- 1State Key Laboratory of Rice Biology and Breeding, Key Laboratory of Molecular Biology of Crop Pathogens and Insects, Ministry of Agriculture, Institute of Insect Sciences, Zhejiang University, Hangzhou, China
- 2Zhejiang Academy of Forestry, Hangzhou, China
- 3Hainan Research Institute, Zhejiang University, Sanya, China
Ceramidases (CDases) are vital sphingolipid enzymes involved in organismal growth and development. They have been reported as key mediators of thermal stress response. However, whether and how CDase responds to heat stress in insects remain unclear. Herein, we identified two CDase genes, C. lividipennis alkaline ceramidase (ClAC) and neutral ceramidase (ClNC), by searching the transcriptome and genome databases of the mirid bug, Cyrtorhinus lividipennis, an important natural predator of planthoppers. Quantitative PCR (qPCR) analysis showed that both ClNC and ClAC were highly expressed in nymphs than in adults. ClAC was especially highly expressed in the head, thorax, and legs, while ClNC was widely expressed in the tested organs. Only the ClAC transcription was significantly affected by heat stress. Knocking down ClAC increased the C. lividipennis nymph survival rate under heat stress. The transcriptome and lipidomics data showed that the RNA interference-mediated suppression of ClAC significantly upregulated the transcription level of catalase (CAT) and the content of long-chain base ceramides, including C16-, C18-, C24-, and C31- ceramides. In C. lividipennis nymphs, ClAC played an important role in heat stress response, and the upregulation of nymph survival rate might be caused by variation in the ceramide levels and transcriptional changes in CDase downstream genes. This study improves our understanding of the physiological functions of insect CDase under heat stress and provides valuable insights into the nature enemy application.
1 Introduction
Rice (Oryza sativa) is the most important food crop in the world with over half of the global population depending on this food resource (Project, 2005; Lou et al., 2014). The brown planthopper (BPH), Nilaparvata lugens, not only directly feeds on the rice plant but also transmits plant viruses (Liu et al., 2018). Since the Green Revolution, pesticide abuse increased the insect drug resistance and caused BPH outbreaks, which have seriously threatened rice production and affected human life (Bottrell and Schoenly, 2012). Thus, the biological control of BPH has increasing importance and is considered the sustainable strategy to avoid the shortcomings of chemical control (Lou et al., 2014). Cyrtorhinus lividipennis (Reuter), a hemipteran predator, is a dominant natural enemy of BPH. It feeds on BPH eggs and nymphs, thus effectively controlling the BPH population and playing an important role in BPH biological control (Matsumura et al., 2005; Sigsgaard, 2007; Preetha et al., 2010; Liu et al., 2018). However, with the acceleration of global warming, thermal stress is weakening the fitness and predatory capacity of C. lividipennis (Bai et al., 2022). Therefore, it is urgently required to investigate how C. lividipennis reacts to heat stress such as identifying the key resistance effectors to increase its survival rate under heat stress and improve its predation performance.
Sphingolipids play structural roles in cellular membranes and act as bioactive signaling molecules involved in multiple cell regulatory functions (Bartke and Hannun, 2009). Ceramides, located in the central part of sphingolipid metabolism, are the precursors of multiple complex sphingolipids (Kitatani et al., 2008; Gault et al., 2010; Young et al., 2013). Many research studies have demonstrated that sphingolipids participate in the regulation of thermal stress response. CDases are the most important metabolic enzymes of ceramides, hydrolyzing ceramides into free fatty acids and sphingosine (Mao and Obeid, 2008). CDases are divided into three subfamilies, including acid CDase (aCDase), neutral CDase (nCDase), and alkaline CDase (alCDase). nCDase have proved to be involved in the heat stress response of BPH (Shi et al., 2018). Serine palmitoyltransferase (SPT), localized in the first step of sphingolipid biosynthesis, is required for the accumulation of trehalose, a thermoprotectant in yeast cells under heat stress (Dickson et al., 1997; Jenkins et al., 1997; Dickson et al., 2006). Sphingosine-1-phosphate (S1P), a product of sphingosine (Sph) phosphorylation, enhanced the survival rate of Arabidopsis cell under heat stress by reducing programmed cell death (Alden et al., 2011). External addition of S1P could reduce the deleterious effect of heat stress on the development of bovine oocytes (Roth and Hansen, 2004). In mouse cells, ceramide and S1P activated the synthesis of heat shock proteins (HSPs) during heat shock response (Chang et al., 1995; Kozawa et al., 1999). The aforementioned data indicate the intimate relationship between sphingolipid metabolism and thermal responses. However, the involvement of sphingolipids in heat resistance in the predator C. lividipennis has not been extensively studied.
In this research, we first identified two CDase homologous genes from the C. lividipennis genomic and transcriptomic databases. Then, the relative expression and phylogenetic analysis of CDases were conducted to understand the characteristics of these enzymes. Using the technology of RNA interference (RNAi), we clarified the biological roles of ClAC in thermal tolerance. Finally, transcriptome and lipid metabolome analyses were performed to reveal the potential regulatory mechanism.
2 Materials and methods
2.1 Insect rearing
C. lividipennis and their prey, BPH, were collected from a paddy field on the Zijingang campus of Zhejiang University, Hangzhou, China. The BPH population was maintained on susceptible rice seedlings of cv. Taichung Native 1 (TN1). C. lividipennis was reared in cages with fresh rice seedlings and sufficient prey. The environmental chamber parameters were set as 26 °C ± 1 °C, 70% ± 10% relative humidity, and a 14:10 h light: dark photoperiod, as described by Bai et al. (2022).
2.2 Sequence analysis
The homologous genes of C. lividipennis CDase were identified in the transcriptome data and genome data through local blast using CDases of Homo sapiens, BPH, and Mus musculus as queries. The phylogenetic analysis of ClAC and ClNC proteins was performed using MEGA X software (http://www.megasoftware.net/). A phylogenetic tree was constructed using the neighbor-joining and Poisson correction methods based on CDase protein sequences and setting the bootstrap value for 1,000 trials.
2.3 Sample collection
The sample collected for the stage-specific expression pattern analysis were eggs (n = 100), first instar nymphs (n = 50), second instar nymphs (n = 50), third instar nymphs (n = 15), fourth instar nymphs (n = 10), fifth instar nymphs (n = 10), and newly emerged females (n = 5) and males (n = 5). Tissues including the head, thorax, leg, integument, midgut, and fat body were dissected from third nymphs (n = 50). The third instar nymphs were collected after exposure under 26 °C and 38 °C for 6 h or 24 h (n = 5) to analyze the differently expressed genes induced by thermal stress. Three biological replicates were collected for each sample, then snap-frozen in liquid nitrogen and stored at −80 °C.
