- Hydrology and Quantitative Water Management Group, Wageningen University, Wageningen, Netherlands
Land-based plastic waste, carried to the sea through rivers, is considered a main source of marine plastic pollution. However, most plastics that leak into the environment never make it into the ocean. Only a small fraction of plastics that are found in the terrestrial and aquatic compartments of river systems are emitted, and the vast majority can be retained for years, decades, and potentially centuries. In this perspective paper we introduce the concept of river systems as plastic reservoirs. Under normal conditions, hydrometeorological variables (such as wind, runoff and river discharge) mobilize, transport and deposit plastics within different river compartments (e.g., riverbanks, floodplains, lakes, estuaries). The emptying of these plastic reservoirs primarily occurs under extreme hydrological conditions (e.g., storms, floods). In this paper we specifically focus on the retention mechanisms within different river compartments, and their effect on the fate of the plastics that are accumulated on various timescales. We aim to introduce the concept of rivers as (long-term) sinks for plastic pollution, and provide suggestions for future research directions.
Introduction
In contrast to what is commonly assumed, most land-based plastic waste does not flow into the ocean. The available observations of plastic pollution within and across river catchments in fact support the hypothesis that most plastics may be retained in and around rivers (e.g., van Emmerik et al., 2019b; Tramoy et al., 2020a; Newbould et al., 2021; Ryan and Perold, 2021). In this paper we present our perspective on what happens to the plastics that do not flow into the sea, and what future work is needed to better understand and quantify the role of rivers as plastic reservoirs. Note that in this paper, we mainly focus on macroplastics (>0.5 cm), although we do include relevant science on other size ranges.
Plastic pollution is an emerging environmental risk due to its negative impact on ecosystem health and human livelihood. Macroplastics in particular can directly (lethally) injure animal and vegetation species, damage vessels and hydraulic infrastructures, and lead to increased urban flood risk due to clogging (van Emmerik and Schwarz, 2020). Plastics enter the environment through various pathways (Figure 1A), including improper disposal, direct dumping, leaking from waste infrastructure or industry, sewage discharge, hydrometeorological variables such as wind and surface runoff, and disasters including floods, storms or landslides (Lechthaler et al., 2020; van Emmerik and Schwarz, 2020). As such, river systems play a major role in transporting land-based plastic waste into the world's oceans.
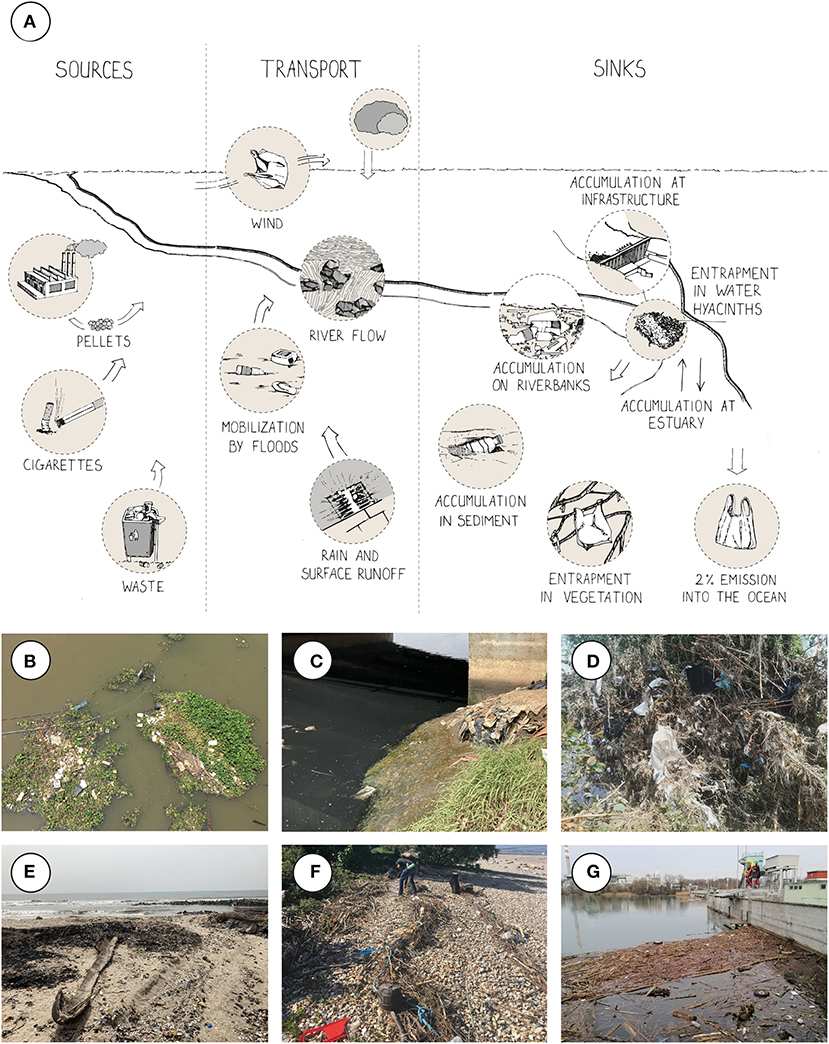
Figure 1. (A) Conceptual overview of sources, transport mechanisms and sinks (retention zones) of river plastic pollution (Graphic design by Cher van den Eng). Sources include mismanaged plastic waste, littering from individuals (e.g., cigarette butts), and leakage from industry (e.g., pellets). Transport processes include wind, surface runoff, river flow dynamics, and floods. Retention zones include infrastructure, stationary and mobile vegetation (the latter illustrated by the water hyacinth), riverbanks, and the estuary. Finally, only <2% is estimated to be emitted into the ocean globally. The photos show examples of plastic retention (B) in water hyacinth (Saigon river, Vietnam), (C) within riverbank sediments, (Odaw river, Ghana), (D) in riparian vegetation (Meuse river, the Netherlands), (E) in the estuary mouth (Odaw river, Ghana), (F) on riverbanks (Seine river, France), and (G) at infrastructure (Rhône river, Switzerland). All photos are taken by the authors.
