- 1Department of Urology, Weill Cornell Medicine, New York Presbyterian Hospital, New York, NY, United States
- 2Department of Undergraduate Studies, Cornell University, Ithaca, NY, United States
- 3Department of Surgery & Perioperative Medicine, University of Texas at Austin Dell Medical School, Austin, TX, United States
- 4Department of Radiology, Weill Cornell Medicine, New York Presbyterian Hospital, New York, NY, United States
- 5Department of Radiology, University of Texas Southwestern Medical Center, Dallas, TX, United States
- 6Columbia University, New York, NY, United States
- 7Promaxo Inc, Oakland, CA, United States
Purpose: Magnetic resonance imaging (MRI)-guided procedures are typically performed under high magnetic field strengths (1.5-3 Tesla) and are expensive. Recently, technological progress has led to MRIs that are low-cost but similarly high performing despite lower field strengths (58-74mT). Our purpose was to evaluate the cost-efficiency of implementing a low-field MRI for targeted prostate cancer biopsy.
Materials and methods: We utilized time-driven activity-based costing (TDABC) to establish the overall costs based on cost rates and total times for every step. Thorough literature searches and consultations with two board certified radiologists, the process maps were created, and the duration of each step was determined by experience and previously published data. A sensitivity cost analysis was also performed to create several cost scenarios for the low-field MRI system.
Results: Total procedure times, performed under moderate sedation, were 61 ± 14.5 mins and 57 ± 23 mins for transperineal targeted biopsy (TPB) with low-field MRI and in-bore MRI-guided transrectal biopsy (IB-TBx) with high-field MRI. In the low-field MRI pathway, 61% of intra-operative time was spent on patient preparation and performing the prebiopsy MRI, however, this step did not require a urologist or radiologist expertise, subsequently keeping costs lower. A sensitivity analysis of the low-field MRI cost at 5% to 50% (in 5% increments) of a high-field MRI produced a difference from $888.13 to $879.18 favoring the lower-field system, respectively.
Conclusions: A portable, low field, office-based MRI system offers a cost-efficient alternative to the high-field MRI. Further studies assessing cost effectiveness while taking into consideration sampling accuracy are needed.
Introduction
There is growing utilization of multiparametric magnetic resonance imaging (mpMRI) as a non-invasive tool in the detection of prostate cancer (PCa) due to its superiority in detecting clinically significant PCa (csPCa) and avoiding overdiagnosis and overtreatment of insignificant PCa (1). The current standard of care, transrectal ultrasound guided (TRUS) biopsy underestimates PCa aggressiveness in 38% of cases and misses up to 52% of clinically significant tumors (1). The addition of mpMRI in the diagnostic pathway of PCa has paved the way for targeted biopsy techniques such as in-bore MRI-guided transrectal biopsy (IB-TBx), ultrasound-guided fusion biopsy (FBx) and ultrasound-guided cognitive biopsy (Cog-TBx). Transperineal targeted biopsy (TPB) has also gained popularity as a feasible and tolerable technique in an ambulatory setting (2). Additionally, mpMRI with biomarkers (i.e. SelectMDx, 4Kscore, ExosomeDx) have demonstrated to help better screen patients for biopsy (3, 4) However, there is no comparative study of IB-TBx with TPB from an oncologic, tolerability, or cost perspective and the adoption of either method is resource and experience dependent (5).
As healthcare reimbursement evolves to become more tied to health-related outcomes, cost must also be considered to evaluate effectiveness of a modality. In biopsy naïve patients, mpMRI has been shown to be cost effective and avoids unnecessary biopsies compared to the standard ultrasound-guided technique when the magnetic resonance imaging (MRI) is used as a screening tool (6). A Markov model looking at the cost-effectiveness of incorporating mpMRI based technique over a 5-, 10-, and 20-year period demonstrated cost differences increasing from $796 at 5 years to $2,187 at 20 years per patient, favoring MRI-based techniques over TRUS (6).
Diagnostic mpMRIs are typically performed under high magnetic field strengths (1.5-3 Tesla[T]) and require a specialized radiology suite with strict safety precautions to house the large system along with costly superconducting magnets, leading to limited accessibility. With healthcare expenditure in mind, technological progress and development led to a paradigm shift advocating for low-cost but high performing designs with clinically applicable portability that allows for in office-use.