2.4 RNA isolation and quantitative real time polymerase chain reaction (qRT-PCR)
Total RNA was isolated using the TRIzol reagent (Invitrogen, Carlsbad, CA, United States), and cDNA was prepared following the manufacturer's protocol using Evo M-MLV RT Mix Kit with gDNA clean (Accurate Biotechnology). The qRT-PCR reaction was prepared with the SYBR Green premix Pro Taq HS qPCR Kit (Accurate Biotechnology) and performed using the CFX96 Real Time System (Bio-Rad Laboratories, Hercules, CA, United States). Each biological replicate had three technical replicates. The primers (Supplementary Table S1) employed for qRT-PCR were designed by Primer3 (v.0.4.0) (https://bioinfo.ut.ee/primer3-0.4.0/) based on the transcriptomic sequences. The standard curve method was employed to calculate the relative transcript levels. The housekeeping gene GAPDH was used as the internal reference gene.
2.5 RNA interference
ClAC and Green Fluorescent Protein (GFP) gene fragments were amplified using primers containing the T7 promoter (Supplementary Table S1). The cloned PCR products were used as templates to synthesize dsRNAs using the Thermo T7 Transcription Kit (TOYOBO). The DNA or RNA concentrations were measured by a NanoDrop 2000 Spectrophotometer (Thermo Fisher Scientific). The dsRNA products were diluted to 1000 μg/μL and stored at −80 °C for subsequent experiments. The dsRNA of ClAC (dsAC) and dsRNA of GFP (dsGFP) were separately microinjected into the mesothorax of third instar nymphs after anesthetizing with CO2. The treated third nymphs recovered in 30 min after microinjection were used for the following experiments. Three biological replicates (n = 5) were randomly collected for the measurement of RNAi efficiency.
2.6 Survival rate
The dsRNA-injected third instar nymphs were separately reared in 38 °C or 26 °C incubators for 12 h intervals to assess their survival rate. Six replicates were performed for each treatment. For each biological replicate, 12–16 injected third instar nymphs were transferred onto one BPH-oviposited rice plant, which was replaced in each glass tube every day to provide enough food resources for C. lividipennis.
2.7 Transcriptome sequencing
The dsAC-injected or dsGFP-injected third nymphs were reared at 38 °C for 24 h, and then sampled for the RNA extraction. Total RNA was extracted from 10 treated individuals for each sample. Three biological replicates were performed for both dsAC and dsGFP treatments. Illumina sequencing and cDNA library construction were carried out at Novogene (Beijing, China). The differentially expressed genes with padj<0.05 and |log2FoldChange|>0 were selected for the Gene Ontology (GO) and Kyoto Encyclopedia of Genes and Genomes (KEGG) analyses.
2.8 Lipidomic profiling
For each sample, 50 mg of dsAC-injected or dsGFP-injected third instar nymphs in total fresh weight were collected after rearing under 38 °C for 24 h. Six biological replicates were performed for both dsAC and dsGFP treatments. The lipid extraction and lipidomic profiling protocols were conducted at Metware Biotechnology laboratories (Wuhan, China). Significantly regulated metabolites between groups were determined by variable importance in projection (VIP) ≥ 1 and |log2 Fold Change| (|Log2FC|) ≥ 1.0.
2.9 Statistical analysis
The statistical values were shown as means ± standard error of the mean (SEM). One-way ANOVA and LSD test (p<0.05) were performed by Data Processing System (DPS) (Tang and Zhang, 2013). Student's t-test was carried out in GraphPad Prism software (Swift, 1997).
3 Results
3.1 Expression patterns and phylogenetic analysis of C. lividipennis CDases
To understand the CDases of C. lividipennis, we totally found two CDases from C. lividipennis (ClAC and ClNC). We analyzed their evolutionary relationships with CDases from humans, mice, plants, fishes, and other insects. The phylogenetic tree showed that ClAC was clustered into the aCDase group and closely related to the aCDases of the hemipteran insects Cimex lectularius and L. striatellus. Meanwhile, ClNC was clustered into the branch of nCDases and closely related to the hemipteran insect C. lectularius (Figure 1A). To further understand the biological function of these two CDases, the relative transcription levels of ClAC and ClNC across different developmental stages or tissues were investigated. The results showed that ClAC was expressed the highest in first and second nymphs, followed by eggs, third nymphs, fourth nymphs, females, and was the lowest in fifth nymphs and males. ClNC was expressed at a higher level in first to fourth nymphs than in eggs and fifth nymphs, and exhibited the lowest expression in adults (Figure 1B, C). These results indicated that ClAC and ClNC were more highly expressed in C. lividipennis early nymphs than adults. However, ClAC was higher expressed in the head, thorax, and legs than that the integument, midgut, and fat body, while ClNC showed no significant transcriptional differences between all studied tissues (Figure 1D, E). In conclusion, C. lividipennis had two CDases: one aCDase (ClAC) and one nCDase (ClNC), and both were conserved among hemipteran insect species. The transcription data on ClAC and ClNC suggested that ClAC and ClNC played different roles throughout the development of C. lividipennis.