Plastic transport processes in river systems are complex. Various hydrometeorological variables have been correlated to the mobilization, transport, dispersion, and accumulation of plastics in rivers. Examples include wind (Bruge et al., 2018; Roebroek et al., 2021a), river flow velocity and discharge (Haberstroh et al., 2021), and precipitation-induced runoff (Treilles et al., 2021). Hydrology is not only important for mobilization and transport processes, but also determines when and where plastics accumulate in river systems (Liro et al., 2020). The combination of river dynamics and characteristics are key to whether plastics are deposited on banks and floodplains, get entrapped in riparian vegetation, settle into the sediment, or get transported to downstream areas (van Emmerik and Schwarz, 2020). Once plastics enter the estuary, the combination of riverine and tidal dynamics determine the fate of (specific) plastics (Figure 1A). A growing amount of evidence suggests that most plastics that enter the environment are never exported into the ocean (van Emmerik et al., 2019b, 2020a; Tramoy et al., 2020a,b; Ryan and Perold, 2021). Globally, only a very small fraction of the mismanaged plastic waste is emitted into the sea (Meijer et al., 2021). The most important science and policy questions therefore remain where the plastic accumulates within river systems, and at what spatial and temporal scales they are retained.
In this perspective paper we elaborate on the hypothesis that rivers act as reservoirs for plastic pollution. We present an overview of the various river compartments that act as accumulation zones for plastic pollution, as well as the retention and (re)mobilization mechanisms. We also elaborate on the fate of plastics retained in these zones, including long-term storage, degradation and remobilization processes. Finally, we present a perspective for future research directions, including specific scientific questions that need to be addressed to effectively prevent, mitigate and reduce plastic pollution in the environment.
Most River Plastics Do Not Flow into the Ocean
On global (Jambeck et al., 2015; Borrelle et al., 2020; Meijer et al., 2021), regional (Bai et al., 2018; van Calcar and van Emmerik, 2019; González-Fernández et al., 2021), and local levels (van Emmerik et al., 2019a; Schöneich-Argent et al., 2020; Vriend et al., 2020), several assessment strategies have been developed to estimate the plastic mass flows from land into rivers, and finally into the ocean. In recent years, the focus on estimating plastic emissions has shifted more toward understanding what happens with plastic within river systems before it makes it into the ocean. Meijer et al. (2021) quantified that globally, less than 2% of the total plastic waste leaked into the environment ends up in the marine environment. Depending on basin characteristics and the locations of plastic leakage, this ratio varies considerably between rivers. For relatively short rivers with high population densities close to the river and the coast, such as found in the Philippines and Indonesia, plastics are more likely to be emitted into the ocean. For long rivers with larger upstream population, infrastructure such as dams, and larger floodplains (for example the Rhône or the Rhine) the likelihood of emission is even less. On a local level, annual emissions of rivers in Jakarta, Indonesia, were compared to the total amount of plastic leaked into the environment, showing that only 3% of the mismanaged plastic waste is transported into the ocean (van Emmerik et al., 2019b). Substantial work on the Seine, France, demonstrated that plastics can have long retention times, already surpassing decades (Tramoy et al., 2020a). Other studies demonstrated the short travel distances of plastic waste once they enter a river system (Weideman et al., 2020; Newbould et al., 2021), suggesting they accumulate somewhere along the river. All available evidence considered, river systems can be a major plastic reservoir, rather than only a source of marine plastic pollution.
Where Are River Plastics Retained?
When rivers do function as reservoirs for plastic pollution, it is key to better understand and quantify where plastics are retained, what factors determine retention, and for how long they are retained. In this section, we provide examples from observational studies that found plastics entrapped in various compartments of river systems, which we categorized into six main retention mechanisms (Figures 1B–G).
Retained on Riverbanks and Floodplains
Plastic pollution found on riverbanks and floodplains originates either from terrestrial pathways (direct littering or dumping) or from riverine transport. Similar to some sediments, macroplastics are more easily deposited on the riverbanks along areas with stagnant water, low flow velocities, low channel slopes and high riparian vegetation densities (Bruge et al., 2018). During floods, riverbank plastic can be transported and deposited in floodplains as well, where it will most likely be buried by natural sediments (Lechthaler et al., 2020; Roebroek et al., 2021b). However, the retention mechanisms and time scales of plastic on riverbanks and in floodplains remain largely unresolved, especially as sedimentation rates and river characteristics play an important, yet unknown role (Lechthaler et al., 2021). Roebroek et al. (2021a) found retention times of shorter than six months, whereas Tramoy et al. (2020b), who investigated the use-by dates on medical packaging, showed that 37% of the litter was discarded before 1984 (Tramoy et al., 2020b). Both the spatiotemporal distribution and the retention times of macroplastics on riverbanks and in floodplains must be further explored in the future, for which we recommend upscaling and expanding methods. The latter may include using expiration dates or the language on packaging, sampling plastics at deeper layers in the riparian zone, and quantifying retention within specific features such as vegetation.
Retained in Plants
Vegetation along the riverbanks, in estuaries and floating at the river surface can function as both a carrier and a reservoir of macroplastics. Variations in river water level can result in the deposition of macroplastics in grass, bushes and trees (Williams and Simmons, 1996). The retention time of plastics trapped in riparian vegetation greatly depends on (seasonally varying) flow dynamics, and the characteristics of the vegetation, such as the height, plant density and spatial configuration, architecture, and geometry. These factors determine to what extent the water interacts with the vegetation, and in turn influences the likelihood of entrapment. A recent study observed that arboreal and shrubby vegetation entrap more macroplastic compared to herbaceous, reed and bush types (Cesarini and Scalici, 2022). The presence of branches, thicker foliage, as well as higher height and density compared to the other vegetation types might play an important role in macroplastic trapping and retention. Moreover, floating aquatic vegetation has been found to trap and carry considerable amounts of macroplastics downstream. In the Saigon river, drifting patches of water hyacinths were found to carry as much as 78% of the floating plastic (Schreyers et al., 2021). However, the effects of bidirectional flows due to the influence of tidal regimes, and the stranding of large hyacinth patches on the riverbanks further complicates this conception of floating vegetation as a transport carrier of plastic into the sea.
