- 1International Crops Research Institute for the Semi-arid Tropics (ICRISAT), Addis Ababa, Ethiopia
- 2Department of Soil and Water Research, Sirinka Agricultural Research Center, Bahir Dar, Ethiopia
- 3Department of Plant Sciences, Woldya University, Mersa, Ethiopia
- 4Research Program on Resilient Farm and Food Systems, Ministry of Agriculture, Participatory Small Scale Irrigation Development Programme, Addis Ababa, Ethiopia
The threat of water scarcity in sub-Saharan Africa is exacerbated by the expanding agricultural water needs, increasing climate variability and inappropriate land use. It calls for technological and institutional innovations to improve water productivity, while sustaining the resources base. This study was undertaken to examine the effect of deficit and supplementary irrigation for staggered production of potato driven by market opportunities at different periods in northeastern Ethiopia. We used potato (Solanum tuberosum) to demonstrate AQUACrop as a tool for improving water productivity during Belg (short) and Meher (long) rainfall seasons. A field experiment was undertaken using supplementary irrigation at different levels of potato crop water satisfaction (50% ETc, 75% ETc, 100% ETc and rainfed conditions) during the Belg (February–May 2020) and Meher (July–October 2020) seasons. Upon proper calibration of AquaCrop for the local potato variety (Belete), long-term simulations revealed that the mean net irrigation requirements were 249 mm during Belg season while the probability of applying supplementary irrigation during Meher was <10% when the effective precipitation was greater than the crop water requirement (ETc) in more than 75% of the years. Although there was significantly higher potato tuber yield from the 100% ETc than that from the 75% ETc, the latter had higher water supply efficiency than the former. Long-term simulations further revealed that the number of rainfall days was more important than the amount of rainfall during the growing period. We engaged water users' associations to employ the recommendation and enforce supplementary irrigation as predicted by the model and we present farmers' response and reasons for resistance toward water saving approaches. Hence, we concluded that 50% ETc and 75% ETc irrigation levels can still be recommended upon proper scheduling to address long dry spells, especially during the middle growth stages in the face of irrigation conflict. However, the institutional settings and market incentives associated with it are the major drivers of adopting improved irrigation water management practices.
Introduction
Irrigation has the potential to boost agricultural productivities in Africa by at least 50 percent (You et al., 2010). Despite the centuries long traditions of using traditional irrigation systems such as spate irrigation in Africa, irrigated agriculture accounts only about 6% of the total cultivated lands until recently. The total irrigated land in Sub-Saharan Africa (SSA) has been only 17% of the total potential irrigated land, thus implying a huge potential yet to be exploited for irrigated food production (Tilman et al., 2011). Comprehensive Africa Agriculture Development Programme (CAADP) consider irrigation as one of the key pillars of agricultural development to address food security and rural poverty. Accelerated irrigation investment in SSA can effectively reduce growing food import dependency from about 54% to as much lower as 17%, thus also reducing the population at risk of hunger and child under-nutrition (Xie et al., 2018). However, the threat of water scarcity in sub-Saharan Africa is exacerbated by the expanding agricultural water needs, increasing climate variability and inappropriate land use. Gnacadja (2013) reported that there will be expansion of drylands and desertification in Africa due to the prevalent climate changes, thus exacerbating water scarcity. The FAO (2013) estimated that by 2030, up to 250 million people in Africa will be living in areas of serious water scarcity, which is likely to displace up to 700 million people as conditions become increasingly unlivable. Hence, it requires technological and institutional innovations to improve water productivity, while sustaining the resources base.
Ethiopia has a considerable water resources potential with about 124.4 billion cubic meter (BCM) river water, 70 BCM lake water, and 30 BCM groundwater resources (Berhanu et al., 2014). Given this huge physical water resources potential, irrigation has recently been considered as the corner stone of the agricultural development in Ethiopia, potentially contributing up to USD 5 billion to the economy and potentially moving up to 6 million households into food security (Awulachew et al., 2005; Wale et al., 2013). Since the 1990's, several small-scale irrigation schemes with irrigation command areas of up to 200 ha area have been established through the support of several bilateral organizations such the International Fund for Agricultural Development (IFAD). Moreover, traditional irrigation schemes have been upgraded. Amede (2015) reported some case examples of community-managed irrigation schemes that have been upgraded from traditional to improved water diversion and distribution systems. However, most of the community-managed irrigation schemes have very low overall performances due to lack of the required technical capacity, institutional challenges and market related risks in Ethiopia (Awulachew and Ayana, 2011; Amede, 2015) and elsewhere in Africa (Bjornlund et al., 2017).
Potato (Solanum tuberosum) is the world's third most important food crop after wheat and rice (Birch et al., 2012). Due to its wider production potential in Ethiopia, there is an increasing trend of potato production and value chain developments, with the production area increased from 62,000 ha to 296,578 ha and annual production increased from 500,000 tons to 3.6 million tons from 2006 to 2016, respectively (CSA (Central Statistical Authority), 2016; FAOSTAT, 2016). Potato offers opportunities as one of the main food security crops in Ethiopia to respond to the rapid population growth (Haverkoert et al., 2012). The crop has a very good prospect in value addition as the urban consumers are diversifying their consumption patterns including branded and packed fresh potatoes, chips and crisps, among others (Haverkoert et al., 2012). Despite the huge potato production potential in the Ethiopian highlands, diseases such as late blight (caused by Phytophthora infestans) and bacterial wilt (caused by Ralstonia solanacearum) are among the major constraints of potato production (Damtew et al., 2018). Smallholder farmers in many parts of the country tried to cope with the diseases by shifting the production from the long rainy season (Meher) to the short rainy season (Belg) (Abdurahman et al., 2017; Damtew et al., 2018). However, serious water stress associated with unreliability of the rainfall during the Belg season has affected potato yield and hence, smallholder farmers are reluctant to grow potato during this season in rainfed systems. The lack of ample production at the end of the Belg season has also caused increased potato prices. Increasing interest to grow potato during the dry seasons to avoid disease and the concomitant challenge of water scarcity at the same period is calling for improved irrigation water management to scale out irrigated potato production in the Ethiopian highlands. Therefore, a combination of long-term agro-meteorological and irrigation water requirement analyses in full and supplemental irrigation are required to determine the amount of irrigation water required for market-oriented potato production system.
Improved irrigation water management based on long-term agro-meteorological analyses requires simple and replicable decision support tools for development practitioners, local researchers, and policy makers. Accordingly, the effects of different irrigation agronomy management techniques and planting periods on yield and water use efficiency should be understood for effective decisions. The FAO's AquaCrop model is one of the most important and widely applicable models to simulate the effects of different irrigation agronomic management practices on crop yields and water use efficiency since the last 10 years (Steduto et al., 2009, 2012). The model requires several parameters and input data to simulate yield response to water for most of the major field crops cultivated worldwide (Steduto et al., 2009, 2012). The model has been aptly calibrated for Mediterranean climate in Spain and could aptly simulate tuber yield, biomass and water productivity values (Montoya et al., 2016). It has also been calibrated and validated under temperate climates (Razzaghi et al., 2017). Upon proper calibration and validations of the model, it has been used to optimize irrigation water application against potato yield at different parts of the world, where some of the calibrated parameters were slightly different (García-Vila and Fereres, 2012; Linker et al., 2018). However, the model has not been previously calibrated and used for simulations of rainfed and irrigated potato in the main potato producing areas of Ethiopia. Therefore, the objective of this study was to conduct long-term simulations and understand the effects of different sowing periods, rainfall patterns and irrigation levels on crop water satisfaction, yield and water use efficiency of potato. Farmers' responses have also been documented to model-based recommendations and facilitate adoption of improved irrigation water management principles.
