- 1Department of Geography and Geosciences, University of Vermont, Burlington, VT, United States
- 2Kansas Geological Survey, University of Kansas, Lawrence, KS, United States
- 3Department of Civil and Environmental Engineering, University of Vermont, Burlington, VT, United States
- 4Department of Civil and Environmental Engineering, Penn State University, University Park, PA, United States
- 5U.S. Geological Survey, Montpelier, VT, United States
The concurrent reduction in acid deposition and increase in precipitation impact stream solute dynamics in complex ways that make predictions of future water quality difficult. To understand how changes in acid deposition and precipitation have influenced dissolved organic carbon (DOC) and nitrogen (N) loading to streams, we investigated trends from 1991 to 2018 in stream concentrations (DOC, ~3,800 measurements), dissolved organic nitrogen (DON, ~1,160 measurements), and dissolved inorganic N (DIN, ~2,130 measurements) in a forested watershed in Vermont, USA. Our analysis included concentration-discharge (C-Q) relationships and Seasonal Mann-Kendall tests on long-term, flow-adjusted concentrations. To understand whether hydrologic flushing and changes in acid deposition influenced long-term patterns by liberating DOC and dissolved N from watershed soils, we measured their concentrations in the leachate of 108 topsoil cores of 5 cm diameter that we flushed with solutions simulating high and low acid deposition during four different seasons. Our results indicate that DOC and DON often co-varied in both the long-term stream dataset and the soil core experiment. Additionally, leachate from winter soil cores produced especially high concentrations of all three solutes. This seasonal signal was consistent with C-Q relation showing that organic materials (e.g., DOC and DON), which accumulate during winter, are flushed into streams during spring snowmelt. Acid deposition had opposite effects on DOC and DON compared to DIN in the soil core experiment. Low acid deposition solutions, which mimic present day precipitation, produced the highest DOC and DON leachate concentrations. Conversely, high acid deposition solutions generally produced the highest DIN leachate concentrations. These results are consistent with the increasing trend in stream DOC concentrations and generally decreasing trend in stream DIN we observed in the long-term data. These results suggest that the impact of acid deposition on the liberation of soil carbon (C) and N differed for DOC and DON vs. DIN, and these impacts were reflected in long-term stream chemistry patterns. As watersheds continue to recover from acid deposition, stream C:N ratios will likely continue to increase, with important consequences for stream metabolism and biogeochemical processes.
1. Introduction
Carbon (C) and nitrogen (N) species influence ecosystem processes and the quality of natural waters, and are thus monitored globally (Smith et al., 2003; Frei et al., 2021). For example, the dissolved fraction of organic carbon (DOC) in streams and rivers plays an important role in the global C cycle (Schlesinger and Melack, 1981; Aufdenkampe et al., 2011; Perdrial et al., 2014). Nitrogen is a limiting nutrient in terrestrial ecosystems and N accumulation can lead to eutrophication with significant ecosystem effects (Fenn et al., 1998). While the total amounts of C and N species impact water quality significantly, their relative proportions, or stoichiometry, are equally important for aquatic productivity (Fenn et al., 1998). Nutrient ratios close to those found in aquatic organisms (i.e., the Redfield ratios C:N:P = 106:16:1) foster aquatic productivity most (Berner and Berner, 2012). Additionally, C:N stoichiometry can be used to make inferences about the energy and nutrient balance within an ecosystem and is greatly impacted by solute inputs, disturbance dynamics, and seasonal hydrologic events (Kincaid et al., 2020; Wymore et al., 2021; Li et al., 2022; Rodríguez-Cardona et al., 2022).
Hydrology is a dominant driver of C and nutrient export at the catchment scale because soil derived constituents are flushed into streams (Raymond and Saiers, 2010; Wilson et al., 2013; Wen et al., 2020; Seybold et al., 2022). Thus, regional changes in timing, composition and amount of precipitation influence total and relative C and N concentrations in streams (Sebestyen et al., 2008; Rodríguez-Cardona et al., 2022). In the northeastern U.S., increases in heavy precipitation (Hayhoe et al., 2007; Galford et al., 2014; Caretta et al., 2022) are likely to impact both C and N export because they are discharge (Q)-dependent constituents. For example, in most catchments DOC increases with Q because DOC tends to be mobilized through direct soil flushing during overland flow or when the water table rises to reach shallow soil horizons (Perdrial et al., 2014; Zhi et al., 2019; Stewart et al., 2022). In snow-dominated catchments, such as those in the northeastern United States, spring snowmelt is a particularly important time for DOC export, because melt waters flush out labile C that accumulated under the snowpack (Brooks and Williams, 1999). Similarly, N can accumulate in snow-covered soil and snowpack and flush out during spring snowmelt (Brooks et al., 1998; Brooks and Williams, 1999; Campbell et al., 2006; Pellerin et al., 2011). During rain-on-snow events, which are increasing in their frequency (Il Jeong and Sushama, 2018), a large amount of N can be exported in a brief period of time (Murray et al., 2022; Seybold et al., 2022).
In the northeastern United States, changing amounts of precipitation is interacting with another driver, i.e., a shift in atmospheric acid deposition. Since the implementation of the 1990 Clean Air Act Amendments, deposition of anthropogenic acids and their dissociation products [e.g., nitrate () and protons (H+)] have decreased, leading to decreases in in streams (Aber et al., 1998; Fenn et al., 1998). While C species are minor in this deposition, this shift has also impacted DOC in streams (Evans et al., 2005; Monteith et al., 2007; Lepistö et al., 2008; Adler et al., 2021). Some studies have established links to C mobilization from soils and show that soil aggregates can become destabilized in low-charge density environments (similar to conditions during recovery from acidification) and release DOC as a result (Cincotta et al., 2019; Adler et al., 2021). Because soil organic matter is a common source for both organic C and N species (Liu et al., 2019), coupled DOC and dissolved organic nitrogen (DON) release during soil aggregate breakup is likely. Indeed, a recent study across biomes demonstrated that recovery from atmospheric acid deposition has led to changes in the organic matter pool and shifts in DOC:DON ratios (Rodríguez-Cardona et al., 2022); however, because dissolved organic nitrogen (DIN) is not a typical aggregating agent (Zhang et al., 2016), aggregate related processes are unlikely to significantly contribute to its release through this process.
Our objective was to investigate the connection between long-term regional-scale atmospheric drivers, stream response and seasonal catchment soil dynamics to disentangle the relative contribution of each driver to the separate and intersecting pathways of DOC, DON, and DIN export. Our study was focused on a forested headwater catchment in northeastern Vermont, United States, Sleepers River Research Watershed (SRRW), which has experienced increases in precipitation, decreases in acid deposition and has high-resolution long-term environmental data recording these changes since the early 1990s (Shanley et al., 2015). To determine the impact of increasing Q on long-term stream water dissolved organic C and N concentrations, we investigated concentration-discharge (C-Q) relation across seasons. To isolate temporal trends in these data independent of Q (e.g., possibly in response to reduced acid deposition), we applied Seasonal Mann-Kendall tests on flow-adjusted data. To assess the combined effect of hydrologic flushing due to increasing precipitation and shifts in acid deposition on mobilization of C and N species from catchment soils, we measured dissolved organic C and N concentrations in the leachate of soil cores that we flushed with solutions simulating high and low acid deposition during four different seasons.
With these approaches we test the following hypotheses: (1) The combined effects of increased precipitation and reduced acid deposition impact dissolved organic C and N species differently, which leads to a shift in C:N stoichiometry in soil and streams. Specifically, because organic species in stream water (DOC and DON) increase with Q (over time and across seasons) and acid deposition decreases soil organic matter solubility, DOC and DON concentrations will increase with increasing Q and decreasing acid deposition; and (2) stream water DIN concentrations will decrease over time with decreasing acid ions (e.g., ) in atmospheric deposition, but will show seasonal variations. However, because DIN is not a strong aggregating agent in soils, experimental manipulations of acid deposition do not impact DIN release through soil aggregate breakup, leading to a further decoupling of C and N response to these drivers and increases in C:N ratios in the long term.
2. Methods and materials
2.1. Study area
SRRW is comprised of nested catchments varying from forested to agricultural landcover. Our main study location was the 40.2-ha (0.402 km2) headwater watershed W-9, which is 100% forested in northern hardwoods (Figure 1). In 1988, the northeast region collectively received an estimated 14 to >20 kg/ha of atmospheric nitrate ion wet deposition; compared to 2018, where levels are estimated to be < 8 kg/ha (Shanley et al., 2004, 2015). Hydrologic data date back to 1960 (Chalmers et al., 2019; Shanley et al., 2022) and biogeochemical data (mostly weekly sampling) date back to 1991 (Matt et al., 2021), representing one of the longest continuous hydrochemical records in the region (Shanley et al., 2022).
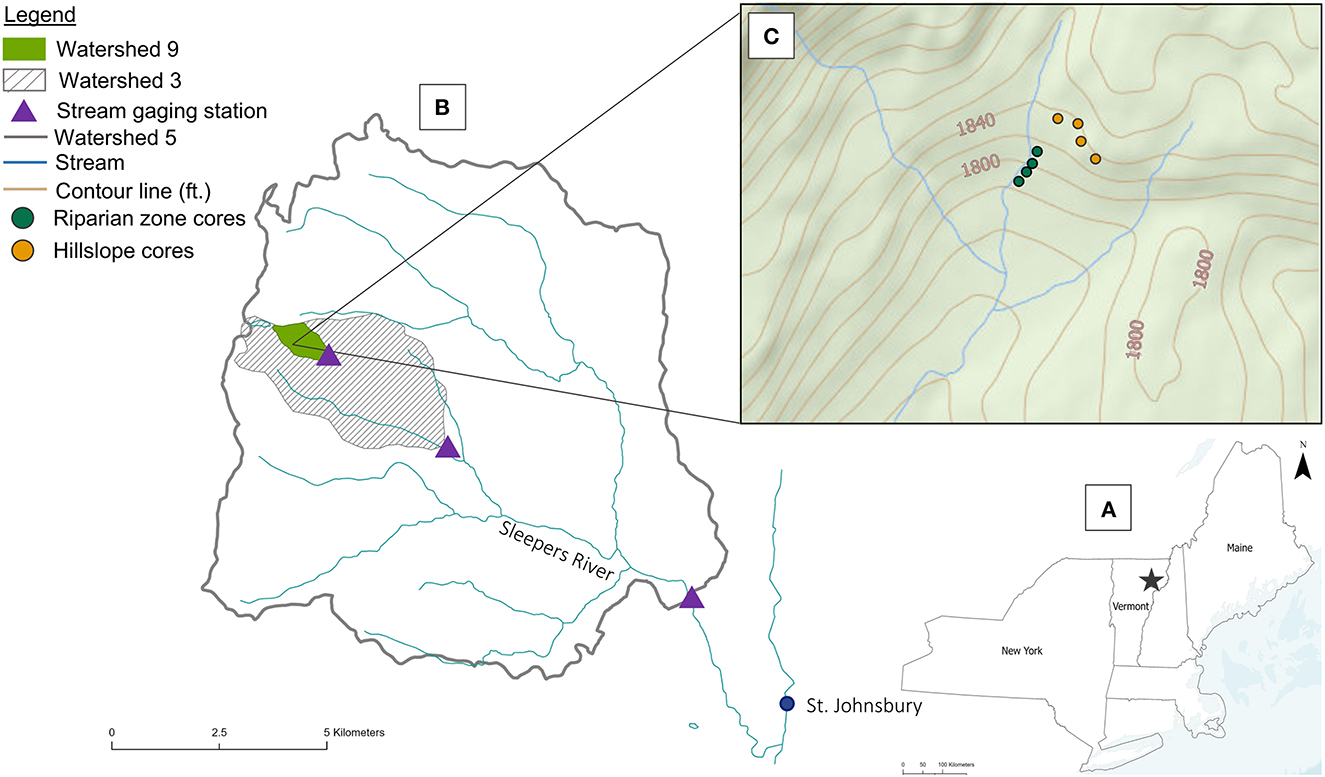
Figure 1. (A) Location of field site in the northeastern United States. (B) Sleepers River Watershed in northeastern Vermont (gray outline), Watershed 3 (gray hatched), and the forested watershed—Watershed 9 (green shaded). (C) Location of soil core sampling sites. Hillslope samples are represented by orange circles, riparian by green circles. Modified from Shanley et al. (2022).
