- 1Instituto de Geografía de la, Universidad Nacional Autónoma de México, Mexico City, Mexico
- 2Posgrado en Ciencias de la Tierra, Universidad Nacional Autónoma de México, Mexico City, Mexico
- 3Departamento de Ingeniería Ambiental-Facultad de Ingeniería Civil, Universidad Autónoma de Nuevo León, San Nicolás de los Garza, Mexico
- 4Laboratorio Variclim, Facultad de Agronomía y Veterinaria, Universidad Autónoma de San Luis Potosí, San Luis Potosí, Mexico
The Monterrey Metropolitan Area (MMA) is located in northeastern Mexico in a semi-arid region. As in other areas of the world, prolonged and severe meteorological droughts are recurrent. Meteorological droughts affect the level of dam reservoirs, rivers, and groundwater (aquifers) in the region to the extent they become hydrological droughts which in turn may contribute to socioeconomic droughts. The recent dry event (2011–2013) in northern Mexico severely affected various socioeconomic sectors and may serve as an example of future climatic and hydrologic conditions in this region. Meteorological droughts in northeastern Mexico, mostly induced by anomalously intense subsidence, frequently result in hydrological droughts and intense pressure in the water resources services of the Monterrey Metropolitan Area (MMA), one of the largest cities in Mexico. Landfalling tropical cyclones entering northeastern Mexico historically have alleviated water crises during prolonged droughts. In 2013, the rains from tropical cyclone Ingrid helped to recover water levels in the system of dam reservoirs that provides water to the MMA. However, water management is only one part of a complex socioeconomic system that must respond to the growing demands for water in a region where aquifers are already overexploited. Trends in the atmospheric circulation indicate that droughts in the region may become more severe in the coming decades. Improved water management programs and protocols should be considered in addition to closer reviews of the hydraulic infrastructure. Water transfers from one region to another should be carefully planned because solving a problem in one place may adversely affect another. The use of climate information may be critical to avoidance of water conflicts in the future.
Introduction
Semi-arid climates are associated with relatively low precipitation, usually between 200 and 700 mm/year, with large climatic contrasts in alternating seasons and years. Water scarcity in these regions has shaped the characteristics of socioeconomic activities. A large percentage of drylands around the world are devoted to agricultural, cattle ranching, or industrial activities. However, rapid demographic growth contributes to more intensive water- consuming activities, which turns into a major problem during prolonged dry periods (UNISDR, 2009). Some water management practices aimed at responding to increasing water demands may result in social, economic, and environmental problems (e.g., Monforte-García et al., 2012) related to over-exploitation of surface water and aquifers. The problem may become more difficult considering published scenarios of climate change for the coming decades (IPCC, 2019).
Meteorological droughts always affect a large number of people with a number of negative impacts (Singh et al., 2014; Center for the Research of Epidemiology of Disasters (CRED), 2015) such as crop-yield failure, erosion of soils, unemployment, lowered incomes, malnutrition, and even psychological problems (Magaña, 2016). Planning water management in semi-arid regions is particularly difficult during anomalous dry periods due to the interests of various stakeholders (Madani et al., 2016) mostly from the agricultural sector. Current drought risk management strategies do not appear to provide an efficient response to the problem (Wilhite et al., 2014) and consequently, the challenges of water management become particularly difficult when the effects of climate change are related to drier conditions (IPCC, 2007; Abu-Allaban et al., 2015).
Water availability changes in time, mainly in relation to low and very-low frequency climate variability, but also in relation to water management practices. Various studies (e.g., Wilhite and Glantz, 1985; Madani et al., 2016) show that meteorological droughts in a vulnerable context, such as that found in semi-arid regions, may result in higher risks of hydrological, agricultural or socioeconomic droughts. The latter occur when water supply cannot meet the demands for water. For example, during meteorological droughts, water shortages in an urban sector increase the risk of socioeconomic droughts, due to the combined effect of a climatic hazard (meteorological drought) occurring in a vulnerable context. Since there is not a universally accepted methodology to estimate vulnerability to meteorological droughts or any other climatic hazard, numerous approaches have been proposed to characterize socioeconomic droughts, mainly based on indices (Eklund and Seaquist, 2015; Shi et al., 2018; Tu et al., 2018). Some approaches specifically concentrate on aspects of vulnerability and resilience of society to meteorological droughts (Mehran et al., 2015; Liu et al., 2020) and make use of indicators to describe socioeconomic droughts by examining the role and condition of local reservoirs and water demands (Huang et al., 2016; Neri and Magaña, 2016). For instance, a region is considered to be more vulnerable to meteorological droughts if aquifers are overexploited, if there are no plans to reduce water consumption, or if there is no use of climate information for prevention actions (CONAGUA, 2014).
In Mexico, the rapid urbanization, insufficient water distribution infrastructure, water quality degradation, competition for water between agriculture and urban areas, and climate change have put more pressure on water management agencies and their plans to prevent or ameliorate the impacts of prolonged meteorological droughts (Magaña, 2016). Consequently, most actions to face drought still focus on compensating economic sectors for their losses in the aftermath of a disaster (Aguilar-Barajas et al., 2016). In the context of a hydrological crisis, preparing for and recovering from meteorological droughts is becoming more and more difficult. Under climate change, northern Mexico will face serious problems in responding to ever-growing water demands (Magaña et al., 2012).
The Monterrey Metropolitan Area (MMA), located in northeastern Mexico (Figure 1), is the nation's third largest urbanized region with a population of around 4.5 million people. Its rapid economic expansion and demographic growth has required increased use of land, infrastructure, and services, but most importantly, water. From 2000 to 2013, water use in the MMA grew by around 45%, while water supply only increased by 12% (CONAGUA, 2014). The Monterrey Water Agency has promoted programs for more efficient use of water resources and constructed large water reservoirs such as El Cuchillo dam reservoir to satisfy the city's constantly growing demand for water (Velasco et al., 2005). But the recent prolonged drought episodes (e.g., 2011–2013) revealed the critical vulnerability of the MMA to precipitation deficit. Recent water infrastructure plans have been made to reduce the risk of socioeconomic droughts including the construction of more dam reservoirs in the zone and new infrastructure development for water transfers from the Huasteca region near the City of San Luis Potosí (around 540 km, from the MMA). The water transfers from distant regions could have important social and environmental (negative) consequences (SADM, 2012).
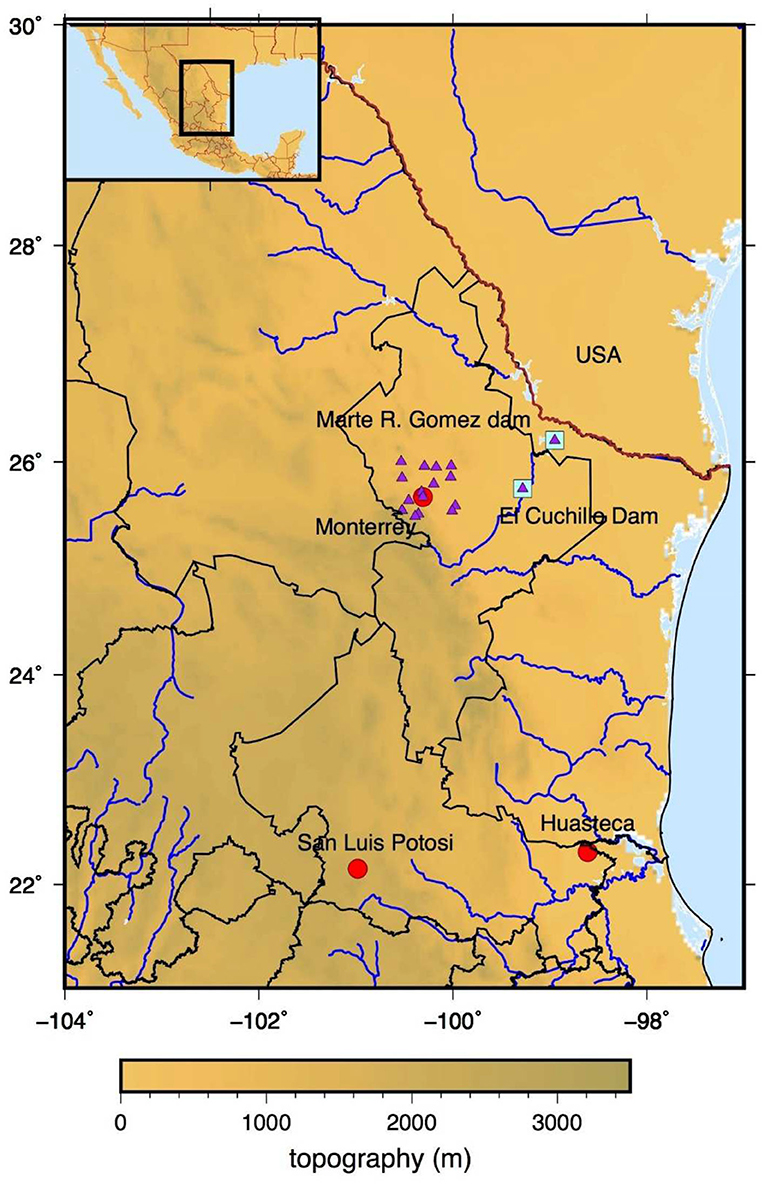
Figure 1. Northeastern Mexico, showing the Monterrey Metropolitan Area (red circle). El Cuchillo Dam reservoir, Marte R. Gomez dam reservoir, and the San Luis Potosi Huasteca area constitute three reference locations for the present study. Shading indicates topography and the purple triangles correspond to the location of the weather stations used in the study.
