- 1Private Laboratory, Warsaw, Poland
- 2Department of Life and Environmental Sciences, Bournemouth University, Poole, United Kingdom
In protists, rare species include dormant organisms and those that do not reach high population abundance, and therefore, are not detected in the samples. These rare microorganisms are part of the so-called ‘rare microbial biosphere’. Although some may occasionally become abundant or dominant under particular environmental conditions, others do have a very rare occurrence, characterized by always having very low populations. The rare protist biosphere represents a significant component of microbial communities, and it is crucial for maintaining ecosystem biodiversity and function. In this study, we aim to demonstrate the richness of the rare ciliate biosphere and hypothesize on the reasons of rarity by combining morphological and ecological details on some exceedingly rare ciliates. The study includes the first report of different morphotypes of Dactylochlamys since 1928, first report of Penardiella undulata since 1930, second report of Penardiella interrupta since 1930, and an undescribed Penardiella species. The first report of Bryophyllum caudatum since 1933, microscopical observations and taxonomical discussion of the rare Legendrea bellerophon and of and undescribed Legendrea species. A new Apertospathula sp. with prokaryotic endosymbionts is described. The rarity of these species is explored and explained by their anaerobic metabolism combined with their prey selection.
Introduction
Although at lower taxonomic ranks microbial life can be less species-rich than some metazoan groups, like Coleoptera beetles for which almost half a million species have been described (May, 1988; Stork, 2018), the diversity of microbes that can be found in even just a single drop of water is stunningly vast, covering protists, bacteria, archaea, fungi, animals, and plants. Globally, one million insect species have been named, and an estimated 0.5 million probably remain undiscovered (Stork, 2018). In contrast, the total number of named free-living ciliate species, one of the most diverse group of protists, is estimated to be no more than a few thousand (Finlay et al., 1996; Fenchel et al., 1997). Among the described and named ciliate species, many constitute rare species that remain understudied and often forgotten, except for descriptions found in old library volumes of publications. An estimated 1.5-28% of all microbes (prokaryotes and eukaryotes) are considered rare taxa due to their scarcity and low abundance during most temporal periods and across most environmental conditions (Finlay et al., 1998; Dunthorn et al., 2014; Shade et al., 2014; Lynch and Neufeld, 2015; Jousset et al., 2017). In protists, rare species include slow-growing organisms that never reach high population densities, like those inhabiting the cold deep oceanic zones, as well as those that survive under unfavorable environmental conditions by remaining dormant or present at very low abundance (Esteban and Finlay, 2003; Galotti et al., 2014). These rare microorganisms are part of the so-called ‘rare microbial biosphere’, which may comprise species key for the functioning of ecosystems (Jousset et al., 2017; Esteban and Fenchel, 2020). Although some may become abundant or dominant locally under stringent environmental conditions that allow their growth, others nonetheless do have a very rare presence, characterized by very low population abundance (Weiss et al., 2022).
In this study we document exceedingly rare and new ciliate species/genera; Three morphotypes of Dactylochlamys, first report of a forgotten Penardiella after 100 years, a new Apertospathula species with thousands of endosymbiotic prokaryotes, report of Legendrea bellerophon and a new Legendrea, and first report of Bryophyllum caudatum since 1933. Members of some of these genera have been reported only a handful of times in published protistological literature, starting with German protozoologist Lauterborn (1901; 1908), followed by Penard (1922); Wetzel (1928); Kahl (1930); Kreutz and Foissner (2006), and more recently by Pomahač et al. (2023). The identity of these ciliates has often confused and eluded researchers, likely due to their unusual morphology, with some suggesting they might be mistaken with larval stages of a suctorian ciliate (Kahl, 1930) or even a flagellate (Penard, 1922). Similarly, the lack of available high-resolution microscopy and molecular data for these organisms has hampered their classification. The limited amount of knowledge available for these remarkable ciliates provide an excellent opportunity to use contemporary methods, including microscopy and phylogenetics, to gain a greater understanding of their ecology, morphology, and taxonomic position within the ciliate tree.
Ciliates belonging to the genus Dactylochlamys, Legendrea, Apertospathula and Penardiella have previously been found inhabiting the loose, organic-rich lower layers of water bodies, known as the sapropel (Lauterborn, 1901), which is typically formed by decaying remains of aquatic plants and animals (Esteban and Fenchel, 2020). Sapropelic habitats are characterized by the absence of oxygen, presence of high levels of sulfides and virtual absence of metazoa. This environment provides a niche for phagotrophic microbial eukaryote predators, like the mentioned genera, that have fermentative metabolisms and obtain nutrients by feeding mostly on anaerobic microbes present in the same environments Weiss et al., 2022). To rediscover these elusive ciliates, we performed intensive sampling and examination of sediments from a variety of freshwater habitats. We hypothesize that the rarity of these ciliates can be explained by their anaerobic metabolism combined with their prey selection.
Materials and methods
Sampling sites
Samples were collected from the sediment of freshwater lakes Las Kabacki (coordinates 52.1254794, 21.0448959), Zalew Bolimowski (coordinates 52.062689, 20.190242), and Park Skaryszewski (coordinates 52.2421, 21.0544), and from a swamp in Gmina Nadarzyn (coordinates 52.094506, 20.779252). All the sampling sites were in the Warsaw area (Poland).
Sample collection
Lake water and sediment sampling was performed using a plastic bottle attached to the end of a telescopic rod. Collected samples were kept in the dark and at room temperature in airtight containers to minimise exposing the samples to oxygen. Shore samples from the swamp were collected from a maximum depth of 30 cm, by dragging a container along the surface sediment and ensuring that the top 10cm of sediment was collected.
Microscopy
Samples were checked daily, in some instances samples were observed for 12 hours after collection to observe the samples more in their “fresh” state. Samples which showed signs of stability were kept often for up to four months. After the initial 12h samples were enriched with boiled wheat grains to encourage growth of dormant ciliates (cysts) and to increase the abundance of rare ciliates. All microscopical imaging, including brightfield, Differential Interference Contrast (DIC) and fluorescence microscopy, was performed using a Zeiss Axioscope 5 with Zeiss Neofluar 10x 0.3NA, Zeiss Neofluar 20x 0.50NA, Zeiss Neofluar 40x 0.75NA and Zeiss 63x 1.40NA Plan-Apochromat objectives.
DNA extraction, amplification, and sequencing
Due to the scarcity of the ciliate, molecular data were obtained from a single one-cell specimen of Dactylochlamys pisciformis, collected from the sediment of the lake in Las Kabacki (see above). Using a glass micropipette, the cell was hand-picked, washed three times with mineral water, and then isolated in an Eppendorf tube and stored at –80°C prior to molecular analysis. Lysis of the cell was achieved by subjecting the cell while still in the Eppendorf tube to five freeze–thaw cycles (between –80°C and room temperature). Total DNA was then amplified from the isolated single-cell sample using the REPLI-g Single Cell Kit (QIAgen), according to the protocol provided by the manufacturer. The 18S rRNA gene was then amplified using PCR, performed using Phusion polymerase and Phusion Green HF Buffer (ThermoScientific), and primers Euk528F (5’- CGGTAATTCCAGCTCC) and U1492R (5’- GGTTACCTTGTTACGACTT) (Edgcomb et al., 2011), which target the V4-V9 region. Amplified DNA from the PCR reaction was purified with a Syngen DNA clean-up Kit and Sanger-sequenced using the same primers that were used for the PCR reaction, performed by the company Genomed S.A. (Poland).
Phylogenetic analysis
NCBI accession numbers for sequences from a previously published phylogeny (Jang et al., 2022) were used to download the corresponding sequences from NCBI. Additional blast searches against the NCBI database were performed using the 18S rRNA gene sequence that was obtained for Dactylochlamys pisciformis in the present study as a query to sample similar sequences. The compiled dataset including the newly obtained D. pisciformis sequence was aligned using mafft-linsi, trimmed using trimAl with -gappyout setting and the resulting alignment used to infer a phylogeny using the program IQ-TREE2 (Minh et al., 2020) with model testing performed using ModelFinder (Kalyaanamoorthy et al., 2017) and performing 1000 Ultrafast bootstraps. Genbank accession number for D. pisciformis in our study is OR119743.
Results and discussion
Ciliates of the genus Dactylochlamys
Amended diagnosis of the genus Dactylochlamys: Free-living anaerobic ciliates that thrive in anoxic freshwater sediments. Cell shape fusiform, sometimes with a posterior end drawn into a “tail-like” extension that can be retracted, resulting in an irregular or ovoid cell shape. Cell covered with longitudinal ribs slightly twisted from the anterior to the posterior end. Each rib bears retractile digitiform extensions that resemble spines due to the presence of an extrusome axis inside, but not all the digitiform extensions may have an extrusome. Uniform ciliature distributed over the surface of the cell, apical cytostome, and dorsal bross present. Cilia are very long and placed between the ribs, one cilium usually accompanying each finger-like extension; denser band of cilia encircle the oral region. Spherical macronucleus. One or more micronuclei present, but not always observable by light microscopy. Single contractile vacuole.
Type species of the genus: Dactylochlamys pisciformis
Dactylochlamys pisciformis Lauterborn, 1901
Cells 60 to 150 µm long, fusiform, with elongated retractable/extendable posterior “tail-like” extension (Figures 1, 2B). Eight to 15 ribs that spiral from the mouth to the posterior end. Ten to 15 finger-like retractable extensions on each ridge, pointing backwards (Figures 1, 2AC). Most but not all finger-like extensions have a single axial extrusome inside; those lacking the extrusome look like membrane foldings (Figures 1, 2C). Somatic cilia very long, resembling flagella, sparce, located between the finger-like extensions and placed at their inner side; not all the finger-like extensions are accompanied by a cilium. Cilia are denser around the oral area. Dorsal bross present. Globules are commonly present in the cytoplasm and in the finger-like extensions. Presence of prokaryotes in the ciliate cytoplasm. Free-living anaerobic ciliate that thrives in anoxic freshwater sediments.
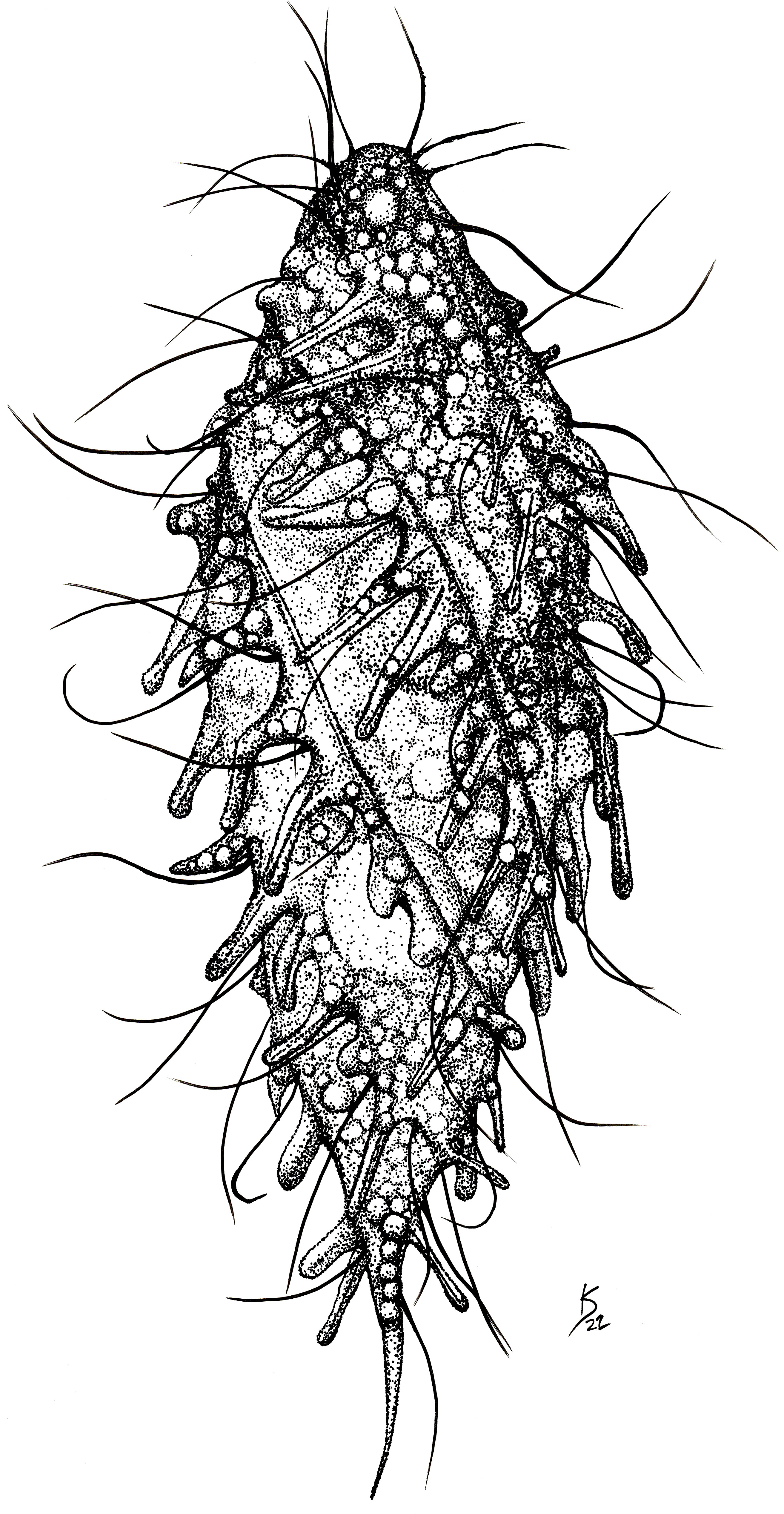
Figure 1 Line drawing of a living individual of the ciliate Dactylochlamys pisciformis. Cell size: 80 µm. Drawing by Katelyn Solbakk.
