- 1CardioMetabolic and Endocrine Institute, Medicine, North Brunswick, NJ, United States
- 2UMDNJ/Rutgers University, New Brunswick, NJ, United States
- 3Department of Geology, University of Peradeniya, Peradeniya, Sri Lanka
- 4Institute of Fundamental Studies, Kandy, Sri Lanka
Importance: More than forty million people from certain tropical countries are at risk of developing a non-conventional form of chronic kidney disease (CKD), CKD of multifactorial etiology (CKDmfo). This is also known as CKD of unknown etiology (CKDu). Worldwide, it kills more than 20,000 people annually.
Findings: CKDmfo is a chronic tubulointerstitial renal disease caused by groundwater-induced hydroxy- and fluorapatite nano-crystals and nano-tube formation in renal tubules and cortical tissues, in conjunction with chronic intravascular volume depletion, chronic renal anaemia, and ischemia. To manifest this gradually developing renal failure, consuming hard water having, higher concentrations of calcium, phosphates, and fluoride for more than 10 years is necessary. The disease progresses when the kidneys fail to repair or due to ongoing renal tissue damage in the presence of micronutrient deficiency, chronic dehydration, renal ischemia, chronic inflammation, and oxidative stress. These prevents regenerations of renal tissues.
Conclusion: Consumption of stagnant groundwater concentrated with ions, like Ca2+, PO4, and F− due to prolonged annual droughts over many years, in conjunction with lesser water intake and chronic dehydration, creates a conducive internal milieu for CaPO4 crystallisation in renal tissues. This proposed primary etiology of the crystal-tubular-nephropathy (CTN) provides an insight into a deeper understanding of the use of cost-effective strategies for prevention, early intervention, and eradication of CKD-CTN. In addition to the nano-crystal/nano-tube concept, we provide supporting scientific evidence that Mg2+ in water and the diet does not promote the disease; instead, it prevents crystal formation and developing CKDmfo, as with some other CKDs.
Relevance: We present novel CaPO4 hydroxyapatite crystal formation concepts in a hyperosmolar fluid in renal tissues that causes CKDmfo. Besides, the protective (e.g., magnesium) and enhancing (e.g., dehydration, drinking stagnant hard water) mechanisms that cause CKD-CTN are explored. A new understanding of causative mechanisms paves a path for cost-effective targeted interventions to prevent and eliminate CKDmfo. These principles apply to all CKDmfo/CKDu-affected countries to protect the renal health of farm labourers and others who regularly engage in physical work in hot and dry environments. Providing affordable potable water, increasing water consumption, and avoiding harmful behaviours are critical measures for eliminating CKDmfo.
Perspective
Question: Why do more than 20,000 people from low-income tropical countries, primarily middle-aged males from farming communities, die from an unusual chronic kidney disease originating from multiple factors and conditions, each year?
Findings: Chronic kidney disease of multifactorial etiology (CKDmfo; CKDu) is an environmentally induced, geogenic—Geological and Biological—chronic tubulointerstitial renal disease. This lethal kidney disease can be cost-effectively prevented and eradicated by implementing straightforward strategic actions, such as public education, providing potable water, and alleviating malnutrition, as described in this article and not by medications, renal dialysis, or constructing renal hospitals.
Implication: For the first time, this study identified a specific cause for the genesis of CKDmfo, designing and enabling of comprehensive, an affirmative and cost-effective public health action for its prevention, which would save livelihoods, the local economy, and thousands of lives.
Introduction
A mysterious chronic kidney disease of multifactorial origin (CKDmfo) or CKD of unknown etiology (CKDu), unrelated to diabetes, hypertension, and other known factors (Wimalawansa, 2016b), threatens the lives of millions of people in tropical countries (Levine et al., 2016; Parameswaran et al., 2020; Redmon et al., 2021). Over 40 million people in several low-income, tropical countries are at risk of developing this unusual kidney disease unrelated to diabetes, hypertension, and other known factors (Wimalawansa, 2016b). CKDmfo is a tubulointerstitial renal disease associated with significant premature deaths (Wimalawansa and Dissanayake, 2019; Wimalawansa, 2020).
The hard water consumed by this vulnerable population is rich in dissolved solids (Abeywickrama et al., 2020). In addition to Ca2+ and Mg2+, water in the affected regions also has higher fluoride concentrations (F−), phosphate () and total dissolved solids (TDS), although not consistently (Wimalawansa and Wimalawansa, 2015a). Those affected live in dry zones at higher elevations above natural and artificial canals. Data suggest that in conjunction with hard water that contains particular ratios of different elements, fluoride consumption (F−) exacerbates this specific type of tubulointerstitial disease (Dissanayake and Chandrajith, 2017). Moreover, the environmental conditions in these regions are conducive to forming nano-mineral calcium phosphate [Ca3(PO4)2; CaPO4] in soft tissues at unique concentrations over an extended period within the renal tubular cells (Wimalawansa and Dissanayake, 2019). These conditions initiate and maintain a persistent low-grade, chronic inflammation in local interstitial tissue, over a period that causes tubular cell fibrosis (Gunawickrama et al., 2021).
Environmental Factors That Initiated CKDmfo—Groundwater, Soil Conditions, and Harsh Weather Conditions
Most CKDmfo-affected regions have low humic, gley soil, and shallow non-permeable hard bedrock underneath. The presence of predominantly clay soil with less sand, coupled with the overextraction of groundwater by agro-wells, may have altered the underground water dynamics in these regions. The natural weathering process constantly releases heavy metals and fluoride into groundwater. In affected countries, the situation aggravates due to governmental neglect and ongoing multiple harmful behaviours of the affected populations rapid progress of agriculture, and other environmental reasons described below.
In countries where CKDmfo is endemic, the affected regions, mostly new settlements, have poor soil drainage. People settled in these new regions (now affected with CKD-CTN) over several decades without proper planning, sanitation, and potable water. This disease neither exclusively affects farmers nor is caused by any agrochemical. In Sri Lanka, thousands of families resettled from the south to the north-central province (NCP), where there are no direct, natural water supply. The water table is deep (groundwater) due to the regolith structure not connecting with the surface water.
New migrants had to dig wells into tapping groundwater for drinking and other purposes. Consequently, thousands of tube wells were constructed in these regions to obtain water for drinking and agricultural purposes. A few years later, the Mahaweli River, the longest river in Sri Lanka, was diverted from the central province to the NCP to facilitate agriculture and other needs (Wimalawansa, 2014a; Weeraratne and Wimalawansa, 2015). The government promised these families supplies of adequate water from the Mahaweli River. However, the water was provided only to low-lying paddy fields and not to living locations. This mass migration-related development program in Sri Lanka was implemented in the late 1970s and accelerated in the 1980s without providing the basic human needs, addressing environmental factors, or assessing groundwater quality (Weeraratne and Wimalawansa, 2015).
The Preamble to CKDmfo
Although the regolith aquifer from the NCP region extends to the south of the country, towards Ampara and Monaragala and partially towards the west towardss the Puttalam district, the soil structures in these areas are distinctly different from the CKDmfo-affected locations. Farmers use similar suites of agrochemicals for farming, significantly with higher concentrations in areas like the upcountry. A key difference is that groundwater in CKDmfo non-endemic regions have neither very hard water nor higher fluoride contents. Therefore, in the absence of mentioned regolith formations, non-endemic regions have minimal or no CKDmfo (Levine et al., 2016; Wimalawansa, 2016a,b; Thammitiyagodage et al., 2017; Edirisinghe et al., 2018; Balasooriya et al., 2020; Gobalarajah et al., 2020; Imbulana and Oguma, 2021).
To complicate matters, while the groundwater is very hard in areas, such as Puttalam and Jaffna districts, it has very low F− content and has a very low prevalence of CKDmfo. These data suggest that drinking hard water alone does not cause CKDmfo (Weeraratne and Wimalawansa, 2015; Wimalawansa, 2015a). Few other locations in the country have high F− (and iron) contents in water, but water is relatively soft, and people living in these areas also have a very low prevalence of CKDmfo. These data show that consuming hard water (Ca2+) or water with higher concentrations of F− alone is insufficient to cause CKDmfo (Wimalawansa, 2016b, 2019a, 2020; Dunuweera et al., 2017; Wimalawansa and Dissanayake, 2019).
The Role of Ca2+, and F– in Renal Nano-Crystal Formation
Besides those agents generating superoxide radicals, excess fluoride (F−) also increases the kidneys' oxidative stress, catalases, and lipid peroxidation (Khalili and Biloklytska, 2009). In one study, rats treated with F− reduce intrinsic antioxidative components, such as glutathione, glutathione reductase, glutathione peroxidase, and superoxide dismutase in renal tissues (Birkner et al., 2006; Bharti et al., 2014). In addition, total phospholipids, phosphatidylethanolamine, phosphatidylcholine, and ubiquinone content in renal tissues are also significantly reduced (Gao et al., 2008; Despres et al., 2019). These data suggest that higher exposure to F− can be highly oxidative and toxic to renal tubules.
Formation of CaPO4-Hydroxyapatite and Fluorapatite
In vivo, renal CaPO4-hydroxyapatite formation is common. However, in general, these structures are unstable [Ca5(PO4)3(OH) = Ca2+ + 3 + OH−]; thus, not sustained. However, when F− substitutes the hydroxyl group in apatite, it creates the more stable fluoroapatite [5Ca2+ + 3 + F−Ca5(PO4)3−F] due to stronger ionic and hydrogen bonds that stabilise the structures. With time, matrix proteins are also incorporated into hydroxy-fluorapatite, strengthening nano-minerals that resist enzymatic degradation (Robinson et al., 2004).
For example, F− integrated into CaPO4 apatite in dental enamel tissues produces crystals resistant to decay. A minute concentration of F− incorporated into dental and skeletal tissues further strengthened the resultant carbonate-apatite crystals resistant to decay (Aoba, 1997). Figure 1 illustrates the multiple mechanisms and pathways of developing nano-mineral crystallisation in renal tubular tissues. These nano-crystals cause chronic low-grade inflammation and oxidative stress, leading to tubular cell apoptosis, fibrosis, and renal failure.
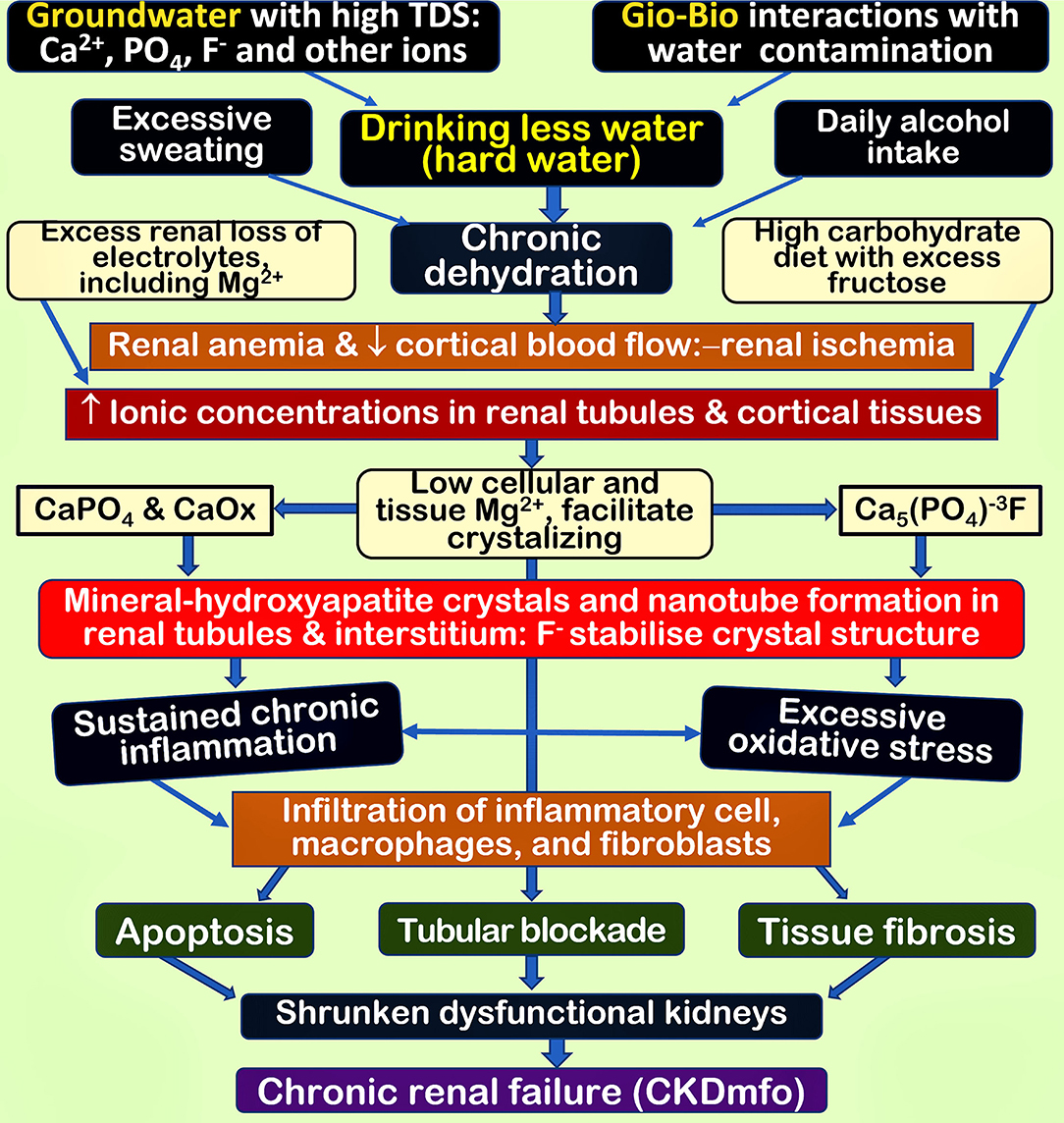
Figure 1. Inter-related mechanisms and multiple pathways leading to the formation of nano-minerals in renal tubular and cortical soft tissues. The fundamental issue is high mineral concentration in the renal cortical fluid and tissues, secondary to ingestion of hard water and chronic dehydration. These multiple factors working together aggravate renal ischemia and anaemia and inflammatory cell infiltration due to nano-mineral precipitation, causing chronic inflammation and oxidative stress. Collectively, these lead to deterioration of renal functions and renal fibrosis, causing irreversible CKD-CTN (CKDmfo) [TDS, total dissolved solids; Ca2+ and , Mg2+, etc.); CaPO4, calcium phosphate; CaOx, calcium oxalate; Mg2+, magnesium; F−, fluoride]. The figure is modified from Wimalawansa and Dissanayake (2019).
Nano-Tube and Crystal Formation in Renal Tissues
Ultrastructural studies in human kidney tissue biopsies of patients with end-stage CKD reported 50- to 1,500-nm multi-lamellar mineral particles within renal tubules. However, no such observations were reported in non-tubular interstitial diseases (Wong et al., 2015). These polycrystalline CaPO4 nano-mineral particles are also found in extracellular matrices and the cytoplasm of tubular cells. When the common form of CKD or CKDmfo advanced to stages beyond stage IIIB, it begins to cause glomerular damage and sclerosis causing proteinuria. Crystalline nephropathies are a unique form of kidney disease characterised by the histologic finding of intra-renal crystal deposition. When some crystals combine with ions like F− in beneficial tubular fluid conditions, crystal precipitation occurs in tubular lumens and surroundings, promoting inflammation, cytotoxicity, and kidney injury (Perazella and Herlitz, 2021).
However, low molecular weight proteins and larger peptides are present in the glomerular filtration and tubular fluids even without glomerular damage: these are secreted or leaked from renal tubular cells. As tubular damage progresses, it increasingly fails to reabsorb larger peptides and proteins secreted from tubular cells, such as liver-type fatty acid-binding protein. These are measurable in urine as markers of renal tubular injury (Birkner et al., 2006), and could also get incorporated into CaPO4 crystals.