In this study, we evaluate cost efficiency of implementing a low field (58mT – 74 mT)) portable open mpMRI by comparing the cost of a high-field MRI-guided IB-TBx procedure to a low-field MRI-guided TPB procedure. We utilized time-driven activity-based costing (TDABC) to establish overall costs based on cost rates and total times for each step of the processes.
Materials and methods
Process map
Two process maps were constructed for biopsy naïve patients undergoing MRI-guided targeted prostate biopsies using a clinical model of two suspicious lesions where three cores were obtained from each lesion.
In-bore biopsy with high-field MRI process map
The first process map (Figure 1) describes patients who underwent a high field (3T) MRI-guided IB-TBx via a transrectal approach.
This procedure was previously described in a single-center, retrospective data consisting of 443 patients who underwent IB-TBx between May 2017 and December 2019 (7). Biopsies were performed under intravenous sedation by one of two radiologists at the institution with more than two years of experience and therefore did not require a pre-procedural anesthesia assessment except in high-risk patients or in patients requesting general anesthesia (<1% of all patients). All patients received antibiotics prophylaxis according to American Urological Association which usually consistent of fluoroquinolone or ceftriaxone in patients with high risk for Escherichia coli resistance (8).
Patients were placed in a prone position. The procedural steps included: (1) performing a prebiopsy fast scan using axial and sagittal T2-weighted images (T2WI) then using the diagnostic mpMRI obtained prior to the procedure day to outline the target area with the DynaTRIM (DynaTRIM®, In vivo, Gainesville, FL) and DynaCAD 5.0 platform, (2) directing the needle guide towards the target lesions according to the coordinates generated by the software, (3) confirming optimal needle-guide/target alignment with T2WI then placing the needle, triggering biopsy gun and performing a lesion-needle location verification with T2WI to ascertain proper sampling.
Transperineal biopsy with a low-field MRI process map
The second process map (Figure 2) describes patients who underwent a low-field MRI-guided TPB (Promaxo Inc., Oakland, CA, USA) (9). The Promaxo system is a single-sided open system with a permanent gradient and a main magnetic field strength operating at 58-74 mT and was cleared by the United States Food and Drug Administration (USFDA) for MRI-guided prostate interventions. Its open configuration accommodates a transperineal biopsy approach which was previously described (9). Data from community urology practice (Mississippi Urology, WIRB #20203968) was used to develop the process map and calculate the procedure duration. The Promaxo DICOM viewer software was utilized in this model and required no specialized software packages such as DynaCAD or DynaTrim. Both experiences and methodology were previously described (9, 10). The procedural steps included: (1) performing prebiopsy scan using T2WI to locate the prostate and ensure proper patient positioning, (2) co-registering T2WI from diagnostic mpMRI on Promaxo DICOM viewer software for template calibration and mapping biopsy coordinates with the physical template, (3) identifying and planning biopsy targets, and (4) triggering biopsy gun. All biopsies were performed with a 20cm biopsy gun and 18-gauge Bard Max-Core Biopsy Instrument (Bard, Covington, GA, USA).
Cost assumptions
Time-driven activity-based costing (TDABC) was used to estimate the resources for each clinical process. Data regarding salaries, equipment and facility cost rates were derived from our institutional accounting (University of Texas Southwestern Medical Center, Weil Cornell Medical Center) data (11). For each process map, a given step’s cost was calculated by multiplying that step’s capacity cost rate for each resource (personnel, equipment, and facilities) by its duration, and taking the sum of these resource costs. The total cost for each biopsy modality was then found by adding each step’s overall cost and the total time for each biopsy procedure and adding the steps’ durations together in a process map. The cost parameters were included from a health enterprise cost accounting perspective and were adjusted to 2020 United States dollars using the consumer price index for medical costs.
(1) Costs and salaries were obtained from the institution’s chief financial officer a capacity cost rate for any given personnel was calculated by dividing their annual salary by their available capacity (in hours) to work over the course of a year.
(2) All personnel were estimated to work 230 days in a year and 8 hours on each of those days and the available capacity was the product of these values.
(3) For costly equipment such as MRIs, an hourly cost rate was calculated by approximating the yearly operating cost was divided by its available capacity considering amortization.
(4) Certain equipment, such as local anesthetic, and antibiotics were assumed to have a negligible cost in comparison to the much larger resource costs.
