rats. Briefly, rat hearts were removed, minced and digested with 0.1% Type II collagenase and 0.25% Trypsin. Cardiomyocytes were isolated by differential detachment and verified under the microscope. Isolated NRCM were cultured in high glucose Dulbecco’s modified Eagle’s medium (DMEM) supplemented with 10% fetal bovine serum (FBS) and 1% penicillin/streptomycin solution in a humidified 5% CO2 incubator (Boxun, Shanghai, China) at 37°C.
Hypoxia/Reoxygenation
A cellular model of H/R was established to mimic myocardial I/R injury in vitro. Briefly, hypoxia was induced by incubation of NRCM cells with glucose-free and serum-free DMEM medium in an anaerobic chamber (95% N2, 5% CO2) at 37°C for 12 h. To mimic reperfusion, the hypoxic NRCM cells were cultured in serum-free DMEM medium (with 5.5 mM glucose) under normoxic conditions for 2 h at 37°C.
D-glucono-1,5-lactone (1 μM) was administrated before the onset of reoxygenation. To investigate whether blocking PI3K/Akt signaling or MEK/ERK signaling could attenuate GDL-conferred cardioprotection against H/R, ERK inhibitor U0126 (10 μM) or PI3K inhibitor Wm (100 nM) administrated concurrently with GDL before the onset of reoxygenation.
Immunoblotting
At the end of reperfusion or reoxygenation, heart tissue or cell culture was collected, and proteins were isolated using radio-immunoprecipitation assay (RIPA) lysis buffer containing protease inhibitor cocktail (Roche), and subsequently quantitated by BCA kit (Pierce Chemical Company, Rockford, IL, United States). Equal amount of proteins were loaded and separated by 10 or 12% polyacrylamide gel electrophoresis (SDS-PAGE), and transferred to polyvinylidene difluoride (PVDF) membranes. The membranes were blocked with 5% skim milk for 1 h, and subsequently incubated with primary antibodies against p-ERK (1:1000; Cell Signaling Technology/CST, Danvers, MA, United States), ERK (1:1000; Proteintech, Wuhan, China), p-Akt (1:1000; CST), Akt (1:1000; CST), Bcl2 (1:1000; Proteintech), Bax (1:1000; Proteintech), Caspase3 (1:1000; Proteintech), The nuclear factor erythroid 2 related factor 2 (NRF2; 1:1000; Proteintech), SOD2 (1:1000; Proteintech) and PKCε (1:1000; Proteintech) overnight at 4°C. Subsequently, the membranes were incubated with appropriate secondary antibodies for 1–2 h. Finally, the blots were visualized by Tanon imaging system (Tanon Science & Technology Co., Ltd., China) and quantified by densitometry using Quantity One software (Bio-Rad, Hercules, CA, United States).
Immunofluorescence
Cardiac tissues were fixed overnight with 4% paraformaldehyde, embedded in paraffin, and sectioned with a microtome in 5 μm slices. Myocardial slices were stained with PKCε antibodies (Proteintech), and photographed by an optical microscope (Nikon, Melville, NY, United States). For the colocalization analysis, Manders’ coefficient was calculated using Coloc2 plugin in FIJI (ImageJ) image processing software.1
Statistical Analysis
All data were presented as means ± SEM and performed using Graph Pad Prism 8.0 software. Student’s t-test or Chi-square test and Fisher’s exact test (for arrhythmia incidence) was used to determine the significant difference among two groups, while one-way ANOVA with Bonferroni’s post hoc test was used among more than two groups. Statistical significance was accepted at P < 0.05.
Results
D-Glucono-1,5-Lactone Exerts Cardioprotection Against Myocardial Ischemia/Reperfusion Injury
D-glucono-1,5-lactone, a derivate of glucose, is a ring-shaped molecule characterized by a tetrahydropyran substituted by three hydroxyl groups, one ketone group, and one hydroxymethyl group (its chemical structure is displayed in Figure 1A). The schematic of the experimental design in vivo is shown in Figure 1B. GDL administration reduced myocardial infarction size in IR + GDL group (25 ± 3%) compared with I/R group (40 ± 2%) (Figure 1C). In line with this, serum levels of cardiac injury markers, LDH and CK-MB were increased following I/R injury, while were decreased with GDL pre-treatment (Figures 1D,E).
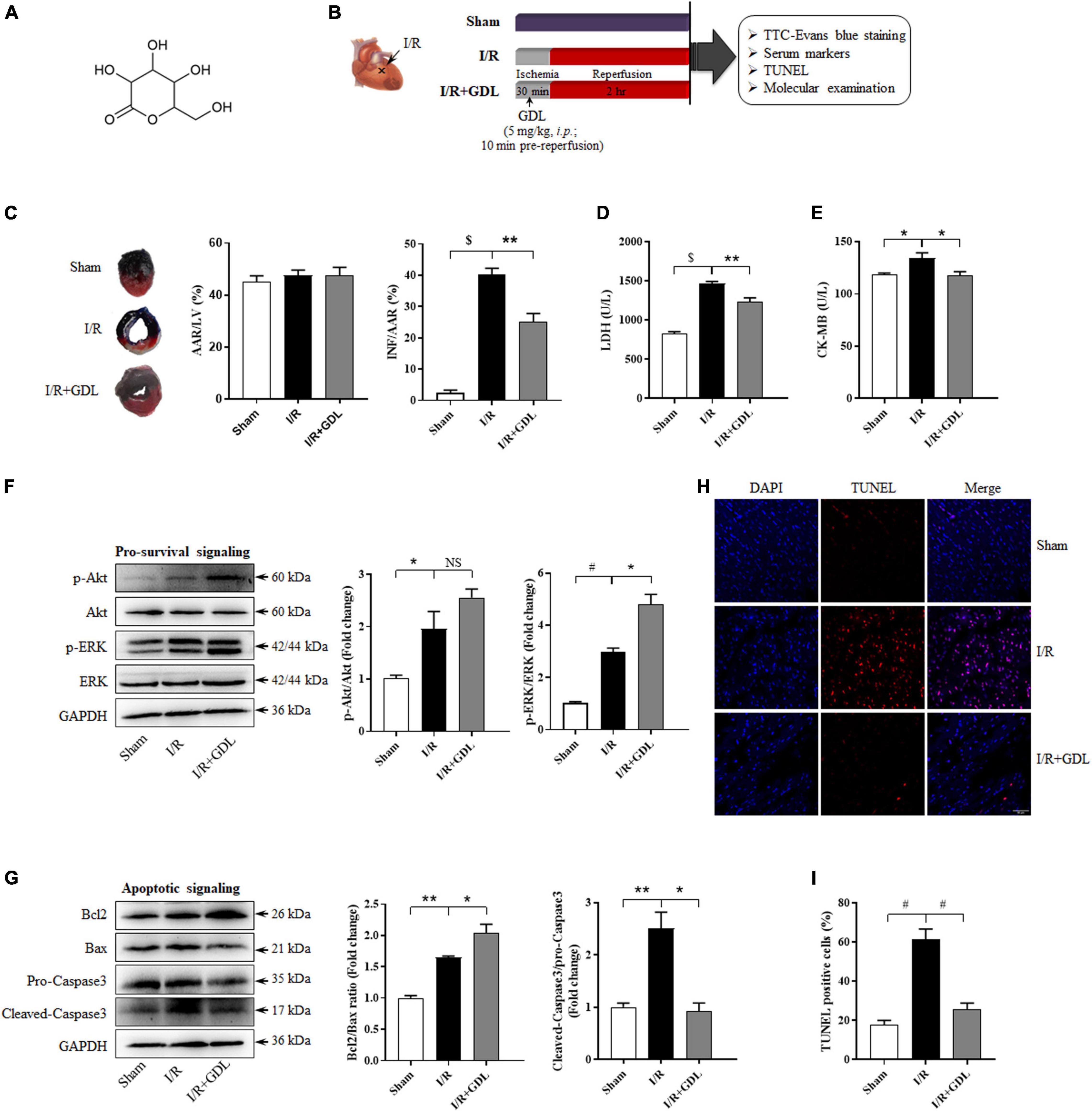
Figure 1. D-glucono-1,5-lactone protected against myocardial ischemia/reperfusion injury in mice. (A) The chemical structure of GDL. Mice were randomly divided into three groups: Sham, I/R group, and I/R + GDL group. (B) The schematic of the experimental design in vivo. (C) Myocardial infarction was assessed by TTC-Evans blue double staining (n = 5). (D) Serum LDH and (E) CK-MB activities were evaluated as an index for cardiac injury via commercial kits (n = 8–12). (F) Representative images and Western blot analysis of pro-survival kinases (ERK and Akt) in cardiac tissues of each group (n = 5). (G) Representative images and Western blot analysis of apoptosis-related proteins (Bcl2, Bax, and Caspase3) in cardiac tissues of each group (n = 5). (H–I) TUNEL staining and quantification of apoptosis in heart tissue section among groups (n = 5; scale bar = 50 μm). *P < 0.05, **P < 0.01, #P < 0.001, and $P < 0.0001 between indicated groups.