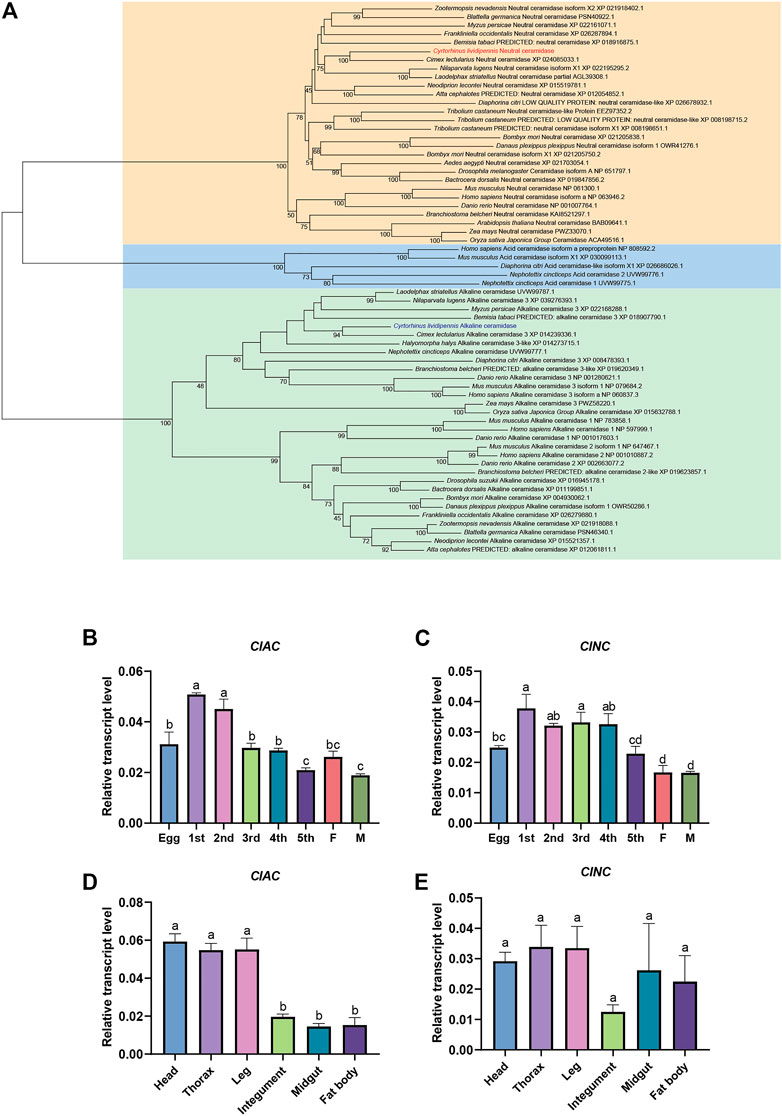
FIGURE 1. Expression patterns and phylogenetic analysis of C. lividipennis ceramidase. (A) Phylogenetic analysis of ClAC and ClNC with the homologous proteins. The neighbor-joining and Poisson correction methods were used to construct the phylogenetic tree based on ceramidase protein sequences. The bootstrap replication value was set to 1000. Relative transcript levels of ClAC (B) and ClNC (C) in eggs, nymphs, and adults. 1st, first instar nymph; 2nd, second instar nymph; 3rd, third instar nymph; 4th, fourth instar nymph; 5th, fifth instar nymph; F, female; M, male. Relative transcript levels ClAC (D) and ClNC (E) in different tissues. The error bar indicates the mean ± SEM of three independent biological replicates. Different letters indicate significant differences (p < 0.05).
3.2 The transcription level of ClAC was significantly increased under heat stress
In order to reveal the relationship between sphingolipid metabolism and insect stress responses, we monitored the transcript levels of ClAC and ClNC after thermal treatment. The transcription of ClAC was first upregulated by two times after stressful heat treatment (38 °C) for 6 h, while it decreased to the normal level in the next 24 h (Figure 2A). Compared with the control group reared at the regular temperature (26 °C), the transcription of ClNC showed no significant differences under heat stress (38 °C) during all 24-hours treatments (Figure 2B). Meanwhile, the transcription levels of other sphingolipid genes, including serine palmitoyltransferase (ClSPT), 3-keto dihydrosphingosine reductase (ClKDSR), sphingolipid delta -desaturase (ClDES), sphingosine kinase (ClSK), sphingosine-1-phosphate phosphatase (ClS1PP), sphingomyelin synthase (ClSMS), sphingomyelinase (ClSMase), and ceramide glucosyltransferase (ClCGT), were also monitored after heat treatment. The results showed that except for ClAC, ClKDSR, and ClS1PP, the sphingolipid genes had no significant transcriptional variation after the high-temperature treatment (Supplementary Figure S1). ClKDSR and ClS1PP were upregulated 1.9 times and 1.7 times, respectively, after stressful heat treatment (38 °C) for 6 h. These data indicated that ClAC, compared to ClNC or other sphingolipid genes, played more critical roles against heat stress in C. lividipennis.
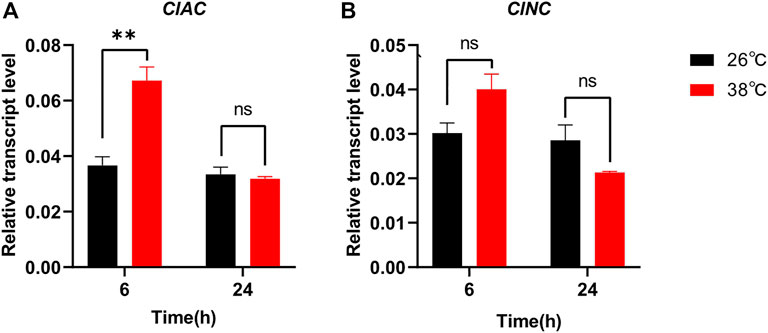
FIGURE 2. Relative transcript levels of ClAC (A) and ClNC (B) after thermal treatment. The gene transcription of C. lividipennis treated at 38 °C was shown by red bars and that at 26 °C by black bars. The error bar indicates the mean ± SEM of three independent biological replicates. Significant differences are indicated by “*” at p<0.05 and “**” at p < 0.01.
3.3 Knocking down ClAC increased the survival rate of C. lividipennis under heat stress
In order to investigate the roles of ClAC in heat stress response, we knocked down the expression of ClAC through RNAi. The relative transcript levels of ClAC were measured at 1 day or 2 days after the injection of dsRNA of ClAC (dsAC). Below 26 °C or 38 °C incubation, the injection of dsAC significantly inhibited the transcription of ClAC, and this transcriptional decline could last for two days (Figure 3A, C). Compared with the control group (the dsGFP-injected nymphs), nymphs with lower ClAC expression (dsAC-injected nymphs) showed no significant difference in the survival rate at 26 °C (Figure 3B). When the rearing temperature was 38 °C, the dsAC-injected C. lividipennis had higher survival rate than the dsGFP-injected group (Figure 3D). This indicated that lower ClAC expression increased the survival rate of C. lividipennis nymphs under heat stress. However, the mechanism through which ClAC mediates the heat stress responses in C. lividipennis is still unknown.
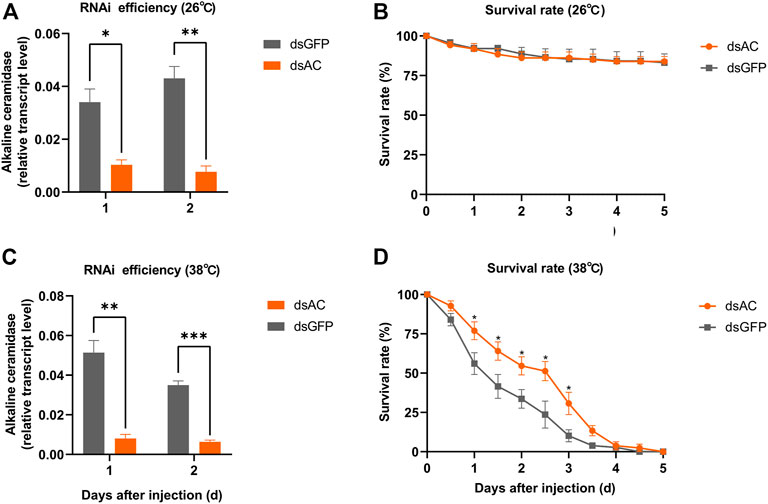
FIGURE 3. Roles of ClAC of C. lividipennis under heat stress. The relative transcription levels of ClAC at 26 °C (A) and 38 °C (C) after dsRNA injection. The error bar indicates the mean ± SEM of three independent biological replicates. The survival rate of third nymphs injected with dsAC (orange) and dsGFP (gray) at 12 h intervals below 26 °C (B) and 38 °C (D). dsAC, dsRNA of ClAC; dsGFP, dsRNA of GFP. The error bar indicates the mean ± SEM of six independent biological replicates (n = 12–16 insects). Significant differences are indicated by “*” at p < 0.05, “**” at p < 0.01, and “***” at p < 0.001.