Retained in Riverbed Sediments
Dense macroplastic items (>1,000 kg/m3) can sink to the river bed in the absence of strong turbulence or currents. These negatively buoyant plastic types include items made of polyvinyl chloride, polyethylene terephthalate, polypropylene and polystyrene. During the transport process, initially buoyant macroplastic items, such as bottles, can fill with water or have biofilm grown on their surface, and thus lose their buoyancy and sink (Gabbott et al., 2020; Lechthaler et al., 2020; Al-Zawaidah et al., 2021). These items can be deposited in areas of the river with low flow velocities (Liro et al., 2020) and high natural sedimentation rates. The macroplastics can either be buried by natural sediments, which increases their remobilization threshold, be transported as bed-load transport, or get resuspended into the water column due to stronger flow velocities or turbulence (van Emmerik and Schwarz, 2020). Possible methods to measure deposited macroplastics include the analysis of dredged sediment (Constant et al., 2021), or analyzing samples from sediment traps (Enders et al., 2019; Saarni et al., 2021). There are only a few measurement methods to quantify macroplastics in riverbed sediments or as bed-load, yet, those are essential to identify macroplastic retention zones and understand their spatiotemporal distribution.
Retained at Infrastructure
Only a small fraction of the world's rivers remain free flowing (Grill et al., 2019). Many river systems have been anthropogenically altered to guarantee shipping, water supply for consumption and irrigation, or generate power. Examples include dams, water inlets, groins, ports, sluices and canals. This type of infrastructure can act as accumulation zones for macroplastics. Larger plastic concentrations have been found upstream of dams, both in surface waters and sediment (Zhang et al., 2015; Castro-Jiménez et al., 2019; Watkins et al., 2019). Macroplastics become trapped around hydroelectric power plants, and are deposited on riverbanks and in sediments around groins (Skalska et al., 2020; Tramoy et al., 2020a). In urban water systems, plastics are trapped by rack structures, pumping stations or around bridges (Tasseron et al., 2020). Consequently, the water system becomes (partially) obstructed, which leads to higher upstream water levels (Honingh et al., 2020). Targeted infrastructure, such as floating litter booms or litter traps, is placed to intentionally accumulate plastics. Besides capturing plastic for removal, these traps also provide a rich source of data. Several assessments to estimate plastic mass transport, identify plastic mass flows, or characterize plastic waste, analyzed waste captured at such infrastructure in the Netherlands (Vriend et al., 2020), France (Gasperi et al., 2014) and Malaysia (Malik et al., 2020). Further work at the river system scale is needed to shed additional light on the role of infrastructure on plastic accumulation, and the plastic mass balance on catchment level.
Retained in Lakes
Exorheic lakes, i.e., lakes that ultimately drain into the ocean, are found in river systems all over the world. Macroplastics have been found afloat in lake surface waters (Faure et al., 2015), buried in bottom lake sediments (Egessa et al., 2020), and deposited on lake shorelines/beaches (Faure et al., 2015; Egessa et al., 2020). These plastics either come from local activities (e.g., littering, fishing gear, direct wastewater drainage from a nearby urban area or direct surface runoff) or have been conveyed by rivers that discharge into the lake. Once a river flows into a lake, the plastics are carried by the lake surface currents and can concentrate in small temporary gyres (Faure et al., 2015). Wind-induced surface currents, especially during storms, also transport and deposit substantial amounts of plastic on the shorelines of lakes (Zbyszewski et al., 2014). The full retention capacity of lakes remains unresolved, and as many river systems worldwide feature lakes, quantifying how much upstream riverine plastics make it through a lake to the downstream river, is crucial for accurate estimates on riverine plastic transport and emissions.
Retained in Estuaries
Estuaries are influenced by tidal dynamics and freshwater discharge, leading to diurnally changing water level, salt concentrations, flow velocity, and flow direction (Savenije, 2012). The scarce observational evidence available suggests that plastic transport and accumulation is impacted by these dynamics at different timescales. Within tidal cycles, plastic flux close to the river mouth has been found to be about the same during both ebb and flood tide, suggesting that the actual net transport from rivers into the sea is very limited (van Emmerik et al., 2020c). As long as the net discharge is low, the plastics accumulating in the estuary have a growing likelihood of beaching on the riverbanks (van Emmerik et al., 2020a), getting entrapped in riparian vegetation (Martin et al., 2020), deposited in the sediment (Acha et al., 2003), or to degrade and fragment into smaller particles (Lebreton et al., 2019). Increased freshwater discharge, especially during flood conditions, may cause a peak of plastic export into the near coastal zone. Other work has suggested that plastics may be retained on estuary shores for years or even longer (Tramoy et al., 2020b). The role of estuaries on plastic transfer between rivers and the ocean, and the time scales of retention, remain largely unresolved. Future work should therefore explore the factors that drive plastic accumulation, (re)mobilization, and the time scales of retention in estuaries.
What Happens to Accumulated Plastics?
In the previous sections we discussed the various plastic transport mechanisms, followed by examples of where and why plastics are entrapped, demonstrating the function of rivers as plastic reservoirs. In this section we further discuss what happens to the retained plastics, and how the reservoirs may be emptied.
Breaking Down Into Smaller Particles
Plastics in river systems are commonly fragments of soft and hard plastics or foam (Castro-Jiménez et al., 2019; Tramoy et al., 2019; van Emmerik et al., 2020a). While some plastic items remain intact in the environment for decades (Tramoy et al., 2020b), others break down into smaller and smaller particles, due to physical fragmentation or chemical degradation (Delorme et al., 2021). Physical fragmentation is caused by mechanical influences such as waves, and abrasion through sediment (Barnes et al., 2009) but also chewing and digestive fragmentation through organisms (Dawson et al., 2018; Mateos-Cárdenas et al., 2020). Chemical degradation processes include biodegradation, photodegradation, thermo-oxidative degradation, thermal degradation, and hydrolysis. Exposure to UV-light and air accelerate the degradation process (Andrady, 2011; Waldschläger et al., 2020). During fragmentation and degradation different processes interact, depending on environmental conditions and properties of the plastic items (Andrady, 2011; Song et al., 2017).