Model assumptions
(1) Pre-biopsy mpMRIs were obtained at the same facility
(2) Costs were based on target biopsy of two lesions with three cores taken from each lesion
(3) IB-TBx was performed transrectally and TPB was performed transperineally
(3) All procedures were performed under moderate sedation
(4) Post-procedure recovery time were the same in both maps
Cost analysis
Given the cost of the low-field MRI system was undefined, multiple replacement cost scenarios as a percentage (5% to 50%) of high-field MRI replacement cost, in increments of 5%, was used to create several cost scenarios for the low-field MRI system. In addition, disposable costs were derived from ultrasound-MRI fusion biopsy system (UroNav, In vivo, Gainesville, FL) given the same instruments were used. The low-field MRI system did not require any offline workstation for segmentation, annotations, or fusion. These scenarios are presented in the supplementals.
Results
On average, the procedure times of the two models were similar within standard deviation, at 61 (± 14.5) minutes with the low-field MRI-guided TPB vs. 57 (± 23) minutes with the high-field MRI-guided IB-TBx. In terms of specialist dedicated time, the total urologist time for the low-field system was 37 minutes or 29.4% of total procedural time and the total radiologist time for the high-field system was 57 mins or 100% of total procedural time. (Table 1). High-field MRI-guided IB-TBx was estimated to cost $2,479.57 per procedure (Table 2).
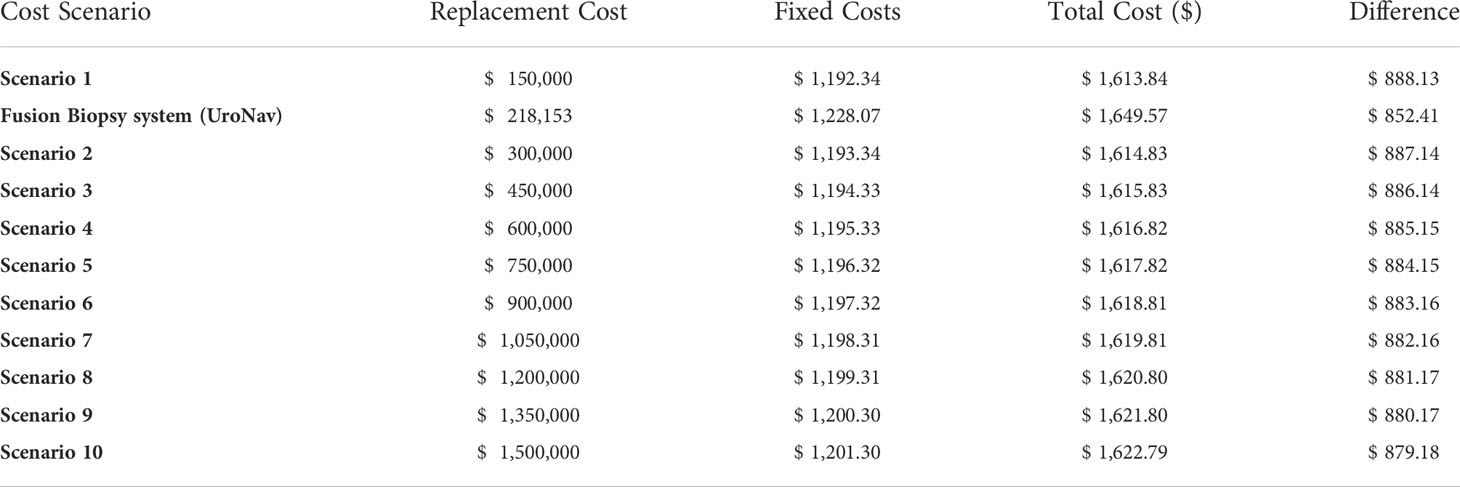
Table 1 Multiple scenario costs of the potential cost of 63mT MRI system by using a sensitivity analysis of the low-field MRI cost at 5% to 50% (in 5% increments) of a high-field MRI produced a difference from $888.13 to $879.18 favoring the lower-field system, respectively.
A sensitivity analysis of the low-field MRI cost at 5% to 50% (in 5% increments) of a high-field MRI produced a difference from $888.13 to $879.18 favoring the lower-field system, respectively. (Supplemental Table 1).
Discussion
We report a theoretical cost comparison of the high-field MRI-guided procedure to the low-field MRI-guided procedure to evaluate the potential cost advantage of implementing a portable, low-field, office-based MRI device. Procedure times were similar when comparing the two systems (61mins vs. 57mins, low-field vs. high-field, respectively). From our analysis, the cost difference between the MRI-targeted pathway stems from on the need for MRI-compatible instruments and the MRI itself.