Prolonged and severe meteorological droughts in northeastern Mexico have resulted in economic, environmental, and social problems (Ortega-Gaucin, 2013) affecting, for instance, water requirements for urban consumption (when demands increase) and reductions in the productive capacity of some of the providers of goods for the MMA (Sisto et al., 2011). The risk of a severe socioeconomic drought appears to be increasing (Sisto et al., 2016). In 1998 and again in 2013, the MMA water supply system almost collapsed. In 2013 the region's main sources of urban water, the El Cuchillo dam reservoir along with other reservoirs were almost depleted. It was only the effect of extreme precipitation from tropical cyclone (TC) Ingrid (more than 500 mm/3 days) that prevented a major disaster. But the high risk of socioeconomic droughts remains as climate change predictions presage a warmer climate with a possibly worsening reduction of surface and groundwater availability. Therefore, new water management plans and measures are critically necessary to reduce the risks of socioeconomic drought (CONAGUA, 2014). In particular, a better understanding is needed regarding how meteorological droughts can evolve into socioeconomic droughts in the MMA region so that a resilient and preventive disaster risk management plan can be developed.
The high risk of the region's vulnerability to socioeconomic droughts is becoming better understood with recent insights from studies of how prolonged meteorological droughts evolve over northern Mexico (Méndez and Magaña, 2010) and on the factors that create such hazards. This knowledge may enable planners to propose better risk management and preventive actions to reduce the negative impacts of droughts, particularly in semi-arid urban centers and in view of climate change. Therefore, the main objective of the present study is to document how meteorological droughts in northeastern Mexico evolve to become socioeconomic droughts affecting the MMA.
Data and Methodology
Characterizing the episodes of drought and impacts requires making use of climatic, environmental, and socioeconomic data. Meteorological droughts are described based on station and gridded precipitation data. Daily precipitation data from 17 weather stations in the MMA and surrounding regions were obtained from the Mexican National Water Commission (CONAGUA) (CONAGUA, 2019) data base (Figure 1) for the period 1960–2015. This information on precipitation is complemented with high spatial resolution analyses obtained from the CMORPH data base (Joyce et al., 2004), corresponding to monthly fields available on 8 × 8 km grids. Precipitation anomalies were compared with gridded Standard Precipitation Indices (SPI-12) available at the SPI-PRECL0p5_12-month of the IRI data base. SPI-12 data have a spatial resolution of 0.5 km by 0.5 km for the 1948–2018 period and are available at: https://iridl.ldeo.columbia.edu/SOURCES/.IRI/.Analyses/.SPI/.SPI-PRECL0p5_12-Month/index.html. Precipitation is significantly intense when tropical cyclones landfall over northeastern Mexico, mainly between May and November (Dominguez and Magaña, 2018). Information on landfalling tropical cyclones is obtained from the NOAA tropical cyclone archive (NOAA, 2019).
The dynamics of prolonged meteorological droughts are analyzed in terms of the low-level moisture flux from the Intra Americas Seas (IAS) into northeastern Mexico, and by examining the intensity of subtropical atmospheric motions. The moisture flux fluctuations are documented using North America Regional Reanalysis (NARR) (Mesinger et al., 2006) for the 1979–2018 periods and NCEP/NCAR Reanalysis data (Kalnay et al., 1996) for the 1948–2018 period. The former allows a high spatial resolution description of the low-level moisture fluxes and atmospheric subsidence at 700 hPa, while the latter allows a lower spatial resolution, but for a longer period. These data are complemented with indices on very low frequency variations of the sea surface temperature over the Atlantic Ocean, corresponding to the Atlantic Multidecadal Oscillation (AMO) (Schlesinger and Ramankutty, 1994). The AMO has been shown to be related to fluctuation in water availability in various regions of North America (Enfield et al., 2001; McCabe et al., 2004) and to changes in hurricane activity over the IAS (Goldenberg, 2001).
Hydrological data from CONAGUA (2020) were used to document changes in monthly water availability at dam reservoirs around the MMA. Water supply for the MMA comes from two main sources: (i) surface water in a system of dam reservoirs that provides almost 55% of water to the urban area, and (ii) groundwater extracted from the aquifer (45%) from more than forty wells in the zone. Water stored at El Cuchillo reservoir (Figure 1) is compared with precipitation data to examine the impacts of meteorological droughts. The effects of water transfers to neighboring regions from El Cuchillo reservoir are also analyzed as a factor that may affect water provision for the urban sector. The case of the severe and prolonged meteorological drought between 2011 and 2013 is examined in detail to illustrate how both drier climatic conditions and water management affected the water provision for the region. This example may serve as a scenario of more frequent conditions in the future under climate change. Estimates of groundwater fluctuations are obtained using data from the Gravity Recovery and Climate Experiment (GRACE) (Rodell and Famiglietti, 2001) which corresponds to changes in equivalent water thickness relative to a time-mean baseline. GRACE data is used to document groundwater changes over the MMA region for the 2000–2016 period.
Finally, information on the demands and provision of water in recent years in the MMA is obtained from the Regional Office of Water in Nuevo Leon (Servicios de Agua y Drenaje de Monterrey). These data serve to describe part of the vulnerability context of the water sector in the MMA in relation to demands and supply.
Results
Climate Variability and Trends
The MMA is located at the foot of the Sierra Madre mountains in the central part of the state of Nuevo León, and the system of dam reservoirs that provide water to the region are further east, so water is pumped to Monterrey using hydraulic infrastructure. Development of the region has taken place around a continuously evolving hydraulic infrastructure, using water from the San Juan River. Maximum temperatures in summer are above 30°C (Figure 2A) and may reach 40°C during warm spells. Mean minimum temperatures during winter, may be slightly above 5°C, but during cold spells they may be close to 0°C. It is during the warmest months of the year, between June and August, that the monthly demands of water in the MMA increase to more than 30 hm3/month. Precipitation in the region is larger in summer, when the interaction between the low-level moisture flux and the Sierra Madre mountains results in precipitation events. Annual precipitation over the region is usually between 400 to 600 mm/year and the orographic forcing determines its large-scale spatial patterns (Figure 2B). The wettest years are the result of sporadic intense rainfall events (Sisto and Ramírez-Orozco, 2015) that serve to recover surface and groundwater levels (Sisto et al., 2016). Even when atmospheric low-level moisture into this region is high (as in July) rainy days are scarce because atmospheric subsidence in the subtropics inhibits deep convective activity (Herrera et al., 2015). In some years though, the contributions of landfalling Tropical Cyclones (TCs) result in large accumulated precipitation, since one single TC may result in more than 300 mm of rain in only a few days. It has been estimated that during wet years, around 30% or more of the total annual precipitation in the region comes from TC rains (Dominguez and Magaña, 2018). However, meteorological droughts continue to be one of the main climatic hazards for the MMA.
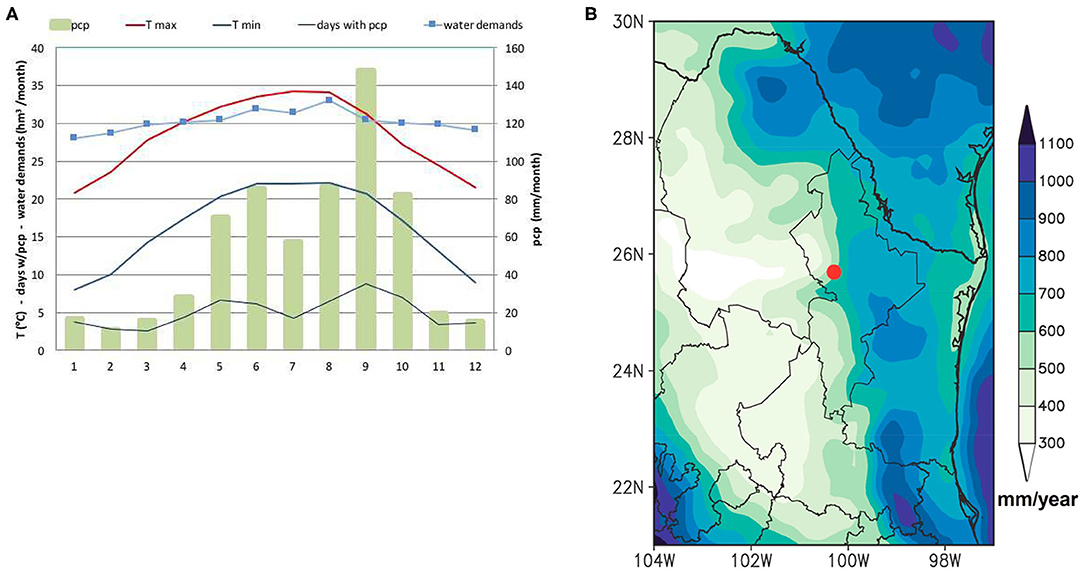
Figure 2. (A) Climograph of the MMA based on CONAGUA weather station data. The red line corresponds to maximum temperature (°C), the blue line corresponds to minimum temperature (°C), and the black line to the average number of days with precipitation. Monthly water demands in the MMA (hm3) are indicated with a blue line with squares. Green columns indicate monthly accumulated precipitation (mm) (B) Annual precipitation over northeastern Mexico based on CMORPH data.