Once broken down into microplastic particles, they could either be deposited in the sediment, be transported in the water column, or ingested by biota (Leslie et al., 2017). The interaction of fragmentation and degradation, and transport of plastics through different river compartments remains largely unknown, and a quantification method for degradation rates has not been found yet (Ford et al., 2022). We do expect smaller particles to be remobilized more easily, travel further downstream, and eventually reach the ocean. Microplastics have been found to be flushed away after floods or storm events (Hurley et al., 2018; Treilles et al., 2022), and do not experience the same interaction with many of the entrapment factors as mentioned in section Where are River Plastics Retained? The plastic reservoir within rivers may therefore be emptied on the form of fragmented secondary microplastic particles.
Retention Leads to Longer Exposure Times
We have seen that plastics accumulate within various compartments in river systems, leading to the exposure of biota to plastics, with all its potential detrimental consequences. The longer plastic accumulates in natural ecosystems, the higher the number of species that will encounter plastics and therefore the higher the chance it leaves a negative impact. Recent evidence shows that macroplastics can be retained in rivers for several decades (Tramoy et al., 2020a), increasing the likelihood of ingestion by biota. In the Amazon, as much as 80% of the fish species had ingested plastic particles (Andrade et al., 2019), which can be life-threatening, especially when (toxic) waterborne contaminants are adsorbed to the plastics (Bellasi et al., 2020). Given the pivotal role of freshwater biota in the food chain, future research must continue to explore the degree and duration of the exposure of biota to plastics in order to clarify the threats they face in different river compartments.
Emptying of the Plastic Reservoir by Extreme Events
During high wind speeds, high rainfall intensities, or high river discharges, accumulated plastics are likely to be remobilized and removed from retention zones. Ample studies have demonstrated an increased riverine plastic transport during flood events (Moore et al., 2011; Jang et al., 2014; Mihai, 2018; van Emmerik et al., 2019c; Roebroek et al., 2021b). Flood events inundate the riverbanks, floodplains and tidal flats and thereby (re)mobilize the plastics that have been accumulating in these reservoirs during normal conditions. Additional plastics also enter the river system during such events. Estuaries that during normal conditions tend to have a zero net transport of plastics into the oceans, export plastics during and just after a flood event (Liro et al., 2020). Even plastics buried within riverbed sediments can get removed from this reservoir when the active sediment layer of the riverbed is destabilized by extremely high water flow velocities (Ockelford et al., 2020). However, extreme events do not solely (re)mobilize plastics, they can lead to plastic deposition as well. When the water flow velocities in the river channel are much higher than above the submerged floodplains, plastics can become transferred from the faster flow (channel) to the slower flow velocity area (floodplain) and settle there (Ciszewski and Grygar, 2016). Another phenomenon, often referred to as the “Christmas tree effect,” whereby plastic debris carried by the flood becomes entrapped in riparian trees and is left behind when the water level drops again, demonstrates the reservoir filling capacity of a flood event (Williams and Simmons, 1996). Future research must explore the relative importance between (re)mobilization and (re)deposition mechanisms during extreme events.
Discussion and Outlook
In this paper we demonstrate the function of rivers as plastic reservoirs, through the retention and release mechanisms in various river compartments (Table 1). One of the crucial open questions that remains are the spatial and temporal scales of the accumulation and retention mechanisms across different river compartments. In estuaries, for example, the retention of floating plastics may be in the order of days (due to the tidal cycle), whereas riverbanks are shown to store plastics for several decades already. These findings suggest that even if no more plastics would leak into the environment as of today, the plastics retained within river systems will continue to have negative impacts, break down in smaller particles, and function as a potential source of marine plastic pollution for the decades and centuries to come. We therefore call on more research on investigating the factors that determine the processes, spatial extent and time scales of retention and release mechanisms of plastics on riverbanks, lakes, estuaries, vegetation, sediment, and infrastructure.
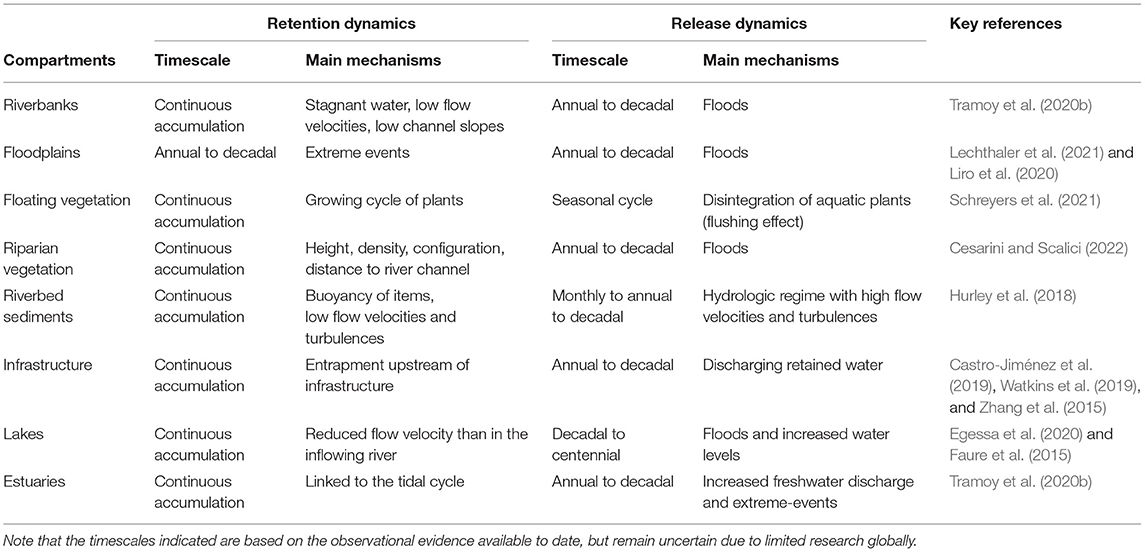
Table 1. Overview of the retention and release dynamics, and their timescales, discussed in this paper.
Detailed investigations of fundamental retention and release mechanisms are complicated due to a lack of observations across all river compartments, and adequate monitoring methods (Broere et al., 2021; van Emmerik et al., 2022). On top of that, most work focuses on tracking specific items (e.g., bottles) through both modeling and observations (Duncan et al., 2020; Newbould et al., 2021), or quantifying plastics in single river cross-sections. Even though such targeted and local studies provide useful snapshots, we advocate for large-scale and holistic studies in which the transport and retention in all river compartments is examined. Furthermore, we reiterate the need for conceptual and observation based approaches to accurately quantify the amount and characteristics of plastics within each compartment, and the mass flow between them (van Emmerik et al., 2022). Assessments that focus on the entire river system can be facilitated through for example large-scale tracker experiments, exploring the use of remote sensing, and promotion of citizen science apps.