Compared to hydroxyapatite, fluoridated nano-minerals are more resilient. Therefore, it facilitates crystal growth and nano-tube formation. This process attracts inflammatory cells and accelerates inflammation (Hodgkins and Schnaper, 2012; Gunawickrama et al., 2021). Later, growing crystals could block renal tubules, and nano-tubes could rupture tubular walls, attracting white blood cells and macrophages. Progressive low-grade chronic inflammation leads to tissue fibrosis and renal failure over a few years. These crystals and nano-tubes are foreign bodies that generate inflammatogenic responses, attracting inflammatory cells and fibroblasts that cause tissue fibrosis.
Immune cells attracted to tubulointerstitial tissues activate tyrosine kinase and caspase-1 and release various inflammatory cytokines fuelling the inflammation, accelerating renal tissue fibrosis (Birkner et al., 2006). Similar nano-mineral mechanisms causing renal toxicity have been described before (Buzea et al., 2007). Table 1 illustrates supporting evidence that CaPO4 nanoparticles are highly likely to cause nano-mineral formation in renal tubules and the interstitial tissues in CKDmfo.
Mechanisms of Formation of Nano-Tubes in Renal Tubules
Groundwater in the CKDmfo-prone dry zonal areas in affected countries is hard (CaCO3), containing high TDS and other components favourable for forming apatite minerals—CaPO4 hydroxyapatite and fluoroapatite (Dissanayake, 2016). Due to the crystal-related inflammation and micro-injuries, tubular epithelial cells undergo structural and functional changes. With tubular cell damage, fibrogenic cells infiltrate into renal parenchymal tissues attracting bioactive inflammatory and oxidative molecules that enhance inflammation and interstitial fibrosis.
With the above-mentioned local damage, tubular cells are subjected to progressive renal injury via epithelial–mesenchymal cell transition, cell-cycle arrest at G1/S and G2/M points, and ongoing oxidative and metabolic abnormalities (Liu et al., 2018). In vivo, these cause tubular cell inflammation, damage, dysfunction, and tubular blockade. Most people having CKD or CKDmfo are malnourished (Kalantar-Zadeh et al., 2003; Gama-Axelsson et al., 2012). Virtually all are micronutrient deficient. In addition to poor diet, impairment of intestinal and renal tubular fractional absorption of minerals, and lack of reabsorption of peptides, proteins makes the condition worse (Zha and Qian, 2017).
Hypocitraturia is a common metabolic risk factor in many persons with renal stones, as Citrate is an inhibitor in Ca stone formation. Increasing urinary citrate can act in renal tubules to reduce calcium oxalate (CaOX) and CaPO4 saturation in tubular fluid and prevent crystal formation and growth. Urinary citrate can increase by ingesting lime juice or potassium citrate (Joshi et al., 2021), which could prevent the adhesion of calcium crystals to tubular cell membranes (Figure 2B) and renal epithelial cells (Zuckerman and Assimos, 2009). A few examples of CaPO4 crystals are illustrated in Figure 2.
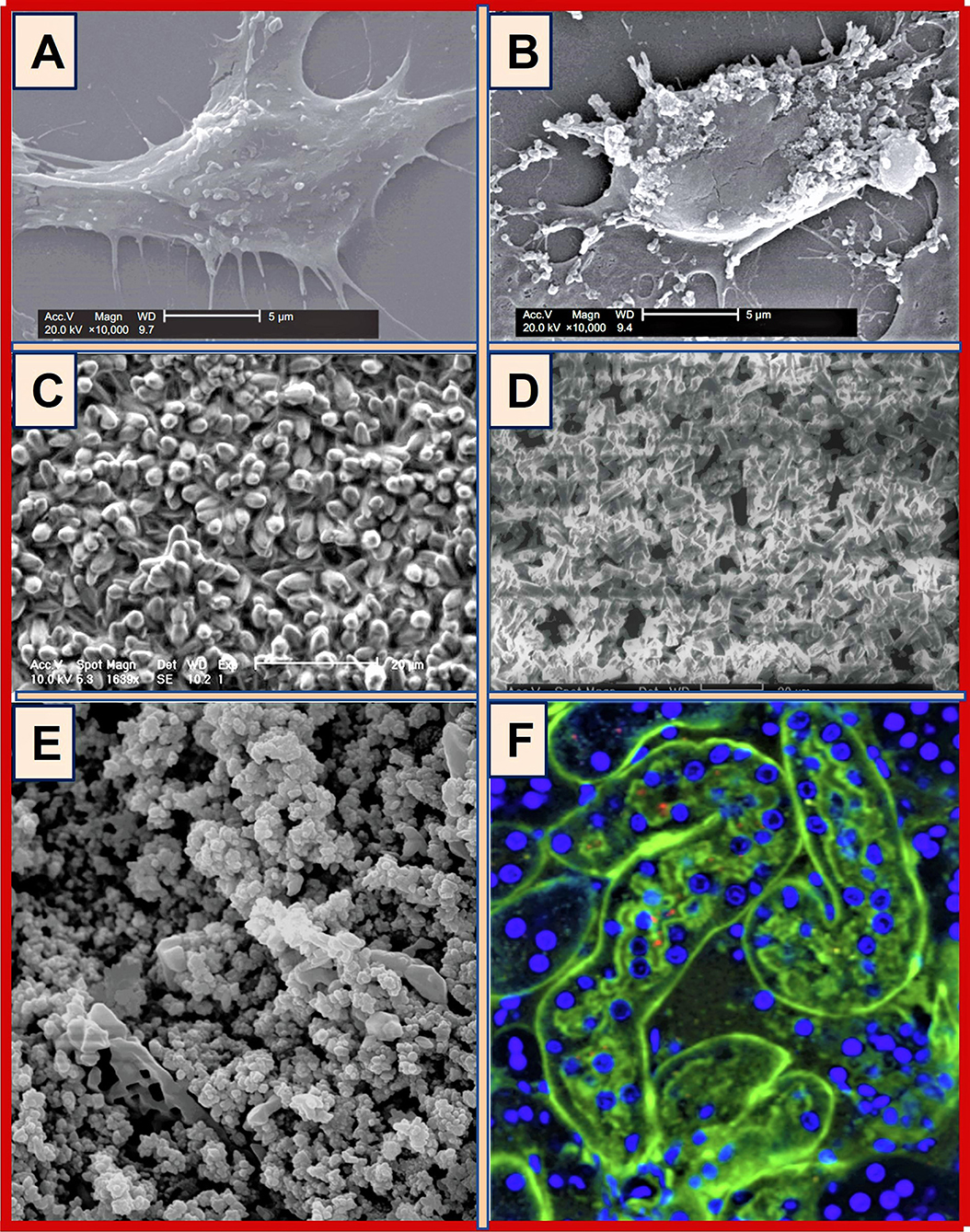
Figure 2. Examples of hydroxyapatite and fluorapatite crystals: (A) CaPO4-crystal formation on the surface of Vero cells treated with H2O2–(A) control; (B) 0.5 mmol/l, H2O2 treatment (scale bars: 5 μm) [COD, calcium oxalate dihydrate; SEM, scanning electron microscopy] (modeled from Gan et al., 2016); (C) SEM images of fluorapatite crystal growth on etched titanium surface; (D) Random growth of fluorapatite crystals on glass (adapted from Czajka-Jakubowska et al., 2009); (E) SEM image of hydroxyapatite in aqueous solution (after Arnich et al., 2003); (F) CaPO4 nano-crystals in renal tissue and tubular cells in mice [CaPO4 crystals in tube lumens (green; FITC-dextran); cell nuclei (blue; Hoechst)] (after Shiizaki et al., 2021).
Excess PO4 Causing Nano-Crystal Formation in the Kidney
Hardness in groundwater is primarily due to calcium and magnesium salts: generally acceptable highest limits in drinking water (less than) are TDS 500 mg/l, calcium 75 mg/l, Mg2+ 50 mg/l, alkalinity 250 mg/l, SO4 200 mg/l, and 0.025 mg/l, and EC 1,800 μS/cm (Unicef/WHO, 2008). However, the water hardness in the CKDmfo-affected NCP regions are often well above 500 mg/l, mostly CaCO3; the concentration of Mg2+ is much less, between 15 and 30 ng/ml, well below the EPA and WHO guidelines (Unicef/WHO, 2008; Wimalawansa, 2016a). However, as described below, concerning CKDmfo, interactions (additive or synergistic effects) between different ion ratios have not been explored (Dunuweera et al., 2017; Wimalawansa, 2019a, 2020; Wimalawansa and Dissanayake, 2019). Figure 3 illustrates a renal biopsy specimen stained for CaPO4 crystals and soft tissues, highlighting the presence of CaPO4 crystals within renal tubules and in surrounding tissues.
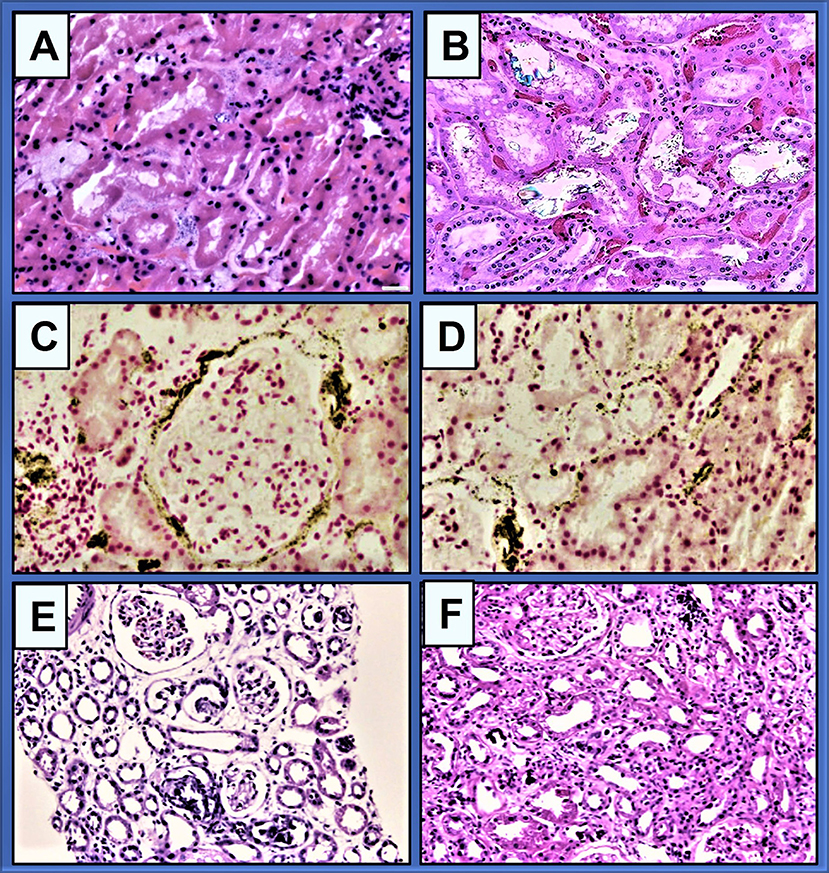
Figure 3. (A) Granular and globular morphologic characteristics of CaPO4 crystal deposition in renal biopsies. Crystals are mildly basophilic on haematoxylin-eosin stained and non-birefringent under polarised light (original magnification, × 400; bar, 20 μm) (after Zhang et al., 2021); (B) Granular crystals in a renal biopsy, in inherited crystalline nephropathy (after Perazella and Herlitz, 2021); (C,D) There are granular CaPO4 crystals in the interstitium and along the tubular basement membranes, peritubular capillaries, and Bowman capsules. Confirmation of CaPO4 using von Kossa staining (magnification, ×400; bar, 20 μm) (after Zhang et al., 2021); (E,F) An example of phosphate nephropathy illustrating intratubular CaPO4 crystals, tubular atrophy, and interstitial fibrosis (haematoxylin and eosin stain: after Fogo, AB. AJKD) (Fogo et al., 2017).
It is unclear whether any food component or malnutrition increase the susceptibility to CKDmfo or chronic disease (poverty-associated) causing malnutrition. When the solubility product of Ca2+ and exceeds the threshold, CaPO4 nanoparticles are formed (Ho et al., 2018). This vicious cycle is facilitated by excess present in water (derived from waterways and reservoirs) and Ca2+ in hard water (Wimalawansa and Dissanayake, 2019) in the affected regions secondary to the overuse of phosphate fertiliser in hilly areas of the country (Wimalawansa, 2015a).
Chronic Dehydration Leads to Saturation of Tubular Fluid CaPO4
Patients with tubular crystallopathy typically have hypokalaemia (potassium, 2.2 m Eq/l), hypochloraemia (chloride, 97 mEq/l), and hypomagnesemia (magnesium, 1.0 mg/dl; 0.41 mmol/l), together with raised serum creatinine and PO4. Secondary to excessive sweating, lesser water intake, and regular alcohol use, maintains chronic persisting dehydration and saturation of the interstitial and tubular fluid microenvironment conducive for precipitation of CaPO4 and CaOx. Consequently, it increases the risk of forming and propagating nano-minerals within tubules, interstitium, and tubular cells (Tiselius, 2013; Sethmann et al., 2017). This phenomenon also increases the risks of vascular calcification with all CKD types, increasing the risks of premature deaths (Birkner et al., 2006).
The key factors essential for triggering nano-tube and nano-crystal formation include chronic dehydration and associated renal ischemia, as well as regular longer-term consumption of hard water with high TDS, CaPO4, and fluoride cause (i.e., transient, or persistent higher concentration of tissue Ca2+ and concentration). The combination of the mentioned multiple factors creates a vicious cycle of enhanced oxidative stress, chronic inflammation (Gunawickrama et al., 2021), and tubular cell fibrosis, causing CKDmfo. Figure 4 summarises the breakdown pathways of the Geo-Bio barrier causing precipitation of nano-minerals in renal tubular tissues leading to chronic inflammation, tissue fibrosis, and renal failure.
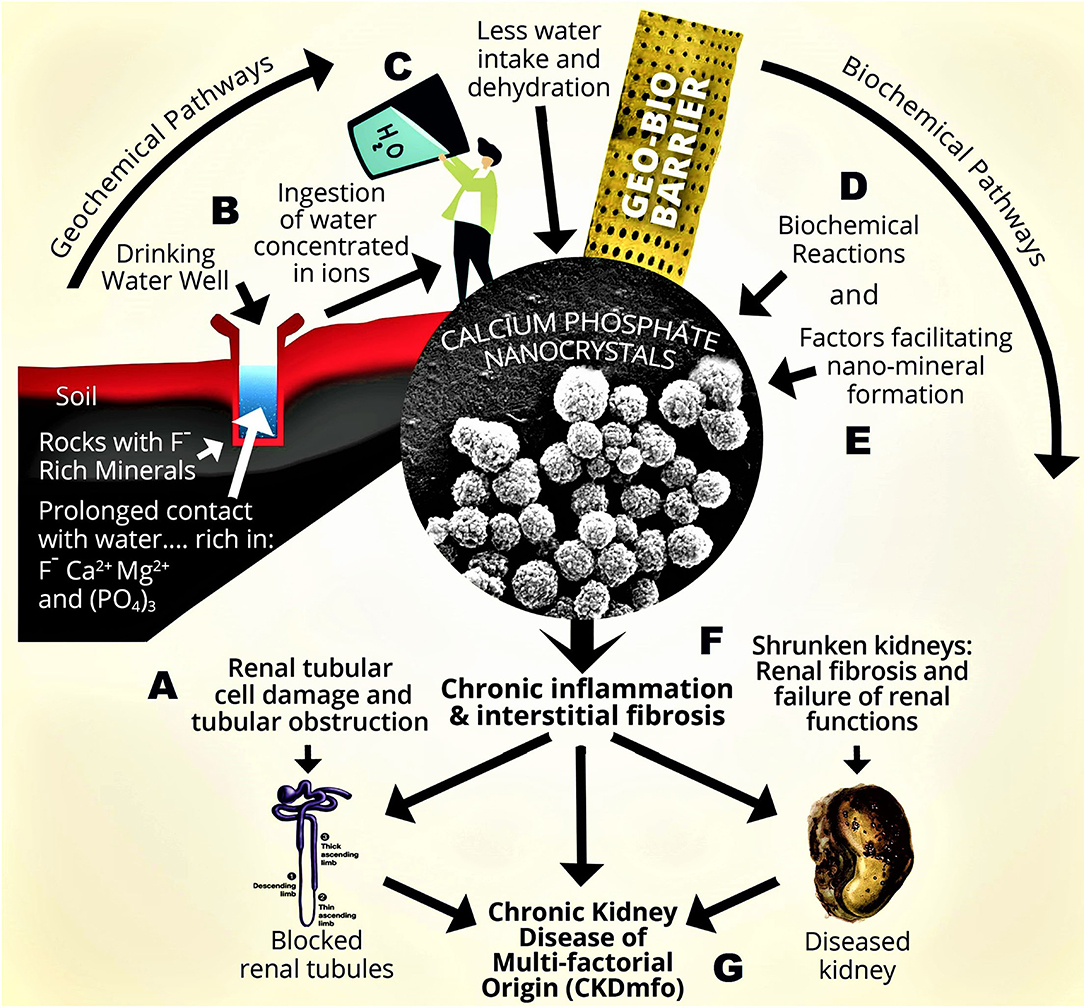
Figure 4. Geological and biological (Geo-Bio) pathways for forming nano-tubes and nano-crystals in chronic kidney disease of CKD-CTN (CKDmfo). A conceptual diagram illustrating pathways for the formation of CaPO4 nano-crystals in kidney tubules causing CKDmfo: (A) Stagnant groundwater is interacting with mineral-rich rocks, containing F−, Ca2+, Mg2+, and . (B) Long duration of water–rock interaction. (C) Consumption of hard water (lesser amounts) with high concentrations of ions. (D) Biochemical reactions and supersaturation, precipitating CaPO4 nano-crystals and nano-tubes in kidney tubules. (E) Factors enhancing the nano-mineral precipitation. (F) Low-grade chronic inflammation, oxidative stress, and tissue fibrosis. (G) Onset of CKDmfo [TDS = total dissolved solids (Ca2+ and , Mg2+, etc.); Mg2+, magnesium; F−, fluoride; CaPO4, calcium phosphate; CaOx, calcium oxalate].