Imbalance between pro-survival and apoptotic signaling is believed to be central to myocyte dysfunction following I/R injury. Thus, we assessed the expression and (or) activity of key players in pro-survival (Akt and ERK) and apoptotic (Bcl2, Bax, and Caspase3) signaling pathways by Western blot analysis, demonstrating that GDL treatment stimulated ERK phosphorylation, especially p42 ERK, whereas no significant difference in Akt expression and phosphorylation was observed between I/R and I/R + GDL mice (Figure 1F). GDL administration following I/R decreased apoptotic signaling in cardiac tissues as evidenced by increased Bcl2/Bax ratio and decreased Caspase3 activation (Figure 1G). In agreement with this, the terminal deoxynucleotidyl transferase (TdT) dUTP nick-end labeling (TUNEL) staining showed that GDL decreased I/R-induced apoptosis in cardiac tissue (Figures 1H,I).
D-Glucono-1,5-Lactone Protects Against Reperfusion-Induced Arrhythmias
Reperfusion-induced arrhythmia is a common clinical manifestation of myocardial I/R injury. The surface ECG was recorded in each group, and arrhythmias were analyzed 30 min post-reperfusion according to the Lambeth conventions (Curtis et al., 2013). ECG showed that arrhythmias were commonly seen during the onset of reperfusion, of which, mostly were premature ventricular contraction (PVC), rarely were ventricular tachycardia (VT), and none were ventricular fibrillation (VF). Representative examples of different ventricular arrhythmias (PVC and VT) were illustrated in Figure 2A. GDL decreased reperfusion-induced arrhythmias (Figure 2B), mainly due to a decrease of PVC but not VT occurrence (Figures 2C,D). Of note, we found that QT interval and corrected QT (QTc) interval, a risk factor for the development of ventricular arrhythmia, were decreased in mice with GDL treatment (Figures 2E,F). Heart rate showed no apparent changes during baseline, ischemia or reperfusion in I/R-injured mice with or without GDL treatment (Figure 2G).
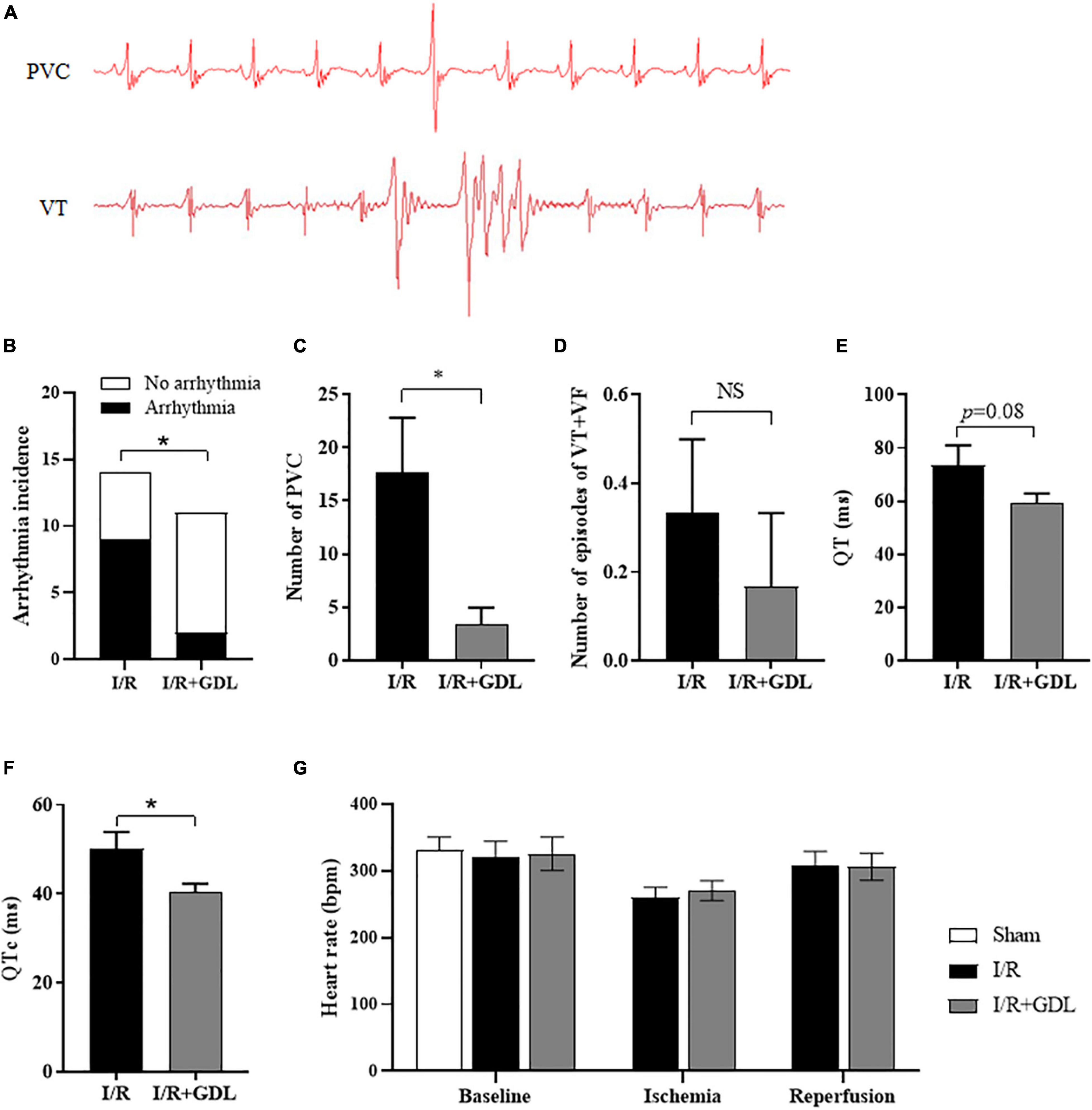
Figure 2. D-glucono-1,5-lactone reduced reperfusion-induced arrhythmias. (A) Representative examples of different ventricular arrhythmias (PVC and VT) during 30 min post-reperfusion. Arrhythmia incidence (B), number of PVC (C), number of VT + VF (D), QT duration (E) and corrected QT (F) in I/R-injured mice in the presence or absence of GDL treatment (n = 6). Heart rate (G) of all sections, including baseline, ischemia and reperfusion (n = 6). *P < 0.05 between indicated groups.
D-Glucono-1,5-Lactone Decreases Oxidative Stress Induced by Ischemia/Reperfusion
Although GDL is known as an antioxidant, its regulatory role in oxidative stress in vivo, especially in the context of I/R, was not investigated. As shown in Figures 3A,B, exposure to I/R displayed an increase of MDA and a decrease of SOD2 activity in either serum or heart tissue. GDL treatment decreased MDA levels both in serum and heart tissue of I/R-injured mice, yet no significant changes of SOD2 activity were observed after GDL treatment (Figures 3A,B).