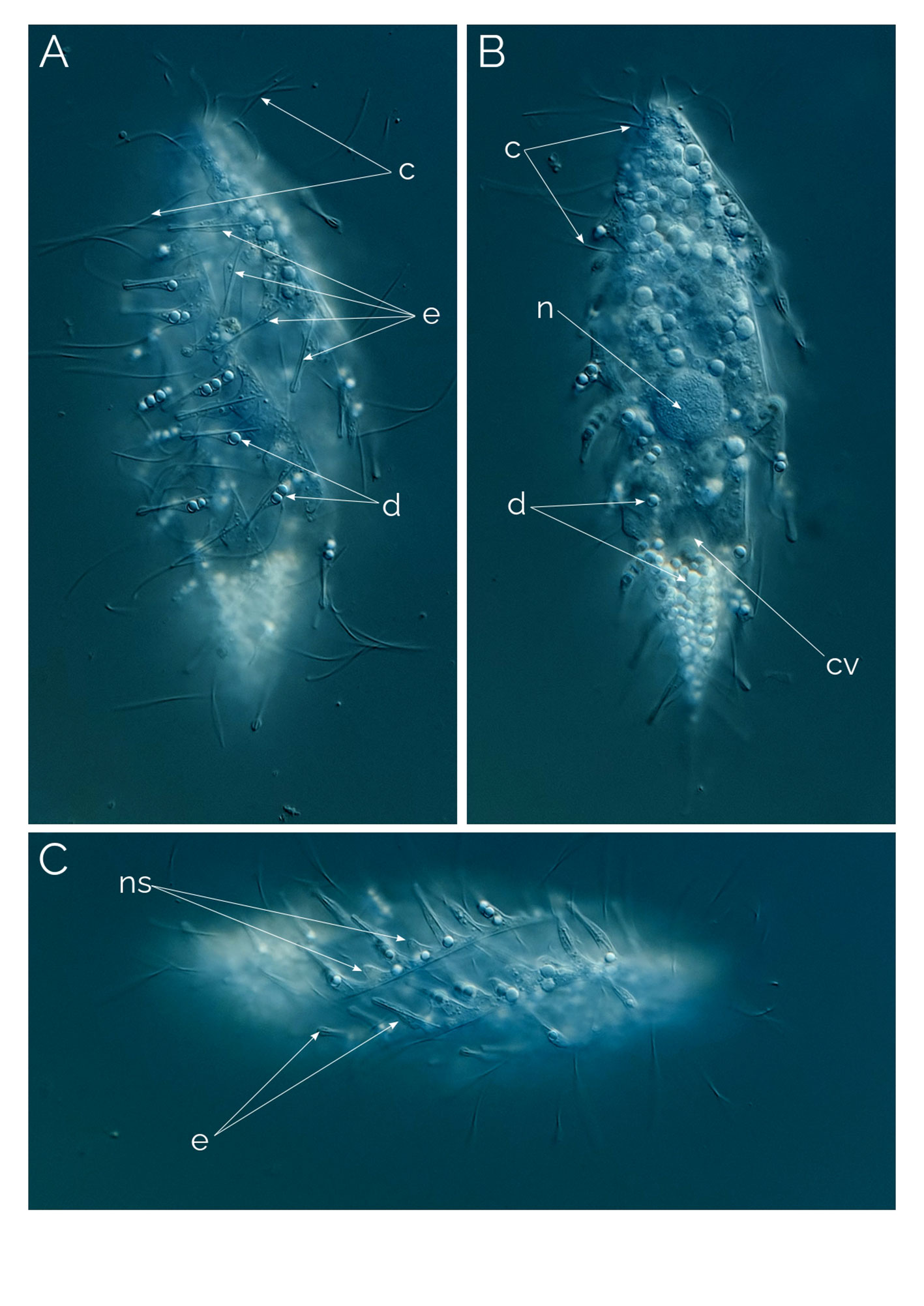
Figure 2 Dactylochlamys pisciformis. Differential Interference Contrast (DIC) images of living cells. (A) Cell showing the characteristic spiral ribs extending from the anterior to the posterior end of the cell, the finger-like extensions with the rod-shaped internal extrusome and the long cilia. (B) Same cell focussing on the cytoplasm and the periphery of the cell. (C) Spiral ribs with finger-like tentacles and the rod-shaped extrusomes; c, cilia; cv, contractile vacuole; e, rod-shaped extrusomes; d, droplets; n, macronucleus; ns, extrusome-less spines. Cell size: 80 µm.
In 1901 Lauterborn (1901) publishes “Die ‘sapropelische’ Lebeweit” (“The Sapropelic Life”), explaining this habitat and describing some species he observed, including the new genus Dactylochlamys. Lauterborn describes D. pisciformis as a new species of a newly erected genus with a brief description of the diagnostic characteristics without any drawings. He remarks on the finger-like structures that are pointing out from the cell surface and he mentions thread-like extrusomes within these finger-like structures, he also shortly mentioned the presence of oil/fat bodies within the cells, described the position of the contractile vacuole posteriorly. He mentions that he could not observe the cell mouth and finishes his article writing that he will be publishing a detailed analysis of Dactylochlamys’ systematic position. The full description comes seven years later, in 1908. Lauterborn publishes another article that includes another short text on Dactylochlamys. Lauterborn doesn’t provide more information about the morphology of Dactylochlamys however he mentions the cell mouth this time as a small depression at the anterior tip of the cell and shows a drawing of the ciliate (Figure 3D). He also speculates about Dactylochlamys being related to Colepina (Coleps). He writes that “Dactylochlamys is one of the most bizarre and striking infusoria I know of” emphasizing the confusing appearance of cells.
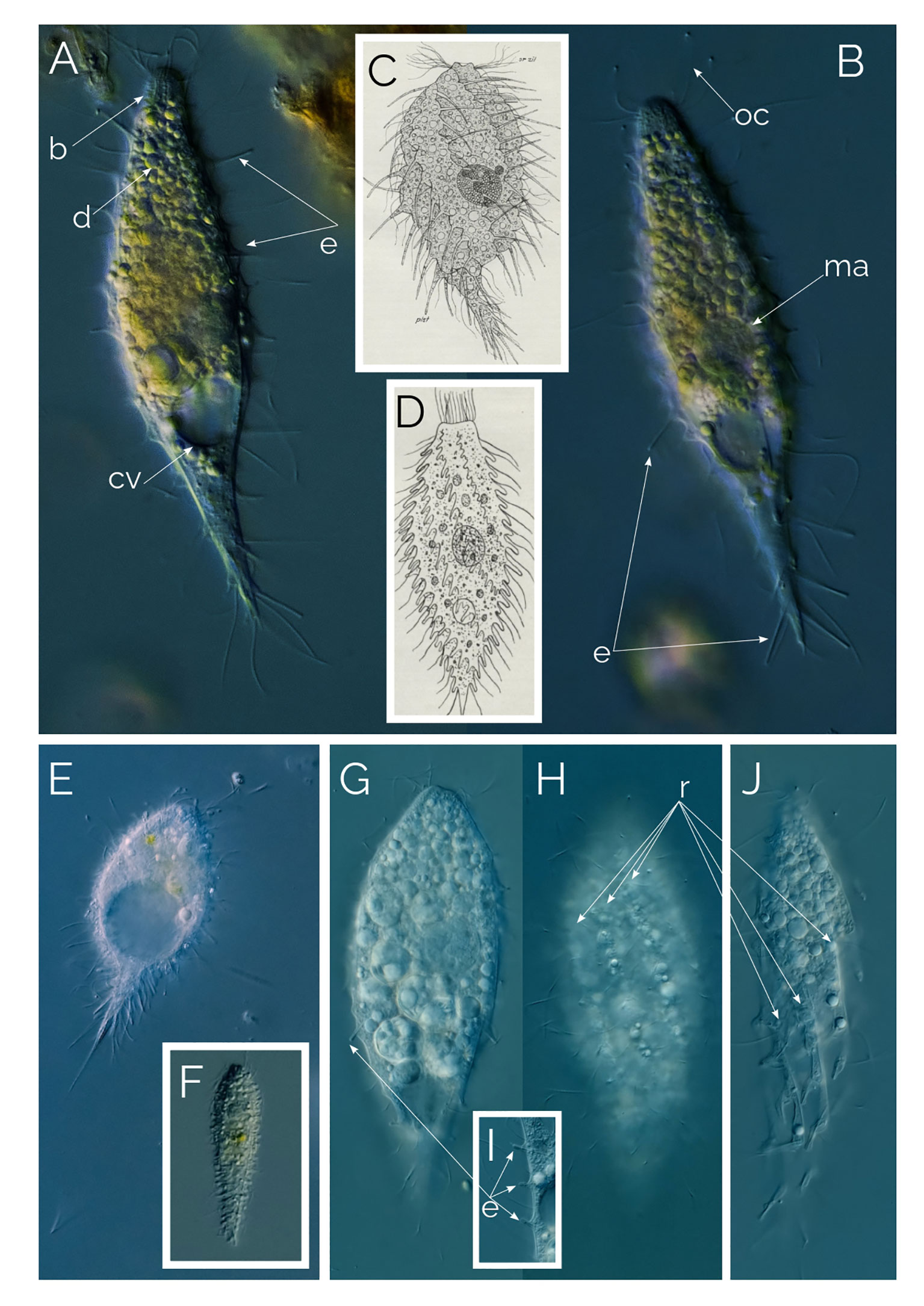
Figure 3 Different morphological variants of Dactylochlamys. Differential Interference Contrast (DIC) images of living cells. (A, B, J), type one. (E, F) type two. (G–I) type three (see text for full explanations); (C): D. hystrix (Wetzel, 1928); (D) D. pisciformis (Lauterborn, 1901); b, bross; cv, contractile vacuole; d, droplet; e, extrusome; ma, macronucleus; oc, oral cilia; r, ridge. Cell sizes: 65-100 µm.
