- 1U. S. Geological Survey, Water Resources Mission Area, Boulder, CO, United States
- 2U. S. Geological Survey, California Water Science Center, Sacramento, CA, United States
- 3U. S. Geological Survey, Oregon Water Science Center, Portland, OR, United States
- 4U. S. Geological Survey, Colorado Water Science Center, Lakewood, CO, United States
- 5U. S. Geological Survey, New Mexico Water Science Center, Albuquerque, NM, United States
- 6U. S. Geological Survey, Wyoming-Montana Water Science Center, Helena, MT, United States
- 7U. S. Geological Survey, Water Resources Mission Area, Reston, VA, United States
- 8U. S. Geological Survey, Kansas Water Science Center, Lawrence, KS, United States
- 9U. S. Geological Survey, Water Resources Mission Area, Burlington, VT, United States
Wildfires pose a risk to water supplies in the western U.S. and many other parts of the world, due to the potential for degradation of water quality. However, a lack of adequate data hinders prediction and assessment of post-wildfire impacts and recovery. The dearth of such data is related to lack of funding for monitoring extreme events and the challenge of measuring the outsized hydrologic and erosive response after wildfire. Assessment and prediction of post-wildfire surface water quality would be strengthened by the strategic monitoring of key parameters, and the selection of sampling locations based on the following criteria: (1) streamgage with pre-wildfire data; (2) ability to install equipment that can measure water quality at high temporal resolution, with a focus on storm sampling; (3) minimum of 10% drainage area burned at moderate to high severity; (4) lack of major water management; (5) high-frequency precipitation; and (6) availability of pre-wildfire water-quality data and (or) water-quality data from a comparable unburned basin. Water-quality data focused on parameters that are critical to human and (or) ecosystem health, relevant to water-treatment processes and drinking-water quality, and (or) inform the role of precipitation and discharge on flow paths and water quality are most useful. We discuss strategic post-wildfire water-quality monitoring and identify opportunities for advancing assessment and prediction. Improved estimates of the magnitude, timing, and duration of post-wildfire effects on water quality would aid the water resources community prepare for and mitigate against impacts to water supplies.
1. Introduction
Wildfires are a natural process in many ecosystems, but their size and severity have increased substantially over the past few decades (Juang et al., 2022; Shuman et al., 2022) and are now widely recognized as a critical risk to water supplies in the western U.S. and many other parts of the world (Bryson, 2021; Fountain, 2021; Nilsen, 2022). Wildfires can lead to increased runoff, erosion, and conveyance of sediment, ash, pollutants, and debris to surface water, resulting in decreased water quality, loss of reservoir storage capacity, stream habitat degradation, and increased treatment costs for drinking water providers (Emelko et al., 2011; Smith et al., 2011; Sham et al., 2013; Bladon et al., 2014; Martin, 2016; Rhoades et al., 2019b). The range of water-quality effects, however, has varied widely, from no noticeable change to orders of magnitude increases in concentrations and yields of sediment, dissolved organic carbon (DOC), nitrate, phosphorus, metals, and other constituents (Rust et al., 2018; Paul et al., 2022). Water providers, reservoir operators, land managers, and emergency response agencies would benefit from improved assessment and prediction of the character, magnitude, and duration of water-quality impacts after wildfire in watersheds across a wide range of ecoregions. A lack of adequate pre- and post-wildfire water-quality data hinders model calibration and adaptation (Basso et al., 2022), assessment of post-wildfire recovery (Hampton et al., 2022), and understanding how wildfires will affect regulatory requirements (Paul et al., 2022). Here we describe a path forward for strategic, consistent post-wildfire water-quality data collection for surface water that, when deployed across a range of ecosystems, will lead to vastly improved assessment and prediction of impacts of wildfire on water supplies.
2. Gaps and barriers in existing post-wildfire water-quality data
Post-wildfire water-quality studies in the past, when conducted, have typically been performed on an ad-hoc, inconsistent basis driven by a local need, available funding, and (or) a particular research question. Therefore, data sets with consistent objectives, approaches, and constituents are rare. The dearth of consistent data is largely related to the challenges in obtaining funding to study rare events (Smith et al., 2011; Patrick et al., 2022) and in measuring the often outsized hydrologic and erosive response to post-wildfire runoff and flooding. Funds related to wildfires were long focused on immediate risk to life and property, with water supplies only recently gaining public attention (Bladon et al., 2014; Martin, 2016; Robinne et al., 2021). The most severe water-quality impacts are often delayed until subsequent high-intensity rainstorms, which can occur months to years after the wildfire (Murphy et al., 2015), by which time funding opportunities and public attention have diminished. Evaluating the effects of wildfires is complicated by a general lack of stream discharge and water-quality data in forested headwaters (Bishop et al., 2008; Krabbenhoft et al., 2022) to provide a pre-wildfire comparison. In addition, because wildfires can reduce the threshold precipitation intensity at which overland flow occurs, post-wildfire stream discharge and sediment concentrations can be orders of magnitude greater than they would have been for similar storms pre-wildfire (Wondzell and King, 2003; Murphy et al., 2015), which poses challenges to current monitoring capabilities. As a result of these challenges, there are numerous gaps in post-wildfire water-quality data (Yu and Cheng, 2008; Rust et al., 2018; Basso et al., 2022; Hampton et al., 2022; Paul et al., 2022; Robinne et al., 2022; Raoelison et al., 2023), as shown in Figure 1.