A low-field system reduces the need for MRI compatible instruments and utilizes a weaker magnet therefore reducing the cost of the device itself. Additionally, it removed the need for a specialized infrastructure to shield from magnetic and electromagnetic disturbances. Because of its portability, it could be utilized in an outpatient setting without the need for added operational costs. Our analysis did not reflect a clinic setting, although this may be the eventual goal. We modeled the cost based on the initial experience thus far with this device which has been in an ambulatory procedural room setting. It is also worth mentioning that a low field system did not require the same tight safety restrictions as high-field MRI, therefore, the need for extra safety training, personnel, and MRI-compatible instruments are no longer necessary. From an opportunity cost standpoint, accessibility to MRI machines has often been the bottleneck in clinical flow resulting in competition between MRI-guided procedures and diagnostic imaging for an MRI spot. Therefore, reducing the cost could increase the physical number of MRIs.
It is also important to note that in the prebiopsy scan in the low-field MRI model, a urologist or radiologist does not have to physically be in the room to perform the initial scan. This can be done by a technician which may reduce personnel cost. Our process map indicates that low-field MRI pathway can be optimized by protocoling the prebiopsy scans since it does not require a specialists’ expertise or time. Nasri et al. recently described the feasibility of integrating the low-field MRI into clinical workflow under sedation (9). Once workflow is established and urologists are more acquainted with the low-field system, transition to fully outpatient procedure with local anesthetic is expected.
Additionally, another benefit of an MRI-based system is the real-time exact lesion correlation. Ultrasound-guided techniques require cross-modality registration of the diagnostic MRI images to the volumetric TRUS image. However, factors such as an abnormally shaped prostate, motion artifact from the patient or deformation of the gland from the probe can easily increase the registration error, thereby adding additional procedure time and possibly affecting cancer detection rates. In fact, Puech et al. compared Cog-TBx with FBx and did not see a significant difference in cancer detection suggesting that detection rate relied heavily on the operator’s experience rather than the software (12). When comparing different registration methods, only an experienced operator significantly reduced registration error (13). Similarly in the ASIST trial, Klotz et al. demonstrated that more experience and higher training were needed to minimize this error (14).
Another interesting technological development in urology is the high-resolution (20MHz) micro-ultrasound system (ExactVu) with impressive spatial resolution to visualize ejaculatory ducts and most importantly suspicious prostatic lesions therefore allowing for targeted biopsies to be performed (15). In a multicenter registry, Klotz et al. demonstrated that micro-ultrasound and mpMRI sensitivity for detecting csPCa was 94% vs. 90%, respectively and the negative predicative value (NPV) was 85% vs. 77%, respectively (16). One of the limitations mentioned in the study is the learning curve. A grading system using the micro-ultrasound has been developed to stratify risk of significant cancer however it has not been validated.
While perks of portable low-field MRI include fewer personnel, it requires the urologist to develop some radiologic expertise. Additionally, utilizing new modalities are often associated with a learning period that would need to be overcome. Chiragzada et al. devised a prostate phantom to assess the accuracy of the Promaxo’s MRI system with four board-certified urologists (17). With the assistance of the system’s imaging and navigation software, the average navigation error was found to be less than 3mm suggesting that the learning curve can be quickly overcome with acceptable results (17). It should be recognized, however, that phantoms convey a best-case scenario not accounting for challenges such as motion and lesion in conspicuity with low magnetic field systems, thus requiring further validation by other studies. It is important to note that one of the advantages of the in-bore approach is that it does not rely on image fusion therefore registration and navigation errors are mitigated.
One of the limitations of the study is that we compared a transrectal to a transperineal approach. IB-TBx is commonly performed in a transrectal approach in the United States, although it can also be performed transperineally or transgluteally. The transglueteal approach is typically reserved for patients with previous rectal surgery or abscess. Our current process map for IB-TBx represents a workflow optimized by a group of radiologists. Therefore, comparing it to the low-field MRI model would provide a more robust comparison. Another limitation is that the cost of the low-field MRI system was depicted in multiple cost scenarios which incorporated disposable costs derived from the UroNav system. A previous paper from our same institution published a mean procedure time of 48.3 mins with transperineal biopsy with UroNav compared to our time of 61 min for low-field, which is a difference of 12.7 mins and within our standard deviation mins (10). Additionally, our study only provided a short-term cost analysis. Details of costs utilized can be found in Supplemental Table 2. A long-term cost analysis is needed as is the incorporation of procedure accuracy in order to assess true cost-effectiveness. We have not published the oncological outcomes of the low-field MRI system. The ability of the system to accuracy diagnose prostate cancer compared to conventional tools can also affect the cost-analysis. Another limitation is that our cost analysis does not account for the learning curve associated with operating a new software system and device which could result in a significantly longer procedure duration.