In between Lauterborn’s, 1901 and 1908 publications, in 1906, Dactylochlamys’ name appears in literature in a French translation of a Russian monograph originally published in 1896. There is a single paragraph about Dactylochlamys, informing the reader that the curious genus could not be studied in sufficient detail by Lauterborn, and its systematic position is not yet determined exactly.
Next account of the genus comes from Penard in 1922. He publishes (Penard, 1922) and includes his observations on D. pisciformis. Penard however mentions the lack of the thread-like structures (extrusomes) inside the finger like structures which Lauterborn did mention, Penard mentions the cell mouth depression, but he adds with a frustrated tone that where there should be “lips” around the depression there was none to be seen. Penard also mentions that the shape of the cell can vary from individual to individual, and questions if there is a [type species] or not because of the differences between the individuals he found.
Dactylochlamys hystrix Wetzel, 1928
In 1928, Wetzel (1928) described a new species, D. hystrix, and compared it to D. pisciformis. He described both species as having similar spiralling “ribs” with inclusions in the cell, a single ellipsoidal macronucleus, and a single round micronucleus. D. hystrix differed from D. pisciformis in terms of cell shape and tentacle size. Wetzel described D. hystrix’s tentacles as with a stiffer core (“nail-like” in D. hystirx vs “finger like” in D. pisciformis) and with a broader extension. Additionally, the size of the posterior cell extension (or “tail”) was.
Our observations suggest that Dactylochlamys is a highly metabolic ciliate capable of altering its shape, rendering the length to width ratio irrelevant for species differentiation (Figures 3, 4). Wetzel (1928) observed the ingestion of rhodobacteria, a finding quoted by Kahl (1930). Pomahač et al. (2023) described D. pisciformis from Germany and the Czech Republic and, like Kahl (1930), considered D. hystrix and D. pisciformis to be conspecific, but did not provide justification for this decision. Future genetic analysis of more morphotypes of Dactylochlamys may help in better understanding both species.
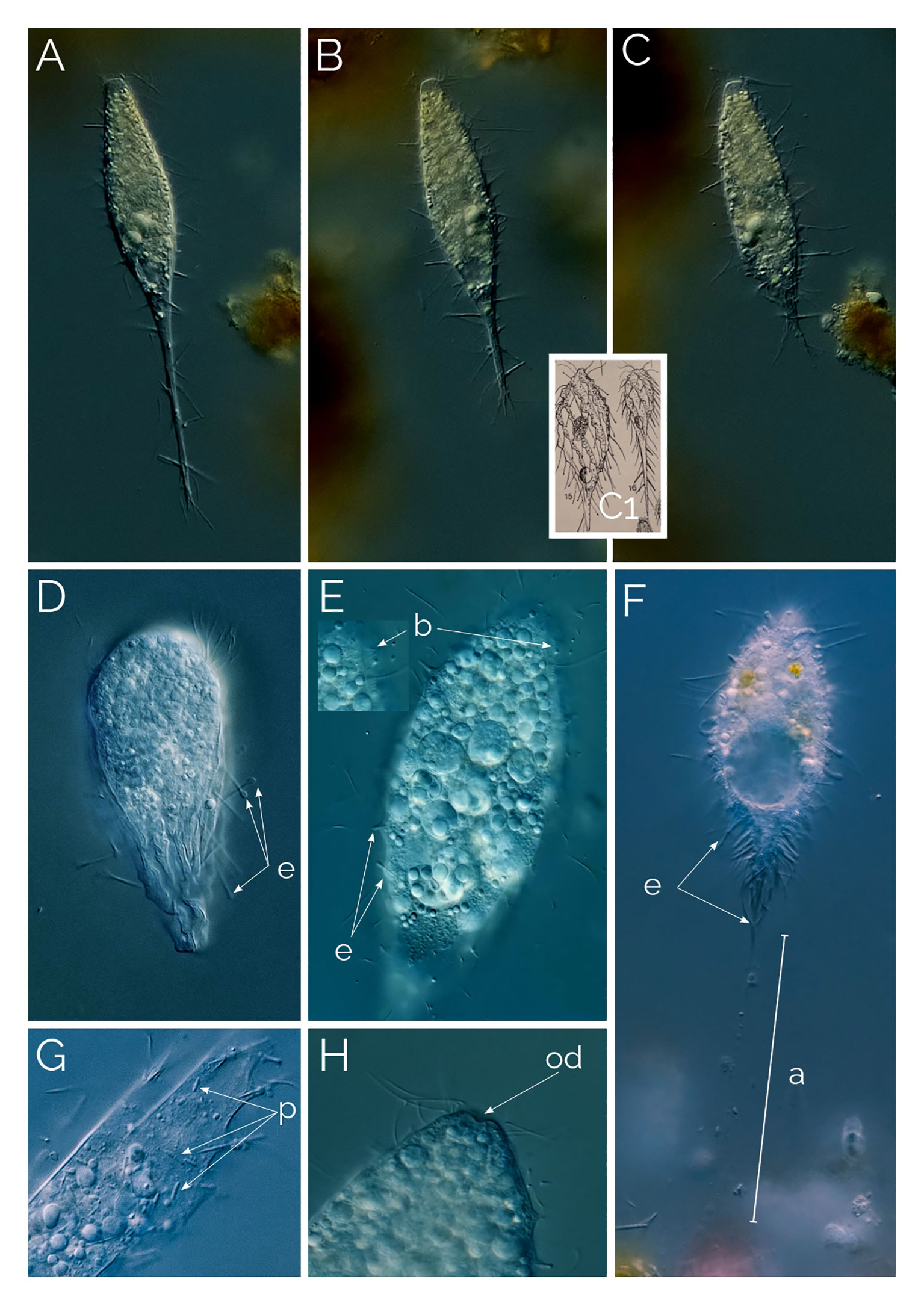
Figure 4 (A–C) cell contracting process in Dactylochlamys. Cell size in (A) from 120 µm to (C) 80 µm. Differential Interference Contrast (DIC) images of living cells. C1, drawing from Kahl, 1930; (D) Suctorial larva of Podophyra soliformis; (E) morphotype three of D. pisciformis (see text); (F) type two Dactylochlamys; a, anchor; b, bross; e, extrusomes; od, oral depression; p, prokaryotes. (G) Cytoplasm of Dactylochlamys pisciformis; (H) Anterior end of D. pisciformis.
Kahl (1930) classified Dactylochlamys under the family Actinobolinidae and was the first to observe its retractile tentacles, an observation we confirmed. In Figures 4A–C, the individual cell changed shape remarkably within ten minutes, as depicted in Kahl’s drawings in Figure 4C1. Kahl noted the absence of a cell mouth and suggested that Dactylochlamys might be a larval form of the suctorian Podophyra soliformis, but we disagree. In Figure 4D, we show that the larval stage of P. soliformis has fundamentally different tentacles from Dactylochlamys. Our molecular data also indicates no close genetic relationship with any suctorian groups available on GenBank. Kreutz and Foissner (2006) provided the first photographs of the genus and detailed morphological information on an internet blog, based on a population from the Simmelried ponds of Germany in 2000. Pomahač et al. (2023) described D. pisciformis populations from Germany and the Czech Republic that are very similar to ours even at the 18S rRNA gene level.
Our observations of Dactylochlamys
In every instance, we found Dactylochlamys in anoxic sediment accompanied by anaerobic ciliate genera, such as Metopus and Caenomorpha. At its peak of abundance, Dactylochlamys was present as one cell per ml. Cells vary in size from 60 to 150 µm, and individual cells can alter their general shape, as can be seen in Figures 4A–C a cell showing high plasticity within a 10-minute period and shortening its “tail” quite significantly. Our strains of Dactylochlamys cells have 8-15 stiff ridges that twist slightly from mouth to tail, on each one of the ridges there are ten to 15 finger-like extensions, that point toward the cell’s posterior end (Figures 1, 2A, C). The finger-like extensions look like spines and may have a single axial extrusome inside (Figure 2C), but some lack the extrusome and look like conspicuous membrane folding (Figure 2C), referred to as “teeth” by Penard (1922). This is also visible in Lauterborn’s drawing, his illustration only shows small spines on the ribs that slight twists on the cell (Figure 3D). Spines and extrusomes are covered with a very thin membrane, and extrusomes can be pushed a few micrometers further by stretching the loose membrane that covers them, which was first observed by Kahl (1930). When the extrusomes are extended, they appear stiffened and make the cell look like a pincushion (Figure 3B).
The cilia are very long relative to cilia of any other ciliates, measuring up to 20 µm and resembling flagella, a feature also mentioned by Lauterborn (1901; 1908) and Penard (1922). These cilia are sparsely distributed across the cell surface, located between the finger-like extensions and positioned at their inner side. Not all the finger-like extensions are accompanied by a cilium (Figure 2). The cilia are denser and longer around the oral area. (Figure 3B).
The observed Dactylochlamys cells contained numerous droplets, possibly fat or oil. Some cells concentrated these droplets at the posterior half (Figure 2B), while others did so at the anterior half (Figure 3A). In some specimens, the spines contained a few spherical droplets as well (Figure 2A). Endosymbiotic prokaryotes were observed within most of the cells, with Figure 4G pointing out the prokaryotes and the middle arrow indicating a dividing prokaryote. The bross is formed by two rows (Figure 3E). The single macronucleus is either spherical or slightly ellipsoid, accompanied by one micronucleus. Each cell had one single posterior contractile vacuole. The mouth depression mentioned by Penard (1922) can be seen in Figure 4H.
We found the first Dactylochlamys cell in April 2020, and since then, we have found three morphological variants in three different localities. All the variants can be seen in Figure 3. The first variant (Figures 3E, F), under low magnification, appears very much like Lauterborn’s illustration of D. pisciformis (Figures 3F vs D), the cells have numerous small tentacles, and under high magnification extrusomes within the tentacles became visible, but they are very easy to miss otherwise. This may explain why Lauterborn (1901) couldn’t see the extrusomes while Penard (1922) did. We observed a population of these cells staying sessile for hours, attached to the debris from their posterior end with a thin thread-like structure (Figure 4F). The cell used in Figure 2 was the isolate for the 18S rRNA gen analysis. The results can be found in Figure 5.