Three prolonged drought periods affecting the MMA have occurred in northern Mexico in recent decades (1998–2001, 2005–2006, and 2011–2013) (Figure 3) in relation to very low frequency climate variability (Méndez and Magaña, 2010). Most frequently, meteorological and hydrological droughts are mitigated thanks to the effect of landfalling TCs (Figure 3) that change the precipitation statistics and water availability (Table 1). In this way, the annual precipitation of dry years may be between 200 and 300 mm/yr, while wet years may be close to 1,000 mm/yr, due to the contribution of those landfalling TCs. This form of climate variability makes seasonal climate forecasts a challenge, consequently making long-term water availability estimates difficult.
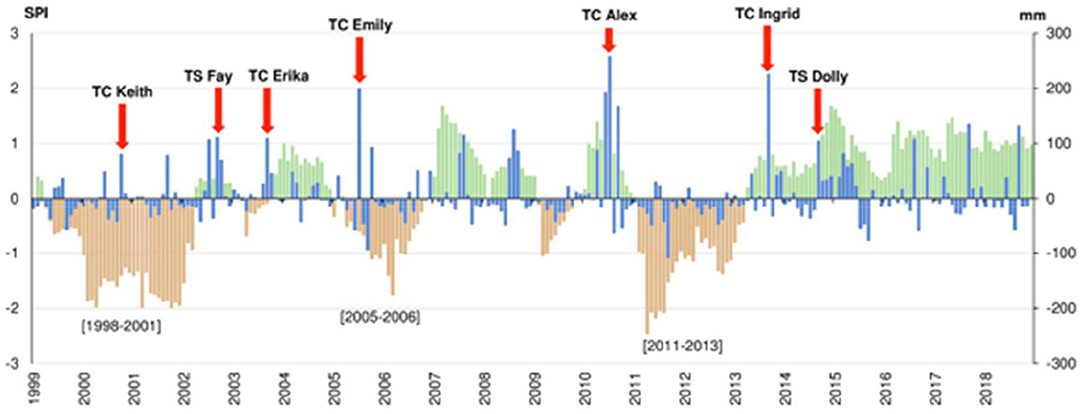
Figure 3. Precipitation anomalies (blue columns) and SPI-12 (green and brown columns) of the MMA. Red arrows correspond to the month when a landfalling TC affected NE Mexico. Numbers in brackets indicate a period of prolonged and severe drought.
One of the most severe droughts in recent years occurred between 2011 and 2013. During some months of the 2011 SPI-12 < −2, which reflected a severe meteorological drought (Figure 3), with important impacts in the streamflow and water reservoirs. The droughts extended over a large part of North America. The anomalously dry conditions lasted for more than 2 years and resulted in a reduction of water availability in the dam reservoirs that provide water to the MMA. It was only the effect of TC Ingrid on September 14-18, 2013, with more than 300 mm/3 days, that mitigated the drought conditions and helped to recover water storage levels, thus alleviating the conditions of a socioeconomic drought (Ortega-Gaucin, 2013). The socioeconomic costs of such dry conditions led the Mexican water agency (CONAGUA, in Spanish) to define public policies for reducing the impacts of drought. The National Program Against Drought (PRONACOSE, in Spanish) defined several actions to respond to socioeconomic droughts in major cities in Mexico, such as the MMA. However, such initiatives lack specific measures to make use of climate information for the prevention or amelioration of the negative impacts of droughts (Magaña, 2016).
In Mexico, it is a common practice to use climate information only to document the evolution of hazards that result in disasters. For instance, meteorological droughts are monitored but seldom predicted for planning water management with a goal of avoiding the occurrence of hydrologic, agricultural, or socioeconomic droughts. Identifying the factors that modulate climate variability serves to increase climate predictability (Krishnamurthy, 2019) which in turn may prove useful while planning water management activities, among other things. Méndez and Magaña (2010) found that very low-frequency variations of climate in northern Mexico were associated with the phases of the Atlantic Multidecadal Oscillation (AMO) and the Pacific Decadal Oscillation (PDO). In northeastern Mexico, the combined effect of AMO and the Caribbean Low Level Jet (CLLJ) modulate low-level moisture flux (Méndez and Magaña, 2010) and are key factors to explain prolonged dry and wet periods over the MMA.
The core of the CLLJ is located over the central Caribbean Sea but extends into the Gulf of Mexico and reaches northeastern Mexico (Figure 4). Its maximum intensity is at 925 hPa as observed in July (Amador, 1998) when the resulting moisture flux over northeastern Mexico maximizes. However, the intense subtropical atmospheric subsidence over this region also maximizes during this time of the year, limiting the favorable conditions for intense precipitation (Figure 5A). The intense atmospheric subsidence results in a relative minimum in precipitation during July, known as the Mid Summer Drought (Magaña et al., 1999; Herrera et al., 2015). It is not until September that atmospheric subsidence weakens and conditions for precipitation become more favorable. More intense precipitation during this time of the year is more probable given the increased chances of landfalling TCs. In this way, it is usually during the second half of the summer season when water availability increases under enhanced runoff.
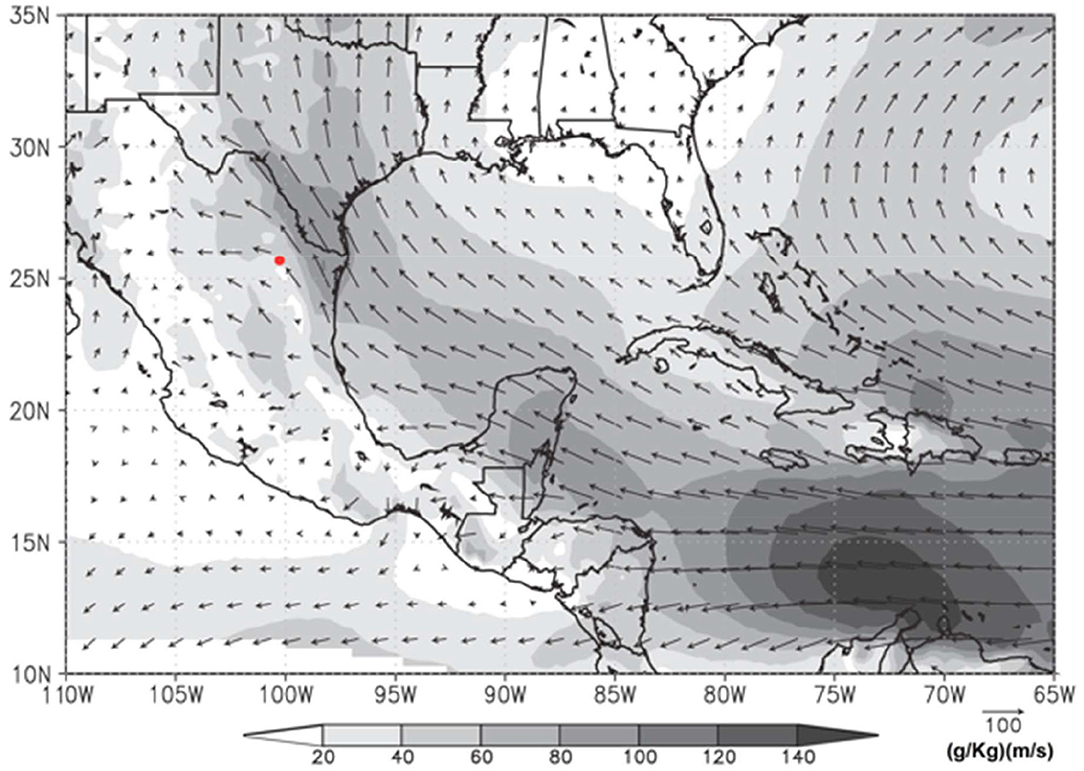
Figure 4. Climatology of the mean moisture flow at 925 hPa for the June-September period. Vector corresponds to moisture flux direction and shades of gray to moisture flux magnitude (g/kg) (m/s).