The bedrock geology of the area is dominantly quartz-mica phyllite with interbedded calcareous granulite (Shanley et al., 2004). The presence of calcite in the parent material distinguishes SRRW from many other northeastern catchments in that the ground and stream water are well-buffered, and deeper soils are not calcium depleted (Armfield et al., 2019). The bedrock is overlain by 1–4 m of basal till emplaced from previous glaciation (Shanley et al., 2004). Dominant soil types include Inceptisols and Spodosols on hillslopes, and Histosols in riparian zones. Forest cover in W-9 consists of sugar maple, beech, yellow birch, and white ash (Shanley et al., 2004). SRRW is located in a humid, temperate region where annual temperatures range from −30 to +30°C (Pellerin et al., 2011). W-9 is a seasonally snow-covered catchment and 20–30% of yearly precipitation accumulates as snow (Shanley et al., 2015). Water yields are dominated by spring snowmelt and event-driven flow; therefore, streamflow and solute concentration have distinctive seasonal patterns (Sebestyen et al., 2008; Pellerin et al., 2011; Shanley et al., 2015; Armfield et al., 2019; Stewart et al., 2022).
2.2. Field sampling and experimental design
To capture seasonal variations in hydrologic drivers and nutrient dynamics, we collected soil cores during fall, winter, spring, and summer (Supplementary Table 1). To investigate how landscape position impacts solute release across seasons, soil cores were sampled from both hillslopes and riparian zones. During each of the sampling campaigns, we collected 27 cores—of which 13 were taken from hillslopes and 14 from riparian zones.
Before collecting cores, we removed leaf litter and forest debris from the soil surface. This was particularly important for fall soils that were covered by a layer of fresh leaf litter. Winter soils were covered by ~120 cm of snow that was removed before sampling. Our experiments focused on the interaction of event waters and shallow soil layers, therefore only the top 10 cm of soil (O and A horizons) was collected by hammering 5 cm diameter PVC pipes into the soil. Cores were carefully removed to maintain the structural integrity of the sample, sealed with parafilm and tape, transported to the laboratory, and stored at 4°C until experimentation within 24 h of collection.
We designed soil core experiments to test the effects of hydrologic flushing events and shifts in solution chemistry on soil DOC, DIN, and DON release. We used solutions that simulated past and present precipitation and soil chemistry conditions at SRRW (Shanley et al., 2004; Armfield et al., 2019) as follows:
• The acidification treatment simulated conditions during the onset of acidification—having high ionic strength (3*10−2 M) from calcium chloride (CaCl2), and low pH (=3 adjusted with hydrochloric acid).
• The recovery treatment simulated reduced acid deposition—having low ionic strength (<10−5 M) and a pH of 5.
• The third treatment (mixed treatment), had a low pH (comparable to the acidification treatment) and low ionic strength (comparable to the recovery treatment), to allow for testing the influence of pH and ionic strength as individual variables.
For flushing experiments, we followed the procedure outlined in Cincotta et al. (2019) and Adler et al. (2021), in which soil cores were positioned vertically and fitted with bottom PVC caps with stop valves to control drainage and the interaction time between the experimental solutions and the soil. Each flushing consisted of the addition of 120 ml of solution to the top of the core, followed by 5 min of interaction time. Gravitationally drained leachate was collected for 4 min into clean HDPE bottles. Leachate of each flushing was collected individually and filtered through a 0.45-μm polyethersulfone filter into combusted glass vials within 24 h of experiments. A schematic of the soil core setup is provided in Supplementary Figure 1. This process was performed twice in sequence to capture “first flush” (i.e., solutes sorbed on soil surfaces and contained in soil pore water) and subsequent solute release. We did not flush soils further because previous studies showed a significant decrease in solute concentration after two flushes (Adler et al., 2021; Bristol, 2021).
2.3. Sample and data analysis
Soil leachate was analyzed for DOC and Total Dissolved Nitrogen (TDN) using a Shimadzu Carbon Analyzer (Shimadzu, Colombia, MD, USA). Anions, including nitrate and nitrite, were measured using a Thermo Scientific Dionex Aquion Ion Chromatography System (Thermo Fisher Scientific, USA), and reported as N (e.g., Nitrate-N). pH was measured using a benchtop pH probe and meter to confirm target pH (±0.1 pH units). DON was calculated by subtracting DIN (i.e., nitrate and nitrite) from TDN. Note that ammonium was below detection limit. To compare results between soil cores, leachate was normalized to the amount of liquid and solid and reported in mg/kg of soil. All statistical analyses were performed using RStudio Version 1.3.1093. Kruskal-Wallis tests were applied to compare soil cores by treatment and landscape position. Statistical significance for difference in medians for treatment and landscape position were determined using an α threshold of 0.05. A post-hoc Dunn test with a Bonferroni adjustment was applied for categorical variables that were detected by the Kruskal-Wallis test to be statistically significant.
2.4. Long-term trend analysis: Flow-adjusted data and seasonal Mann-Kendall tests
To investigate stream response to long-term shifts in atmospheric drivers we used two main approaches on the stream solute concentration dataset from SRRW W-9 (Matt et al., 2021; Shanley et al., 2021, 2022). The datasets comprise grab sample concentrations from September 30, 1991 to December 26, 2018 for DOC and DIN and from February 22, 2005 to December 26, 2018 for DON with 3,799 measurements for DOC, 2,131 for DIN, and 1,155 for DON. The first approach investigated how stream solute concentrations vary with variations in Q. For this we performed linear regressions on the relationship between instantaneous log-transformed DOC, DIN, and DON concentrations (mg/L) with corresponding instantaneous log-Q measurements at the sampling time and plotted these by meteorological season.
Our second approach evaluated temporal trends in stream water solute concentrations independent of Q. For this we applied a Locally Weighted Scatterplot Smoothing (LOWESS) regression on log-transformed DOC, DON, and DIN concentrations and Q, using a smoothing pattern coefficient (f = 0.67), and extracted the residuals (Supplementary Figure 2). Flow-adjusted concentrations (DOCFA, DINFA, DONFA) were obtained from reordering the LOWESS residuals according to the corresponding time stamp for each observation (Hirsch and Slack, 1984; Helsel et al., 2020).
To correct for seasonal dependence, we performed monotonic trend analysis for time series, or Seasonal Mann-Kendall statistical tests, to detect upward (positive) or downward (negative) trends in flow-adjusted concentrations independent of seasonal influence (Hirsch and Slack, 1984). Positive and negative trends were identified by the normalized test statistic, Kendall's tau (tau = ±1), where positive trends have a tau-value >0.05 and negative trends <0.05, with a statistically significant α threshold of 0.05. Finally, we applied a Pettitt test to identify change points (trend shifts) in continuous time series data that otherwise could be missed from monotonic trend analysis (Pettitt, 1979).
3. Results
3.1. Long-term stream chemistry: C-Q relationships
The C-Q response and concentration variability changed seasonally for most of the investigated solutes at SRRW. However, DOC and DON had similar export behavior across seasons. In all seasons, DOC and DON had positive C-Q slopes, indicating enrichment with increasing Q, but C-Q slopes for DON were much closer to zero, indicating a more constant response with changing Q (Figure 2). DOC concentrations were generally highest and most variable in summer and fall and DON concentrations were most variable in fall and winter (Table 1). In contrast to DOC and DON, the directionality of DIN C-Q slopes varied across seasons but were positive in fall and spring. The highest DIN concentrations occurred in winter and spring.