Materials and Methods
Description of the Study Area
This study was undertaken in Northeastern Ethiopia at Barneb 2 community-managed irrigation scheme geographically located at 10.854 Latitude and 39.393 longitude (Figure 1). The study area has a mean altitude of 2,910 m above sea level. It has a humid agro-climate with an aridity index (ratio of mean annual precipitation to mean annual reference evapotranspiration) of 0.79 and bimodal rainfall pattern (Figure 2). The monthly rainfall is characterized by very high annual variability, with a coefficient of variability of >200% during the dry months (October–January). The mean daily minimum temperature varied between 5oC (December)−12oC (June) while the mean daily maximum temperature varied between 19oC (August)−24oC (May). The soils of the study site are dominated by vertisols with a dominant soil textural class of clay loams. The soil water properties of the area are presented in Table 1.
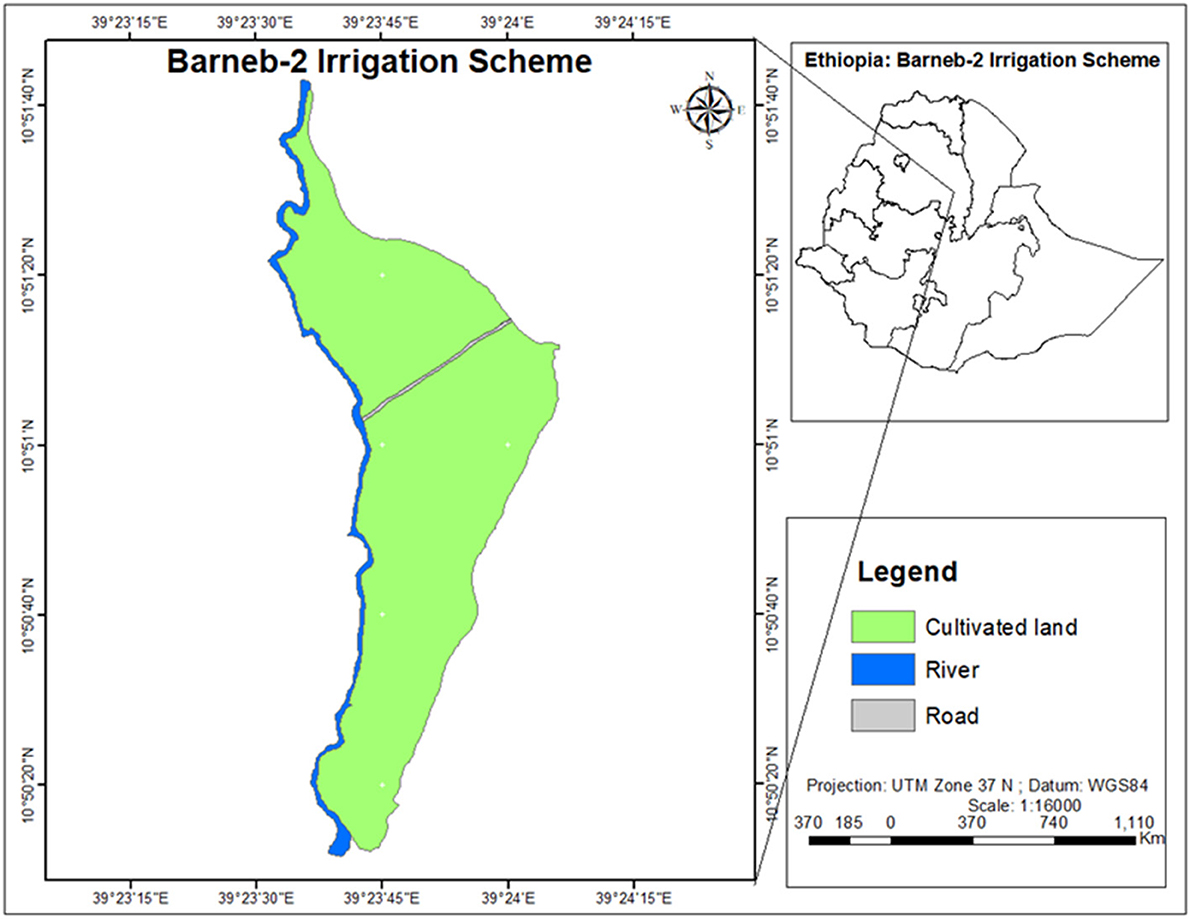
Figure 1. Location of the Barneb 2 community-managed small-scale irrigation scheme in Northeastern Ethiopian highlands.
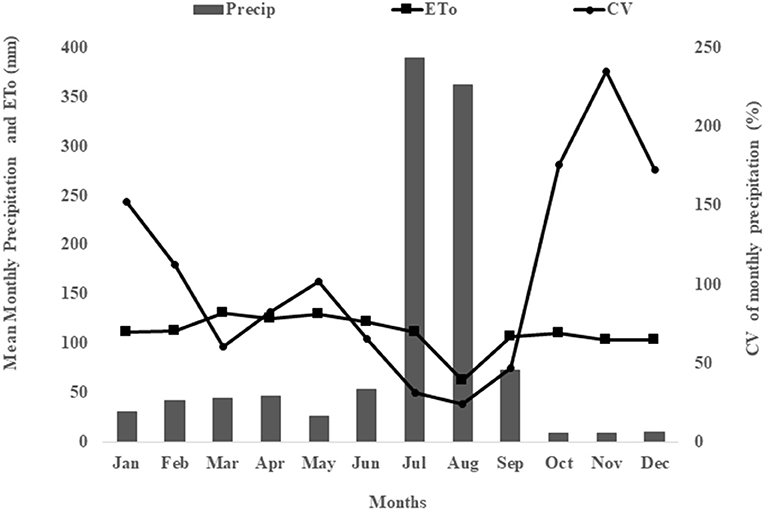
Figure 2. Mean monthly precipitation, reference evapotranspiration, and Coefficient of Variability computed from long-term daily meteorological data during 2001–2020 for Barneb 2 small-scale irrigation scheme in Northeastern Ethiopia.

Table 1. Soil physical properties of the experimental fields at Barneb 2 small-scale irrigation scheme in Northeastern Ethiopia.
Being one of the recently built small-scale irrigation schemes, the Barneb 2 community managed small-scale irrigation scheme was constructed by the Federal Government of Ethiopia in 2012 through the financial support of the International Fund for Agricultural Development (IFAD). Although it was designed by diverting water from Barneb perennial river to irrigate 160 ha, it is currently irrigating only about 107 ha which is managed by about 592 farmers, 111 of whom are women-headed. Irrigation is mainly applied during January/February–May/June as the farmers are trying to escape frost damages during October-December. Yet, there is growing challenge in terms of accessing enough irrigation water due to competing interests among farmers residing at both upstream and downstream of the Barneb 2 scheme.
Description of AquaCrop and Test of transferability
Model Description and Data Inputs
The FAO's AquaCrop model evolved from the approach to simulate crop yields in response to water (Doorenbos and Kassam, 1979) to a methodology for assessing the effects of water stress on yield and water use efficiency (Hsiao et al., 2009; Raes et al., 2009; Steduto et al., 2012). AquaCrop uses only the productive transpiration (Tr) and a normalized water productivity (WP*, biomass per unit of cumulative transpiration, g/cm3) to estimate the total crop biomass (Steduto et al., 2009; Eq. 1). It employs WP* as a conservative parameter (Steduto et al., 2007) by adjusting the crop water productivity for a given crop in each climatic condition. A harvest index (HI) is used to estimate the harvestable yield (Y) from the total biomass (B) using Eq. 2. The model depends on canopy progression and senescence model as the basis for the estimate of Tr and its separation from evaporation (E) (Raes et al., 2009; Steduto et al., 2009).
where Tr is crop transpiration (in mm) and WP* is water productivity parameter in units of kg (biomass) m−2 (land area) mm−1 (mm of cumulated water transpired over the time period in which the biomass is produced).