3.4 Transcriptome analysis
In order to further understand the mediating role of ClAC in heat responses, the transcriptome of third nymphs at the first day after dsRNA injection was analyzed. A total of 1214 differentially expressed genes (DEGs) were detected between the two groups. Compared with dsGFP-injected nymphs, the transcription of 286 genes was upregulated, while that of 928 genes were downregulated in dsAC-injected third nymphs (Figure 4A). KEGG enrichment revealed that the DEGs were mainly involved in peroxisome (nine DEGs), cutin, suberine, and wax biosynthesis (seven DEGs), and longevity regulating pathways (nine DEGs) (Figure 4B). In the longevity regulating pathway, the transcription of catalase (CAT), alcohol-forming fatty acyl-CoA reductase (FARD-1), and stearoyl-CoA desaturase (FAT-6) were significantly varied (Supplementary Figure S2). GO enrichment analysis showed that DEGs were mostly clustered in the structural molecule activity, oxidoreductase activity, and extracellular region (Figure 4C). To reveal the relationship between ClAC and oxidoreductase, we quantified the transcript levels of oxidoreductase-related genes including two CAT (ClCAT1 and ClCAT2), two glutathione peroxidases (ClGPX1 and ClGPX2), five peroxidases (ClPOD1 to ClPOD5), and five superoxide dismutases (ClSOD1 to ClSOD5). The transcription of ClCAT1 instead of other genes was significantly increased by 2.2 times in the dsAC-injected third nymphs (Supplementary Figure S3). Moreover, we quantified the relative transcription levels of other sphingolipid genes after knocking down ClAC. The qPCR results showed that the transcript level of ClKDSR and acid sphingomyelinase 2 (ClaSMase2) were upregulated by 1.9 times and 1.3 times, respectively, while other sphingolipid genes had no significant transcription difference between the dsAC-injected nymphs (Supplementary Figure S4).
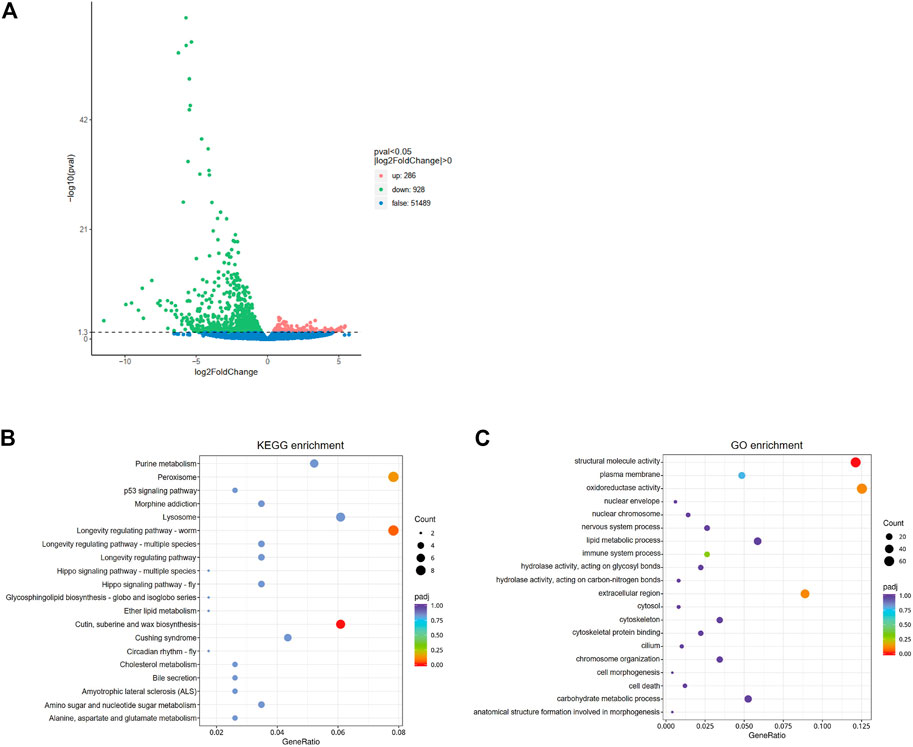
FIGURE 4. Analysis of comparative transcriptome data. (A) Volcano plot of differentially expressed genes (DEGs) between dsAC and dsGFP treatments. The red, green, and blue dots represent significantly upregulated genes, significantly downregulated genes, and non-significantly different genes, respectively. DEGs are filtered based on the criteria of padj<0.05 and |log2FoldChange|>0. The most enriched KEGG pathway (B) and GO terms (C) of DEGs. The sizes of the dots represent the number of DEGs, and the colors represent the enrichment level of DEGs.
3.5 Lipid profiling analysis
Lipid profiling was conducted after the transcriptional inhibition of ClAC to investigate the metabolic functions of ClAC and reveal the network between sphingolipids and other metabolites. A total of 32 differential lipid metabolites (DLMs) were detected, of which 19 DLMs were significantly increased and 13 DLMs were significantly decreased (Supplementary Figure S5A). The KEGG enrichment of DLMs showed that 13 of the 32 DLMs were enriched in the sphingolipid pathway (41%) (Supplementary Figure S5B). These sphingolipids included 10 ceramides (Cer), 1 ceramide-1-phosphate (CerP), and 2 glucosylceramides (GlucoCer). Cer(t18:0/24:0(2OH)) and Cer(t18:0/24:0) were upregulated by 3.9 and 2.1 times, respectively. Ceramides, including Cer(d18:1/18:1), Cer(d18:1/18:0), Cer(d18:1/16:0), Cer(d18:1/24:1), and Cer(d18:1/18:2) were increased by 3.8, 3.8, 5.2, 7.5, and 2.3 times, respectively. The ultra-long chain ceramide Cer(d28:2/31:1(2OH)) was significantly increased by 2.2 times. A trace amount of dihydroceramide Cer(d18:0/16:0(2OH)) (0.23 nmol/g) was detected in dsAC-injected nymphs after high-temperature treatment, while none were observed in the dsGFP-injected nymphs. The levels of Cer(d18:2/16:0) and CerP(d18:1/18:1) were significantly decreased by 41% and 48%, respectively. Meanwhile, GlucoCer(d18:1/22:1) and GlucoCer(d18:1/24:1) were significantly increased by 5.2 and 4.6 times, respectively (Figure 5). In conclusion, most ceramides were increased after ClAC inhibition, and ClAC mainly mediated the levels of C18 sphingo-based ceramides and their glycosylation derivatives (GlucoCers) in the response to heat stress.