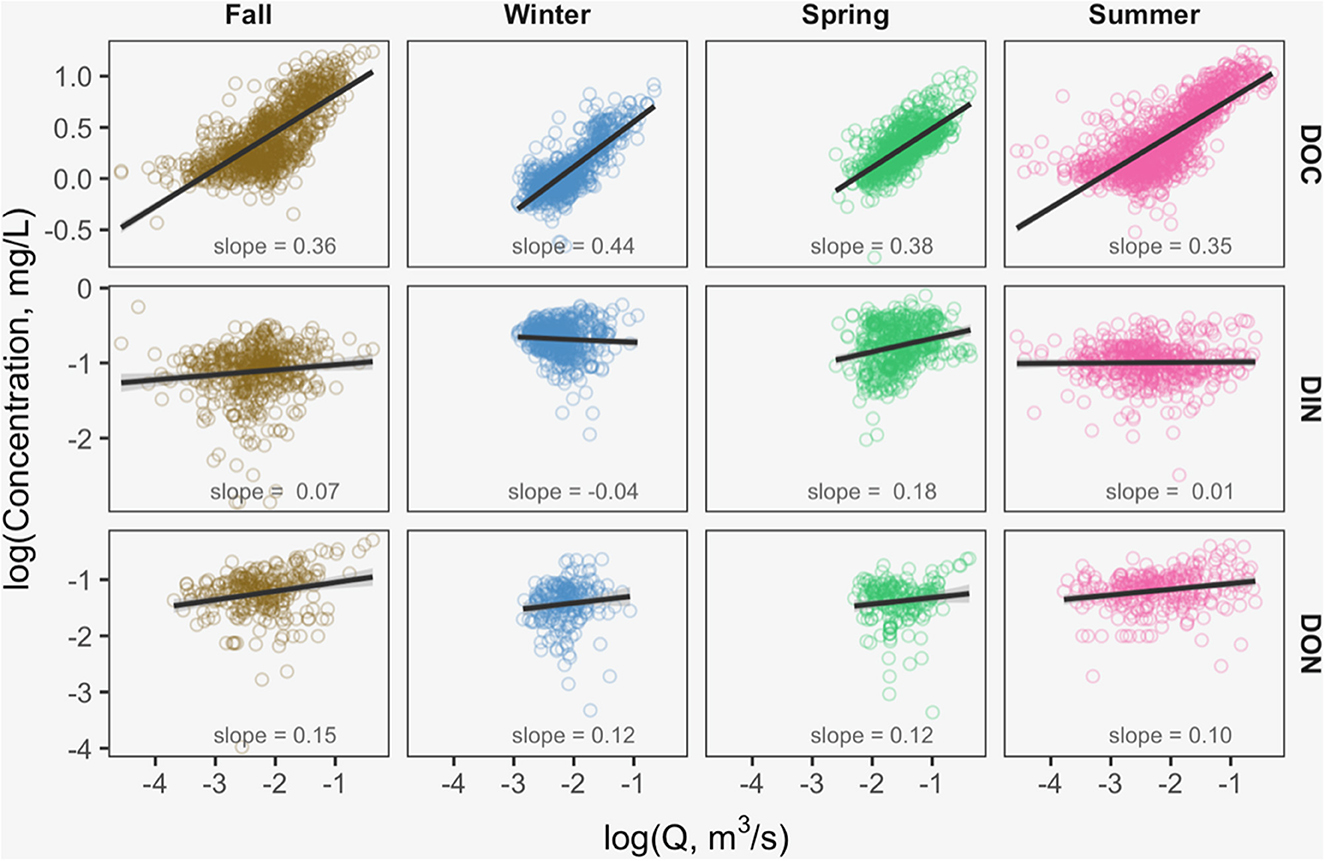
Figure 2. Seasonal concentration-discharge (C-Q) behavior for log-transformed dissolved organic carbon (DOC), dissolved inorganic nitrogen (DIN), and dissolved organic nitrogen (DON) concentrations in mg/L vs. log-transformed Q in cubic meters per second (m3/s). Seasons are represented by color. The slope of the log C-log Q relationship is shown and describes the directionality of the relationship. Positive slopes indicate an enrichment response. Negative slopes indicate a dilution response. Slope values near zero indicate a constant response.
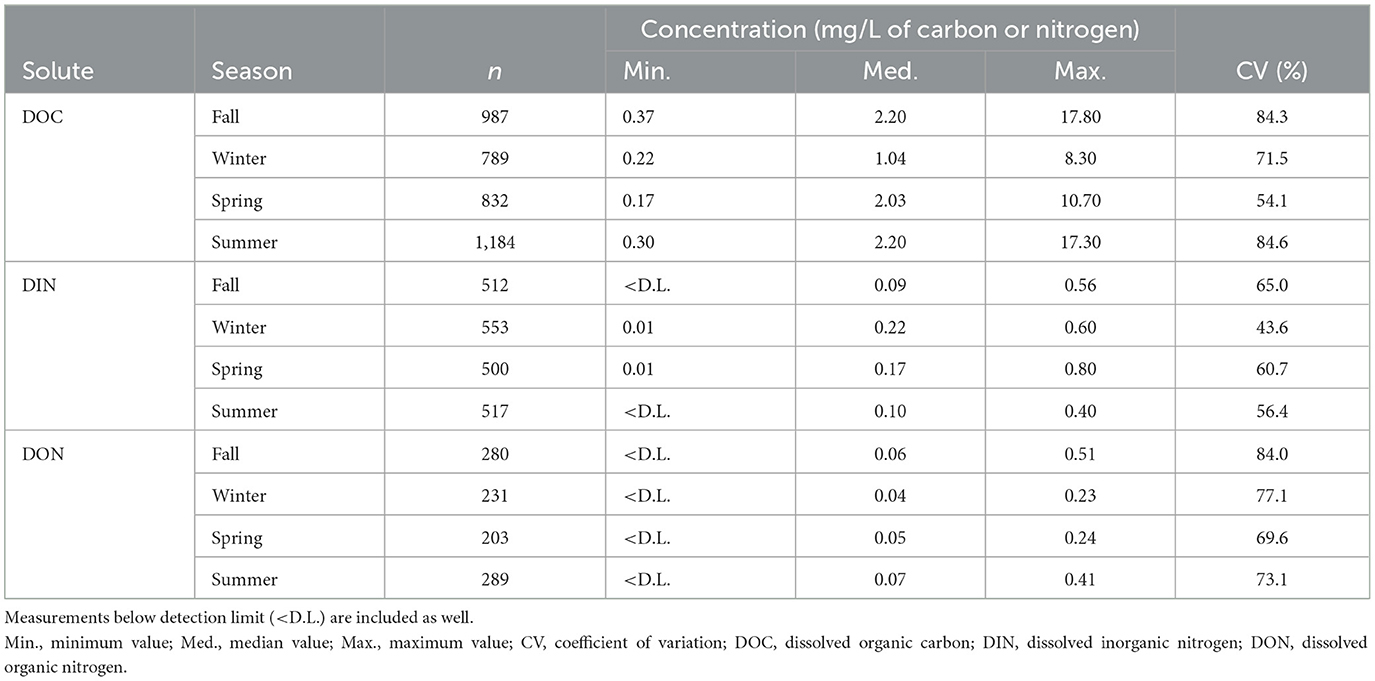
Table 1. Number of observations (n) by solutes and season and statistical information for concentration-discharge relationships.
3.2. Long-term stream chemistry: Flow-adjusted trends
DOCFA, DINFA, and DONFA showed significant but contrasting temporal trends. DOCFA generally increased across the period of observation between 1991 and 2018 (tau = 0.116, p < 0.001, Figure 3A), but no single season drove this increase (seasonally independent DOCFA trends were insignificant; Supplementary Figures 2–4). Pettitt change point detection analysis for DOCFA revealed a trend shift in 2005. Prior to 2005, the strength of the trend (tau = 0.08, p < 0.001) was greater than for the 2005–2018 period (tau = 0.07, p < 0.001, Figure 3A). In contrast, DINFA generally decreased between 1991 and 2018 (tau = −0.19, p < 0.001, Figure 3B) and significant decreases were observed across all seasons (Supplementary Figure 4). Pettitt change point detection analysis for DINFA revealed a trend shift in 2002. Prior to 2002, the DINFA trend was negative (tau = −0.08, p = 0.006). After 2002, the trend for DINFA was positive (tau = 0.06, p = 0.01), but the signal was more variable. Specifically, DINFA oscillated from 2004 to 2007, dramatically decreased after 2007, increased again until 2015, and decreased until the end of the record (Figure 3B). DON data were not available prior to 2005 so we could not analyze the longer-term trends. However, the DONFA trend after 2005 was positive (tau = 0.026), and flow-adjusted concentrations mirrored the general oscillating pattern observed for DINFA (Figure 3C). Further, the 2014 peak in DONFA occurred in all seasons that year (Supplementary Figure 5). The general trend of increasing DOC concentrations and decreasing DIN concentrations led to an increase in C:N ratios (tau of seasonal Mann Kendall = 0.23), with most of the increase happening since 2015 (Figure 3D).
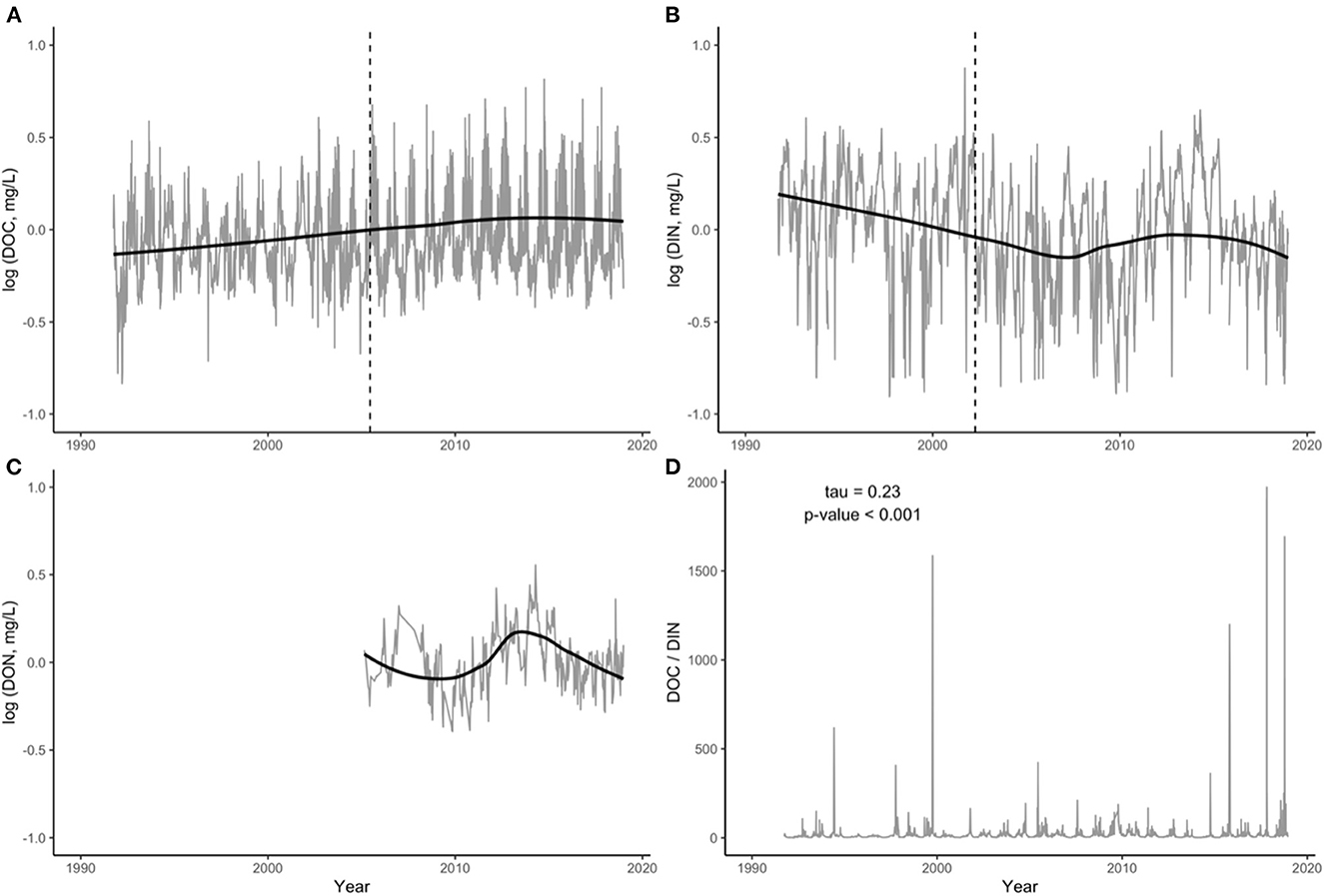
Figure 3. Long-term trends in (A) flow-adjusted dissolved organic carbon (DOC) concentrations, (B) flow-adjusted dissolved inorganic nitrogen (DIN) concentrations, (C) flow-adjusted dissolved organic nitrogen (DON) concentrations, and (D) C:N ratios calculated from DOC and DIN concentrations. For DOCFA and DINFA, change points (indicated by vertical dashed lines) were found in the early 2000s and trends both before and after the change points are statistically significant (α ≤ 0.05) and positive for all, except negative for DIN before the change point. Seasonal Mann Kendall tests on C:N ratios indicate a positive trend (tau = 0.23) over the time series.