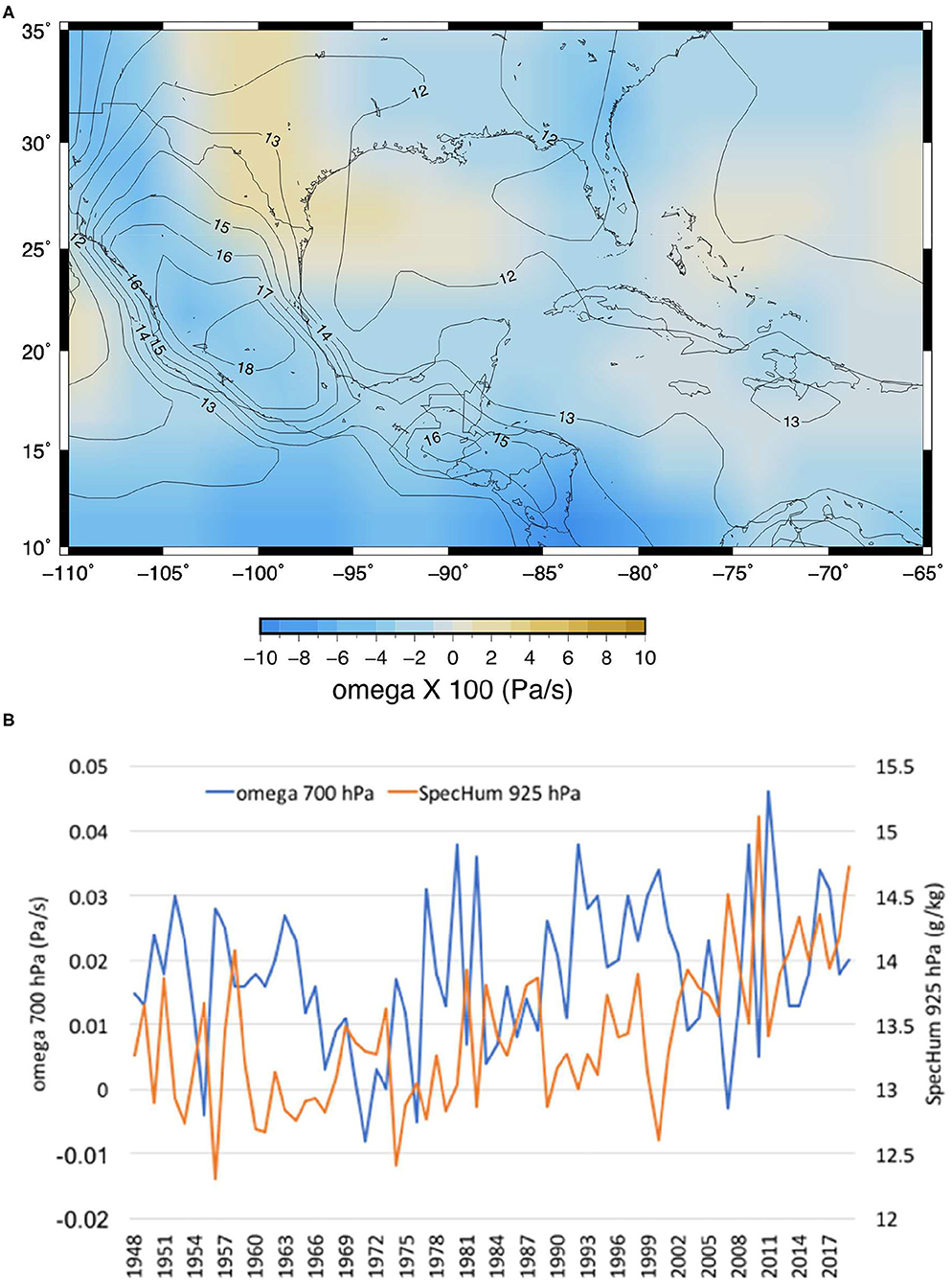
Figure 5. (A) Climatology of omega vertical velocity (Pa/s) at 700 hPa over the IAS, Mexico and Central America between June and September. Tan (blue) color indicates regions of atmospheric subsidence (ascending) motions. Isolines correspond to specific humidity (g/kg) at 925 hPa for the same months. (B) Time series (1948–2019) of vertical ascending motions (Pa/s) at 700 hPa (blue line) and specific humidity (g/kg) at 925 hPa (orange line) over NE Mexico (at 100°W, 25°N).
On interannual time scales, more intense atmospheric subsidence and weak specific humidity flowing at low atmospheric levels over northeastern Mexico combine to produce meteorological droughts. Some of the more severe droughts in this region show that such conditions were present in 1999–2002 or in 2011–2013 (Figure 5B). However, when the moisture flux is strong and atmospheric subsidence weakens, the chances of wet conditions increase. In recent decades (since 1970) such interannual variations are occurring in what appears to be a positive trend of more intense atmospheric subsidence (positive omega at 700 hPa) along with more low-level moisture flow into northeastern Mexico. This trend may lead to more frequent dry periods, but also higher chances of extreme precipitation events, particularly in relation to TC occurrences in the region. This tendency may be considered a regional climate change scenario or it could be part of the very low frequency climate variability of the region.
The modulation of climate phases by the AMO shows that its positive phases in recent decades have coincided with drier conditions in northern Mexico. A warmer tropical and subtropical Atlantic is negatively correlated with the intensity of the CLLJ, which in turn may modulate the formation of TCs over the Intra Americas Seas. The chances of TC formation over the IAS increase when the positive phase of the AMO is present (Goldenberg, 2001). However, it is the intensity of the moisture flux over northeastern Mexico that appears to be determinant of a dry or wet period over northeastern Mexico (Figure 6). An anomalously strong CLLJ induces more atmospheric humidity conditions. Although a stronger CLLJ inhibits the formation of TCs over the IAS due to vertical wind shear, it increases moisture flow and the intensity of precipitation, as has been observed in recent years.
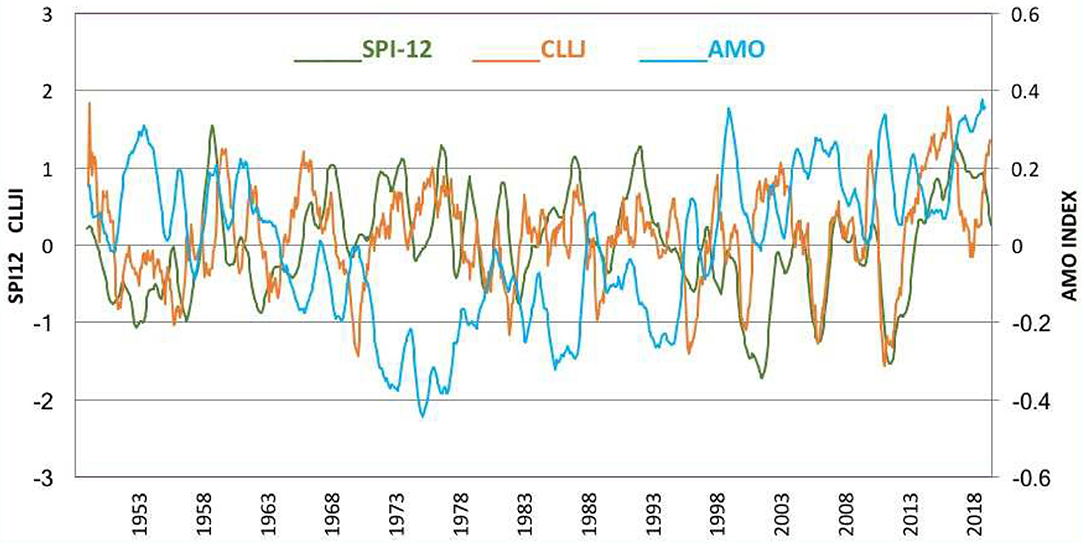
Figure 6. Time series of the smoothed (moving avg. 12 points) version of the: SPI-12 index around the MMA (green line) of the CLLJ index (red line) and the AMO index (blue line).
Given the recent decadal variations and long-term trends in atmospheric circulations in relation to the AMO and the CLLJ, it is possible that drier conditions will be observed in northeastern Mexico in the coming decades. The intensity of the CLLJ correlates with the phases of the AMO. A positive phase of the AMO tends to increase the chances of a weak CLLJ and more episodes of intense droughts in northeastern Mexico, only to be alleviated by the occurrence of a landfalling TC over the same region. However, predicting chances of landfalling TCs is more difficult than an outlook of the positive phase of the AMO. When the SPI-12 over northeastern Mexico is compared with the Caribbean Low-Level Jet Index (CLLJI) (Wang, 2007) it is observed that a weak (or intense) CLLJ is related to a dry (or wet) summer over the region of the MMA, in agreement with the results observed by Méndez and Magaña (2010). Therefore, the positive trend to a warmer Atlantic Ocean may result in higher chances of drought over NE Mexico in the coming years or decades with consequent impacts. The onset of a positive phase in the AMO appears to occur previous to a weakening of the CLLJ and the onset of the negative SPI-12 in northeastern Mexico, providing some elements of predictability of meteorological droughts. Closer re-analysis of very long-term AMO predictions (e.g., Wei et al., 2017) may generate better estimates of possible future prolonged droughts over the MMA, and, along with adequate vulnerability analyses (Neri and Magaña, 2016), the chances of socioeconomic droughts.
