- 1Division of Pulmonology, Department of Internal Medicine, Medical University of Graz, Graz, Austria
- 2Ludwig Boltzmann Institute for Lung Vascular Research, Graz, Austria
- 3Institute for Medical Informatics, Statistics and Documentation, Medical University of Graz, Graz, Austria
- 4Faculty of Physics, Babes-Bolyai University Cluj-Napoca, Cluj-Napoca, Romania
- 5Division of General and Thoracic Surgery, University Hospital Krems, Karl Landsteiner University of Health Sciences, Krems an der Donau, Austria
- 6Division of Thoracic Surgery, Department of Surgery, Medical University of Vienna, Vienna, Austria
- 7Experimental Anesthesiology, Department of Anesthesiology and Intensive Care Medicine, Medical University of Graz, Graz, Austria
Background: NT-proBNP and GDF-15 are established blood-derived biomarkers for risk assessment in pulmonary hypertension (PH), despite limited sensitivity and specificity. Apelin has a crucial function in endothelial homeostasis, thus it might represent a new biomarker for PH. However, there are numerous circulating apelin isoforms, and their potential role in this setting is unknown. This study evaluated different apelin isoforms in PH patients and prospectively evaluated the role of apelin-17 in comparison with NT-proBNP and GDF-15 as diagnostic marker in idiopathic pulmonary arterial hypertension (IPAH).
Methods: Based on our pilot study, we performed a power calculation for apelin-13, apelin-17, apelin-36, as predictor of IPAH vs healthy controls. Apelin-17 provided the best discriminatory power, and accordingly, we enrolled n = 31 patients with IPAH and n = 31 matched healthy controls in a prospective study. NT-proBNP and GDF-15 was determined in all patients. ROC curve analysis was performed to assess the diagnostic value of the markers and their combinations.
Results: Apelin-17, NT-proBNP, and GDF-15 were significantly elevated in IPAH patients as compared to controls (p < .001). Apelin-17 detected IPAH with a sensitivity of 68% and a specificity of 93% at a cut-off value of >1,480 pg/ml (AUC 0.86, 95%CI:0.76–0.95) as compared to GDF-15 (sensitivity 86%; specificity 72%, AUC 0.81 (95%CI:0.7–0.92)) and NT-proBNP (sensitivity 86%; specificity 72% (AUC 0.85, 95%CI:0.75–0.95)). Combinations of these markers could be used to increase either specificity or sensitivity.
Conclusion: Apelin-17 appears to be suitable blood derived diagnostic marker for idiopathic pulmonary arterial hypertension.
1 Introduction
A biomarker aims to improve prognosis prediction or risk assessment or to be of diagnostic value. In pulmonary hypertension (PH) patients several blood-derived biomarkers have been predictive for prognosis e.g. creatinine, troponins, red cell distribution width, GDF-15, endostatin, free fatty acids, YKL-40, and uric acid to natriuretic peptides (Torbicki et al., 2003; Leuchte et al., 2007; Njaman et al., 2007; Nickel et al., 2008; Shah et al., 2008; Rhodes et al., 2011; Heresi et al., 2012; Chen et al., 2014; Damico et al., 2015; Simpson et al., 2019; Geenen et al., 2020; Huai et al., 2021; Hendriks et al., 2022), howeverin inthe international guidelines only NT-proBNP and BNP are recommended. (Humbert et al., 2022). Biomarkers that have a unique diagnostic value are missing. However, there are reasons to beleieve that apelin is a promising candidate.
There is growing evidence that the APJ/apelin axis, closely related to the ACE2 system, plays an essential role in maintaining pulmonary vascular endothelial cell homeostasis by positively influencing survival, proliferation, and migration (Alastalo et al., 2011). APJ belongs to the G-protein coupled receptors, and its role in the pulmonary circulation has been extensively reviewed (Andersen et al., 2011; Yang et al., 2015; Chatterjee et al., 2020; Liu et al., 2020). The APJ receptor is abundant in the pulmonary vasculature on endothelial and smooth muscle cells. Its endogenous ligand, apelin, is a promising candidate biomarker for PH. However, it has at least 46 isoforms with different biological functions (Mesmin et al., 2011).
The apelin gene encodes the 77-amino acid preproapelin, cleaved at its C-terminus to produce the 55-amino acid prohormone apelin-55. The intracellular processing of apelin-55 results in many bioactive residues circulating in the body. Although they could all be potential biomarkers for PH, there is limited data available. In most of these studies, only one isoform was measured, either apelin-13 or apelin-12, and the cohorts were small and quite heterogeneous. Chandra et al. found that apelin-12 levels were lower in n = 23 PH patients than in controls (Chandra et al., 2011). These data demonstrate that disruption of apelin signaling can exacerbate PH mediated by decreased activation of AMP-activated kinase and eNOS, and they identify this pathway as a potentially important therapeutic target for treatment of this refractory human disease. Others found reduced apelin-36 plasma levels in patients with parenchymal lung diseases and PH patients (Goetze et al., 2006). However, there is no data on the diagnostic value of other circulating apelin isoforms and the studies were not designed to evaluate apelin as a diagnostic biomarker for PH. Particularly apelin-17 appears to be of interest, as this isoform has the highest binding potency to the APJ receptor (Yang et al., 2017a; Read et al., 2020).
We prospectively investigated apelin-17 as a diagnostic biomarker in IPAH patients compared to matched controls and found that the accuracy in PH detection was comparable to NT-proBNP and GDF-15.