3.3. Soil core experiments: DOC, DIN, DON leachate by season, treatment, and landscape position
Soil core leachate DOC concentrations varied notably by season, but the treatment effect was only significant for winter samples (Kruskal-Wallis, p = 0.04). Leachate DOC concentrations from fall soils were low among treatments (1–17 mg/kg; Figure 4). Leachate DOC concentrations in winter soil had the highest mean concentration from the recovery treatment for both hillslopes [35.2 mg/kg, standard deviation (sd) = 31.1] and riparian zones (20.0 mg/kg, sd = 19.1), and these concentrations were significantly greater than for the acidification treatment (Dunn, p = 0.04; Figure 4). Spring soils had the lowest DOC concentrations, ranging from 0.9 to 10 mg/kg (Figure 4). Summer leachate DOC concentrations were generally high, ranging from 0.8 to 27 mg/kg (Figure 4). Irrespective of treatment, the highest DOC concentrations were found in leachate from hillslope soils in the fall and winter and riparian soils in the summer.
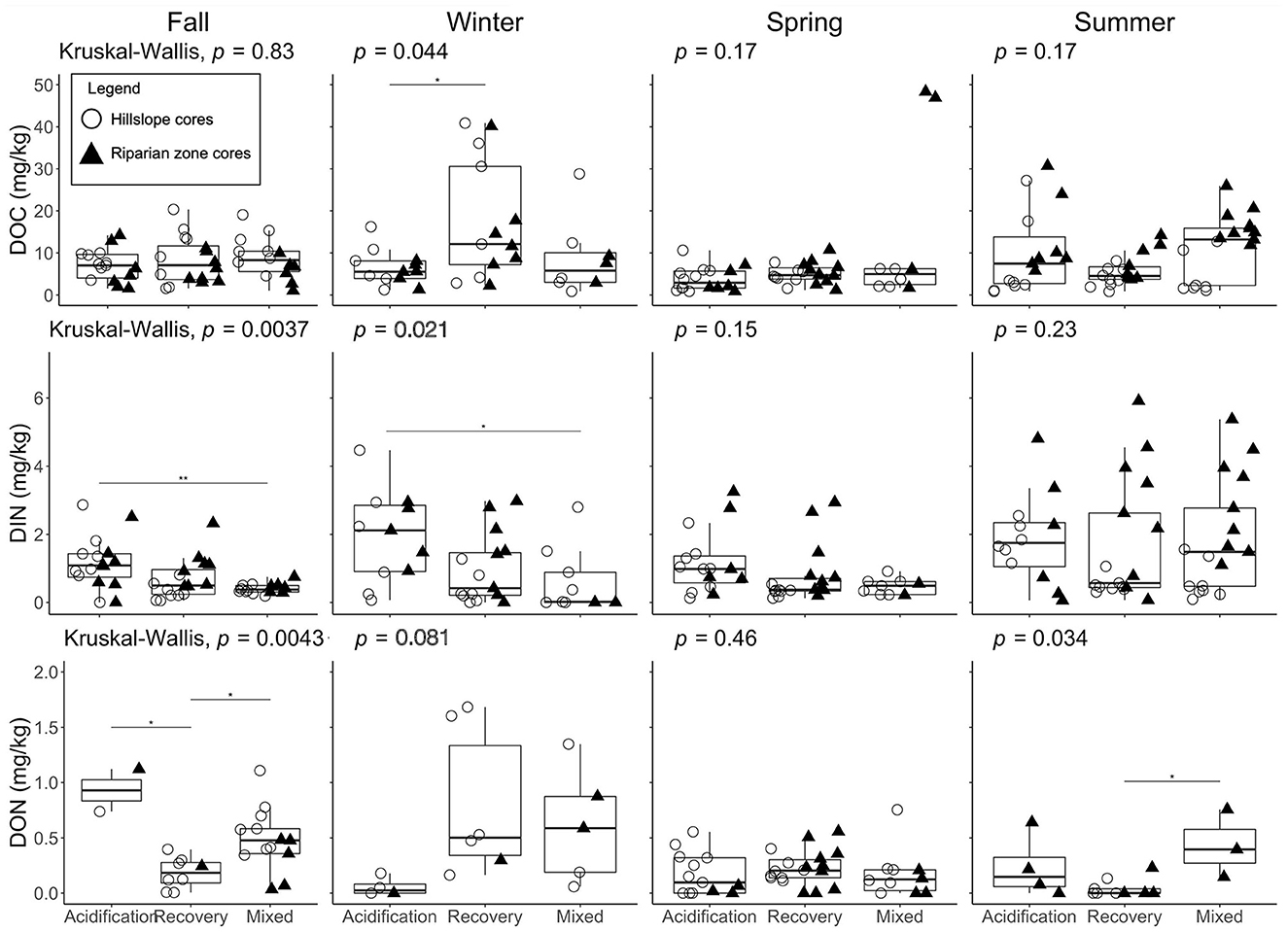
Figure 4. Seasonal (left to right) dissolved organic carbon (DOC), dissolved inorganic nitrogen (DIN), and dissolved organic nitrogen (DON) concentrations (top to bottom) from soil core leaching experiments. Boxplots are ordered by soil core treatment. Kruskal Wallis tests were used to determine statistically significant (p ≤ 0.05) differences in means between each treatment. A post-hoc Dunn test with a Bonferroni correction was applied to seasons with a significant Kruskal Wallis p-value. Brackets indicate between which treatments were significant, and asterisks denote significance of treatments as follows: *p ≤ 0.05, **p ≤ 0.01. Symbols represent the landscape position where the core was collected; open circles represent hillslopes; and solid triangles represent riparian zones. Box plots depict the minimum, first quartile, median, third quartile, and maximum, with outliers depicted as single points.
DIN concentrations in leachate varied considerably by season and in some cases by treatment. For example, DIN concentrations in fall leachates were generally low (0–2.9 mg/kg), but leachate from the acidification treatment had the highest mean concentration (1.16 mg/kg, sd = 0.77) and was significantly different than concentrations in the leachate from the mixed treatment (Dunn, p = 0.003; Figure 4). The acidification treatment for winter soils also had the highest leachate DIN concentrations (maximum of 4.5 mg/kg; hillslope mean = 1.81 mg/kg, sd = 1.72; riparian mean = 3.72 mg/kg, sd = 4.17) and leachate DIN concentrations from these soils were significantly higher than DIN in leachates from the mixed treatment, which had the lowest mean DIN concentrations for winter soils (Dunn, p = 0.02; Figure 4). The mean DIN concentration from the recovery treatment was between those from the acidification and mixed treatments and ranged from 0.2 mg/kg for hillslopes to 1.5 mg/kg in riparian zones. Spring soils had the lowest leachate DIN concentrations (maximum of 2.33 mg/kg) and there were no significant differences among treatments (Figure 4). Leachate DIN concentrations from summer soils did not differ among treatments, but were frequently higher than in other seasons, with the highest concentration from riparian soils (4.8 mg/kg; Figure 4).
There were more seasonal and treatment differences for DON than for DOC and DIN. The highest mean DON concentration in fall leachate was from the acidification treatment (0.93 mg/kg, sd = 0.27), which was significantly greater than the mean recovery concentration (0.18 mg/kg, sd = 0.14; Dunn, p = 0.01; Figure 4). The second highest mean DON concentration was in leachate from the mixed treatment (0.48 mg/kg, sd = 0.28), which was also significantly greater than the mean recovery concentration (Dunn, p = 0.02). Similar to DOC and DIN, DON leachate concentrations from winter soils (especially hillslopes) were high compared to other seasons (up to 1.68 mg/kg) but did not vary significantly by treatment (Figure 4). Leachate from spring soils yielded low concentrations (maximum of 0.51 mg/kg) and there were no treatment differences (Figure 4). Leachate from summer soils, especially riparian soils, were generally higher than for other seasons (up to 0.76 mg/kg; Figure 4). The highest mean DON concentration was in leachate from the mixed treatment (0.43 mg/kg, sd = 0.30), which was significantly greater than recovery concentrations (Dunn, p = 0.04).
4. Discussion
Overlapping drivers such as shifts in acid deposition and increased precipitation impact stream water solute patterns in complex ways (Freeman et al., 2001; Worrall et al., 2004; Eimers et al., 2008a,b; Clark et al., 2010), making it challenging to predict water quality in light of continued changes. Our overarching objective was to investigate the connection between regional drivers, catchment dynamics, and differential stream response, in order to disentangle the C and N response to these drivers and assess the effect on C:N stoichiometry.
4.1. Patterns in stream water: Do C and N respond differently to overlapping drivers?
To identify general patterns in long-term trends and C-Q relation, we first investigated the Q control on DOC and N-species in long-term datasets and found variability in C-Q patterns for all investigated solutes with pronounced seasonal differences (Figure 2). DOC and DON export behavior was similar in most seasons, with concentrations increasing with Q, though less so for DON. This was consistent with our hypothesis that DOC and DON would originate from a common, soil-derived source and increase in concentration as streams connected to upper soil horizons at higher flows. Most of the higher flows occurred in spring and summer during snowmelt events and rainstorms. Such events are particularly important for soil flushing and mobilizing substantial amounts of DOC and other soil-derived solutes to streams (Boyer et al., 1997; Sebestyen et al., 2008; Pellerin et al., 2011). Due to dilution from snowmelt, stream concentrations were often lower in winter and spring compared to other seasons, however, because of the large volume of water exported, this time is a significant export period for soil-derived solutes (Pellerin et al., 2011; Seybold et al., 2022). Higher concentrations in summer and fall mean that rainstorms also export a significant amount of these solutes despite the shorter-lived nature of storms vs. snowmelt (Kincaid et al., 2020). This is in agreement with predictions that DOC export is likely to increase as the result of longer growing seasons (Shanley et al., 2015).
