- 1Okinawa Churashima Research Institute, Okinawa Churashima Foundation, Motobu, Japan
- 2Okinawa Churaumi Aquarium, Okinawa Churashima Foundation, Motobu, Japan
- 3Okinawa Churashima Foundation Veterinary Hospital, Okinawa Churashima Foundation, Motobu, Japan
Shark-specific artificial uterine fluid is a recently developed material suitable for maintaining viviparous shark embryos outside the maternal body. Previous studies confirmed that this fluid greatly extends the captive maintenance period of the embryonic slendertail lantern shark (Etmopterus molleri) from <1 week to several months. Its application, however, has been limited to late-term embryos. This study reports the first application of this fluid on mid-term embryos with long external gill filaments. The incubation was found to be successful, with the embryos growing to their natural birth size after 1 year. A high mortality of mid-term embryos was observed during incubation due to pressure ulcers. This indicates that not only appropriate chemical environment but also appropriate physical environment is critical for the stable maintenance of mid-term embryos.
1 Introduction
An artificial uterus is a next-generation incubator used in the medical care of premature birth fetuses and conservation breeding of viviparous animals in public zoos and aquaria [e.g., (1)]. Recent studies by the authors proposed a new artificial uterine system for viviparous elasmobranchs (sharks and batoids). This method is characterized by the usage of “artificial uterine fluid,” a urea-containing liquid that has osmotic pressure and salinity nearly equivalent to those of shark blood plasma (2). In the first application of this method at the Okinawa Churaumi Aquarium (Okinawa, Japan) from 2020 to 2021, two embryonic slendertail lantern sharks, Etmopterus molleri, were successfully maintained for ~5 months until they reached normal birth size (2). These specimens, however, failed to adapt to a seawater environment after “delivery.” Thereafter, in the second round of incubation from 2021 to 2022, an alternative seawater adaptation protocol was applied to the embryos, allowing the stable maintenance of juveniles after delivery (3).
Despite the high efficacy of artificial uterine fluid, as confirmed in previous studies, one of the concerns with this fluid is its applicability to early- to mid-term embryos. Considering the previous studies focused only on late-term embryos—from the very late period of stage 32 to the end of stage 34, the applicability of the fluid to embryos at earlier stages currently remains hypothetical (2). It is generally accepted that embryonic physiological mechanisms dynamically change with its development. Studies on the oviparous cloudy catshark (Scyliorhinus torazame) showed that the mechanisms of osmoregulation, respiration, and nutrient absorption shift from embryonic mode to post-hatched mode in the middle of embryonic development when the respiratory slits of the egg capsule open [e.g., (4–6)]. Similar physiological shifts are also likely to occur in viviparous sharks, and if so, it is possible that the environmental conditions applied to late-term embryos in Tomita et al. (2) cannot be used to maintain earlier-term embryos.
This study reports a third-round incubation experiment on a slendertail lantern shark conducted from 2022 to 2023. This experiment is distinguished from the previous experiments in that it started the incubation half a year earlier in the development process than the previous experiments.
2 Materials and methods
2.1 Embryos
A total of 33 slender-tail lantern shark embryos originating from five females were used in this study. Local fishers caught these females during hook-and-line fishing on April 28, 2022, in the sea near Okinawa Island, Japan. They were transported in a cooler box filled with cold seawater but died within 6 h of returning to the port and were donated to the Okinawa Churaumi Aquarium. After confirming embryonic survival with ultrasonography, the uterus containing three to four embryos each was opened with tweezers and a scalpel. The anterior or posterior portion of the uterus was then cut off with surgical scissors and the embryos were released into a Petri dish. The embryos were immediately introduced into the incubation chamber of the artificial uteri in the Okinawa Churaumi Aquarium.
For embryonic developmental staging, the criteria proposed by Ballard et al. (7) were used to divide embryonic development into 34 stages. These criteria were originally developed for the oviparous catshark Scyliorhinus canicula, but are widely used for elasmobranchs in general.
2.2 Experimental design
Embryonic incubation was conducted using two artificial uteri with the same systems used by Tomita et al. (3). The system details have been described by Tomita et al. (2). The embryos were placed separately in small (350–500 mL) glass or ceramic containers in the incubation chamber of the artificial uterus. The number of embryos in each container varied between one and three depending on the size of the container. The upper side of the containers was covered with a plastic net (mesh size = 1.5 mm), allowing free exchange of the incubation fluid between the inside and outside of the containers. The containers, including the embryos, were flexibly transferred across the artificial uteri depending on the condition of the embryos and the artificial uterus.