It is not unusual that farm labourers in the CKDmfo-affected regions are chronically dehydrated. They regularly experience excessive sweating due to exposure to harsh, warm, and humid weather conditions, consume less fluid due to unpalatable hard water, and the daily use of illicit alcohol daily. These conditions are favourable in generating concentrated urine and tissue fluids in the renal cortical regions, driving nano-mineral formation (Wimalawansa, 2014a). Renal tissue nano-minerals are not visible by standard radiological methodologies. However, when nano-crystals grow above 1 mm (as in soft tissue and coronary calcification), they become visible with high-density imaging methods, such as computed tomography.
Local Conditions in the Kidney That Facilitate Crystallisation
There are several types of calcium-related nephropathies. Common types of renal tubular crystals formation are crystal nephropathy (type 2), damage to renal vasculature (type 1), and urinary urolithiasis (type 3); all these cause renal injuries that can lead to CKD (Mulay and Anders, 2017). Crystals formed by minerals, proteins, endogenous metabolites, or exogenous drugs and toxins also could cause tubular crystallopathies (Mulay and Anders, 2017).
Formation of crystals and other microparticles causes renal damage via different mechanisms. For example, the NLRP3 inflammasome causes apoptosis via a pathway through a speck-like protein containing CARD/caspase-1 (NLRP3/ASC/caspase-1) (Schroder and Tschopp, 2010; Mulay and Anders, 2017) and exert cytotoxicity through the necroptosis signalling pathway (Mulay and Anders, 2017), whereas vitamin D has a profound immunomodulatory effect in suppressing NLRP3/ASC/caspase-1 and toll-like receptor-induced harm (Matias et al., 2021; Nunes et al., 2022)—therefore is a potential mode for therapeutic intervention. However, slowly growing crystal-mediated granuloma formation, as with CKDmfo, is present in chronic crystallo-nephropathies that lead to CKD and renal fibrosis.
Other Causes and Conditions That Increase the Risk of CaPO4 Crystal Nephropathy
As described in the introduction, water hardness or F− alone does not cause CKDmfo. For example, in Sri Lanka, in Jaffna, Ampara, and Puttalam districts, water hardness is >1,000 mg/l. In CKDmfo-unaffected areas like hill-country, farmers use higher agrochemical quantitates without CKDmfo (Wimalawansa, 2016a). While the natural phosphorus levels are <0.03 mg/l, orthophosphate levels vary between 0.005 and 0.05 mg/l [4], but what is clinically meaningful is the total content. In CKDmfo-affected regions, the average concentration of reservoirs water is higher, between 0.8 and 1.4 mg/l (Dharma-Wardana et al., 2015; Wimalawansa, 2015a). Nevertheless, concentrations alone are not too high to cause health hazards. Meanwhile, the consumption of hard water (Ca2+), coupled with intake of higher concentrations of ± F− for more than a decade in the presence of a favourable milieu increases the risks for CaPO4 crystallisation in renal tubules.
Metallothioneins I/II (MT) have protective and anti-apoptotic effects in renal proximal tubular cells. The expression of these molecules suppresses the excessive oxidative stress, including that induced by F− (Chmielewska et al., 2015). In addition, endogenous antioxidants and MTs induced by zinc (Zn) and selenium (Se) protect against oxidation and ischemic injuries (Birkner et al., 2006). Meanwhile, there is no credible scientific evidence that arsenic, heavy metals, or agrochemicals cause CKDmfo. Even if small quantities of any of these get absorbed into the gastrointestinal tract or absorbed, there are built-in inherent protective mechanisms, such as chelation, enzymatic reactions, and detoxication (MTs) in the gut, liver, and kidneys that would eliminate most of these (Goyer, 1989; Hma et al., 2018).
It is noteworthy that the WHO recommended water quality standards are focused on individual elements without considering potential added and synergic effects between macro and micro-trace elements (1997; Wasana et al., 2017) or changes of effects from pH. Nevertheless, there are many biological and physiological examples where such interactions can be beneficial or harmful. Based on small-scale rat studies using hard water (Ca2+ or Mg2+), potential additive effects of F− have been reported with Mg2+ and suggested as a cause for CKDmfo. However, these models neither reflect the reality of ion–ion interactions (see above) nor reflect the human behaviours in affected populations (Wasana et al., 2017; Torreggiani et al., 2020).
Mg Deficiency Contributes to Nano-Crystal Formation and CKDmfo
Mg2+ is an important macro-mineral that is essential for enzymatic reactions. In renal tissues, it competes with Ca2+ in determining the rate and the concentrations of accumulation of Ca2+ and homeostasis of elements. Higher concentrations of Mg2+ antagonise Ca2+, preventing CaPO4 and CaCO3 nano-crystal formation. In contrast, chronic hypomagnesemia increases the risk of CaPO4 nano-crystal formation in renal tissues. Moreover, in rats fed on an Mg2+-deficient diet, the deficiency-associated complications are accentuated by low dietary potassium. Thus, the functions of these cations and anions are interlinked. Therefore, using these ions individually in experiments to assess their functions can be misleading.
While high dietary calcium intake reduces plasma Mg2+ (Ericsson et al., 1986), supplementation of Mg2+ or low-dose fluoride treatment mitigates the accumulation of unphysiological calcium levels in renal tissue. Researchers need to incorporate the mentioned critical and complex status related to dietary and drinking water and ion–ion interactions. Therefore, studies should not be designed in isolation but should consider potential additive or competitions of ions in vivo physiological situations.
Hundreds of studies have reported the renal protective effects of Mg2+, including renal electrolyte handling and maintenance of in vivo electrolyte balances of critical elements, such as Na+, K+, , Mg2+, and Ca2+, vital for health (Wassenaar et al., 2018; Mazzaferro et al., 2021). Nevertheless, due to hormonal abnormalities, such electrolyte imbalances (i.e., dyshomeostasis) are common in all CKD types, especially tubulointerstitial diseases (CKD-CTN) such as CKDmfo. These get worsened in the presence of malnutrition (Wang et al., 2018; Bousquet-Santos et al., 2019), which is universally present in persons with CKDmfo. While Mg2+ supplementation is helpful, the ideal way to manage electrolyte homeostasis is through a balanced diet (Anupama et al., 2020).
Does Magnesium Contribute to the Causation of CKDmfo?
Hypomagnesemia worsens several common disorders, such as diabetes, metabolic syndrome, parathyroid and other endocrine disorders, ostoporosis, and all of CKD types, leading to poor clinical outcomes (Al and Rodriguez, 2013). In vitro and in vivo models demonstrated that tissue hypomagnesaemia worsens several disorders, including CKD, cardiovascular diseases, vascular and soft tissue calcification, fatigue and depression, and impair bone-mineral metabolism (Al and Rodriguez, 2013; Galassi and Cozzolino, 2014; Floege, 2015; Wassenaar et al., 2018). Therefore, it is difficult to envisage how a smaller increase in the concentration of Mg2+ in drinking water as postulated by some (minute quantities compared to Mg2+ ingested via food (Wimalawansa, 2016a; Liyanage et al., 2022) could cause CKDmfo (Wasana et al., 2016, 2017). As illustrated in Table 2, one needs to be concerned with hypomagnesemia and not excess Mg2+ in the circulation and kidneys.
Hypomagnesemia increases the risk of CaPO4 nano-crystal formation in renal tissues. Whereas Mg2+, alpha-Klotho, vitamin K, and zinc prevent CaPO4-nano-crystal formation in the kidney, FGF23 and calciprotein particles modulate this process (Vervloet, 2019). Mg2+ deficiency can be detrimental to most organ systems, including endocrine, bone, renal, and cardiovascular (Table 2). It is a common and effective way to use metallic element-based binders, such as Mg2+, aluminum, and Ca2+, to bind to prevent the load and reduce CKD-metabolic bone disease (CKD-MBD) (Vervloet, 2019; Pendon-Ruiz De Mier et al., 2020). In addition, Mg2+ supplementation reduces vascular calcification, prevents renal function deterioration, and stabilises CKD-MBD. Mg2+ also improves all hormonal actions, including calcitriol, and its (vitamin D) receptor functions. These are also important in CKDmfo (Galassi and Cozzolino, 2014; Pendon-Ruiz De Mier et al., 2020; Kula and Bansal, 2021).
While it may not be fully implementable due to poverty, changing dietary habits, including clean (potable) water intake, is essential to preventing deterioration CKDmfo. Such interventions improve the general condition of those affected and reverse early renal abnormalities in the initial stages of the disease. In contrast, many other studies confirmed improved general wellbeing and reversing the initial stages of CKD and CKDmfo when supplemented with magnesium or magnesium-based phosphate-binding agents (Al and Rodriguez, 2013; Floege, 2015). Table 2 illustrates the potential causes of Mg2+ depletion and hypomagnesemia in CKD patients.
As illustrated in Table 2, in addition to acute and chronic diseases, there are specific conditions and circumstances where hypomagnesemia is manifest. Except for inherited/genetic diseases, most others are easily modifiable.
Contaminated Groundwater vs. Spring Water
Ongoing seismic and geochemical processes aid the erosion of underground bedrocks, releasing various ionic components into the water, including CaPO4 and F−, into groundwater (Mikac et al., 2011). During the construction of deep tube wells, some tap into F−-rich groundwater pockets, pumping out water containing high content of F− and other contaminants (Wimalawansa, 2020). In parallel, the PO4 concentrations in surface (e.g., reservoirs) and groundwater have increased due to the excessive application of agrochemicals in agriculture in distinct regions (Wimalawansa, 2016b).
The increasing demand for water that began in the 1960s to resettle communities and vast expansion of agriculture needed to overexploit groundwater in the CKDmfo-affected dry zonal regions (Panabokke, 2007). Besides the CKDmfo-affected regions in Sri Lanka, there are shallow regolith aquifer (between 5 and 20 m) and a deeper fracture zone aquifer (between 30 and 40 m) (Imbulana and Oguma, 2021). Most large agro-wells and domestic dug wells in this region are tapped into regolith aquifers (Pathmarajah, 2002).
The occurrence of higher hardness (the equivalent of CaCO3, above 385 mg/l) and alkalinity (CaCO3, above 365 mg/l) and high TDS are salient parts of groundwater in CKD-affected villages. In comparison, although present in some regions, salinity and ionicity and higher Mg2+ contents do not directly contribute to the causation of CKDmfo. Ongoing chemical weathering and dissolution of carbonates and silicates rocks [Ca-Mg-HCO3] represent the geochemistry of groundwater. Continual weathering of carbonate and silicate components in regolith aquifers in the CKDmfo-affected regions, enhanced by overexploitation, evaporation, and cation ion exchange, all significantly influences the hardness, electrical conductivity (EC), and dissolved components in hard, groundwater, raising Ca2+, Mg2+, and HCO3 concentrations (Elango and Kannan, 2007) in affected countries.
Isotopic studies suggested that CKDmfo-endemic villages are associated with groundwater sources and not fed directly with rain or surface water (Edirisinghe et al., 2018). Therefore, ions and chemical pollutants get considerably concentrated in groundwater during the dry season, increasing the risks for CKDmfo (Wimalawansa, 2020). In contrast, those who consume surface or spring water have a significantly lower incidence of CKDmfo. Besides, the prevalence of CKDmfo is higher in those who consume water from deep wells connected with the stagnant aquifer (Balasubramanya et al., 2020) but not with shallow wells.
There are dozens of natural springs amid CKDmfo-affected regions. Springwater quality is far better than groundwater and does not require processing before consumption. Residents who consume water from such natural springs are rarely affected by CKDmfo even though these villages are located adjoining those with a high incidence of CKDmfo (Jayasekara et al., 2013; Wimalawansa, 2015b; Wimalawansa and Wimalawansa, 2015b). Isotopic studies demonstrate that such natural springs are not connected to the groundwater aquifers (Wimalawansa, 2016b; Wickramarathna et al., 2017; Edirisinghe et al., 2018).
Higher total hardness in water is universal in the CKDmfo-affected regions (Weaver et al., 2015). In contrast to higher Mg2+ content reported in some groundwater samples (Edirisinghe et al., 2018), others have reported lower Mg2+ concentrations in samples from CKDu-endemic areas compared with the non-endemic areas (Dissanayake and Chandrajith, 2007; Wasana et al., 2016). Thus, having elevated Mg2+ in groundwater is incidential, and, not common to all affected villages. In contrast, most CKDmfo-affected locations have higher F− concentrations in groundwater (Wimalawansa, 2016b; Imbulana and Oguma, 2021). As described below, Mg2+ protects renal tissues partly from its antagonistic effects on Ca2+ and PO4 (Ter Braake et al., 2017; Sakaguchi et al., 2018).
The Amount of Mg2+ Derived From Drinking Water
Most CKDmfo-endemic regions have regolith aquifers with higher, Ca2+, Mg2+, F−, and bicarbonate concentrations. Increases in ions in stagnant water are due to prolonged contact time with an aquifer, weathering of underground rocks, and evapotranspiration. Importantly, those wells connected to regolith aquifers used by residents in the affected locations are not connected to the surface agricultural water table/sources, such as water reservoirs and irrigation canals, as reported by isotopic tracer studies (Rango et al., 2015; Wickramarathna et al., 2017; Edirisinghe et al., 2018).
The finding of marginally higher Mg2+ in some water samples (but within the WHO-recommended upper limit) (Wasana et al., 2016, 2017; Liyanage et al., 2022) seems coincidental and non-significant on clinical grounds (Balasooriya et al., 2020) and remains a hypothesis. Reported rat studies suggested a connection between hard water Mg2+/F− and CKDmfo (Thammitiyagodage et al., 2017; Wasana et al., 2017). One study used water from an endemic region, while the other used laboratory-made, hard water (Wasana et al., 2016, 2017; Thammitiyagodage et al., 2017). While being attractive, such data are too remote to conclude the causation of CKDmfo, especially with hundreds of other scientific studies confirming the protective nature of Mg2+ on renal functions.