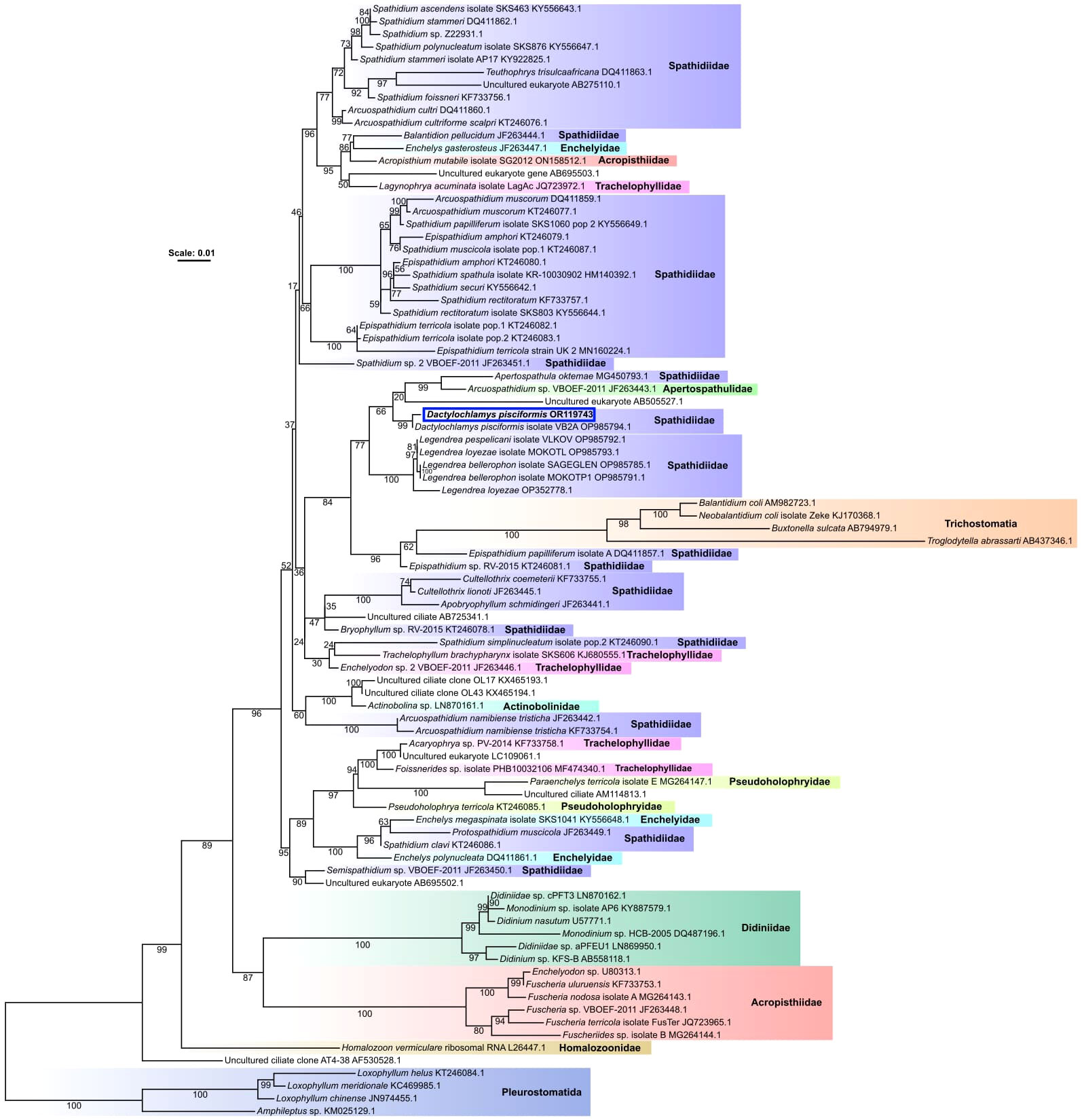
Figure 5 An unrooted species phylogeny of ciliates from several groups belonging to the class Litostomatea. The phylogeny was inferred from a trimmed nucleotide alignment of 18S rRNA genes comprised of 1459 sites using the model GTR+F+I+G4, chosen as the best fitting model according to the Bayesian Information Criterion by the program ModelFinder (Kalyaanamoorthy et al., 2017), implemented within IQ-TREE2 (Minh et al., 2020). Support values are percentages of 1000 ultrafast bootstrap replicates (Hoang et al., 2018), with well-supported branches having ≥ 95% ultrafast bootstrap support. Clades are labeled with family names according to the NCBI taxonomy database. Scale bar represents the number of substitutions per site.
The second variant shown in Figures 3G, H is a larger Dactylochlamys with very shallow spines on the ridge, instead of tentacles, the spines contain a single extrusome. In most cases, only the tip of the extrusome is visible, and the rest extends into the cytoplasm. The tip of the tentacles has a little knob-like membrane bulge in some cases (Figure 3I), which might be why Kahl’s (1930) drawings of Dactylochlamys appear with the knob at the tip of the tentacles (Figure 4C1). In Figure 3G, the focus plane slices the cell, and in Figure 3H, the focus plane is on the surface of the cell, showing the shallow spines.
The third cell variant is depicted in Figures 3A, B, J. The cells are slender with prominent ridges, and the tentacles extend out stiffly but can also be contracted (Figures 4A–C). When examined without a high-resolution microscope objective, this morphotype resembled D. hystrix, with stiffer nail-like tentacles and no extrusomes (as described by Wetzel, 1928). However, when checked with greater resolving power, thin extrusomes were visible inside the tentacles (Figure 3J).
Figures 1, 2, 3E, F depict the typical morphotype of Dactylochlamys pisciformis, as observed by various previous authors. Understanding the concept of species within this genus is particularly challenging for two main reasons. Firstly, the genus is exceptionally rare, posing difficulties for comprehensive study. Secondly, the morphology of Dactylochlamys presents issues due to its plasticity, leading to significant variations in cell shape within the same population. For instance, Figures 3G, H could represent a well-fed version of Figure 3J. As the cell expands the prominent rows of ridges seen in Figure 3J disappear. This study demonstrates the existence of morphologically distinct Dactylochlamys. However, we only have 18S rRNA evidence for the morphotype depicted in Figure 2, which has a 99% similarity to the strain described by Pomahač et al. (2023) (Figure 5).
Ciliates of the genus Penardiella
Penardiella undulata Kahl, 1930
The cell is oval and laterally flattened, with the anterior region slightly truncated (Figure 6). The prominent unciliated ridge travels around the perimeter of the cell and undulates on the ventral side of the ciliate (Figures 6B, D, F), but on the dorsal side the ridge makes an inward turn and does not connect with the mouth (Figures 6E, F). The oral aperture is situated toward the dorsal edge on the apical part of the ridge, and the oral area bears two types of extrusomes. Extrusomes line the ridge.
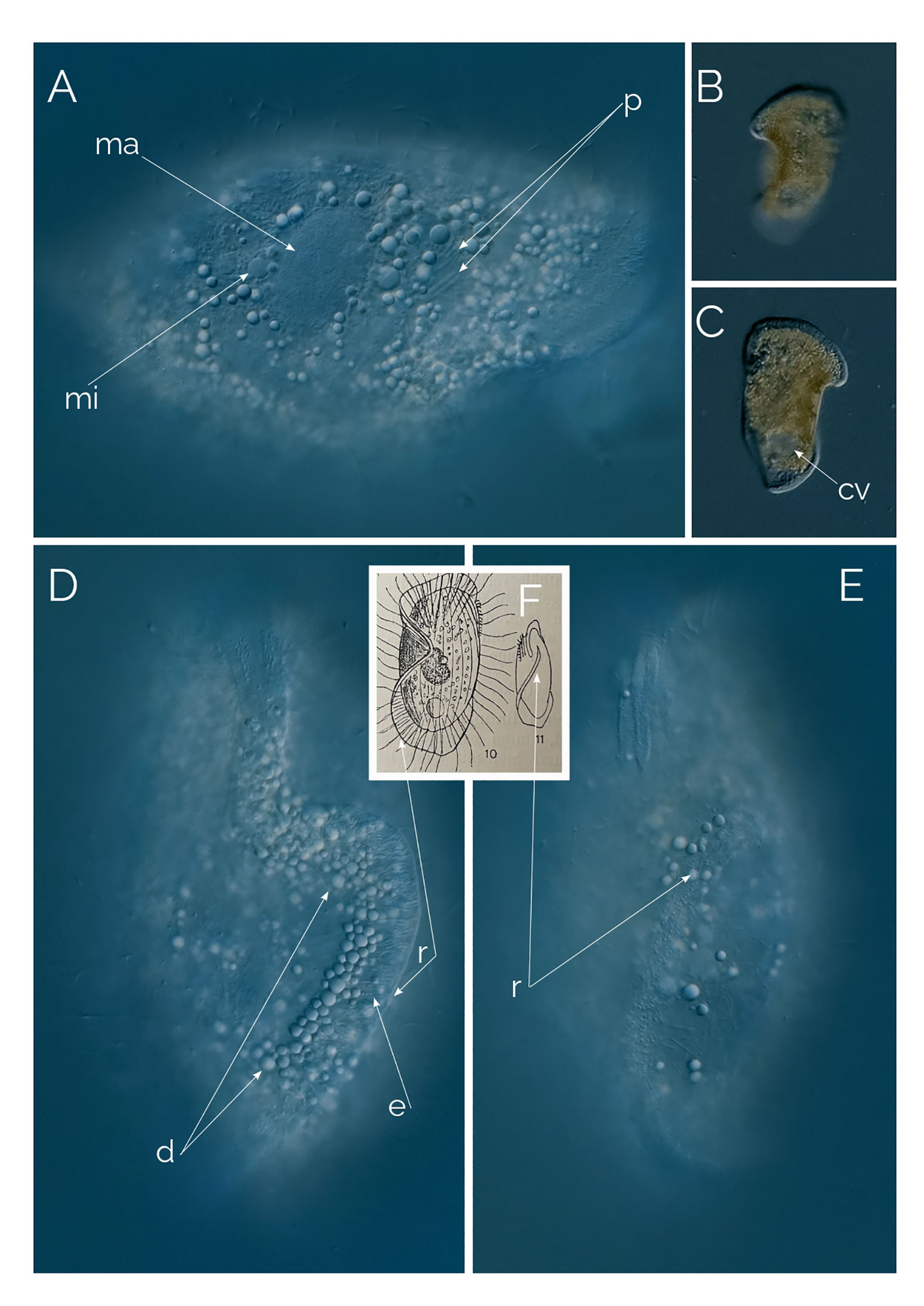
Figure 6 (A–E) Penardiella undulata. Differential Interference Contrast (DIC) images of living cells. (F) drawing from Kahl, 1930; cv, contractile vacuole; d, droplet; e: extrusome; ma, macronucleus; mi, micronucleus; p, prokaryotes; r, ridge. Cell size: 180 µm.
Kahl created the genus Penardiella in 1930 by moving two species of Legendrea described by Penard under the new genus, Penardiella crassa and Penardiella interrupta (Figure 7), and he described a new species he called P. undulata. There are very few records of Penardiella over the last 90 years or so. One of the three species of the genus, P. undulata, has never been reported since Kahl’s discovery in 1930. We found many individuals periodically from a single, small pond rich in decaying organic matter from Las Kabacki, where the ciliate was encountered more frequently during winter. P. undulata has a frill-like ridge, circling ventrally around the cell but instead of connecting, the ridge makes a subtle but characteristic inward turn. Kahl’s drawing and our microphotograph shows the cell from the dorsal side (Figure 6F). Kahl (1930) reported occasional finds of P. undulata and remarked on the lack of noticeable morphological variations. We agree with this observation, as all the individuals we encountered had the same ridge organization.
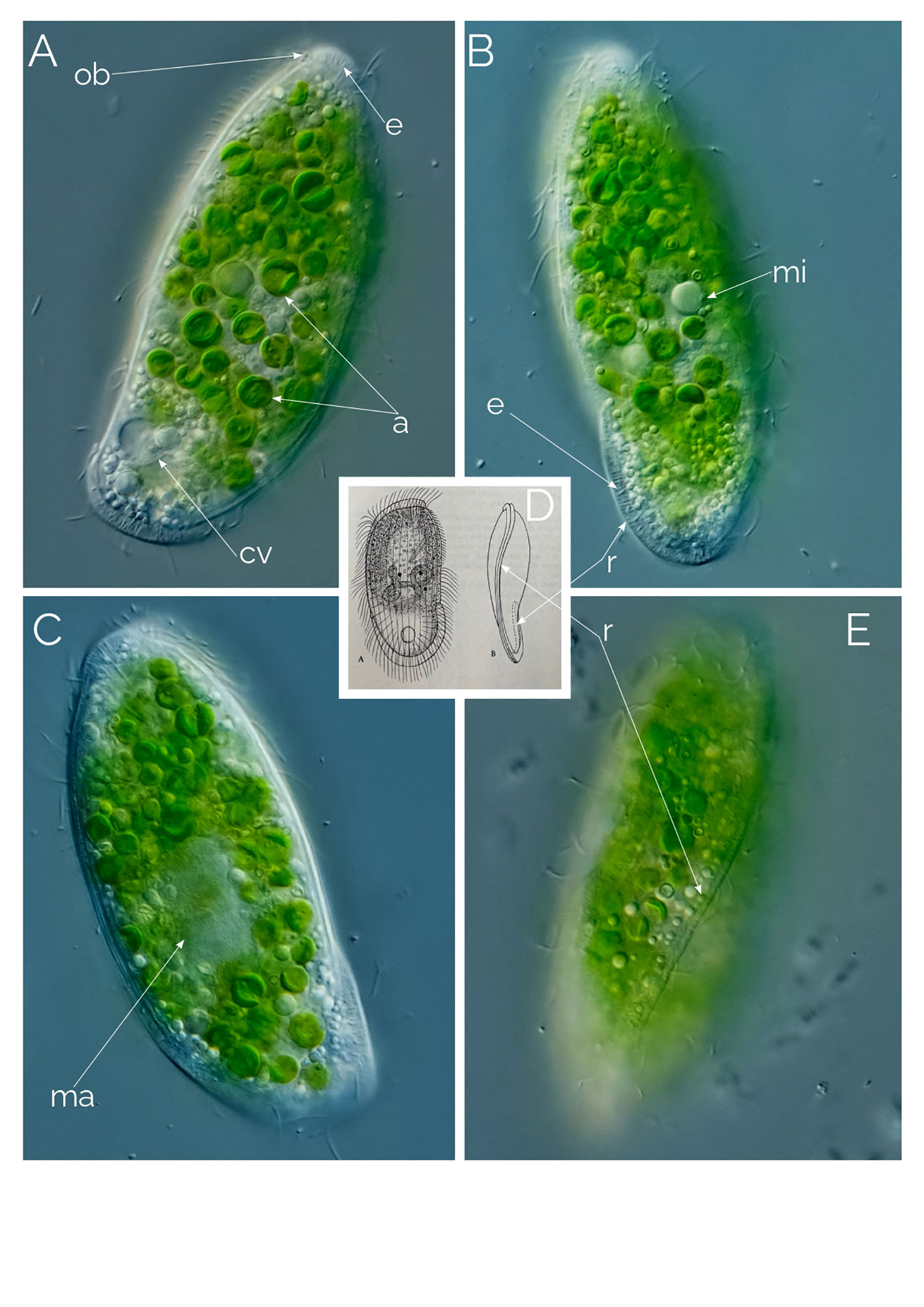
Figure 7 (A–C, E) Penardiella interrupta. Differential Interference Contrast (DIC) images of living cells; a, endosymbiotic algae; cv, contractile vacuole; e: extrusome; ma, macronucleus; mi, micronucleus(?); ob, oral bulge; r, ridge. (D) from Kahl (1930). Cell size: 90 µm.