Water Availability and Management in the MMA
Under average conditions, a water balance in a semi-arid zone indicates that it is through sporadic intense precipitation events that water availability in the region (infiltration and runoff) increases. During prolonged meteorological droughts, as in 2011–2013, extreme precipitation events were scarce leading to drier conditions and a marked decrease in water availability. In Mexico, to avoid a socioeconomic drought when levels of the dam reservoirs drop, greater extractions of water by means of wells have been implemented. This has been the recurrent water management policy of the MMA (CONAGUA, 2014).
The supply of water for the MMA depends on both surface water from a system of dam reservoirs and ground water from numerous wells: 43 deep-wells between 700 and 1,000 m, 65 shallow-wells with depths of <100 m, as well as one infiltration gallery (SADM, 2012). La Boca dam, began operations in 1961 with capacity of 39.5 Mm3; Cerro Prieto dam, began operations in 1983 with capacity of 300.0 Mm3; and El Cuchillo dam, began operations in 1995 with capacity of 1,123.0 Mm3. The most important dam reservoir for water provision to the MMA is El Cuchillo. It was built in 1995, but the filling of this water reservoir was slow due to a prolonged and severe drought between 1998 and 2001 (Figure 7). Since then, large fluctuations in water availability in El Cuchillo have occurred mainly in relation to wet and dry periods, but also in relation to water management practices, such as transfers of water to the neighboring dam reservoir, Marte R. Gomez. In 2013, El Cuchillo levels dropped to levels that placed water supply for the MMA at risk. The need to reduce the risk of socioeconomic droughts in the MMA led to the development of public policies aimed at maintaining water provision (CONAGUA, 2014). However, rapid demographic and urban growth continues and has resulted in difficulties in responding to water demands, particularly during severe meteorological droughts. Water availability in dam reservoirs is a crucial element in reducing vulnerability to meteorological droughts (Mehran et al., 2015). Anomalous low levels in dam reservoirs or aquifers make a region more vulnerable to prolonged precipitation deficits (Neri and Magaña, 2016). Water transfers from the El Cuchillo dam reservoir to the Marte R. Gomez dam reservoir (which is fed by the San Juan river) (Figure 1) are a common practice, but during dry years, they may produce major drops in the water levels in the El Cuchillo dam reservoir (Figure 7), as happened in the late summer of 2013. When precipitation is more than 100 mm/month, there are important increases in water levels in the El Cuchillo and Marte R. Gomez dam reservoirs. However, after the onset of severe and prolonged drought, as in 2011, water levels in the El Cuchillo dropped from around 1,200 hm3 to around 800 hm3 in 2 years. In late 2012, in the middle of a severe drought, a transfer of water of around 500 hm3 to the Marte R. Gomez dam reservoir led to extremely low levels of water in the El Cuchillo dam reservoir (<200 hm3) that almost made the surface water supply system collapse. It was only the landfalling TC Ingrid, which brought more than 300 mm of rain in 3 days, that helped the system to recover to levels of around 800 hm3 by the end of that year. A steady recovery of the water levels in the El Cuchillo dam reservoir took place after that thanks to a period of positive anomalies in precipitation between 2014 and 2016.
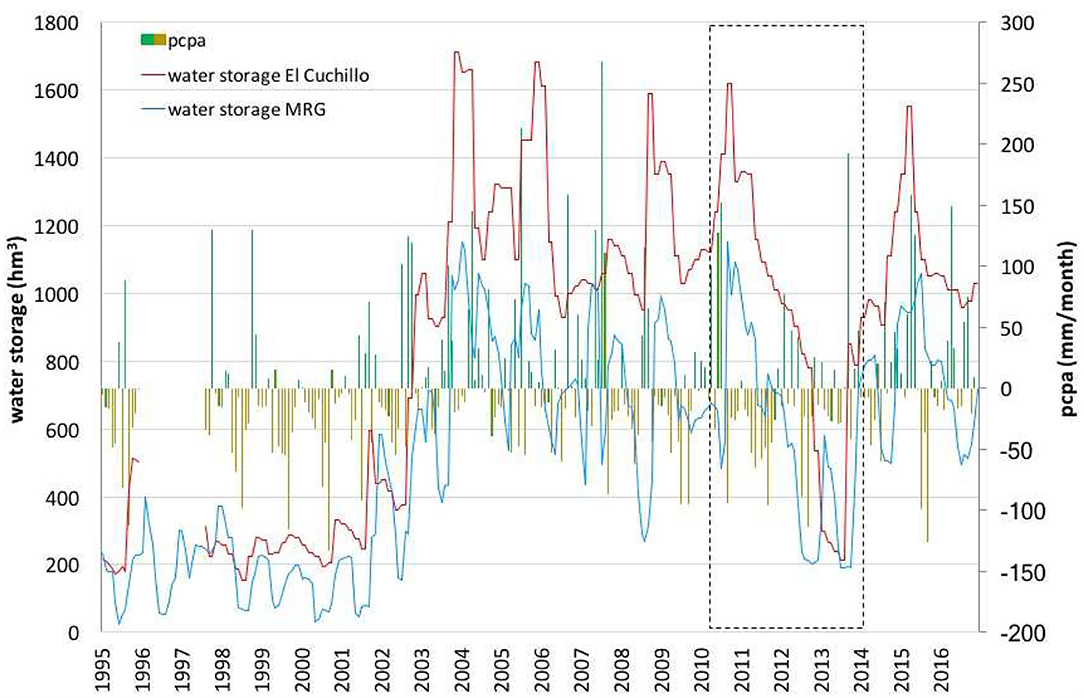
Figure 7. Monthly precipitation anomalies (mm/month) at El Cuchillo dam reservoir (green- brown bars) and monthly water storage (thousands of cubic meters) at El Cuchillo and Marte R. Gomez dam reservoirs, between 1995 and 2016. The dashed line box indicates the periods of a prolonged meteorological drought between the occurrence of landfalling TCs. (Data source: BANDAS, CONAGUA).
The mean annual water provision to the MMA is around 380 hm3/yr. Around 55% of that water is obtained from the system of dam reservoirs in the region, mostly from the El Cuchillo (64% of the dam reservoir system). In 2013, the low water storage in the El Cuchillo and in the other dam reservoirs almost resulted in a severe socioeconomic drought. The situation was avoided with increased groundwater exploitation. Using groundwater estimates from remote-sensing monitoring applications such as GRACE, it is possible to estimate the fluctuations in groundwater levels in the regional aquifer over the MMA. The Water Equivalent Thickness anomaly from a long-term average is understood from a review of the GRACE inferred observations of the sum of all the water storage compartments at and below the Earth's surface, including soil moisture, groundwater in shallow and deep aquifers, canopy water, and also the content of surface water bodies such as rivers, lakes and occasionally flooded wetlands. The impact of the 2011–2013 meteorological drought in the groundwater levels corresponded to a decrease of around 50%, which was twice as large as in previous years. The anomalously low levels of surface water led to increased pumping of water from the aquifer that, along with reduced recharge, resulted in a significant depletion of water in the aquifer. It was only thanks to the impact of TC Ingrid that a partial recovery of the aquifer took place (Figure 8). After Ingrid, ground water levels recovered but not to average values, which places the region in a highly vulnerable condition if a new severe or/and prolonged meteorological drought occurs.
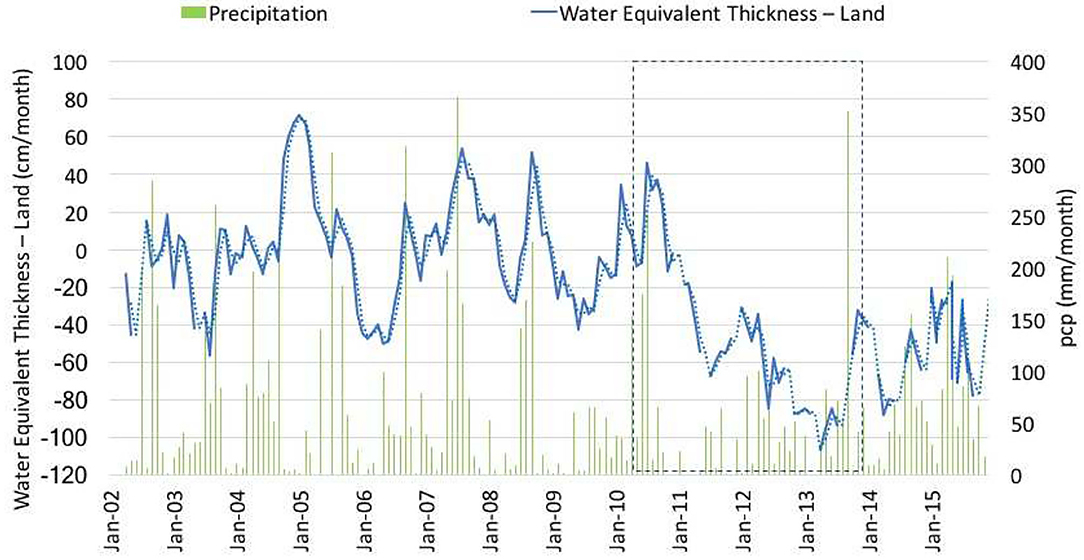
Figure 8. Water equivalent thickness (cm) over the MMA (Data GRACE-JPL). The dotted line corresponds to a smoothed version of the original data, as mean of interpolating missing data. The green bars correspond to monthly precipitation over the MMA. The dashed-line box indicates the periods of a prolonged meteorological drought between the occurrence of TCs.