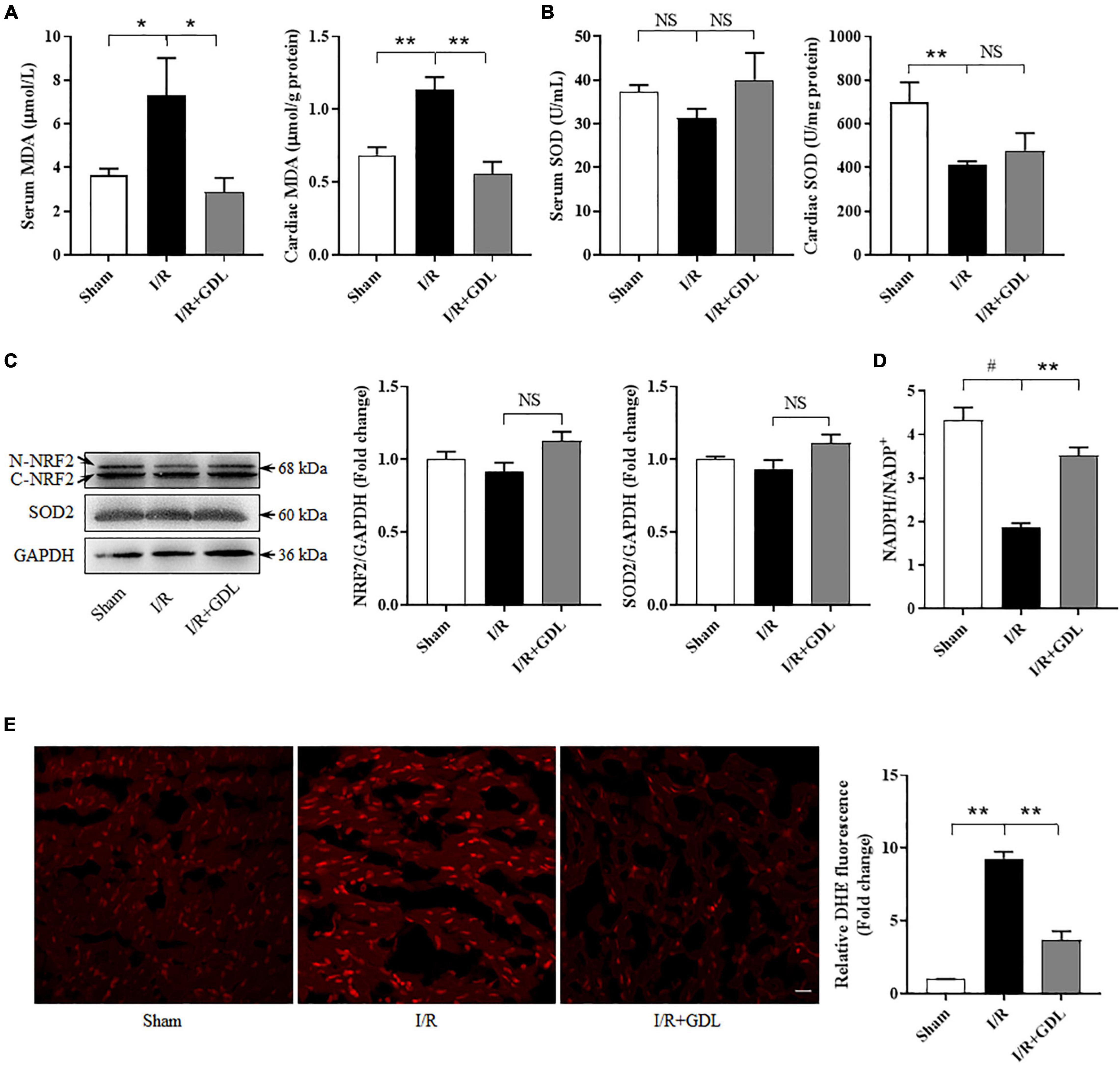
Figure 3. D-glucono-1,5-lactone attenuated reperfusion-induced oxidative stress. (A) MDA levels in serum and cardiac tissue in sham, I/R, and I/R + GDL groups (n = 4–6). (B) SOD activities in serum and cardiac tissue in sham, I/R, and I/R + GDL groups (n = 6–7). (C) Representative images and Western blot analysis of NRF2 and SOD2 expression in sham, I/R, and I/R + GDL groups (n = 5). (D) NADPH/NADP+ ratio among groups (n = 3–4). (E) DHE staining of heart tissue section among groups (n = 3; scale bar = 20 μm). *P < 0.05, **P < 0.01, and #P < 0.001 between indicated groups. NS, not significant.
Oxidative stress refers to the pathological process of tissue damage caused by excessive ROS production and/or reduced ROS scavenging capacity. We first evaluated the expression of antioxidant enzymes including NRF2 and SOD2. In line with the results of our studies regarding to SOD activities, no apparent changes of NRF2 and SOD2 expression were observed among groups (Figure 3C). NADPH, a key component in the cellular anti-oxidative defense system, was decreased in the heart of I/R mice, an effect which could be reversed by GDL treatment (Figure 3D). Next, we measured total ROS levels using DHE staining, showing that GDL treatment decreased I/R-induced ROS levels (Figure 3E).
D-Glucono-1,5-Lactone Increases Cell Viability of Neonatal Rat Cardiomyocytes Cells Following Hypoxia/Reoxygenation Injury
Next, we evaluated the protective effect of GDL and the underlying mechanism in vitro. Our results demonstrated that high concentration of GDL (>100 μM) had a cytotoxic effect on NRCM cells, whereas GDL concentrations at 1 μM and 10 μM did not show this adverse effect (Figure 4A). In the context of H/R injury, GDL concentrations at 1 μM, 10 μM, 100 μM and 1 mM showed beneficial effects against H/R injury, an effect that was diminished when GDL concentration was at 10 mM (Figure 4B). Therefore, GDL concentration in the following experiments was set to be 1 μM when GDL exerted cardioprotective effects against H/R injury and had less cytotoxic effect. As expected, GDL (1 μM) treatment decreased apoptotic signaling in H/R-injured NRCM cells, as evidenced by decreased Caspase3 activation and increased Bcl2/Bax ratio (Figure 4C). In agreement with this result, GDL treatment activated pro-survival signaling including ERK and Akt (Figure 4D).
