- 1Canine Performance Sciences, Auburn University College of Veterinary Medicine, Auburn, AL, United States
- 2Department of Anatomy, Physiology, Pharmacology, Auburn University College of Veterinary Medicine, Auburn, AL, United States
- 3School of Forensic Sciences, Oklahoma State University—Center for Health Sciences, Stillwater, OK, United States
- 4Federal Bureau of Investigation Laboratory Division, Washington D.C., DC, United States
Effective explosives detection requires dogs to generalize their response to untrained variations of targets that are related to those with which they were trained. Previous research suggests that dogs tend to be highly specific to their trained odors, and are sensitive to alterations in odor profiles. Triacetone triperoxide (TATP) is an increasingly popular homemade explosive due to the widespread accessibility of starting materials. The large variety of reagent sources and production approaches yields high variability in deployed formulations. Whether dogs trained with pure forms of TATP generalize to other variations is unknown, representing a potentially significant security gap. In the current study, we tested dogs (n = 11) previously trained to detect pure TATP with four variants: diacetone diperoxide (DADP), a homologue often created as a TATP byproduct, and three different clandestine TATP formulations designed to emulate those used by terrorists or insurgents. On average, dogs detected each untrained variant at rates equivalent to the trained TATP (ps > 0.07), with individual variability in first-trial alerts for some of the variants. Chemical analyses paralleled the canine results, showing distinct similarities and differences. For the TATP samples, the laboratory-grade was the purest sample tested and did not contain DADP or the TATP homologue that the three clandestine versions showed in their respective headspace profiles. The headspace results showed that each sample could be clearly identified as TATP, yet they showed recognizable differences due to their individual syntheses. These findings suggest that training on pure TATP may be effective for generalization to untrained variants. Further research is necessary to identify factors that influence individual variation in generalization between dogs, as well as other explosives.
1 Introduction
Dogs trained for the detection of explosives and other threats continue to represent the most reliable and effective detection method currently available (Furton and Myers, 2001; Harper et al., 2005). Because the odor profile of targets encountered operationally are likely to vary in some way from that of targets used in training, a critical aspect of optimal detection is generalization to novel (i.e., untrained) variants of target odors (Moser et al., 2019). This ability is especially critical for the detection of devices utilizing homemade explosives (HMEs), which can vary widely in chemical composition due to regional availability and access to starting materials (Harper et al., 2005; Kopp, 2008; Steinkamp et al., 2016). A number of studies have indicated that dogs tend to be highly specific and sensitive to changes in target odor composition, showing low levels of generalization to untrained variations of a target (Oxley and Waggoner, 2009; Cerna et al., 2011; Lazarowski and Dorman, 2014; Lazarowski et al., 2015; Hall and Wynne, 2018).
Triacetone triperoxide (TATP) is a popular HME among terrorists due to its ease of manufacture using readily available starting materials, and has been implicated in several high-profile attacks in recent decades (Kopp, 2008; Espinosa-Fuentes et al., 2011). Bombing intelligence reports indicate that terrorists employ various formulations of TATP, which vary based on material procurement resources and specific recipes found on the internet. Depending on the formulation and materials used, the final product will include TATP, as well as other chemicals, resulting in a target odor signature that contains TATP and additional chemically produced volatile organic compounds (VOCs) left over in the product. Diacetone diperoxide (DADP), a TATP homologue, is sometimes inadvertently made as a byproduct during TATP production (Espinosa-Fuentes et al., 2011) (Figure 1). Therefore, there is potentially wide variability in the TATP odor profile that detection dogs may encounter in operations, which may differ significantly from the pure laboratory-grade TATP profile used in training due to safety reasons. It is unknown if different TATP formulations produce VOC signature profiles that alter the perception of a TATP target and ultimately the response of the canine, which may represent a significant security gap. Thus, the goal of the current study was to examine whether dogs trained to detect pure laboratory-grade TATP generalize across TATP and DADP formulations containing impurities in order to identify potential security gaps to be addressed in training.
2 Materials and Methods
2.1 Target Odors
There were two baseline TATP targets used in the study with which dogs had prior experience. The first was a 1 g sample of solid, laboratory-grade TATP synthesized by an FBI Explosives Chemist. Throughout the study, the TATP was contained in an anti-static vial with a 13-hole lid. This configuration is used by the FBI’s National Canine Improvised Explosives Detection Program for safety. The second baseline target was vaporous TATP delivered via a polymer odor-capture-and-release (POCR) training aid, an absorption-based training aid created for safe and effective TATP odor delivery through desorption. The POCR was created using proprietary methods similar to those described by MacCrehan et al. (2018) in which the polymer, in this case polydimethylsiloxane (PDMS), is placed in a 2 oz tin (PaperMart®) and exposed to TATP vapor for a set period of time and under specified conditions.
Four probe odors (i.e., TATP variants with which dogs had no prior experience) were tested in the current study, each presented in 1 g amounts inside an anti-static vial with a 13-hole lid (consistent with the laboratory-grade TATP sample): laboratory-grade DADP and three clandestine-grade formulations of TATP, all synthesized by an FBI Explosives Chemist. For the clandestine-grade formulations of TATP, all chemicals were purchased from Amazon.com or Giant Food of Maryland, LLC in order to best simulate the household products that would be used by a terrorist, criminal, or amateur chemist. Recipe ingredients are not disclosed due to security concerns related to the hazardous nature of the materials. The laboratory-grade formulations of TATP and DADP were pure formulations due to the pure grade of chemicals used in the synthesis and the washing/crystallization procedures. The clandestine formulations of TATP each used differing low-grade chemicals. One remained un-washed; the other two were washed under different conditions.
2.2 Chemical Analysis
Headspace was collected from the samples by weighing 0.50 g of each TATP and DADP sample into separate antistatic vials (ESD Plastic Containers, Linda, CA; part no. VL80L-BAS). Each vial was placed in a separate 16 oz metal jar (Paulie Jar) and allowed to equilibrate for 1 hour. At the end the equilibration period, each jar was flushed to exhaustion by pulling 500 ml of air using a gas-tight syringe which was inserted through a septum in the jar lid. The 500 ml of air was then injected into a 1 L Tedlar bag (SKC Inc.). The bags were transferred from the secure synthesizing location to the laboratory for analysis. Before destroying the samples, 0.03 g of each explosive powder was dissolved in 4 ml of acetonitrile, creating solutions of approximately 7,500 ppm. The laboratory-grade TATP and DADP samples were each collected in a different location on a separate day. Blanks were also taken in each sampling space using the same Tedlar bag method: the synthesizing location, the TATP laboratory, and the DADP laboratory.