DIN concentrations, in contrast, were highest in winter and spring (Figure 2), a signal that is consistent with previous studies in the area that found stream nitrate elevated as a result of rain-on-snow events and early snowmelt (Sebestyen et al., 2008; Pellerin et al., 2011; Casson et al., 2019; Kincaid et al., 2020; Seybold et al., 2022). DIN concentrations, however, did not vary systematically with Q, and in contrast to the organic solutes, soil flushing might not be the dominant control. Instead, seasonal controls are important for DIN export, particularly after DIN accumulates in soils during winter and subsequent snowmelt and spring rains export the DIN prior to the growing season when biological demand is low (Brooks et al., 1998, 2011). Prior to the prolonged snowmelt period, rain-on-snow events are particularly hot moments of DIN export, contributing up to 90% of winter nitrate export in seasonally snow-covered catchments (Casson et al., 2014). These events need to be considered carefully with respect to future patterns in stream N, as rain-on-snow events are projected to become more abundant with winter climate change (Il Jeong and Sushama, 2018; Seybold et al., 2022).
To assess the presence or significance of drivers—other than the often dominant Q control on stream solutes, we also investigated temporal trends in flow-adjusted concentrations. Our findings of increasing DOCFA (Figure 3A) over several decades are in agreement with previous work that identified a significant positive trend for DOC at SRRW that has been attributed to reduced acid deposition (Cincotta et al., 2019; Adler et al., 2021; Bristol, 2021; Huntington and Shanley, 2022); DONFA was not available for this time period. However, this increase is not driven significantly by any specific season (Supplementary Figure 3), which indicates that a number of processes likely contribute to this pattern. Contrary to the increase in DOCFA, DINFA showed a general, significant decrease over past decades (Figure 3B), which is consistent with decreases in atmospheric nitrate deposition across other watersheds in the northeast (Eshleman et al., 2013; Frei et al., 2021), and is reflected by decreases in all seasons (Supplementary Figure 4). Overall, the impact of both drivers on increasing DOC, together with the deposition-related decreases in nitrate led to a significant increase in C:N ratios especially since 2015 (Figure 3D).
The large fluctuations for DINFA and DONFA after the mid-2000s are superimposed on the general decrease of N in streams and point to additional dynamics independent of shifts in deposition. This pattern is a regional phenomenon that is also apparent, for example, at nearby Hubbard Brook Experimental Forest. The stream N fluctuations do not match up with regional shifts in precipitation chemistry, no exact cause has been identified. However, the moderate drought in late 2009 and 2010, followed by an exceptionally wet year in 2013–2014 across New England may have contributed (U.S. Drought Monitor, 2023; Supplementary Figure 6). Periods of drought reduce soil microbial activity and plant uptake (Rupp et al., 2021) and lead to accumulation of N that is available for flushing thereafter (McClain et al., 2003; Rupp et al., 2021). Indeed, a recent study found that dry periods followed by heavy rainfall can disrupt the water-solute balance in soils, and lead to exceptionally high N export in streams (Loecke et al., 2017; Rupp et al., 2021). The decoupling of C and N dynamics is interesting and points to complex dynamics that require further investigation at a regional scale.
Even though the flow-adjustment method controls for the variability in DOC and N concentrations in stream water with fluctuating Q, this does not mean that observed changes in these constituents are not related to shifts in precipitation amounts. For example, increased precipitation can lead to anoxic conditions with increases in soil moisture, reducing carbon respiration (Wen et al., 2020), but having less effect on the processing of N forms. In this scenario, DOC can accumulate despite warm summer temperatures and nitrate would be reduced to gaseous forms (Hungat et al., 2003; McNeill and Unkovich, 2007). Indeed, summer DOC concentrations were elevated at high flow, which is in agreement with previous studies (Ryan et al., 2021, 2022). Nitrate concentrations were also high, albeit more variable, which might signal that denitrification was spatially and/or temporally more variable. Additionally, recent research emphasized the role of throughfall as an important source of soil and stream dissolved organic matter during summer months when leaves are out (Ryan et al., 2021, 2022). The influx of throughfall is relatively enriched in C and might contribute to observed patterns.
4.2. The role of soil derived C and N: Indications of co-variations in pathways
To test our hypotheses on the pathways of DOC, DON, and DIN from soil flushing and shifts in precipitation chemistry, we conducted soil core experiments with solutions representing high and low acid deposition. These experiments allowed us to simulate intense rain/snowmelt with general conditions of past acidification, with high ionic strength and low pH, as well as current conditions with higher pH and lower ionic strength. Because availability of solutes for mobilization is a function of seasonal dynamics of production, removal, and catchment connectivity (Bernhardt et al., 2017), we conducted these experiments across seasons and landscape positions. Specifically, we hypothesized that organic species (DOC and DON) are preferentially leached from soils with solutions that simulate reduced acid deposition, as these are common constituents in soil aggregates that become unstable in these conditions. In contrast, because DIN is not a strong aggregating agent, we hypothesized that simulated shifts in acid deposition during experiments do not impact DIN release, but that seasonal variations dominate.
Interestingly, the only scenario that aligned with our prediction that leachate DOC concentrations would be higher in the recovery treatment than the acidification treatment was with the winter soils. We surmise this is the case because shifts in precipitation chemistry influence soil DOC availability by affecting soil aggregate stability-instability processes. Indeed, others have found that soils at SRRW treated with a low charge density solution released more DOC and had smaller aggregates after treatment than those treated with a high charge density solution (Cincotta et al., 2019; Adler et al., 2021). In fall soils a large proportion of the soil DOC pool is likely highly soluble DOC derived from freshly fallen plant and leaf detritus (Goodale et al., 2009; Wilson et al., 2013). Hence, any additional DOC released from soil aggregates in the presence of the recovery treatment solution was likely masked by this larger DOC pool. Interestingly, DON concentrations were highest in the fall acidification treatment, suggesting additional processes may impact DON mobilization in fall, though the small sample size for that treatment prevents us from claiming anything conclusively (Figure 4). Regardless, in winter, the labile DOC pool derived from fall plant detritus has been exported or immobilized. Consequently, when winter soils are exposed to the recovery solution, solutes sorbed to soil aggregates and particles are released into soil solution. We observed this in winter soils for both DOC and DON. This is consistent with a previous study that found high organic solute mobilization from winter soil experiments (Bristol, 2021).
The increased release of organic species from winter soils has important implications for DOC and DON export given that the largest hydrological event in this seasonally snow-dominated system is spring snowmelt (Perdrial et al., 2014). During snowmelt, the entire catchment typically becomes hydrologically connected to the stream (Pellerin et al., 2011), and solutes that accumulated or were released from soil aggregates are exported from the catchment. We suspect we did not see a similar response in spring soils because these soils may have already been flushed during snowmelt at our site. Previous flushing from snowmelt would also explain why all investigated solutes had relatively low concentrations across treatments (Figure 4).
As hypothesized, DIN concentrations in leachate were strongly impacted by seasons and were generally low in spring and fall and high in summer and winter. Even though we did not actively simulate the variety of biogeochemical and hydrological processes across seasons, the conditions of sampled soils are a result of these combined processes prior to sampling, and we interpret our results in this context. For example, elevated temperatures and active vegetation make summer a biogeochemically active time. Riparian zones remain wet and have ideal conditions for biogeochemical cycling (Bernhardt et al., 2017). The high DIN and DOC release from riparian soils indicates that biogeochemical cycling was highly active, but that neither aerobic organic matter respiration nor anaerobic nitrate reduction dominated as these processes would have resulted in lower concentrations of both species.
Contrary to our hypotheses, soil DIN responded strongly to treatments and released more nitrate in acidification solution in most seasons but especially from winter soils (Figure 4). One likely explanation is that the chloride in our treatment solutions, which has greater affinity for soil anion exchange sites than nitrate, replaced nitrate on exchange sites and caused the leachate to become enriched with nitrate (Allred, 2007). The ion-exchange process is relatively fast and would be captured in our relatively short (minutes) experiment duration. These results are interesting in the context of reduced acid deposition, as, broadly speaking, the acidification treatments simulate conditions of the past, where precipitation and soil pH were lower and charge density was higher. At this time, streams were already impacted by substantial amounts of DIN from the atmosphere (Lawrence et al., 2020), but our results suggest that soil chemical conditions may have contributed to additional DIN release, exacerbating N concentrations in streams. In this case, shifts in acid deposition affected soil processes that influence both C and N mobilization in opposite directions, amplifying respective trends and contributing to further increases in C:N ratios. Another option might be that our experiments simply mobilized legacy nitrate that had accumulated from past deposition (or simply over winter) and is released when ions with higher affinity for soil exchange sites are added to the system. This process is worthwhile considering across other sites and we encourage the use of our data in combination with other data to assess C:N dynamics at a regional scale.
4.3. The connection between long-term data and experimental results
Our long-term data assessment offers insights into general annual and seasonal patterns, but the attribution of specific processes can be difficult. Our soil core experiments offer insights on specific processes. Together, these approaches investigate the combined effect of hydrological processes and shifts in precipitation composition.
For example, our soil core experiments on winter soils simulate hydrologic flushing via snowmelt, which is the most important hydrological event in these systems (Sebestyen et al., 2008; Shanley et al., 2015; Seybold et al., 2022). Winter soil leachate was particularly high in DOC, which is significant because this source is readily flushed to streams by snowmelt. Indeed, many studies show that consistent snowpack enhances DOC exports during spring snowmelt (Brooks and Williams, 1999; Brooks et al., 2011; Viglietti et al., 2014; Winnick et al., 2017) because soils are insulated, and in the absence of hydrologic events, accumulate DOC (Groffman et al., 2001). The fact that conditions of low acid deposition (recovery treatment) led to the highest liberation of DOC and DON from soils indicates that soil processes might have contributed to the long-term increase in stream DOC for decades (unfortunately we do not have access to long-term DON data). However, trend analyses by season do not indicate spring (or any other season) as primarily responsible for the observed increase in DOC (Supplementary Figure 3), suggesting that the effect of reduced acid deposition is consistent across seasons.
Our experimental results also provide insights into stream DIN responses. For example, high concentrations of DIN in leachate from winter soil cores suggest that accumulated N in winter soils contributes to high stream DIN concentrations during spring snowmelt (Figure 2). Further, though it is widely accepted that acidic atmospheric deposition enrich streams with DIN, high DIN concentrations in leachate from our acidification treatment (Figure 4) suggest that soil processes (e.g., ion exchange) contributed additional DIN at the beginning of the record. Thus, the subsequent decline in stream DIN concentrations we observed (Figure 3B) is likely the result of shifts in precipitation chemistry and the reduced contribution of soil-derived DIN. However, we want to emphasize that atmospheric deposition is only one driver for catchment N cycling (Lawrence et al., 2020) and that our experiments do not capture many aspects of the conditions during peak acid deposition (e.g., the more complex precipitation composition).