At the urban level, water provision, consumption, treatment, and re-use have been increasing in recent decades along with rapid demographic growth. Since the second half of the twentieth century, the rapid increase in the demands for water have been met by construction of new hydraulic infrastructure such as dam reservoirs (Figure 9). Since the 1960s, part of the water supply for the MMA has been provided by large water reservoirs. The increasing water demands of recent decades are expected to be satisfied by new hydraulic developments in the region. However, during meteorological drought periods this source of water may become limited and more groundwater extraction will have to be used to compensate for the surface water deficit in dam reservoirs. Precipitation in the region exhibits very low frequency fluctuations throughout the decades. For instance, during the 1950s and early 1960s, northern Mexico experienced a severe prolonged drought period when evaporation levels were high. In the MMA during that period, annual precipitation was around 400 mm/yr and evaporation around 2,700 mm/yr, which implies larger losses of surface water. From the early 1960s until the late 1980s, wet periods were observed with annual precipitation of between 600 and 700 mm/yr and potential evaporation around 2,500 mm/yr. Since the mid-1990s until recently, a new dry period has been experienced, with intermediate and relatively short wet periods (2003–2005 and 2013–2015) and where potential evaporation was relatively low, 2,200–2,400 mm/yr. Maximum temperatures in the El Cuchillo dam reservoir region have not shown a trend to higher values in recent decades (not shown) and this may control evaporation. The existence of two large dam reservoirs in the region since 1983 and 1994 may be one factor causing the local temperatures to maintain within a range (28°-32°C from 1950 to 2018) and relatively low evaporation rates, factors which should be explored, since this could be relevant for the water balance in large reservoirs.
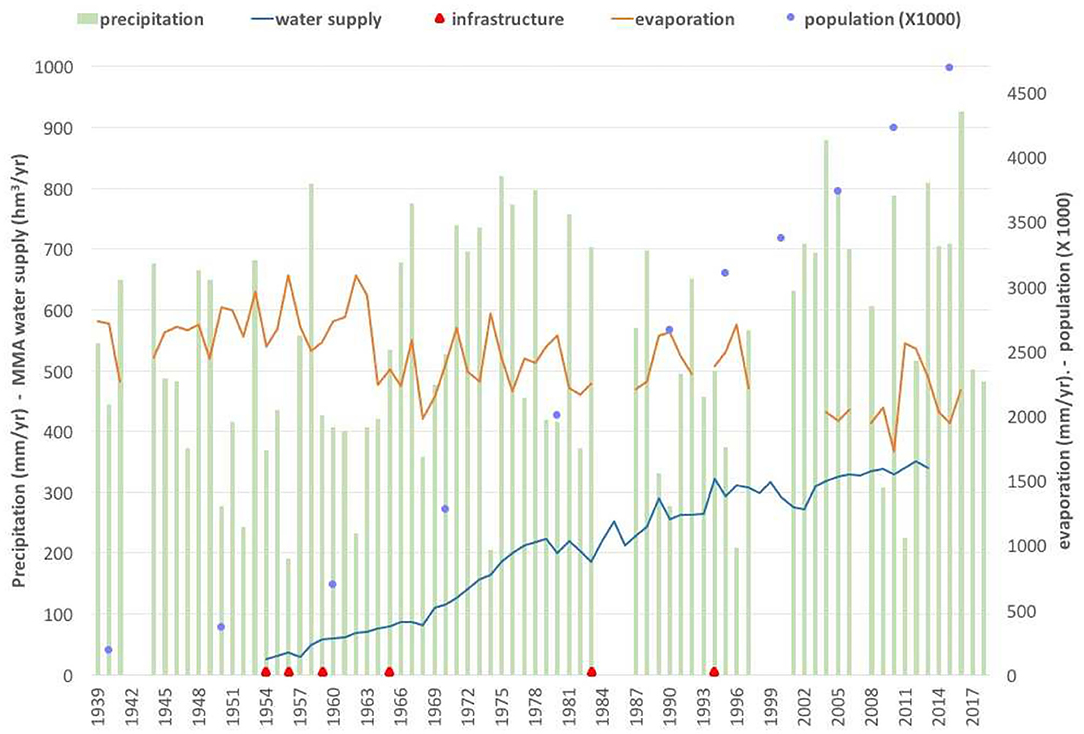
Figure 9. Annual water supply (hm3) (yellow line) surface water supply (red line) and groundwater supply (blue line) for the MMA. The green dots indicate population of the MMA and the purple dots correspond to years when hydraulic infrastructure was implemented for water supply for the MMA.
A management strategy that depends on surface water requires aquifers to be in good condition to make them a resilience factor in case of drought. Therefore, more attention should be given in developing plans for the use of surface and groundwater, considering the very low frequency variability of the climate, especially in relation to prolonged drought periods. In one possible planning strategy, the water provision system for the MMA could rely more on surface water during wet periods, thus allowing the aquifers to recover to average or above average levels. And during dry periods, water provision for the MMA would rely on groundwater, as has happened in recent decades.
During warm periods, domestic water consumption increases by 10–15% (Figure 2A). For instance, during summer, water demands in the MMA increase around 79.2 hm3 on average. This implies that water demands rise as a function of maximum temperature and therefore, it may be expected that in a warmer climate, the provision of potable water increases, since, regardless of the storage in water reservoirs, consumption will be higher. The rapid demographic and economic growth in the MMA, along with warmer temperatures due to climate change, have increased the demand for water and have led to the development of more hydraulic infrastructure. There are new hydraulic projects to maintain the provision of potable water to the urban area, since some consider that meteorological droughts constitute a major hazard that cannot be successfully managed with the current sources of water (CONAGUA, 2014). However, for those projects to be efficient and resilient, climate information schemes should be included during planning stages, mainly in relation to very low frequency climate variability and prolonged meteorological droughts.
Discussion and Conclusions
The recent experience of a severe prolonged drought during the 2011–2013 period, and the impacts on the water supply for the urban population have led water agencies to consider how climate change increases the risk of socioeconomic droughts. Current water management practices, including water transfers from one region to another, should be carefully examined in the coming years. Large scale water transfer projects such as those from the Panuco river, near the San Luis Potosí Huasteca region, could be only a partial solution to the potential impacts of climate change, particularly when meteorological droughts affect central and northern Mexico. This type of solution, under the paradigm of more water to offer instead of new water management strategies, may result in social conflicts with neighboring states. Even though the Water Agency of Monterrey has already implemented several water use efficiency measures, new local strategies in water management are necessary, such as the use of improved climate information for long-term planning. The climatic conditions of northeastern part of Mexico exhibit predictability on very long-term time scales, which may serve to better define water management strategies combining surface and ground water provision.
On interannual and interdecadal time scales, SST anomalies appear to modulate atmospheric circulations that reflect as enhanced subsidence larger low-level moisture flows over northern Mexico (Méndez and Magaña, 2010). This is in agreement with findings that show that in recent decades, atmospheric subsidence in subtropical regions appears to be more intense due to a stronger Hadley cell (Quan et al., 2004). This may result in more frequent periods of drought for northern Mexico which could be mitigated by the occurrence of intense precipitation events (Figure 10) (e.g., landfalling TCs). But, dependence on the occurrence of TCs to recover water levels in dam reservoirs could place the regional water system at risk of socioeconomic droughts. Seasonal climate predictions of climate change scenarios of landfalling TCs are extremely uncertain.