The contribution of Mg2+ from drinking water to daily intake is negligible. One average-sized banana supplies more minerals and micronutrients than the total daily intake of such from water. The average amount of Mg2+ intake from 2–l drinking water is between 20 and 30 ng/ml (even in water from CKDmfo-affected locations) (Balasooriya et al., 2020), while a medium-sized banana provides ~65 mg (Wimalawansa, 2016a). Therefore, it is hard to envisage how <20 mg/l in drinking water (Wickramarathna et al., 2017) would cause CKDmfo, even if interactions could be present. The tolerable upper limit of Mg2+ supplementation is 350. Consuming higher doses than this without Mg2+ losing disorders, as illustrated in Table 2, for a prolonged period can cause hypermagnesemia (Wyskida et al., 2012). Like other minerals, prolonged hypermagnesemia increases morbidities and all-cause mortality (Galan Carrillo et al., 2021).
The Role of Mg2+ in Renal Diseases
In addition to maintaining homeostatic and physiologic balances in the body, Mg2+ facilitates and functions as a cofactor in biochemical and enzymatic reactions, neural conduction, and the release of peptides and hormones and activating receptors (Greco, 2012). Normal serum magnesium levels are between 1.56 and 2.68 mg/dl (Gragossian et al., 2021). The kidney's pathological handling of Mg2+ can result in either hypomagnesemia or hypermagnesemia (Cunningham et al., 2012; Massy and Drueke, 2015; Quinones et al., 2019; Van Laecke, 2019). Serum Mg2+ reflects poorly, the body storage of magnesium.
Hypomagnesemia is present when the serum total magnesium concentration drops below 1.50 mg/dl. It can indirectly lower serum and tissue potassium and increase serum calcium concentrations, further impairing electrolyte imbalance (Quinones et al., 2019). Clinical signs and symptoms of severe hypomagnesemia include tremors, generalised muscular weakness, cardiac arrhythmia or ischemia, dysfunction of hormones, and death (Cunningham et al., 2012). In addition, persistent hypomagnesemia significantly increases vascular calcification, endothelial dysfunction, cardiovascular diseases (Van Laecke, 2019), increase vulnerability to infections, and all-cause mortality, especially in those with CKD stages 3 and above (Quinones et al., 2019; Azem et al., 2020).
Hypomagnesemia and Hyperphosphatemia Exacerbate CKDmfo
In addition, hypomagnesemia increases the intracellular Ca2+ concentration through negative-regulation of calcium channels, suppressing N-methyl-D-aspartate receptors and nuclear factor-kappaB, phagocytic cells. The resultant increase of Ca2+ activates the renin–angiotensin hormonal system raising blood pressure and further reduce the blood supply to renal cortical regions, aggravating renal tubular ischemia and fibrosis (Rayssiguier et al., 2006). Such reactions generate an internal milieu beneficial for growth and aggregation of nano-mineral apatite causing renal tubular blockade, nano-ruptures of tubules with inflammation, tubular cell fibrosis, and death. Collectively, these escalate renal ischemia and inflammation and reduce renal functions. CaPO4 crystals get attached to TLR4 on tubular cells and undergo endocytosis at their brush borders, triggering a vicious cycle of inflammation and fibrosis (Figure 5; Moe, 2021).
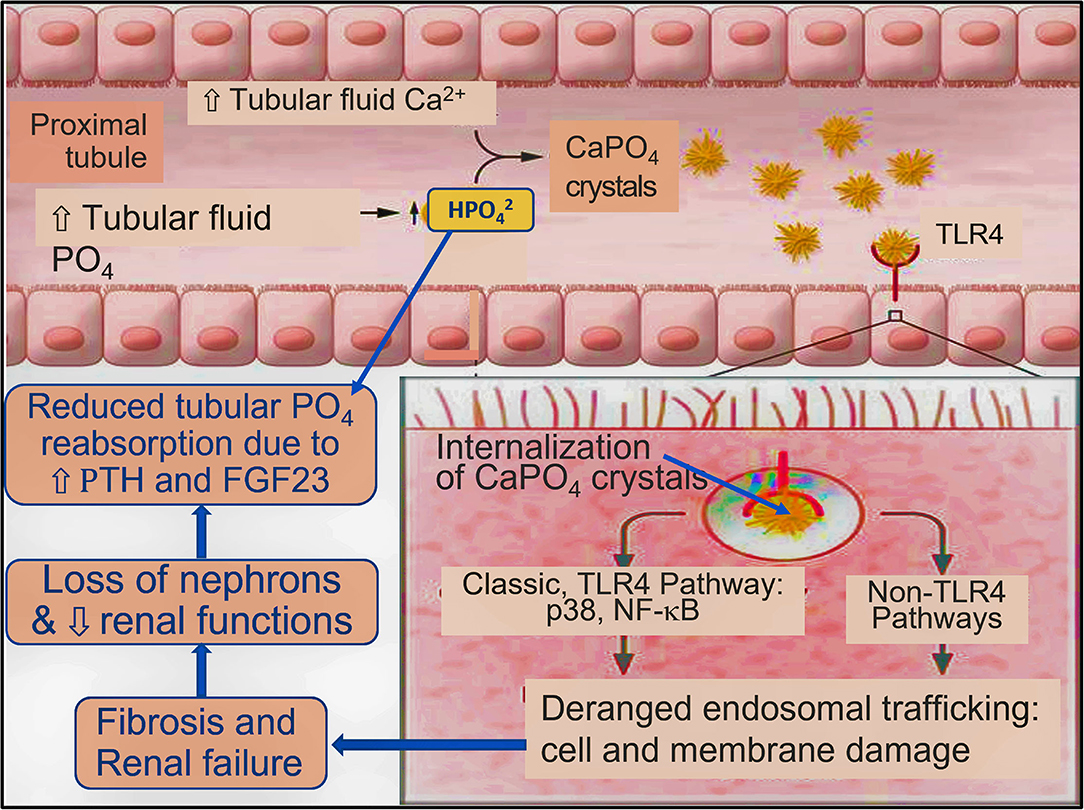
Figure 5. Postulated generic model for renal tubular crystalloid-nephropathy and progression of CKD [concept by Shiizaki et al. (2021) and modified from Moe (2021)]. Increased tubular fluid PO4 reacts with Ca2+ to form CaPO4 crystals and transforms to hydroxyapatite.
Those with CKD show raised parathyroid hormone levels (secondary or tertiary hyperparathyroidism) and FGF23, reduced PO4 reabsorption, and increased luminal PO4 concentration, which likely to worsen CKD (Moe, 2021). Once CaPO4 reaches a threshold in tubular or tissue fluids, crystallisation begins; crystals bind to cell-surface brush-border TLR4 on tubular cells, causing tubular damage (Moe, 2021). Crystals are internalised, initiating inflammation fibrosis in the tubule interstitium, and reducing renal functions, as shown in Figure 5.
Irrespective of the cause, the progression of CKD is associated with worsening vascular calcification, imbalances of mineral metabolism (CKD-MBD), inflammation, increased oxidative and metabolic stress, coagulation abnormalities, endothelial dysfunction, and accumulation of uremic toxins (Van Laecke, 2019). Hypomagnesemia worsens the mentioned pathologies and associated comorbidities through multiple mechanisms that worsen underlying nephropathy (Cunningham et al., 2012; Rodelo-Haad et al., 2020). These lead to poor clinical outcomes, including premature death, as in CKD-CTN (Quinones et al., 2019).
Contrary to some beliefs, dietary Mg2+ and slightly elevated serum Mg2+ are protective (Van Laecke, 2019). Besides, Mg2+ supplementation was reported to reduce or reverse vascular calcifications and CKD-associated deaths. This partly occurs through inhibition of the Wnt/β-catenin signalling pathway (Rodelo-Haad et al., 2020). Severe hypermagnesemia is rare and almost always observed in patients with end-stage renal failure who consume higher doses of magnesium supplements or magnesium-containing antacids (Van Laecke, 2019). Adverse effects of hypermagnesemia include nausea, vomiting, flushing, lethargy, and hypotension (Cunningham et al., 2012; Nishikawa et al., 2018; Rodelo-Haad et al., 2020).
Low Mg2+ and Klotho and High PO4 Further Aggravate CKDmfo
The average plasma inorganic PO4 concentration in adults is between 2.5 and 4.5 mg/dl: a level higher than 4.5 mg/dl is considered hyperphosphatemic. The average dietary phosphate intake is ~1,000 mg/day. At physiological pH of 7.4, PO4 exists as both H2 and H and acts as an extracellular fluid buffer (Peacock, 2021). Either PO4 overload or hypomagnesemia can aggravate renal failure. Mg2+ deficiency causes hypovitaminosis D, worsens kidney damage, induced by an increased tubular load of PO4, as in CKDmfo and other crystalloid nephropathies (Cunningham et al., 2012).
On the other hand, Mg2+ supplements can counteract or reverse the adverse effects from excess PO4 on renal tubular cells and reduce vascular calcification. Besides, in vitro data show that adequate Mg2+ alleviates proximal tubular cell injury from high PO4 (Sakaguchi et al., 2018). Therefore, in geographical regions with high PO4 content in drinking water, as in most CKDmfo-endemic regions, one should consider using magnesium supplements to counteract adverse effects of phosphate in the kidney.
Renal α-Klotho is a co-receptor for fibroblast growth factor-23 (FGF23) synthesised in bone. FGF23 is a phosphaturic hormone that regulates 1,25(OH)2D and involves ageing. A high tubular PO4 load in CKD reduces renal tubular expression of α-Klotho, a renal protective molecule. FGF23 increases renal PO4 excretion by reducing type IIa Na+/PO4 co-transporter expression in proximal tubular cells (Bergwitz and Juppner, 2010). In early CKD, the circulating FGF23 rises to counteract hyperphosphatemia. Increased FGF23 enhances urinary fractional excretion of PO4 (Sakan et al., 2014) but suppresses 1α-hydroxylase enzyme, thus reducing circulatory 1,25(OH)2D (calcitriol) concentration and stimulating PTH release (secondary hyperparathyrodisum) (Bergwitz and Juppner, 2010).
Additional Factors Contributing to the Genesis of CKDmfo
Current data suggest that the final common pathway of renal tubular cell damage and apoptosis occurs via oxidative stress (Figure 1). As a part of the intervention, it is necessary to modify in vivo conditions that favour the formation of nano-minerals. These include preventing dehydration, dietary modifications, alleviating chronic inflammation, and renal anaemia. In addition, other innovative approaches are also necessary to minimise renal tubular cell oxidative stress and mitochondrial dysfunction.
Dietetic Fructose in Renal Tubular CaPO4 Nanotube Formation
Rats fed with an Mg-deficient diet developed hypomagnesemia, reduced renal tissue Mg2+, and increased serum ionised Ca2+ concentrations. The condition worsens in rats fed with refined sugars, glucose, or fructose, than those with complex carbohydrates (Koh et al., 1989). In the fructose-fed group, renal Ca2+ content was eight times greater than in the control group.
In addition, there are negative synergistic interactions between low Mg2+ (high Ca2+) with excess fructokinase activity in kidneys (Andres-Hernando et al., 2017; Wrighton, 2017). The risk for mineral crystallisation is increased due to chronic dehydration and associated low urinary output (i.e., concentrated urine), which worsen the clinical condition (Garcia-Arroyo et al., 2016; Roncal-Jimenez et al., 2016). The presence of chronically high-concentrated urine due to dehydration, in conjunction with hypercalciuria, low urinary Mg2+-to-Ca2+ ratio, and renal interstitial or urine pH below 6.8 is highly conducive to precipitating CaPO4 crystals, even in the absence of hypercalcaemia (Koh et al., 1989). Low urinary Mg2+ and high-fructose diets further aid the mineral crystallisation process in kidneys.
Impairment of Mitochondrial Functions Causing Tubular Failure
Mitochondrial dysfunctions in renal tubular cells are partly due to ROS-mediated oxidative stress and chronic inflammation: these contribute to renal tubular cell dysfunction, atrophy, and fibrosis (Ye et al., 2015). Excess ROS generated via toxins, infections, metabolic derangements, micronutrient deficiency such as vitamin D and E, and dietary and in vivo synthesis of antioxidants (Wimalawansa, 2019d), reduce the generation of ATP (Bataille et al., 2011; Guan et al., 2015). The diminished availability of ATP will continue to impair renal tubular cell functions.
Multiple mechanisms, including mitochondrial chaperone prohibitin, protect tubular epithelial cells from oxidative stress-induced damage by directly inhibiting the oxidative process, preventing mitochondria dysfunction, and inhibiting cell apoptosis (Ye et al., 2015). Therefore, increasing mitochondria prohibitin in renal tubular cells could be used to alleviate nephrotoxic agent-induced tubular nephropathies.
In parallel, the synthetic antioxidants, N-acetyl-L-cysteine (NAC), a glutathione (GSH) precursor and a stimulator, could reduce intracellular oxidative stress by working through multiple mechanisms. The latter includes attenuating c-Jun NH2-terminal kinase and p38 mitogen-activated protein kinase phosphorylation, scavenging ROS, and activating extracellular regulated kinase 1/2 (ERK1/2), providing cytoprotection for renal epithelial cells (Zhang et al., 2011).
Probable Cause for CKDmfo
Dietary Factors Increasing the Risk of CKDmfo
The diets of individuals in CKDmfo-affected regions are high in carbohydrates, fewer fats, protein, and micronutrients. Besides, a considerable proportion of calories come from fructose, with enhanced fructokinase activity that negatively affects renal functions. High concentrations of Ca2+, PO4, and F− with low Mg2+ are conducive to precipitating CaPO4/hydroxyapatite and fluoroapatite nano-crystals in tubular fluid and renal cortical tissue (Aihara et al., 2003; Schepers et al., 2003; Khan, 2004, 2010; Czajka-Jakubowska et al., 2009; Khaskhali et al., 2009; Roncal Jimenez et al., 2014; Gan et al., 2016; Skwarek et al., 2017; Ter Braake et al., 2018; Wang, 2021). Table 3 illustrates the proposed causes in chronological order (earliest to present) assorted to date with related references.
Worldwide, about 30% of patients with end-stage renal disease have no specific underlying nephropathy diagnosis, and up to 15% of these cohorts carry genetic mutations such as HNF1β mutations (Tin et al., 2015). Examples of other rare developmental abnormalities associated with nephropathies are PAX2, EYA1, and GATA3 mutations: many of these conditions are associated with hypomagnesemia, which increases the the risk of developing all CKDs. However, the incidence is so rare, thus genetic screening is not warranted.
Scientists have proposed several theories to explain the mechanisms of bio-calcification (Miller et al., 2004; Yiu et al., 2015). When CaPO4 crystals form in renal tubules and interstitial tissues, they activate the Juxta-Glomerular apparatus, and other hormonal mechanisms that increase blood pressure and reduce the blood supply to kidneys. They reduce the Ca and PO4 fractional reabsorption, enhance fluid PO4, and modulate ionised calcium. This vicious cycle further increases the proximal tubular fluid CaPO4 saturation (Robertson, 2015), desirable for crystallisation (Linnes et al., 2013).
The formation of nano-crystallisation and hydroxyapatite nanoparticle scallops in the kidney is facilitated by higher inorganic Ca2+ and PO4 concentrations (Martel et al., 2014). In the presence of a high ambient F− concentration, hydroxyapatite changes into more stable fluorapatite (Wong et al., 2015) Ca5(PO4)3F + 9F− → Ca5F10 + 3().
Fluorapatite crystals are hard but inflammatogenic, attracting white blood and other immune cells. The reported nanoparticles are composed of polycrystalline CaPO4 crystals similar to bone mineral. The nano-crystals and nano-tubules are deposited within renal tubules and extracellular tissues surrounding renal tubules (Wong et al., 2015).