The connection between atmospheric inputs, soil processes and stream water response during times of high acid deposition and low acid deposition can be conceptualized into two main time periods and major soil processes (Figure 5). High charge density soil solution during high acid deposition contribute to soil aggregate stabilization and relatively less DOC release, however, ion exchange is promoted and based on affinity. In contrast, during decreased acid deposition, charge density in soils decreases and contributes to dispersion of soil aggregates that release DOC and DON, while ion exchange is less important.
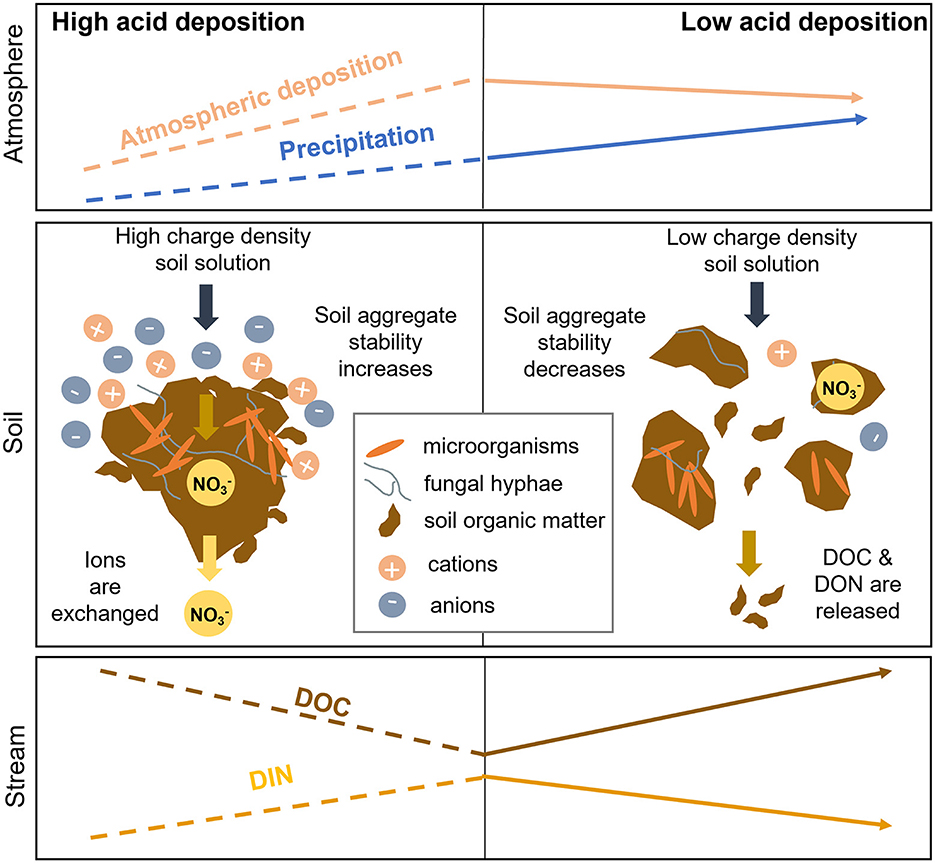
Figure 5. Conceptual model of the connection between atmospheric inputs (top panel), soil processes (middle panel) and stream water response (bottom panel) during times of high acid deposition (left panel) and low acid deposition (right panel). Data on atmospheric inputs and stream response during the onset of acid deposition are sparse and thus indicated by dashed lines, while decrease in acid deposition is well documented and trends are indicated with solid lines. During high acid deposition, high charge density of soil solution stabilizes soil aggregates and colloidal associations, but high ion concentration leads to ion exchange [exemplified for nitrate ()]. In contrast, low acid deposition leads to lower charge density of soil solution, causing aggregate destabilization and ensuing release of dissolved and colloidal dissolved organic matter and dissolved organic nitrogen [dissolved organic carbon (DOC) and dissolved organic nitrogen (DON)] from soil organic matter. DIN, dissolved inorganic nitrogen.
In this context, it is important to consider the limitations of this study. For example, although SRRW provides a high-frequency record of stream chemistry since 1990, the record still has gaps. Importantly, TDN was not measured prior to 2005 and therefore we cannot be certain how DON concentrations behaved during the first half of the record. Also, our soil core experiments only capture a single year of seasonal data, and our results are not representative of an entire season, but rather are a snapshot in time. Higher frequency sampling of soil cores would provide a more complete picture of seasonal soil dynamics.
Our long-term data analysis and soil core experiment demonstrate that the concurrent recovery from acid deposition and increase in heavy precipitation frequency affected C and N concentrations in opposing directions. This multi-decade synchronous increase in DOC concentrations and decrease in DIN has led to a progressive increase in stream C:N ratios at SRRW. Between 1997 and 2000, the C:N ratio averaged 9:1 (sd = 131), and in the period between 2015 and 2018 the ratio increased to an average 34:1 (sd = 163) (Figure 3D). The previous ratio is closer to the Redfield ratio (7:1) that is ideal for aquatic productivity (Kincaid et al., 2020), hence this shift might suggest a potential decrease in aquatic productivity and algal blooms. Indeed, in a meta study across latitudes, Rodríguez-Cardona et al. (2022) found that DOC:DON molar concentrations increased in about a third of the investigated 74 streams.
However, we also observed strong seasonal effects, and winter processes are particularly important because solutes accumulate in soil. The increasing number of rain-on-snow events and mid-winter thaws transport C and especially nitrate to streams. These results are consistent with another meta study (Seybold et al., 2022) that found disproportionate nitrate exports linked to winter events, thus surges and crashes of DIN can lead to punctuated drops in C:N albeit to general increases in these ratios at the annual scale. Together, the evolving C:N ratio and shifts in the total N pool in streams will likely have implications for stream metabolism and biogeochemical reaction rates that are difficult to predict (Wymore et al., 2015; Bernhardt et al., 2018; Rodríguez-Cardona et al., 2022).
5. Conclusions
Disentangling C and N responses to specific regional drivers (shifts in soil solution and/or hydrology) is a challenge, but catchment specific studies can provide insight on the coupled and intersecting pathways of C and N that influence C:N ratios. Specifically, the impact of shifts in soil pH and ionic strength on C and N mobility are dependent upon spatial dynamics (landscape position) and temporal patterns (seasonality and hydrology). Thus, C and N responses to reduced acid deposition may be superimposed on responses to increases in precipitation.
The interdependency of drivers can produce large variability of biogeochemical response across scales, but soil core experiments on nutrient liberation can provide insights on specific processes. Our results indicate that soils with high anion exchange capacities may be less resilient to precipitation extremes compared to soils with low anion exchange capacities.
Decreased acid deposition will not cause increases in DOC exports indefinitely, however, increased precipitation in the northeastern United States will likely continue to mobilize DOC at this watershed. Atmospheric deposition of N will continue to decrease, thus C:N ratios will continue to increase in general, which may counteract conditions for eutrophication and algal blooms in receiving water bodies.
Winter processes are particularly important, as solutes accumulate in soil during this season. Mid-winter thaws and rain-on-snow events are becoming more common, offering transport mechanisms of C and N species to streams. With disproportionate nitrate exports linked to winter events, and surges and crashes of DIN similar to those we observed at SRRW in 2014, subsequent punctuated drops in C:N ratios may become more frequent and support eutrophication in ways that will be difficult to predict. Such rapid changes in stoichiometry may have important consequences for aquatic communities and water quality in the future.
Data availability statement
The datasets presented in this study can be found in online repositories. The names of the repository/repositories and accession number(s) can be found below: The stream water dataset used for DOC, DIN, DON timeseries trends is available at https://doi.org/10.5066/P9380HQG. All experimental data are available on EarthChem https://doi.org/10.26022/IEDA/112721.
Author contributions
MR was responsible for sample collection, preparation, analyses, production of both figures, and tables as well as primary authorship. JP as Master's thesis advisor and project lead aided in interpretation, authorship, laboratory training, and allowed laboratory use. KU, ES, DK, JS, LL, and BS provided essential expertise to the interpretation of the results. All authors contributed to the article and approved the submitted version.
Funding
This work was supported by the National Science Foundation under Grant Nos. EAR2012123, EAR2012080, and EAR1724171.
Acknowledgments
The authors thank Megan Petrine, Lindsey Stinson, Bryan Stolzenburg, Dina Cordes, and Serena Matt who assisted with field and laboratory work. We thank Elliott Bloom, who assisted with data collection in lab and field and was instrumental in registering samples with SESAR and uploading data to EarthChem. We thank Marie English and Carol Adair's lab for providing laboratory space and guidance for sample analysis. Sleepers River infrastructure and data acquisition are supported by USGS Ecosystems Mission Area Climate Research and Development. Any use of trade, firm, or product names is for descriptive purposes only and does not imply endorsement by the U.S. Government.
Conflict of interest
The authors declare that the research was conducted in the absence of any commercial or financial relationships that could be construed as a potential conflict of interest.
Publisher's note
All claims expressed in this article are solely those of the authors and do not necessarily represent those of their affiliated organizations, or those of the publisher, the editors and the reviewers. Any product that may be evaluated in this article, or claim that may be made by its manufacturer, is not guaranteed or endorsed by the publisher.
Supplementary material
The Supplementary Material for this article can be found online at: https://www.frontiersin.org/articles/10.3389/frwa.2023.1065300/full#supplementary-material
References
Aber, J. D., McDowell, W., Nadelhoffer, K., Magill, A., and andBerntson, G. (1998). Nitrogen saturation in temperate forest ecosystems. BioScience 48, 921. doi: 10.2307/1313296
Adler, T., Underwood, K. L., Rizzo, D. M., Harpold, A., Sterle, G., Li, L., et al. (2021). Drivers of dissolved organic carbon mobilization from forested headwater catchments: a multi scaled approach. Front. Water 3, e578608. doi: 10.3389/frwa.2021.578608
Allred, B. J. (2007). Effects of nitrate concentration and ionic strength on nitrate anion exclusion under unsaturated flow conditions. Soil Sci. 172, 75. doi: 10.1097/ss.0b013e31814cee75
Armfield, J. R., Perdrial, J. N., Gagnon, A., Ehrenkranz, J., Perdrial, N., Cincotta, M., et al. (2019). Does stream water composition at sleepers river in vermont reflect dynamic changes in soils during recovery from acidification? Front. Earth Sci. 6, e00246. doi: 10.3389/feart.2018.00246
Aufdenkampe, A. K., Mayorga, E., Raymond, P. A., Melack, J. M., Doney, S. C., Alin, S. R., et al. (2011). Riverine coupling of biogeochemical cycles between land, oceans, and atmosphere. Front. Ecol. Environ. 9, 53. doi: 10.1890/100014
Berner, E. K., and Berner, R. A. (2012). Global Environment. Water, Air and Geochemical Cycles. Princeton; Oxford: Princeton University Press.