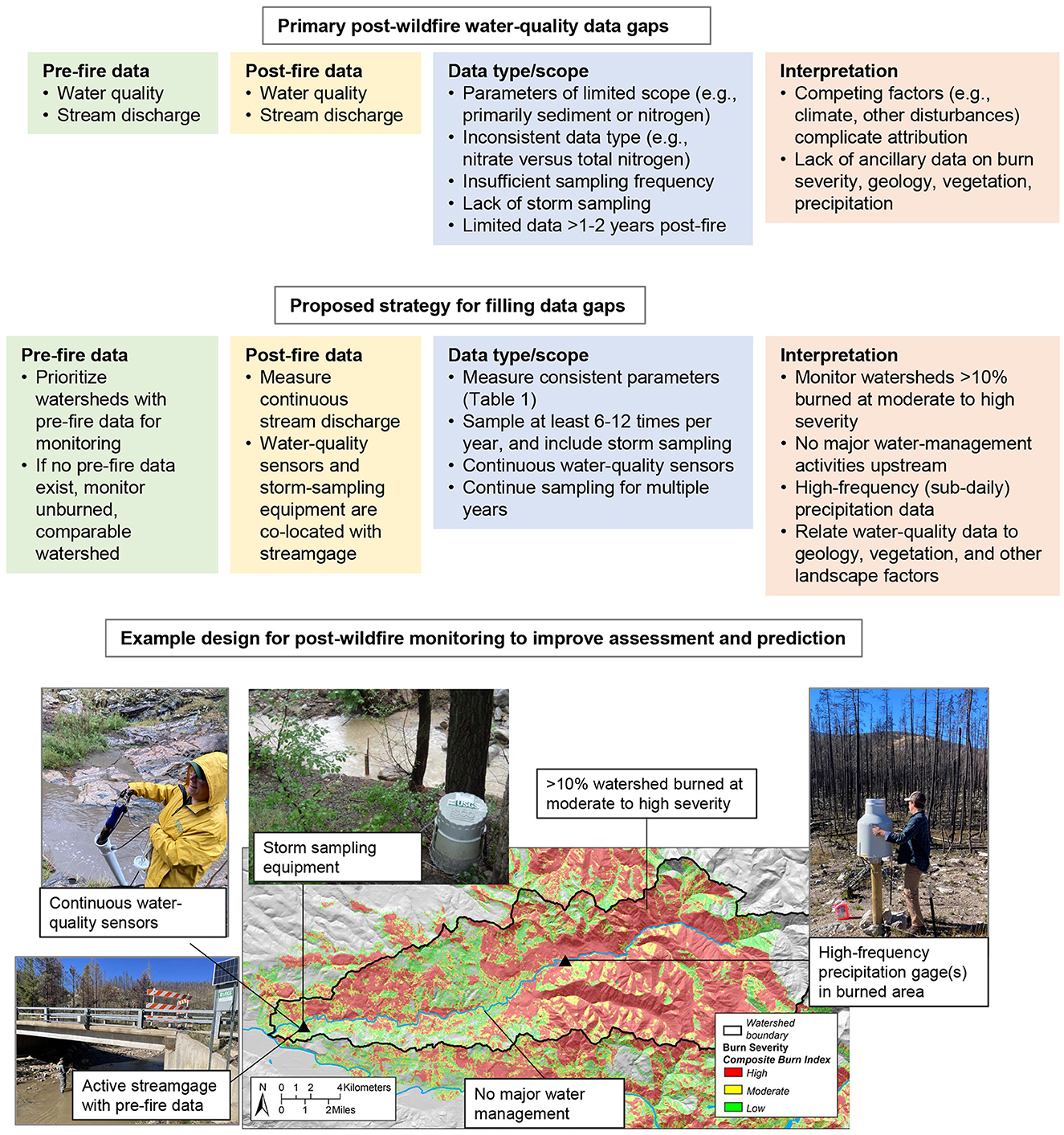
Figure 1. Post-wildfire water-quality gaps and proposed gap-filling strategy. [Composite burn index (Key and Benson, 2006) rates burn severity for substrates (litter, duff, fuel, and soil) and vegetation on a continuous scale from 0 (unburned) to 3 (high burn severity). Map prepared by Max Schwid; photographs by Sheila Murphy and Mike Kohn].
Data-related gaps highlighted in Figure 1 result in a lack of consistent data needed for the development of models and assessments that could provide actionable guidance for water-supply agencies that is broadly applicable across regions. Data consistency issues also hinder comparisons between different studies, and derived estimates of the magnitude, timing, and duration of post-wildfire effects on water quality have high uncertainty.
3. Proposed strategy
Closing the aforementioned water-quality data gaps requires strategic monitoring to produce consistent, unified datasets. Optimal collection of these new datasets involves careful selection of sampling locations and measurement of consistent parameters at similar temporal scales. We present the following strategy for selecting locations and methodologies for data collection that enable identification of regional insights into effects of wildfire on surface water quality.
3.1. Monitoring locations
Post-wildfire water-quality data are needed from sites with a diversity in climate, land use, geology, and vegetation to build a foundation for discerning regional differences in effects on hydrogeochemical and ecological systems. With hundreds of wildfires burning U.S. watersheds each year, it would be impossible to monitor every stream within or downstream of a burned area. Selection of monitoring locations based on the following optimal criteria would strengthen assessment and prediction of post-wildfire water quality (Figure 1):
• Active (or recently active) streamgage with pre-fire data. Discharge is critical to understanding water quality. Ideally, the site would have a stream discharge record longer than 10 years, collected within the last 30 years, to allow separation of climate variability from wildfire impacts.
• Continuous water-quality sensors and storm-sampling equipment can be co-located with streamgage and can be accessed frequently. Due to the critical role that storm events play in the transport of post-wildfire ash and sediment, high-frequency data collection that covers all ranges of stream discharge is most useful. It must be possible to access the equipment safely in all weather conditions.