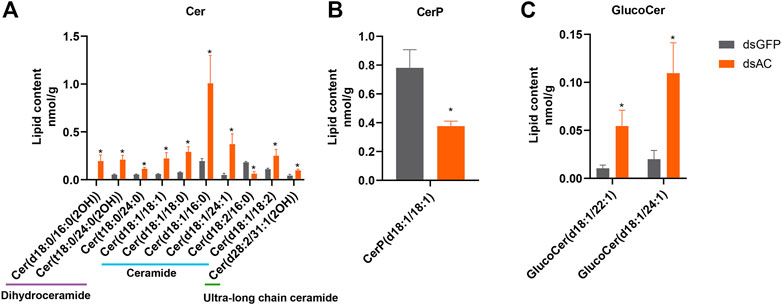
FIGURE 5. Sphingolipid levels after dsAC- and dsGFP-injection. The levels of ceramides (A), ceramide-1-phosphate (B), and glucoceramide (C) in C. lividipennis nymphs after dsAC- and dsGFP-injection. dsAC-injected group, orange column; dsGFP-injected group, gray column. Significant differences are shown by “*” at VIP (VIP ≥ 1) and absolute log2FC (|log2FC| ≥ 1.0).
4 Discussion
CDases are classified according to their optimal pH for enzymatic activity (Mao et al., 2001). The types of CDase vary among different organisms. Therefore, it is necessary to investigate the CDase categories for their functional analysis. Five CDase homologs genes have been identified from humans, including one aCDase, one nCDase, and three alCDases (Coant et al., 2017). Two CDases were found in the hemipteran insect Nephotettix cincticeps (Zhang et al., 2021). Similar to C. lividipennis, the nCDase and alCDase of Drosophila (CDase and Dacer) were reported, whereas no aCDase homologs have been identified (Yoshimura et al., 2002; Acharya and Acharya, 2005; Yuan et al., 2011). The nCDase and alCDase were also reported in the hemipteran insect Laodelphax striatellus (Zhou et al., 2013; Zhang et al., 2021). The phylogenetic analysis demonstrated that CDases are highly conserved between insects, while the biological function of different type CDases vary among different insect species. The alCDase of Drosophila (Dacer) was highly expressed in the pupal stage and significantly affected the longevity of Drosophila (Yang et al., 2010; Zhang et al., 2019). The nCDase of Tribolium castaneum showed high expression in adults, but its biological functions had not been demonstrated (Zhou et al., 2011).The nCDase of BPH was highly expressed in female adults and played essential roles in the reproduction of the insect (Shi et al., 2018; Shi et al., 2021). Different from BPH or Tribolium castaneum, both ClNC and ClAC had high expression in the early nymph stages. The relative transcript levels of genes involved in the de novo biosynthetic pathway and sphingomyelinase pathway were tested to observe ceramide metabolism across C. lividipennis different developmental stages (Supplementary Figure S6). In the de novo biosynthetic pathway, ClSPT2, ClKDSR, and ClCS were highly expressed in eggs, followed by nymphs and females, and exhibited the lowest levels in males. ClDES was highly expressed in nymphs, while was lowest in eggs and adults. It suggested high levels of ceramides might synthesize from the de novo biosynthetic pathway. In the sphingomyelinase pathway, sphingomyelinases (ClnSMase, ClaSMase1, and ClaSMase2) hydrolyze sphingomyelin to ceramide, while sphingomyelin synthases (ClSMS) synthesize ceramide into sphingomyelin (Gault et al., 2010). The relative transcription data showed that ClnSMase was highly expressed in eggs and nymphs, and lowest in adults. Coordinated with ClAC and ClNC, the expression of ClaSMase1 and ClaSMase2 were highest in the first nymph, decreased from the first to fifth nymph, and lowest in eggs and adults, indicating ClAC or ClNC might hydrolyze the ceramides produced by ClSMases. Moreover, ClSMS was also higher expressed in first nymphs than in other development stages. These results showed that the sphingolipid genes are more highly expressed in early nymph stages than in the adults, indicating the ceramide metabolism was more active in C. lividipennis nymphs. In order to maintain a balance of ceramide metabolism, ClAC and ClNC were supposed to be highly expressed to degrade the excessive ceramides in nymphs. In BPH, the transcript level of nCDase was upregulated under heat stress and knocking down of nCDase increased the female survival rate (Shi et al., 2018). Meanwhile, our results showed that ClAC instead of ClNC responded to the thermal threat in C. lividipennis, suggesting that ClNC played different roles together with ClAC, and the function of ClNC need to be further investigated.
In the present study, we attempted to explain how ClAC mediated the high temperature resistance in C. lividipennis through transcriptome and lipodomics analysis. For the transcription level, the inhibition of ClAC directly mediated the transcription of longevity-related genes and other sphingolipid metabolism-related genes (ClKDSR and ClaSMase2) to control the hyper thermal responses in C. lividipennis. As previously reported, thermal stress induces the generation of reactive oxygen species (ROS), including superoxide anion (O2−), hydroxyl radicals (−OH), and hydrogen peroxide (H2O2), which can cause oxidative damages and lead to cell death in living organisms (Cui et al., 2011). Antioxidants are necessary to reduce the content of ROS and improve the cellular fitness. Enzymatic antioxidants, such as SOD, CAT, POD, and GPX, have been identified as ROS scavengers (Wang et al., 2001). CAT as one of the primary antioxidant enzymes protects organisms from oxidative damage by catalyzing hydrogen peroxide into water and oxygen (Wang et al., 2001). CAT was also reported to play important roles in the thermal tolerance of insects. Suppressing CAT significantly reduced the survival rate of Myzus persicae under heat stress (Li et al., 2021). In the whitefly Bemisia tabaci, CAT help to adapt to high temperature by scavenging excessive ROS (Liang et al., 2022). The transcript level and activities of CAT were enhanced to reduce the production of ROS in mutant Dacer, aiding resistance to paraquat-induced oxidative stress (Yang et al., 2010; Zhang et al., 2019). Therefore, we speculated that the high expression of CAT after ClAC inhibition in C. lividipennis might enhance the antioxidant ability of nymphs against the abundant ROS caused by heat stress. However, sphingolipids as the non-enzymatic antioxidants, especially for the ceramides, have been demonstrated to mediate oxidoreductase and maintain cellular redox homeostasis by regulating antioxidant enzymes (García-Caparrós et al., 2021). C2 ceramide inhibited ROS production and cell death in H2O2-treated rat primary astrocytes by increasing the expression of phase II antioxidant enzymes, including heme oxygenase-1 (HO-1), NAD(P)H:quinine oxidoreductase 1 (NQO1), and superoxide dismutase (SOD), attesting to the therapeutic potential of C2 ceramide for various oxidative stress-associated diseases (Jung et al., 2016). The treatment of ceramide and ascorbic acid increased the activities of POD and SOD, thus significantly reduced the oxidative damages and maintained the storage quality of strawberries (Zhao et al., 2019). Taken together, the change in ceramide levels after ClAC inhibition might mediate the expression of CAT and affect the survival rate of C. lividipennis under heat stress. Moreover, the ClKDSR and ClaSMase2 closely regulated the ceramide levels through ClAC than other sphingolipid gene in C. lividipennis.