Bernhardt, E. S., Blaszczak, J. R., Ficken, C. D., Fork, M. L., Kaiser, K. E., Seybold, C. E., et al. (2017). Control points in ecosystems: moving beyond the hot spot hot moment concept. Ecosystems 20, 665–682. doi: 10.1007/s10021-016-0103-y
Bernhardt, E. S., Heffernan, J. B., Grimm, N. B., Stanley, E. H., Harvey, J. W., Arroita, M., et al. (2018). The metabolic regimes of flowing waters. Limnol. Oceanogr. 63, S99–S118. doi: 10.1002/lno.10726
Boyer, E. W., Hornberger, G. M., Bencala, K. E., and McKnight, M. D. (1997). Response characteristics of DOC flushing in an alpine catchment. Hydrol. Proces. 11, 1635–1647. doi: 10.1002/(SICI)1099-1085(19971015)11:12andlt;1635::AID-HYP494andgt;3.0.CO;2-H
Bristol, C. (2021). Assessing The Impact Of Changes In Acid Deposition On Dissolved Organic Carbon Mobilization From Two Forested Headwater Catchments: A Combined Lab And Field Study. Burlington, VT: University of Vermont.
Brooks, P. D., Grogan, P., Templer, P. H., Groffman, P., Öquist, M. G., Schimel, J., et al. (2011). Carbon and nitrogen cycling in snow-covered environments. Geogr. Compass 5, 682–699. doi: 10.1111/j.1749-8198.2011.00420.x
Brooks, P. D., and Williams, M. W. (1999). Snowpack controls on nitrogen cycling and export in seasonally snow-covered catchments. Hydrol. Proces. 13, 2177–2190. doi: 10.1002/(SICI)1099-1085(199910)13:14/15<2177::AID-HYP850>3.0.CO;2-V
Brooks, P. D., Williams, M. W., and Schmidt, K. S. (1998). Inorganic nitrogen and microbial biomass dynamics before and during spring snowmelt. Biogeochemistry 43, 1–15. doi: 10.1023/A:1005947511910
Campbell, J. L., Mitchell, M. J., and Mayer, B. (2006). Isotopic assessment of NO3– and SO42– mobility during winter in two adjacent watersheds in the Adirondack Mountains. J. Geophys. Res. Atmosp. 111, 208. doi: 10.1029/2006JG000208
Caretta, M. A., Mukherji, A., Arfanuzzaman, M., Betts, R. A., Gelfan, A., Hirabayashi, Y., et al. (2022). “Water,” in Climate Change 2022: Impacts, Adaptation and Vulnerability, eds H.-O. Pörtner, D. C. Roberts, M. Tignor, E. S. Poloczanska, K. Mintenbeck, A. Alegría, M. Craig, S. Langsdorf, S. Löschke, V. Möller, A. Okem, B. Rama Cambridge (New York, NY: IPPC), 551–712.
Casson, N. J., Eimers, M. C., and Watmough, A. S. (2014). Sources of nitrate export during rain-on-snow events at forested catchments. Biogeochemistry 120, 23–36. doi: 10.1007/s10533-013-9850-4
Casson, N. J., Eimers, M. C., Watmough, S. A., and Richardson, C. M. (2019). The role of wetland coverage within the near-stream zone in predicting of seasonal stream export chemistry from forested headwater catchments. Hydrol. Proces. 33, 1465–1475. doi: 10.1002/hyp.13413
Chalmers, A. T., Shanley, J. B., Denner, J., and Clark, F. S. (2019). Snow Depth and Snow Water Equivalents at Sleepers River Research Watershed. Danville, VT: U. S. G. Survey.
Cincotta, M. M., Perdrial, J. N., Shavitz, A., Libenson, A., Landsman-Gerjoi, M., Perdrial, N., et al. (2019). Soil aggregates as a source of dissolved organic carbon to streams: an experimental study on the effect of solution chemistry on water extractable carbon. Front. Environ. Sci. 7, 172. doi: 10.3389/fenvs.2019.00172
Clark, J. M., Bottrell, S. H., Evans, C. D., Monteith, D. T., Bartlett, R., Rose, R., et al. (2010). The importance of the relationship between scale and process in understanding long-term DOC dynamics. Sci. Total Environ. 408, 2768–2775. doi: 10.1016/j.scitotenv.2010.02.046
Eimers, M. C., Watmough, S. A., Buttle, J. M., and Dillon, J. P. (2008a). Examination of the potential relationship between droughts, sulphate and dissolved organic carbon at a wetland-draining stream. Global Change Biol. 14, 938–948. doi: 10.1111/j.1365-2486.2007.01530.x
Eimers, M. C., Watmough, S. A., and Buttle, M. J. (2008b). Long-term trends in dissolved organic carbon concentration: a cautionary note. Biogeochemistry 87, 71–81. doi: 10.1007/s10533-007-9168-1
Eshleman, K. N., Sabo, R. D., and Kline, M. K. (2013). Surface water quality is improving due to declining atmospheric N deposition. Environ. Sci. Technol. 47, 12193–12200. doi: 10.1021/es4028748
Evans, C. D., Monteith, D. T., and Cooper, M. D. (2005). Long-term increases in surface water dissolved organic carbon: Observations, possible causes and environmental impacts. Environ. ion 137, 55–71. doi: 10.1016/j.envpol.2004.12.031
Fenn, M. E., Poth, M., Aber, J., Baron, J., and Bormann, B. (1998). Nitrogen excess in North American ecosystems: predisposing factors, ecosystem responses and management strategies. Ecolog. Applicat. 8, 706. doi: 10.1890/1051-0761(1998)008[0706:NEINAE]2.0.CO;2
Freeman, C., Evans, C. D., Monteith, D. T., Reynolds, B., and Fenner, N. (2001). Export of organic carbon from peat soils. Nature 412, 785–785. doi: 10.1038/35090628
Frei, R. J., Lawson, G. M., Norris, A. J., Cano, G., Vargas, M. C., Kujanp,ää, E., et al. (2021). Limited progress in nutrient pollution in the U.S. caused by spatially persistent nutrient sources. PLoS ONE 16, e0258952. doi: 10.1371/journal.pone.0258952
Galford, G. L., Hoogenboom, A., Carlson, S., Ford, S., Nash, J., Palchak, E., et al. (2014). Considering Vermont's Future in a Changing Climate: The First Vermont Climate Assessment. Burlington, VT: Gund Institute for Ecological Economics.
Goodale, C. L., Thomas, S. A., Fredriksen, G., Elliott, E. M., Flinn, K. M., Butler, T. J., et al. (2009). Unusual seasonal patterns and inferred processes of nitrogen retention in forested headwaters of the Upper Susquehanna River. Biogeochemistry 93, 197–218. doi: 10.1007/s10533-009-9298-8
Groffman, P. M., Driscoll, C. T., Fahey, T. J., Hardy, J. P., Fitzhugh, R. D., Tierney, L. G., et al. (2001). Colder soils in a warmer world: a snow manipulation study in a northern hardwood forest ecosystem. Biogeochemistry 56, 135–150. doi: 10.1023/A:1013039830323
Hayhoe, K., Wake, C. P., Huntington, T. G., Luo, L., Schwartz, M., Sheffield, J., et al. (2007). Past and future changes in climate and hydrological indicators in the U.S. Northeast. Clim. Dynam. 28, 381–407. doi: 10.1007/s00382-006-0187-8
Helsel, D. R., Hirsch, R. M., Ryberg, K. R., Archfield, S. A., and Gilroy, J. E. (2020). Statistical methods in water resources. U.S. geological survey techniques and methods. Book 4, 458. doi: 10.3133/tm4A3
Hirsch, R. M., and Slack, J. R. (1984). A nonparametric trend test for seasonal data with serial dependence. Water Resour. Res. 20, 727–732. doi: 10.1029/WR020i006p00727
Hungat, B. A., Dukes, J., Shaw, M., Luo, Y., and Field, C. (2003). Nitrogen and climate change. Science 302, 1512. doi: 10.1126/science.1091390
Huntington, T. G., and Shanley, J. B. (2022). A systematic increase in the slope of the concentration discharge relation for dissolved organic carbon in a forested catchment in Vermont, USA. Sci. Total Environ. 844, 156954. doi: 10.1016/j.scitotenv.2022.156954
Il Jeong, D., and Sushama, L. (2018). Rain-on-snow events over North America based on two Canadian regional climate models. Clim. Dynam. 50, 303–316. doi: 10.1007/s00382-017-3609-x
Kincaid, D. W., Seybold, E. C., Adair, E. C., Bowden, W. B., Perdrial, J. N., Vaughan, M. C. H., et al. (2020). Land use and season influence event-scale nitrate and soluble reactive phosphorus exports and export stoichiometry from headwater catchments. Water Resour. Res. 56, e2020WR.027361. doi: 10.1029/2020WR027361
Lawrence, G. B., Scanga, S. E., and Sabo, D. R. (2020). Recovery of Soils From Acidic Deposition May Exacerbate Nitrogen Export From Forested Watersheds. J. Geophys. Res. Biogeosci. 125, e2019JG.005036. doi: 10.1029/2019JG005036
Lepistö, A., Kortelainen, P., and Mattsson, T. (2008). Increased organic C and N leaching in a northern boreal river basin in Finland. Global Biogeochem. Cycles 22, 3175. doi: 10.1029/2007GB003175
Li, L., Stewart, B., Zhi, W., Sadayappan, K., Ramesh, S., Kerins, D., et al. (2022). Climate controls on river chemistry. Earth's Future. 10, e2021ED002603. doi: 10.1029/2021EF002603
Liu, M., Han, G., and Zhang, Q. (2019). Effects of soil aggregate stability on soil organic carbon and nitrogen under land use change in an erodible region in Southwest China. Int. J. Environ. Res. Public Health 16, 3809. doi: 10.3390/ijerph16203809
Loecke, T. D., Burgin, A. J., Riveros-Iregui, D. A., Ward, A. S., Thomas, S. A., Davis, C. A., et al. (2017). Weather whiplash in agricultural regions drives deterioration of water quality. Biogeochemistry 133, 7–15. doi: 10.1007/s10533-017-0315-z
Matt, S., Shanley, J. B., Chalmers, A. T., Sebestyen, S. D., Merriam, J. L., Bailey, S. W., et al. (2021). Aqueous Chemistry Database, Sleepers River Research Watershed, Danville, Vermont, 1991-2018. Vermont, VT: U.S. G. Survey data release. doi: 10.5066/P9380HQG
McClain, M. E. B. E., Dent, C. L., Gergel, S. E., Grimm, N. B., Groffman, P. M., Hart, S. C., et al. (2003). Biogeochemical hot spots and hot moments at the interface of terrestrial and aquatic ecosystems. Ecosystems 6, 301–312. doi: 10.1007/s10021-003-0161-9
McNeill, A., and Unkovich, M. (2007). “The nitrogen cycle in terrestrial ecosystems,” in Nutrient Cycling in Terrestrial Ecosystems, eds P. Marschner and Z. Rengel (Springer Berlin Heidelberg), 37–64.