AquaCrop is organized based on the soil–crop–atmosphere continuum, thus encompassing (i) the soil, with its water balance; (ii) the plant, with its growth, development, and yield processes; and (iii) the atmosphere, with its thermal regime, rainfall, evaporative demand, and carbon dioxide concentration (Hsiao et al., 2009; Raes et al., 2009; Steduto et al., 2012). Therefore, the model uses climate, crop, irrigation, soil, field management, and initial soil water conditions as inputs. The user should calibrate the soil fertility level of the study area. The climate files encompass (i) minimum and maximum air temperature, (ii) reference evapotranspiration (ETo) which can be estimated from daily temperature, solar radiation, relative humidity and wind speed, (iii) rainfall, all daily values, and the CO2 concentration which can be found as the default value of Mauna Loa Observatory records in Hawaii (Steduto et al., 2009). The daily climate data for the period 2001–2020 was imported for long-term simulations in this study. Hence, the daily ET0 could be computed based on the FAO PenMan Montheith equation as described in detail in Allen et al. (1998). As the AquaCrop model uses crop input file of both conservative and user-specific parameters, some of the latter are cultivar specific. Table 2 presented the conservative and user-specific crop parameters for potato used in this study.
The irrigation menu enables the user to determine the net irrigation and suggest different levels (full and deficit irrigation) in different schedules. Runoff was described with a curve number (CN) value of 77 based on the soil hydrologic unit, topography and traditional tillage in the study area (USDA, 1964). The essential input soil parameters were determined for the study area (Table 1) and they were used for all historical simulations. For the historical simulations, the initial soil water content was determined based on onset of the rainfall season for a given year. AquaCrop enables users to apply user-specific criteria for determination of onsets.
Model Calibration and Validation
FAO's AquaCrop model was previously parameterized for potato and had been used in different localities for simulations of rainfed and irrigated potato production systems (Montoya et al., 2016; Razzaghi et al., 2017). In this study, the model calibration and validation were undertaken for a local potato variety (belete) which is currently widely grown in Ethiopia due to its high yield, better dry matter content and good market demands (Abebe et al., 2013). The calibration of soil fertility followed the procedure by FAO (2009a). During the Feb 22–June 21, 2020 experimental season, there were two types of experiments. The first experimental plots of 3.5 m X 3 m were set in the field with two treatments and three replications mainly to calibrate the FAO's AquaCrop model. The two treatments are one with an optimum fertility level (reference field) and another one without fertilizer (calibration field). For the reference field, the recommended fertilizer rate and about 15 tons/ha rate of cattle manure was applied, and nothing was applied on the calibration fields. Water was applied regularly to keep the soil water content near field capacity in both the reference and calibration fields. The second experimental plots of 3.5 m X 3 m were set up in four irrigation treatments. These irrigation treatments satisfy different levels of the potato crop water requirement (100% ETc, 75% ETc, 50% ETc and 0% ETc). The 0% ETc represented the non-irrigated potato field which was entirely dependent on the natural rainfall (Table 3). Each treatment was replicated four times, resulting in a total of 16 plots (4 treatments X 4 replications).

Table 3. Irrigation treatments set up during the field experiment (Feb 22–June 22, 2020) at Barneb 2 small-scale irrigation scheme in Northeastern Ethiopia.
The irrigation amount and scheduling were determined based on the procedure previously used by Leskovar et al. (2012) and Assefa et al. (2016). Hence, the amount and frequency of irrigation was determined based on daily potato crop evapotranspiration (ETc) for a well-watered crop, that is the product of the daily reference evapotranspiration (ETo) and potato crop coefficient (Kc) at different developmental stages (Eq. 3; Allen et al., 1998).
The FAO ETo calculator Version 3.1 was employed to estimate the daily values of the reference evapotranspiration (ETo) from the observed daily values of relative humidity and maximum and minimum temperature (FAO, 2009b). In this study, only the actual daily rainfall, relative humidity, and minimum and maximum temperature values were measured from an adjacent meteorological station previously installed by Sirinka Agricultural Research center. Moreover, a conventional rain gauge and thermometer were installed as part of the experiment for verifications of the data. However, solar radiation and wind speed were not measured. As Biazin and Stroosnijder (2012), we estimated the solar radiation using ETo calculator based on the latitude, longitude and elevation values that were given as inputs to the software (FAO, 2009b). A similar approach was previously followed for the estimation of ETo without using solar radiation (Biazin and Stroosnijder, 2012). Once the 100% ETc for the unstressed fields was determined, the stressed values of ETc (75% ETc and 50% ETc) were determined accordingly as percentages of the actual ETc. The actual irrigation amounts were, therefore, the results of the differences between ETc and the effective precipitation. The calculation of the effective precipitation followed the USDA procedure (USDA, 1970; SCS, 1993). Figure 3 depicted the climatic parameters and irrigation amount during the two experimental seasons, Feb 22–June 22 and July 10–Nov 10, 2020.
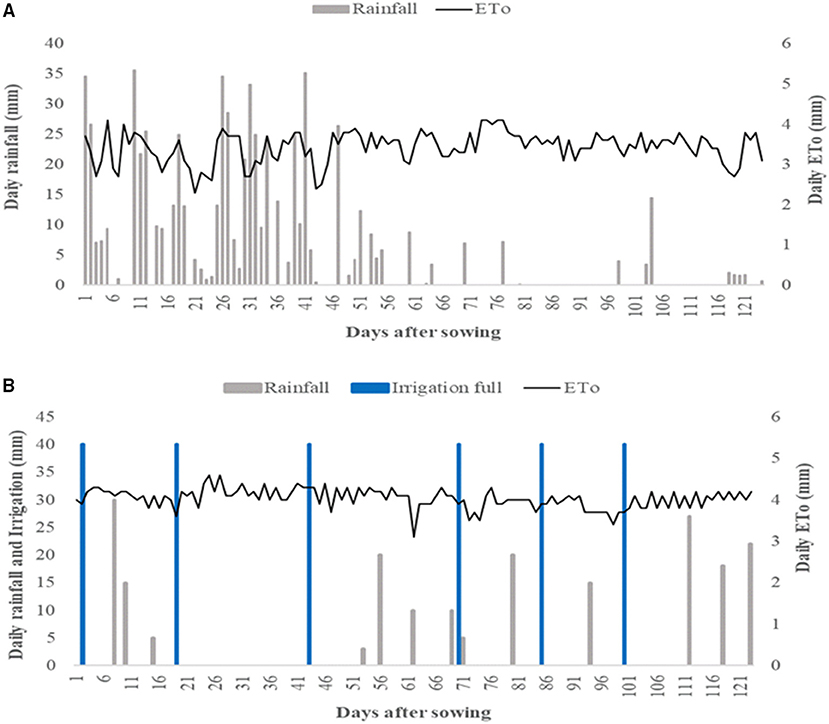
Figure 3. Daily precipitation, reference evapotranspiration (ETo) and applied irrigation (100% ETc, mm) during the cropping season Feb 22–June 21, 2020 (A) and July 10–Nov 10 (B) at Barneb 2 small-scale irrigation scheme in Northeastern Ethiopia.
Crop data collected includes phenological characteristics (user-specific parameters, Table 2), canopy development and yield parameters. These parameters were collected from the calibration and validation experimental fields where potato was planted in locally recommended spacing of 0.6 m between rows and 0.4 m between plants in a row. The biomass harvested at the end of the crop cycle from the calibration field expressed as a percentage of the biomass from the reference field was considered as an expression of the soil fertility level (FAO, 2009b). FAO (2009a) suggested potato water productivity normalized for ETo and CO2 (gram/m2) in the ranges of 14–18. However, Montoya et al. (2016) used Wp* value of 19 in Spain. For the early maturing local variety, we have managed to properly simulate the yield at a Wp* value of 16.