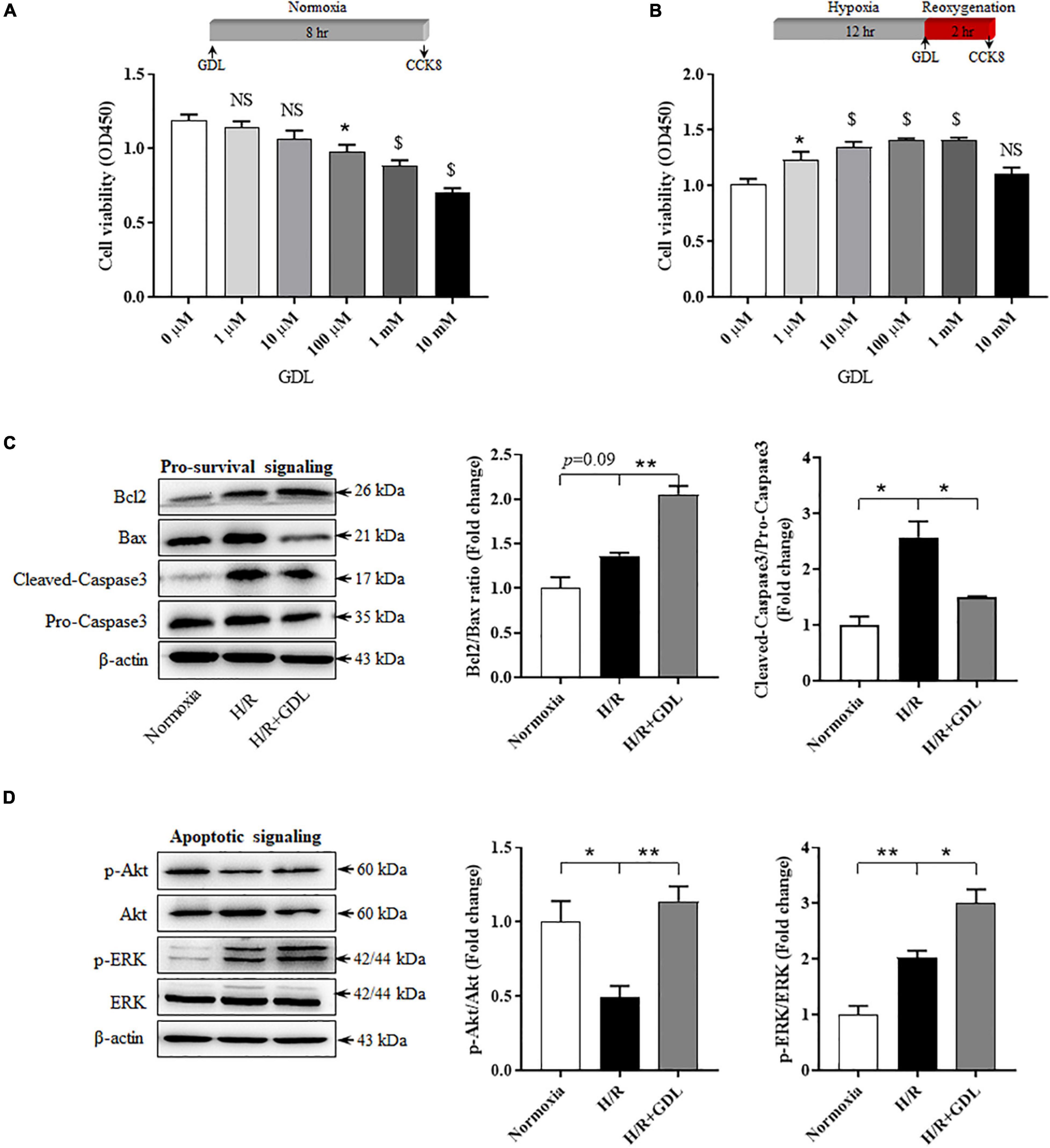
Figure 4. D-glucono-1,5-lactone enhanced cell viability of NRCM following H/R injury. (A) Dose-dependent effect of GDL (0 μM, 1 μM, 10 μM, 100 μM, 1 mM, and 10 mM) on cell viability of NRCM cells by CCK-8 test. (B) Dose-dependent effect of GDL (0 μM, 1 μM, 10 μM, 100 μM, 1 mM, and 10 mM) on cell viability of H/R-injured NRCM cells by CCK-8 test. (C) Representative images and Western blot analysis of apoptosis-related proteins (Bcl2, Bax, and Caspase3) in normoxia, H/R and H/R + GDL groups. (D) Representative images and Western blot analysis of pro-survival kinases (ERK and Akt) among groups. *P < 0.05, **P < 0.01, and $P < 0.0001 between indicated groups. Data shown are representive of five independent experiments.
Inhibition of Extracellular Signal-Regulated Kinase Signaling via U0126 Attenuates D-Glucono-1,5-Lactone Protection Against Hypoxia/Reoxygenation Injury
Both in vivo and in vitro studies have shown that GDL-conferred cardioprotection was associated with increased pro-survival signaling (ERK or Akt). CCK-8 assay showed that blocking ERK signaling by its inhibitor U0126 attenuated GDL-induced cell survival, whereas inhibiting PI3K/Akt signaling via Wortmannin did not show this effect (Figure 5A). Supportively, GDL treatment activated ERK signaling, reaching a peak at 2 h (Figure 5B). In addition, either low or high concentration of GDL strongly activated ERK signaling after treating cardiomyocytes for 2 h (Figure 5C). Western blot analysis showed that blocking ERK signaling attenuated GDL-induced inhibition of Caspase3, yet had no significant effect on Bcl2/Bax ratio (Figures 5D–G).
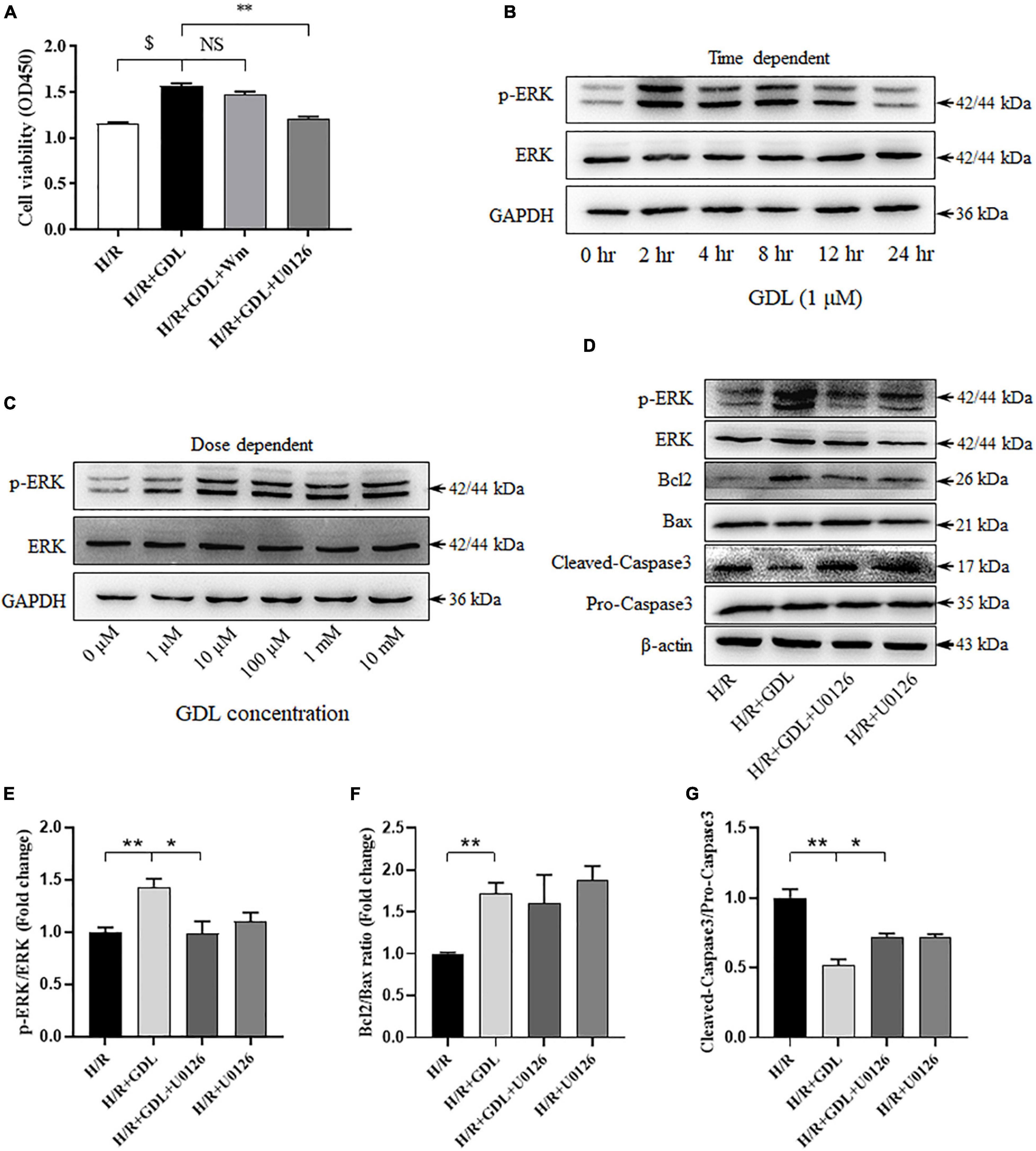
Figure 5. Extracellular signal-regulated kinase signaling contributed to GDL-conferred cardioprotection against H/R injury. (A) The effect of MEK/ERK inhibitor U0126 (10 μM) or PI3K/Akt inhibitor Wortmannin (Wm; 100 nM) on GDL-induced cardioprotection against H/R injury. (B) Time-dependent effect of GDL (0, 2, 4, 8, 12, and 24 h) on ERK expression and phosphorylation by Western blot analysis. (C) Dose-dependent effect of GDL (0 μM, 1 μM, 10 μM, 100 μM, 1 mM and 10 mM) on ERK expression and phosphorylation by Western blot analysis. (D–G) Representative images and Western blot analysis of apoptosis-related proteins (Bcl2, Bax, and Caspase3) and pro-survival ERK signaling in H/R, H/R + GDL, H/R + GDL + U0126, H/R + U0126 groups. *P < 0.05 and **P < 0.01 between indicated groups. NS, not significant. Data shown are representive of five independent experiments.