• At least 10% of watershed is burned at moderate to high severity. Many studies have indicated that forest disturbances affect watershed response, and a range of disturbance thresholds has been reported in the literature. For example, some studies documented effects on annual water yield when 20% or more of a watershed is disturbed (Bosch and Hewlett, 1982; Wine and Cadol, 2016; Hallema et al., 2018); other work has reported that as little as 7–10% of watershed disturbance may cause a hydrologic effect (Buma and Livneh, 2017; Beyene et al., 2021). The proposed minimum percentage of the watershed burned at moderate to high severity would allow us to identify thresholds for post-wildfire water-quality effects across a range of constituents and ecoregions.
• Watershed has no major water-management activities upstream of the monitoring location. Water management, including diversions, reservoirs, and wastewater plants, can obscure wildfire impacts to stream discharge or water quality. While monitoring wildfire-affected basins with water resources management would be beneficial to provide critical operational guidance to water providers, active pre-wildfire or post-wildfire water management can make data less useful for model and assessment development.
• High-frequency (sub-daily) precipitation data can be obtained within the burned area. These data would allow correlation of water quality with hydrologic drivers, such as rainfall intensity (Murphy et al., 2015), and comparison to pre-wildfire rainfall-runoff response. Precipitation frequency estimates (Perica et al., 2013), and (or) gridded data, such as PRISM (Daly et al., 1994) or Daymet (Thornton et al., 2012) may be used to guide precipitation gage locations and density. However, gridded data usually lack sufficient temporal resolution and are less accurate in areas of steep mountains and lack of precipitation gages (Murphy et al., 2017), which is a common scenario in burned forests. On-the-ground precipitation monitoring can also fill gaps in weather radar or better capture high-intensity rainfall (e.g., Nikolopoulos et al., 2015; Bernard and Gregoretti, 2021).
• Pre-wildfire water-quality data and (or) water-quality data from a comparable unburned basin are available. Pre-wildfire water-quality data, collected for many years and across a range of stream discharge, would best enable the separation of the effect of wildfire from other climate or landscape factors. However, these data are scarce (Rust et al., 2018; Raoelison et al., 2023), and requiring such data would eliminate most watersheds from selection. Water-quality data collected on the same stream, upstream from the burned area, and (or) from a nearby, unburned watershed with similar landscape (land cover, geology, vegetation, etc.) and climate can also aid in the interpretation of post-wildfire effects (Hewlett, 1971; Rice, 1979).
3.2. Monitoring design and frequency
A combination of continuous in-stream sensors and water-quality samples (across a range of discharge values) is needed to capture the full range of post-wildfire response and to facilitate diagnosing connections and dependencies between watershed processes and constituent fate and transport. In-stream sensors can provide high-frequency data (depending on local precipitation regime, 1–15-min measurement intervals may be needed) for certain constituents of interest, are valuable for capturing transient events, and require minimal field staff. Water-quality samples are needed to capture a broader range of parameters, and to correlate with and correct sensor data; for example, fluorescent dissolved organic matter measurements must be corrected for interference in highly turbid waters (Saraceno et al., 2017). Storm sampling with automatic samplers (triggered by water level and (or) turbidity) allows the collection of water samples during high-flow events that may be too dangerous or short-lived to collect with routine sampling, and can capture very turbid events, when sensors can be damaged and (or) monitoring capabilities can be exceeded. Following best practices for water-quality sample collection and processing, such as the U.S. Geological Survey's National Field Manual for the Collection of Water-Quality Data (U. S. Geological Survey, 2018), ensures site intercomparability. Hydrologic and geomorphic response can be amplified after wildfire; thus, the use of rugged, secure, non-contact, and (or) inexpensive monitoring equipment can reduce the financial risk of equipment damage or loss. Rust et al. (2018) advocated for collection of post-wildfire water-quality data at a frequency of at least six times per year and ideally 12 times per year; we agree that 12 samples per year, across a range of hydrologic conditions, is an ideal minimum value, but we believe that storm sampling is critical, with at least 30–50% of the samples collected during storm events. Perennial streams generally have greater relevance to water supply, and thus would serve as an initial focus.
Ideally, monitoring stream discharge and water quality would begin immediately after wildfire and continue for many years. Magnitudes of response for certain constituents of concern, such as nitrate, DOC, and some metals are typically greatest in the initial rainstorms (“first flush”) following wildfire containment; thus, depending on the timing of wildfire and seasonality of precipitation in the ecoregion, equipment deployment as soon as possible after the wildfire provides the best opportunity to capture initial responses. However, we acknowledge the immense challenge this can pose because of difficulty in obtaining appropriate access and funding after wildfire (Smith et al., 2011; Patrick et al., 2022). Post-wildfire hydrologic and water-quality effects tend to be greatest in the first 3 years after wildfire, though in some regions debris flow risk (and thus sediment transport) may increase 5–10 years post-wildfire due to root decomposition (Wondzell and King, 2003). Very few studies have monitored post-wildfire water quality for >5 years (Rust et al., 2018; Hampton et al., 2022). Thus, maintaining post-wildfire water-quality monitoring for longer periods would allow us to improve our ability to assess “recovery,” which will vary by constituent (Minshall et al., 1989; Silins et al., 2009; Emelko et al., 2016; Rhoades et al., 2019a) and ecoregion.
3.3. Water-quality parameters and relevant measurements
The most useful water-quality parameters to measure after wildfire are those that are critical to human and (or) ecosystem health, relevant to water-treatment processes and drinking-water quality, have a watershed-specific regulatory requirement, and (or) inform the role of precipitation and discharge on flow paths and water quality. Parameter selection must balance the collection of essential data with fiscal and practical constraints; therefore, we have divided these parameters into two tiers. Tier one parameters are considered the highest priority for assessing and modeling impacts of wildfire on water quality; tier two parameters would provide additional information relevant to water treatment, aquatic life, and (or) flow paths and lay the groundwork for next-generation modeling capabilities, but substantially increase monitoring costs.