As part of the model validation, Root Mean Square Error (RMSE, Eq. (4), percent deviation (Dev. %, Eq. 5) and coefficient of determination (R2) were determined for biomass and tuber yields as previously used by Montoya et al. (2016) for potato and Biazin and Stroosnijder (2012) for maize.
where Si and Mi are the simulated and measured values, respectively, and n is the number of observations. The model fit improves as RMSE approaches zero. The unit for RMSE is the same as that for Si and Mi.
Moreover, percent deviation (Dev%) was used (Eq. 3).
Simulating Potato Water Satisfaction From Natural Rainfall, Net Irrigation Required and Yields in Response to Long-Term Climate at Different Growing Seasons
Upon proper calibration and validation, the AquaCrop model was employed to simulate potato yield in response to rainfed and irrigated potato production following successful planting dates during both the Belg (January/February–May/June) and Meher (Jun/July–Sep/October) seasons. Accordingly, 20 years daily climate data (2001–2020) representing the study area was collected from the National Meteorological Agency. Therefore, the effects of different sowing periods, rainfall amount and number of rainfall days during the growing period (counting between planting and harvesting periods) on the yield and water use efficiency of potato was simulated. The successful planting dates also known as successful onset of the rains was previously defined for different crops in Ethiopia (Raes et al., 2004; Tesfaye and Walker, 2004; Araya and Stroosnijder, 2011; Biazin and Stroosnijder, 2012) or elsewhere in Africa (Sivakumar, 1988; Barron et al., 2003; Raes et al., 2004). Therefore, the successful planting dates or onsets for potato was defined through a combination of the aforementioned literature and discussions with the local farmers, and field observations during 2019 and 2020 experimental seasons. Hence, a sowing date for potato during the occasion after January 1 was determined when the rainfall accumulated in six consecutive days is at least 20 mm (<0.85 mm d−1). Given that there can be one or more sowing dates per season; one or more simulations were made for onsets between January 1 and February 28/29 for the Belg season and between June 1 and July 31 for the Meher season. Hence, up to 4 consecutive sowing dates were determined per season per year using AquaCrop thus making the first sowing date as the initial search date for the criterion. Similar approaches were undertaken previously in Ethiopia (Biazin and Stroosnijder, 2012) and elsewhere in the world (Geerts et al., 2010; Mhizha, 2010). The total rainfall, number of rainfall days and net irrigation requirements determined for each growing length that encompassed the 123 days between the date of onset as simulated by the model and harvest in the historical simulations. Accordingly, potato tuber yield and water use efficiency were simulated under both rainfed (without any irrigation) and irrigated (irrigation applied to supplement the natural rainfall) conditions for the historical simulations of both the growing seasons. The water use efficiency under rainfed (WUErainfed) conditions (was computed based on the simulated tuber yield and amount of evapotranspired water (ETc) during the growing period (Eq. 6). Therefore, this was undertaken for both the small and main rainfall seasons.
where Y is the simulated tuber yield without irrigation during the potato growing period and ETc is the amount of water evapotranspierd during the growing period.
Finally, the water supply efficiency (WSE) of the supplemental irrigation system was computed as follows (Eq. 7). This was, therefore, undertaken for both the full (100%ETc) and deficit (75%ETc) irrigation conditions.
Pi is the production increase (kg) due to supplemental irrigation and calculated as the difference between the yield from the rainfed and irrigated conditions. Iw is the irrigation water applied (m3) during the whole crop growing season.
Identifying Incentive Mechanisms and Barriers of Adoption for Deficit Irrigation
A detailed household survey was undertaken with about 30 households that represented about 5% of the small-scale irrigation user households and who are members of the irrigation water users' associations (IWUA) at Barneb 2 irrigation scheme. Hence, the perceptions of farmers and their constraints in adapting partial irrigation and employing improved water management in potato farming could be assessed. The assessment focused on identifying the major technical, institutional and market-related incentives and constraints and solicit the priority areas of interventions to enhance water productivity at farm and scheme scales. Moreover, using focus group discussions, qualitative data was collected on perceptions and constraints from 24 irrigation users, facilitated in two groups of 12 persons each. The qualitative and quantitative assessments enabled to prioritize the major biophysical, technical, institutional and market related challenges.
Results and Discussions
Model Calibrations and Validations
The AquaCrop model was aptly calibrated for adjustment of some user-specific parameters of potato based on canopy progression determined from a reference field (Figure 4). Many of the cultivar-specific parameters such as time from sowing to senescence and harvest, maximum canopy cover, and harvest index have been aptly determined from the experimental fields. Parameters such as conservative water productivity, root zone expansion, canopy decline coefficients and soil fertility levels were adjusted and calibrated to fit the model (Table 2). There was a very good agreement between the measured and simulated canopy cover values for both the reference (non-stressed) and calibrated (stressed) fields (Figure 4). The coefficient of determination (R2) between the observed and simulated values of canopy cover was 0.99 for the reference field and 0.97 for the calibrated field, respectively, implying the best fit to determine the calibrated parameters. The ratio of the total biomass from the calibrated field to that from the reference field was 0.69 implying that the fertility stress of the study area was 31%. Upon proper calibrations, the potato crop parameters for the specific variety were within the ranges recommended by FAO (2009).
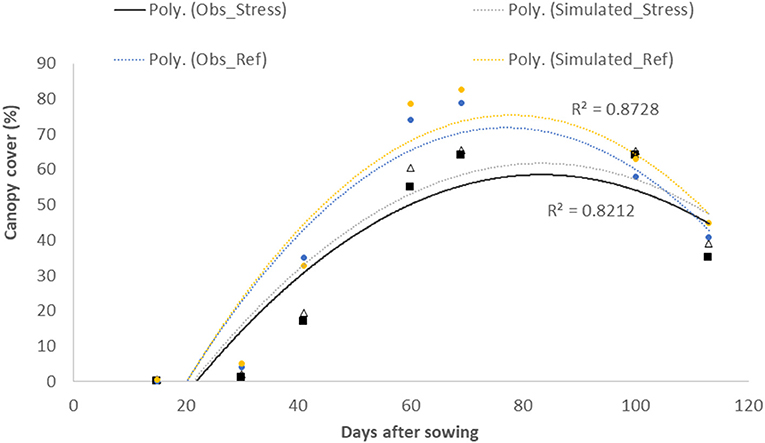
Figure 4. Simulated and observed potato canopy cover progression under a non-stressed fully irrigated and fertilized reference fields (RF) and non-fertilized but fully irrigated calibration fields (stressed calibration field) during 2010 at Barneb 2 Irrigation Scheme in Northeastern Ethiopia. The markers indicate the dates when canopy cover was measured for both the reference fields (Ref) which were fully fertilized with optimum irrigation and stressed fields (stress) which were optimally irrigated but not fertilized. The trend lines indicate the polynomial lines that best fit canopy development with developmental stages.
The total fresh tuber and biomass yields of potato were appropriately simulated for both irrigated (Feb-June, 2020) and rainfed (July–Nov, 2020) conditions as implied by the acceptable ranges of RMSE and Dev% (Table 4). The RMSE and percent deviation values were in comfortably acceptable ranges which are not far from previous simulations in different agro-climatic conditions elsewhere in the world (Montoya et al., 2016; Razzaghi et al., 2017). The model could appropriately simulate the effect of the serious cold stress on tuber yield of potato. The potato tuber yield obtained from the rainfed system during the Meher season was only about 34% of the yield obtained from the Belg season (Table 4). This is mainly attributed to high incidence of frost damage during the former as it was manifested by the severe defoliation of leaves at the maturation stage. The local farmers have seriously complained about the high risk of frost for many crops including potato. Frost can cause substantial loss of foliage, leading to a reduction in photosynthate production and hence yield (Francois et al., 1999; Condori et al., 2014).