Protein Kinase C Is a Potential Target of D-Glucono-1,5-Lactone by Bioinformatics Analysis and Molecular Docking Studies
To further understand how GDL regulates ERK signaling, we used Swiss Target Prediction databases2 to sort out the potential targets of GDL. Species were selected as “Homo sapiens,” and a total of 23 targets were obtained under the condition of probability greater than 0.1 (Supplementary Table 1). Among them, Protein kinase C (PKC) isoforms, including PKCδ, PKCα, PKCγ, PKCε, PKCη, PKCθ, stood out as the most possible target for GDL.
Next, Autodock Vina was used to predict the binding modes of GDL with PKC (Trott and Olson, 2009). The crystal structure of PKC (PDB code 1XJD) was obtained from the Protein Data Bank (PDB), and GDL was docked into PKC structure by Autodock Vina. Among twenty conformation generated, the best one has been nominated based on lowest binding energy (-5.0 Kcal/mol). Protein-ligand interaction of docked complex was visualized by PyMOL software3 (Figure 6A). The hydrogen bonds were analyzed by PyMOL software, showing GDL interacts with PKC by forming hydrogen bonds with Q688, F691, and K413 (Figure 6B). Electrostatic surface of PKC was generated by APBS tool, showing GDL buried in a positive charged pocket of PKC (Figure 6C).
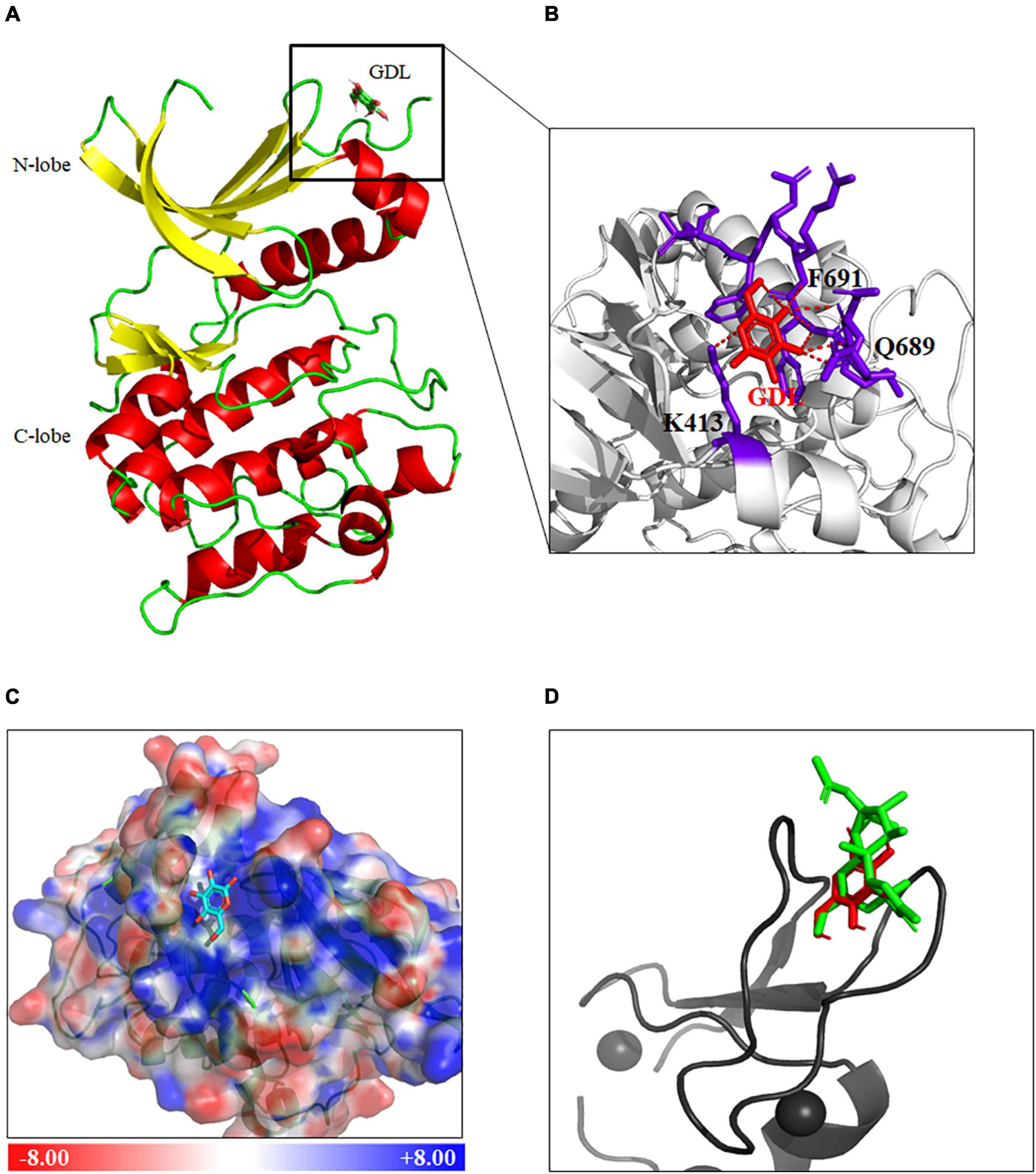
Figure 6. Protein kinase C is a putative target of GDL by bioinformatics analysis and molecular docking studies. (A) Docking of GDL into PKC (PDB code 1XJD) by AutoDock Vina, and the protein-ligand interaction of PKC and GDL was visualized by PyMOL (www.pymol.org). (B) Formation of hydrogen bonds between GDL and PKC (Red, GDL). (C) Electrostatic surface of PKC structure generated by APBS tool (Blue, positive charge; red, negative charge; Cyan, GDL). (D) Docking of GDL into PKC (PDB code 1PTR) by AutoDock Vina, and comparison of docking poses in PKC between Phorbol-13-acetate (Green) and GDL (Red).
The NMR structure of PKC binding with Phorbol-13-acetate (PDB code 1PTR) has been revealed before, thus, we try to compare the binding poses of GDL with Phorbol-13-acetate. GDL was docked into the crystal structure of PKCδ C1 domain by Autodock Vina (binding energy -5.0 Kcal/mol), and we can see GDL occupied the same position in PKC structure as Phorbol-13-acetate after structural alignment (Figure 6D).
Protein Kinase C Isoform PKCε Is Considered as the Direct Target of D-Glucono-1,5-Lactone by Functional Identification
Protein kinase C family falls into three groups: the classical PKC (cPKC; α, βI, βII, and γ), atypical PKC (aPKC; ζ and λ/ι) or novel PKC (nPKC; δ, ε, η, θ, μ, and υ). To investigate whether or which PKC isoform contributes to GDL-induced ERK phosphorylation and cardioprotection, we used different PKC inhibitors, including Chelerythrine (a general PKC inhibitor), Gö6976 (an inhibitor of cPKC), Rottlerin (an inhibitor of PKCδ), and PKCεV1-2 (a specific inhibitor of PKCε). Western blot analysis showed that Chelerythrine (5 μM), Gö6976 (1 μM), and Rottlerin (25 μM) had no effect on GDL-induced ERK phosphorylation, whereas PKCε specific inhibitor PKCεV1-2 (20 μM) attenuated this effect (Figures 7A,B). Immunofluorescence staining showed that i.p. administration of GDL in mice increased PKCε translocation into nuclear (Figures 7C,D). Cell viability assay showed that PKCεV1-2 attenuated GDL-conferred cardioprotection against H/R injury (Figure 7E).