Additional parameters not listed in (Table 1) may also be desired due to local circumstances and (or) stakeholder needs, or to inform specific types of models, but can be cost-prohibitive. Parameters that could inform the evaluation of water-quality and ecosystem impacts include organic contaminants (e.g., polycyclic aromatic hydrocarbons [PAHs]), and other compounds that may be present in the wildland/urban interface (Paul et al., 2022). In addition to changes in DOC concentrations, carbon character can be altered by wildfire, with implications for potential disinfection byproducts in drinking water (Wang et al., 2015; Hohner et al., 2016); therefore, carbon spectral fluorescence fingerprinting (Carpenter et al., 2022) could aid in assessing impacts of wildfire on drinking water supplies. Studies of fish populations, macroinvertebrate populations, stream metabolism rates, and harmful algal blooms (HABs), particularly regarding physiological tools and biomarkers, would assist in modeling wildfire effects on stream ecosystems (Gomez Isaza et al., 2022).
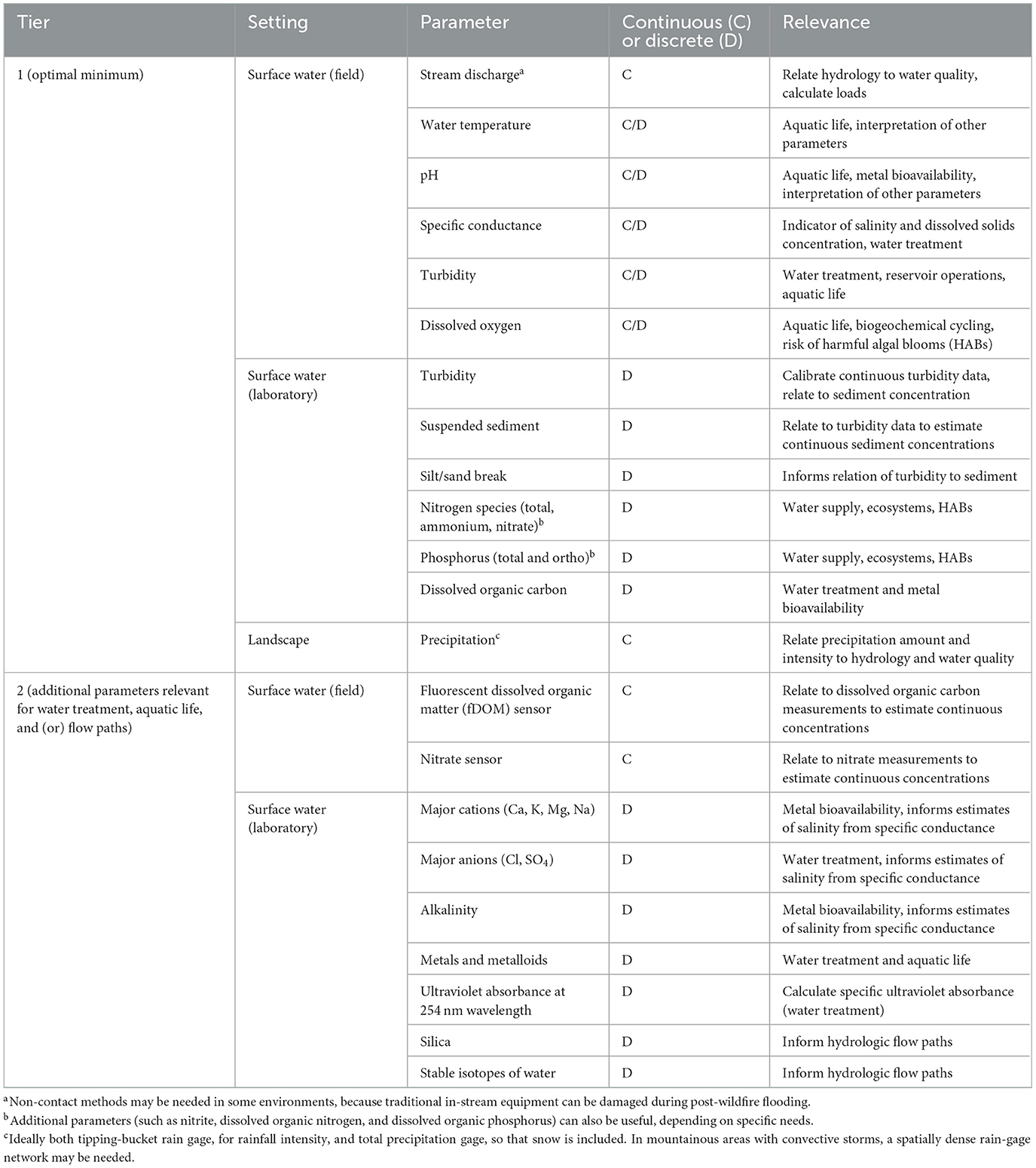
Table 1. Critical parameters to measure after wildfire to improve assessment and prediction of water quality.
Post-wildfire assessment and modeling efforts would also be improved by collecting more accurate measurements of spatial precipitation patterns [by deploying precipitation gages or weather radar (NOAA-USGS Debris Flow Task Force, 2005)] and runoff events [measurement of both stage and velocity by noncontact radar provides useable hydraulic information when channel morphology changes rapidly (Fulton et al., 2020)], along with measurements that inform infiltration and flow paths [e.g., air temperature, soil-hydraulic properties controlling infiltration (Ebel, 2013, 2020), soil moisture sensors, and cameras aimed at burned hillslopes]. Detailed analysis of local geology and soils, vegetation types, and burn severity (Cerrato et al., 2016; Rahman et al., 2018) facilitates identification of critical drivers in different ecosystems. Finally, high-resolution, repeat land surface measurements derived from remote sensing datasets (including imagery or light detection and ranging (LiDAR) datasets) would further improve understanding of post-wildfire runoff and land surface change (East et al., 2021; Morell et al., 2021; Rengers et al., 2021), and impacts on post-wildfire water quality.