2 Methods
2.1 Prospective study
In our prospective study, we enrolled a total of n = 31 IPAH patients from our pulmonary hypertension clinic and n = 31 age- and sex-matched healthy controls between January 2013 and January 2014. IPAH was diagnosed if the patient met the hemodynamic criteria: mean pulmonary arterial pressure (mPAP) ≥ 25 mmHg, pulmonary vascular resistance (PVR)> 3WU, pulmonary arterial wedge pressure (PAWP) ≤ 15 mmHg and relevant diseases of the left heart, lung, liver, kidney, and blood as well as perfusion defects on the lung perfusion scan were excluded. The other inclusion criteria were a written informed consent for the study and for the storage of blood samples in the biobank, age >18 years and no clinical signs of acute cardiac decompensation.
Exclusion criteria were withdrawal of consent or evidence of significant left heart, lung, liver, kidney or blood disease. Peripheral blood was collected from an antecubital vein into 9 ml Vacutainer® tubes with separating gel. After the initial 21 IPAH patients and their age- and sex-matched controls (Supplementary Figure S2 in the Supporting Information), due to slow recruitment, we enrolled the same 10 IPAH patients and 10 controls who had already participated in the pilot study, on a de novo basis. This adaptive design allowed us to include a sufficient number of patients in a reasonable period of time (Leon et al., 2011; Whitehead et al., 2016). All samples were reversibly anonymized, and investigators were blinded to clinical data. The primary endpoint was the accuracy of apelin-17 to discriminate IPAH patients from controls. In addition, we exploratively included all eligible CTEPH patients during the enrollment period and tested the same potential biomarkers. Subjects gave written informed consent, and the study was approved by the local ethics committee (23–408 ex 10/11).
Diagnostic workup of IPAH and CTEPH patients was performed according to the ERS/ESC guidelines and the protocol of the sixth World Symposium for Pulmonary Hypertension proceedings (Simonneau et al., 2019; Humbert et al., 2022). Right heart catheterization was performed on a regular basis when pulmonary hypertension was suspected based on unexplained dyspnoea or the results of transthoracic Doppler echocardiography, ECG, or thoracic imaging. Throughout the acquisition period, the same experienced team performed diagnostic procedures in a standardized manner. Patients were usually admitted 1 day prior to the RHC, where the actual blood tests, ECG, and chest X-ray were performed. Diagnostic workup included high-resolution computed tomography of the chest, lung perfusion scintigraphy, pulmonary function tests and exercise testing. All subjects gave written informed consent for the clinical procedures. For the right heart catheter investigation after local anaesthesia, the jugular vein was approached using the Seldinger technique. A balloon-tipped Swan-Ganz catheter was inserted until the wedge position was achieved. The zero reference line was placed at the midthoracic level (Kovacs et al., 2014).
2.2 Biomarker assessment
Serum samples were stored at room temperature for 30 min, then centrifuged at 1500 G for 10 min and aliquoted to 250 µl. All samples were stored at -80°C in the Biobank of the Medical University of Graz until analysis. For the determination of apelins and GDF-15, commercially available enzyme-linked immunosorbent assays (ELISA) were used (apelin-13: Cusabio Biotech Co., LTD., Cat. No. CSB-E13072h), (apelin-17: Antibodies online Cat. No. ABIN6953820 for the pilot study and USCN Life Science Inc., Cat. No. CED065Hu for the prospective study), (apelin-36: Cusabio Biotech Co., LTD., Cat. No. CSB-E13566h), (GDF-15: R&D, Cat. No. DGD150). According to the manufacturer, no significant cross-reactivity or interference was observed between apelin-17 and analogues. NT-proBNP was determined during routine testing at the hospital’s central laboratory (Roche, Cobas).
2.3 Pilot study
To test the utility of apelins as possible biomarkers for PH, we performed a pilot study with 10 IPAH patients and 10 matched controls, in which we examined serum levels of apelin-12, apelin-13, apelin-17, apelin-36, NT-proBNP, and GDF-15 (Foris et al., 2013). We found that several apelin isoforms were elevated in IPAH, with apelin-17 having the best discriminatory power (Supplementary Figure S1 in the Supporting Information). Apelin-12 was below the limit of detection in most patients and was therefore not further investigated.
2.4 Statistical analysis
The power analysis for apelin-17, providing 80% discriminatory power for detecting differences at an alpha level of 0.05, yielded a sample size of n = 31 IPAH patients and n = 31 healthy controls. Data are presented as means ± standard deviation or median and interquartile range (IQR). Statistical analysis was performed using GraphPad Prism software (Version 5.04, GraphPad Software Inc., La Jolla, California) and SPSS (Version 23, SPSS Inc., Chicago, IL, USA). For the analysis of differences between groups, Kruskal–Wallis test was used followed by Dunn´s multiple comparison test. To analyse the association between parameters, Pearson correlation analysis was used for normally distributed values and Spearman correlation for non-normally distributed values. ROC analysis was performed to determine the diagnostic value of apelin-13, apelin-17, apelin-36, GDF-15 and NT-proBNP. The Area Under the Curve (AUC) and corresponding 95% confidence intervals were calculated. The best cut-off score was calculated according to the Youden index. p < 0.05 was considered statistically significant.
3 Results
Patient characteristics of the N = 31 enrolled IPAH patients, with their age- and sex-matched healthy controls and the 24 CTEPH patients are shown in Table 1.