Semi-quantitative headspace analysis was performed using solid phase microextraction-gas chromatography-mass spectrometry (SPME-GC-MS; Agilent 6,890 with a 5,973 mass selective detector). A conditioned divinylbenzene/carboxen/polydimethylsiloxane (DVB/CAR/PDMS) SPME fiber (Millipore Sigma) was exposed to the air in each Tedlar bag for 15 min. The fiber was then desorbed for 5 min in the GC inlet at 225°C with a 5:1 split. The GC column used was a 6 m DB-5MS column with 0.25 mm ID (Agilent J&W). The oven was held to 50°C for 1.5 min, then ramped to 150°C at 25 °C/min, where it was held for an additional minute. The samples were analyzed in scan mode (m/z 42.0–300.0). When scanning for DADP, the fiber desorption was delayed by 1 min in comparison to the TATP scanning samples to ensure it would be detected.
The solutions were analyzed qualitatively using direct analysis in real time-mass spectrometry (DART-MS; JEOL AccuTOF-DART-4G). In positive mode, the ionization of TATP and DADP occurred by forming the adducts [TATP + NH4]+ (m/z 240) and [DADP + NH4]+ (m/z 165), respectively, by introducing a 1:10 solution of ammonium hydroxide in methanol simultaneously with the sample. This was not required in negative mode. The mass spectrometer scanned from m/z 40.0000 through 600.0000. Orifice 1 operated at 20 V, while orifice 2 and the rings lens each operated at 5 V. The interface was heated to 200°C with an aluminum cap. Each run began with polyethylene glycol (PEG) as a calibration, followed by the introduction of each solution in sequence using separate capillary tubes. Samples were introduced in triplicate.
2.3 Canine Testing
2.3.1 Dogs and Housing
Eleven Labrador retrievers (8 M/3 F) between 1 and 6 years old (mean age: 3.16) from the Auburn University College of Veterinary Medicine (AUCVM) Canine Performance Sciences (CPS) detection dog program participated in this study. Dogs were housed in individual indoor/outdoor runs within the kennel complex at the AUCVM. All activities were approved and monitored by the AUCVM Institutional Animal and Care Use Committee in accordance with the U.S. Animal Welfare Act (IACUC # 2017-3124). The AUCVM is an Association for Assessment and Accreditation of Laboratory Animal Care International (AAALAC) accredited facility.
All dogs had previous experience detecting the odor of TATP (both laboratory grade and the POCR training aid) as well as various commercial/military explosive odors typical of those operational detection dogs are trained on. This training was not a part of the current study and varied across dogs. Therefore, as an additional confirmation of dogs’ proficiency in detecting TATP for the purpose of the current study, baseline trials were included in the test session (described below). Importantly, dogs did not have experience with any peroxide-based explosives other than TATP.
2.3.2 Generalization Testing
Testing occurred in a dedicated training building at Auburn University’s Canine Performance Sciences. For safety reasons, odor stimuli were presented inside a cinder block (cut in half horizontally) enclosed in a specially constructed wooden box (31.75 × 31.74 cm), with wire mesh screens on the sides (Figure 2). This design allowed access to the odor while preventing physical contact between the dog and the substance, as well as blast containment in the event of a detonation. Each box was positioned on top of a cart with the base of the cart approximately 7 cm from the ground. Boxes were arranged in a ten-position circular array within an 8 × 10 m arena, created using the interior walls of the building and 1.2 m high wooden panel walls (Figure 3). Dogs were previously trained to systematically search the array off leash from first to last position in a counter-clockwise manner. After searching the last position, the handler called the dog out to prevent returning to previous positions.
Test sessions were conducted double blind, with the dogs working off leash. The handler remained outside of the test arena, seated behind the arena wall for the duration of all trials out of the dog’s sight. At the start of each trial, the handler sent the dog into the arena to sample the ten positions one at a time in a counter-clockwise manner. An experimenter inside a control room adjacent to the arena viewed the dog through a one-way mirror (Figure 3). The experimenter signaled the outcome of each trial to the handler using a remote light system that was only visible to the handler. If the dog made a correct indication (a sit response, operationally defined as full contact of the hindquarters on the ground for any duration in front of the target position [e.g., Moser et al. (2020)] or searched the last position with no false alerts on a non-target trial, the experimenter signaled with a green light and the handler recalled the dog and delivered a reward (play with a ball). If the dog searched the last position and had not indicated to a target present, the experimenter signaled with a red light and the handler called the dog out of the arena without delivering a reward. False indications (sitting at a position that did not contain a target) were ignored by the handler and the dog was allowed to continue searching the rest of the array to ensure exposure to potential targets in subsequent positions. An observer who was blind to the presence and location of targets, positioned behind a panel wall in one corner of the arena, scored whether and at which location dogs made a response. Additionally, the blind observer noted change of behavior (COB), a characteristic change in ongoing search behavior (e.g., direction, head snap) indicative of target odor recognition (SWGDOG, 2011; Minhinnick, 2016).
Two test sessions were conducted over two consecutive days, with two sets of trials per session. All dogs ran the first set of trials, followed by a break of approximately 1 hour, and then all dogs ran the second set. Two handlers alternated between dogs.
Session 1 began with a baseline measure of each dog’s ability to detect the odor of TATP, to ensure that potential failures in responding to the probed variants were due to a generalization failure rather than a deficiency in recognizing TATP. The session began with one trial presenting the POCR training aid, followed by three laboratory-grade TATP and one non-target (“blank”) trial in randomized sequence. Throughout all testing, targets appeared in a randomly selected position with distractors in all other positions. The blank trial contained all distractors (see below). If a dog missed any of the baseline target trials, the trial was repeated (occurred only once). Following this set of trials, the first probe odor (DADP) was presented across three consecutive trials. All dogs completed the baseline and DADP trials before advancing. Next, all dogs were tested on Formulation 1 of the clandestine TATP recipes across three consecutive trials. On the following day, Session 2 began with one baseline and one blank trial in randomized order, followed by three consecutive trials of Formulation 2. After all dogs completed Formulation 2 trials, Formulation 3 was tested across three consecutive trials. Responses to probe odors were reinforced like baseline trials to minimize disruption of performance. If a dog missed two consecutive probe trials, a baseline trial was inserted to maintain motivation (occurred twice, once each for two different dogs, both for the odor of DADP). All target odors were presented in identical plastic antistatic containers, which were also used as distractors (see below).