4. Opportunities
While the post-wildfire water-quality datasets described here would serve as the keystone for assessment and prediction of wildfire impacts on water supplies, there are many additional opportunities for improving our understanding of the nexus of wildfire, water, and society (Martin, 2016). Water-quality monitoring in watersheds that are vulnerable to wildfire, including parameters listed in (Table 1), would provide critical baseline data for assessing how wildfires impact watersheds. Improved post-wildfire water-modeling capacity, driven by data integration that reduces prediction uncertainty, would further advance the ability of the wildfire-science community to provide actionable intelligence for water supply planning and protection (e.g., Neris et al., 2021; Nyman et al., 2021; Steblein et al., 2021; Robinne et al., 2022). Remotely sensed characterization of post-wildfire water quality could provide a globally available sentinel of water-supply hazards. Recent work has shown that satellite remote sensing can provide spatial and temporal estimates of post-wildfire turbidity (Cira et al., 2022) and phytoplankton blooms (Tang et al., 2021). Post-wildfire changes in nutrient loads may alter chlorophyll-a, colored dissolved organic matter, and HABs in receiving waters that could be detected by satellite remote sensing (Cira et al., 2022).
Key near-term opportunities from strategic post-wildfire water-quality monitoring include:
• Identifying regional factors that dampen or amplify the effects of wildfire on water quality to provide rapid, actionable guidance for water-supply planning and protection.
• Integrating water-quality datasets into model development and evaluation.
• Linking in-situ water-quality measurements with remotely sensed water-quality indicators.
• Advances in in-stream sensor technology during high-turbidity events.
The next generation of strategic post-wildfire water-quality monitoring can extend beyond the priorities outlined here. We focus on in-stream processes, but understanding wildfire effects on reservoirs is an important next step in post-wildfire monitoring (Nunes et al., 2018; Basso et al., 2021; Paul et al., 2022). Groundwater, which supplies water to over 2 billion people and accounts for up to a third of global water withdrawals (Famiglietti, 2014), can be detrimentally affected by wildfire (Mansilha et al., 2020), yet there is almost no data or guidance on potential wildfire impacts on public or private supply wells. The present state of knowledge cannot provide reliable estimates of the downstream water-quality extent beyond the burned area (Martin, 2016), yet this guidance is clearly needed. Finally, we have focused on perennial streams due to their greater importance to water supplies, but intermittent and ephemeral streams can also convey sediment and other water-quality constituents to streams following wildfire (MacNeille et al., 2020) and thus can be important to study in some basins.
Wildfire is often not the sole terrestrial disturbance affecting water-supply watersheds; pre- and post-wildfire logging, reforestation, mining, and other activities can confound interpretations of wildfire impacts (e.g., Silins et al., 2009; Harrison et al., 2014; Rhoades et al., 2018; Stevens-Rumann et al., 2018; Murphy et al., 2020; Burke et al., 2021), especially when such disturbances overlap in space and time. Compound stressors can extend to atmospheric processes such as elevated wet and dry deposition of constituents before wildfire (Riggan et al., 1994; Burke et al., 2010; Heindel et al., 2022) and smoke effects (Williamson et al., 2016; Evans et al., 2021; Boyer et al., 2022). Climate variability and change also confound detecting wildfire effects, and future shifts in precipitation regimes (Touma et al., 2022) may affect constituent mobilization. The need for tracking the source and transformation of constituents from hillslopes to channels to lentic water bodies has been identified as a critical gap (e.g., Nunes et al., 2018).
Priority areas for longer-term post-wildfire water-quality monitoring and assessment include:
• Tracking constituent sources from hillslopes and into channels, including in-channel sources such as stored sediment or hyporheic exchange processes, and their transformation during transport.
• Connecting post-wildfire stream water quality to processes and conditions in downstream receiving waters such as reservoirs and estuaries, thus linking hillslopes, lotic, and lentic water bodies for different constituents and river corridor structures.
• Measuring wildfire effects on groundwater quantity and quality.
• Separating out the confounding influence of multiple, overlapping disturbances and land-use legacies.
• Establishing mechanisms for adequately funding post-wildfire monitoring campaigns.
Data availability statement
The original contributions presented in the study are included in the article/supplementary material, further inquiries can be directed to the corresponding author.
Ethics statement
Written informed consent was obtained from the individual(s) for the publication of any potentially identifiable images or data included in this article.
Author contributions
SM and BE conceptualized the paper. SM led the design and drafting of the manuscript with BE and DM. All authors contributed to the article and approved the submitted version.
Funding
This work was supported by the U.S. Geological Survey National Water Quality Program.
Acknowledgments
Feedback from Michelle Hornberger, R. Blaine McCleskey, and Amanda Hohner helped to significantly improve this manuscript. Any use of trade, firm, or product names is for descriptive purposes only and does not imply endorsement by the U. S. Government.
Conflict of interest
The authors declare that the research was conducted in the absence of any commercial or financial relationships that could be construed as a potential conflict of interest.
Publisher's note
All claims expressed in this article are solely those of the authors and do not necessarily represent those of their affiliated organizations, or those of the publisher, the editors and the reviewers. Any product that may be evaluated in this article, or claim that may be made by its manufacturer, is not guaranteed or endorsed by the publisher.