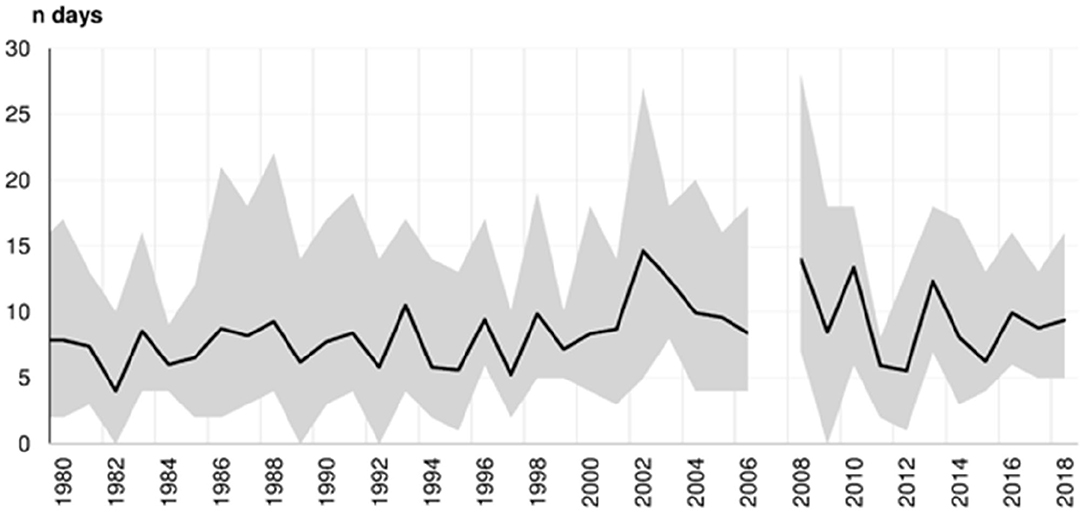
Figure 10. Average number of days per year with precipitation above 15 mm/day in the MMA. The shading corresponds to the range in the number of days in 17 weather stations in the MMA. The gap around 2007 corresponds to missing (incomplete) daily data in most stations in the region.
It is necessary to incorporate a component of drought risks in the region's water management systems. Monitoring and predicting the phases of the AMO and the intensity of the CLLJ may serve to improve water management practices. Meteorological droughts are slow-onset hazards, whose impacts depend on people's vulnerabilities (socioeconomic conditions, lack of strategies and plans, limited institutional capacities, and resources). Depending on the vulnerability context, an anomalously prolonged precipitation deficit may result in hydrological, agricultural, or socioeconomic droughts. Understanding these vulnerabilities to drought may allow government authorities and the public to undertake effective drought mitigation and preparedness measures.
In addition to more efficient water use practices, new alternatives to respond to the growing demands of water in the MMA should be considered: (i) water management planning cognizant of interannual and interdecadal climate variability, (ii) long term water planning considering the role of TCs as providers of water and the trends in the hydrologic cycle and climate change adaptation measures, and (iii) the use of seasonal and long-term climate prediction in a socioeconomic drought risk model. The consideration of such strategic measures may result in a new paradigm for water management in Mexico and other semi-arid regions. Water supply in a water crisis context is exacerbating social, economic, and environmental problems. The MMA could benefit from novel approaches in finding alternative sources of water that complement the existing ones. The advancement of water technology in the near future may include: groundwater artificial recharge (Jones, 2011), atmospheric water vapor processors for potable water production (Wahlgren, 2001), or improved refinement of water programs. The possibilities of reducing the water crisis in these Mexican regions are still ample but require accepting the existence of non-traditional solutions to the water crisis.
Data Availability Statement
The original contributions presented in the study are included in the article/supplementary material, further inquiries can be directed to the corresponding authors.
Author's Note
The problem of socioeconomic droughts in large cities in northern Mexico, a semi-arid region is acquiring more relevance in the Agenda of Water Agencies in Mexico, considering the growing water crisis in the country. Most water management strategies to deal with meteorological droughts focus on responding to the adverse climatic conditions, with a high economic cost. Climatic information is usually referenced to describe the adverse conditions in the water sector, but rarely used to prevent the negative consequences of meteorological droughts for instance. The present study analyzes the way in which very low frequency climate variability results in droughts in a semiarid region of Mexico, and how such knowledge could be used to prevent some of the negative consequences of droughts, for instance in large urban centers. The analysis shows that it is not only the long period fluctuations in climate, but also, the trends, probably related to climate change, what should be addressed by water managers in the near future, leading to potential adaptation measures at the regional level.
Author Contributions
All authors listed have made a substantial, direct and intellectual contribution to the work, and approved it for publication.
Funding
CONACYT Fondo: M0037FORDECYT 000000000297506 Seguridad hídrica en ciudades 001 DIAGNÓSTICO. EH was financially supported with CONACYT scholarship 208423.
Conflict of Interest
The authors declare that the research was conducted in the absence of any commercial or financial relationships that could be construed as a potential conflict of interest.
Acknowledgments
This study was financially supported by CONACYT (Mexico) under Grant FORDECYT 297506. The technical assistance of Gustavo Vázquez is appreciated. We are indebted to the Servicios de Agua y Drenaje de Monterrey I.P.D. (Monterrey Water Agency) for kindly providing information on water consumption in the MMA. We appreciate several valuable discussions with Aldo Ramirez, Ismael Aguilar, and Jurgen Mahlknecht.
References
Abu-Allaban, M., El-Naqa, A., and Jaber, M. (2015). Water scarcity impact of climate change in semi-arid regions: a case study in Mujib basin, Jordan. Arab. J. Geosci. 8, 951–959. doi: 10.1007/s12517-014-1266-5
Aguilar-Barajas, I., Sisto, N. P., Magaña-Rueda, V., Ramírez, A. I., and Mahlknecht, J. (2016). Drought policy in Mexico: a long, slow march toward an integrated and preventive management model. Water Policy 18, 107–121. doi: 10.2166/wp.2016.116
Amador, J. A. (1998). A climatic feature of the tropical Americas: the trade wind easterly jet. Top. Meteor. Oceanogr. 5, 91–102.
Center for the Research of Epidemiology of Disasters (CRED) (2015). The Human Cost of Weather Related Disasters: 1995-2015. The UN Office for Disaster Risk Reduction. Available online at: https://www.unisdr.org/files/46796_cop21weatherdisastersreport2015.pdf (accessed March 22, 2020).
CONAGUA (2014). Programa de Medidas Preventivas y de Mitigación de la Sequía, Ciudad de Monterrey, N. L., Organismo de Cuenca Río Bravo. 76pp (In Spanish). Available online at: https://www.gob.mx/cms/uploads/attachment/file/99851/PMPMS_Monterrey_NL.pdf (accessed June 12, 2020).
CONAGUA (2019). Información Estadística Climatológica. Gobierno de México (In Spanish). Available online at: Available online at: https://smn.conagua.gob.mx/es/climatologia/informacion-climatologica/informacion-estadistica-climatologica (accessed June 12, 2020).
CONAGUA (2020). Banco Nacional de Datos de Aguas Superficiales (BANDAS). Subdirección General Técnica. Gerencia de Aguas Superficiales. Available online at: http://www.conagua.gob.mx/CONAGUA07/Contenido/Documentos/Portada%20BANDAS.htm (accessed March 23, 2020).
Dominguez, C., and Magaña, V. (2018). The role of tropical cyclones in precipitation over the tropical and subtropical North America. Front. Earth Sci. 6:19. doi: 10.3389/feart.2018.00019
Eklund, L., and Seaquist, J. (2015). Meteorological, agricultural and socioeconomic drought in the Duhok Governorate, Iraqi Kurdistan. Nat. Hazards 76, 421–441. doi: 10.1007/s11069-014-1504-x
Enfield, D. B., Mestas-Nunez, A. M., and Trimble, P. J. (2001). The Atlantic Multidecadal Oscillation and its relationship to rainfall and river flows in the continental U.S. Geophys. Res. Lett. 28, 2077–2080. doi: 10.1029/2000GL012745
Goldenberg, S. B. (2001). The recent increase in Atlantic hurricane activity: causes and implications. Science 293, 474–479. doi: 10.1126/science.1060040
Herrera, E., Magaña, V., and Caetano, E. (2015). Air–sea interactions and dynamical processes associated with the midsummer drought. Int. J. Climatol. 35, 1569–1578. doi: 10.1002/joc.4077
Huang, S. Z., Huang, Q., Leng, G. Y., and Liu, S. Y. (2016). A nonparametric multivariate standardized drought index for characterizing socioeconomic drought: a case study in the Heihe River Basin. J. Hydrol. 542, 875–883. doi: 10.1016/j.jhydrol.2016.09.059
IPCC (2019). Climate Change and Land: an IPCC special report on climate change, desertification, land degradation, sustainable land management, food security, and greenhouse gas fluxes in terrestrial ecosystems, eds. P.R. Shukla, J. Skea, E. Calvo Buendia, V. Masson-Delmotte, H.-O. Pörtner, D. C. Roberts, P. Zhai, R. Slade, S. Connors, R. van Diemen, M. Ferrat, E. Haughey, S. Luz, S. Neogi, M. Pathak, J. Petzold, J. Portugal Pereira, P. Vyas, E. Huntley, K. Kissick, M. Belkacemi, J. Malley. In press.