2.3.3 Controls
Distractor odors were present in all non-target positions to serve as negative controls for calculating specificity/false alarm rate. Distractors included household products (e.g., tools, office supplies, cleaning products), food items (e.g., flavorings, cooking liquids), and items related to training and testing (e.g., Nitrile gloves, empty clean containers identical to those used to contain target odors, cloth bags used in the dogs’ regular training, PDMS material used to create the POCR training aids). On each trial, at least two distractors were moved to a new position and distractors were continuously rotated in and out from a larger pool of distractors. Additionally, novel distractors were implemented to ensure that potential generalization responses to the probes did not occur based solely on the novelty of the probe odor. Four novel distractors (one for each probe) that the dogs had not previously experienced in training or in other test trials (acetone, gun oil, WD40, and Flex Seal) were presented once each for each dog. Acetone was specifically selected as it is a common household product that is chemically similar to the odors tested in order to determine whether it was a factor in generalization to the targets. Two novel distractors were presented each day, on a randomly selected trial and in a pseudo randomly selected position (if presented on a target trial, the novel distracter was placed in a position ahead of the target position to ensure it was encountered). All novel distractors, and some regular distractors, were presented in the same antistatic container that held all of the target odors during testing.
To control for potential scent marking by the dogs on the boxes, odors (targets and distractors) were moved to new positions by removing the odor container from the box and placing it in a different box, so that any marking or contamination remained on the exterior of the box and did not vary systematically with the targets. On every trial at least two items (one target and one distractor, or two distractors on blank trials) were replaced or moved to a different position.
2.3.4 Canine Performance Scoring and Analysis
On each trial, dogs’ responses were scored as a hit (response to a position containing a target), miss (no response to a position containing a target), false alarm (response to a position not containing a target), correct rejection (no response to a position containing a distractor), and COB. For analyses purposes COB were considered a miss but are reported for each target. Sensitivity (i.e., hit rate) for each target was calculated as total hits out of total presentations of the target. False alarm rate was calculated as total false alarms out of total opportunities for a false alarm (i.e., number of positions searched). Specificity was calculated as total correct rejections out of total positions searched across all trials.
A generalized linear mixed-effects model (GLMM) with a binomial distribution [lme4 package (Bates et al., 2015)] was used to analyze responses (binary variable; 1 = hit, 0 = miss) as a function of the fixed factor of target odor (POCR, TATP, DADP, Formulation 1, 2, or 3). Trial number was included as an additional fixed factor to assess effects of within-session learning, as well as the interaction between target odor and trial to assess learning across presentations specific to each odor. Dog ID was included as a random factor to control for effects of repeated testing. Additionally, to assess first-trial performance, we used a GLMM with a binomial distribution to analyze responses (hit or miss) on the first encounter of each target odor. Sensitivity to each test odor was also compared to the novel distractor false alarm rate using paired samples t-tests. The alpha level was set to 0.05 for all analyses. Analyses were performed in the R statistical program (Version February 1, 5033, RStudio) and SPSS Version 25. Data represent the mean (±SEM) unless otherwise noted.
3 Results
3.1 Chemical Analysis
Figure 4 shows the TATP headspace results. Figure 4A shows the peak areas of recovered TATP and a TATP conformer. The TATP conformer identified is not a contaminant and is naturally present in TATP samples (Denekamp et al., 2005). Notably, Formulations 1, 2, and 3 each contained a compound that was a probable TATP homologue (Figure 4B). This compound, absent in the laboratory-grade sample, had a mass spectrum consistent with a TATP homologue. A higher peak area for each compound was collected from Formulations 1 and 2 than from Formulation 3 and the laboratory-grade sample, indicating that these two formulations have a higher headspace concentration. A small amount of TATP was detected in both blanks. This was expected given the large quantities of TATP synthesized and stored in each location; however, given the difference in recovery between the samples and the blanks, it was assumed to not affect the qualitative results.
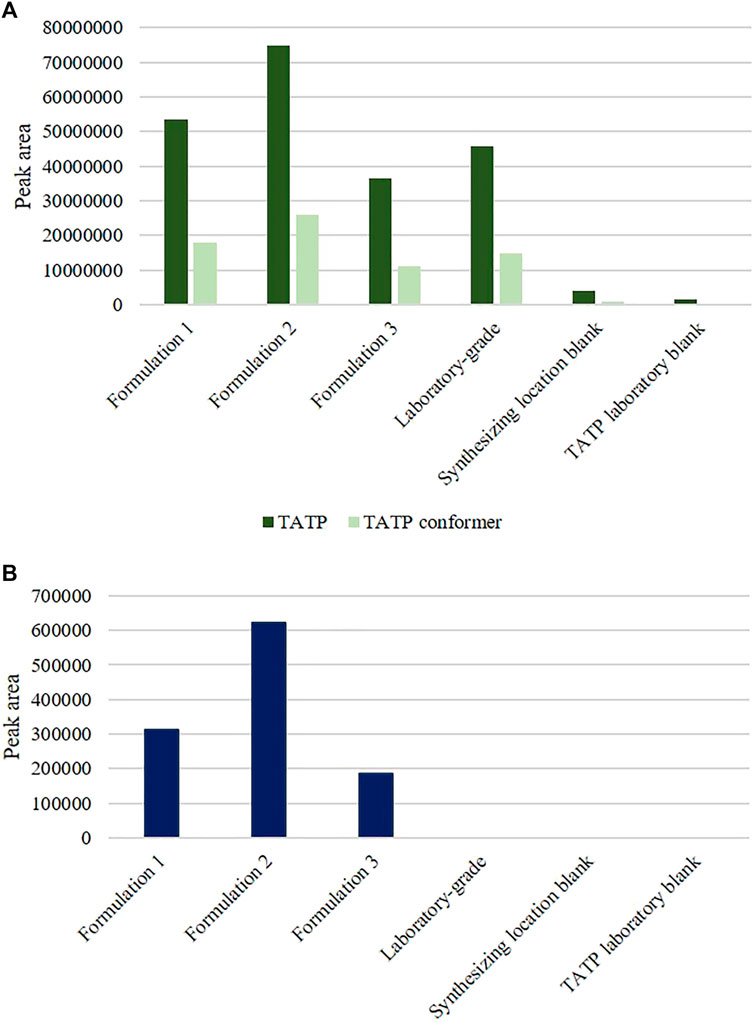
FIGURE 4. Headspace results for the tested TATP formulations showing peak areas (in arbitrary units) for (A) TATP and the TATP conformer and (B) the probable TATP homologue.