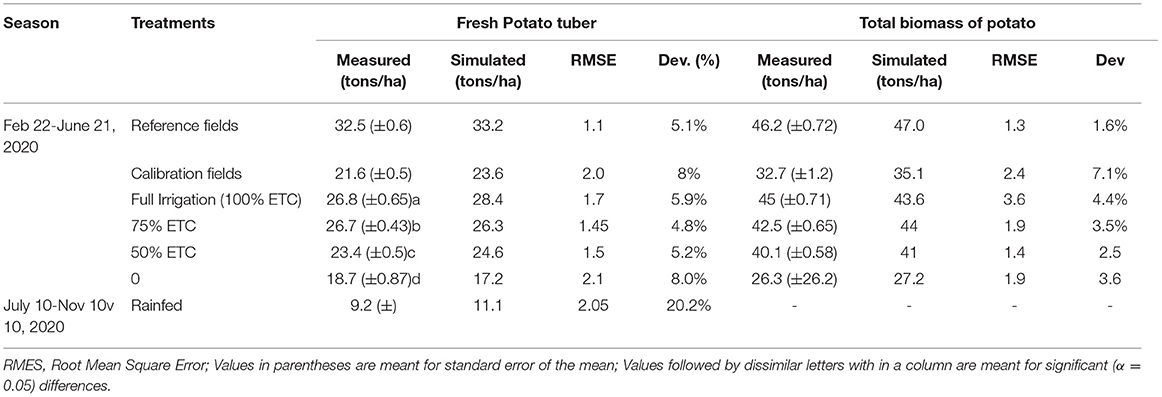
Table 4. Simulated and measured values of potato tuber yield and total biomass from experimental plots during Feb 22–June, 2020 and July 10–Nov 10, 2020 at Barneb 2 irrigation Scheme in northeastern Ethiopia.
Simulated Crop Water Satisfaction, Net Irrigation Requirement and Yield in Response to Different Rainfall Patterns and Growing Seasons
The Potato Water Satisfaction From Rainfall and Net Irrigation Water Requirements During the Small and Main Rainfall Seasons
The results of long-term historical agro-meteorological analyses revealed that the precipitation following planting of potato at the onset dates during January and February (Belg season) can supply only 44% of the potato water requirement, implying that more than half of the water requirement should come from irrigation (Table 5), along with improved water management interventions. On the other hand, the probability of sowing potato following successful onsets during Jan-February was 47%, implying that during 53% of the years the smallholder farmers should depend on irrigation at the time of sowing before the end of February. When the sowing date is pushed from January to April, the total amount of rainfall during the potato growing period significantly (R2 = 0.8) increased while the net irrigation requirement moderately decreased (R2 = 0.3) (Figure 5A). However, sowing of potato after the end of February has two disadvantages: (1) the farmers will miss the good market around June and beginning of May; (2) planting of second rotational crops during June and July will not be possible thus pushing planting to August and September which is literally impossible in the area due to end of season drought, and severe frost during October–December. The net irrigation requirement is very low while growing of potato during June/July-September/October (Figure 5B) although there is a serious challenge of frost especially after September thus substantially reducing the yield. Previous studies elsewhere in Ethiopia indicated that the Belg rainfall is less predictable and dependable (Tesfaye and Walker, 2004; Assefa et al., 2016; Gummadi et al., 2018).
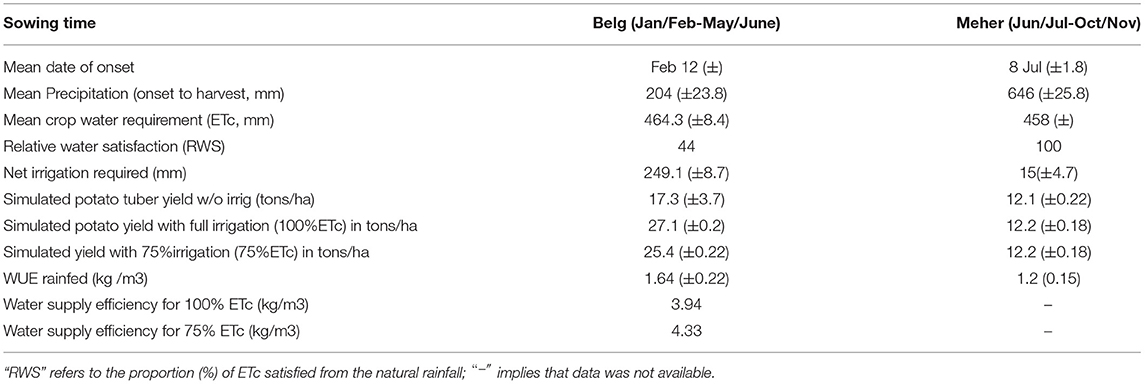
Table 5. Mean precipitation, water requirement (ETc), relative water satisfaction (RWS) from the natural rainfall based on long-term weather data (2001–2020) and net irrigation requirement of the potato crop during the small rainfall (Belg) and main rainfall (Meher) seasons at Barneb 2 small-scale irrigation scheme in Northeastern Ethiopia.
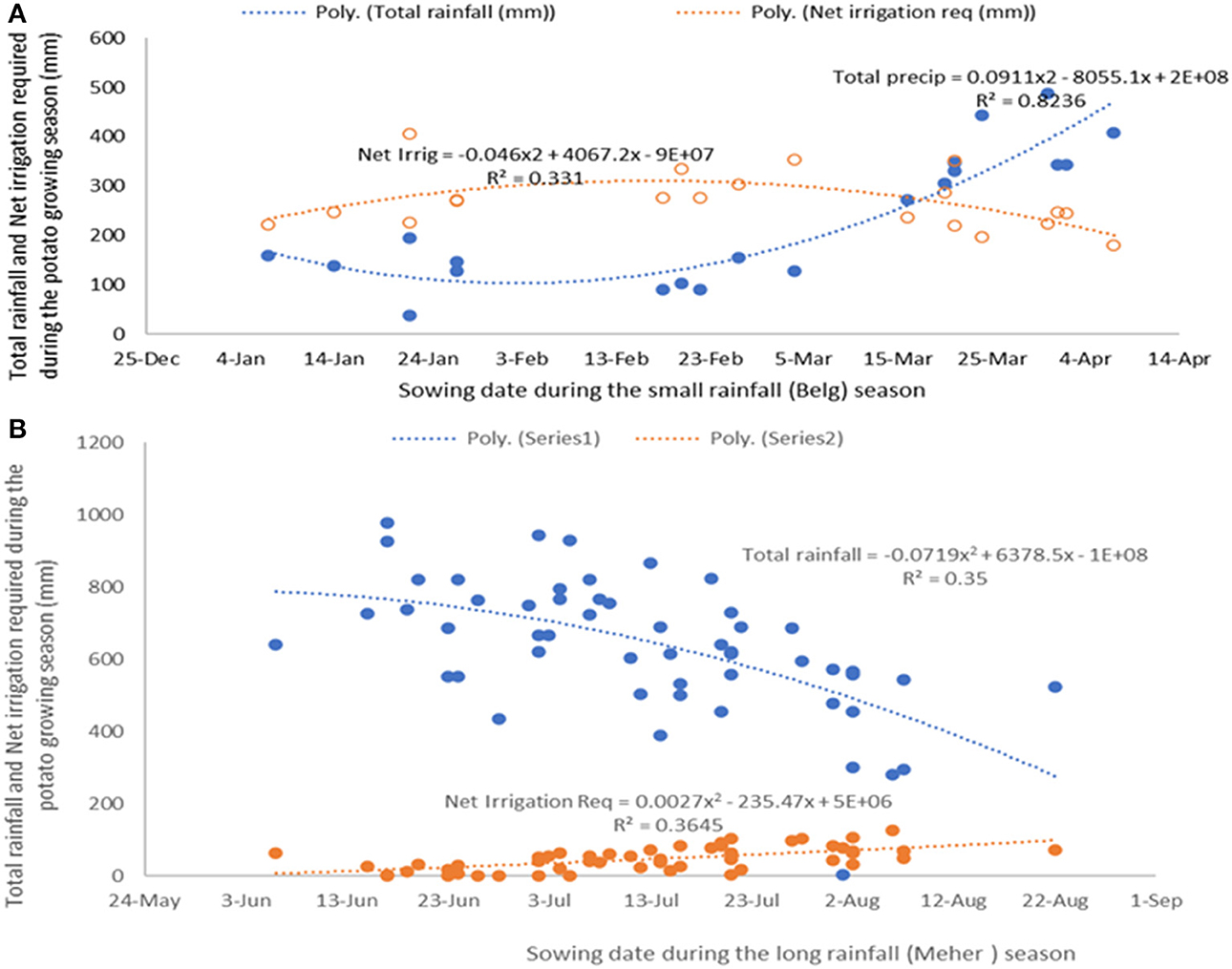
Figure 5. Total precipitation during the potato growing period and net irrigation requirements as affected by time of sowing (onset) for both the Belg (A) and Meher (B) seasons at Barneb 2 irrigation scheme in Northeastern Ethiopia. Simulations were made based on daily climate data of 20 years (2001–2020).