The chemical environment of the incubation fluid was changed over time following the protocol described by Tomita et al. (3). This was a two-step process that included stable maintenance and pre-adaptation periods. The stable maintenance period was characterized by embryonic maintenance in an artificial uterine fluid with the same chemical composition as described by Tomita et al. (2). In contrast, the pre-adaptation period was characterized using an incubation fluid whose chemical composition was shifted from that of artificial uterine fluid to seawater in a stepwise manner. The incubation fluid was prepared by mixing artificial uterine fluid and seawater in various proportions during the process; the proportion of artificial uterine fluid decreased from 100 to 75, 50, 25, 12.5, and 6.3% in volume, and conversely, that of seawater increased from 0 to 25, 50, 75, 87.5, and 93.7%.
The fluid chemistry was measured twice daily (9:00 and 16:00) throughout the incubation period using an automated clinical chemistry analyzer (DRI-CHEM NX600, Fujifilm Co., Tokyo, Japan). The temperature of the fluid was maintained at 12°C.
When the external yolk sacs of the embryos were fully absorbed, they were released from the incubation container into a 500 L seawater tank set outside of the artificial uterus. In analogy to natural delivery, during which the embryo is released from a spatially restricted environment and obtains relevant freedom in locomotion, this process is defined as artificial “delivery”.
3 Results
3.1 Embryonic incubation
The embryos had the following morphological characteristics at the start of captive maintenance: (1) long external gill filaments projected from the gill slits and spiracles; (2) arched mouth opening; (3) no coloration on the body surface except for the black ring-like pigmentation surrounding the pupil; (4) presence of pectoral, pelvic, dorsal, and caudal fin lobes; and (5) presence of a small bud of rostral protrusion (Figure 1A). This indicated that they belonged to stage 31. The pigment covered the entire eyeball after ~100 days of incubation. Body pigmentation begins at the basal part of the dorsal and caudal fins. The external gill filaments then regressed (stage 32, Figures 1B–D). Regression of the external gill filaments was completed after ~300 days of incubation, after which the external yolk sac became much smaller (stage 33, Figures 1E, F). The external yolk sac was completely absorbed just before the “delivery”, presenting a body morphology identical to a newborn (stage 34). The size of the embryos increased from ~3–15 cm in total length throughout the incubation period. The changes in the chemical composition of the artificial uterus are presented in the lower panel of Figure 1.