Figure 5 shows the results for the DADP and clandestine TATP formulations. DADP in the headspace of the laboratory-grade DADP was an order of magnitude above any other sample. DADP was detected in the headspace of each of the clandestine formulations of TATP (Formulations 1, 2, and 3), but was absent in the headspace of the laboratory-grade TATP. Acetone was detected in all five of the samples. Acetone is a chemical precursor and was either a residual or decomposition product. Chlorinated acetones were not observed, though they have been identified in previous studies (Oxley et al., 2013; Fitzgerald and Bilusich, 2012). Interestingly, while Formulation 3 had the lowest amount of recovered TATP and conformer compared to the other formulations, it had the highest amount of DADP and acetone. This was assumed to be due to residual or contaminant compounds that drove the decomposition and conversion of TATP to DADP. Residual compounds were likely the result of the clandestine recipes used that did not wash or recrystallize the material for purification. The DADP sample did contain a small amount of TATP and acetone, though the peak areas were smaller than the amount present in the blanks. It is therefore probable that this TATP and the acetone came from the sampling space rather than the sample itself.
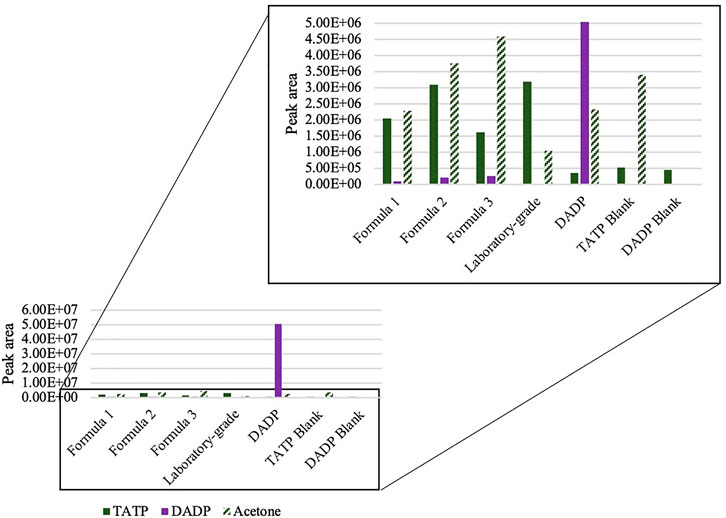
FIGURE 5. Headspace results for the tested TATP formulations and DADP showing peak areas (in arbitrary units) for TATP, DADP, and acetone. The DADP signal is given in an inset for easier visualization.
Figure 6 and Figure 7 show the DART-MS mass spectra taken in positive and negative modes, respectively. Figure 6 results agree with the headspace results, showing that Formulation 3 was more similar to the laboratory-grade TATP than the other formulations. Formulations 1 and 2 were more similar to each other. The laboratory-grade sample has the fewest ions detected in comparison to the other four samples, showing its purity. Negative mode was used to monitor the presence or absence of residual acid in the samples (Figure 7). No acid was detected. The DART-MS mass spectra showed that there were distinct differences between each formulation.
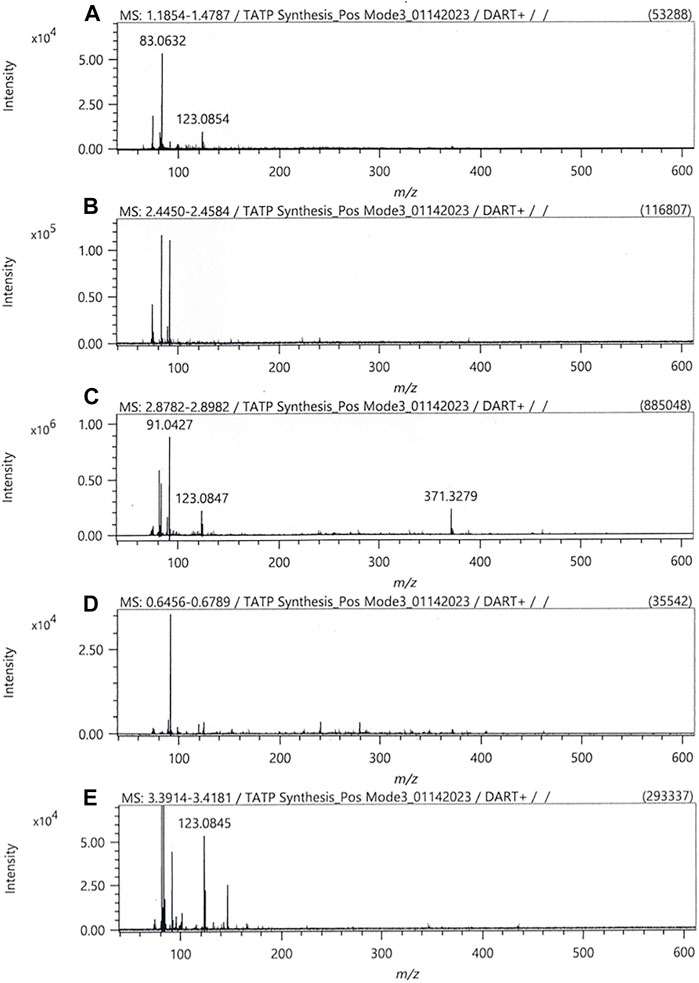
FIGURE 6. DART-MS mass spectra for Formulation 1 (A), Formulation 2 (B), Forumation 3 (C), Laboratory-grade TATP (D), and Laboratory-grade DADP (E), taken in positive mode.