Potato Yield and Water Use Efficiency in Response to Long-Term Rainfall and Supplementary Irrigation During the Small and Main Rainfall Seasons
Table 5 presented simulated potato tuber yields, water use efficiency and irrigation water supply efficiency for the Belg and Meher seasons. The long-term mean simulated tuber yield of supplementarily irrigated potato was 57% higher than that from the rainfed potato. Although the yield from 100% ETc irrigation was 7% higher than that from the deficit irrigation (75% ETc), the water supply efficiency of the latter was slightly greater than the former implying that deficit irrigation could be recommended during severe competition for irrigation water in the study area. This implies that there is the need for further studies having intermediate irrigation levels to get the optimum level that compromises between yield reductions and higher water supply efficiency. A previous study in Iran revealed that the highest water use efficiency in the field conditions occurred under 75% of water requirement although the highest tuber yield was obtained from the 100% ETC (Afshar et al., 2014). A previous study in the Ethiopian highlands also confirmed that applying 75% of the potato crop water requirement has better water use efficiency than optimal or “no stress” irrigation thus implying that deficit irrigation can be a good water management technique to save irrigation water without reducing the yield of potato (Kifle and Gebretsadikan, 2016). The simulated yield of potato was more strongly correlated to the number of rainfall days than the total rainfall amount during the growing season implying the need for proper scheduling (Figure 6). Potato being moderately sensitive to water stress (Bowen, 2003; FAO, 2009a), long dry spells during the growing season can severely affect the yield, tuber size and quality despite the high amount of total rainfall during the rainfall season. Water stress at the middle growth stage when there is high tuberization rate could significantly affect yield of potato (Kifle and Gebretsadikan, 2016).
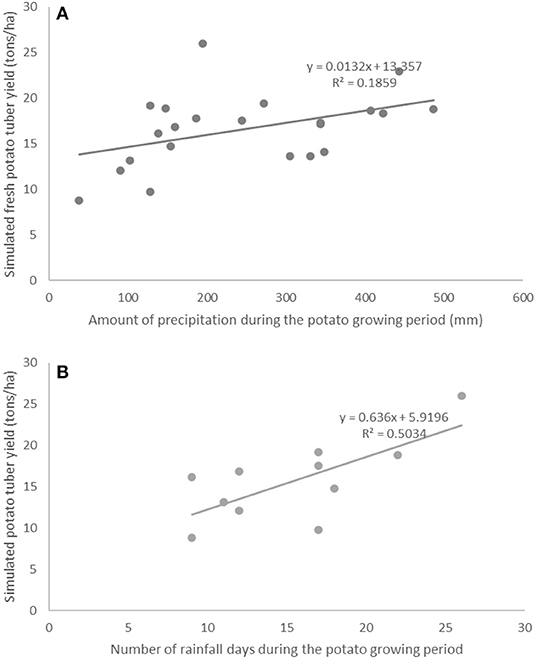
Figure 6. Simulated potato tuber yield in response to total rainfall (A) and number of rainfall days (B) during the Belg season at Barneb 2 irrigation scheme in Northeastern Ethiopia. Simulations were made based on daily climate data of 20 years (2001–2020).
Institutional and Market Aspects Affecting the Aqua Crop Recommendations
The smallholder irrigation users in the study area have faced a list of challenges and constraints affecting the proper implementations of improved irrigation agronomic practices, water saving deficit irrigation practices, and market gains (Table 6). About 93% of the respondents preferred to grow cereal crops such as wheat and barley to vegetables and tubers such as potato. All the respondents believed that wheat and barley withstand drought and variable climatic risks better than vegetables. According to the focus group discussants wheat and barley have less production and market risks than potato. The discussants further agreed that wheat could be produced with supplementary irrigation or under relatively lower rainfall amounts than vegetables and tubers during the small rainfall (Belg) season. Cereals would not also require new skills, experience relatively lower damages by pest/disease, and have better shelf life. Despite these advantages of growing cereals, the yield and income from irrigated vegetables and tubers such as potato was perceived to be higher than cereals. Based on a detailed analysis of the major institutional challenges for irrigation schemes in Ethiopia, Amede (2015) concluded that improving water productivity of horticultural crops and addressing the yield gaps require appropriate institutional and technical interventions. Upon proper skill-based trainings for the irrigation water users' association (IWUA) focusing on improved irrigation agronomy and field water management techniques, institutional linkages between the IWUA and the authorized seed certification agency was established. Accordingly, proper implementation of improved potato production techniques for farmer-based potato seed supply was found feasible both during the Belg season under deficit irrigation and Meher season under rainfed systems. The feedback of the IWUA to potato seed production using improved irrigation agronomy was found to be promising as the willingness to engage in potato seed production using improved irrigation agronomy and deficit irrigation systems increased by 3.5 times during the second season. Lack of access to quality seed and employing proper water management being a continuing challenge (Hirpa et al., 2010), small-scale potato seed production could be possible by employing proper implementations of irrigation agronomy.
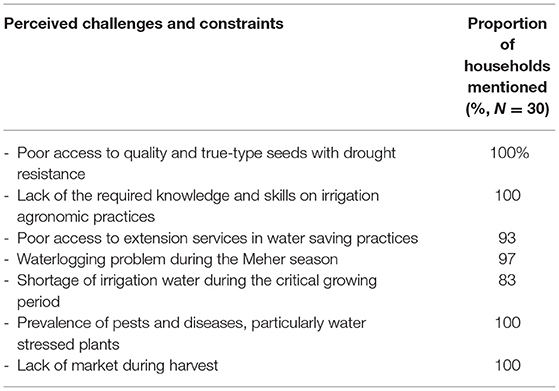
Table 6. Perceived challenges and constraints for adopting deficit irrigation by smallholder irrigation user farmers at Barneb 2 small-scale irrigation scheme in Northeastern Ethiopia.
Conclusion
Upon proper calibration and validations, the FAO's AquaCrop model could aptly simulate the relative water satisfaction, net irrigation requirements and yield of potato in response to different times of sowing, number of rainfall days and total rainfall during the potato growing periods. The long-term agro-meteorological analyses revealed that the precipitation following planting of potato at the onset dates during Belg (January and February planting) could supply an average of 44% of the potato water requirement implying the high probability of need for irrigation. During 53% of the years, the smallholder farmers should apply irrigation at the time of sowing during January/February. The long-term mean net irrigation requirements were 249 mm during Belg season while the probability of applying supplementary irrigation during June-September was <10% when the effective precipitation was greater than the crop water requirement (ETc) in more than 75% of the years. Despite the slightly higher yields of potato from 100% ETc than the 75% ETc, the latter had higher water supply efficiency than the former. Simulated yield was more strongly and positively correlated to the number of rainfall days than the total amount of rainfall. This implied that the adverse effect of long dry spells during the potato growing season on yield and yield components should be addressed through supplementary irrigation. Hence, we concluded that 50% ETc and 75% ETc irrigation levels can still be applicable with minor yield declines, but with AquaCrop recommended scheduling to address long dry spells, especially during the middle stages of potato growth, accompanied by improved water management interventions. It is also important to identify incentive mechanisms for farmers to adopt improved water management intervention to minimize risks of deficit irrigation. The capacity development and institutional linkages to initiate the IWUA for farmer-based potato seed production encouraged more farmers due to market incentives. We conclude that AquaCrop model can be used as a decision support tool, but appropriate participation of the irrigation water users' association and considerations of institutional factors such as improving access to extension and market incentives were crucial in decision making. The outcome of the long-term simulation can be used to undertake forecasted yields in response to different time of planting and irrigation levels as part of the market-oriented production system.