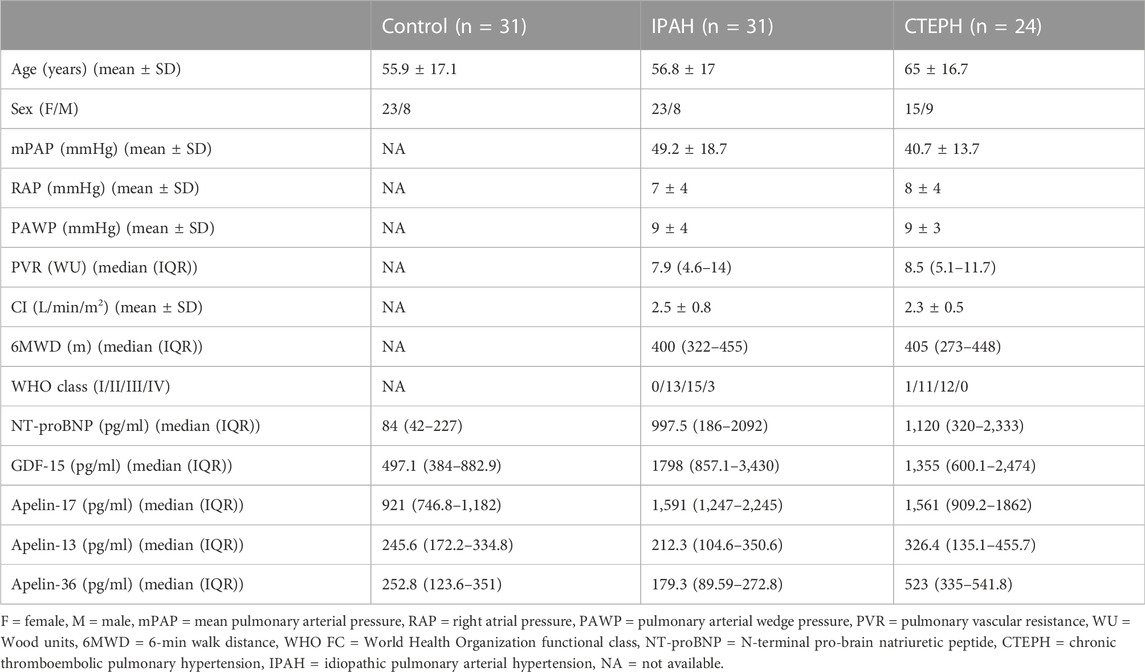
TABLE 1. Patients’ characteristics. Values are expressed as mean ± standard deviation (mean ± SD) or median and interquartile range (median (IQR)).
3.1 Serum levels of biomarkers
Apelin-17 (minimum detectable dose: 48.2 pg/ml) was detectable in all samples, except for one healthy control, and levels were elevated in both IPAH and CTEPH, compared to controls (IPAH vs CTRL vs CTEPH: median = 1591 pg/ml (IQR: 1,247–2,245) vs 921 pg/ml (IQR: 746.8–1,182) vs 1561 pg/ml (IQR: 909.2–1862), p < 0.0001 for IPAH vs control and p < 0.001 for CTEPH vs control, Figure 1A) with no significant differences between IPAH and CTEPH. Apelin-13 was detectable in all but one IPAH patient and showed no differences between the groups (Figure 1B).
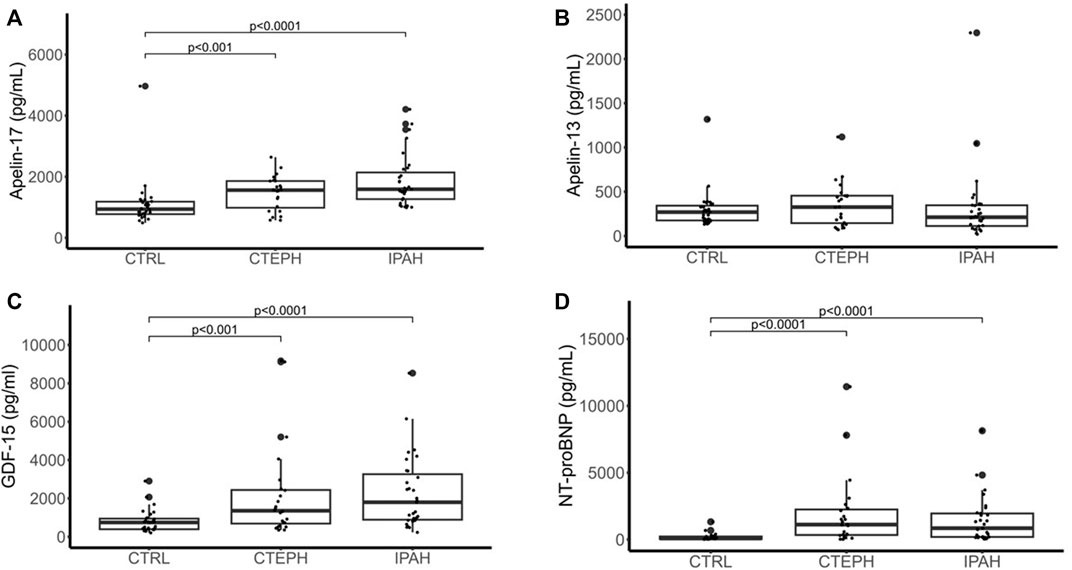
FIGURE 1. Serum levels of apelin-17 (A), apelin-13 (B), GDF-15 (C) and NT-proBNP (D) in n = 31 IPAH patients, n = 31 controls as well as n = 24 CTEPH patients represented as Whisker-Blot plots. Points above or below the plots represent outliers. p < 0.05 was considered statistically significant.
GDF-15 (mean minimum detectable dose: 2 pg/ml) was detectable in all patients and turned out to be increased in both IPAH and CTEPH as compared to control (IPAH vs CTRL vs CTEPH: median = 1798 pg/ml (IQR: 857–3,430) vs 497 pg/ml (IQR: 384–883) vs 1,355 pg/ml (IQR: 600–2,474); IPAH vs CTRL p < 0.0001, CTEPH vs CTRL p < 0.001, Figure 1C), with no significant differences between CTEPH and IPAH.