Urology has been at the forefront of technological advances since it pioneered progression in the field of robotics. Unlike high-field diagnostic MRI, a low field MRI designed for interventions can support more MRI-guided case volumes. Its portability and accessibility can change diagnostic and therapeutic algorithms.
Conclusion
The selection of patients based on their individual characteristics and onsite cost-benefit analysis are important aspects of healthcare. From a short-term perspective, it may be efficient to implement a portable, low-field, office-based MRI device. However, the sampling accuracy of the portable, lower cost system needs to be established and compared to the IB-TBx before head-to-head cost utility analyses can be made. Ultimately, the preference for a technique should rely on local experience and availability of equipment. The development of novel imaging techniques may lead to further refinements in this important diagnostic pathway.
Data availability statement
The raw data supporting the conclusions of this article will be made available by the authors, without undue reservation.
Author contributions
BC had full access to all the data in the study and takes responsibility for the integrity of the data and the accuracy of the data analysis; study concept and design: CS, AL, ZC, DM, DC, SV, PS and BC; Acquisition of data: CS, AL, DC, AN, and DK; analysis and interpretation of data: CS, AL, SV, and PS; drafting of the manuscript: CS and ZC; critical revision of the manuscript for important intellectual content: CS, AL, ZC, DM, DC, AN, DK, SV, PS and BC; Supervision: BC. All authors contributed to the article and approved the submitted version.
Conflict of interest
DM is a consultant for Promaxo. AN, DK, SV, and PS are Promaxo employees. BC is a consultant for Allergan and Boston Scientific, and Promaxo.
The remaining authors declare that the research was conducted in the absence of any commercial or financial relationships that could be construed as a potential conflict of interest.
Publisher’s note
All claims expressed in this article are solely those of the authors and do not necessarily represent those of their affiliated organizations, or those of the publisher, the editors and the reviewers. Any product that may be evaluated in this article, or claim that may be made by its manufacturer, is not guaranteed or endorsed by the publisher.
Supplementary material
The Supplementary Material for this article can be found online at: https://www.frontiersin.org/articles/10.3389/fruro.2022.966862/full#supplementary-material
References
1. Ahmed HU, El-Shater Bosaily A, Brown LC, Gabe R, Kaplan R, Parmar MK, et al. Diagnostic accuracy of multi-parametric MRI and TRUS biopsy in prostate cancer (PROMIS): A paired validating confirmatory study. Lancet (2017) 389(10071):815–22. doi: 10.1016/S0140-6736(16)32401-1
2. Bass EJ, Donaldson IA, Freeman A, Jameson C, Punwani S, Moore C, et al. Magnetic resonance imaging targeted transperineal prostate biopsy: a local anaesthetic approach. Prostate Cancer Prostatic Dis (2017) 20(3):311–7. doi: 10.1038/pcan.2017.13
3. Busetto GM, Del Giudice F, Maggi M, De Marco F, Porreca A, Sperduti I, et al. Prospective assessment of two-gene urinary test with multiparametric magnetic resonance imaging of the prostate for men undergoing primary prostate biopsy. World J Urol (2021) 39(6):1869–77. doi: 10.1007/s00345-020-03359-w
4. de la Calle CM, Fasulo V, Cowan JE, Lonergan PE, Maggi M, Gadzinski AJ, et al. Clinical utility of 4Kscore((R)), ExosomeDx and magnetic resonance imaging for the early detection of high grade prostate cancer. J Urol (2021) 205(2):452–60. doi: 10.1097/JU.0000000000001361
5. Berry B, Parry MG, Sujenthiran A, Nossiter J, Cowling TE, Aggarwal A, et al. Comparison of complications after transrectal and transperineal prostate biopsy: A national population-based study. BJU Int (2020) 126(1):97–103. doi: 10.1111/bju.15039
6. Callender T, Emberton M, Morris S, Pharoah PDP, Pashayan N. Benefit, harm, and cost-effectiveness associated with magnetic resonance imaging before biopsy in age-based and risk-stratified screening for prostate cancer. JAMA Netw Open (2021) 4(3):e2037657. doi: 10.1001/jamanetworkopen.2020.37657
7. Subramanian N, Recchimuzzi DZ, Xi Y, Diaz de Leon A, Chen H, Xie D, et al. Impact of the number of cores on the prostate cancer detection rate in men undergoing in-bore magnetic resonance imaging-guided targeted biopsies. J Comput Assist Tomogr (2021) 45(2):203–9. doi: 10.1097/RCT.0000000000001115
8. Bhanot N, Sahud AG, Sepkowitz D. Best practice policy statement on urologic surgery antimicrobial prophylaxis. Urology (2009) 74(1):236–7. doi: 10.1016/j.urology.2009.03.025
9. Nasri JW, Vinayak G, Parekha S, Adams JD, Kumar D, Venkataraman SS, et al. OFFICE-BASED, POINT-OF-CARE, LOW-FIELD MRI SYSTEM TO GUIDE PROSTATE INTERVENTIONS: RECENT DEVELOPMENTS. Eur Med J (2021) 9:83–90.