The absence of such data fail to provide reliable information to understand multifactorial complex interactions or generate clinically meaningful evidence to make firm conclusions (Wimalawansa, 2016b). At times, such data can be even misleading. Therefore, studying compounds in isolation in complex diseases like CKDmfo should be avoided. One example is ayurvedic medications, where effectiveness cannot be demonstrable by studying their isolated compounds. Similarly, based on relatively higher concentrations of Mg2+ and F− in drinking water (Wickramarathna et al., 2017) and a couple of laboratory rat studies (Wasana et al., 2017) that have not been reproduced, it is inappropriate to make the sweeping conclusion that Mg2+ and F− interactions cause CKDmfo (Wimalawansa and Dissanayake, 2019).
Novel Targets for Interventions—Effective Disease Modulators
There are highly cost-effective anti-inflammatory and anti-oxidant dietary supplements available that prevent generation of reactive oxygen species (ROS), as well as agents targeted to eliminate the vicious cycles of oxidative stress and chronic inflammation and to correct mitochondrial dysfunction. Most of these interventions are economical and widely available but not routinely used in treating persons with CKDmfo.
Renal tubular cells are metabolically highly active, and their transmembrane receptor-based transportation functions are energy dependent, based on the availability of ATP—energy. Meanwhile, the renal tubular reabsorption of ions such as Ca2+, , and Mg2+ occurs in nephrons through different ion channels and transporters, which are also energy-requiring processes (Blaine et al., 2015). Because of the high energy requirements of renal tubular cells, there are abundant mitochondria present in the renal tubular cells for continuously generating ATP/energy for the ongoing active solute filtration and absorption process (Ralto et al., 2019).
Tubular cell-directed nephrotoxins and ROS, reduce mitochondrial respiration. Consequently, it continues to deplete ATP (but not Na-K-ATPase-driven increased consumption) and increase the intracellular accumulation of lactic dehydrogenase and non-esterified fatty acids, dampening the mitochondrial respiratory functions. Tubular cell hypoxia, partly due to renal anaemia, tissue fibrosis, and intrarenal hypertension, further lessens the intracellular synthesis of ATP, and GSH induce tubular epithelial cell apoptosis. However, this process is antagonised by extracellular acidosis (around pH 6.7): compounds that chelate intracellular Ca2+ and improve oxygenation of tubular cells could reverse the pathology (Zager et al., 2008)—another potential target for intervention.
Limitations of This Study and Why Most Published Hypotheses Are Incorrect
The primary limitation of this study is the limited confirmatory renal ultrastructural data on the formation of nano-crystals and nano-tubes in CKDu, which is currently under investigation. Meanwhile, none of the other theories or hypotheses illustrated in Table 1 could explain the entire process of tubular dysfunction fibrosis leading to CKDmfo. There is no credible, published data that any agrochemical, heavy metals, arsenic, algae, or fungal toxins or ayurvedic medications cause CKDu/CKDmfo.
Based on the above scientific data and understanding of the biology and physiology of magnesium on renal functions, the idea that higher Mg2+ intake via drinking water (or food) (Wasana et al., 2016, 2017; Dharma-Wardana, 2018) causes CKDu is improbable. On the contrary, X-ray diffraction and energy-dispersive spectroscopy demonstrated that Mg2+ prevents CaPO4 crystal formation in media and vascular systems associated with CKD (Ter Braake et al., 2018).
Mg2+ reduces hydroxyapatite formation and significantly decreases Ca2+ and PO4 fractions in crystals, including extracellular space, thus reducing the risks for in vivo crystallisation (Ter Braake et al., 2018). In summation, all biological, physiological, in vitro, and in vivo evidence suggests that Mg2+ protects renal tissues from injuries, among others preventing crystal formation. CKDu/CKDmfo is a Geo-Bio-mediated, naturally induced chronic disease (Figure 4) that arises from multiple factors. It is 100% preventable with proactive measures, as described here and previously (Wimalawansa, 2019a,b, 2020; Wimalawansa and Dissanayake, 2019).
Discussion
CKDmfo is an environmentally induced preventable chronic disease (Wimalawansa, 2015c, 2016b). It is predominant in remotely located economically poor farming and related communities and associated with drinking groundwater, harmful behaviour, and other factors. This chronic tubulointerstitial disease is caused by consuming naturally contaminated groundwater, which aids the formation of CaPO4 crystals in the kidneys. In the favourable micro-environmental conditions in vivo, nano-mineral crystal formation occurs in vulnerable renal tissue. This process is likely to be modulated by additive or synergistic interactions between concentrated Ca2+, PO4, F−, and other ions, especially when tissue and intracellular Mg2+ concentrations are suboptimal. These conditions induce hydroxyapatite and fluoroapatite nano-crystal formation (Wimalawansa, 2015b; Wimalawansa and Dissanayake, 2019), creating a progressive, negative vicious cycle of tubular cell dysfunction, apoptosis, fibrosis, and CKD-CTN.
Irrespective of underlying reasons, changes in rain patterns and associated extended dry seasons, inferior quality groundwater, and lack of potable water negatively affect human and animal health. Because of the close association of the Geo-Bio pathway (Figure 4) leading to diseases, populations who live in intimate association with the immediate geo-environment are the most vulnerable.
While tropical countries are particularly affected by climate change, it is unknown whether this is related to CKD-CTN. In certain equatorial countries over the past four decades, the annual dry period has extended from 6 to 8 months, associated with an increase of ~1°C of average temperature. In addition, overexploitation of groundwater has adversely affected the available water supplies in the dry zonal regions, as in Sri Lanka and other CKD-CTN affected countires. The fatal disease was initiated following the migration of people into new territories (now affected with CKD-CTN), without proper environmental assessments and preparations, and acquired harmful behaviour over the past four decades. Consuming contaminated groundwater for many years derived from deep stagnant dug wells in higher elevations (i.e., away from tanks and streams) probably causes this disease (Wimalawansa, 2015b, 2016b; Wimalawansm, 2015).
As described, multiple approaches are necessary to overcome this silent, ongoing epidemic of CKD-CTN in affected countries (Wimalawansa, 2015b, 2016b). Since CKD-CTN is closely related to the environment where the affected people live for decades and consume natural groundwater, lifestyles, drinking water, and dietary habits must address systematically to overcome the adverse effects on the kidney and other vital organs. Providing financial assistance, renal protective medications (i.e., renal replacement therapy) (Varughese et al., 2020), and dialysis would never prevent the CKD-CTN. Governments of the affected countries must focus on disease prevention: the right approach and solutions based on the mentioned root cause to eradicate CKD-CTN (Wimalawansm, 2015).
The data described here demonstrate that (A) consumption of stagnant groundwater (hard water with higher concentrations of CaPO4 ± fluoride over many years, (B) chronic dehydration due to multiple reasons, and (C) the biological conditions and the local micro-environment in renal tissue being conducive to form nano-crystals could cause this deadly renal disease. Besides, the provision of reverse osmosis-purified clean water (Greenlee et al., 2009; Wimalawansa, 2013b, 2018) over 3 years significantly reduced (~50%) the incidence of CKDmfo. Putting data together facilitated the identification of mentioned factors that fulfilled the Bradford Hill criteria as the probable cause for the CKD-CTN (CKDmfo/CKDu). Providing potable water with low concentration of Ca2+, PO4, and F− and other contaminants and supplementing Mg2+ or adding it to RO, drinking water are the most cost-effective solutions to overcome this, otherwise deadly disease.
Conclusion
The novel concepts described here would leap forward in understanding the etiology of CKDmfo and taking affirmative action to prevent its occurrence and save lives. We have provided evidence that nano-crystallisation of CaPO4-hydroxyapatite and fluorapatite in renal tubular tissues is critical for developing CKD-CTN (CKDmfo/CKDu). In addition, we have provided overwhelming scientific evidence that higher amounts of Mg2+ help to prevent deterioration of CKD and CKD-CTN. Meanwhile, there is no credible evidence that excess Mg2+ contributes to or cause CKD-CTN.
Irrespective of the occupation, CKD-CTN affects those subjects when the internal environment in the kidney is conducive for CaPO4-hydroxyapatite crystal precipitation (Wimalawansa and Dissanayake, 2019). It is not a disease that exclusively affects those working in agriculture. People who have no connection with agriculture or agrochemicals also get the disease if the conditions are right. Consequently, agriculture- or agrochemical-based derogative terminology and acronyms should not be used to describe this disease (Ileperuma et al., 2018). The present findings enable respective administrations and charitable foundations to provide cost-effective modalities to prevent renal damage and reverse the early renal failure of this fatal disease.
In addition to a district- or country-wide broader chronic disease prevention program (Wimalawansa, 2015c), an area-wide cost-efficient strategy for early diagnosis and prevention of CKD-CTN is essential to rescue renal functions before irreversible harm occurs (Wimalawansa, 2015b, 2019c). Taking proper and proactive actions can remove the risks for more than forty million people and prevent an estimated 1.5 million premature deaths over the next few decades from CKD-CTN in affected countries. A proactive and cost-effective approach will save funds, opportunity costs, and lives in affected countries. The costs and opportunity costs that are associated with CKD-CTN can be further reduced by executing a broader chronic disease prevention program, in parallel with others. Such would help millions of families affected with CKD-CTN, in tropical countries.
Besides, the described concepts and findings will pave the path to develop highly effective, targeted solutions at a minimal cost to protect the health and lives of the working-age population, who regularly engage in physical work in a harsh environment. Spending 10% of the current healthcare expenditure on the above-mentioned disease prevention programs could lead to elimination of the CKD-CTN. Interventions need to encompass multiple cost-effective strategies, such as providing potable water, alleviating malnutrition, dietary habits, modifying harmful lifestyles, and educating the public and professionals on minimising chronic disease risks. These are critical for altering the current negative trend to save lives.
Author Contributions
SW wrote the paper and related research contributions, while CD provided intellectual input and editing and both authors approved it for publication.
Conflict of Interest
The authors declare that the research was conducted in the absence of any commercial or financial relationships that could be construed as a potential conflict of interest.
Publisher's Note
All claims expressed in this article are solely those of the authors and do not necessarily represent those of their affiliated organizations, or those of the publisher, the editors and the reviewers. Any product that may be evaluated in this article, or claim that may be made by its manufacturer, is not guaranteed or endorsed by the publisher.
Acknowledgments
The author appreciates the helpful input of Dr. Nimal Chandrasena.
References
(1997). National kidney foundation report on dialyzer reuse. Task force on reuse of dialyzers, council on dialysis, national kidney foundation. Am. J. Kidney Dis. 30, 859–871. doi: 10.1016/S0272-6386(97)90096-2
Abeywickrama, H. M., Wimalasiri, S., Koyama, Y., Uchiyama, M., Shimizu, U., Kakihara, N., et al. (2020). Quality of life and symptom burden among chronic kidney disease of uncertain etiology (ckdu) patients in girandurukotte, Sri Lanka. Int. J. Environ. Res. Public. Health. 17, 41. doi: 10.3390/ijerph17114041
Aihara, K., Byer, K. J., and Khan, S. R. (2003). Calcium phosphate-induced renal epithelial injury and stone formation: involvement of reactive oxygen species. Kidney Int. 64, 1283–1291. doi: 10.1046/j.1523-1755.2003.00226.x
Al, M. D. F., and Rodriguez, M. (2013). Magnesium - its role in Ckd. Nefrologia 33, 389–399. doi: 10.3265/Nefrologia.pre2013.Feb.11840
Ananda Jayalal, T. B., Mahawithanage, S. T. C., Senanayaka, S., and Dassanayaka, P. B. (2020). Evidence of selected nephrotoxic elements in Sri Lankan human autopsy bone samples of patients with ckdu and controls. Bmc Nephrol. 21, 384. doi: 10.1186/s12882-020-02049-4
Andres-Hernando, A., Li, N., Cicerchi, C., Inaba, S., Chen, W., Roncal-Jimenez, C., et al. (2017). Protective role of fructokinase blockade in the pathogenesis of acute kidney injury in mice. Nat. Commun. 8, 14181. doi: 10.1038/ncomms14181
Anupama, P. H., Prasad, N., Nzana, V. B., Tiwari, J. P., Mathew, M., and Abraham, G. (2020). Dietary management in slowing down the progression Of Ckdu. Indian J. Nephrol. 30, 256–260. doi: 10.4103/ijn.IJN_366_18
Aoba, T. (1997). The effect of fluoride on apatite structure and growth. Crit. Rev. Oral. Biol. Med. 8, 136–153. doi: 10.1177/10454411970080020301
Arnich, N., Lanhers, M. C., Laurensot, F., Podor, R., Montiel, A., and Burnel, D. (2003). In Vitro and in vivo studies of lead immobilization by synthetic hydroxyapatite. Environ. Pollut. 124, 139–149. doi: 10.1016/S0269-7491(02)00416-5
Azem, R., Daou, R., Bassil, E., Anvari, E. M., Taliercio, J. J., Arrigain, S., et al. (2020). Serum magnesium, mortality and disease progression in chronic kidney disease. Bmc Nephrol. 21, 49. doi: 10.1186/s12882-020-1713-3