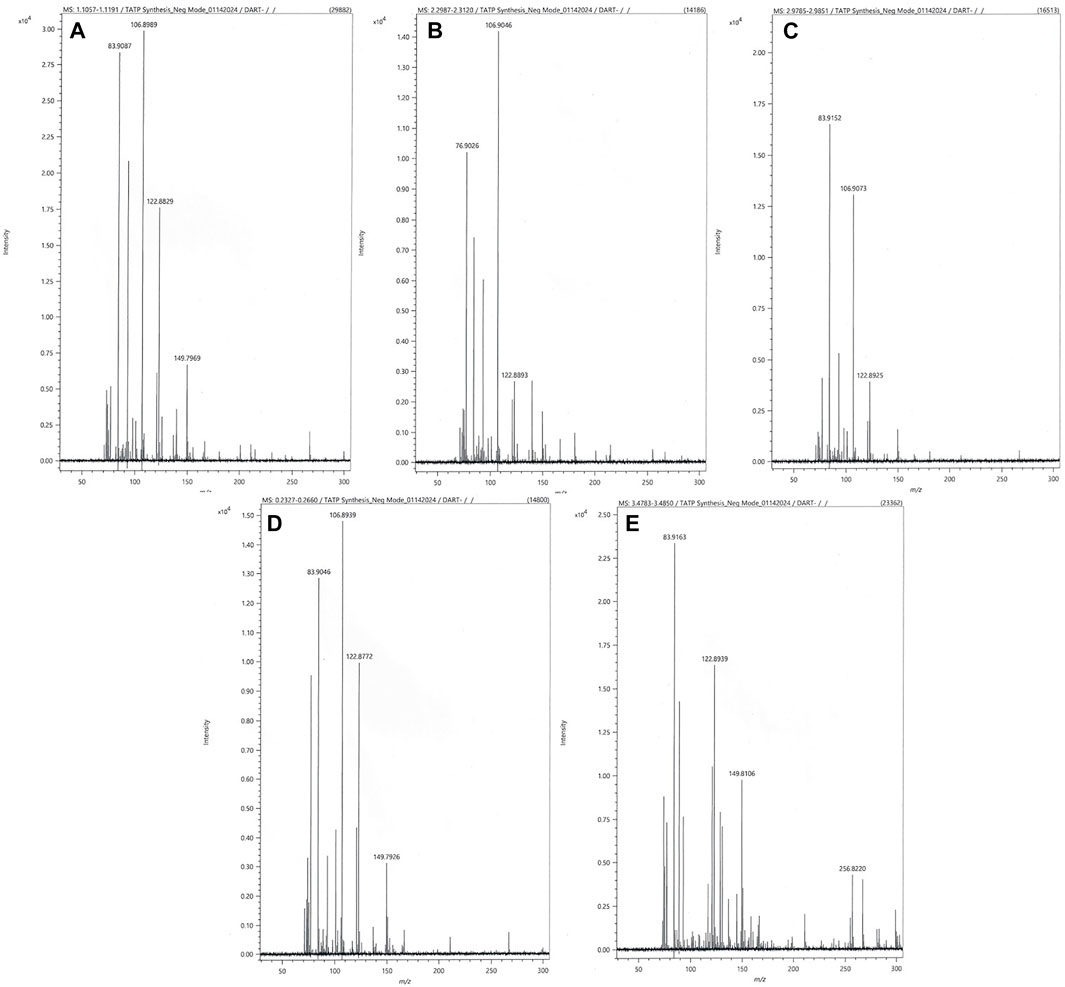
FIGURE 7. DART-MS mass spectra for Formulation 1 (A), Formulation 2 (B), Forumation 3 (C), Laboratory-grade TATP (D), and Laboratory-grade DADP (E), taken in negative mode.
3.2 Canine Testing
Baseline trials confirmed that dogs were proficient in detecting the trained TATP odor, with average sensitivities of 100% (±0) and 96.36% (±2.33) for the POCR training aid and laboratory-grade TATP, respectively. Average specificity overall was 97.75 (±0.60).
Figure 8 shows average sensitivity for each of the tested targets. Average sensitivity did not differ between any of the test or baseline targets (GLMM: zs < 1.81, ps > 0.07; Table 1). The overall false alarm rate across testing was low (1.85%), and average response rates to each test odor were significantly higher than the average response rate to novel distractors (13.64%, ±6.18, ps < 0.001). There were a total of six responses (WD40: 3, Flex Seal: 2, acetone: 1) and one COB (acetone) across the 44 novel distractor presentations.
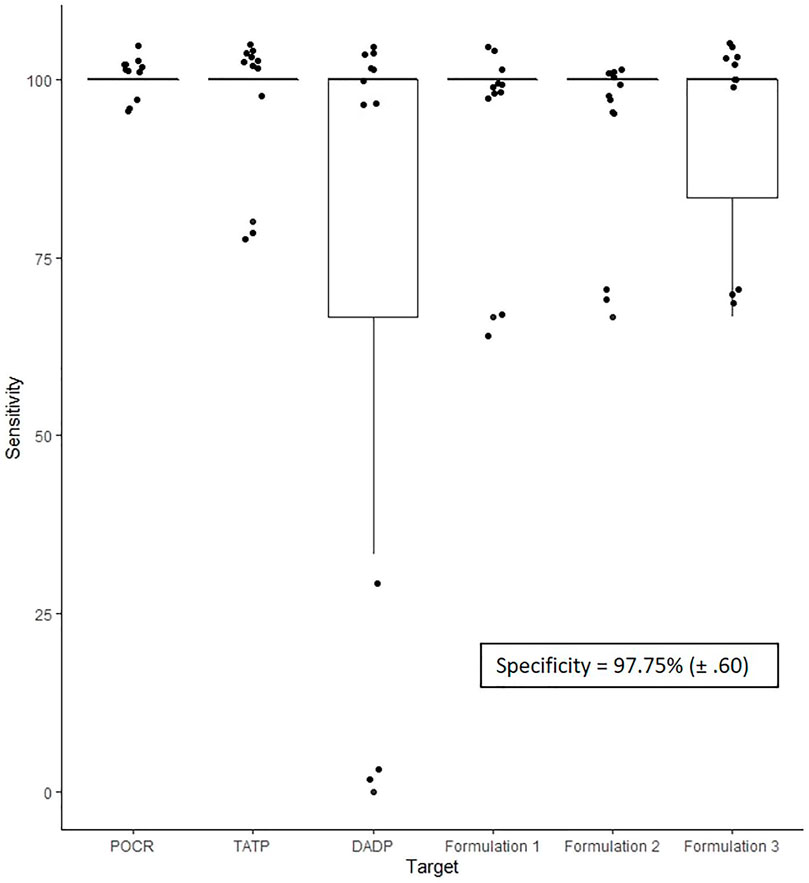
FIGURE 8. Distribution of canine sensitivity (percentage of correct responses) for each target. Horizontal lines represent medians, boxes represent the interquartile range, and whiskers represent the range of values within 1.5 X interquartile range. Dots are individual dog averages across each trial for the corresponding target, jittered to reduce over-plotting.