We observed 10 individuals, always in the anoxic sediment. The size of the individuals ranged from 100 to 250 µm, always the same shape and orientation of the ridge. The macronucleus is ellipsoidal and accompanied by one single micronucleus (Figure 6A). The ridge is without cilia and bears long extrusomes (Figure 6D). The cytoplasm is full of refractive droplets, like the oil droplets from some anaerobic ciliates (Figure 6D).
Penardiella interrupta Kahl, 1930
This species has only been sighted once, by Kreutz in 2009 (personal website) as far as we are aware since Kahl (1930). We only found four cells of P. interrupta in Glinianki Zielonka (Poland). The cells are around 100 µm in length and have around 20 kineties (Figure 7). The mouth slit is followed by a curved line of extrusomes that semicircles the cell, running from the ventral side and ending at the middle of the dorsal side. The mouth bears two types of extrusomes, long and short. The macronucleus appears nodular (Figure 7C), and the bross has three rows. There is one single, small, posterior contractile vacuole. In our samples we always found P. interrupta with endosymbiotic algae (Figure 7).
Undescribed Penardiella sp.
We found this ciliate alongside P. interrupta in samples from Glinianki Zielonka (Poland). Just like other Penardiella species, the mouth is followed by a ridge of short extrusomes (Figure 8). We found 20 individuals altogether. The cell size is 120 µm and appears like an arrowhead with two blades as the extrusome ridges line almost perpendicular to the mouth slit (Figure 8C). Three types of extrusomes are present; two of them are associated with the mouth and the ridge, the other one is scattered in the cytoplasm (Figure 8F). The extrusomes in the mouth are longer than the ones that support the ridge (Figure 8G). The macronucleus is single and ellipsoidal in shape, the micronucleus was not observed. The ciliate swims quite fast, while rotating on the long axis.
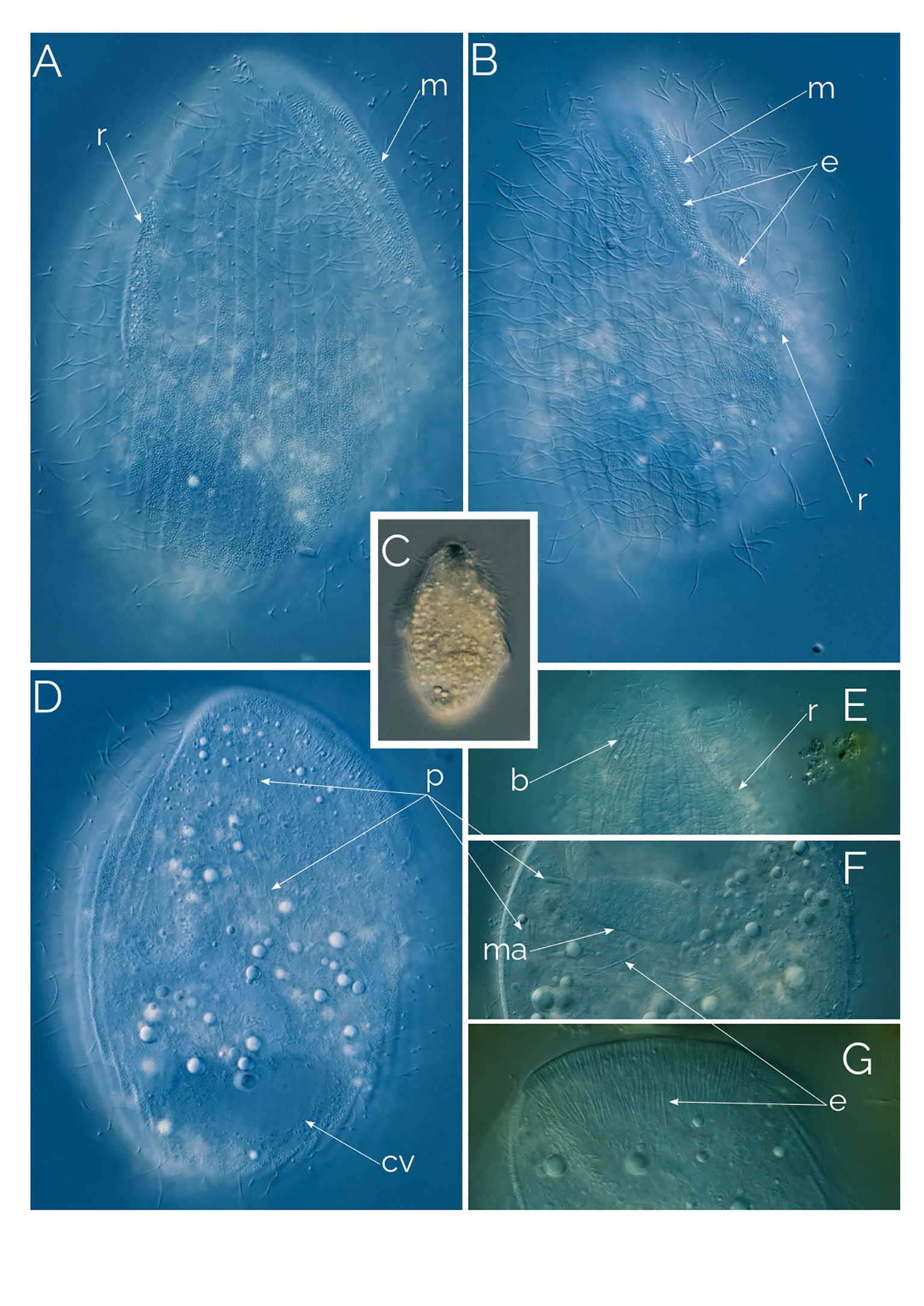
Figure 8 (A–G) Undescribed Penardiella. Differential Interference Contrast (DIC) images of living cells; b, bross; cv, contractile vacuole; e, extrusomes; m, mouth; ma: macronucleus; p, prokaryotes; r, ridge. Cell size: 120 µm.
Bryophyllum caudatum Gelei, 1933
Ciliates of this genus have a cell shape that is irregularly oval and laterally flattened. The ventral edge is convex on which the long slit-like oral aperture is situated. The oral bulge is a characteristic feature of the genus, occupying the entire cell length and curving around the posterior end. In Bryophyllum caudatum, the oral bulge continues beyond the posterior end of the cell, to coil around itself, forming a tail-like extension that varies in development (Figure 9; Gelei, 1933). The oral bulge is supported by long extrusomes in the oral area (Figure 9A). Contractile vacuoles are in bundles (Figures 9D, H). The macronucleus is elongated and ribbon-like (Figure 9H). As it was described by Gelei (1933), a mane-like cilia zone accompanies the “lip bulge” (Figures 9J, L as cz1). This zone consists of ciliary rows. The ciliary rows are more densely ciliated on the left side of the lip bulge, while the cilia rows on the right side consist of fewer cilia (Figure 9L). The ciliary rows on the right side run parallel to each other and end abruptly all on the same line, while the ciliary rows on the left spread evenly across the mouth and connect with the circumoral kinety (Figure 9L). Another ciliary zone is positioned dorsolaterally, this zone runs across the cell as three long ciliary rows and a single ciliary row which ends halfway through (Figures 9J, K as cz2).
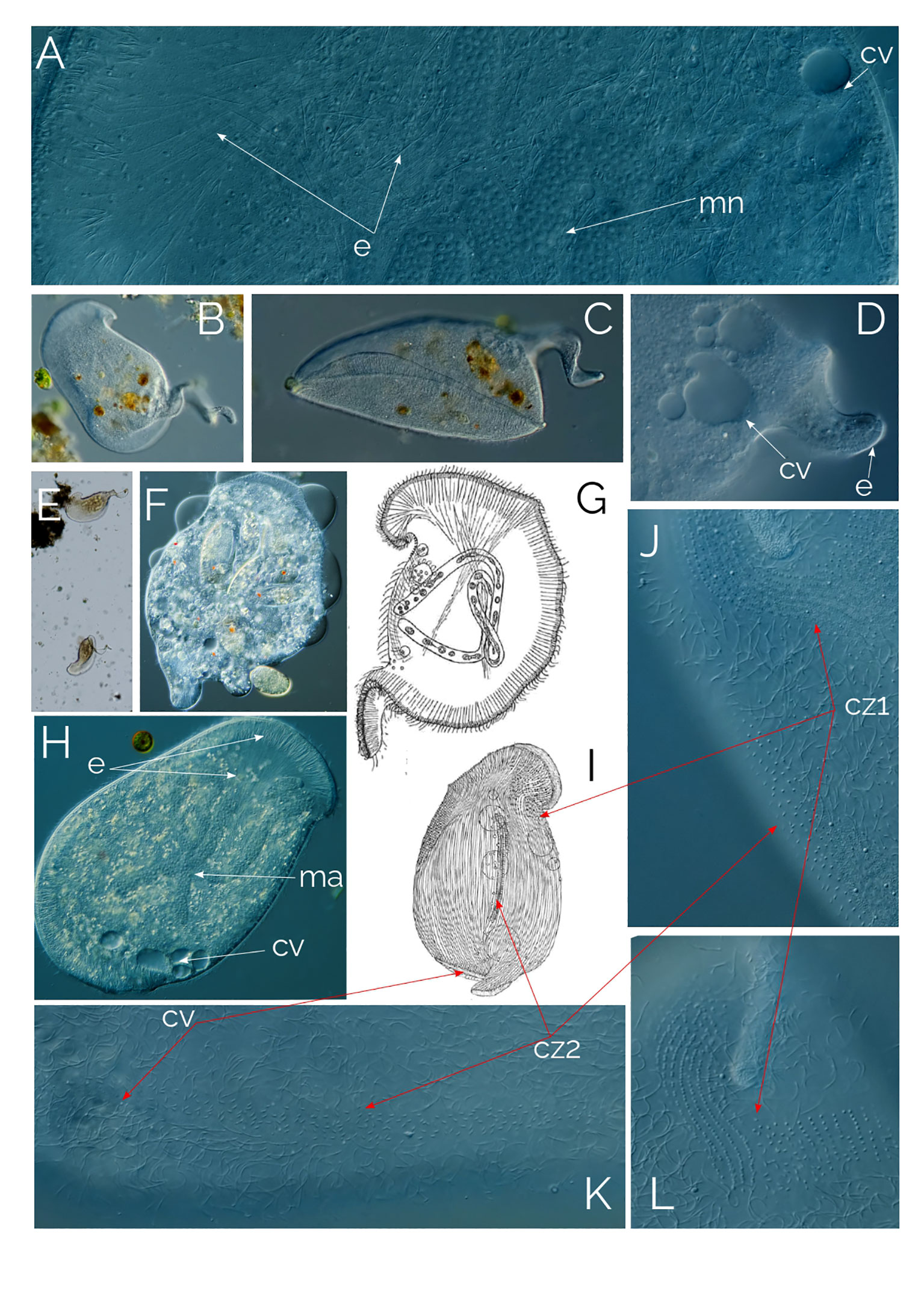
Figure 9 (A–F, H, J–L) Bryophyllum caudatum; cz1, ciliary zone #1; cz2, ciliary zone #2. cv, contractile vacuole; e, extrusomes; ma, macronucleus. (G) drawing from Gelei (1933), cell in vivo. (I) cell after “Sublimat-Silber-Gold” (Gelei, 1933). Cell size: 400 µm.