Balasooriya, S., Munasinghe, H., Herath, A. T., Diyabalanage, S., Ileperuma, O. A., Manthrithilake, M., et al. (2020). Possible links between groundwater geochemistry and chronic kidney disease of unknown etiology (ckdu): an investigation from the ginnoruwa region In Sri Lanka. Expoure Health 12, 823–834 doi: 10.1007/s12403-019-00340-w
Balasubramanya, S., Stifel, D., Horbulyk, T., and Kafle, K. (2020). Chronic kidney disease and household behaviours in sri lanka: historical choices of drinking water and agrochemical use. Econ. Hum. Biol. 37, 100862. doi: 10.1016/j.ehb.2020.100862
Bandara, J. M., Wijewardena, H. V., Bandara, Y. M., Jayasooriya, R. G., and Rajapaksha, H. (2011). Pollution of river mahaweli and farmlands under irrigation by cadmium from agricultural inputs leading to a chronic renal failure epidemic among farmers in Ncp, Sri Lanka. Environ. Geochem. Health. 33, 439–453. doi: 10.1007/s10653-010-9344-4
Bandara, J. M., Wijewardena, H. V., Liyanege, J., Upul, M. A., and Bandara, J. M. (2010). Chronic renal failure in Sri Lanka caused by elevated dietary cadmium: trojan horse of the green revolution. Toxicol. Lett. 198, 33–39. doi: 10.1016/j.toxlet.2010.04.016
Bataille, A. M., Maffeo, C. L., and Renfro, J. L. (2011). Avian renal proximal tubule urate secretion is inhibited by cellular stress-induced amp-activated protein kinase. Am J Physiol Renal. Physiol. 300, F1327–F1338. doi: 10.1152/ajprenal.00680.2010
Bergwitz, C., and Juppner, H. (2010). Regulation of phosphate homeostasis by pth, vitamin D, And Fgf23. Annu. Rev. Med. 61, 91–104. doi: 10.1146/annurev.med.051308.111339
Bharti, V. K., Srivastava, R. S., Kumar, H., Bag, S., Majumdar, A. C., Singh, G., et al. (2014). Effects of melatonin and epiphyseal proteins on fluoride-induced adverse changes in antioxidant status of heart, liver, and kidney of rats. Adv. Pharmacol. Sci. (2014). 14, 532969. doi: 10.1155/2014/532969
Birkner, E., Grucka-Mamczar, E., Zwirska-Korczala, K., Zalejska-Fiolka, J., Stawiarska-Pieta, B., Kasperczyk, S., et al. (2006). Influence of sodium fluoride and caffeine on the kidney function and free-radical processes in that organ in adult rats. Biol. Trace. Elem. Res. 109, 35–48. doi: 10.1385/BTER:109:1:035
Blaine, J., Chonchol, M., and Levi, M. (2015). Renal control of calcium, phosphate, and magnesium homeostasis. Clinic. J. Am. Soc. Nephrol. 10, 1257–1272. doi: 10.2215/CJN.09750913
Bousquet-Santos, K., Costa, L., and Andrade, J. M. L. (2019). Nutritional status of individuals with chronic renal failure in hemodialysis in the unified health system. Cien. Saude. Colet. 24, 1189–1199. doi: 10.1590/1413-81232018243.11192017
Branca, J. J. V., Morucci, G., Maresca, M., Tenci, B., Cascella, R., Paternostro, F., et al. (2018). Selenium and zinc: two key players against cadmium-induced neuronal toxicity. Toxicol. In Vitro 48, 159–169. doi: 10.1016/j.tiv.2018.01.007
Buzea, C., Ivan, I., Pacheco Blandino, I. I. P., and Kevin Robbie, K. (2007). Nanomaterials and nanoparticles: sources and toxicity. Biointerphases, 2, Mr17–Mr172. doi: 10.1116/1.2815690
Chandrajith, R., Dissanayake, C. B., Ariyarathna, T., Herath, H. M., and Padmasiri, J. P. (2011). Dose-Dependent Na And Ca in fluoride-rich drinking water–another major cause of chronic renal failure in tropical arid regions. Sci. Total Environ. 409, 671–675. doi: 10.1016/j.scitotenv.2010.10.046
Chang, J. C., and Yang, H. Y. (2021). Epidemiology of chronic kidney disease of undetermined aetiology in Taiwanese farmers: a cross-sectional study from changhua community-based integrated screening programme. Occup. Environ. Med. 78, 849–858. doi: 10.1136/oemed-2021-107369
Chen, W., Krage, N., Wu, L., Pan, G., Khosrivafard, M., and Chang, A. C. (2008). Arsenic, cadmium, and lead in california cropland soils: role of phosphate and micronutrient fertilisers. J. Environ. Qual. 37, 689–695. doi: 10.2134/jeq2007.0444
Chmielewska, M., Symonowicz, K., Pula, B., Owczarek, T., Podhorska-Okolow, M., Ugorski, M., et al. (2015). Expression of metallothioneins i and ii in kidney of doxorubicin-treated rats. Exp. Toxicol. Pathol. 67, 297–303. doi: 10.1016/j.etp.2015.01.006
Cunningham, J., Rodriguez, M., and Messa, P. (2012). Magnesium in chronic kidney disease stages 3 and 4 and in dialysis patients. Clin. Kidney J. 5, I39–I51. doi: 10.1093/ndtplus/sfr166
Czajka-Jakubowska, A. E., Liu, J., Chang, S. R., and Clarkson, B. H. (2009). The effect of the surface characteristics of various substrates on fluorapatite crystal growth, alignment, and spatial orientation. Med. Sci. Monit. 15, Mt84-8. Available online at: https://pubmed.ncbi.nlm.nih.gov/19478709/
Desalegn, B., Nanayakkara, S., Harada, K. H., Hitomi, T., Chandrajith, R., Karunaratne, U., et al. (2011). Mycotoxin detection in urine samples from patients with chronic kidney disease of uncertain etiology in Sri Lanka. Bull. Environ. Contam. Toxicol. 87, 6–10. doi: 10.1007/s00128-011-0301-4
Despres, A. A., Perrot, N., Poulin, A., Tastet, L., Shen, M., Chen, H. Y., et al. (2019). Lipoprotein(A), Oxidized phospholipids, and aortic valve microcalcification assessed by 18f-sodium fluoride positron emission tomography and computed tomography. Cjc. Open. 1, 131–140. doi: 10.1016/j.cjco.2019.03.004
Dharmaratne, R. W. (2015). Fluoride in drinking water and diet: the causative factor of chronic kidney diseases in the North Central Province Of Sri Lanka. Environ. Health Prev. Med. 20, 237–242. doi: 10.1007/s12199-015-0464-4
Dharma-Wardana, M. W., Amarasiri, S. L., Dharmawardene, N., and Panabokke, C. R. (2015). Chronic kidney disease of unknown aetiology and ground-water ionicity: study based on Sri Lanka. Environ. Geochem. Health 37, 221–231. doi: 10.1007/s10653-014-9641-4
Dharma-Wardana, M. W. C. (2018). Chronic kidney disease of unknown etiology and the effect of multiple-ion interactions. Environ. Geochem. Health. 40, 705–719. doi: 10.1007/s10653-017-0017-4
Dissanayake, C., and Chandrajith, R. (2017). Groundwatr fluoride as a geochemical marker in the etiology of chronic kidney disease of unknown origin in Sri Lanka. Ceyl. J. Sci. 46, 312. doi: 10.4038/cjs.v46i2.7425
Dissanayake, C. B. (2016). Medical geology-a futuristic discipline (Editoril). Ceylon J. Sci. 45, 1–3. doi: 10.4038/cjs.v45i3.7395
Dissanayake, C. B., and Chandrajith, R. (2007). Medical geology in tropical countries with special reference to Sri Lanka. Environ Geochem Health 29, 155–162. doi: 10.1007/s10653-006-9070-0
Dissanayake, C. B. C. (2019). Fluoride and hardness in groundwater of tropical regions - review of recent evidence indicating tissue calcification and calcium phosphate nanoparticle formation in kidney tubules. Cey. J. Sci. 48, 197–207. doi: 10.4038/cjs.v48i3.7643
Diyabalanage, S., Navarathna, T., Abeysundara, H. T., Rajapakse, S., and Chandrajith, R. (2016). Trace Elements In Native And Improved Paddy Rice From Different Climatic Regions of Sri Lanka: implications for public health. Springerplus 5, 1864. doi: 10.1186/s40064-016-3547-9
Dunuweera, R., Shimomura, M., Priyankarage, J.v, Jayasingha, P., and Wimalawansa, S.j (2017). Chronic kidney disease of multifunctional origin (ckdmfo) prevailing in Sri Lanka: re-evaluated. World J. Pharma. Res. 6, 33–66.
Edirisinghe, E., Manthrithilake, H., Pitawala, H., Dharmagunawardhane, H. A., and Wijayawardane, R. L. (2018). Geochemical and isotopic evidences from groundwater and surface water for understanding of natural contamination in chronic kidney disease of unknown etiology (Ckdu) endemic zones in Sri Lanka. Isotopes Environ. Health Stud. 54, 244–261. doi: 10.1080/10256016.2017.1377704
Elango, L., and Kannan, R. (2007). Rock-water interaction and its control on chemical composition of groundwater. Dev. Environ. Sci. 5, 229–243. doi: 10.1016/S1474-8177(07)05011-5
Ericsson, Y., Luoma, H., and Ekberg, O. (1986). Effects of calcium, fluoride and magnesium supplementations on tissue mineralization in calcium- and magnesium-deficient rats. J. Nutr. 116, 1018–1027. doi: 10.1093/jn/116.6.1018
Faleel, R. A., and Jayawardena, U. A. (2020). Progression of potential etiologies of the chronic kidney disease of unknown etiology in Sri Lanka. J. Environ Sci Health C Toxicol Carcinog 38, 362–383. doi: 10.1080/26896583.2020.1852012
Fernando, W., Nanayakkara, N., Gunarathne, L., and Chandrajith, R. (2020). Serum And Urine fluoride levels in populations of high environmental fluoride exposure with endemic Ckdu: a case-control study from Sri Lanka. Environ. Geochem. Health 42, 1497–1504. doi: 10.1007/s10653-019-00444-x
Floege, J. (2015). Magnesium in Ckd: more than a calcification inhibitor? J. Nephrol. 28, 269–277. doi: 10.1007/s40620-014-0140-6
Fogo, A. B., Lusco, M. A., Najafian, B., and Alpers, C. E. (2017). Ajkd atlas of renal pathology: nephrocalcinosis and acute phosphate nephropathy. Am. J. Kidney Dis. 69, E17–E18. doi: 10.1053/j.ajkd.2017.01.005
Galan Carrillo, I., Vega, A., Goicoechea, M., Shabaka, A., Gatius, S., Abad, S., et al. (2021). Impact of serum magnesium levels on kidney and cardiovascular prognosis and mortality in ckd patients. J. Ren. Nutr. 31, 494–502. doi: 10.1053/j.jrn.2020.09.004
Galassi, A., and Cozzolino, M. (2014). Magnesium: a renewed player of vascular ageing in diabetic ckd patients? Clin. Kidney J. 7, 93–96. doi: 10.1093/ckj/sfu011
Gama-Axelsson, T., Heimburger, O., Stenvinkel, P., Barany, P., Lindholm, B., and Qureshi, A. R. (2012). Serum albumin as predictor of nutritional status in patients with Esrd. Clin. J. Am. Soc. Nephrol. 7, 1446–1453. doi: 10.2215/CJN.10251011
Gan, Q. Z., Sun, X. Y., Bhadja, P., Yao, X. Q., and Ouyang, J. M. (2016). Reinjury risk of nano-calcium oxalate monohydrate and calcium oxalate dihydrate crystals on injured renal epithelial cells: aggravation of crystal adhesion and aggregation. Int. J. Nanomed. 11, 2839–2854. doi: 10.2147/IJN.S104505
Gao, Q., Liu, Y. J., and Guan, Z. Z. (2008). Oxidative stress might be a mechanism connected with the decreased alpha 7 nicotinic receptor influenced by high-concentration of fluoride in sh-sy5y neuroblastoma cells. Toxicol. In Vitro 22, 837–843. doi: 10.1016/j.tiv.2007.12.017
Garcia-Arroyo, F. E., Cristobal, M., Arellano-Buendia, A. S., Osorio, H., Tapia, E., Soto, V., et al. (2016). Rehydration with soft drink-like beverages exacerbates dehydration and worsens dehydration-associated renal injury. Am. J. Physiol. Regul. Integr. Comp. Physiol. 311, R57–65. doi: 10.1152/ajpregu.00354.2015
Gautam, S., and Khapunj, A. (2021). Prevalence of hypomagnesemia among elderly patients attending a tertiary care center: a descriptive cross-sectional study. Jnma. J. Nepal. Med. Assoc. 59, 35–38. doi: 10.31729/jnma.5764
Gobalarajah, K., Subramaniam, P., Jayawardena, U. A., Rasiah, G., Rajendra, S., and Prabagar, J. (2020). Impact of water quality on chronic kidney disease of unknown etiology (Ckdu) in thunukkai division in Mullaitivu district, Sri Lanka. Bmc. Nephrol. 21, 507. doi: 10.1186/s12882-020-02157-1
Goyer, R. A. (1989). Mechanisms of lead and cadmium nephrotoxicity. Toxicol. Lett. 46, 153–162. doi: 10.1016/0378-4274(89)90124-0
Gragossian, A., Bashir, K., and Friede, R. (2021). Hypomagnesemia [Online]. Available online at: https://www.Ncbi.Nlm.Nih.Gov/Books/Nbk500003/#:~:text=Hypomagnesemia%20is%20an%20electrolyte%20disturbanceRenal%20losses%2c%20and%20other%20conditions (accessed December 12, 2021).
Greco, D. S. (2012). Endocrine causes of calcium disorders. Top Compan. Anim. Med. 27, 150–155. doi: 10.1053/j.tcam.2012.11.001
Greenlee, L. F., Lawler, D. F., Freeman, B. D., Marrot, B., and Moulin, P. (2009). Reverse osmosis desalination: water sources, technology, and today's challenges. Water Res. 43, 2317–2348. doi: 10.1016/j.watres.2009.03.010
Guan, S. S., Sheu, M. L., Wu, C. T., Chiang, C. K., and Liu, S. H. (2015). Atp synthase subunit-beta down-regulation aggravates diabetic nephropathy. Sci. Rep. 5, 14561. doi: 10.1038/srep14561
Gunatilake, S., Seneff, S., and Orlando, L. (2019). Glyphosate's synergistic toxicity in combination with other factors as a cause of chronic kidney disease of unknown origin. Int. J. Environ. Res. Public Health 16, 324. doi: 10.3390/ijerph16152734
Gunawickrama, S., Hewavitharana, K. I. G., Nanayakkara, P., and Gunawickrama, K. B. S. (2021). Chronic kidney disease of unknown etiology (Ckdu) In Sri Lanka: hematological changes and pro-inflammation suggest likely predictors of advance disease, as renal outcomes show prevalent normoalbuminuria. Diseases 10, 1002. doi: 10.3390/diseases10010002
Hma, S. H., Kawakami, T., Nagasawa, S., Serikawa, Y., Motoyama, A., Chaminda, G. G. T., et al. (2018). Arsenic, cadmium, lead, and chromium in well water, rice, and human urine in sri lanka in relation to chronic kidney disease of unknown etiology. J. Water Health 16, 212–222. doi: 10.2166/wh.2018.070
Ho, S. P., Chen, L., Allen, F. I., Hsi, R. S., Shimotake, A. R., Wiener, S. V., et al. (2018). Architecture-guided fluid flow directs renal biomineralization. Sci. Rep. 8, 14157. doi: 10.1038/s41598-018-30717-x
Hodgkins, K. S., and Schnaper, H. W. (2012). Tubulointerstitial injury and the progression of chronic kidney disease. Pediatric. Nephrol. 27, 901–909. doi: 10.1007/s00467-011-1992-9
Ileperuma, O., Weeraratne, S., and Wimalawansa, S. J. (2018). Acronyms, cinac, acn, kducal or nucal and so on are inappropriate to use for describing Ckdu. J. Epidemiol. Commun. Health 72, 967–968. doi: 10.1136/jech-2018-210959
Ileperuma, O. A., Dharmagunawardhane, H. A., and Herath, K. P. R. P. (2009). Dissolution of aluminium from sub-standard utensils under high fluoride stress: a possible risk factor for chronic renal failure in The North-Central Province. J.Natn.Sci.Foundation Sri Lanka 37, 219–222. doi: 10.4038/jnsfsr.v37i3.1217
Imbulana, S., and Oguma, K. (2021). Groundwater as a potential cause of chronic kidney disease of unknown etiology (Ckdu) In Sri Lanka: a review. J. Water Health 19, 393–410. doi: 10.2166/wh.2021.079
Jayasekara, J. M., Dissanayake, D. M., Adhikari, S. B., and Bandara, P. (2013). Geographical distribution of chronic kidney disease of unknown origin in north central region of Sri Lanka. Ceylon. Med. J. 58, 6–10. doi: 10.4038/cmj.v58i1.5356
Jayasekera, J., Dissnayake, D.m, Ratnayake, P., Wikramasinghe, W., and Radella, Y.a, Palugaswewa, W. B. (2012). The Effects from concenrated water on reservoirs of high prevelance areas on ckd of unknonw origin in Sri Lanka On Mice (Abst.) [Online]. Colombo, Sri Lanka: Sri Lanka medical association annual scientific sessions.