For the metabolite level, ceramides are not only the essential structural compositions of plasma membranes (Fabri et al., 2020), but also the signaling molecules mediating cellular growth, apoptosis, or death (Okazaki et al., 1998). Ceramides were demonstrated to play important roles in stress tolerance. The loss of the ceramide synthase gene hyl-2 made Caenorhabditis elegans more sensitive to heat shock and anoxia, which indicated that the specific ceramides synthesized by hyl-2 were required for the stress responses (Menuz et al., 2009). The C16-ceramide synthesized by ceramide synthase 6 had anti-apoptotic roles in tumor cells when encountering endoplasmic reticulum (ER) stress (Senkal et al., 2010). The variation in ceramide species and concentration affected the plasma membrane fluidity. Due to the reduced ceramide levels after ceramide transfer protein (CERT) inhibition, the vesicles were loosely packed, less homogenous, and non-discrete in the plasma membrane of Dcert (Rao et al., 2007). The increased fluidity of plasma membrane made Dcert mutants more susceptible to oxidative damage (Rao et al., 2007). In addition, ceramides with different acyl-chains had different impact on the membrane biophysical properties. Sandra et al. found that saturated ceramides (C16-, C18-, and C24-) were preferred to form gel domains over unsaturated ceramides (C18:1- and C24:1-) in phosphatidylcholine model membranes, and the gel domain formed by C16-ceramide was larger and more stable than that formed by C18-ceramide (Pinto et al., 2011). In the present study, the increased ceramide levels proved the hydrolysis function of ClAC as the CDase in C. lividipennis. Saturated Cer (d18:1/16:0) was the most abundant species and unsaturated Cer (d18:1/24:1) was the most significantly increased species after ClAC inhibition, indicating that Cer (d18:1/16:0) and Cer (d18:1/24:1) were the dominant ceramide species catalyzed by ClAC. Cer (d18:1/16:0) reached the highest level compared to other ceramides and might be associated with plasma membrane fluidity. Thus, we proposed that this molecule might play an important role in enhancing the cell membrane stability and in turn upregulate the resistance to oxidative stress.
Most entomology studies have profiled CDases in the model insect D. melanogaster and other insect pests such as BPH. However, the CDases profiles have not been studied in natural enemy insects. We found two CDases, ClAC and ClNC, in C. lividipennis and demonstrated that ClAC is essential in heat stress response. Metabolically, ClAC mediated the ceramide levels and directly regulated the oxidoreductase-related genes at the transcription level. Our results suggested that inhibiting ClAC increased the survival rate of C. lividipennis nymphs, which was regulated by the alteration of ceramide levels and ClAC downstream genes. This study validated the ClAC functions and provides valuable information for improving the heat stress tolerance of C. lividipennis and other natural enemies of pest insects. Further study should be conducted to construct a sphingolipid gene network to enhance sphingolipid applications in green and sustainable biological pest control.
Data availability statement
The datasets presented in this study can be found in online repositories. The names of the repository/repositories and accession number(s) can be found at: https://www.ncbi.nlm.nih.gov/bioproject/PRJNA932139.
Author contributions
MC, X-XS, and Z-RZ conceived and proposed the idea and designed the study. MC performed the experiments and data analysis. MC and X-XS contributed to writing and reading the manuscript. NW, CZ, Z-YS, and W-WZ provided guidance and assistants for the experiment. All authors contributed to the article and approved the submitted version.
Funding
This work was financially supported by the National Natural Science Foundation of China (Grant Nos. 31901875 and 31871962).
Acknowledgments
The authors thank YL Bai, YD Zhang, and other laboratory mates for their kind cooperation during the research.
Conflict of interest
The authors declare that the research was conducted in the absence of any commercial or financial relationships that could be construed as a potential conflict of interest.
Publisher’s note
All claims expressed in this article are solely those of the authors and do not necessarily represent those of their affiliated organizations, or those of the publisher, the editors, and the reviewers. Any product that may be evaluated in this article, or claim that may be made by its manufacturer, is not guaranteed or endorsed by the publisher.