Bryophyllum caudatum has not been found since Gelei (1933), but over the past two years, we have occasionally found this tailed Bryophyllum in the water column of a pond in Las Kabacki (Poland), although with low abundance (one cell per ml). The ciliate is large, up to 500 µm, and the tail length ranges from a small bump on the posterior end to almost as long as the organism itself (Figures 9B–E). B. caudatum thrived in the sample containers after every sampling for some weeks, probably due to the plankton net samples concentrated in rotifers, which is the food source of the ciliate (Figure 9F). The mouth is slit-like, armed with long extrusomes that extend halfway into the cell (Figure 9H). Where the mouth slit ends, the shorter extrusome band continues to circle the cell until the opposite posterior end of the cell (Figure 9H). From here, the extrusome band continues to extend to form the coiled tail at the posterior end of the ciliate (Figure 9D).
We were able to observe the longer tailed individuals with a magnifying glass in our culture containers as Gelei had done 90 years ago (1933). The ciliates crawled on the sediment surface, on the container’s surface and upside down under the water-air surface. Gelei (1933) also explained that the ciliary rows on the right side of the lip bulge have fewer cilia compared to the left side because the ciliate crawls on the right side. We do not know what the function of the tail is; it might even be a “left over” after cell division. Figure 9G shows the ciliate in vivo, while Figure 9I shows the ciliate with “Sublimat-Silber-Gold” staining (Gelei, 1933). Figures 9K, L shows the ciliary zones in vivo.
Ciliates of the genus Apertospathula sp.
This ciliate, which we currently identifying as an undescribed species of the genus Apertospathula, is 150-200 µm in length and 60 µm in width, being 30 µm at the narrower posterior cell region in fusiform cells (Figure 10). At low magnification, the cell is fusiform, wider anteriorly (Figure 10G). However, the most remarkable characteristic of the ciliate is revealed only at high magnification. Under high magnification, it is apparent that the cell volume is filled with 2-3 µm-long prokaryotes, thousands of them packed in the ciliate’s cytoplasm (Figure 10). The ciliate macronucleus is ellipsoidal, accompanied by three micronuclei (Figure 10C). The ciliate has 10-16 somatic kineties (12 rows in Figure 10A) and a single posterior contractile vacuole. The ciliate has an incomplete oral bulge (Figure 10C), as described for the genus Apertospathula (Foissner et al., 2002). The bross is present and has three rows (Figure 10D). The ciliate is anaerobic and always found with anaerobic ciliate genera like Metopus, Caenomoprha and Brachonella. The ciliate is never abundant, usually as one cell per ml, when found.
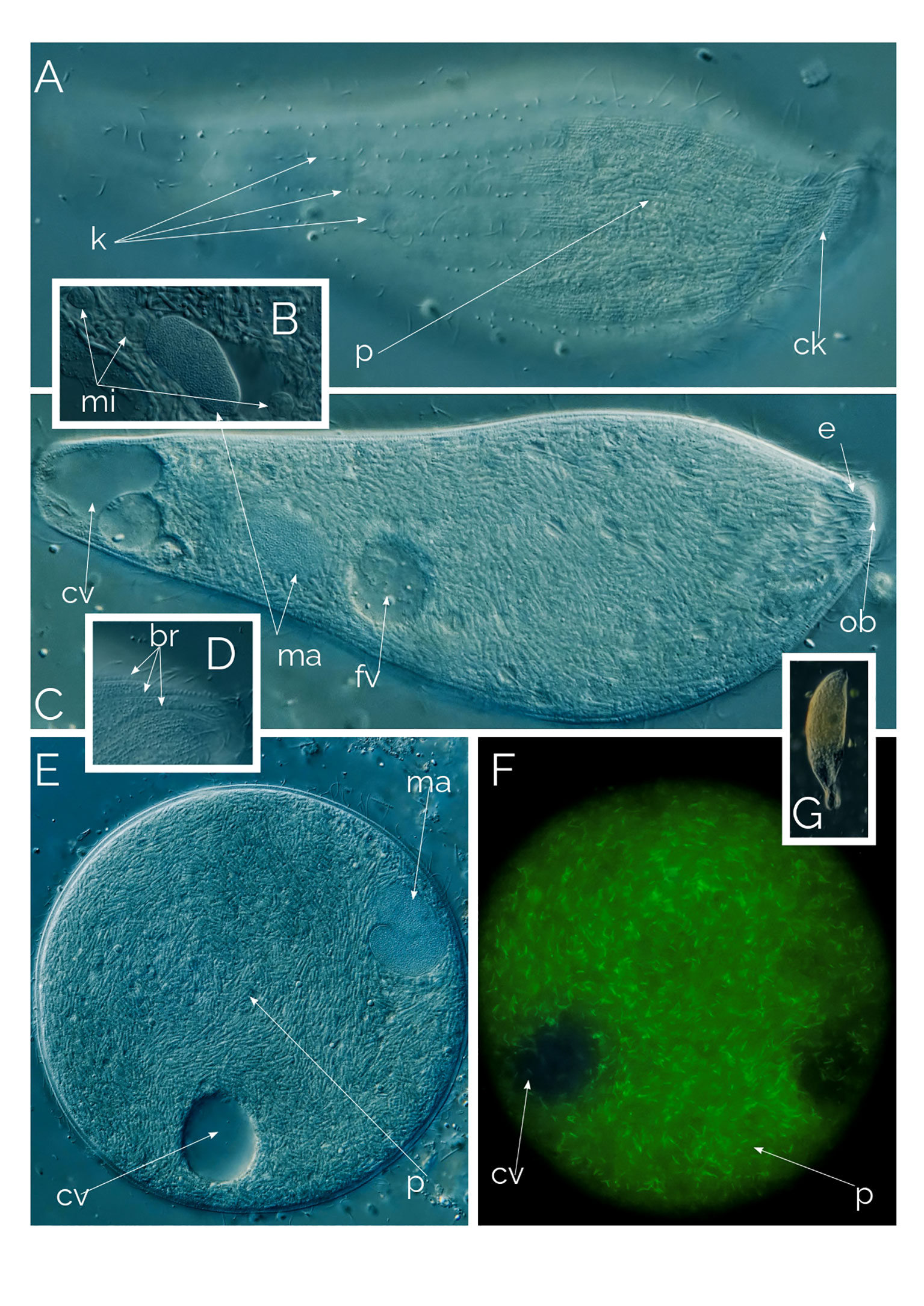
Figure 10 Undescribed Apertospathula. (A–D, G) Differential Interference Contrast (DIC) images of living cells; (E, F) cyst (F as seen under autofluorescence); br, bross; ck, circumoral kinety; cv, contractile vacuole; fv, food vacuole; e, extrusomes; k, somatic kineties; ma, macronucleus; mi, micronucleus; ob, oral bulge; p, prokaryotes. Cell size: 170 µm.
We came across many cysts accompanied by active individuals; the cysts were easy to recognize because of the endosymbiotic prokaryotes (Figure 10E). Under UV excitation, the cysts lit up with autofluorescence of the prokaryotes (Figures 9, 10F) and autofluorescence is like methanogenic archaea’s (Esteban and Fenchel, 2020). Interestingly the cysts show a high degree of cytoplasmic streaming, the cytoplasm of the cyst continuously swirls, the prokaryotes slide on each other. In cases of colpodid ciliate cysts (unpub. results), the cytoplasmic streaming only becomes noticeable when the ciliate starts to prepare for excystment but in Apertospathula’s case cysts were swirling for days without excysting. We also observed a similar motion with inactive (but not encysted) Legendrea bellerophon and L. loyezae, both ciliates carry endosymbiotic prokaryotes (see below and Weiss et al., 2022). We speculate that the motion of the cytoplasm may be triggered by the nutrient and resource needs of the prokaryotic endosymbionts.
Apertospathula are predatory raptorial ciliates, that feed not just on other protists but also on neighboring metazoa (Foissner et al., 2002). In our Apertospathula species, we have not seen a food vacuole with any recognizable content, which may indicate that the endosymbiotic prokaryotes are meeting the metabolic demands of the host (Fenchel and Finlay, 1991; Fenchel and Finlay, 1995).
Legendrea bellerophon Penard, 1914
These ciliates, like the closely related Legendrea loyezae (Weiss et al., 2022), have two distinct morphologies: one that is motile and another that is motionless. The cell size is 100 to 180 µm. The motile (free-swimming) ciliate is oval, with protruding papillae (Figure 11) that contain extrusomes. The motionless form is stationary and is the feeding stage of the ciliate; the cell settles on sediment or debris, flattened in shape, and starts stretching its papillae to develop long thin tentacles very similar to those of L. loyezae (Weiss et al., 2022). The tentacles extend slowly until they are fully stretched and the ciliate stays like that waiting for prey to collide with them (Figures 11H, J). For the motile phase, the cell contracts the tentacles and swims while rotating on its long axis (Figures 11A–C, I). When the ciliate extends the tentacles, it takes a spherical shape as small as 100 µm; however, when the cell is in the swimming state, it can be as large as 300 µm. There is one posterior contractile vacuole (Figure 11J). The cytostome is apical and bross has three rows (Figure 11D). Macronucleus is sausage shaped (Figure 11A). L. bellerophon is anaerobic and thrives in anoxic freshwater sediments.