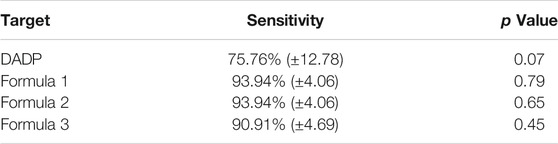
TABLE 1. Average (±SEM) sensitivity for each odor tested, and p values of GLMM for each probe odor in comparison to the pure laboratory-grade TATP sample.
There was no significant effect of trial number (GLMM: z = 0.03, p = 0.98) or interaction between target and trial number (ps >0.94), indicating that performance was not the result of within-session learning. However, responses on the first encounter of DADP and Formulation 3 were significantly lower than baseline response rates (GLMM: z = -2.60, p = 0.01 and z = -2.60, p = 0.01, respectively), while first-trial responses to Formulations 1 and 2 did not differ from baseline (ps > 0.06). At the individual level, 8/11 dogs responded on the first encounter of DADP, with two of the three dogs that did not respond showing a COB (as observed by the blind evaluator) to the target. Nine of the 11, 11/11, and 8/11 dogs responded on the first encounter of Formulations 1, 2, and 3, respectively, with no COBs observed.
4 Discussion
The current study evaluated dogs’ tendency to respond to untrained formulaic variations of TATP and related compounds after training on pure laboratory-grade TATP. Given the popularity of TATP-based HMEs and the wide variability in their manufacture, determining whether detection dogs successfully generalize to variations of the target odor profile without explicit training is important for guiding training practices. We assessed generalization using complementary canine and chemical analyses to evaluate behavioral generalization to probed target odors and compared odor profiles of the targets using chemical headspace analysis.
The qualitative DART-MS results showed that each formulation of TATP and DADP had a distinct profile. The headspace results identified a probable TATP homologue present in each of the clandestine formulations that was absent in the pure laboratory-grade sample. Also, DADP was present in each of the clandestine formulations, but was absent in the pure laboratory-grade sample. All of the samples did contain acetone, however. Oxley et al. (2013) determined that TATP in the presence of residual acid will decompose into DADP (Oxley et al., 2013). It is likely that the clandestine formulations synthesized here maintained residual compounds that facilitated TATP decomposition and conversion into DADP, leading to the presence of DADP, the homologue, and the decomposition product acetone. The presence of these compounds also makes the explosive more unstable, and therefore more dangerous. The laboratory-grade TATP did not contain the homologue or DADP, representing a purer formulation that is safer for use in canine training. Despite the differences in profiles, there were similarities that could be used to identify each as a formulation of TATP.
Responses by dogs previously trained on the odor of TATP to variants of TATP were overall high, reflecting the chemical analyses of TATP, where TATP was the highest recovered compound despite the precursor quality and synthesis. Across test trials, average response rates to the untrained probes were similar to response rates to the trained TATP targets. The false alarm rate was negligible and generalization rates were significantly higher than responses to the novel distractors, indicating that responses were controlled by the perceptual similarity of the odor rather than a lack of specificity. While the response rate to DADP was lower than the other targets, the difference was not significant and was driven by a small number of dogs. However, further analyses of dogs’ responses to the first presentation of each probe revealed that positive alerts to the first exposures to DADP and Formulation 3 were significantly lower than baseline detection. These results were supported by the headspace results, showing that Formulation 3 contained higher levels of DADP and acetone, and lower levels of TATP in comparison to the other TATP formulations tested. For these odors, subsequent exposures appeared to be necessary for dogs to confidently alert to the odor. This finding highlights the importance of isolating first-trial performance in generalization studies in order to uncover effects potentially masked by repeated exposure and reinforcement of the tested odor. Nevertheless, for these two odors, eight of the 11 dogs positively alerted to the odor on the first exposure, which could be considered a moderate rate of generalization. Further, two of the dogs that failed to perform the trained alert response to DADP demonstrated a behavioral indication of target odor recognition (i.e., COB). Importantly, this behavior was reported by a blind observer, and similar behavior towards distracting novel odors were only observed once out of 44 novel distractor presentations. Thus, given that in operational settings COBs are considered sufficient for the handler to interpret the presence of a threat and respond accordingly, a handler may not wait for a dog to exhibit a trained final response after showing a COB. Thus, the COBs observed for DADP could functionally be considered positive responses, resulting in a 91% response rate. However, the lower response rates by some dogs for DADP and Formulation 3 indicate that individual variability can exist in the perceptual similarity between TATP and these targets. Interestingly, individual differences in generalization appeared to be consistent across odors, as three first-trial misses across the four probed odors were by the same dog, and another dog was responsible for two of the first-trial misses. Other studies have reported individual differences in olfactory generalization that could be attributed to training history, breed, or other factors (DeGreeff et al., 2020), though breed and life history were largely homogenous in our sample. Further research is needed to determine factors influencing variability in generalization as observed in the current study, and whether our obtained results are generalizable to other populations of detection dogs.
Responses across the clandestine formulations of TATP tested here were roughly the same as responses to the laboratory-grade TATP, suggesting that the variations in preparation had little effect on dogs’ perception of the target odor. Caution must be used when extrapolating these results to all TATP formulations as only three specific formulations were tested and it is possible that dogs may not readily generalize to all formulas. However, these results suggest “safer” laboratory-grade TATP formulations routinely used in canine training provide a representative odor profile for generalization to other variations dogs may encounter in operations. The finding that safer TATP formulations routinely used in training can be effective reduces the need for explosive ordnance disposal teams and bomb technicians to assume greater risks with more dangerous impure TATP formulations.
Our findings that dogs generalized at high rates across the four variants is somewhat in contrast to the existing, though relatively limited, literature on canine olfactory generalization. For the most part, previous studies evaluating dogs’ tendency to respond to untrained variants of a target odor suggest that dogs are highly specific and sensitive to differences in target odor profiles, and largely do not generalize at operationally acceptable levels. This finding is most commonly demonstrated in examinations of dogs’ ability to generalize from a single component to a mixture containing the component, or vice versa (Gadberry et al., 2018; Hall and Wynne, 2018; Lazarowski and Dorman, 2014; Lazarowski et al., 2015). In the case of mixtures, failure to generalize could be due to a number of factors such as more salient components overshadowing others, chemical interactions between the components resulting in changes to their odor profile, or low discriminability between components due to their molecular similarity (Moser et al., 2019; Simon et al., 2018). Generalization or discrimination between odorants can also be influenced by their chemical relation. For example, dogs are more likely to generalize between compounds of similar carbon chain lengths (Hall et al., 2016), which appears to be a greater predictor of generalization than functional group commonality (DeGreeff et al., 2020). While generalization within other functional groups was not tested in the current study, it is possible that within a given functional group generalization is more likely than between functional groups. This would explain why there was moderate generalization between the acetone peroxides tested in this study, though this hypothesis should be tested further.