Tracker experiments, both using GPS trackers and marked items, have yielded new insights in the transport and accumulation processes, demonstrating that the travel distance is limited in many cases. River focused experiments have used relatively low number of trackers (less than a hundred), and large-scale experiments using thousands of trackers have to date only been used at sea (e.g., Duncan et al., 2020; Schöneich-Argent and Freund, 2020; Tramoy et al., 2020b; Newbould et al., 2021; Ryan and Perold, 2021). Close-range and satellite remote sensing also offer new avenues for large-scale data collection. Marine plastic debris can already be observed from space using Sentinel-2 imagery (Biermann et al., 2020). Rivers are generally smaller and more dynamic systems, requiring more research to fully explore the potential for river plastic monitoring from space. Lab experiments to establish hyperspectral reflectance databases will accelerate the development of new sensors, and disentangle and downscale the aggregated signals observed from space (Tasseron et al., 2021). Finally, several citizen science mobile applications facilitate rapid upscaling of data collection of plastic pollution on land (Litterati; Ballatore et al., 2021) and in river systems (CrowdWater; van Emmerik et al., 2020b). Projects that target specific locations have been shown to stimulate the use of such apps and rapidly increase observations done by citizen scientists (Tasseron et al., 2020).
With this paper we aim to demonstrate the current state of the science on river plastic pollution, and elaborate on the hypothesis that rivers function as plastic reservoirs. River plastic pollution and hydrology are unequivocally connected, emphasizing the need for more transdisciplinary studies to improve the understanding of this emergent pollutant.
Author Contributions
TvE, YM, RH, KW, and LS: conceptualization, formal analysis, investigation, writing—original draft, and writing—review and editing. All authors contributed to the article and approved the submitted version.
Funding
The work of TvE was supported by the Veni Research Program, the River Plastic Monitoring Project with project number 18211, which was (partly) financed by the Dutch Research Council (NWO). The work of LS was supported by NWO Open Mind grant 18127. The work of KW was supported by Investment Plan for strengthening the Technical Sciences at Wageningen University.
Conflict of Interest
The authors declare that the research was conducted in the absence of any commercial or financial relationships that could be construed as a potential conflict of interest.
Publisher's Note
All claims expressed in this article are solely those of the authors and do not necessarily represent those of their affiliated organizations, or those of the publisher, the editors and the reviewers. Any product that may be evaluated in this article, or claim that may be made by its manufacturer, is not guaranteed or endorsed by the publisher.
Acknowledgments
We thank Cher van den Eng for providing the artwork for Figure 1. We thank Paul Vriend and Lourens Meijer for providing valuable input on an earlier version of this manuscript. We are grateful to the three reviewers and the editor whose suggestions helped improving the paper.
References
Acha, E. M., Mianzan, H. W., Iribarne, O., Gagliardini, D. A., Lasta, C., and Daleo, P. (2003). The role of the Rio de la Plata bottom salinity front in accumulating debris. Mar. Pollut. Bull. 46, 197–202. doi: 10.1016/S0025-326X(02)00356-9
Al-Zawaidah, H., Ravazzolo, D., and Friedrich, H. (2021). Macroplastics in rivers: present knowledge, issues and challenges. Environ. Sci. 23:535, doi: 10.1039/D0EM00517G
Andrade, M. C., Winemiller, K. O., Barbosa, P. S., Fortunati, A., Chelazzi, D., Cincinelli, A., et al. (2019). First account of plastic pollution impacting freshwater fishes in the Amazon: ingestion of plastic debris by piranhas and other serrasalmids with diverse feeding habits. Environ. Pollut. 244, 766–773. doi: 10.1016/j.envpol.2018.10.088
Andrady, A. L. (2011). Microplastics in the marine environment. Mar. Pollut. Bull. 62, 1596–1605. doi: 10.1016/j.marpolbul.2011.05.030
Bai, M., Zhu, L., An, L., Peng, G., and Li, D. (2018). Estimation and prediction of plastic waste annual input into the sea from China. Acta Oceanologica Sinica 37, 26–39. doi: 10.1007/s13131-018-1279-0
Ballatore, A., Verhagen, T. J., Li, Z., and Cucurachi, S. (2021). This city is not a bin: crowdmapping the distribution of urban litter. J. Ind. Ecol. 1–16. Available online at: https://doi.org/10.1111/jiec.13164
Barnes, D. K., Galgani, F., Thompson, R. C., and Barlaz, M. (2009). Accumulation and fragmentation of plastic debris in global environments. Philos. Trans. R. Soc. Lond., B, Biol. Sci. 364, 1985–1998. doi: 10.1098/rstb.2008.0205
Bellasi, A., Binda, G., Pozzi, A., Galafassi, S., Volta, P., and Bettinetti, R. (2020). Microplastic contamination in freshwater environments: a review, focusing on interactions with sediments and benthic organisms. Environments 7:30. doi: 10.3390/environments7040030
Biermann, L., Clewley, D., Martinez-Vicente, V., and Topouzelis, K. (2020). Finding plastic patches in coastal waters using optical satellite data. Sci. Rep. 10, 1–10. doi: 10.1038/s41598-020-62298-z
Borrelle, S. B., Ringma, J., Law, K. L., Monnahan, C. C., Lebreton, L., McGivern, A., et al. (2020). Predicted growth in plastic waste exceeds efforts to mitigate plastic pollution. Science 369, 1515–1518. doi: 10.1126/science.aba3656
Broere, S., van Emmerik, T., González-Fernández, D., Luxemburg, W., de Schipper, M., Cózar, A., et al. (2021). Towards underwater macroplastic monitoring using echo sounding. Front. Earth Sci. 9:628704. doi: 10.3389/feart.2021.628704
Bruge, A., Barreau, C., Carlot, J., Collin, H., Moreno, C., and Maison, P. (2018). Monitoring litter inputs from the Adour River (Southwest France) to the marine environment. J. Mar. Sci. Eng. 6:24. doi: 10.3390/jmse6010024
Castro-Jiménez, J., González-Fernández, D., Fornier, M., Schmidt, N., and Sempéré, R. (2019). Macro-litter in surface waters from the Rhone River: plastic pollution and loading to the NW Mediterranean Sea. Mar. Pollut. Bull. 146, 60–66. doi: 10.1016/j.marpolbul.2019.05.067
Cesarini, G., and Scalici, M. (2022). Riparian vegetation as a trap for plastic litter. Environ. Pollut. 292:118410.