Jones, A. (2011). “Sustaining groundwater resources: a critical element in the global water crisis,” in Institute of Geography and Earth, ed AA Jones (Springer), 228. doi: 10.1007/978-90-481-3426-7
Joyce, R. J., Janowiak, J. E., Arkin, P. A., and Xie, P. (2004). CMORPH: a method that produces global precipitation estimates from passive microwave and infrared data at high spatial and temporal resolution. J. Hydromet. 5, 487–503. doi: 10.1175/1525-7541(2004)005andlt;0487:CAMTPGandgt;2.0.CO;2
Kalnay, E., Kanamitsu, M., Kistler, R., Collins, W., Deaven, D., Gandin, L., et al. (1996). The NCEP/NCAR 40-year reanalysis project. Bull. Amer. Meteor. Soc. 77, 437–70. doi: 10.1175/1520-0477(1996)077<0437:TNYRP>2.0.CO;2
Krishnamurthy, V. (2019). Predictability of weather and climate. Earth Space Sci. 6, 1043–1056. doi: 10.1029/2019EA000586
Liu, S. N., Shi, H. Y., and Sivakumar, B. (2020). Socioeconomic drought under growing population and changing climate: a new index considering the resilience of a regional water resources system. J. Geophys. Res. Atmos. 125:e2020JD033005. doi: 10.1029/2020JD033005
Madani, K., AghaKouchak, A., and Mirchi, A. (2016). Iran's socio-economic drought: challenges of a water-bankrupt nation. Iranian Stud. 49, 997–1016. doi: 10.1080/00210862.2016.1259286
Magaña, V. (2016). Considerations for a research program on drought in Mexico. Tecnología y Ciencias del Agua. 6, 115–133. Available online at: http://revistatyca.org.mx/ojs/index.php/tyca/article/view/1274 (accessed June 23, 2020).
Magaña, V., Amador, J. A., and Medina, S. (1999). The midsummer drought over Mexico and Central America. J. Climate 12, 1577–1588. doi: 10.1175/1520-0442(1999)012<1577:TMDOMA>2.0.CO;2
Magaña, V., Neri, C., and Zermeño, D. (2012). Climate change scenarios and potential impacts on water availability in northern Mexico. Clim. Res. 51, 171–184. doi: 10.3354/cr01080
McCabe, G. J., Palecki, M. A., and Betancourt, J. L. (2004). Pacific and Atlantic Ocean influences on multidecadal drought frequency in the United States. PNAS 101, 4136–4141. doi: 10.1073/pnas.0306738101
Mehran, A., Mazdiyasni, O., and AghaKouchak, A. (2015). A hybrid framework for assessing socioeconomic drought: linking climate variability, local resilience, and demand. J. Geophys. Res. 120, 7520–7533. doi: 10.1002/2015JD023147
Méndez, M., and Magaña, V. (2010). Regional aspects of prolonged meteorological droughts over Mexico and Central America. J. Climate. 23, 1175–1188. doi: 10.1175/2009JCLI3080.1
Mesinger, F., DiMego, G., Kalnay, E., and Mitchell, K. (2006). North American regional reanalysis. Bull. Amer. Meteor. Soc. 87, 343–360. doi: 10.1175/BAMS-87-3-343
Monforte-García, G., Aguilar-Benitez, I., and González-Gaudiano, E. (2012). Limitaciones de una gestión sectorizada para la sustentabilidad del agua: Caso Monterrey, México. Revista Bitácora Urbano Territorial 20, 53–63.
Neri, C., and Magaña, V. (2016). Estimation of vulnerability and risk to meteorological drought in Mexico. Wea. Climate Soc. 8, 95–110. doi: 10.1175/WCAS-D-15-0005.1
NOAA (2019). NHC Data Archive. Available online at: https://www.nhc.noaa.gov/data/ (accessed July 20, 2020).
Quan, X., Diaz, H., and Hoerling, M. (2004). “Changes in the Tropical Hadley Cell since 1950,” in The Hadley Circulation: Present, Past and Future,. eds Henry F. Diaz and Raymond S. Bradley (Boston: Kluwer Academic Publishers), 85–120. doi: 10.1007/978-1-4020-2944-8_4
Rodell, M., and Famiglietti, J. S. (2001). An analysis of terrestrial water storage variations in Illinois with implications for the Gravity Recovery and Climate Experiment (GRACE). Wat. Resour. Res. 37, 1327–1340. doi: 10.1029/2000W.R.900306
SADM Servicios de Agua y Drenaje de Monterrey (2012). Proyecto Monterrey VI, Manifestación de Impacto Ambiental. Available online at: http://sinat.semarnat.gob.mx/dgiraDocs/documentos/nl/estudios/2012/19NL2012H0018.pdf (accessed March 23, 2020).
Schlesinger, M., and Ramankutty, N. (1994). An oscillation in the global climate system of period 65–70 years. Nature 367, 723–726. doi: 10.1038/367723a0
Shi, H. Y., Chen, J., Wang, K. Y., and Niu, J. (2018). A new method and a new index for identifying socioeconomic drought events under climate change: a case study of the East River basin in China. Sci. Total Environ. 616, 363–375. doi: 10.1016/j.scitotenv.2017.10.321
Singh, Naveen, P., Bantilan, C., and Byjesh, K. (2014). Vulnerability and policy relevance to drought in the semi-arid tropics of Asia – A retrospective analysis. Weather Clim. Extremes 3, 54–61. doi: 10.1016/j.wace.2014.02.002
Sisto, N., Ramirez, A., Aguilar-Barajas, I., and Magana-Rueda, V. (2016). Climate threats, water supply vulnerability and the risk of a water crisis in the Monterrey Metropolitan Area (Northeastern Mexico). Phys. Chem. Earth Parts A/B/C. 91, 2–9. doi: 10.1016/j.pce.2015.08.015
Sisto, N. P., Guajardo-Quiroga, R., and Aguilar-Barajas, I. (2011). Estimación de los impactos económicos de una sequía (Estimating the economic impacts of drought. Tecnología y Ciencias del Agua. 2:abril-junio. Available online at: http://www.revistatyca.org.mx/ojs/index.php/tyca/article/view/20/16
Sisto, N. P., and Ramírez-Orozco, A. I. (2015). “Flash floods in the Monterrey Metropolitan Area, Mexico, Lessons from Hurricane Alex,” in Water and Cities in Latin America: Challenges for Sustainable Development, ed I. Aguilar-Barajas (Abingdon: Routledge), 149–165.
Tu, X. J., Wu, H. O., Singh, V. P., Chen, X., Lin, K., Xie, Y., et al. (2018). Multivariate design of socioeconomic drought and impact of water reservoirs. J. Hydrol. 566, 192–204. doi: 10.1016/j.jhydrol.2018.09.012
UNISDR (2009). Drought Risk Reduction Framework and Practices: Contributing to the Implementation of the Hyogo Framework for Action. United Nations secretariat of the International Strategy for Disaster Reduction (Geneva), 213.
Velasco, I., Ochoa, L., and Gutiérrez, C. (2005). Sequía, un problema de perspectiva y gestión. Región Y Sociedad 17:34. doi: 10.22198/rys.2005.34.a615
Wahlgren, R. (2001). Atmospheric water vapor processor designs for potable water production. Water Res. 35, 1–22. doi: 10.1016/S0043-1354(00)00247-5
Wang, C. (2007). Variability of the caribbean low-level Jet and its relations to climate. Climate Dyn. 29, 411–422. doi: 10.1007/s00382-007-0243-z
Wei, M., Li, Q., Xin, X., Zhou, W., Han, Z., Luo, Y., et al. (2017). Improved decadal climate prediction in the North Atlantic using EnOI-assimilated initial condition. Sci. Bull. 62, 1142–1147. doi: 10.1016/j.scib.2017.08.012
Wilhite, D. A., and Glantz, M. H. (1985). Understanding the Drought Phenomenon: the role of definitions. Water Int. 10, 111–120. doi: 10.1080/02508068508686328
Keywords: water availability, dams, climate change, socioeconomic drought risk, meteorological drought
Citation: Magaña V, Herrera E, Ábrego-Góngora CJ and Ávalos JA (2021) Socioeconomic Drought in a Mexican Semi-arid City: Monterrey Metropolitan Area, a Case Study. Front. Water 3:579564. doi: 10.3389/frwa.2021.579564
Received: 02 July 2020; Accepted: 06 January 2021;
Published: 28 January 2021.
Edited by:
Haiyun SHI, Southern University of Science and Technology, ChinaReviewed by:
Zhaoqiang Zhou, Southern University of Science and Technology, ChinaNajiba Chkir, University of Sfax, Tunisia
Copyright © 2021 Magaña, Herrera, Ábrego-Góngora and Ávalos. This is an open-access article distributed under the terms of the Creative Commons Attribution License (CC BY). The use, distribution or reproduction in other forums is permitted, provided the original author(s) and the copyright owner(s) are credited and that the original publication in this journal is cited, in accordance with accepted academic practice. No use, distribution or reproduction is permitted which does not comply with these terms.
*Correspondence: Víctor Magaña, dmljdG9ybXJAdW5hbS5teA==