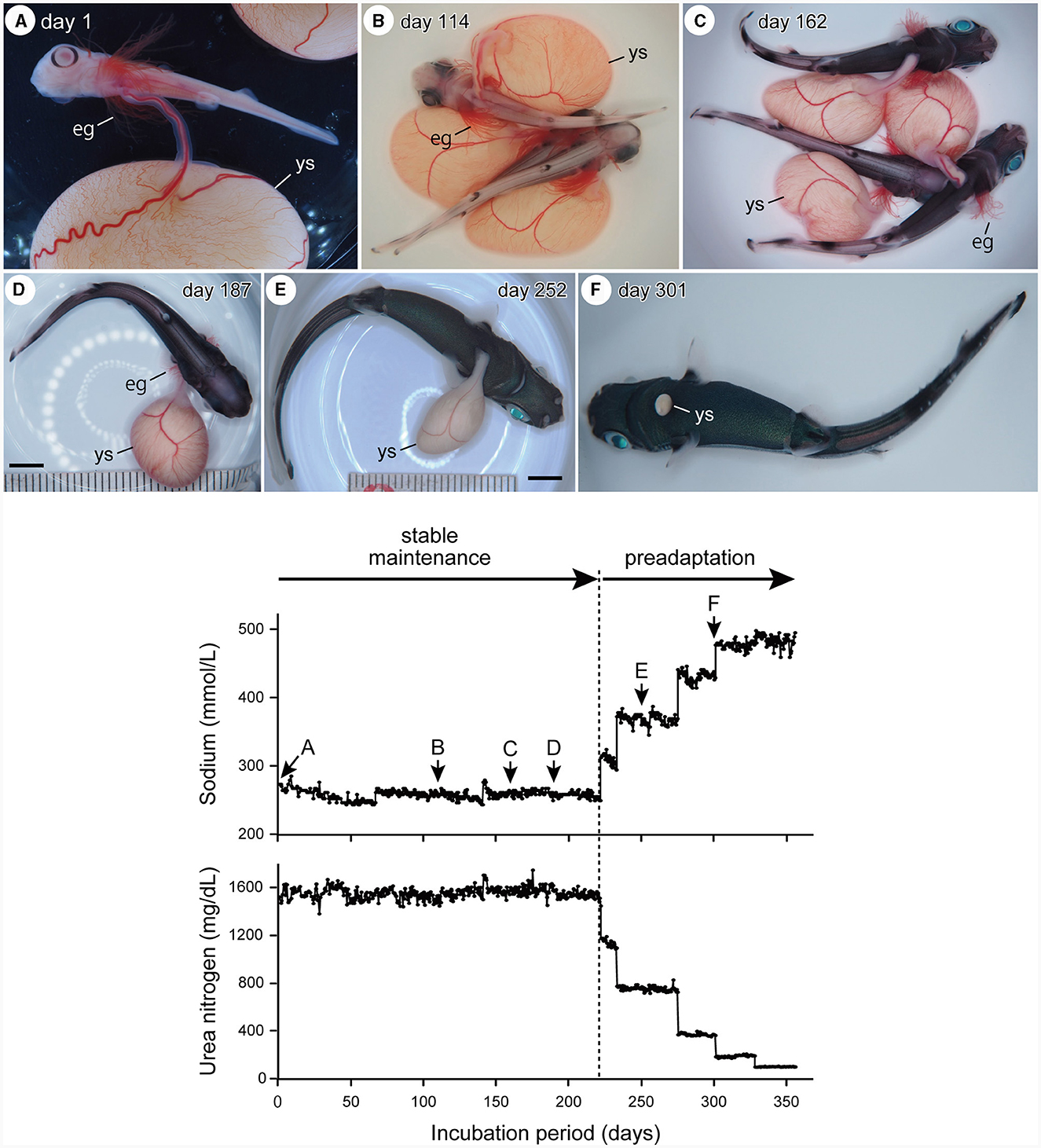
Figure 1. Upper panel: temporal changes in embryo external morphology through incubation, showing a retraction of external gill filaments (eg), reduction of external yolk-sac (ys), and change in body coloration (A, stage 31; B–D, stage 32: E, F, stage 33). Scale bars = 1 cm. Lower panel: temporal change in the chemical composition (sodium and urea) of the incubation fluid before artificial “delivery.” During the stable incubation period, the embryos were maintained in the artificial uterine fluid of which the salinity and osmotic pressure were equivalent to shark blood plasma. During the preadaptation process, the embryos were maintained in the fluid in which the sodium and urea concentrations were shifted toward the seawater. Alphabets (A–F) attached to the sodium graph indicate the timing at which the corresponding embryo photographs in the upper panel were taken.
A total of three embryos reached birth size. Two were delivered on April 10th, 2023 (embryonic incubation period of 347 days), and the remaining were delivered on April 18th, 2023 (embryonic incubation period of 355 days). These three embryos were moved from the artificial uterus and maintained in a 1,000 L seawater tank. They spent most of the time resting on the floor of the seawater tank and sometimes swam actively in response to light stimuli. The first meal comprising a mixture of minced chub mackerel, Scomber japonicus, and minced sakura shrimp, Lucensosergia lucens was fed to the specimens on the eighth day after “delivery”.
3.2 Notes on the embryonic mortalities
Most embryonic mortalities due to pressure sores occurred in stage 31 embryos (Figure 2). A total of 14 specimens (42% of the original number of specimens) died in the first month, and an additional six specimens (18% of the original number of specimens) in the next month. The following processes were observed in the embryo before death: (1) petechiae appeared on the surface of the external yolk sac. These petechiae generally appeared on the yolk sac membrane where the wall of the incubation container was in contact. (2) The number and size of the petechiae increased over time, covering a large area of the external yolk sac (Figures 2A, C). (3) The external yolk sac ruptured (Figure 2B) and the embryo died. This type of death ceased during stage 32.