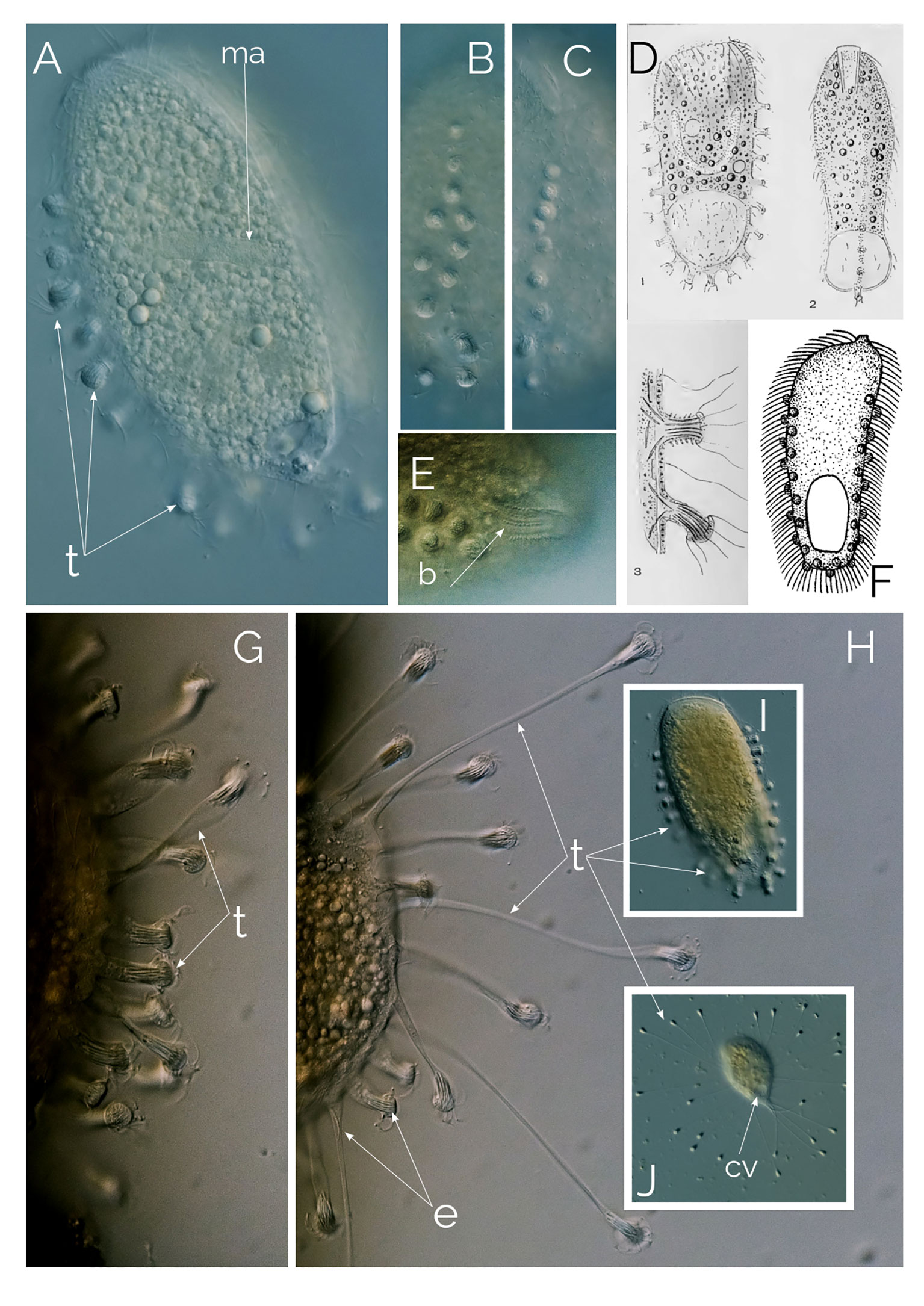
Figure 11 Legendrea bellerophon. Differential Interference Contrast (DIC) images of living cells; (A–C, I) motile, free-swimming stage with contracted tentacles. (D) from Penard (1914). (E): showing dorsal bross. (F): Holophrya ornata from (Stokes, 1887). (G, H, J): motionless stage showing the extended tentacles; b, bross; cv, contractile vacuole; ma, macronucleus; t, tentacles. Cell size: The swimming ciliate is up to 300 µm; the motionless, sedentary ciliate is 100-180 µm, excluding the extended tentacles.
L. bellerophon is a very rare ciliate (we found six cells in two years), which has only been reported a handful of times, the most comprehensive description of the species is from Penard (1914). After discovering this ciliate, Penard diligently examined the species, reporting his observations in a 27-page-long paper, providing thorough detailed descriptions, showing different forms of the ciliate, size, and shape of the extrusomes (Penard, 1914), accompanied with excellent illustrations (Figure 11D).
A recent paper by Pomahač et al. (2023) states that the ciliate described by Penard (1914) was first published by Stokes (1887) as Holophrya ornata based on an inconclusive illustration (Figure 11F). Based on this single assumption the authors renamed L. bellerophon as Legendrea ornata. Unfortunately, Stokes (1887) did not describe the characteristic contractile tentacles of L. bellerophon, the cell size of H. ornata is half that of L. bellerophon, and the oral bulge of H. ornata is entirely different from that of L. bellerophon. Furthermore, while Stokes (1887) described the extrusome bundles of H. ornata as subtle, they are very visible and distinctive in L. bellerophon (Figure 11A), and the extrusome bundles do not necessarily zigzag as in H. ornata’s drawing from Stokes (1887) (Figure 11F). Kahl (1930) considered Holophrya ornata Stokes, 1887 a potential parasite. With all this evidence, it is inadequate to synonymize L. bellerophon with Holophrya ornata due to the absence of descriptive material for H. ornata Stokes, 1887. Any comparisons with it can only be hypothetical.
Legendrea sp.
We found a ciliate similar to, but distinct from, Legendrea pes pelicanis Penard, 1922 (Figure 12C). The ciliate was 250-300 µm long and had an abundance of 1cell/ml, when present in the samples. The ciliate has pimple-like extrusome bundles that are typical of the genus Legendrea. However, unlike other Legendrea species such as L. bellerophon and L. loyezae, the extrusome bundles do not extend to develop into tentacles. Instead, they remain as pimples that encircle the entire perimeter of the ciliate’s cell (Figures 12A, B).
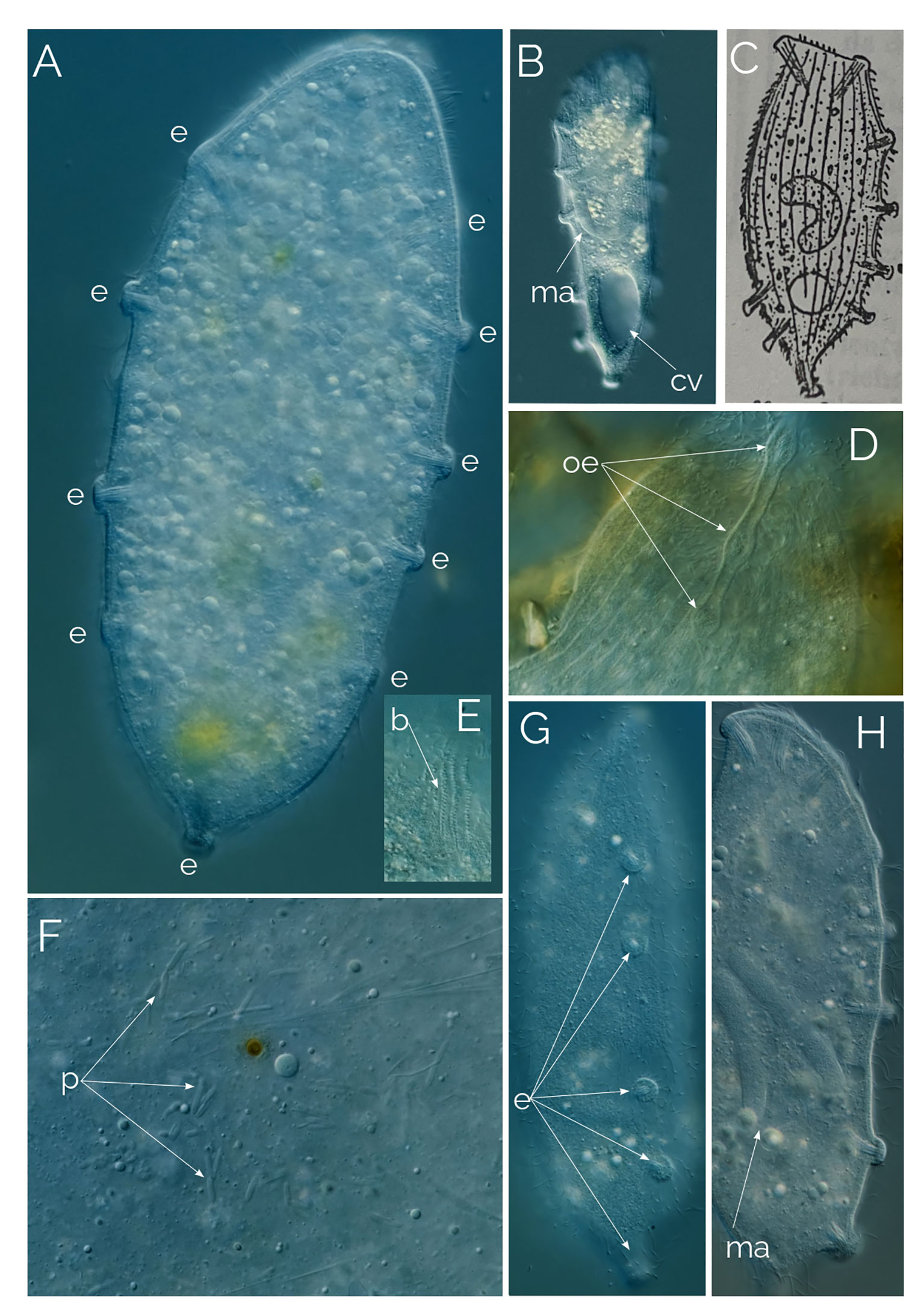
Figure 12 Undescribed Legendrea. Differential Interference Contrast (DIC) images of living cells. Cell size: 280 µm. (A, B) extrusome pimples surrounding the entire perimeter of the cell. (C) Legendrea pes pelicanis from Penard (1922); b: bross; cv, contractile vacuole; e: extrusome bundles; ma, macronucleus; oe, oral extrusome bundles; p, prokaryotes. (D) close up image of the oral extrusome bundles; (E) Bross; (F) Cytoplasm with endosymbiotic prokaryotes; (G) Side view of the cell showing five extrusome bundles; (H) Extrusome bundles (or pimples) located along the ventral side of the cell.
In 2023, Pomahač et al. (2023) also described a ciliate that has extrusome pimples around the entire perimeter of the cell and identified it as L. pespelicanis Penard, 1922. However, in Penard’s L. pes pelicanis (Penard, 1922), the pimple-like extrusome bundles cover only two-thirds of the cell perimeter, which is why it is called “pes pelicanis” as it resembles the talon-less part of the pelican foot (Figure 12C). L. pespelicanis in Pomahač et al. (2023) closely resembles our Legendrea sp. Therefore, we consider both ciliates to be conspecific and a distinct species from L. pes pelicanis.
What makes some ciliates rare?
The detection and isolation of the ciliates described in the present study required long hours of careful microscopic investigation of pond sapropel over the course of two years, during which only a few individual cells were acquired in most instances. Looking for these rare ciliates is extremely challenging, considering the volume of freshwater that had to be investigated to find those cells. Since cell division is the only route to increase the number of individuals in a ciliate population (Long and Zufall, 2010; Boscaro and Keeling, 2023), the rarity of a microbe must correlate with its growth rate and the factors that limit its growth. Anaerobic ciliates that inhabit the sapropel have a lower growth efficiency than their aerobic counterparts, with only 10% of energy gained from their food, compared to 40% in aerobic ciliates (Fenchel and Finlay, 1995; Fenchel and Finlay, 2018). Although most anaerobic ciliates feed exclusively on the abundant prokaryotes that thrive in the anoxic sediments, some predatory anaerobic ciliates have more complex feeding behaviors that impact their population size and, consequently, their rarity. One such trait is food selection and the response to famine conditions caused by food depletion (Fenchel and Finlay, 2018; Esteban and Fenchel, 2020).
Predatory raptorial ciliates capture individual prey and rely on food resources that are patchy and less abundant compared to filter-feeding (bacterivorous) ciliates. Aerobic raptorial ciliates adapt to patchy food resources by actively seeking out their prey, increasing the likelihood of finding it (Esteban and Fenchel, 2020). In contrast, many anaerobic ciliates, with low energy metabolisms, have adapted to the patchiness of food resources by developing a motionless, energy-conserving stage. One such ciliate, Legendrea bellerophon, remains motionless for extended periods, waiting for prey to collide with its extendable tentacles. It feeds almost exclusively on microscopic metazoa, such as rotifers and gastrotrichs (Penard, 1914; Pomahač et al., 2023) that occasionally descend from the oxic layers. These ciliates can survive for several weeks without cell division or food (unpublished observations), which may facilitate the eventual encounter with rare prey and therefore ensure the ciliates’ long-term survival. Our hypothesis is that the anaerobic metabolism of these ciliates, combined with their selective feeding habits, explains their rarity. Developing novel methods to enrich for and ideally also culture rare microbes could improve our ability to study them and therefore lead to a far greater understanding of their biology (Lewis et al., 2021).