Jayasumana, C., Gunatilake, S., and Senanayake, P. (2014). Glyphosate, hard water and nephrotoxic metals: are they the culprits behind the epidemic of chronic kidney disease of unknown etiology in sri lanka? Int. J. Environ. Res. Public Health 11, 2125–2147. doi: 10.3390/ijerph110202125
Jia, H., Qian, H., Qu, W., Zheng, L., Feng, W., and Ren, W. (2019). Fluoride occurrence and human health risk in drinking water wells from southern edge of chinese loess plateau. Int. J. Environ. Res. Public Health 16, 1683. doi: 10.3390/ijerph16101683
Joshi, A., Tallman, J. E., Calvert, J. K., Brewer, T., Miller, N. L., Yang, L., et al. (2021). Complementary and alternative medicine use in first-time and recurrent kidney stone sormers. Urology 156, 58–64. doi: 10.1016/j.urology.2021.05.084
Kalantar-Zadeh, K., Stenvinkel, P., Pillon, L., and Kopple, J. D. (2003). Inflammation and nutrition in renal insufficiency. Adv. Ren. Replace The.r 10, 155–169. doi: 10.1053/j.arrt.2003.08.008
Khalili, J., and Biloklytska, H. F. (2009). Influence of supplementary vitamins and minerals on lipid peroxidation and redox state in heart, kidney and liver of rats exposed to fluoride. Fiziol Zh 55, 75–80. Available online at: https://www.ncbi.nlm.nih.gov/pubmed/20201392
Khan, S. R. (2004). Crystal-induced inflammation of the kidneys: results from human studies, animal models, and tissue-culture studies. Clin. Exp. Nephrol. 8, 75–88. doi: 10.1007/s10157-004-0292-0
Khan, S. R. (2010). Nephrocalcinosis in animal models with and without stones. Urol. Res. 38, 429–438. doi: 10.1007/s00240-010-0303-4
Khaskhali, M. H., Byer, K. J., and Khan, S. R. (2009). The effect of calcium on calcium oxalate monohydrate crystal-induced renal epithelial injury. Urol. Res. 37, 1–6. doi: 10.1007/s00240-008-0160-6
Koh, E. T., Reiser, S., and Fields, M. (1989). Dietary fructose as compared to glucose and starch increases the calcium content of kidney of magnesium-deficient rats. J. Nutr. 119, 1173–1178. doi: 10.1093/jn/119.8.1173
Kula, A. J., and Bansal, N. (2021). Magnesium and cardiovascular disease in ckd: the mysteries of a humble divalent cation. Kidney Med. 3, 162–164. doi: 10.1016/j.xkme.2021.02.002
Kulathunga, M., Wijayawardena, M. A. A., Naidu, R., Wimalawansa, S. J., and Wijeratne, A. W. (2020). Association between body mass index and estimated glomerular filtration rate in patients with chronic kidney disease of unknown aetiology in Sri Lanka. Environ. Geochem. Health 472, 7. doi: 10.1007/s10653-019-00472-7
Levine, K. E., Redmon, J. H., Elledge, M. F., Wanigasuriya, K. P., Smith, K., Munoz, B., et al. (2016). Quest to identify geochemical risk factors associated with chronic kidney disease of unknown etiology (ckdu) in an endemic region of Sri Lanka-a multimedia laboratory analysis of biological, food, and environmental samples. Environ. Monit. Assess 188, 548. doi: 10.1007/s10661-016-5524-8
Linnes, M. P., Krambeck, A. E., Cornell, L., Williams, J. C. Jr., Korinek, M., Bergstralh, E. J., et al. (2013). Phenotypic characterization of kidney stone formers by endoscopic and histological quantification of intrarenal calcification. Kidney Int. 84, 818–825. doi: 10.1038/ki.2013.189
Liu, B. C., Tang, T. T., Lv, L. L., and Lan, H. Y. (2018). Renal tubule injury: a driving force towards chronic kidney disease. Kidney Int. 93, 568–579. doi: 10.1016/j.kint.2017.09.033
Liyanage, D. N. D., Diyabalanage, S., Dunuweera, S. P., Rajapakse, S., Rajapakse, R. M. G., and Chandrajith, R. (2022). Significance of mg-hardness and fluoride in drinking water on chronic kidney disease of unknown etiology in monaragala, Sri Lanka. Environ. Res. 203, 111779. doi: 10.1016/j.envres.2021.111779
Martel, J., Peng, H. H., Young, D., Wu, C. Y., and Young, J. D. (2014). Of nanobacteria, nanoparticles, biofilms and their role in health and disease: facts, fancy and future. Nanomedicine. 9, 483–499. doi: 10.2217/nnm.13.221
Massy, Z. A., and Drueke, T. B. (2015). Magnesium and cardiovascular complications of chronic kidney disease. Nat. Rev. Nephrol. 11, 432–442. doi: 10.1038/nrneph.2015.74
Matias, M. L., Romao-Veiga, M., Ribeiro, V. R., Nunes, P. R., Gomes, V. J., Devides, A. C., et al. (2021). Progesterone and vitamin d downregulate the activation of the nlrp1/nlrp3 inflammasomes and Tlr4-Myd88-Nf-kappab pathway in monocytes from pregnant women with preeclampsia. J. Reprod. Immunol. 144, 103286. doi: 10.1016/j.jri.2021.103286
Mazzaferro, S., De Martini, N., Cannata-Andia, J., Cozzolino, M., Messa, P., Rotondi, S., et al. (2021). Focus on the possible role of dietary sodium, potassium, phosphate, magnesium, and calcium on ckd progression. J. Clin. Med. 10, 958. doi: 10.3390/jcm10050958
Mcdonough, L. K., Meredith, K. T., Nikagolla, C., Middleton, R. J., Tan, J. K., Ranasinghe, A. V., et al. (2020). The water chemistry and microbiome of household wells in medawachchiya, Sri Lanka, an area with high prevalence of chronic kidney disease of unknown origin (Ckdu). Sci. Rep. 10, 18295. doi: 10.1038/s41598-020-75336-7
Mikac, I., Fiket, Z., Terzic, S., Baresic, J., Mikac, N., and Ahel, M. (2011). Chemical indicators of anthropogenic impacts in sediments of the pristine karst lakes. Chemosphere 84, 1140–1149. doi: 10.1016/j.chemosphere.2011.04.027
Miller, V. M., Rodgers, G., Charlesworth, J. A., Kirkland, B., Severson, S. R., Rasmussen, T. E., et al. (2004). Evidence of nanobacterial-like structures in calcified human arteries and cardiac valves. Am. J. Physiol. Heart Circ. Physiol. 287, H1115–H1124. doi: 10.1152/ajpheart.00075.2004
Moe, O. W. (2021). A generic crystallopathic model for chronic kidney disease progression. J. Clin. Invest. 131, 1858. doi: 10.1172/JCI151858
Mondal, D., Dutta, G., and Gupta, S. (2016). Inferring the fluoride hydrogeochemistry and effect of consuming fluoride-contaminated drinking water on human health in some endemic areas of Birbhum District, West Bengal. Environ. Geochem. Health 38, 557–576. doi: 10.1007/s10653-015-9743-7
Mulay, S. R., and Anders, H. J. (2017). Crystal nephropathies: mechanisms of crystal-induced kidney injury. Nat. Rev. Nephrol. 13, 226–240. doi: 10.1038/nrneph.2017.10
Murray, K. O., Fischer, R. S., Chavarria, D., Duttmann, C., Garcia, M. N., Gorchakov, R., et al. (2015). Mesoamerican nephropathy: a neglected tropical disease with an infectious etiology? Microbes Infect. 17, 671–675. doi: 10.1016/j.micinf.2015.08.005
Muthusinghe, D. S., Shimizu, K., Lokupathirage, S. M. W., Wei, Z., Sarathkumara, Y. D., Fonseka, G. R. A., et al. (2021). Identification of novel rodent-borne orthohantaviruses in an endemic area of chronic kidney disease of unknown etiology (Ckdu) In Sri Lanka. Viruses 13, 1984. doi: 10.3390/v13101984
Nanayakkara, S., Senevirathna, S., Harada, K. H., Chandrajith, R., Nanayakkara, N., and Koizumi, A. (2020). The influence of fluoride on chronic kidney disease of uncertain aetiology (Ckdu) In Sri Lanka. Chemosphere 257, 127186. doi: 10.1016/j.chemosphere.2020.127186
Nanayakkara, S., Senevirathna, S. T., Abeysekera, T., Chandrajith, R., Ratnatunga, N., Gunarathne, E. D., et al. (2014). an integrative study of the genetic, social and environmental determinants of chronic kidney disease characterised by tubulointerstitial damages in the north central region Of Sri Lanka. J. Occup. Health 56, 28–38. doi: 10.1539/joh.13-0172-OA
Nishikawa, M., Shimada, N., Kanzaki, M., Ikegami, T., Fukuoka, T., Fukushima, M., et al. (2018). The characteristics of patients with hypermagnesemia who underwent emergency hemodialysis. Acute Med. Surg. 5, 222–229. doi: 10.1002/ams2.334
Nunes, P. R., Romao-Veiga, M., Matias, M. L., Ribeiro, V. R., De Oliveira, L., Peracoli, J. C., et al. (2022). Vitamin D decreases expression Of Nlrp1 And Nlrp3 inflammasomes in placental explants from women with preeclampsia cultured with hydrogen peroxide. Hum. Immunol. 83, 74–80. doi: 10.1016/j.humimm.2021.10.002
Panabokke, C. R. (2007). Groundwater Conditions In Sri Lanka, Colombo. Sri Lanka: National Science Foundation Of Sri Lanka.
Parameswaran, S., Rinu, P. K., Kar, S. S., Harichandrakumar, K. T., James, T. D., Priyamvada, P. S. P., et al. (2020). A Newly Recognized Endemic Region Of Ckd Of Undetermined Etiology (Ckdu) In South India-"Tondaimandalam Nephropathy. Kidney Int. Rep. 5, 2066–2073. doi: 10.1016/j.ekir.2020.08.032
Pathmarajah, S. (2002). Use Of Groundwater For Agriculture In Sri Lanka. Aesl/Pgia: Pgia, Sri Lanka.
Peacock, M. (2021). Phosphate metabolism in health and disease. Calcif Tissue Int 108, 3–15. doi: 10.1007/s00223-020-00686-3
Peiris-John, R. J., Wanigasuriya, J. K., Wickremasinghe, A. R., Dissanayake, W. P., and Hittarage, A. (2006). Exposure to acetylcholinesterase-inhibiting pesticides and chronic renal failure. Ceylon Med. J. 51, 42–43. doi: 10.4038/cmj.v51i1.1382
Pendon-Ruiz De Mier, M. V., Rodelo-Haad, C., Diaz-Tocados, J. M., Munoz-Castaneda, J. R., and Rodriguez, M. (2020). Magnesium: an old player revisited in the context of Ckd-Mbd. Clin Chim Acta 501, 53–59. doi: 10.1016/j.cca.2019.11.037
Perazella, M. A., and Herlitz, L. C. (2021). The crystalline nephropathies. Kidney Int. Rep. 6, 2942–2957. doi: 10.1016/j.ekir.2021.09.003
Petropoulos, Z. E., Ramirez-Rubio, O., Scammell, M. K., Laws, R. L., Lopez-Pilarte, D., Amador, J. J., et al. (2021). Climate trends at a hotspot of chronic kidney disease of unknown causes in Nicaragua, 1973-2014. Int. J. Environ. Res. Public Health 18, 5418. doi: 10.3390/ijerph18105418
Prasanthi, R. P., Devi, C. B., Basha, D. C., Reddy, N. S., and Reddy, G. R. (2010). Calcium and zinc supplementation protects lead (Pb)-induced perturbations in antioxidant enzymes and lipid peroxidation in developing mouse brain. Int. J. Dev. Neurosci. 28, 161–167. doi: 10.1016/j.ijdevneu.2009.12.002
Quinones, H., Hamdi, T., Sakhaee, K., Pasch, A., Moe, O. W., and Pak, C. Y. C. (2019). Control of metabolic predisposition to cardiovascular complications of chronic kidney disease by effervescent calcium magnesium citrate: a feasibility study. J. Nephrol. 32, 93–100. doi: 10.1007/s40620-018-0559-2
Rajapaksha, H., Pandithavidana, D. R., and Dahanayake, J. N. (2021). Demystifying chronic kidney disease of unknown etiology (Ckdu): computational interaction analysis of pesticides and metabolites with vital renal enzymes. Biomolecules 11. doi: 10.3390/biom11020261
Ralto, K. M., Rhee, E. P., and Parikh, S. M. (2019). Nad(+) homeostasis in renal health and disease. Nat. Rev. Nephrol. 6, 216. doi: 10.1038/s41581-019-0216-6
Rango, T., Jeuland, M., Manthrithilake, H., and Mccornick, P. (2015). Nephrotoxic contaminants in drinking water and urine, and chronic kidney disease in rural Sri Lanka. Sci. Total Environ. 518, 574–585. doi: 10.1016/j.scitotenv.2015.02.097
Rayssiguier, Y., Gueux, E., Nowacki, W., Rock, E., and Mazur, A. (2006). High fructose consumption combined with low dietary magnesium intake may increase the incidence of the metabolic syndrome by inducing inflammation. Magnes. Res. 19, 237–243.
Redmon, J. H., Levine, K. E., Lebov, J., Harrington, J., and Kondash, A. J. (2021). A Comparative review: chronic kidney disease of unknown etiology (ckdu) research conducted in Latin America Versus Asia. Environ. Res. 192, 110270. doi: 10.1016/j.envres.2020.110270
Robertson, W. G. (2015). Potential role of fluctuations in the composition of renal tubular fluid through the nephron in the initiation of randall's plugs and calcium oxalate crystalluria in a computer model of renal function. Urolithiasis 43, 93–107. doi: 10.1007/s00240-014-0737-1
Robinson, C., Connell, S., Kirkham, J., Brookes, S. J., Shore, R. C., and Smith, A. M. (2004). The effect of fluoride on the developing tooth. Caries Res. 38, 268–276. doi: 10.1159/000077766
Rodelo-Haad, C., Pendon-Ruiz De Mier, M. V., Diaz-Tocados, J. M., Martin-Malo, A., Santamaria, R., Munoz-Castaneda, J. R., et al. (2020). The role of disturbed mg homeostasis in chronic kidney disease comorbidities. Front. Cell Dev. Biol. 8, 543099. doi: 10.3389/fcell.2020.543099
Rodrigo, C., Nawarathne, P., and Jayasinghe, S. (2021). Chronic interstitial nephritis in agricultural communities (Cinac) and lysosomal tubulopathy: is there a place for anti-oxidants? Med. Hypotheses 146, 110414. doi: 10.1016/j.mehy.2020.110414
Roncal Jimenez, C. A., Ishimoto, T., Lanaspa, M. A., Rivard, C. J., Nakagawa, T., Ejaz, A. A., et al. (2014). Fructokinase activity mediates dehydration-induced renal injury. Kidney Int. 86, 294–302. doi: 10.1038/ki.2013.492
Roncal-Jimenez, C. A., Ishimoto, T., Lanaspa, M. A., Milagres, T., Hernando, A. A., Jensen, T., et al. (2016). Aging-associated renal disease in mice is fructokinase dependent. Am. J. Physiol. Renal Physiol. 311, F722–F730. doi: 10.1152/ajprenal.00306.2016
Sakaguchi, Y., Hamano, T., and Isaka, Y. (2018). Magnesium and progression of chronic kidney disease: benefits beyond cardiovascular protection? Adv. Chronic Kidney Dis. 25, 274–280. doi: 10.1053/j.ackd.2017.11.001
Sakan, H., Nakatani, K., Asai, O., Imura, A., Tanaka, T., Yoshimoto, S., et al. (2014). Reduced renal alpha-klotho expression in ckd patients and its effect on renal phosphate handling and vitamin D metabolism. Plos One 9, E86301. doi: 10.1371/journal.pone.0086301
Schepers, M. S., Duim, R. A., Asselman, M., Romijn, J. C., Schroder, F. H., and Verkoelen, C. F. (2003). Internalization Of Calcium Oxalate Crystals By Renal Tubular Cells: A Nephron segment-specific process? Kidney Int. 64, 493–500. doi: 10.1046/j.1523-1755.2003.00107.x
Schroder, K., and Tschopp, J. (2010). The inflammasomes. Cell 140, 821–832. doi: 10.1016/j.cell.2010.01.040
Sethmann, I., Wendt-Nordahl, G., Knoll, T., Enzmann, F., Simon, L., and Kleebe, H. J. (2017). Microstructures of randall's plaques and their interfaces with calcium oxalate monohydrate kidney stones reflect underlying mineral precipitation mechanisms. Urolithiasis 45, 235–248. doi: 10.1007/s00240-016-0925-2
Shiizaki, K., Tsubouchi, A., Miura, Y., Seo, K., Kuchimaru, T., Hayashi, H., et al. (2021). Calcium phosphate microcrystals in the renal tubular fluid accelerate chronic kidney disease progression. J. Clin. Invest. 131, 693. doi: 10.1172/JCI145693
Skwarek, E., Bolbukh, Y., and Janush, W. (2017). Hydroxyapatite composites with multiwalled carbon nanotubes. Adsorpt. Sci. Technol. 35, 534–544. doi: 10.1177/0263617417700637
Stalin, P., Purty, A. J., and Abraham, G. (2020). Distribution and determinants of chronic kidney disease of unknown etiology: a brief overview. Indian J. Nephrol. 30, 241–244. doi: 10.4103/ijn.IJN_313_18
Sunil-Chandra, N. P., Jayaweera, J., Kumbukgolla, W., and Jayasundara, M. (2020). Association of hantavirus infections and leptospirosis with the occurrence of chronic kidney disease of uncertain etiology in the North Central Province of Sri Lanka: a prospective study with patients and healthy persons. Front. Cell Infect. Microbiol. 10, 556737. doi: 10.3389/fcimb.2020.556737
Ter Braake, A. D., Shanahan, C. M., and De Baaij, J. H. F. (2017). Magnesium counteracts vascular calcification: passive interference or active modulation? Arterioscler. Thromb. Vasc. Biol. 37, 1431–1445. doi: 10.1161/ATVBAHA.117.309182
Ter Braake, A. D., Tinnemans, P. T., Shanahan, C. M., Hoenderop, J. G. J., and De Baaij, J. H. F. (2018). Magnesium prevents vascular calcification in vitro by inhibition of hydroxyapatite crystal formation. Scientific Rep. 8, 2069. doi: 10.1038/s41598-018-20241-3
Thammitiyagodage, M. G., Gunatillaka, M. M., Ekanayaka, N., Rathnayake, C., Horadagoda, N. U., Jayathissa, R., et al. (2017). Ingestion of dug well water from an area with high prevalence of chronic kidney disease of unknown etiology (Ckdu) and development of kidney and liver lesions in rats. Ceylon Med. J. 62, 20–24. doi: 10.4038/cmj.v62i1.8428
Tin, A., Grams, M. E., Maruthur, N. M., Astor, B. C., Couper, D., Mosley, T. H., et al. (2015). Results from the atherosclerosis risk in communities study suggest that low serum magnesium is associated with incident kidney disease. Kidney Int. 87, 820–827. doi: 10.1038/ki.2014.331
Tiselius, H. G. (2013). the role of calcium phosphate in the development of randall's plaques. Urolithiasis 41, 369–377. doi: 10.1007/s00240-013-0602-7
Torreggiani, M., Fois, A., D'alessandro, C., Colucci, M., Orozco Guillen, A. O., Cupisti, A., et al. (2020). Of mice and men: the effect of maternal protein restriction on offspring's kidney health. are studies on rodents applicable to chronic kidney disease patients? a narrative review. Nutrients 12, 614. doi: 10.3390/nu12061614
Unicef/WHO (2008). Progress On Drinking Water And Sanitation: Special Focus On Sanitation. Unicef/Who.