NT-proBNP (normal range: less than 150 pg/ml) was not available in two IPAH patients, one CTEPH patient and six healthy controls and turned out to be increased in both IPAH and CTEPH as compared to control (IPAH vs CTRL vs CTEPH: median = 997.5 pg/ml (IQR: 186–2092) vs 84 pg/ml (IQR: 42–227) vs 1,120 pg/ml (IQR: 320–2,333); IPAH vs CTRL p < 0.0001, CTEPH vs CTRL p < 0.0001, Figure 1D), with no significant differences between CTEPH and IPAH.
Apelin-36 (minimum detectable dose: 14.2 pg/ml) turned out to be undetectable in a relatively large number of subjects (n = 7 controls, n = 2 IPAH and n = 16 CTEPH). If undetectable values are neglected, there was a significant difference between CTEPH and IPAH (CTEPH vs IPAH: 523 pg/ml (IQR: 335–542) vs 179 pg/ml (IQR: 90–273) p < 0.05), but this result does not allow to draw any conclusions.
3.2 Diagnostic value of biomarkers
To assess the diagnostic accuracy of our markers in predicting IPAH compared with controls, we calculated the area under the curve (AUC) for the receiver operating curve (ROC). Apelin-17 distinguished IPAH from controls with an AUC of 0.86 (95%CI: 0.76–0.95)). At a cut-off value of 1,480 pg/ml, IPAH was detected with a sensitivity and specificity of 68% and 93%, respectively. Apelin-13 and apelin-36 did not predict IPAH. GDF-15 detected IPAH with an AUC of 0.81 (95%CI: 0.7–0.92). At a cut-off value of 855 pg/ml, sensitivity and specificity were 86% and 72%, respectively (Figure 2 A). NT-proBNP detected IPAH with an AUC of 0.84 (95%CI: 0.75–0.95) and at a cut-off value of 140 pg/ml, the sensitivity and specificity were 86% and 72%, respectively (Table 2).
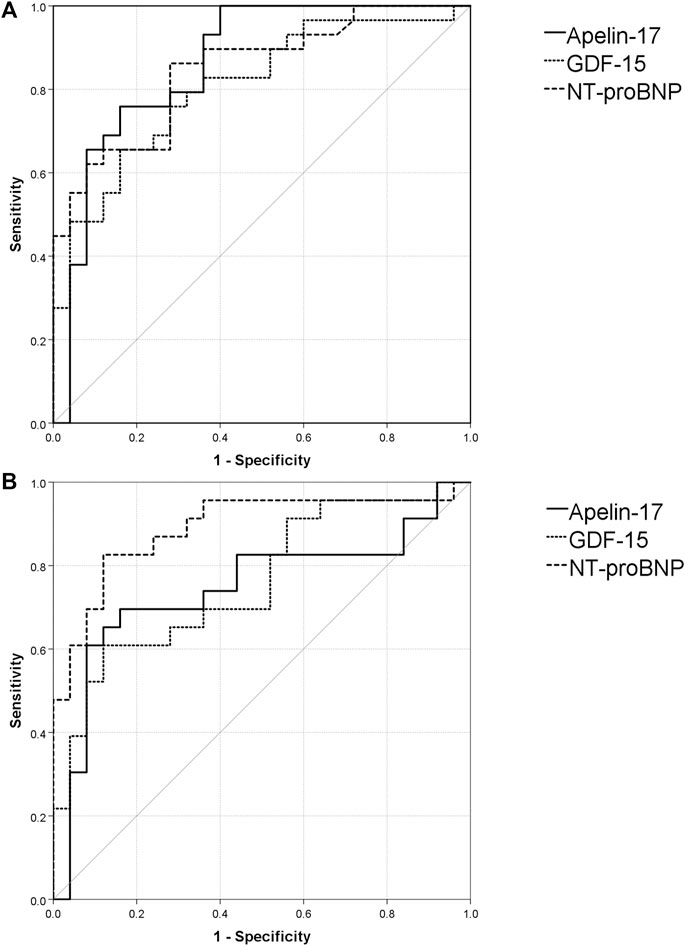
FIGURE 2. Receiver operating curves and area under the curve analysis of the investigated markers. Values of sensitivity (%) are represented on the Y axis. X axis represents 100% - specificity (%). (A). ROC curves for apelin-17, GDF-15 and NT-proBNP for IPAH (B). ROC curves for apelin-17, GDF-15 and NT-proBNP for CTEPH.
In CTEPH, apelin-17 (AUC 0.75, 95%CI: 0.60–0.89; best cut-off: >1,270 pg/ml; sensitivity 71%; specificity 87%) and apelin-36 (AUC: 0.84, 95%CI: 0.88–1.00; best cut-off: >320 pg/ml; sensitivity 86%; specificity: 77%) distinguished CTEPH from healthy controls. GDF-15 distinguished CTEPH from controls with an AUC of 0.73 (95%CI: 0.59–0.87) and at a cut-off of 1,220 pg/ml, sensitivity and specificity were 58% and 84%, respectively (Figure 2B). NT-proBNP distinguished CTEPH from controls with an AUC of 0.89 (95%CI: 0.79–0.99) and at a cut-off of 305 pg/ml, the sensitivity and specificity were 83% and 88%, respectively.