Supplementary material
The Supplementary Material for this article can be found online at: https://www.frontiersin.org/articles/10.3389/fphys.2023.1160846/full#supplementary-material
References
Acharya, U, and Acharya, JK (2005). Enzymes of sphingolipid metabolism in Drosophila melanogaster. Cell Mol Life Sci 62 (2), 128–42. doi:10.1007/s00018-004-4254-1
Alden, KP, Dhondt-Cordelier, S, McDonald, KL, Reape, TJ, Ng, CK-Y, McCabe, PF, et al. (2011). Sphingolipid long chain base phosphates can regulate apoptotic-like programmed cell death in plants. Biochemical and Biophysical Research Communications 410 (3), 574–80. doi:10.1016/j.bbrc.2011.06.028
Bai, YL, Quais, MK, Zhou, WW, and Zhu, ZR (2022). Consequences of elevated temperature on the biology, predation, and competitiveness of two mirid predators in the rice ecosystem. Journal of Pest Science 95, 901–16. doi:10.1007/s10340-021-01414-y
Bartke, N, and Hannun, YA (2009). Bioactive sphingolipids: metabolism and function. Journal of Lipid Research 50, S91–S96. doi:10.1194/jlr.R800080-JLR200
Bottrell, DG, and Schoenly, KG (2012). Resurrecting the ghost of green revolutions past: The brown planthopper as a recurring threat to high-yielding rice production in tropical Asia. Journal of Asia-Pacific Entomology 15 (1), 122–40. doi:10.1016/j.aspen.2011.09.004
Chang, Y, Abe, A, and Shayman, JA (1995). Ceramide formation during heat shock: a potential mediator of alpha B-crystallin transcription. The Proceedings of the National Academy of Sciences 92 (26), 12275–9. doi:10.1073/pnas.92.26.12275
Coant, N, Sakamoto, W, Mao, C, and Hannun, YA (2017). Ceramidases, roles in sphingolipid metabolism and in health and disease. Advances in Biological Regulation 63, 122–31. doi:10.1016/j.jbior.2016.10.002
Cui, Y, Du, Y, Lu, M, and Qiang, C (2011). Antioxidant responses of Chilo suppressalis (Lepidoptera: Pyralidae) larvae exposed to thermal stress. Journal of Thermal Biology 36 (5), 292–7. doi:10.1016/j.jtherbio.2011.04.003
Dickson, RC, Nagiec, EE, Skrzypek, M, Tillman, P, Wells, GB, and Lester, RL (1997). Sphingolipids are potential heat stress signals in Saccharomyces. Journal of Biological Chemistry 272 (48), 30196–200. doi:10.1074/jbc.272.48.30196
Dickson, RC, Sumanasekera, C, and Lester, RL (2006). Functions and metabolism of sphingolipids in Saccharomyces cerevisiae. Progress in Lipid Research 45 (6), 447–65. doi:10.1016/j.plipres.2006.03.004
Fabri, J, de Sa, NP, Malavazi, I, and Del Poeta, M (2020). The dynamics and role of sphingolipids in eukaryotic organisms upon thermal adaptation. Progress in Lipid Research 80, 101063. doi:10.1016/j.plipres.2020.101063
García-Caparrós, P, Filippis, LD, Gul, A, Hasanuzzaman, M, Ozturk, M, Altay, V, et al. (2021). Oxidative Stress and Antioxidant Metabolism under Adverse Environmental Conditions : a Review. The Botanical Review 87, 421–66. doi:10.1007/s12229-020-09231-1
Gault, CR, Obeid, LM, and Hannun, YA (2010). “An overview of sphingolipid metabolism: from synthesis to breakdown,” in Sphingolipids as Signaling and Regulatory Molecules Editors C Chalfant, and MD Poeta (New York: Springer New York), 1–23.
Jenkins, GM, Richards, A, Wahl, T, Mao, C, Obeid, L, and Hannun, Y (1997). Involvement of yeast sphingolipids in the heat stress response of Saccharomyces cerevisiae. Journal of Biological Chemistry 272 (51), 32566–72. doi:10.1074/jbc.272.51.32566
Jung, JS, Choi, MJ, Ko, HM, and Kim, HS (2016). Short-chain C2 ceramide induces heme oxygenase-1 expression by upregulating AMPK and MAPK signaling pathways in rat primary astrocytes. Neurochemistry International 94, 39–47. doi:10.1016/j.neuint.2016.02.004
Kitatani, K, Idkowiak-Baldys, J, and Hannun, YA (2008). The sphingolipid salvage pathway in ceramide metabolism and signaling. Cellular Signalling 20 (6), 1010–8. doi:10.1016/j.cellsig.2007.12.006
Kozawa, O, Niwa, M, Matsuno, H, Tokuda, H, Miwa, M, Ito, H, et al. (1999). Sphingosine 1-phosphate induces heat shock protein 27 via p38 mitogen-activated protein kinase activation in osteoblasts. Journal of Bone and Mineral Research 14 (10), 1761–7. doi:10.1359/jbmr.1999.14.10.1761
Li, MY, Wang, Y, Lei, X, Xu, CT, Wang, DD, Liu, S, et al. (2021). Molecular characterization of a catalase gene from the green peach aphid (Myzus persicae). Archives of Insect Biochemistry and Physiology 108 (2), e21835. doi:10.1002/arch.21835
Liang, P, Ning, J, Wang, W, Zhu, P, Gui, L, Xie, W, et al. (2022). Catalase promotes whitefly adaptation to high temperature by eliminating reactive oxygen species. Insect Science 2022, 1–16. doi:10.1111/1744-7917.13157
Liu, SY, Zhao, J, Hamada, C, Cai, WL, Khan, M, Zou, YL, et al. (2018). Identification of attractants from plant essential oils for Cyrtorhinus lividipennis, an important predator of rice planthoppers. Journal of Pest Science 92 (2), 769–80. doi:10.1007/s10340-018-1054-1
Lou, Y-G, Zhang, G-R, Zhang, W-Q, Hu, Y, and Zhang, J (2014). Reprint of: Biological control of rice insect pests in China. Biological Control 68, 103–16. doi:10.1016/j.biocontrol.2013.09.018
Mao, C, and Obeid, LM (2008). Ceramidases: regulators of cellular responses mediated by ceramide, sphingosine, and sphingosine-1-phosphate. Biochimica et Biophysica Acta (BBA) - Molecular and Cell Biology of Lipids 1781 (9), 424–34. doi:10.1016/j.bbalip.2008.06.002
Mao, C, Xu, R, Szulc, ZM, Bielawska, A, Galadari, SH, and Obeid, LM (2001). Cloning and characterization of a novel human alkaline ceramidase. A mammalian enzyme that hydrolyzes phytoceramide. The Journal of Biological Chemistry 276 (28), 26577–88. doi:10.1074/jbc.M102818200
Matsumura, M, Urano, S, and Suzuki, Y (2005). “Evaluating augmentative releases of the mirid bug Cyrtorhinus lividipennis to suppress brown planthopper Nilaparvata lugens in open paddy fields.” In. Rice is Life: Scientific Perspectives for the 21st Century. (Manila: International Rice Research Institute).