10. Cricco-Lizza E, Wilcox Vanden Berg RN, Laviana A, Pantuck M, Basourakos SP, Salami SS, et al. Comparative effectiveness and tolerability of transperineal MRI-targeted prostate biopsy under local versus sedation. Urology (2021) 155:33–8. doi: 10.1016/j.urology.2021.06.023
11. Kaplan RS, Anderson SR. Time-driven activity-based costing. Harv Bus Rev (2004) 82(11):131–138, 150.
12. Puech P, Rouviere O, Renard-Penna R, Villers A, Devos P, Colombel M, et al. Prostate cancer diagnosis: multiparametric MR-targeted biopsy with cognitive and transrectal US-MR fusion guidance versus systematic biopsy–prospective multicenter study. Radiology (2013) 268(2):461–9. doi: 10.1148/radiol.13121501
13. Hale GR, Czarniecki M, Cheng A, Bloom JB, Seifabadi R, Gold SA, et al. Comparison of elastic and rigid registration during magnetic resonance Imaging/Ultrasound fusion-guided prostate biopsy: A multi-operator phantom study. J Urol (2018) 200(5):1114–21. doi: 10.1016/j.juro.2018.06.028
14. Klotz L, Lughezzani G, Maffei D, Sanchez A, Pereira JG, Staerman F, et al. Comparison of micro-ultrasound and multiparametric magnetic resonance imaging for prostate cancer: A multicenter, prospective analysis. Can Urol Assoc J (2021) 15(1):E11–E16. doi: 10.5489/cuaj.6712
15. Rohrbach D, Wodlinger B, Wen J, Mamou J, Feleppa E. High-frequency quantitative ultrasound for imaging prostate cancer using a novel micro-ultrasound scanner. Ultrasound Med Biol (2018) 44(7):1341–54. doi: 10.1016/j.ultrasmedbio.2018.02.014
16. Klotz L, Pond G, Loblaw A, Sugar L, Moussa M, Berman D, et al. Randomized study of systematic biopsy versus magnetic resonance imaging and targeted and systematic biopsy in men on active surveillance (ASIST): 2-year postbiopsy follow-up. Eur Urol (2020) 77(3):311–17. doi: 10.1016/j.eururo.2019.10.007
Keywords: prostate cancer, cost-effectiveness, MRI, biopsy, transperineal
Citation: Sze C, Cheema Z, Laviana A, Margolis D, Costa DN, Satya P, Nacev A, Kumar D, Venkataraman SS and Chughtai B (2022) Cost-efficient analysis of a portable low field magnetic resonance imaging system for prostate cancer diagnosis. Front. Urol. 2:966862. doi: 10.3389/fruro.2022.966862
Received: 11 June 2022; Accepted: 08 August 2022;
Published: 20 September 2022.
Edited by:
Shawn Dason, The Ohio State University, United StatesReviewed by:
Martina Maggi, Sapienza University of Rome, ItalyMatthew Liew, Royal Albert Edward Infirmary, United Kingdom
Copyright © 2022 Sze, Cheema, Laviana, Margolis, Costa, Satya, Nacev, Kumar, Venkataraman and Chughtai. This is an open-access article distributed under the terms of the Creative Commons Attribution License (CC BY). The use, distribution or reproduction in other forums is permitted, provided the original author(s) and the copyright owner(s) are credited and that the original publication in this journal is cited, in accordance with accepted academic practice. No use, distribution or reproduction is permitted which does not comply with these terms.
*Correspondence: Bilal Chughtai, YmljOTAwOEBtZWQuY29ybmVsbC5lZHU=