A model was used to combine markers to increase sensitivity or specificity. Three positive criteria, apelin-17 > 1,480 pg/ml, NT-proBNP >140 pg/ml, and GDF-15 > 855 pg/ml, in the same patient, were associated with a specificity of 96% and a sensitivity of 41% for IPAH vs control. When two markers were above the cut-off, the specificity and sensitivity were 80% and 86% for IPAH vs control. When only one marker was above the cut-off, IPAH was detected with a sensitivity of 100% and a specificity of 60%. For CTEPH, the same marker combination (3 of 3) with adjusted thresholds resulted in a specificity of 100% and a sensitivity of 43%. Two positive criteria were associated with a sensitivity of 78% and a specificity of 88%, and one positive criterion (NT-proBNP >305 pg/ml, or apelin-17 > 1,270 pg/ml, or GDF-15 > 1,220 pg/ml) with a sensitivity of 91% and a specificity of 72% (Table 3).

TABLE 3. A combination model for predicting IPAH and CTEPH vs. controls using different cut-offs for IPAH (apelin-17 > 1,480 pg/ml, NT-proBNP >140 pg/ml, GDF-15 > 855 pg/ml) and CTEPH (NT-proBNP >305 pg/ml, apelin-17 > 1,270 pg/ml, GDF-15 > 1,220 pg/ml).
3.3 Correlation analysis
In IPAH, apelin-17 was not significantly correlated with demographic data or hemodynamics. In contrast, GDF-15 was positively correlated with PAWP (ρ = 0.46), NT-proBNP (ρ = 0.56), uric acid (ρ = 0.63) and WHO class (ρ = 0.57). NT-proBNP was positively correlated with PAWP (ρ = 0.48), RAP (ρ = 0.64), WHO FC (ρ = 0.47), bilirubin (ρ = 0.52), uric acid (ρ = 0.63) and GDF-15 (ρ = 0.56). Moreover, it was negatively correlated with CO (ρ = -0.6), CI (ρ = -0.65) and 6MWD (ρ = -0.42) (Supplementary Table S1 in the Supporting Information).
In CTEPH, apelin-17 was positively correlated (p < 0.05) with markers of right heart failure (RAP ρ = 0.49, NT-proBNP ρ = 0.46), uric acid (ρ = 0.46) and bilirubin (ρ = 0.44). GDF-15 was negatively correlated with resting arterial pCO2 (ρ = -0.56) and 6-min walk distance (ρ = -0.54). There were also significant correlations with mPAP (ρ = 0.44), RAP (ρ = 0.44), PVR (ρ = 0.4), and NT-proBNP (ρ = 0.61) (Supplementary Table S2 in the Supporting Information). NT-proBNP was significantly correlated with mPAP (ρ = 0.75), PVR (ρ = 0.72), CI (ρ = -0.44), 6MWD (ρ = - 0.42), pCO2 (ρ = -0.59), bilirubin (ρ = 0.5), uric acid (ρ = 0.44), apelin-17 (ρ = 0.46) and GDF-15 (ρ = 0.61).
4 Discussion
4.1 Circulating apelin isoforms
We prospectively investigated the diagnostic value of apelin-17 for the diagnosis of IPAH compared with age-and sex-matched controls and compared this to NT-proBNP and GDF-15, the most established markers of PAH, to better interpret and classify our results. As a main finding, we found that apelin-17 distinguished IPAH from controls with an AUC comparable to NT-proBNP and GDF-15. In addition, we found that our CTEPH cohort, when compared to the controls, was identified by serum apelin-17 with an accuracy comparable to IPAH. This finding suggests that apelin-17 may not only be a marker for IPAH but for pulmonary hypertension, in general. In patients with three positive markers for PH (apelin-17 and GDF-15 and NT-proBNP), the specificity for PH was very high, but at the expense of sensitivity. In patients with at least one positive marker, the sensitivity for PH was 100%, but at the expense of specificity.
Apelin isoforms have been discussed as potential new biomarkers for PH but have not been investigated in a prospective study after a formal power calculation. A previous study by Zhen et al. demonstrated the presence of at least apelin-13, apelin-36 and apelin-17 in the plasma of healthy controls and pyroglutamil apelin-13 was proved to be the major isoform (Zhen et al., 2013). Apelin-12 was rapidly degraded under physiological conditions, consistent with the observation from our pilot study that apelin-12 was detectable in only 2/10 samples.
In our prospective cohort, in contrast to NT-proBNP and GDF-15, apelin-17 was not significantly correlated with any of the pulmonary hemodynamic markers in IPAH. This suggests that it may be independent of the actual cardiac and vascular stress and might rather represent the remodeling activity. However, this is speculative as in CTEPH, apelin-17 was significantly correlated with hemodynamic parameters. These differences in the apelin-17 associations between IPAH and CTEPH could be due to different underlying pathological disease mechanisms or the small sample size.
We did not aim to assess if apelin-17 may be a prognostic marker as well, however it is tempting to speculate that elevated levels may be present in the whole disease course since PH is a progressive vasculopathy. Therefore, future studies assessing apelin-17 as a prognostic biomarker in PH are warranted.