References
Basso, M., Mateus, M., Ramos, T. B., and Vieira, D. C. S. (2021). Potential post-fire impacts on a water supply reservoir: an integrated watershed-reservoir approach. Front. Environ. Sci. 9, 684703. doi: 10.3389/fenvs.2021.684703
Basso, M., Serpa, D., Mateus, M., Keizer, J. J., and Vieira, D. C. S. (2022). Advances on water quality modeling in burned areas: a review. PLOS Water. 1, e0000025. doi: 10.1371/journal.pwat.0000025
Bernard, M., and Gregoretti, C. (2021). The use of rain gauge measurements and radar data for the model-based prediction of runoff-generated debris-flow occurrence in early warning systems. Water Res. Res. 57, e2020. doi: 10.1029/2020WR027893
Beyene, M. T., Leibowitz, S. G., and Pennino, M. J. (2021). Parsing weather variability and wildfire effects on the post-fire changes in daily stream flows: a quantile-based statistical approach and its application. Water Res. Res. 57, e2020. doi: 10.1029/2020WR028029
Bishop, K., Buffam, I., Erlandsson, M., Fölster, J., Laudon, H., Seibert, J., et al. (2008). Aqua Incognita: the unknown headwaters. Hydrol. Process. 22, 1239–1242. doi: 10.1002/hyp.7049
Bladon, K. D., Emelko, M. B., Silins, U., and Stone, M. (2014). Wildfire and the future of water supply. Environ. Sci. Tech. 48, 8936–8943. doi: 10.1021/es500130g
Bosch, J. M., and Hewlett, J. D. (1982). A review of catchment experiments to determine the effect of vegetation changes on water yield and evapotranspiration. J. Hydrol. 55, 3–23. doi: 10.1016/0022-1694(82)90117-2
Boyer, E. W., Moritz, M. A., and Brown, M. G. (2022). Smoke deposition to water surfaces drives hydrochemical changes. Hydrol. Process. 36, e14626. doi: 10.1002/hyp.14626
Bryson, D. (2021). U.S. West Faces Little-Known Effect of Raging Wildfires: Contaminated Water. London: Reuters.
Buma, B., and Livneh, B. (2017). Key landscape and biotic indicators of watersheds sensitivity to forest disturbance identified using remote sensing and historical hydrography data. Environ. Res. Lett. 12, 074028. doi: 10.1088/1748-9326/aa7091
Burke, M. P., Hogue, T. S., Ferreira, M., Mendez, C. B., Navarro, B., Lopez, S., et al. (2010). The effect of wildfire on soil mercury concentrations in Southern California watersheds. Water, Air. Soil Pollut. 212, 369–385. doi: 10.1007/s11270-010-0351-y
Burke, W. D., Tague, C., Kennedy, M. C., and Moritz, M. A. (2021). Understanding how fuel treatments interact with climate and biophysical setting to affect fire, water, and forest health: a process-based modeling approach. ront. For. Glob. Change 3, 591162. doi: 10.3389/ffgc.2020.591162
Carpenter, K. D., Kraus, T. E. C., Hansen, A. M., Downing, B. D., Goldman, J. H., Haynes, J., et al. (2022). Sources and Characteristics of Dissolved Organic Carbon in the McKenzie River, Oregon, Related to the Formation of Disinfection By-Products in Treated Drinking Water, Scientific Investigations Report 2022-5010. U.S. Geological Survey, Reston, VA, United States. p. 50. doi: 10.3133/sir20225010
Cerrato, J. M., Blake, J. M., Hirani, C., Clark, A. L., Ali, A.-M. S., Artyushkova, K., et al. (2016). Wildfires and water chemistry: effect of metals associated with wood ash. Environm. Sci. 18, 1078–1089. doi: 10.1039/C6EM00123H
Cira, M., Bafna, A., Lee, C. M., Kong, Y., Holt, B., Ginger, L., et al. (2022). Turbidity and fecal indicator bacteria in recreational marine waters increase following the 2018 Woolsey fire. Sci. Rep. 12, 2428. doi: 10.1038/s41598-022-05945-x
Daly, C., Neilson, R. P., and Phillips, D. L. (1994). A statistical-topographic model for mapping climatological precipitation over mountainous terrain. J. Appl. Meteorol. 33, 140–158.
East, A. E., Logan, J. B., Dartnell, P., Lieber-Kotz, O., Cavagnaro, D. B., McCoy, S. W., et al. (2021). Watershed sediment yield following the 2018 carr fire, Whiskeytown National recreation area, Northern California. Earth Space Sci. 8, e2021. doi: 10.1029/2021EA001828
Ebel, B. A. (2013). Wildfire and aspect effects on hydrologic states after the 2010 Fourmile Canyon Fire. Vadose Zone J. 12, 1. doi: 10.2136/vzj2012.0089
Ebel, B. A. (2020). Temporal evolution of measured and simulated infiltration following wildfire in the Colorado Front Range, USA: shifting thresholds of runoff generation and hydrologic hazards. J. Hydrol. 585, 124765. doi: 10.1016/j.jhydrol.2020.124765
Emelko, M. B., Silins, U., Bladon, K. D., and Stone, M. (2011). Implications of land disturbance on drinking water treatability in a changing climate: demonstrating the need for “source water supply and protection” strategies. Water Res. 45, 461–472. doi: 10.1016/j.watres.2010.08.051
Emelko, M. B., Stone, M., Silins, U., Allin, D., Collins, A. L., Williams, C. H. S., et al. (2016). Sediment-phosphorus dynamics can shift aquatic ecology and cause downstream legacy effects after wildfire in large river systems. Glob. Chang. Biol. 22, 1168–1184. doi: 10.1111/gcb.13073
Evans, J. S., Norman, A.-L., and Reid, M. L. (2021). Evidence of smoke from wildland fire in surface water of an unburned watershed. Water Res. Res. 57, e2021. doi: 10.1029/2021WR030069
Famiglietti, J. S. (2014). The global groundwater crisis. Nat. Clim. Chang. 4, 945–948. doi: 10.1038/nclimate2425
Fountain, H. (2021). Wildfires Threaten Urban Water Supplies, Long After the Flames are Out. Manhattan, New York City: The New York Times.