Data Availability Statement
The raw data supporting the conclusions of this article will be made available by the authors, without undue reservation.
Author Contributions
BB has been the principal investigator of this research thus leading the research design, setting up the experiment and leading the data collection and analyses. TA has been providing high level technical support for the proper establishment of the experiments, data analyses and manuscript preparations. SW, GT, and NA have made very good contributions in the data collection, analyses and manuscript preparations. All authors contributed to the article and approved the submitted version.
Funding
This study was financially supported by the Participatory Small scale Irrigation Development Program (PASIDP) of the Ministry of Agriculture which has been initiated through the financial support of the International Fund for Agricultural Development (IFAD).
Conflict of Interest
The authors declare that the research was conducted in the absence of any commercial or financial relationships that could be construed as a potential conflict of interest.
Publisher's Note
All claims expressed in this article are solely those of the authors and do not necessarily represent those of their affiliated organizations, or those of the publisher, the editors and the reviewers. Any product that may be evaluated in this article, or claim that may be made by its manufacturer, is not guaranteed or endorsed by the publisher.
Acknowledgments
The authors are thankful to the ICRISAT-PASIDP collaboration program through the project entitled on Enhancing Efficiency of small-scale irrigation schemes in Ethiopia. The authors gratefully thank field data collectors, and the smallholder farmers who volunteered their fields for the field experimentations and calibrations of the AquaCrop model.
References
Abdurahman, A., Griffin, D., Elphinstone, J., Struik, P. C., Schulz, S., Schulte-Geldermann, E., et al. (2017). Molecular characterization of Ralstonia solanacearum strains from Ethiopia and tracing potential source of bacterial wilt disease outbreak in seed potatoes. Plant Pathol. 66, 826–834. doi: 10.1111/ppa.12661
Abebe, T., Wongchaochant, S., and Taychasinpitak, T. (2013). Evaluation of specific gravity of potato varieties in Ethiopia as a criterion for determining processing quality. Kasetsart J. (Nat. Sci.) 47, 30–41.
Afshar, A., Afsharmanesh, G. Z., Adeli, M., and Malekian, A. (2014). Assessment of AquaCrop model in the simulation of potato yield and water use efficiency under different water regimes. J. Biol. Environ. Sci. 8, 79–86. Available online at: http://hdl.handle.net/11452/17387
Allen, R .G., Pereira, L. S., Raes, D., and Smith, M. (1998). Crop Evapotranspiration: Guidelines for Computing Crop Water Requirements: Irrigation and Drainage Paper No. 56. Rome: FAO.
Amede, T. (2015). Technical and institutional attributes constraining the performance of small-scale irrigation in Ethiopia. Water Resour. Rural Dev. 6, 78–91. doi: 10.1016/j.wrr.2014.10.005
Araya, A., and Stroosnijder, L. (2011). Assessing drought risk and irrigation needs in northern Ethiopia. Agric. For. Meteorol. 151, 425–436. doi: 10.1016/j.agrformet.2010.11.014
Assefa, S., Biazin, B., Muluneh, A., Yimer, F., and Haileslassie, A. (2016). Rainwater harvesting for supplemental irrigation of onions in the southern dry lands of Ethiopia. Agric. Water Manage. 178, 325–334. doi: 10.1016/j.agwat.2016.10.012
Awulachew, S. B., and Ayana, M. (2011). Performance of irrigation: an assessment at different scales in Ethiopia. Exp. Agric. 47, 57–69. doi: 10.1017/S0014479710000955
Awulachew, S. B., Merrey, D. J., Kamara, A. B., Van Koopen, B., De, V.ries, Penning, F., and Boelle, E. (2005). Experiences and Opportunities for Promoting Small-Scale/Micro Irrigation and Rainwater Harvesting for Food Security in Ethiopia. Colombo: IWMI Working Paper. 98.
Barron, J., Rockstrom, J., Gichuki, F., and Hatibu, N. (2003). Dry-spell analysis and maize yields for two semi-arid locations in east Africa. Agric. For. Meteorol. 117, 23–37.
Berhanu, B., Seleshi, Y., and Melesse, A. M. (2014). “Surface water and groundwater resources of Ethiopia: potentials and challenges of water resources development,” in Nile River Basin: Ecohydrological Challenges, Climate Change and Hydropolitics, eds A. M. Melesse, W. Abtew, and S. G. Setegn (London: Springer), 91–118. doi: 10.1007/978-3-319-02720-3_6
Biazin, B., and Stroosnijder, L. (2012). To tie or not to tie ridges for water conservation in Rift Valley drylands of Ethiopia. Soil Tillage Res. 124, 83–94. doi: 10.1016/j.still.2012.05.006
Birch, P. R. J., Bryan, G., Fenton, B., Gilroy, E. M., Hein, I., Jones, J. T., et al. (2012). Crops that feed the world 8: potato: are the trends of increased global production sustainable? Food Secur. 4, 477–508. doi: 10.1007/s12571-012-0220-1
Bjornlund, H., van Rooyen, A., and Stirzaker, R. (2017). Profitability and productivity barriers and opportunities in small-scale irrigation schemes. Int. J. Water Resour. Dev. 33, 690–704. doi: 10.1080/07900627.2016.1263552
Bowen, W. T. (2003). “Water productivity and potato cultivation,” in Water Productivity in Agriculture: Limits and Opportunities for Improvements, eds J. W. Kijne, R. Barker and D. Molder (Colombo: IWMI), 229–238. doi: 10.1079/9780851996691.0229
Condori, B., Hijmans, R. J., Ledent, J. F., and Quiroz, R. (2014). Managing potato biodiversity to cope with frost risk in the high Andes: a modeling perspective. PLoS ONE 9:e81510. doi: 10.1371/journal.pone.0081510
CSA (Central Statistical Authority) (2016). Agricultural Sample Survey 2015/16 (2008 E.C) Volume V. Report on Area, Production, and Farm Management Practice of Belg Season Crops for Private Peasant Holdings. Statistical Bulletin, Vol. 578. Addis Ababa: CSA. 18–19.
Damtew, E., Tafesse, S., Lie, R., Mierlo, B. V., Lemaga, B., Sharma, K., et al. (2018). Diagnosis of management of bacterial wilt and late blight in potato in Ethiopia: a systems thinking perspective. Wageningen J. Life Sci. 86–87, 12–24. doi: 10.1016/j.njas.2018.03.003
Doorenbos, J., and Kassam, A. H. (1979). Yield Response to Water. Irrigation and Drainage Paper no. 33. Rome: FAO.
FAO (2009b). The ETo Calculator: Evapotranspiration From a Reference Surface, Reference Manual. Rome: FAO. 1–38.
FAO (2013). Hot Issues: Water Scarcity. Available online at: http://www.fao.org/nr/water/issues/scarcity.html (accessed December 10, 2020).
FAOSTAT (2016). Food and Agriculture Organization (FAO) of the United Nations. Retrieved from http://www.fao.org/faostat/en/#data/QC (accessed December 08, 2020).