4.2 Role of apelin in the circulation
The importance of apelin-17 is supported by in vitro evidence that this isoform has the highest affinity for the APJ receptor (Yang et al., 2017b). In addition, there are several studies showing that synthetized apelin-17 analogs have potent effects on the APJ receptor (Iturrioz et al., 2010; Gerbier et al., 2017; McKinnie et al., 2017; Fischer et al., 2020; Flahault et al., 2021). However, only few studies investigating circulating apelin have distinguished between isoforms. Under normoxic conditions, an apelin mixture consisting of many isoforms inhibited the vasoconstriction of pulmonary arteries as shown by wire myography under normoxic but not under hypoxic conditions (Andersen et al., 2009). In another approach, estradiol administration to male SuHx-PH rats decreased RV proapoptotic signaling and RV apelin RNA levels (Frump et al., 2015). Elabela/Toddler is another apelin receptor ligand that showed similar effects to apelin in PH models despite lacking sequence similarity (Yang et al., 2017a). Thus, apelin acts as a vasoactive and likely anti-remodeling mediator in the pulmonary circulation. There are only a few therapeutic approaches, and most of them were made with apelin-13, which improved vascular remodeling in mice exposed to chronic hypoxia (Fan et al., 2020) [pyr1]-Apelin-13 infusion decreased PVR and increased cardiac output in an acute study of 19 PAH patients (Brash et al., 2018). This indicates that the upregulation of apelin in pulmonary hypertension represents a negative feedback loop, comparable to natriuretic peptides.
4.3 Limitations
As a limiting factor, the number of patients in our study was small, but this was consistent with our power calculation and yielded a highly significant result. At the time of recruitment, PH was defined as mPAP ≥25 mmHg hence we did not include patients who had a mPAP >20 mmHg but less than 25 mmHg. Some of the prospectively enrolled patients had already participated in the pilot study. However, these patients were enrolled in the prospective study on a de novo basis and the previous results were blinded to the investigators. Most patients were on targeted PAH therapy and over time, medication changed in several patients. Therefore, it cannot be excluded that medication affected the apelin levels. However, it seems very unlikely that this explains the significant differences between PH patients and controls. We enrolled only patients with severe pulmonary hypertension and healthy controls and found strong contrasts in apelin-17 levels. It is possible that the discriminatory power would be lower in patients with mild PH or other forms of PH. However, the same applies to the established markers NT-proBNP and GDF-15, which did not perform better than apelin-17 as biomarker for PH.
5 Conclusion
Based on this prospective study, apelin-17 is a diagnostic marker for IPAH with a diagnostic performance comparable to the established biomarkers NT-proBNP and GDF-15. The study suggests that this is not specific for IPAH but may be also true for CTEPH.
Data availability statement
The original contributions presented in the study are included in the article/Supplementary Material, further inquiries can be directed to the corresponding author.
Ethics statement
The studies involving human participants were reviewed and approved by the Ethics Committee of the Medical University of Graz. The patients/participants provided their written informed consent to participate in this study.
Author contributions
VF, ZB, AA, and HO provided substantial contributions to the conception an design of the work. VF, PD, GK, BG, WK, and HO provided substantial contributions to the acquisition, analysis, and interpretation of data. ZB and AA performed the statistical analysis. VF, GK, AA, BZ, PD, BG, AO, and HO revised the manuscript critically for important intellectual content.
Funding
The study was founded by the Ludwig Boltzmann Institute for Lung Vascular Research and by the Medical University of Graz.
Acknowledgments
The blood samples used in this study were stored at the Biobank Graz.
Conflict of interest
The authors declare that the research was conducted in the absence of any commercial or financial relationships that could be construed as a potential conflict of interest.
Publisher’s note
All claims expressed in this article are solely those of the authors and do not necessarily represent those of their affiliated organizations, or those of the publisher, the editors and the reviewers. Any product that may be evaluated in this article, or claim that may be made by its manufacturer, is not guaranteed or endorsed by the publisher.
Supplementary material
The Supplementary Material for this article can be found online at: https://www.frontiersin.org/articles/10.3389/fphys.2022.986295/full#supplementary-material
References
Alastalo T., Li M., Perez Vde J., Pham D., Sawada H., Wang J., et al. (2011). Disruption of pparγ/β-catenin-mediated regulation of apelin impairs BMP-induced mouse and human pulmonary arterial EC survival. J. Clin. Investigation 121 (9), 3735–3746. doi:10.1172/JCI43382
Anderson C., Hilberg O., Mellemkjær S., Nielsen-Kudsk J., Simonsen U. (2011). Apelin and pulmonary hypertension. Pulm. Circ. 1 (3), 334–346. doi:10.4103/2045-8932.87299
Anderson C., Markvardsen L., Hilberg O., Simonsen U. (2009). Pulmonary apelin levels and effects in rats with hypoxic pulmonary hypertension. Respir. Med. 103 (11), 1663–1671. doi:10.1016/j.rmed.2009.05.011
Brash L., Barnes G., Brewis M., Church A., Gibbs S., Howard L., et al. (2018). Short-term hemodynamic effects of apelin in patients with pulmonary arterial hypertension. JACC. Basic Transl. Sci. 3 (2), 176–186. doi:10.1016/j.jacbts.2018.01.013
Chandra S., Razavi H., Kim J., Agrawal R., Kundu R., de Jesus Perez V., et al. (2011). Disruption of the apelin-APJ system worsens hypoxia-induced pulmonary hypertension. Arteriosclerosis, Thrombosis, Vasc. Biol. 31 (4), 814–820. doi:10.1161/ATVBAHA.110.219980
Chatterjee P., Gheblawi M., Wang K., Vu J., Kondaiah P., Oudit G. (2020). Interaction between the apelinergic system and ACE2 in the cardiovascular system: Therapeutic implications. Clin. Sci. 134 (17), 2319–2336. London, England. doi:10.1042/CS20200479
Chen G., Yang T., Gu Q., Ni X., Zhao Z., Ye J., et al. (2014). Elevated plasma YKL-40 as a prognostic indicator in patients with idiopathic pulmonary arterial hypertension. Respirol. Carlt. Vic.) 19 (4), 608–615. doi:10.1111/resp.12283
Damico R., Kolb T., Valera L., Wang L., Housten T., Tedford R., et al. (2015). Serum endostatin is a genetically determined predictor of survival in pulmonary arterial hypertension. Am. J. Respir. Crit. Care Med. 191 (2), 208–218. doi:10.1164/rccm.201409-1742OC
Fan J., Fan X., Guang H., Shan X., Tian Q., Zhang F., et al. (2020). Upregulation of MiR-335-3p by NF-?b transcriptional regulation contributes to the induction of pulmonary arterial hypertension via APJ during hypoxia. Int. J. Biol. Sci. 16 (3), 515–528. doi:10.7150/ijbs.34517
Fischer C., Lamer T., Fernandez K., Gheblawi M., Wang W., Pascoe C., et al. (2020). Optimizing PEG-extended apelin analogues as cardioprotective drug leads: Importance of the KFRR motif and aromatic head group for improved physiological activity. J. Med. Chem. 63 (20), 12073–12082. doi:10.1021/acs.jmedchem.0c01395
Flahault A., Girault-Sotias P., Keck M., Alvear-Perez R., De Mota N., Estéoulle L., et al. (2021). A metabolically stable apelin-17 analog decreases AVP-induced antidiuresis and improves hyponatremia. Nat. Commun. 12 (1), 305. doi:10.1038/s41467-020-20560-y
Foris V., Kovacs G., Marsh L., Kwapiszewska G., Tscherner M., Avian A., et al. (2013). Determination of apelin isoforms in patients with pulmonary hypertension. Am. J. Respir. Crit. Care Med. 187, 2559.