Fulton, J. W., Mason, C. A., Eggleston, J. R., Nicotra, M. J., Chiu, C.-L., Henneberg, M. F., et al. (2020). Near-field remote sensing of surface velocity and river discharge using radars and the probability concept at 10 U.S. Geological Survey streamgages. Remote Sensing. 12, 1296. doi: 10.3390/rs12081296
Gomez Isaza, D. F., Cramp, R. L., and Franklin, C. E. (2022). Fire and rain: a systematic review of the impacts of wildfire and associated runoff on aquatic fauna. Glob. Chang. Biol. 28, 2578–2595. doi: 10.1111/gcb.16088
Hallema, D. W., Sun, G., Caldwell, P. V., Norman, S. P., Cohen, E. C., Liu, Y., et al. (2018). Burned forests impact water supplies. Nat. Commun. 9, 1307. doi: 10.1038/s41467-018-03735-6
Hampton, T. B., Lin, S., and Basu, N. B. (2022). Forest fire effects on stream water quality at continental scales: a meta-analysis. Environ. Res. Lett. 17, 064003. doi: 10.1088/1748-9326/ac6a6c
Harrison, E. T., Dyer, F., Wright, D. W., and Levings, C. (2014). Historical land-use influences the long-term stream turbidity response to a wildfire. Environ. Manage. 53, 393–400. doi: 10.1007/s00267-013-0193-7
Heindel, R. C., Murphy, S. F., Repert, D. A., Wetherbee, G. A., Liethen, A. E., Clow, D. W., et al. (2022). Elevated nitrogen deposition to fire-prone forests adjacent to urban and agricultural areas, Colorado Front Range, USA. Earth's Fut. 10, e2021. doi: 10.1029/2021EF002373
Hewlett, J. D. (1971). Comments on the catchment experiment to determine vegetal effects on water yield. JAWRA. 7, 376–381. doi: 10.1111/j.1752-1688.1971.tb05920.x
Hohner, A. K., Cawley, K., Oropeza, J., Summers, R. S., and Rosario-Ortiz, F. L. (2016). Drinking water treatment response following a Colorado wildfire. Water Res. 105, 187–198. doi: 10.1016/j.watres.2016.08.034
Juang, C. S., Williams, A. P., Abatzoglou, J. T., Balch, J. K., Hurteau, M. D., and Moritz, M. A. (2022). Rapid growth of large forest fires drives the exponential response of annual forest-fire area to aridity in the Western United States. Geophys. Res. Lett. 49, e2021. doi: 10.1029/2021GL097131
Key, C. H., and Benson, N. C. (2006). “Landscape assessment,” in Firemon: Fire Effects Monitoring and Inventory System, Lutes, D. C., Keane, R.E., Caratti, J.F., Key, C.H., Benson, N.C., Sutherland, S., and Gangi, L. (Fort Collins, CO: US Department of Agriculture, Forest Service, Rocky Mountain Research Station) p. 1–55.
Krabbenhoft, C. A., Allen, G. H., Lin, P., Godsey, S. E., Allen, D. C., Burrows, R. M., et al. (2022). Assessing placement bias of the global river gauge network. Nat. Sust. 5, 586–592. doi: 10.1038/s41893-022-00873-0
MacNeille, R. B., Lohse, K. A., Godsey, S. E., Perdrial, J. N., and Baxter, C. V. (2020). Influence of drying and wildfire on longitudinal chemistry patterns and processes of intermittent streams. Front. Water 2, 563841. doi: 10.3389/frwa.2020.563841
Mansilha, C., Melo, A., Martins, Z. E., Ferreira, I. M. P. L. V. O., Pereira, A. M., and Espinha Marques, J. (2020). Wildfire effects on groundwater quality from springs connected to small public supply systems in a peri-urban forest area (Braga Region, NW Portugal). Water. 12, 1146. doi: 10.3390/w12041146
Martin, D. A. (2016). At the nexus of fire, water and society. Philos. Trans. R. Soc. 371, 20150172. doi: 10.1098/rstb.2015.0172
Minshall, G. W., Brock, J. T., and Varley, J. D. (1989). Wildfires and Yellowstone's stream ecosystems. Bioscience. 39, 707–715. doi: 10.2307/1311002
Morell, K. D., Alessio, P., Dunne, T., and Keller, E. (2021). Sediment recruitment and redistribution in mountain channel networks by post-wildfire debris flows. Geophys. Res. Lett. 48, e2021. doi: 10.1029/2021GL095549
Murphy, S. F., McCleskey, R. B., Martin, D. A., Holloway, J. M., and Writer, J. H. (2020). Wildfire-driven changes in hydrology mobilize arsenic and metals from legacy mine waste. Sci. Total Environ. 743, 140635. doi: 10.1016/j.scitotenv.2020.140635
Murphy, S. F., Stallard, R. F., Scholl, M. A., González, G., and Torres-Sánchez, A. J. (2017). Reassessing rainfall in the Luquillo Mountains, Puerto Rico: local and global ecohydrological implications. PLoS ONE. 12, e0180987. doi: 10.1371/journal.pone.0180987
Murphy, S. F., Writer, J. H., McCleskey, R. B., and Martin, D. A. (2015). The role of precipitation type, intensity, and spatial distribution in source water quality after wildfire. Environ. Res. Lett. 10, 084007. doi: 10.1088/1748-9326/10/8/084007
Neris, J., Santin, C., Lew, R., Robichaud, P. R., Elliot, W. J., Lewis, S. A., et al. (2021). Designing tools to predict and mitigate impacts on water quality following the Australian 2019/2020 wildfires: Insights from Sydney's largest water supply catchment. Integr. Environ. Assess. Manag. 17, 1151–1161. doi: 10.1002/ieam.4406
Nikolopoulos, E. I., Borga, M., Creutin, J. D., and Marra, F. (2015). Estimation of debris flow triggering rainfall: influence of rain gauge density and interpolation methods. Geomorphology. 243, 40–50. doi: 10.1016/j.geomorph.2015.04.028
Nilsen, E. (2022). Disaster Upon Disaster: Wildfires are Contaminating the West's Depleting Water With Ashy Sludge. Marietta: CNN.