Francois, C., Bosseno, R., Vacher, J. J., and Seguin, B. (1999). Frost risk mapping derived from satellite and surface data over Bolivian Altiplano. Agric. For. Meteorol. 95, 113–137. doi: 10.1016/S0168-1923(99)00002-7
García-Vila, M., and Fereres, E. (2012). Combining the simulation crop model AquaCrop with an economic model for the optimization of irrigation management at farm level. Eur. J. Agron. 36, 21–31. doi: 10.1016/j.eja.2011.08.003
Geerts, S., Raes, D., and Garcia, M. (2010). Using AquaCrop to derive deficit irrigation schedules. Agric. Water Manage. 98, 213–216. doi: 10.1016/j.agwat.2010.07.003
Gnacadja, L. (2013). Land Degradation: The Hidden Face of Water Scarcity Harvard International Review. Cambridge, MA. 35, 50–54.
Gummadi, S., Rao, K. P. C., Seid, J., Legesse, G., Kadiyala, M. D. K., Takele, R., et al. (2018). Spatio-temporal variability and trends of precipitation and extreme rainfall events in Ethiopia in 1980–2010. Theor. Appl. Climatol. 134, 1315–1328. doi: 10.1007/s00704-017-2340-1
Haverkoert, A., Koesveld, F. V., Scheppers, H., Wijnands, J., Wustman, R., and Zhang, X. (2012). Potato Prospects for Ethiopia: On the Road to Value Addition. Wageningen: Wageningen University and Research Center, PPO Publication no. 528. 1–66.
Hirpa, A., Miranda, P. M., Meuwissen, M. P. M., Tesfaye, A., Willemen, J. M., Lommen, W. J. M., et al. (2010). Analysis of seed potato systems in Ethiopia. Am. J. Pot. Res.87, 537–552. doi: 10.1007/s12230-010-9164-1
Hsiao, T. C., Heng, L. K., Steduto, P., Rojas-Lara, B., Raes, D., and Fereres, E. (2009). AquaCrop ? The FAO crop model to simulate yield response to water III. Parameterization and testing for maize. Agronomy J. 101, 448–459.
Kifle, M., and Gebretsadikan, T. G. (2016). Yield and water use efficiency of furrow irrigated potato underregulated deficit irrigation, Atsibi-Wemberta, North Ethiopia. Agric. Water Manage. 170, 133–139. doi: 10.1016/j.agwat.2016.01.003
Leskovar, D. I., Agehara, S., Crop CoeffiYoo, K., and Pascuala-Seva, N. (2012). Crop Coefficient-based Deficit Irrigation and Planting Density for Onion: Growth, Yield, and Bulb Quality. HortScience. 47, 31–37.
Linker, R., Ioslovich, L., Sylaios, G., Plauborg, F., and Battilani, A. (2018). Optimal model-based deficit irrigation scheduling using AquaCrop:A simulation study with cotton, potato and tomato. Agric. Water Manage. 163, 236–243. doi: 10.1016/j.agwat.2015.09.011
Mhizha, T. (2010). Increase of Yield Stability by Staggering the Sowing Dates of Different Varieties of Rainfed Maize in Zimbabwe. PhD thesis, Belgium: Katholieke Universiteit Leuven.
Montoya, F., Camargo, D., Ortega, J. F., Córcoles, J. I., and Domínguez, A. (2016). Evaluation of Aquacrop model for a potato crop under different irrigation conditions. Agric. Water Manage. 164, 267–280. doi: 10.1016/j.agwat.2015.10.019
Raes, D., Sithole, A., Makarau, A., and Milford, J. (2004). Evaluation of first sowing dates recommended by criteria currently used in Zimbabwe. Agric. Water Manage. 125, 177–185. doi: 10.1016/j.agrformet.2004.05.001
Raes, D., Steduto, P., Hsiao, T. C., and Fereres, E. (2009). AquaCrop–the FAO crop model to simulate yield response to water II. Main algorithms and soft ware description. Agron. J. 101, 438–447. doi: 10.2134/agronj2008.0140s
Razzaghi, F., Zhou, Z., Andersen, M. N., and Plauborg, F. (2017). Simulation of potato yield in temperate condition by the AquaCrop model. Agric. Water Manage. 191, 113–123. doi: 10.1016/j.agwat.2017.06.008
SCS (1993). Irrigation water requirements. National Engineering Handbook, Part 623. Washington, DC: Soil Conservation Service, US Dept. Of Agriculture. 284.
Sivakumar, M. V. K. (1988). Predicting rainy season potential from the onset of rains in Southern Sahelian and Sudanian climatic zones of West Africa. Agric. For. Meteorol. 42, 295–305. doi: 10.1016/0168-1923(88)90039-1
Steduto, P., Hsiao, T. C., and Fereres, E. (2007). On the conservative behaviour of biomass water productivity. Irrigation Sci. 25, 189–207. doi: 10.1007/s00271-007-0064-1
Steduto, P., Hsiao, T. C., Fereres, E., and Raes, D. (2012). Crop Yield Response to Water. FAO Irrigation and Drainage Paper 66. Rome: FAO. 1–233.
Steduto, P., Hsiao, T. C., Raes, D., and Fereres, E. (2009). AquaCrop – the FAO crop model to simulate yield response to water I. Concepts and underlying principles. Agron. J. 101, 426–437. doi: 10.2134/agronj2008.0139s
Tesfaye, K., and Walker, S. (2004). Matching of crop and environment for optimal water use: the case of Ethiopia. Phys. Chem. Earth 29, 1061–1067. doi: 10.1016/j.pce.2004.09.024
Tilman, D., Balzer, C., Hill, J., and Befort, B. L. (2011). Global food demand and the sustainable intensification of agriculture. Proc. Natl. Acad. Sci. U. S. A. 108, 20260–20264.
USDA (United States Department of Agriculture). (1964). “Estimation of direct runoff from storm rainfall,” in National Engineering Handbook (Washington, DC: Soil Conservation Service, USDA). p. 1–24.
USDA. (1970). Irrigation Water Requirements. Technical Release no. 21. USDA. Washington, DC: Soil Conservation Service.
Wale, A., Collick, A. S., Rossiter, D. G., Langan, S., and Steenhuis, T. S. (2013). “Realistic assessment of irrigation potential in the Lake Tana basin, Ethiopia,” in Rainwater management for resilient livelihoods in Ethiopia: Proceedings of the Nile Basin Development Challenge Science Meeting, Addis Ababa, 9–10 July 2013. NBDC Technical Report 5, ed M. Wolde. Nairobi, Kenya: ILRI.
Xie, H., Perez, N., Anderson, W., Ringler, C., and You, L. (2018). Can Sub-Saharan Africa feed itself? The role of irrigation development in the region's drylands for food security. Water Int. 43, 796–814. doi: 10.1080/02508060.2018.1516080
Keywords: potato, supplementary irrigation, small rainfall (Belg) season, water use efficeincy, irrigation water users' assoication
Citation: Biazin B, Wondatir S, Tilahun G, Asaro N and Amede T (2021) Using AquaCrop as a Decision-Support Tool for Small-Scale Irrigation Systems Was Dictated by the Institutional and Market Incentives in Ethiopia. Front. Water 3:664127. doi: 10.3389/frwa.2021.664127
Received: 04 February 2021; Accepted: 23 July 2021;
Published: 18 August 2021.
Edited by:
Silvio Gumiere, Laval University, CanadaReviewed by:
Carsten Montzka, Julich-Forschungszentrum, Helmholtz-Verband Deutscher Forschungszentren (HZ), GermanyThiago Gumiere, Laval University, Canada
Copyright © 2021 Biazin, Wondatir, Tilahun, Asaro and Amede. This is an open-access article distributed under the terms of the Creative Commons Attribution License (CC BY). The use, distribution or reproduction in other forums is permitted, provided the original author(s) and the copyright owner(s) are credited and that the original publication in this journal is cited, in accordance with accepted academic practice. No use, distribution or reproduction is permitted which does not comply with these terms.
*Correspondence: Birhanu Biazin, Qi50ZW1lc2dlbkBjZ2lhci5vcmc=