Van Laecke, S. (2019). Hypomagnesemia and hypermagnesemia. Acta. Clin. Belg. 74, 41–47. doi: 10.1080/17843286.2018.1516173
Varughese, S., Agarwal, S. K., Raju, T. R., and Khanna, T. (2020). Options of renal replacement therapy in Ckdu. Indian J. Nephrol. 30, 261–263. doi: 10.4103/ijn.IJN_396_19
Vervloet, M. (2019). Modifying phosphate toxicity in chronic kidney disease. Toxins (Basel) 11, 5022. doi: 10.3390/toxins11090522
Wang, A. Y., Kalantar-Zadeh, K., Fouque, D., Wee, P. T., Kovesdy, C. P., Price, S. R., et al. (2018). Precision medicine for nutritional management in end-stage kidney disease and transition to dialysis. Semin. Nephrol. 38, 383–396. doi: 10.1016/j.semnephrol.2018.05.008
Wang, M. (2021). Calcium phosphate crystals: targets for renoprotection. Nat. Rev. Nephrol. 17, 572. doi: 10.1038/s41581-021-00472-w
Wanigasuriya, K. (2012). Aetiological factors of chronic kidney disease in the north central province of sri lanka: a review of evidence to-date. J. Coll. Commun. Physic. Sri Lanka 17, 15–20. doi: 10.4038/jccpsl.v17i1.4931
Wanigasuriya, K. P., Peiris, H., Ileperuma, N., Peiris-John, R. J., and Wickremasinghe, R. (2008). Could ochratoxin a in food commodities be the cause of chronic kidney disease in Sri Lanka? Trans. R. Soc. Trop. Med. Hyg. 102, 726–728. doi: 10.1016/j.trstmh.2008.04.007
Wanigasuriya, K. P., Peiris-John, R. J., and Wickremasinghe, R. (2011). Chronic kidney disease of unknown aetiology in sri lanka: is cadmium a likely cause? Bmc. Nephrol. 12, 32. doi: 10.1186/1471-2369-12-32
Wanigasuriya, K. P., Peiris-John, R. J., Wickremasinghe, R., and Hittarage, A. (2007). Chronic renal failure in north central Province of Sri Lanka: an environmentally induced disease. Trans. R. Soc. Trop. Med. Hyg. 101, 1013–1017. doi: 10.1016/j.trstmh.2007.05.006
Wasana, H. M., Aluthpatabendi, D., Kularatne, W. M., Wijekoon, P., Weerasooriya, R., and Bandara, J. (2016). Drinking water quality and chronic kidney disease of unknown etiology (Ckdu): synergic effects of fluoride, cadmium and hardness of water. Environ. Geochem. Health 38, 157–168. doi: 10.1007/s10653-015-9699-7
Wasana, H. M., Perera, G. D., Gunawardena, P. S., Fernando, P. S., and Bandara, J. (2017). Who Water Quality standards vs synergic effect(S) of fluoride, heavy metals and hardness in drinking water on kidney tissues. Sci. Rep. 7, 42516. doi: 10.1038/srep42516
Wassenaar, E., O'melia, A. M., and Mehler, P. S. (2018). A causality dilemma: arfid, malnutrition, psychosis, and hypomagnesemia. Int. J. Eat. Disord. 51, 1113–1116. doi: 10.1002/eat.22939
Weaver, V. M., Fadrowski, J. J., and Jaar, B. G. (2015). Global dimensions of chronic kidney disease of unknown etiology (Ckdu): a modern era environmental and/or occupational nephropathy? Bmc. Nephrol. 16, 145. doi: 10.1186/s12882-015-0105-6
Weeraratne, S., and Wimalawansa, S. J. (2015). A major irrigation project (accelerated mahaweli programme) and the chronic kidney disease of multifactorial origin in Sri Lanka. Int. J. Environ. Agricult. Res. 1, 16–27.
Who-CKDu-Final Report: Jayathilaka, N., Mendis, S., Mehta, F. R., Dissanayake, L. J., and Janakan, N. (2013). Who Sri Lanka Ckdu Report: Chronic Kidney Disease Of Unknown Aetiology (Ckdu): A New Threat To Health. Available online at: [http://dh-web.org/place.names/posts/index.html#ckdu]; http://www.lankaweb.com/news/items12/WHO%20final%20report%20on%20CKDu%20SL.pdf; http://dh-web.org/place.names/posts/index.html#ckdu
Wickramarathna, S., Balasooriya, S., Diyabalanage, S., and Chandrajith, R. (2017). Tracing Environmental aetiological factors of chronic kidney diseases in the dry zone of Sri Lanka-a hydrogeochemical and isotope approach. J. Trace Elem. Med. Biol. 44, 298–306. doi: 10.1016/j.jtemb.2017.08.013
Wimalawansa, S., and Wimalawansa, S. J. (2015a). Protection of watersheds, and control and responsible use of fertiliser to prevent phosphate eutrophication ofreservoirs. Int. J. Res. Environ. Sci. 1, 1–18.
Wimalawansa, S. A., and Wimalawansa, S. J. (2014a). Agrochemical-related environmental pollution: effects on human health. Glob. J. Biol. Agricult. Health Sci. 3, 72–83.
Wimalawansa, S. A., and Wimalawansa, S. J. (2014b). Impact of changing agricultural practices on human health: chronic kidney disease of multi-factorial origin In Sri Lanka. Wudpecker J. Agricult. Res. 3, 110–124.
Wimalawansa, S. A., and Wimalawansa, S. J. (2016). Environmentally induced, occupational diseases with emphasis on chronic kidney disease of multifactorial origin affecting tropical countries. Ann. Occup. Environ. Med. 28, 33. doi: 10.1186/s40557-016-0119-y
Wimalawansa, S. J. (2013a). Environmental Pollution-Related Chronic Kidney Disease Of Multi-Factoril Origin. Annual Olcott Oration-2013. Available online at: https://wimalawansa.org/sites/all/files/Olcott_Oration_2013_b.pdf; Colombo, Sri Lanka [Online].
Wimalawansa, S. J. (2013b). Purification of contaminated water with reverse osmosis: effective solution of providing clean water for human needs in developing countries. J. Emerg. Technol. Adv. Eng. 3, 75–89.
Wimalawansa, S. J. (2014a). Escalating chronic kidney diseases in sri lanka: causes, solutions and recommendations. Environ. Health Preventi. Med. 19, 375–394. doi: 10.1007/s12199-014-0395-5
Wimalawansa, S. J. (2014b). “Prevention of Ckd of multi-factorial origin (Ckd-mfo): issues, gravity, and the importance of early diagnosis,” in 5th International Conference On Sustainable Built Environment; “Environment Pollution Of Prevention Of Ckd-Mfo In Sri Lanka, Kandy: Icsbe, p. 22–26.
Wimalawansa, S. J. (2015a). Agrochemicals and chronic kidney disease of multifactorial origin: environmentally induced occupational exposure disease. Int. J. Nephrol. Kidney Fail. 1, 1–9. doi: 10.16966/2380-5498.111
Wimalawansa, S. J. (2015b). Escalating chronic kidney diseases of multi-factorial origin (ckd-mfo) in Sri Lanka: causes, solutions, and recommendations-update and responses. Environ. Health. Prev. Med. 20, 152–157. doi: 10.1007/s12199-015-0447-5
Wimalawansa, S. J. (2015c). Strategic framework for managing non communicable diseases: preventing chronic kidney disease of multifactorial origin (Ckdmfo/Ckdu) as an example. Chronic. Dis. Int. 2, 1–9.
Wimalawansa, S. J. (2016a). Effect of water hardness on non-communicable diseases including chronic kidney disease of multifactorial origin (Ckdmfo/Ckduo). J. Environ. Health Sci. Eng. 2, 1–11. doi: 10.15436/2378-6841.16.029
Wimalawansa, S. J. (2016b). The role of ions, heavy metals, fluoride, and agrochemicals: critical evaluation of potential aetiological factors of chronic kidney disease of multifactorial origin (Ckdmfo/Ckdu) and recommendations for its eradication. Environ. Geochem. Health 38, 639–678. doi: 10.1007/s10653-015-9768-y
Wimalawansa, S. J. (2018). A cost-effective, interim solution to overcome water-pollution related human diseases. Euro. J. Biomedical Pharma. Sci 5, 988–998. Available online at: http://ejbps.com/ejbps/abstract_id/3934
Wimalawansa, S. J. (2019a). Molecular and cellular toxicity of fluoride in mystery, tubulointerstitial chronic kidney disease: a systematic review. Rev. Environ. Sci. Bio/Technol. 3, 33. doi: 10.1007/s11157-019-09521-0
Wimalawansa, S. J. (2019b). Public health interventions for chronic diseases: cost-benefit modelizations for eradicating chronic kidney disease of multifactorial origin (Ckdmfo/ Ckdu) From Tropical Countries. Heliyon 5, E02309. doi: 10.1016/j.heliyon.2019.e02309
Wimalawansa, S. J. (2019c). Public health interventions for chronic diseases: cost–benefit modelizations for eradicating chronic kidney disease of multifactorial origin from tropical countries. Heliyon. 19, 60. doi: 10.2139/ssrn.3350560
Wimalawansa, S. J. (2019d). Vitamin D deficiency: effects on oxidative stress, epigenetics, gene regulation, and ageing. Biology (Basel) 8, Pii: E30. doi: 10.3390/biology8020030
Wimalawansa, S. J. (2020). Does fluoride cause the mysterious chronic kidney disease of multifactorial origin? Environ. Geochem. Health. 3, 503. doi: 10.1007/s10653-019-00503-3
Wimalawansa, S. J., and Dissanayake, C. B. (2019). Factors affecting the environmentally induced, chronic kidney disease of unknown aetiology in dry zonal regions in tropical countries—novel findings. Environments 7, 1–26. doi: 10.3390/environments7010002
Wimalawansa, S. J., and Wimalawansa, S. A. (2015b). Chronic kidney disease of multifactorial origin (CKDmfo) In Sri Lanka: escalating incidence and long-term survival estimates. J. Nephrology and Urology Research 22, 1–17.
Wimalawansm, S. J. (2015). Strategic framework for managing non-communicable diseases: preventing chronic kidney disease of multifactorial origin In Sri Lanka as an example. Chronic. Dis. Int. 2, 9.
Wong, T. Y., Wu, C. Y., Martel, J., Lin, C. W., Hsu, F. Y., Ojcius, D. M., et al. (2015). Detection and characterization of mineralo-organic nanoparticles in human kidneys. Sci. Rep. 5, 15272. doi: 10.1038/srep15272
Wrighton, K. H. (2017). Acute kidney injury: benefits of blocking fructokinase. Nat. Rev. Nephrol. 13, 192. doi: 10.1038/nrneph.2017.23
Wyskida, K., Witkowicz, J., Chudek, J., and Wiecek, A. (2012). Daily magnesium intake and hypermagnesemia in hemodialysis patients with chronic kidney disease. J. Ren. Nutr. 22, 19–26. doi: 10.1053/j.jrn.2011.03.001
Ye, J., Li, J., Xia, R., Zhou, M., and Yu, L. (2015). Prohibitin protects proximal tubule epithelial cells against oxidative injury through mitochondrial pathways. Free Radic. Res. 49, 1393–1403. doi: 10.3109/10715762.2015.1075654
Yiu, A. J., Callaghan, D., Sultana, R., and Bandyopadhyay, B. C. (2015). Vascular calcification and stone disease: a new look towards the mechanism. J. Cardiovasc. Dev. Dis. 2, 141–164. doi: 10.3390/jcdd2030141
Zager, R. A., Johnson, A. C., Naito, M., and Bomsztyk, K. (2008). Maleate nephrotoxicity: mechanisms of injury and correlates with ischemic/hypoxic tubular cell death. Am. J. Physiol. Renal Physiol. 294, F187–F197. doi: 10.1152/ajprenal.00434.2007
Zha, Y., and Qian, Q. (2017). Protein nutrition and malnutrition in Ckd And Esrd. Nutrients, 9, 208. doi: 10.3390/nu9030208
Zhang, F., Lau, S. S., and Monks, T. J. (2011). The Cytoprotective Effect Of N-Acetyl-L-cysteine against ros-induced cytotoxicity is independent of its ability to enhance glutathione synthesis. Toxicol. Sci. 120, 87–97. doi: 10.1093/toxsci/kfq364
Zhang, M., Zhou, W., Liu, S., and Hao, C. (2021). Phosphate nephropathy in gitelman syndrome. Kidney Med 3, 864–865. doi: 10.1016/j.xkme.2021.04.021
Zuckerman, J. M., and Assimos, D. G. (2009). Hypocitraturia: pathophysiology and medical management. Rev. Urol. 11, 134–144. Available online at: https://www.ncbi.nlm.nih.gov/pubmed/19918339
Keywords: chronic renal failure, CKDu and CKDmfo, environment, fluoride, geochemistry, hard water, nephropathy, tropical countries
Citation: Wimalawansa SJ and Dissanayake CB (2022) CaPO4-Mediated CKD of Crystallo-Tubular-Nephropathy [CKD-CTN]—A Crystal and Nanotube-Induced Geo-Environmental Disease. Front. Water 4:816643. doi: 10.3389/frwa.2022.816643
Received: 16 November 2021; Accepted: 10 February 2022;
Published: 29 April 2022.
Edited by:
Katherine A. James, University of Colorado Anschutz Medical Campus, United StatesReviewed by:
Richard Johnson, University of Colorado Boulder, United StatesPhuong-Thu Pham, University of California, Los Angeles, United States
Copyright © 2022 Wimalawansa and Dissanayake. This is an open-access article distributed under the terms of the Creative Commons Attribution License (CC BY). The use, distribution or reproduction in other forums is permitted, provided the original author(s) and the copyright owner(s) are credited and that the original publication in this journal is cited, in accordance with accepted academic practice. No use, distribution or reproduction is permitted which does not comply with these terms.
*Correspondence: Sunil J. Wimalawansa, suniljw@hotmail.com