Ciszewski, D., and Grygar, T. M. (2016). A review of flood-related storage and remobilization of heavy metal pollutants in River Systems. Water Air Soil Pollut. 227:239doi: 10.1007/s11270-016-2934-8
Constant, M., Alary, C.l., De Waele, I., Dumoulin, D., Breton, N., and Billon, G. (2021). To what extent can micro- and macroplastics be trapped in sedimentary particles? A case study investigating dredged sediments. Environ. Sci. Technol. 55, 5898–5905 doi: 10.1021/acs.est.0c08386
Dawson, A. L., Kawaguchi, S., King, C. K., Townsend, K. A., King, R., Huston, W. M., et al. (2018). Turning microplastics into nanoplastics through digestive fragmentation by Antarctic krill. Nat. Commun. 9, 1–8. doi: 10.1038/s41467-018-03465-9
Delorme, A. E., Koumba, G. B., Roussel, E., Delor-Jestin, F., Peiry, J. L., Voldoire, O., et al. (2021). The life of a plastic butter tub in riverine environments. Environ. Pollut. 287:117656. doi: 10.1016/j.envpol.2021.117656
Duncan, E. M., Davies, A., Brooks, A., Chowdhury, G. W., Godley, B. J., Jambeck, J., et al. (2020). Message in a bottle: open source technology to track the movement of plastic pollution. PLoS ONE 15:e0242459. doi: 10.1371/journal.pone.0242459
Egessa, R., Nankabirwa, A., Basooma, R., and Nabwire, R. (2020). Occurrence, distribution and size relationships of plastic debris along shores and sediment of northern Lake Victoria. Environ. Pollut. 257:113442. doi: 10.1016/j.envpol.2019.113442
Enders, K., Käppler, A., Biniasch, O., Feldens, P., Stollberg, N., Lange, X., et al. (2019). Tracing microplastics in aquatic environments based on sediment analogies. Sci. Rep. 9:15207doi: 10.1038/s41598-019-50508-2
Faure, F., Demars, C., Wieser, O., Kunz, M., and De Alencastro, L. F. (2015). Plastic pollution in Swiss surface waters: nature and concentrations, interaction with pollutants. Environ. Chem. 12, 582–591. doi: 10.1071/EN14218
Ford, H. V., Jones, N. H., Davies, A. J., Godley, B. J., Jambeck, J. R., Napper, I. E., et al. (2022). The fundamental links between climate change and marine plastic pollution. Sci. Total Environ. 806:1150392. doi: 10.1016/j.scitotenv.2021.150392
Gabbott, S., Russell, C., Yohan, Y., and Zalasiewicz, J. (2020). The geography and geology of plastics: their environmental distribution and fate. Plast. Waste Recycl. 2020, 33–63 doi: 10.1016/B978-0-12-817880-5.00003-7
Gasperi, J., Dris, R., Bonin, T., Rocher, V., and Tassin, B. (2014). Assessment of floating plastic debris in surface water along the Seine River. Environ. Pollut. 195, 163–166. doi: 10.1016/j.envpol.2014.09.001
González-Fernández, D., Cózar, A., Hanke, G., Viejo, J., Morales-Caselles, C., Bakiu, R., et al. (2021). Floating macrolitter leaked from Europe into the ocean. Nat. Sustain. 4, 474–483. doi: 10.1038/s41893-021-00722-6
Grill, G., Lehner, B., Thieme, M., Geenen, B., Tickner, D., Antonelli, F., et al. (2019). Mapping the world's free-flowing rivers. Nature 569, 215–221. doi: 10.1038/s41586-019-1111-9
Haberstroh, C. J., Arias, M. E., Yin, Z., and Wang, M. C. (2021). Effects of urban hydrology on plastic transport in a subtropical river. ACS EST Water 8, 1714–1727. doi: 10.1021/acsestwater.1c00072
Honingh, D., van Emmerik, T., Uijttewaal, W., Kardhana, H., Hoes, O., and van de Giesen, N. (2020). Urban river water level increase through plastic waste accumulation at a rack structure. Front. Earth Sci. 8:28. doi: 10.3389/feart.2020.00028
Hurley, R., Woodward, J., and Rothwell, J. J. (2018). Microplastic contamination of river beds significantly reduced by catchment-wide flooding. Nat. Geosci. 11, 251–257. doi: 10.1038/s41561-018-0080-1
Jambeck, J. R., Geyer, R., Wilcox, C., Siegler, T. R., Perryman, M., Andrady, A., et al. (2015). Plastic waste inputs from land into the ocean. Science 347, 768–771. doi: 10.1126/science.1260352
Jang, Y. C., Lee, J., Hong, S., Mok, J. Y., Kim, K. S., Lee, Y. J., et al. (2014). Estimation of the annual flow and stock of marine debris in South Korea for management purposes. Mar. Pollut. Bull. 86, 505–511. doi: 10.1016/j.marpolbul.2014.06.021
Lebreton, L., Egger, M., and Slat, B. (2019). A global mass budget for positively buoyant macroplastic debris in the ocean. Sci. Rep. 9, 1–10. doi: 10.1038/s41598-019-49413-5
Lechthaler, S., Esser, V., Schüttrumpf, H., and Stauch, G. (2021). Why analysing microplastics in floodplains matters: application in a sedimentary context. Environ. Sci. 23, 117–131 doi: 10.1039/D0EM00431F
Lechthaler, S., Waldschläger, K., Stauch, G., and Schüttrumpf, H. (2020). The way of macroplastic through the environment. Environments 7:73doi: 10.3390/environments7100073
Leslie, H. A., Brandsma, S. H., Van Velzen, M. J. M., and Vethaak, A. D. (2017). Microplastics en route: field measurements in the Dutch river delta and Amsterdam canals, wastewater treatment plants, North Sea sediments and biota. Environ. Int. 101, 133–142. doi: 10.1016/j.envint.2017.01.018
Liro, M., van Emmerik, T., Wyzga, B., Liro, J., and Miku,ś, P. (2020). Macroplastic storage and remobilization in rivers. Water 12:2055. doi: 10.3390/w12072055