Frump A., Goss K., Vayl A., Albrecht M., Fisher A., Tursunova R., et al. (2015). Estradiol improves right ventricular function in rats with severe angioproliferative pulmonary hypertension: Effects of endogenous and exogenous sex hormones. Am. J. Physiology. Lung Cell. Mol. Physiology 308 (9), L873–L890. doi:10.1152/ajplung.00006.2015
Geenen L., Baggen V., Kauling R., Koudstaal T., Boomars K., Boersma E., et al. (2020). Growth differentiation factor-15 as candidate predictor for mortality in adults with pulmonary hypertension. Heart (British Card. Soc. 106 (6), 467–473. doi:10.1136/heartjnl-2019-315111
Gerbier R., Alvear-Perez R., Margathe J., Flahault A., Couvineau P., Gao J., et al. (2017). Development of original metabolically stable apelin-17 analogs with diuretic and cardiovascular effects. FASEB J. Official Publ. Fed. Am. Soc. Exp. Biol. 31 (2), 687–700. doi:10.1096/fj.201600784R
Goetze J. P., Rehfeld J. F., Carlsen J., Videbaek R., Andersen C., Boesgaard S., et al. (2006). Apelin: A new plasma marker of cardiopulmonary disease. Regul. Pept. 133 (1–3), 134–138. doi:10.1016/j.regpep.2005.09.032
Hendriks P., van de Groep L., Veen K., Meertens S., Boersma E., Boomars K., et al. (2022). Prognostic value of brain natriuretic peptides in patients with pulmonary arterial hypertension: A systematic review and meta-analysis. Am. Heart J. 250, 34–44. doi:10.1016/j.ahj.2022.05.006
Heresi G., Tang W., Aytekin M., Hammel J., Hazen S., Dweik R. (2012). Sensitive cardiac troponin I predicts poor outcomes in pulmonary arterial hypertension. Eur. Respir. J. 39 (4), 939–944. doi:10.1183/09031936.00067011
Huai X., Sun Y., Sun X., Wu W., Wang L., Jiang R., et al. (2021). The effect of docosahexaenoic acid on predicting the survival of patients with idiopathic pulmonary arterial hypertension. Ann. Transl. Med. 9 (12), 995. doi:10.21037/atm-21-2479
Humbert M., Kovacs G., Hoeper M. M., Badagliacca R., Berger R., Brida M., et al. (2022). 2022 ESC/ERS Guidelines for the diagnosis and treatment of pulmonary hypertension. Eur. Respir. J., 2200879. Online ahead of print. doi:10.1183/13993003.00879-2022
Iturrioz X., Gerbier R., Leroux V., Alvear-Perez R., Maigret B., Llorens-Cortes C. (2010). By interacting with the C-terminal phe of apelin, Phe255 and Trp259 in helix VI of the apelin receptor are critical for internalization. J. Biol. Chem. 285 (42), 32627–32637. doi:10.1074/jbc.M110.127167
Kovacs G., Avian A., Pienn M., Naeije R., Olschewski H. (2014). Reading pulmonary vascular pressure tracings. How to handle the problems of zero leveling and respiratory swings. Am. J. Respir. Crit. Care Med. 190 (3), 252–257. doi:10.1164/rccm.201402-0269PP
Leon A. C., Davis L. L., Kraemer H. C. (2011). The role and interpretation of pilot studies in clinical research. J. Psychiatric Res. 45 (5), 626–629. doi:10.1016/j.jpsychires.2010.10.008
Leuchte H., El Nounou M., Tuerpe J, Hartmann B., Baumgartner R., Vogeser M., et al. (2007). N-terminal pro-brain natriuretic peptide and renal insufficiency as predictors of mortality in pulmonary hypertension. Chest 131 (2), 402–409. doi:10.1378/chest.06-1758
Liu W., Yan J., Pan W., Tang M. (2020). Apelin/elabela-APJ: A novel therapeutic target in the cardiovascular system. Ann. Transl. Med. 8 (5), 243. doi:10.21037/atm.2020.02.07
McKinnie S., Wang W., Fischer C., McDonald T., Kr K., Iturrioz X., et al. (2017). Synthetic modification within the “RPRL” region of apelin peptides: Impact on cardiovascular activity and stability to neprilysin and plasma degradation. J. Med. Chem. 60 (14), 6408–6427. doi:10.1021/acs.jmedchem.7b00723
Mesmin C., Fenaille F., Becher F., Tabet J-C., Ezan E. (2011). Identification and characterization of apelin peptides in bovine colostrum and milk by liquid chromatography-mass spectrometry. J. Proteome Res. 10 (11), 5222–5231. doi:10.1021/pr200725x
Nickel N., Kempf T., Tapken H., Tongers J., Laenger F., Lehmann U., et al. (2008). Growth differentiation factor-15 in idiopathic pulmonary arterial hypertension. Am. J. Respir. Crit. Care Med. 178 (5), 534–541. doi:10.1164/rccm.200802-235OC