A few limitations to the current study should be noted. First, despite the dogs being reared and trained under the same conditions, there was some variability in the exact odors dogs had been previously trained to detect. Similarly, the amount of prior experience with TATP odor varied across dogs. Future research should strive to control training history as much as possible, or systematically vary previous training to determine its effect on generalization. For example, overtraining on a specific stimulus can narrow the generalization gradient (Moser et al., 2019). Therefore, our results cannot speak to the minimum amount of training needed to yield generalization across the targets tested. Nonetheless, results from our study have valuable implications as the number and types of odors dogs in our study were trained to detect resembled that of operational detection canines that are typically trained to detect a multitude of odors.
Additional limitations are inherent to the challenges of testing olfactory generalization in dogs. Primarily, the forced choice fixed-sampling procedure is a relatively artificial scenario compared to real-world operational searches that detection dogs perform. However, the external validity tradeoff in using more sterile laboratory settings is the ability to control environmental conditions and presentations of targets and non-targets, allowing for more precise accuracy metrics. Further, drawbacks of using forced choice procedures can be addressed by making the probability of encountering a target in a given trial or location unpredictable (for example, by including blanks as in the current study, or by allowing for multiple targets in the same trial) (Lazarowski et al., 2020).
Finally, including baseline trials in the test sessions was important for providing a check of dogs’ ability to detect the trained target, which was necessary for evaluating responses to the untrained probes, and were also used to maintain motivation in the event that dogs did not respond to multiple probes. However, while priming dogs with a trained target odor may enhance detection of that particular odor (Goldblatt et al., 2009), it is possible that the inclusion of the baseline trials attenuated responses to variants that followed.
5 Conclusion
The current study was the first examination, to our knowledge, of canine olfactory generalization within a class of peroxide-based explosives, adding to the literature on generalization in detection dogs which has been explored for various, decreasingly common, oxidizers (Degreeff et al., 2018; Lazarowski and Dorman, 2014; Lazarowski et al., 2015; Hall and Wynne, 2018). We found that detection dogs trained with the odor of pure laboratory-grade TATP generalized to untrained formulaic variations of TATP and a TATP homologue (DADP) at levels consistent with their trained target, indicating that modifications in manufacture did not produce significant changes in dogs’ perception of the odor profile. These findings were further confirmed by the chemical analyses showing that the formulations had distinct profiles, yet were similar enough to be identified by dogs as TATP. Further, the laboratory-grade TATP was purer than the other formulations, meaning it was more stable. These results suggest that detection dogs may be effectively trained to detect a range of TATP HMEs using safer laboratory-grade TATP training aids without compromising their ability to generalize to other variants. Further research is needed to determine whether these results are generalizable to other explosive classes and populations of detection dogs.
Data Availability Statement
The raw data supporting the conclusion of this article will be made available by the authors, without undue reservation.
Ethics Statement
The animal study was reviewed and approved by the Auburn University Institutional Animal and Care Use Committee.
Author Contributions
Conceptualization, CA and JB; Methodology, JB, SK, LL, and AS; Data collection, JB, LL, AS, and KV; formal analysis, SK, LL, and AS; writing—original draft preparation, CA, SK, LL, and AS; writing—review and editing, all authors; Supervision, CA, JB, MS, KV, and PW. All authors have read and agreed to the published version of the manuscript.
Funding
This research was funded by FBI Weapons of Mass Destruction Directorate, Emerging Threats and Technologies Unit, Chemical Biological Advanced Threat Detection, Grant number DJF-18-2300-PR-0004815.
Conflict of Interest
The authors declare that the research was conducted in the absence of any commercial or financial relationships that could be construed as a potential conflict of interest.
Publisher’s Note
All claims expressed in this article are solely those of the authors and do not necessarily represent those of their affiliated organizations, or those of the publisher, the editors and the reviewers. Any product that may be evaluated in this article, or claim that may be made by its manufacturer, is not guaranteed or endorsed by the publisher.
Acknowledgments
We thank Auburn University Canine Performance Science canine instructors Terry Fischer, Jenn Jankiewicz, and Laura Reeder for the preparation and handling of dogs in the study, and Bart Rogers for assistance with data collection. We also thank Dr. Lee Palmer for providing stand-by emergency care during the study.
References
Bates, D., Mächler, M., Bolker, B., and Walker, S. (2015). Fitting Linear Mixed-Effects Models Usinglme4. J. Stat. Soft. 67 (1), 1–48. doi:10.18637/jss.v067.i01
Cerna, K., Pinc, L., and Pachman, J. (2011). Ability of Explosives Detector Dogs to Generalize Odor of TNT. New Trends Res. Energetic Mater. Proc. Semin. 2, 542–549.
Degreeff, L. E., Peranich, K., and Simon, A. (2018). Detection of Ammonium Nitrate Variants by Canine: A Study of Generalization between like Substances. Washington, DC: Naval Research Laboratory.
DeGreeff, L. E., Simon, A. G., Peranich, K., Holness, H. K., Frank, K., and Furton, K. G. (2020). Generalization and Discrimination of Molecularly Similar Odorants in Detection Canines and the Influence of Training. Behav. Process. 177 (May), 104148. doi:10.1016/j.beproc.2020.104148
Denekamp, C., Gottlieb, L., Tamiri, T., Tsoglin, A., Shilav, R., and Kapon, M. (2005). Two Separable Conformers of TATP and Analogues Exist at Room Temperature. Org. Lett. 7 (12), 2461–2464. doi:10.1021/ol050801c
Espinosa-Fuentes, E. A., Peña-Quevedo, A. J., Pacheco-Londoño, L. C., Infante-Castillo, R., and Hernández-Rivera, S. P. (2011). “A Review of Peroxide Based Homemade Explosives: Characterization and Detection,” in Explosive Materials: Classification, Composition and Properties; Chemical Engineering Methods and Technology Series (Hauppauge, NY: Nova Science Publishers, Inc), 259–282.