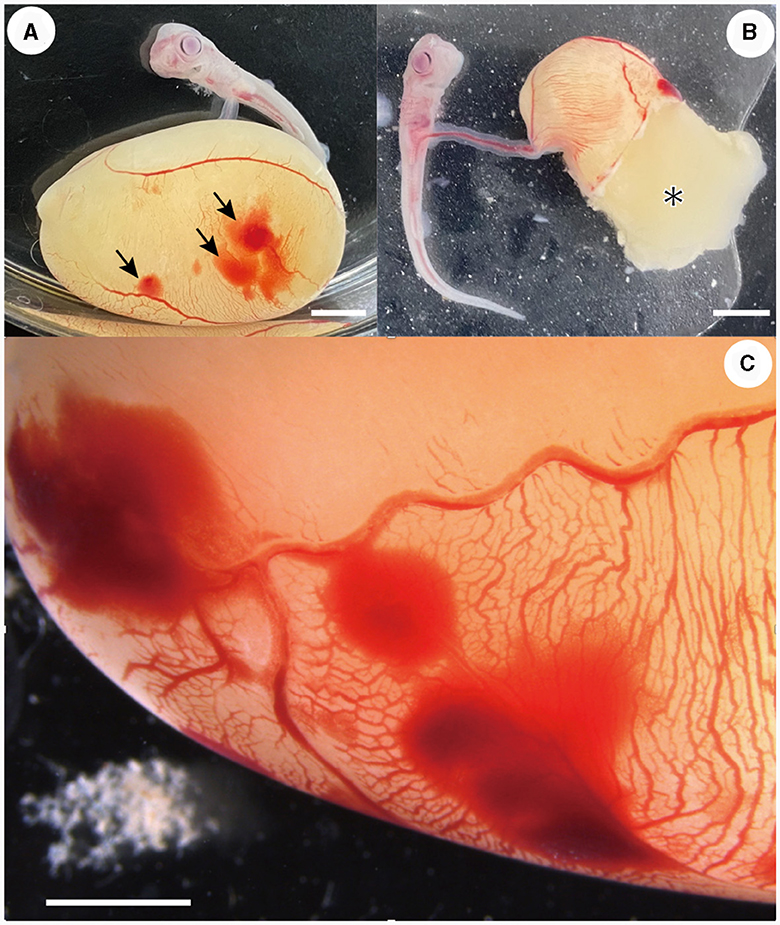
Figure 2. Embryos that died due to pressure ulcers. (A) Petechiae (arrows) were observed on the external yolk-sac membrane (day 16 of incubation). (B) External yolk sac was ruptured and yolk (*) was released (day 14 of incubation). (C) Close-up view of the petechiae occurred on the external yolk-sac (day 5 of incubation). Scale bars = 5 mm.
Unexpected mass mortality occurred on July 27th, 2022 (day 91 of incubation) in the artificial uterus. On the morning of that day (9:00 a.m.), six embryos were found dead, and one died the following day. No pre-mortality symptoms (e.g., bacterial or ciliate infections on the skin surface) were observed under an optical microscope, suggesting environmental reasons (e.g., temporal anoxia). This suggestion is further supported by the observation that these dead embryos were incubated in a relatively deep container (depth >8.5 cm), which may have a low fluid exchange rate between the inside and outside of the container, and the embryos incubated in a shallower container (depth < 5.5 cm) survived in the same artificial uterus. However, the exact cause was undetermined. There were no abnormalities in ammonia, salinity, or urea concentrations that were detected in the incubation fluid before or after this event.
4 Discussion
This study is the first to confirm that the artificial uterine fluid developed by Tomita et al. (2) has the ability to maintain mid-term (stage 31) embryos. The 355 days of embryonic incubation achieved in this study is possibly the longest reliable record for viviparous elasmobranchs and other vertebrates (1, 8, 9). Although there are no published data on the gestation period of slendertail lantern sharks, it was assumed that the gestation period of the species was ~1.5 years. This is based on the observation that pregnant females caught in November, 6 months before incubation commenced, include ovulated eggs with no visible embryo (probable stage 1–2 embryo; TT, pers. obs.). Considering this gestational period, the incubation period of the study corresponded to approximately two-thirds of the entire gestational period. This estimate is supported by data from the oviparous sharks Scyrhiorhinus canicula and Chilloscyllium punctatum, whose embryonic development from stages 31–34 accounts for 62 and 68% of the entire developmental process, respectively (7, 10).
Despite the success of long-term embryonic incubation, the technical issue of high mortality in mid-term embryos due to pressure ulcers persists. Such death ceased in embryos after the late portion of stage 32, where the body movement became strong enough to lift and rotate the external yolk sac, suggesting that the removal of permanent pressure on the yolk sac is effective in avoiding this problem. Based on this expectation, the container with the embryo was gently shaken to detach the external yolk sac surface from the container wall once or twice a week after the first case of pressure ulcer was observed. This procedure, however, did not stop the symptoms, and its efficacy could not be quantitatively evaluated in this study. This problem may be avoided in future studies by coating the inner wall of the container with a soft and low-friction material such as mucin hydrogel, which surrounds the embryo in the egg capsule of oviparous elasmobranchs (11).