Several other factors may also contribute to the rarity of ciliates (Weisse, 2014). Evolutionary, historical and ecological processes may limit their development potential, confining them to specific environments (Foissner et al., 2007; Hines et al., 2016). Niche conservatism, which suggests that species distribution patterns are influenced by ancestral climatic affinities, has also been proposed as a growth limiting factor for some soil protists (Fernández et al., 2022). However, there is insufficient published data on this concept for ciliates, making it an interesting area for future research. The rare ciliates presented in this study are not confined to the geographical locations where we found them.
Data availability statement
The datasets presented in this study can be found in online repositories. The names of the repository/repositories and accession number(s) can be found in the article/supplementary material.
Author contributions
JW: Conceptualization, Writing – original draft, Writing – review & editing, Methodology. GE: Conceptualization, Writing – original draft, Writing – review & editing, Supervision, Validation.
Funding
The author(s) declare that no financial support was received for the research, authorship, and/or publication of this article.
Acknowledgments
We are grateful to Katelyn Solbakk for making the illustration shown in Figure 1 and to Ela Gaworska for sample collection. We are grateful to Anna Karnkowska and Marta Sałek, Institute of Evolutionary Biology, Faculty of Biology, University of Warsaw (Poland), for kindly offering to do the molecular work and provide us with the 18S rRNA sequencing. We are also thankful to William Lewis, The Waller Lab, Department of Biochemistry, Hopkins Building, University of Cambridge (UK) for very helpful discussions on the first draft of the manuscript and for providing Figure 5.
Conflict of interest
The authors declare that the research was conducted in the absence of any commercial or financial relationships that could be construed as a potential conflict of interest.
The author(s) declared that they were an editorial board member of Frontiers, at the time of submission. This had no impact on the peer review process and the final decision.
Publisher’s note
All claims expressed in this article are solely those of the authors and do not necessarily represent those of their affiliated organizations, or those of the publisher, the editors and the reviewers. Any product that may be evaluated in this article, or claim that may be made by its manufacturer, is not guaranteed or endorsed by the publisher.
References
Boscaro V., Keeling P. J. (2023). How ciliates got their nuclei. PNAS 120, e222181812. doi: 10.1073/pnas.2221818120
Dunthorn M., Stoeck T., Clamp J., Warren A., Frédéric Mahé F. (2014). Ciliates and the rare biosphere: A review. J. Euk. Microbiol. 61, 404–409. doi: 10.1111/jeu.12121
Edgcomb V., Orsi W., Bunge J., Jeon S., Christen R., Leslin C., et al (2011). Protistan microbial observatory in the Cariaco Basin, Caribbean. I. Pyrosequencing vs Sanger insights into species richness. ISME J. 5 (8), 1344–1356. doi: 10.1038/ismej.2011.6
Esteban G. F., Fenchel T. M. (2020). Ecology of Protozoa. The Biology of Free-living Phagotrophic Ciliates. 2nd ed (Switzerland: Springer Nature), 186 pp.
Esteban G. F., Finlay B. J. (2003). Cryptic freshwater ciliates in a hypersaline lagoon. Protist 154, 411–418. doi: 10.1078/143446103322454149
Fenchel T., Esteban G. F., Finlay B. J. (1997). Local versus global diversity of microorganisms: Cryptic diversity of ciliated protozoa. Oikos 80, 220–225. doi: 10.2307/3546589
Fenchel T., Finlay B. J. (1995). Ecology and evolution in anoxic worlds. Oxford Series in Ecology and Evolution (Oxford: Oxford University Press), pp 276.
Fenchel T., Finlay B. J. (2018). “Free-living protozoa with endosymbiotic methanogens,” in (Endo)symbiotic methanogenic archaea, 2nd edition. Ed. Hackstein J. H. P. (Oxford University Press: Springer), pp 1–pp12.
Fenchel T. M., Finlay B. J. (1991). Synchronous division of an endosymbiotic methanogenic bacterium in the anaerobic ciliate Plagiopyla frontata Kahl. J. Eukaryotic Microbiol. 38, 22–28. doi: 10.1111/j.1550-7408.1991.tb04790.x
Fernández L. D., Seppey C. V. W., Singer D., Fournier B., Tatti D., Mitchell E. A. D., et al. (2022). Niche conservatism drives the elevational diversity gradient in major groups of free-living soil unicellular eukaryotes. Microb. Ecol. 83, 459–469. doi: 10.1007/s00248-021-01771-2
Finlay B. J., Corliss J. O., Esteban G., Fenchel T. (1996). Biodiversity at the microbial level: The number of free-living ciliates in the biosphere. Q. Rev. Biol. 71, 221–237. doi: 10.1086/419370
Finlay B. J., Esteban G. F., Fenchel T. (1998). Protozoan diversity: converging estimates of the global number of free-living ciliate species. Protist 149, 29–37. doi: 10.1016/S1434-4610(98)70007-0
Foissner W., Agatha S., Berger H. (2002). Soil Ciliates (Protozoa, Ciliophora) from Namibia (Southwest Africa), with emphasis on two contrasting environments, the Etosha Region and the Namib Desert. Denisia 5, 1–1080.
Foissner W., Chao A., Katz L. A. (2007). “Diversity and geographic distribution of ciliates (Protista: Ciliophora),” in Protist Diversity and Geographical Distribution. Topics in Biodiversity and Conservation, vol. vol 8. Eds. Foissner W., Hawksworth D. L. (Dordrecht: Springer).
Galotti A., Finlay B. J., Francisco Jiménez-Gómez F., Guerrero F., Esteban G. F. (2014). Most ciliated protozoa in extreme environments are cryptic in the seed-bank. Aquat. Microb. Ecol. 72, 187–193. doi: 10.3354/ame01699
Gelei J. V. (1933). Ciliatenfauna der Umgebung von Szeged II, Bryophyllum. Archiv für Protistenkunde 81, 201–230.
Hines H. N., McCarthy P. J., Esteban G. F. (2016). The first record for the Americas of Loxodes rex, a flagship ciliate with an alleged restricted biogeography. AMicrob. Ecol. 71 (1), 5–8. doi: 10.1007/s00248-015-0656-x
Hoang D. T., Chernomor O., Von Haeseler A., Minh B. Q., Vinh L. S. (2018). UFBoot2: improving the ultrafast bootstrap approximation. Mol. Biol. Evol. 35, 518–522. doi: 10.1093/molbev/msx281
Jang S. W., Omar A., Nam S. W., Jung J.-H. (2022). Morphology and Molecular Phylogeny of Fuscheriides baugilensis sp. nov. (Protozoa, Ciliophora, Haptorida) from South Korea. Diversity 14, 70. doi: 10.3390/d14020070
Jousset A., Bienhold C., Chatzinotas A., Gallien L., Gobet A., Kurm V., et al. (2017). Where less may be more: how the rare biosphere pulls ecosystems strings. ISME J. 11, 853–862. doi: 10.1038/ismej.2016.174
Kahl A. (1930). Urtiere oder Protozoa. I: Wimpertiere oder Ciliata (Infusoria). Eine Bearbeitung der freilebenden und ectocommensalen Infusorien der Erde, unter Ausschluss der marinen Tintinnidae. Vier Teile. Allgemeiner Teil und Prostomata. Ed. Dahl F. (Jena: Die Tierwelt Deutschlands und der angrenzenden Meeresteile, Gustav Fischer).
Kalyaanamoorthy S., Minh B. Q., Wong T. K., Von Haeseler A., Jermiin L. S. (2017). ModelFinder: fast model selection for accurate phylogenetic estimates. Nat. Methods 14 (6), 587–589. doi: 10.1038/nmeth.4285
Kreutz M., Foissner W. (2006). The Sphagnum ponds of Simmelried in Germany: a biodiversity hot-spot for microscopic organisms. Protozoological Monogr. 3, 1–267.
Lauterborn R. (1908). Protozoen-Studien. V. Teil. Zur Kenntnis einiger Rhizopoden und Infusorien aus dem Gebietedes Oberrheins. (Zeitschrift für wissenschaftliche Zoologie), 90, 645–669.
Lewis W. H., Tahon G., Geesink P., Sousa D. Z., Ettema T. J. (2021). Innovations to culturing the uncultured microbial majority. Nat. Rev. Microbiol. 19, 225–240. doi: 10.1038/s41579-020-00458-8
Long H., Zufall R. A. (2010). Diverse modes of reproduction in the marine free-living ciliate Glauconema trihymene. BMC Microbiol. 10, 108. doi: 10.1186/1471-2180-10-108
Lynch M., Neufeld J. (2015). Ecology and exploration of the rare biosphere. Nat. Rev. Microbiol. 13, 217–229. doi: 10.1038/nrmicro3400
May R. M. (1988). How many species are there on Earth? Science 241, 1441–1449. doi: 10.1126/science.241.4872.1441
Minh B. Q., Schmidt H. A., Chernomor O., Schrempf D., Woodhams M. D., Von Haeseler A., et al. (2020). IQ-TREE2: new models and efficient methods for phylogenetic inference in the genomic era. Mol. Biol. Evol. 37 (5), 1530–1534. doi: 10.1093/molbev/msaa015
Penard E. (1914). Un curieux Infusoire Legendrea bellerophon. Rev. Suisse Zool. 22, 407–432. (Plates of drawings are found at the end of the volume).
Pomahač O., Méndez-Sánchez D., Poláková K., Müller M., Solito M.-M., Bourland W. A., et al. (2023). Rediscovery of remarkably rare anaerobic tentaculiferous ciliate genera Legendrea and Dactylochlamys (Ciliophora: Litostomatea). Biology 12, 707. doi: 10.3390/biology12050707
Shade A., Jones S. E., Caporaso J. G., Hendelsman J., Knight R., Fierer N., et al. (2014). Conditionally rare taxa disproportionately contribute to temporal changes in microbial diversity. Mbio 5, e01371–e01314. doi: 10.1128/mBio.01371-14
Stork N. E. (2018). How many species of insects and other terrestrial arthropods are there on Earth? Annu. Rev. Entomol. 63, 31–45. doi: 10.1146/annurev-ento-020117-043348
Weiss J., Andreou D., Esteban G. F. (2022). The extraordinarily rare Legendrea loyezae Fauré-Fremiet 1908 (Haptoria, Ciliophora). Protist 173, 12591. doi: 10.1016/j.protis.2022.125912
Weisse T. (2014). Ciliates and the rare biosphere —community ecology and population dynamics. J. Euk. Microbiol. 61, 419–433. doi: 10.1111/jeu.12123
Keywords: anaerobic ciliates, microbial diversity, Apertospathula, Legendrea, Penardiella
Citation: Weiss J and Esteban GF (2024) Tracking down the rare ciliate biosphere. Front. Protistol. 1:1308546. doi: 10.3389/frpro.2023.1308546
Received: 06 October 2023; Accepted: 04 December 2023;
Published: 08 January 2024.
Edited by:
Renata Matoničkin Kepčija, University of Zagreb, CroatiaReviewed by:
Leonardo D. Fernández, Universidad Bernardo O’Higgins, ChileAdriana Vallesi, University of Camerino, Italy
Copyright © 2024 Weiss and Esteban. This is an open-access article distributed under the terms of the Creative Commons Attribution License (CC BY). The use, distribution or reproduction in other forums is permitted, provided the original author(s) and the copyright owner(s) are credited and that the original publication in this journal is cited, in accordance with accepted academic practice. No use, distribution or reproduction is permitted which does not comply with these terms.
*Correspondence: Genoveva F. Esteban, Z2VzdGViYW5AYm91cm5lbW91dGguYWMudWs=