Fitzgerald, M., and Bilusich, D. (2012). The Identification of Chlorinated Acetones in Analyses of Aged Triacetone Triperoxide (TATP). J Forensic Sci. 57 (5), 1299–1302. doi:10.1111/j.1556-4029.2012.02239.x
Furton, K., and Myers, L. J. (2001). The Scientific Foundation and Efficacy of the Use of Canines as Chemical Detectors for Explosives. Talanta 54 (3), 487–500. doi:10.1016/S0039-9140(00)00546-4
Gadberry, J., Good, K., Lawhon, S., and Siers, L. (2018). Explosive Detection Canines: An Examination of the Stew Training Technique. Working Dog Mag., 25–29.
Goldblatt, A., Gazit, I., and Terkel, J. (2009). “Olfaction and Explosives Detector Dogs,” in Canine Ergonomics: The Science of Working Dogs (Boca Raton, USA: CRC Press), 135–174. doi:10.1201/9781420079920.ch8
Hall, N. J., Collada, A., Smith, D. W., and Wynne, C. D. L. (2016). Performance of Domestic Dogs on an Olfactory Discrimination of a Homologous Series of Alcohols. Appl. Anim. Behav. Sci. 178, 1–6. doi:10.1016/j.applanim.2016.03.016
Hall, N. J., and Wynne, C. D. L. (2018). Odor Mixture Training Enhances Dogs' Olfactory Detection of Home-Made Explosive Precursors. Heliyon 4 (12), e00947. doi:10.1016/j.heliyon.2018.e00947
Harper, R., Almirall, J., and Furton, K. (2005). Identification of Dominant Odor Chemicals Emanating from Explosives for Use in Developing Optimal Training Aid Combinations and Mimics for Canine Detection. Talanta 67 (2), 313–327. doi:10.1016/j.talanta.2005.05.019
Kopp, C. (2008). Technology of Improvised Explosive Devices. Defence Today. Available at: http://www.ausairpower.net/SP/DT-IED-1007.pdf.
Lazarowski, L., and Dorman, D. C. (2014). Explosives Detection by Military Working Dogs: Olfactory Generalization from Components to Mixtures. Appl. Anim. Behav. Sci. 151, 84–93. doi:10.1016/j.applanim.2013.11.010
Lazarowski, L., Foster, M. L., Gruen, M. E., Sherman, B. L., Fish, R. E., Milgram, N. W., et al. (2015). Olfactory Discrimination and Generalization of Ammonium Nitrate and Structurally Related Odorants in Labrador Retrievers. Anim. Cogn. 18 (6), 1255–1265. doi:10.1007/s10071-015-0894-9
Lazarowski, L., Krichbaum, S., DeGreeff, L. E., Simon, A., Singletary, M., Angle, C., et al. (2020). Methodological Considerations in Canine Olfactory Detection Research. Front. Vet. Sci. 7 (July), 1–17. doi:10.3389/fvets.2020.00408
MacCrehan, W. A., Young, M., and Schantz, M. (2018). Measurements of Vapor Capture-and-Release Behavior of PDMS-Based Canine Training Aids for Explosive Odorants. Forensic Chemistry 11, 58–64. doi:10.1016/j.forc.2018.09.002
Minhinnick, S. (2016). “Statistical Reliability Confounders and Improvement in Advanced Dog Training: Patterns, Routines, Targets, Alerts, Distractors, Reinforcement, and Other Issues,” in Canine Olfaction Science and Law (Boca Raton, USA: CRC Press), 197–212.
Moser, A. Y., Bizo, L., and Brown, W. (2019). Olfactory Generalization in Detector Dogs. Animals (Basel) 9, 1–12. doi:10.3390/ani9090702
Moser, A. Y., Brown, W. Y., Bizo, L. A., Andrew, N. R., and Taylor, M. K. (2020). Biosecurity Dogs Detect Live Insects after Training with Odor-Proxy Training Aids: Scent Extract and Dead Specimens. Chem. Senses 45 (January), 179–186. doi:10.1093/chemse/bjaa001
Oxley, J. C., Smith, J. L., Steinkamp, L., and Zhang, G. (2013). Factors Influencing Triacetone Triperoxide (TATP) and Diacetone Diperoxide (DADP) Formation: Part 2. Propellants, Explosives, Pyrotechnics 38 (6), 841–851. doi:10.1002/prep.201200215
Oxley, J. C., and Waggoner, L. P. (2009). “Detection of Explosives by Dogs,” in Aspects of Explosives Detection (Amsterdam: Elsevier), 27–40. doi:10.1016/b978-0-12-374533-0.00003-9
Simon, A. G., Degreeff, L. E., Peranich, K., and Furton, K. G. (2018). Canine Generalization to Molecularly Similar Odors and Odor Mixtures. Washington, D.C., USA: Naval Research Laboratory.
Steinkamp, F. L., DeGreeff, L. E., Collins, G. E., and Rose-Pehrsson, S. L. (2016). Factors Affecting the Intramolecular Decomposition of Hexamethylene Triperoxide Diamine and Implications for Detection. J. Chromatogr. A 1451, 83–90. doi:10.1016/j.chroma.2016.05.013
SWGDOG (2011). SC1-abcdefghijk-Terminology. Available at: http://swgdog.fiu.edu/approved-guidelines/sc1_terminology_abcdefghijk.pdf (Accessed January 21, 2019).
Keywords: detection dogs, generalization, olfaction, explosives, triacetone triperoxide (TATP), diacetone diperoxide (DADP)
Citation: Lazarowski L, Simon A, Krichbaum S, Angle C, Singletary M, Waggoner P, Van Arsdale K and Barrow JA (2021) Generalization Across Acetone Peroxide Homemade Explosives by Detection Dogs. Front. Anal. Sci. 1:797520. doi: 10.3389/frans.2021.797520
Received: 18 October 2021; Accepted: 19 November 2021;
Published: 15 December 2021.
Edited by:
James J. Harynuk, University of Alberta, CanadaReviewed by:
Amritha Mallikarjun, University of Pennsylvania, United StatesTim Edwards, University of Waikato, New Zealand
Copyright © 2021 Lazarowski, Simon, Krichbaum, Angle, Singletary, Waggoner, Van Arsdale and Barrow. This is an open-access article distributed under the terms of the Creative Commons Attribution License (CC BY). The use, distribution or reproduction in other forums is permitted, provided the original author(s) and the copyright owner(s) are credited and that the original publication in this journal is cited, in accordance with accepted academic practice. No use, distribution or reproduction is permitted which does not comply with these terms.
*Correspondence: Lucia Lazarowski, bHVjaWEubGF6YXJvd3NraUBhdWJ1cm4uZWR1