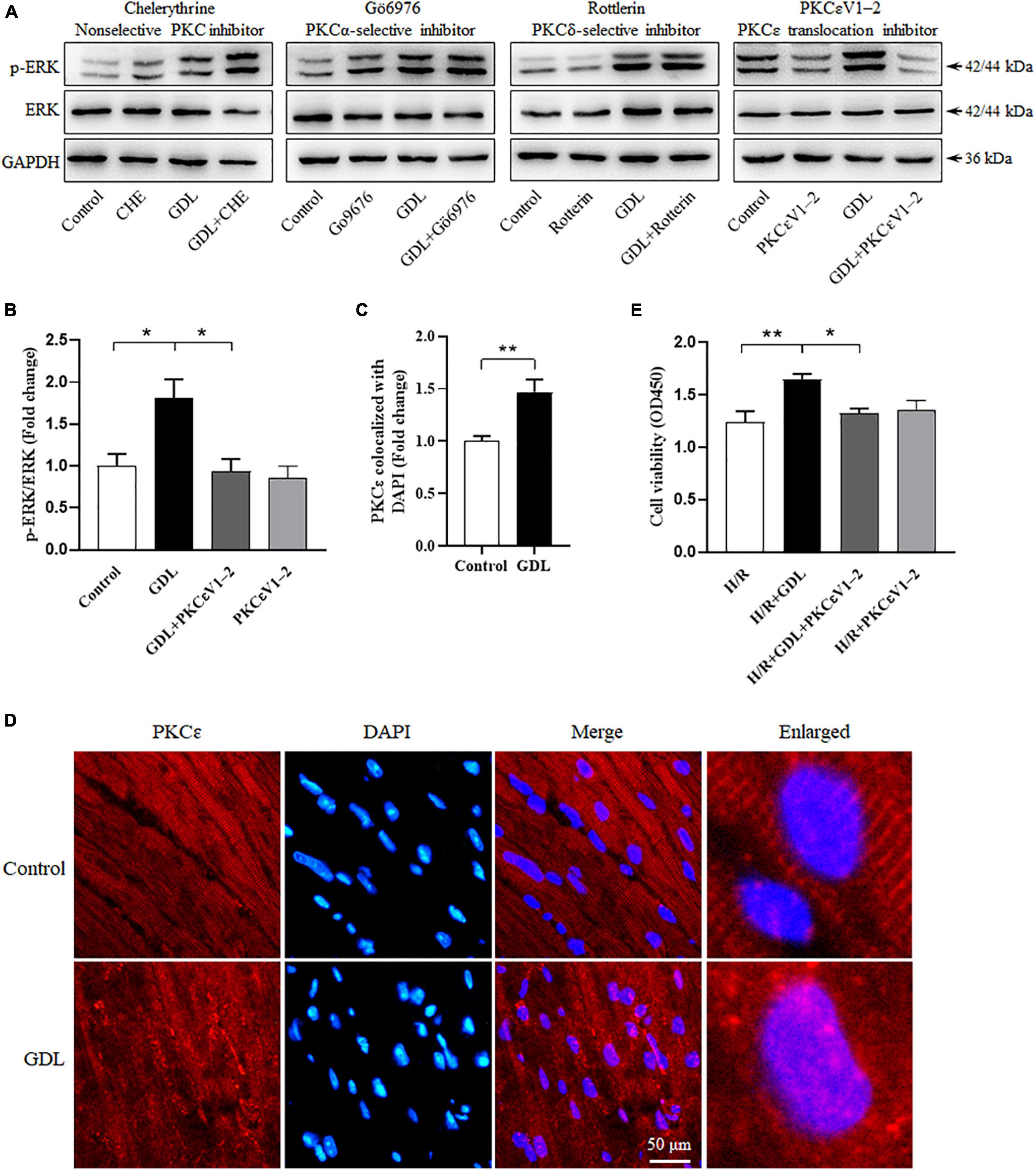
Figure 7. Protein kinase C isoform PKCε is identified as the direct target of GDL by functional analysis. (A) Representative Western blot images of ERK expression and phosphorylation in H/R-injured NRCM cells following the treatment of PKC inhibitors (Chelerythrine, Gö6976, Rottlerin or PKCεV1-2). (B) Quantification analysis of ERK phosphorylation in H/R-injured NRCM cells following PKCεV1-2 treatment. (C,D) Immunofluorescence staining of PKCε nuclear translocation in the heart of mice treated with or without GDL. (E) The effect of PKCεV1-2 on GDL-induced cardioprotection against H/R injury. *P < 0.05 and **P < 0.01 between indicated groups. Data shown are representive of five independent experiments.
Discussion
D-glucono-1,5-lactone is a widely used food additive and presents in a variety of natural products and fermented food. However, its roles in cardiovascular diseases remain elusive. In this study, using a mouse model of I/R injury and a cellular model of H/R mimicking this process, we revealed that GDL administration (5 mg/kg, i.p.) attenuated I/R injury and reperfusion-induced arrhythmias, and pharmacological inhibition of ERK signaling attenuated GDL-conferred cardioprotection. Bioinformatics analysis and molecular docking studies revealed that PKC is a potential target for GDL, and subsequent functional analysis demonstrated PKC isoform PKCε as its direct target. Taken together, our results demonstrated that natural product GDL acts as a direct activator of PKCε and, thus, provides cardioprotection against I/R injury via activation of ERK signaling (Figure 8).
In human body, besides derived from foods, GDL is also a metabolite of gut microbiota, thus having the capacity to affect physiological and pathophysiological processes when the intestinal homeostasis is altered. Zhao et al. (2018) demonstrated that fecal GDL increased about twofold in normal human subjects after half-marathon race compared with those before race. A datasets of caecal metabolites of normal diet and high-fat-diet (HFD) mice (MTBLS545) available from Metabolights4 was analyzed and profiled, demonstrating an increase of caecal GDL levels in HFD-induced obese mice (Supplementary Figure 1A; Zheng et al., 2017). Of note, another dataset of metabolomics of mice inoculated with human microbiota available from Metabolomics Workbench (ST0007455) showed that an increase of GDL levels in peripheral blood at 35 days post-inoculation of human microbiota compared with that at 6 days (Supplementary Figure 1B). These results demonstrated that GDL might be a critical mediator between gut microbiota and human health.
D-glucono-1,5-lactone is known as a potent antioxidant mainly for two reasons: (1) GDL is a natural PHA, which is capable of chelating metals and may function as a free radical scavenger (Bernstein et al., 2004); (2) GDL regulates redox balance via entering into the PPP pathway (Wushensky et al., 2018). Supportively, we found that GDL treatment decreased oxidative stress in reperfusion injured mice, which is probably due to the increase of NADPH production and the decrease of ROS production (Figure 3). NADPH is a major component to detoxify ROS by reducing glutathione, and decreased NADPH is associated with the loss of antioxidant activity and the increase of ROS production. Our results suggested that GDL increased NADPH/NADP+ ratio in cardiomyocytes from I/R-injured mice, thereby enhancing cellular ROS-scavenging capacity. However, the detailed mechanism how GDL increased NADPH production needs further elucidation.
MEK/ERK signaling and PI3K/Akt signaling are two major components of the pro-survival reperfusion injury salvage kinase (RISK) signaling pathway. Our results demonstrated that GDL exerts cardioprotection via ERK but not Akt signaling. Our in vivo experiments showed that GDL treatment activated ERK but not Akt signaling in mice subjected to I/R (Figure 1F). Consistently, GDL treatment increased ERK signaling in H/R-injured NRCM cells (Figure 4D). In addition, GDL treatment activated ERK in a time-dependent manner, reaching a peak value at 2 h post treatment (Figure 5B). However, our results also showed that high concentration of GDL (>10 μM) is toxic for cardiomyocytes (Figure 4A), probably via different mechanisms.