Njaman W., Iesaki T., Iwama Y., Takasaki Y., Daida H. (2007). Serum uric acid as a prognostic predictor in pulmonary arterial hypertension with connective tissue disease. Int. Heart J. 48 (4), 523–532. doi:10.1536/ihj.48.523
Read C., Yang P., Kuc R., Nyimanu D., Williams T., Glen R., et al. (2020). Apelin peptides linked to anti-serum albumin domain Antibodies retain affinity in vitro and are efficacious receptor agonists in vivo. Basic & Clin. Pharmacol. Toxicol. 126, 96–103. doi:10.1111/bcpt.13227
Rhodes C., Wharton J., Howard L., Gibbs J., Wilkins M. (2011). Red cell distribution width outperforms other potential circulating biomarkers in predicting survival in idiopathic pulmonary arterial hypertension. Heart (British Card. Soc. 97 (13), 1054–1060. doi:10.1136/hrt.2011.224857
Shah S. J., Thenappan T., Rich S., Lu T., Archer S. L., Gomberg-Maitland M. (2008). Association of serum creatinine with abnormal hemodynamics and mortality in pulmonary arterial hypertension. Circulation 117 (19), 2475–2483. doi:10.1161/CIRCULATIONAHA.107.719500
Simonneau G., Montani D., Celermajer D., Denton C., Ma G., Krowka M., et al. (2019). Haemodynamic definitions and updated clinical classification of pulmonary hypertension. Eur. Respir. J. 53 (1), 1801913. doi:10.1183/13993003.01913-2018
Simpson C., Damico R., Hummers L., Khair R., Kolb T., Hassoun P., et al. (2019). Serum uric acid as a marker of disease risk, severity, and survival in systemic sclerosis-related pulmonary arterial hypertension. Pulm. Circ. 9 (3), 2045894019859477. doi:10.1177/2045894019859477
Torbicki A., Kurzyna M., Kuca P., Fijałkowska A., Sikora J., Florczyk M., et al. (2003). Detectable serum cardiac troponin T as a marker of poor prognosis among patients with chronic precapillary pulmonary hypertension. Circulation 108 (7), 844–848. doi:10.1161/01.CIR.0000084544.54513.E2
Whitehead A. L., Julious S. A., Cooper C. L., Campbell M. J. (2016). Estimating the sample size for a pilot randomised trial to minimise the overall trial sample size for the external pilot and main trial for a continuous outcome variable. Stat. Methods Med. Res. 25 (3), 1057–1073. doi:10.1177/0962280215588241
Yang P., Kuc R., Brame A., Dyson A., Singer M., Glen R., et al. (2017b). [Pyr 1]Apelin-13 (1-12) is a biologically active ACE2 metabolite of the endogenous cardiovascular peptide [pyr 1]Apelin-13. Front. Neurosci. 11, 92. doi:10.3389/fnins.2017.00092
Yang P., Maguire J., Davenport A. (2015). Apelin, elabela/toddler, and biased agonists as novel therapeutic agents in the cardiovascular system. Trends Pharmacol. Sci. 36 (9), 560–567. doi:10.1016/j.tips.2015.06.002
Yang P., Read C., Kuc R., Buonincontri G., Southwood M., Torella R., et al. (2017a). Elabela/toddler is an endogenous agonist of the apelin APJ receptor in the adult cardiovascular system, and exogenous administration of the peptide compensates for the downregulation of its expression in pulmonary arterial hypertension. Circulation 135 (12), 1160–1173. doi:10.1161/CIRCULATIONAHA.116.023218
Keywords: apelin-17, NT-proBNP, GDF-15, blood derived biomarker, diagnosis, idiopathic pulmonary arterial hypertension
Citation: Foris V, Kovacs G, Avian A, Bálint Z, Douschan P, Ghanim B, Klepetko W, Olschewski A and Olschewski H (2023) Apelin-17 to diagnose idiopathic pulmonary arterial hypertension: A biomarker study. Front. Physiol. 13:986295. doi: 10.3389/fphys.2022.986295
Received: 04 July 2022; Accepted: 15 December 2022;
Published: 04 January 2023.
Edited by:
Tzong-Shyuan Lee, National Taiwan University, TaiwanReviewed by:
Silvia Ulrich, University Hospital Zürich, SwitzerlandElena Goncharova, University of California, Davis, United States
Copyright © 2023 Foris, Kovacs, Avian, Bálint, Douschan, Ghanim, Klepetko, Olschewski and Olschewski. This is an open-access article distributed under the terms of the Creative Commons Attribution License (CC BY). The use, distribution or reproduction in other forums is permitted, provided the original author(s) and the copyright owner(s) are credited and that the original publication in this journal is cited, in accordance with accepted academic practice. No use, distribution or reproduction is permitted which does not comply with these terms.
*Correspondence: Vasile Foris, dmFzaWxlLmZvcmlzQG1lZHVuaWdyYXouYXQ=