The present study sheds light on the poorly understood uterine function of the lantern shark, especially the nutrient supply to embryos. This development in elasmobranchs was originally found in myliobatiform stingrays. The inner wall of the uterus is covered with numerous filamentous structures (trophonemata) that secrete lipid-rich “milk”. The embryo grows to a large size at the magnitude of 1,680–4,900% in weight by drinking this fluid [e.g., (12)]. Similar nutrient supply functions were later confirmed in a wide range of elasmobranch taxa, although the amount and composition of nutrients and secretion mechanisms vary greatly among different taxa [e.g., (13)]. The only study on this function in lantern sharks was conducted by Cotton et al. (14). They compared the organic weight of embryonic great lantern sharks (Etmopterus princeps) at different developmental stages and imply the possibility of some level of nutrient transfer from the mother to the embryo. The pattern of organic weight change is, however, determined not only by the amount of maternal nutrient input but also by other factors, such as gestation period and embryonic metabolic rate. Their hypothesis, thus, remains controversial. Interestingly, the incubated embryos that did not acquire any external nutrients grew to ~15 cm in total length. This size matches the natural birth size reported for this species (15). It is, therefore, likely that the maternal nutrient supply, when present, was small in amount in the slender-tail lantern shark, and its absence had little impact on the embryonic growth of this species. In species in which the uterus exhibits strong nourishing capabilities, it will be necessary to add a nutrient supply function to the current artificial uterine system, a task our research group is currently focusing on.
Data availability statement
The original contributions presented in the study are included in the article/supplementary material, further inquiries can be directed to the corresponding author.
Ethics statement
Ethical review and approval were not required for the animal study because the maintenance and handling of fish in this study were conducted in strict accordance with the guidelines for animal experiments of the Okinawa Churashima Foundation, with the same consideration of animal care and welfare as that for “higher” vertebrates (reptiles, birds, and mammals), as per the guidelines stipulated. The approval from the Institutional Animal Care and Use Committee of Okinawa Churashima Foundation, which is required for higher vertebrates, is waived for “lower” vertebrates including fishes. The studies were conducted in accordance with the local legislation and institutional requirements.
Author contributions
TT: Conceptualization, Data curation, Formal analysis, Funding acquisition, Investigation, Methodology, Validation, Visualization, Writing – original draft. MT: Conceptualization, Data curation, Formal analysis, Investigation, Methodology, Resources, Supervision, Validation, Writing – review & editing. AK: Data curation, Formal analysis, Investigation, Methodology, Resources, Validation, Visualization, Writing – review & editing. KMu: Data curation, Formal analysis, Investigation, Methodology, Validation, Visualization, Writing – review & editing. KMi: Data curation, Formal analysis, Investigation, Validation, Writing – review & editing. KS: Conceptualization, Methodology, Supervision, Validation, Writing – review & editing.
Funding
The author(s) declare financial support was received for the research, authorship, and/or publication of this article. This study was supported by JSPS KAKENHI (grant numbers 19K04064 and 23K05930).
Acknowledgments
We thank Hiromi Morota, Hiroko Takaoka, Toshiki Higa, and other staff members of the Okinawa Churaumi Aquarium for their assistance in maintaining the artificial uterus. We also thank Nagisa Yano, Sayuri Kino, and Keiichi Ueda (Okinawa Churashima Foundation Veterinary Hospital) for their assistance with the operation of DRI-CHEM NX600.
Conflict of interest
The authors declare that the research was conducted in the absence of any commercial or financial relationships that could be construed as a potential conflict of interest.
Publisher's note
All claims expressed in this article are solely those of the authors and do not necessarily represent those of their affiliated organizations, or those of the publisher, the editors and the reviewers. Any product that may be evaluated in this article, or claim that may be made by its manufacturer, is not guaranteed or endorsed by the publisher.