Extracellular signal-regulated kinase signaling was highly associated with the biological effects of lactone, yet the actual effects (inhibitory or activated) of lactones on ERK signaling is inconsistent depending on the lactone type and cell type. GDL is a δ-lactone, and we showed that GDL increased ERK phosphorylation in vivo and in vitro (Figures 1, 4, 5). In line with our results, Emblicanin A and Emblicanin B, δ-lactones from Emblica officinalis, up-regulated ERK signaling (Sumitra et al., 2009). Macro-cyclic lactone bryostatin1 activated PKC and downstream ERK in many cell types (Zhang and Liu, 2002). However, Goniothalamin and Rasfonin, also members of δ-lactone, were found to inhibit ERK phosphorylation in different cancer cells (Xiao et al., 2014; Innajak et al., 2016). Moreover, the effects of sesquiterpene lactones on ERK signaling were widely explored, showing different effects following different sesquiterpene lactone treatment (Kim et al., 2005, 2008; Michalak et al., 2019).
Protein kinase C is involved in a variety of cellular processes including cell proliferation, differentiation, hypotrophy, apoptosis, and modulates various signaling pathways including ERK signaling pathway (Singh et al., 2017). Herein, Swiss target prediction showed that PKC isoforms are potential targets of GDL, which was further confirmed by functional identification. Subsequently, the interaction of PKC with GDL was simulated by using molecular docking Autodock Vina, demonstrating that GDL could bind to the C1 domain of PKC, resulting in its activation, which is consistent with other PKC activators DAG or phorbol ester (Zhang et al., 1995). Of note, it has been reported that diacylglycerol-lactone 130C037 has different affinity for PKC isoforms (higher affinity for PKCδ and lower affinity for PKCα) (Pu et al., 2005), showing its binding preference to PKC isoforms. Likewise, functional analysis showed that GDL activated ERK signaling mainly via PKCε but not other PKC isoforms (Figure 7). In the context of myocardial I/R injury, PKC isoforms are believed to have distinct roles in regulating reperfusion injury (Budas et al., 2007; Chen et al., 2021). Generally speaking, inhibition of PKCα, PKCβ, and PKCδ attenuated I/R injury, while activation of PKCε protects the heart against reperfusion injury (Budas et al., 2007; Kong et al., 2008). In this study, we found that GDL stimulated ERK phosphorylation and resultant cardioprotection mainly via activating PKCε (Figure 7). These data suggested that GDL has a clinical potential as a cardioprotective drug against I/R injury.
Both bioinformatics analysis and functional identification showed GDL is a potent activator of PKCε, thereby exerts cardioprotection against I/R injury; however, direct biochemical evidence for the binding of GDL to PKCε is still limited, and needs further exploration.
Conclusion
Taken together, our results demonstrated that a natural metabolite GDL provides cardioprotection against myocardial I/R injury via targeting PKC and subsequent activation of ERK signaling.
Data Availability Statement
The datasets presented in this study can be found in online repositories. The names of the repository/repositories and accession number(s) can be found in the article/Supplementary Material.
Ethics Statement
The animal study was reviewed and approved by the Animal Care Committee of Northwestern Polytechnical University.
Author Contributions
XZ, JX, and XQ took the responsibility for study design. XQ, BL, and FG performed the experiments and data acquisition. XQ, JX, ZC, and YH analyzed the data. XQ and XZ wrote the manuscript. All authors contributed to manuscript revision, read, and approved the submitted version.
Funding
This work was supported by the National Natural Science Foundation of China (Grant Numbers 31871172, 32000808, 31871146, and 81800360).
Conflict of Interest
The authors declare that the research was conducted in the absence of any commercial or financial relationships that could be construed as a potential conflict of interest.
Publisher’s Note
All claims expressed in this article are solely those of the authors and do not necessarily represent those of their affiliated organizations, or those of the publisher, the editors and the reviewers. Any product that may be evaluated in this article, or claim that may be made by its manufacturer, is not guaranteed or endorsed by the publisher.
Supplementary Material
The Supplementary Material for this article can be found online at: https://www.frontiersin.org/articles/10.3389/fphys.2022.856699/full#supplementary-material
Abbreviations
AAR, area at risk; CHE, Chelerythrine; DHE, Dihydroethidium; ECG, electrocardiogram; ERK, extracellular signal-regulated kinase; GDL, D-glucono-1,5-lactone; HR, heart rate; H/R, hypoxia/reoxygenation; I/R, ischemia/reperfusion; LDH, lactate dehydrogenase; LV, left ventricle; MDA, malondialdehyde; NRF2, the nuclear factor erythroid 2 related factor 2; PHA, polyhydroxy acid; PKC, protein kinase C; PVC, premature ventricular contraction; ROS, reactive oxygen species; SOD, superoxide dismutase; TUNEL, the terminal deoxynucleotidyl transferase (TdT) dUTP nick-end labeling; VF, ventricular fibrillation; VT, ventricular tachycardia.
Footnotes
- ^ https://imagej.net/Coloc2
- ^ http://swisstargetprediction.ch/
- ^ www.pymol.org
- ^ https://www.ebi.ac.uk/metabolights/index
- ^ https://www.metabolomicsworkbench.org/
References
Audina, M. (2021). A review on anti-aging properties of polyhydroxy acid. World J. Pharm. Res. 10, 137–141.