Malik, N. K. A., Abd Manaf, L., Jamil, N. R., Rosli, M. H., Ash'aari, Z. H., and Adhar, A. S. M. (2020). Variation of floatable litter load and its compositions captured at floating debris boom (FDB) structure. J. Mater. Cycles Waste Manag. 22, 1744–1767. doi: 10.1007/s10163-020-01065-8
Martin, C., Baalkhuyur, F., Valluzzi, L., Saderne, V., Cusack, M., Almahasheer, H., et al. (2020). Exponential increase of plastic burial in mangrove sediments as a major plastic sink. Sci. Adv. 6:eaaz5593. doi: 10.1126/sciadv.aaz5593
Mateos-Cárdenas, A., O'Halloran, J., van Pelt, F. N., and Jansen, M. A. (2020). Rapid fragmentation of microplastics by the freshwater amphipod Gammarus duebeni (Lillj.). Sci. Rep. 10, 1–12. doi: 10.1038/s41598-020-69635-2
Meijer, L. J., van Emmerik, T., van der Ent, R., Schmidt, C., and Lebreton, L. (2021). More than 1000 rivers account for 80% of global riverine plastic emissions into the ocean. Sci. Adv. 7:eaaz5803. doi: 10.1126/sciadv.aaz5803
Mihai, F.-C. (2018). Rural plastic emissions into the largest mountain lake of the Eastern Carpathians. R. Soc. Open Sci. 5:172396. doi: 10.1098/rsos.172396
Moore, C. J., Lattin, G. L., and Zellers, A. F. (2011). Quantity and type of plastic debris flowing from two urban rivers to coastal waters and beaches of Southern California. J. Integr. Coast. Zone Manag. 11, 65–73. doi: 10.5894/rgci194
Newbould, R. A., Powell, D. M., and Whelan, M. J. (2021). Macroplastic debris transfer in rivers: a travel distance approach. Front. Water 3:724596. doi: 10.3389/frwa.2021.724596
Ockelford, A., Cundy, A., and Ebdon, J. E. (2020). Storm response of fluvial sedimentary microplastics. Sci. Rep. 10:1865doi: 10.1038/s41598-020-58765-2
Roebroek, C. T., Harrigan, S., Van Emmerik, T. H., Baugh, C., Eilander, D., Prudhomme, C., et al. (2021b). Plastic in global rivers: are floods making it worse? Environ. Res. Lett. 16:025003. doi: 10.1088/1748-9326/abd5df
Roebroek, C. T., Hut, R., Vriend, P., De Winter, W., Boonstra, M., and Van Emmerik, T. H. (2021a). Disentangling variability in riverbank macrolitter observations. Environ. Sci. Technol. 55, 4932–4942. doi: 10.1021/acs.est.0c08094
Ryan, P. G., and Perold, V. (2021). Limited dispersal of riverine litter onto nearby beaches during rainfall events. Estuarine Coast. Shelf Sci. 251:107186. doi: 10.1016/j.ecss.2021.107186
Saarni, S., Hartikainen, S., Meronen, S., Uurasjärvi, E., Kalliokoski, M., and Koistinen, A. (2021): Sediment trapping - an attempt to monitor temporal variation of microplastic flux rates in aquatic systems. Environ. Pollut. 274:116568. doi: 10.1016/j.envpol.2021.116568
Savenije, H. H. G. (2012). Salinity and Tides in Alluvial Estuaries, 2nd Edn. Delft: Delft University of Technology.
Schöneich-Argent, R. I., Dau, K., and Freund, H. (2020). Wasting the North Sea?–a field-based assessment of anthropogenic macrolitter loads and emission rates of three German tributaries. Environ. Pollut. 263:114367. doi: 10.1016/j.envpol.2020.114367
Schöneich-Argent, R. I., and Freund, H. (2020). Trashing our own “backyard” –investigating dispersal and accumulation of floating litter from coastal, riverine, and offshore sources in the German Bight using a citizen science-based wooden drifter recapture approach. Mar. Environ. Res. 162:105115. doi: 10.1016/j.marenvres.2020.105115
Schreyers, L., van Emmerik, T., Luan Nguyen, T., Castrop, E., Phung, N. A., Kieu-Le, T. C., et al. (2021). Plastic plants: the role of water hyacinths in plastic transport in tropical rivers. Front. Environ. Sci. 9:177. doi: 10.3389/fenvs.2021.686334
Skalska, K., Ockelford, A., Ebdon, J. E., and Cundy, A. B. (2020). Riverine microplastics: Behaviour, spatio-temporal variability, and recommendations for standardised sampling and monitoring. J. Water Process Eng. 38:101600. doi: 10.1016/j.jwpe.2020.101600
Song, Y. K., Hong, S. H., Jang, M., Han, G. M., Jung, S. W., and Shim, W. J. (2017). Combined effects of UV exposure duration and mechanical abrasion on microplastic fragmentation by polymer type. Environ. Sci. Technol. 51, 4368–4376. doi: 10.1021/acs.est.6b06155
Tasseron, P., van Emmerik, T., Peller, J., Schreyers, L., and Biermann, L. (2021). Advancing floating macroplastic detection from space using experimental hyperspectral imagery. Remote Sens. 13:2335. doi: 10.3390/rs13122335
Tasseron, P., Zinsmeister, H., Rambonnet, L., Hiemstra, A. F., Siepman, D., and van Emmerik, T. (2020). Plastic hotspot mapping in urban water systems. Geosciences 10:342. doi: 10.3390/geosciences10090342
Tramoy, R., Colasse, L., Gasperi, J., and Tassin, B. (2019). Plastic debris dataset on the Seine river banks: plastic pellets, unidentified plastic fragments and plastic sticks are the Top 3 items in a historical accumulation of plastics. Data Brief 23:103697. doi: 10.1016/j.dib.2019.01.045
Tramoy, R., Gasperi, J., Colasse, L., Silvestre, M., Dubois, P., Noûs, C., et al. (2020a). Transfer dynamics of macroplastics in estuaries–new insights from the Seine estuary: part 2. Short-term dynamics based on GPS-trackers. Mar. Pollut. Bull. 160:111566. doi: 10.1016/j.marpolbul.2020.111566