References
1. Otway NM, Ellis MT. Construction and test of an artificial uterus for ex situ development of shark embryos. Zoo Biol. (2012) 31:197–205. doi: 10.1002/zoo.20422
2. Tomita T, Toda M, Murakumo K, Kaneko A, Yano N, Nakamura M, et al. Five-month incubation of viviparous deep-water shark embryos in artificial uterine fluid. Front Mar Sci. (2022) 9:825354. doi: 10.3389/fmars.2022.825354
3. Tomita T, Toda M, Kaneko A, Murakumo K, Miyamoto K, Sato K. Successful delivery of viviparous lantern shark from an artificial uterus and the self-production of lantern shark luciferin. PLoS ONE. (2023) 18:e0291224. doi: 10.1371/journal.pone.0291224
4. Tomita T, Nakamura M, Sato K, Takaoka H, Toda M, Kawauchi J, et al. Onset of buccal pumping in catshark embryos: how breathing develops in the egg capsule. PLoS ONE. (2014) 9:e109504. doi: 10.1371/journal.pone.0109504
5. Takagi W, Kajimura M, Tanaka H, Hasegawa K, Ogawa S, Hyodo S. Distributional shift of urea production site from the extraembryonic yolk sac membrane to the embryonic liver during the development of cloudy catshark (Scyliorhinus torazame). Com Biochem Physiol Part A Mol Integr Physiol. (2017) 211:7–16. doi: 10.1016/j.cbpa.2017.05.019
6. Honda Y, Ogawa N, Wong MK-S, Tokunaga K, Kuraku S, Hyodo S, et al. Molecular mechanism of nutrient uptake in developing embryos of oviparous cloudy catshark (Scyliorhinus torazame). PLoS ONE. (2022) 17:e0265428. doi: 10.1371/journal.pone.0265428
7. Ballard WW, Mellinger J, Lechenault H. A series of normal stages for the development of Scyliorhinus canicula, the lesser spotted dogfish (Chondrichthyes: Scyliorhinidae). J Exp Zool. (1993) 267:318–36. doi: 10.1002/jez.1402670309
8. Gilbert PW. The ability of yolk-sac dogfish pups to survive outside the uterus. Bull Mt Desert Isl Biol Lab. (1959) 1959:68.
9. Evans DH, Oikari A, Kormanik GA, Mansberger L. Osmoregulation by the prenatal spiny dogfish, Squalus acanthias. J Exp Biol. (1982) 101:295–305. doi: 10.1242/jeb.101.1.295
10. Onimaru K, Motone F, Kiyatake I, Nishida K, Kuraku S. A staging table for the embryonic development of the brownbanded bamboo shark (Chiloscyllium punctatum). Dev Dyn. (2018) 247:712–23. doi: 10.1002/dvdy.24623
11. Lenain E, Henderson AC. Egg-jelly production and composition in an oviparous chondrichthyan, Scyliorhinus canicula (Linnaeus, 1758). J Appl Ichthyol. (2020) 36:675–81. doi: 10.1111/jai.14080
12. Hamlett WC, Musick JA, Eulitt AM, Jarrell RL, Kelly MA. Ultrastructure of uterine trophonemata, accommodation for uterolactation, and gas exchange in the southern stingray, Dasyatis americana. Can J Zool. (1996) 74:1417–30. doi: 10.1139/z96-157
13. Tomita T, Nakamura M, Nozu R, Ogawa N, Toda M, Sato K. Mode of uterine milk secretion in the white shark. Anat Rec. (2022) 305:1724–31. doi: 10.1002/ar.24860
14. Cotton CF, Grubbs RD, Dyb JE, Fossen I, Musick JA. Reproduction and embryonic development in two species of squaliform sharks, Centrophorus granulosus and Etmopterus princeps: evidence of matrotrophy? Deep Sea Res. (2015) 115:41–54. doi: 10.1016/j.dsr2.2014.10.009
Keywords: artificial uterus, artificial uterine fluid, conservation breeding, elasmobranch, lantern shark
Citation: Tomita T, Toda M, Kaneko A, Murakumo K, Miyamoto K and Sato K (2024) One-year extra-uterine life support for viviparous shark embryos: first technological application to mid-term embryos. Front. Fish Sci. 2:1352871. doi: 10.3389/frish.2024.1352871
Received: 09 December 2023; Accepted: 30 January 2024;
Published: 15 February 2024.
Edited by:
Julia Liv Yvonne Spaet, University of Cambridge, United KingdomReviewed by:
Wataru Takagi, The University of Tokyo, JapanJose Castro, Southeast Fisheries Science Center (SEFSC), United States
Copyright © 2024 Tomita, Toda, Kaneko, Murakumo, Miyamoto and Sato. This is an open-access article distributed under the terms of the Creative Commons Attribution License (CC BY). The use, distribution or reproduction in other forums is permitted, provided the original author(s) and the copyright owner(s) are credited and that the original publication in this journal is cited, in accordance with accepted academic practice. No use, distribution or reproduction is permitted which does not comply with these terms.
*Correspondence: Taketeru Tomita, dC10b21pdGFAb2tpY2h1cmEuanA=