Bernstein, E. F., Brown, D. B., Schwartz, M. D., Kaidbey, K., and Ksenzenko, S. M. (2004). The polyhydroxy acid gluconolactone protects against ultraviolet radiation in an in vitro model of cutaneous photoaging. Dermatol. Surg. 30, 189–196. doi: 10.1111/j.1524-4725.2004.30060.x
Bowes, L. (2013). The science of hydroxy acids: mechanisms of action, types and cosmetic applications. J. Aesthet. Nurs. 2, 77–81. doi: 10.12968/joan.2013.2.2.77
Budas, G. R., Churchill, E. N., and Mochly-Rosen, D. (2007). Cardioprotective mechanisms of PKC isozyme-selective activators and inhibitors in the treatment of ischemia-reperfusion injury. Pharmacol. Res. 55, 523–536. doi: 10.1016/j.phrs.2007.04.005
Chen, L., Shi, D., and Guo, M. (2021). The roles of PKC-δ and PKC-ε in myocardial ischemia/reperfusion injury. Pharmacol. Res. 170:105716. doi: 10.1016/j.phrs.2021.105716
Curtis, M. J., Hancox, J. C., Farkas, A., Wainwright, C. L., Stables, C. L., Saint, D. A., et al. (2013). The Lambeth conventions (II): guidelines for the study of animal and human ventricular and supraventricular arrhythmias. Pharmacol. Ther. 139, 213–248. doi: 10.1016/j.pharmthera.2013.04.008
Geng, T., Shen, W.-W., Wang, J.-J., Huang, W.-Z., Wang, Z.-Z., and Xiao, W. (2018). Research development of ginkgo terpene lactones. Zhongguo Zhong Yao Za Zhi 43, 1384–1391. doi: 10.19540/j.cnki.cjcmm.20180312.001
Innajak, S., Mahabusrakum, W., and Watanapokasin, R. (2016). Goniothalamin induces apoptosis associated with autophagy activation through MAPK signaling in SK-BR-3 cells. Oncol. Rep. 35, 2851–2858. doi: 10.3892/or.2016.4655
Kim, S. H., Danilenko, M., and Kim, T. S. (2008). Differential enhancement of leukaemia cell differentiation without elevation of intracellular calcium by plant-derived sesquiterpene lactone compounds: HL-60 cell differentiation and sesquiterpene lactones. Br. J. Pharmacol. 155, 814–825. doi: 10.1038/bjp.2008.319
Kim, S. H., Oh, S. M., and Kim, T. S. (2005). Induction of human leukemia HL-60 cell differentiation via a PKC/ERK pathway by helenalin, a pseudoguainolide sesquiterpene lactone. Eur. J. Pharmacol. 511, 89–97. doi: 10.1016/j.ejphar.2005.02.008
Kong, L., Andrassy, M., Chang, J. S., Huang, C., Asai, T., Szabolcs, M. J., et al. (2008). PKCβ modulates ischemia-reperfusion injury in the heart. Am. J. Physiol. Heart Circ. Physiol. 294, H1862–H1870. doi: 10.1152/ajpheart.01346.2007
Li, X., Huang, L., Liu, G., Fan, W., Li, B., Liu, R., et al. (2020). Ginkgo diterpene lactones inhibit cerebral ischemia/reperfusion induced inflammatory response in astrocytes via TLR4/NF-κB pathway in rats. J. Ethnopharmacol. 249:112365. doi: 10.1016/j.jep.2019.112365
Liu, Q., Jin, Z., Xu, Z., Yang, H., Li, L., Li, G., et al. (2019). Antioxidant effects of ginkgolides and bilobalide against cerebral ischemia injury by activating the Akt/Nrf2 pathway in vitro and in vivo. Cell Stress Chaperones 24, 441–452. doi: 10.1007/s12192-019-00977-1
Liu, Y. B., Yu, B., Li, S. F., Fan, Y., Han, W., Yu, J. B., et al. (2011). Mechanisms mediating the cardioprotective effects of rapamycin in ischaemia-reperfusion injury. Clin. Exp. Pharmacol. Physiol. 38, 77–83. doi: 10.1111/j.1440-1681.2010.05467.x
Makowski, L., Zhou, C., Zhong, Y., Kuan, P. F., Fan, C., Sampey, B. P., et al. (2014). Obesity increases tumor aggressiveness in a genetically engineered mouse model of serous ovarian cancer. Gynecol. Oncol. 133, 90–97. doi: 10.1016/j.ygyno.2013.12.026
Michalak, B., Piwowarski, J. P., Granica, S., Waltenberger, B., Atanasov, A. G., Khan, S. Y., et al. (2019). Eupatoriopicrin inhibits pro-inflammatory functions of neutrophils via suppression of IL-8 and TNF-alpha production and p38 and ERK 1/2 MAP kinases. J. Nat. Prod. 82, 375–385. doi: 10.1021/acs.jnatprod.8b00939
Moreno, K. X., Harrison, C. E., Merritt, M. E., Kovacs, Z., Malloy, C. R., and Sherry, A. D. (2017). Hyperpolarized δ-[1-13C]gluconolactone as a probe of the pentose phosphate pathway. NMR Biomed. 30:e3713. doi: 10.1002/nbm.3713
Pu, Y., Perry, N. A., Yang, D., Lewin, N. E., Kedei, N., Braun, D. C., et al. (2005). A novel diacylglycerol-lactone shows marked selectivity in vitro among C1 domains of protein kinase C (PKC) isoforms α and δ as well as selectivity for RasGRP compared with PKCα. J. Biol. Chem. 280, 27329–27338. doi: 10.1074/jbc.M414132200
Singh, R. M., Cummings, E., Pantos, C., and Singh, J. (2017). Protein kinase C and cardiac dysfunction: a review. Heart Fail. Rev. 22, 843–859. doi: 10.1007/s10741-017-9634-3
Sumitra, M., Manikandan, P., Gayathri, V. S., Mahendran, P., and Suguna, L. (2009). Emblica officinalis exerts wound healing action through up-regulation of collagen and extracellular signal-regulated kinases (ERK1/2). Wound Repair Regen. 17, 99–107. doi: 10.1111/j.1524-475X.2008.00446.x
Trott, O., and Olson, A. J. (2009). AutoDock Vina: improving the speed and accuracy of docking with a new scoring function, efficient optimization, and multithreading. J. Comput. Chem. 31, 455–461. doi: 10.1002/jcc.21334
Wang, G., Dai, G., Song, J., Zhu, M., Liu, Y., Hou, X., et al. (2018). Lactone component from Ligusticum chuanxiong alleviates myocardial ischemia injury through inhibiting autophagy. Front. Pharmacol. 9:301. doi: 10.3389/fphar.2018.00301
Wu, D., and Cederbaum, A. I. (2003). Alcohol, oxidative stress, and free radical damage. Alcohol Res. Health 27, 277–284.
Wushensky, J. A., Youngster, T., Mendonca, C. M., and Aristilde, L. (2018). Flux connections between gluconate pathway, glycolysis, and pentose–phosphate pathway during carbohydrate metabolism in Bacillus megaterium QM B1551. Front. Microbiol. 9:2789. doi: 10.3389/fmicb.2018.02789
Xiao, Z., Li, L., Li, Y., Zhou, W., Cheng, J., Liu, F., et al. (2014). Rasfonin, a novel 2-pyrone derivative, induces ras-mutated Panc-1 pancreatic tumor cell death in nude mice. Cell Death Dis. 5:e1241. doi: 10.1038/cddis.2014.213
Zhang, G., Kazanietz, M. G., Blumberg, P. M., and Hurley, J. H. (1995). Crystal structure of the Cys2 activator-binding domain of protein kinase Cδ in complex with phorbol ester. Cell 81, 917–924. doi: 10.1016/0092-8674(95)90011-X
Zhang, J., Zhang, L., Shi, L., Zhao, Z., Xu, H., Liang, F., et al. (2017). Parthenolide attenuates cerebral ischemia/reperfusion injury via Akt/GSK-3β pathway in PC12 cells. Biomed. Pharmacother. 89, 1159–1165. doi: 10.1016/j.biopha.2017.03.009
Zhang, W., and Liu, H. T. (2002). MAPK signal pathways in the regulation of cell proliferation in mammalian cells. Cell Res. 12, 9–18. doi: 10.1038/sj.cr.7290105
Zhao, X., Zhang, Z., Hu, B., Huang, W., Yuan, C., and Zou, L. (2018). Response of gut microbiota to metabolite changes induced by endurance exercise. Front. Microbiol. 9:765. doi: 10.3389/fmicb.2018.00765
Zheng, X., Huang, F., Zhao, A., Lei, S., Zhang, Y., Xie, G., et al. (2017). Bile acid is a significant host factor shaping the gut microbiome of diet-induced obese mice. BMC Biol. 15:120. doi: 10.1186/s12915-017-0462-7
Keywords: gluconolactone, Ischemia/Reperfusion injury, PKC, ERK, ROS
Citation: Qin X, Liu B, Gao F, Hu Y, Chen Z, Xu J and Zhang X (2022) Gluconolactone Alleviates Myocardial Ischemia/Reperfusion Injury and Arrhythmias via Activating PKCε/Extracellular Signal-Regulated Kinase Signaling. Front. Physiol. 13:856699. doi: 10.3389/fphys.2022.856699
Received: 17 January 2022; Accepted: 22 February 2022;
Published: 14 March 2022.
Edited by:
Chun Yang, Nanjing Medical University, ChinaReviewed by:
Eylem Taskin, Adiyaman University, TurkeyAntonio Rodríguez-Sinovas, Vall d’Hebron Research Institute (VHIR), Spain
Copyright © 2022 Qin, Liu, Gao, Hu, Chen, Xu and Zhang. This is an open-access article distributed under the terms of the Creative Commons Attribution License (CC BY). The use, distribution or reproduction in other forums is permitted, provided the original author(s) and the copyright owner(s) are credited and that the original publication in this journal is cited, in accordance with accepted academic practice. No use, distribution or reproduction is permitted which does not comply with these terms.
*Correspondence: Jie Xu, eHVqaWVmbW11QDEyNi5jb20=; Xing Zhang, WmhhbmdYaW5nQGZtbXUuZWR1LmNu
†These authors have contributed equally to this work