Tramoy, R., Gasperi, J., Colasse, L., and Tassin, B. (2020b). Transfer dynamic of macroplastics in estuaries—New insights from the Seine estuary: Part 1. Long term dynamic based on date-prints on stranded debris. Mar. Pollut. Bull. 152:110894. doi: 10.1016/j.marpolbul.2020.110894
Treilles, R., Gasperi, J., Gallard, A., Mohamed, S. A. A. D., Rachid, D. R. I. S., Partibane, C., et al. (2021). Microplastics and microfibers in urban runoff from a suburban catchment of Greater Paris. Environ. Pollut. 287:117352. doi: 10.1016/j.envpol.2021.117352
Treilles, R., Gasperi, J., Tramoy, R., Dris, R., Gallard, A., Partibane, C., et al. (2022). Microplastic and microfiber fluxes in the Seine River: flood events versus dry periods. Sci. Total Environ. 805:150123. doi: 10.1016/j.scitotenv.2021.150123
van Calcar, C. J., and van Emmerik, T. H. M. (2019). Abundance of plastic debris across European and Asian rivers. Environ. Res. Lett. 14:124051. doi: 10.1088/1748-9326/ab5468
van Emmerik, T., Loozen, M., Van Oeveren, K., Buschman, F., and Prinsen, G. (2019b). Riverine plastic emission from Jakarta into the ocean. Environ. Res. Lett. 14:084033. doi: 10.1088/1748-9326/ab30e8
van Emmerik, T., Roebroek, C., De Winter, W., Vriend, P., Boonstra, M., and Hougee, M. (2020a). Riverbank macrolitter in the Dutch Rhine–Meuse delta. Environ. Res. Lett. 15:104087. doi: 10.1088/1748-9326/abb2c6
van Emmerik, T., and Schwarz, A. (2020). Plastic debris in rivers. WIREs Water 7:e1398doi: 10.1002/wat2.1398
van Emmerik, T., Seibert, J., Strobl, B., Etter, S., Den Oudendammer, T., Rutten, M., et al. (2020b). Crowd-based observations of riverine macroplastic pollution. Front. Earth Sci. 8:298. doi: 10.3389/feart.2020.00298
van Emmerik, T., Strady, E., Kieu-Le, T. C., Nguyen, L., and Gratiot, N. (2019a). Seasonality of riverine macroplastic transport. Sci. Rep. 9, 1–9. doi: 10.1038/s41598-019-50096-1
van Emmerik, T., Tramoy, R., van Calcar, C., Alligant, S., Treilles, R., Tassin, B., et al. (2019c). Seine plastic debris transport tenfolded during increased river discharge. Front. Mar. Sci. 6:642. doi: 10.3389/fmars.2019.00642
van Emmerik, T., Van Klaveren, J., Meijer, L. J., Krooshof, J. W., Palmos, D. A. A., and Tanchuling, M. A. (2020c). Manila river mouths act as temporary sinks for macroplastic pollution. Front. Mar. Sci. 7:770. doi: 10.3389/fmars.2020.545812
van Emmerik, T., Vriend, P, and Copius Peereboom, E. (2022). Roadmap for long-term macroplastic monitoring in rivers. Front. Environ. Sci. 9:802245. doi: 10.3389/fenvs.2021.802245
Vriend, P., Van Calcar, C., Kooi, M., Landman, H., Pikaar, R., and Van Emmerik, T. (2020). Rapid assessment of floating macroplastic transport in the Rhine. Front. Mar. Sci. 7:10. doi: 10.3389/fmars.2020.00010
Waldschläger, K., Born, M., Cowger, W., Gray, A., and Schüttrumpf, H. (2020). Settling and rising velocities of environmentally weathered micro-and macroplastic particles. Environ. Res. 191:110192. doi: 10.1016/j.envres.2020.110192
Watkins, L., McGrattan, S., Sullivan, P. J., and Walter, M. T. (2019). The effect of dams on river transport of microplastic pollution. Sci. Total Environ. 664:834–840. doi: 10.1016/j.scitotenv.2019.02.028
Weideman, E. A., Perold, V., and Ryan, P. G. (2020). Limited long-distance transport of plastic pollution by the Orange-Vaal River system, South Africa. Sci. Total Environ. 727:138653. doi: 10.1016/j.scitotenv.2020.138653
Williams, A. T., and Simmons, S. L. (1996). The degradation of plastic litter in rivers: implications for beaches. J. Coastal Conserv. 2, 63–72. doi: 10.1007/BF02743038
Zbyszewski, M., Corcoran, P. L., and Hockin, A. (2014). Comparison of the distribution and degradation of plastic debris along shorelines of the Great Lakes, North America. J. Great Lakes Res. 40, 288–299. doi: 10.1016/j.jglr.2014.02.012
Keywords: macroplastic, hydrology, marine debris, plastic soup, pollution, water quality
Citation: van Emmerik T, Mellink Y, Hauk R, Waldschläger K and Schreyers L (2022) Rivers as Plastic Reservoirs. Front. Water 3:786936. doi: 10.3389/frwa.2021.786936
Received: 30 September 2021; Accepted: 22 December 2021;
Published: 26 January 2022.
Edited by:
Boris M. Van Breukelen, Delft University of Technology, NetherlandsReviewed by:
Luca Solari, University of Florence, ItalyEllen Wohl, Colorado State University, United States
Mauricio Arias, University of South Florida, United States
Copyright © 2022 van Emmerik, Mellink, Hauk, Waldschläger and Schreyers. This is an open-access article distributed under the terms of the Creative Commons Attribution License (CC BY). The use, distribution or reproduction in other forums is permitted, provided the original author(s) and the copyright owner(s) are credited and that the original publication in this journal is cited, in accordance with accepted academic practice. No use, distribution or reproduction is permitted which does not comply with these terms.
*Correspondence: Tim van Emmerik, dGltLnZhbmVtbWVyaWtAd3VyLm5s