Monteith, D. T., Stoddard, J. L., Evans, C. D., de Wit, H. A., Forsius, M., Hogasen, T., et al. (2007). Dissolved organic carbon trends resulting from changes in atmospheric deposition chemistry. Nature 450, 537–540. doi: 10.1038/nature06316
Murray, D. S., Shattuck, M. D., McDowell, W. H., and Wymore, S. A. (2022). Nitrogen wet deposition stoichiometry: the role of organic nitrogen, seasonality, and snow. Biogeochemistry 160, 301–314. doi: 10.1007/s10533-022-00966-0
Pellerin, B. A., Saraceno, J. F., Shanley, J. B., Sebestyen, S. D., Aiken, G. R., Wollheim, W. M., et al. (2011). Taking the pulse of snowmelt: in situ sensors reveal seasonal, event and diurnal patterns of nitrate and dissolved organic matter variability in an upland forest stream. Biogeochemistry 108, 183–198. doi: 10.1007/s10533-011-9589-8
Perdrial, J., McIntosh, J., Harpold, A., Brooks, P. D., Zapata-Rios, X., Ray, J., et al. (2014). Stream water carbon controls in seasonally snow-covered mountain catchments: impact of inter-annual variability of water fluxes, catchment aspect and seasonal processes. Biogeochemistry 118, 273–290. doi: 10.1007/s10533-013-9929-y
Pettitt, A. (1979). A non-parametric approach to the change-point problem. J. R. Stat. Soc. Series C (Appl. Statist.) 28, 126–135. doi: 10.2307/2346729
Raymond, P. A., and Saiers, J. E. (2010). Event controlled DOC export from forested watersheds. Biogeochemistry 100, 197–209. doi: 10.1007/s10533-010-9416-7
Rodríguez-Cardona, B. M., Wymore, A. S., Argerich, A., Barnes, R. T., Bernal, S., Brookshire, E. N. J., et al. (2022). Shifting stoichiometry: long-term trends in stream-dissolved organic matter reveal altered C:N ratios due to history of atmospheric acid deposition. Global Change Biol. 28, 98–114. doi: 10.1111/gcb.15965
Rupp, H., Tauchnitz, N., and Meissner, R. (2021). The effects of soil drying out and rewetting on nitrogen and carbon leaching—results of a long-term lysimeter experiment. Water. 13, 2601. doi: 10.3390/w13182601
Ryan, K. A., Adler, T., Chalmers, A., Perdrial, J., Shanley, J. B., Stubbins, A., et al. (2021). Event scale relationships of DOC and TDN fluxes in throughfall and stemflow diverge from stream exports in a forested catchment. J. Geophys. Res. Biogeosci. 126, e2021JG.006281. doi: 10.1029/2021JG006281
Ryan, K. A., Adler, T., Chalmers, A. T., Perdrial, J., Sebestyen, S., Shanley, J. B., et al. (2022). Optical properties of dissolved organic matter in throughfall and stemflow vary across tree species and season in a temperate headwater forest. Biogeochemistry. doi: 10.1007/s10533-022-00985-x
Schlesinger, W. H., and Melack, J. M. (1981). Transport of organic carbon in the world's rivers. Tellus 33, 172–187. doi: 10.1111/j.2153-3490.1981.tb01742.x
Sebestyen, S. D., Boyer, E. W., Shanley, J. B., Kendall, C., Doctor, D. H., Aiken, G. R., et al. (2008). Sources, transformations, and hydrological processes that control stream nitrate and dissolved organic matter concentrations during snowmelt in an upland forest. Water Resour. Res. 44, W12410. doi: 10.1029/2008WR006983
Seybold, E., Dwivedi, R., Musselman, K., Kincaid, D., Schroth, A., Classen, A., et al. (2022). Winter runoff events pose an unquantified continental-scale risk of high wintertime nutrient export. Environ. Res. Lett. 17, 104044. doi: 10.1088/1748-9326/ac8be5
Shanley, J. B., Chalmers, A. T., Denner, J. C., and Clark, F. S. (2021). Five-minute Discharge; Daily Precipitation, Stream Runoff, and Maximum and Minimum Air Temperature; and Annual Precipitation and Runoff for W-9 Catchment, Sleepers River Research Watershed near Danville, Vermont, 1991-2018. Vermont, VT: U. S. G. Survey.
Shanley, J. B., Chalmers, A. T., Denner, J. C., Clark, S. F., Sebestyen, S. D., Matt, S., et al. (2022). Hydrology and biogeochemistry datasets from Sleepers River Research Watershed, Danville, Vermont, USA. Hydrol. Proces. 36, e14495. doi: 10.1002/hyp.14495
Shanley, J. B., Kram, P., Hruska, J., and Bullen, D. T. (2004). A biogeochemical comparison of two well-buffered catchments with contrasting histories of acid deposition. Water Air Soil Pollut.: Focus 4, 325–342. doi: 10.1023/B:WAFO.0000028363.48348.a4
Shanley, J. B., Sebestyen, S. D., McDonnell, J. J., McGlynn, B. L., and Dunne, T. (2015). Water's way at sleepers river watershed – revisiting flow generation in a post-glacial landscape, Vermont USA. Hydrol. Proces. 29, 3447–3459. doi: 10.1002/hyp.10377
Smith, K. A., Ball, T., Conen, F., Dobbie, K. E., Massheder, J., Rey, A., et al. (2003). Exchange of greenhouse gases between soil and atmosphere: interactions of soil physical factors and biological processes. Eur. J. Soil Sci. 54, 779. doi: 10.1046/j.1351-0754.2003.0567.x
Stewart, B., Shanley, J. B., Kirchner, J. W., Norris, D., Adler, T., Bristol, C., et al. (2022). Streams as mirrors: reading subsurface water chemistry from stream chemistry. Water Resour. Res. 58, e2021WR.029931. doi: 10.1029/2021WR029931
U.S. Drought Monitor. (2023). Northeast Percent Area in U.S. Drought Monitor Categories. Time Series | U.S. Drought Monitor. Available online at: https://droughtmonitor.unl.edu/DmData/TimeSeries.aspx (accessed February 28, 2023).
Viglietti, D., Freppaz, M., Filippa, G., and Zanini, E. (2014). Soil C and N response to changes in winter precipitation in a subalpine forest ecosystem, NW Italy. Hydrol. Proces. 28, 5309–5321. doi: 10.1002/hyp.10008
Wen, H., Perdrial, J., Abbott, B. W., Bernal, S., Dupas, R., Godsey, S. E., et al. (2020). Temperature controls production but hydrology regulates export of dissolved organic carbon at the catchment scale. Hydrol. Earth Syst. Sci. 24, 945–966. doi: 10.5194/hess-24-945-2020
Wilson, H. F., Saiers, J. E., Raymond, P. A., and Sobczak, V. W. (2013). Hydrologic drivers and seasonality of dissolved organic carbon concentration, nitrogen content, bioavailability, and export in a forested New England Stream. Ecosystems 16, 604–616. doi: 10.1007/s10021-013-9635-6
Winnick, M. J., Carroll, R. W. H., Williams, K. H., Maxwell, R. M., Dong, W., Maher, K., et al. (2017). Snowmelt controls on concentration-discharge relationships and the balance of oxidative and acid-base weathering fluxes in an alpine catchment, East River. Colorado. 53, 2507–2523. doi: 10.1002/2016WR019724
Worrall, F., Ron, H., Evans, C. D., Watts, C. D., Adamson, J., Neal, C., et al. (2004). Trends in dissolved organic carbon in UK rivers and lakes. Biogeochemistry 70, 369–402. doi: 10.1007/s10533-004-8131-7
Wymore, A. S., Fazekas, H. M., and McDowell, H. W. (2021). Quantifying the frequency of synchronous carbon and nitrogen export to the river network. Biogeochemistry 152, 1–12. doi: 10.1007/s10533-020-00741-z
Wymore, A. S., Rodríguez-Cardona, B., and McDowell, H. W. (2015). Direct response of dissolved organic nitrogen to nitrate availability in headwater streams. Biogeochemistry 126, 1–10. doi: 10.1007/s10533-015-0153-9
Zhang, S., Wang, R., Yang, X., Sun, B., and Li, Q. (2016). Soil aggregation and aggregating agents as affected by long term contrasting management of an Anthrosol. Sci. Rep. 6, 39107. doi: 10.1038/srep39107
Keywords: carbon, nitrogen, stoichiometry, overlapping drivers, long-term data, experiments, stream, soil
Citation: Ruckhaus M, Seybold EC, Underwood KL, Stewart B, Kincaid DW, Shanley JB, Li L and Perdrial JN (2023) Disentangling the responses of dissolved organic carbon and nitrogen concentrations to overlapping drivers in a northeastern United States forested watershed. Front. Water 5:1065300. doi: 10.3389/frwa.2023.1065300
Received: 09 October 2022; Accepted: 20 February 2023;
Published: 13 March 2023.
Edited by:
Paul D. Wagner, University of Kiel, GermanyReviewed by:
Alissa M. White, University of Montana, United StatesRichard James Cooper, University of East Anglia, United Kingdom
Copyright © 2023 Ruckhaus, Seybold, Underwood, Stewart, Kincaid, Shanley, Li and Perdrial. This is an open-access article distributed under the terms of the Creative Commons Attribution License (CC BY). The use, distribution or reproduction in other forums is permitted, provided the original author(s) and the copyright owner(s) are credited and that the original publication in this journal is cited, in accordance with accepted academic practice. No use, distribution or reproduction is permitted which does not comply with these terms.
*Correspondence: Julia N. Perdrial, SnVsaWEuUGVyZHJpYWxAdXZtLmVkdQ==; Manya Ruckhaus, bXJ1Y2toYXVzMUBnbWFpbC5jb20=
†ORCID: Erin C. Seybold orcid.org/0000-0002-0365-2333
Kristen L. Underwood orcid.org/0000-0003-3008-3057
Bryn Stewart orcid.org/0000-0002-3199-0129
Dustin W. Kincaid orcid.org/0000-0003-1640-685X
James B. Shanley orcid.org/0000-0002-4234-3437
Li Li orcid.org/0000-0002-1641-3710