Menuz, V, Howell, KS, Gentina, S, Epstein, S, Riezman, I, Fornallaz-Mulhauser, M, et al. (2009). Protection of C. elegans from anoxia by HYL-2 ceramide synthase. Science 324 (5925), 381–4. doi:10.1126/science.1168532
Okazaki, T, Kondo, T, Kitano, T, and Tashima, M (1998). Diversity and Complexity of Ceramide Signalling in Apoptosis. Cellular Signalling 10 (10), 685–92. doi:10.1016/s0898-6568(98)00035-7
Pinto, SN, Silva, LC, Futerman, AH, and Prieto, M (2011). Effect of ceramide structure on membrane biophysical properties: the role of acyl chain length and unsaturation. Biochimica et Biophysica Acta 1808 (11), 2753–60. doi:10.1016/j.bbamem.2011.07.023
Preetha, G, Stanley, J, Suresh, S, and Samiyappan, R (2010). Risk assessment of insecticides used in rice on miridbug, Cyrtorhinus lividipennis Reuter, the important predator of brown planthopper, Nilaparvata lugens (Stal). Chemosphere 80 (5), 498–503. doi:10.1016/j.chemosphere.2010.04.070
Project, IRGS (2005). The map-based sequence of the rice genome. Nature 436 (7052), 793–800. doi:10.1038/nature03895
Rao, RP, Yuan, C, Allegood, JC, Rawat, SS, Edwards, MB, Wang, X, et al. (2007). Ceramide transfer protein function is essential for normal oxidative stress response and lifespan. The Proceedings of the National Academy of Sciences 104 (27), 11364–9. doi:10.1073/pnas.0705049104
Roth, Z, and Hansen, PJ (2004). Sphingosine 1-phosphate protects bovine oocytes from heat shock during maturation. Biological of Reproduction 71 (6), 2072–8. doi:10.1095/biolreprod.104.031989
Senkal, CE, Ponnusamy, S, Bielawski, J, Hannun, YA, and Ogretmen, B (2010). Antiapoptotic roles of ceramide-synthase-6-generated C16-ceramide via selective regulation of the ATF6/CHOP arm of ER-stress-response pathways. The FASEB Journal 24 (1), 296–308. doi:10.1096/fj.09-135087
Shi, XX, Huang, YJ, Begum, MA, Zhu, MF, Li, FQ, Zhang, MJ, et al. (2018). A neutral ceramidase, NlnCDase, is involved in the stress responses of brown planthopper, Nilaparvata lugens (Stal). Scientific Reports 8 (1), 1130. doi:10.1038/s41598-018-19219-y
Shi, XX, Zhu, MF, Wang, N, Huang, YJ, Zhang, MJ, Zhang, C, et al. (2021). Neutral Ceramidase Is Required for the Reproduction of Brown Planthopper, Nilaparvata lugens (Stal). Frontiers in Physiology 12, 629532. doi:10.3389/fphys.2021.629532
Sigsgaard, L (2007). Early season natural control of the brown planthopper, Nilaparvata lugens: the contribution and interaction of two spider species and a predatory bug. Bulletin of Entomological Research 97 (5), 533–44. doi:10.1017/S0007485307005196
Swift, ML (1997). GraphPad prism, data analysis, and scientific graphing. Journal of chemical information and computer sciences 37 (2), 411–2. doi:10.1021/ci960402j
Tang, QY, and Zhang, CX (2013). Data Processing System (DPS) software with experimental design, statistical analysis and data mining developed for use in entomological research. Insect Science 20 (2), 254–60. doi:10.1111/j.1744-7917.2012.01519.x
Wang, Y, Oberley, LW, and Murhammer, DW (2001). Antioxidant defense systems of two lipidopteran insect cell lines. Free Radic Biol Med 30 (11), 1254–62. doi:10.1016/s0891-5849(01)00520-2
Yang, Q, Gong, ZJ, Zhou, Y, Yuan, JQ, Cheng, JA, Tian, L, et al. (2010). Role of Drosophila alkaline ceramidase (Dacer) in Drosophila development and longevity. Cell Mol Life Sci 67 (9), 1477–90. doi:10.1007/s00018-010-0260-7
Yoshimura, Y, Okino, N, Tani, M, and Ito, M (2002). Molecular cloning and characterization of a secretory neutral ceramidase of Drosophila melanogaster. Journal of Biochemical 132 (2), 229–36. doi:10.1093/oxfordjournals.jbchem.a003215
Young, MM, Kester, M, and Wang, HG (2013). Sphingolipids: regulators of crosstalk between apoptosis and autophagy. Journal of Lipid Research 54 (1), 5–19. doi:10.1194/jlr.R031278
Yuan, C, Rao, RP, Jesmin, N, Bamba, T, Nagashima, K, Pascual, A, et al. (2011). CDase is a pan-ceramidase in Drosophila. Molecular Biology of the Cell 22 (1), 33–43. doi:10.1091/mbc.E10-05-0453
Zhang, CH, Zhang, MJ, Shi, XX, Mao, C, and Zhu, ZR (2019). Alkaline Ceramidase Mediates the Oxidative Stress Response in Drosophila melanogaster Through Sphingosine. Journal of Insect Science 19 (3), 13. doi:10.1093/jisesa/iez042
Zhang, MJ, Shi, XX, Bai, YL, Zhou, WW, and Zhu, ZR (2021). Sphingolipid composition and metabolism differ in three auchenorrhynchous pests of rice. Journal of Asia-Pacific Entomology 24 (3), 772–9. doi:10.1016/j.aspen.2021.06.013
Zhao, HN, Liu, SW, Chen, MG, Li, J, Huang, DD, and Zhu, SH (2019). Synergistic effects of ascorbic acid and plant-derived ceramide to enhance storability and boost antioxidant systems of postharvest strawberries. Journal of the Science of Food and Agriculture 99 (14), 6562–71. doi:10.1002/jsfa.9937
Zhou, Y, Lin, XW, Yang, Q, Zhang, YR, Yuan, JQ, Lin, XD, et al. (2011). Molecular cloning and characterization of neutral ceramidase homologue from the red flour beetle, Tribolium castaneum. Biochimie 93 (7), 1124–31. doi:10.1016/j.biochi.2011.03.009
Keywords: ceramidase, Cyrtorhinus lividipennis, heat stress, catalase, ceramide, nature enemy
Citation: Chen M, Shi X-X, Wang N, Zhang C, Shi Z-Y, Zhou W-W and Zhu Z-R (2023) Alkaline ceramidase (ClAC) inhibition enhances heat stress response in Cyrtorhinus lividipennis (Reuter). Front. Physiol. 14:1160846. doi: 10.3389/fphys.2023.1160846
Received: 07 February 2023; Accepted: 24 April 2023;
Published: 09 May 2023.
Edited by:
Bimalendu B. Nath, Savitribai Phule Pune University, IndiaReviewed by:
Ali R. Bandani, University of Tehran, IranKai Liu, Zhongkai University of Agriculture and Engineering, China
Copyright © 2023 Chen, Shi, Wang, Zhang, Shi, Zhou and Zhu. This is an open-access article distributed under the terms of the Creative Commons Attribution License (CC BY). The use, distribution or reproduction in other forums is permitted, provided the original author(s) and the copyright owner(s) are credited and that the original publication in this journal is cited, in accordance with accepted academic practice. No use, distribution or reproduction is permitted which does not comply with these terms.
*Correspondence: Zeng-Rong Zhu, enJ6aHVAemp1LmVkdS5jbg==; Xiao-Xiao Shi, c2hpeGlhbzY2NTZAMTYzLmNvbQ==