NOAA-USGS debris-Flow task Force. (2005). NOAA-USGS debris-Flow Warning System- Final Report, Circular 1283. U.S. Geological Survey, Reston, VA, United States. p. 47. doi: 10.3133/cir1283
Nunes, J. P., Doerr, S. H., Sheridan, G., Neris, J., Santín, C., Emelko, M. B., et al. (2018). Assessing water contamination risk from vegetation fires: Challenges, opportunities and a framework for progress. Hydrol. Process. 32, 687–694. doi: 10.1002/hyp.11434
Nyman, P., Yeates, P., Langhans, C., Noske, P. J., Peleg, N., Schärer, C., et al. (2021). Probability and consequence of postfire erosion for treatability of water in an unfiltered supply system. Water Res. Res. 57, 2019WR026185. doi: 10.1029/2019WR026185
Patrick, C. J., Hensel, E., Kominoski, J. S., Stauffer, B. A., and McDowell, W. H. (2022). Extreme event ecology needs proactive funding. Front. Ecol. Environ. 20, 496–497. doi: 10.1002/fee.2569
Paul, M. J., LeDuc, S. D., Lassiter, M. G., Moorhead, L. C., Noyes, P. D., and Leibowitz, S. G. (2022). Wildfire induces changes in receiving waters: a review with considerations for water quality management. Water Res. Res. 58, e2021. doi: 10.1029/2021WR030699
Perica, S., Martin, D., Pavlovic, S., Roy, I., St. Laurent, M., Trypaluk, C., et al. (2013). NOAA Atlas 14, Precipitation-Frequency Atlas of the United States, Volume 8 Version 2.0: Midwestern States (Colorado, Iowa, Kansas, Michigan, Minnesota, Missouri, Nebraska, North Dakota, Oklahoma, South Dakota, Wisconsin). Silver Spring, Maryland: National Oceanic and Atmospheric Administration.
Rahman, A., El Hayek, E., Blake, J. M., Bixby, R. J., Ali, A.-M., Spilde, M., et al. (2018). Metal reactivity in laboratory burned wood from a watershed affected by wildfires. Environ. Sci. Technol. 52, 8115–8123. doi: 10.1021/acs.est.8b00530
Raoelison, O. D., Valenca, R., Lee, A., Karim, S., Webster, J. P., Poulin, B. A., et al. (2023). Wildfire impacts on surface water quality parameters: cause of data variability and reporting needs. Environ. Pollut. 317, 120713. doi: 10.1016/j.envpol.2022.120713
Rengers, F. K., McGuire, L. A., Kean, J. W., Staley, D. M., Dobre, M., Robichaud, P. R., et al. (2021). Movement of sediment through a burned landscape: Sediment volume observations and model comparisons in the San Gabriel Mountains, California, USA. J. Geophysical Res. 126, e2020. doi: 10.1029/2020JF006053
Rhoades, C., Pelz, K., Fornwalt, P., Wolk, B., and Cheng, A. (2018). Overlapping bark beetle outbreaks, salvage logging and wildfire restructure a lodgepole pine ecosystem. Forests. 9, 101. doi: 10.3390/f9030101
Rhoades, C. C., Chow, A. T., Covino, T. P., Fegel, T. S., Pierson, D. N., and Rhea, A. E. (2019a). The legacy of a severe wildfire on stream nitrogen and carbon in headwater catchments. Ecosystems. 22, 643–657. doi: 10.1007/s10021-018-0293-6
Rhoades, C. C., Nunes, J. P., Silins, U., and Doerr, S. H. (2019b). The influence of wildfire on water quality and watershed processes: new insights and remaining challenges. Int. J. Wildland Fire. 28, 721–725. doi: 10.1071/WFv28n10_FO
Rice, R. M. (1979). A Watershed's Response to Logging and Roads: South Fork of Caspar Creek, California, 1967-1976. Berkeley, CA: U.S. Department of Agriculture, Forest Service, Pacific Southwest Forest and Range Experiment Station.
Riggan, P. J., Lockwood, R. N., Jacks, P. M., Colver, C. G., Weirich, F., DeBano, L. F., et al. (1994). Effects of fire severity on nitrate mobilization in watersheds subject to chronic atmospheric deposition. Environ. Sci. Technol. 28, 369–375. doi: 10.1021/es00052a005
Robinne, F.-N., Hallema, D. W., Bladon, K. D., Flannigan, M. D., Boisram,é, G., Bréthaut, C. M., et al. (2021). Scientists' warning on extreme wildfire risks to water supply. Hydrol. Process. 35, e14086. doi: 10.1002/hyp.14086
Robinne, F.-N., Paquette, C., Hallema, D. W., Bladon, K. D., and Parisien, M.-A. (2022). Baseline geographic information on wildfire-watershed risk in Canada: needs, gaps, and opportunities. Can. Water Resour. J. 47, 1–18. doi: 10.1080/07011784.2022.2032367
Rust, A. J., Hogue, T. S., Saxe, S., and McCray, J. (2018). Post-fire water-quality response in the western United States. Int. J. Wildland Fire 27, 203–216. doi: 10.1071/WF17115
Saraceno, J. F., Shanley, J. B., Downing, B. D., and Pellerin, B. A. (2017). Clearing the waters: Evaluating the need for site-specific field fluorescence corrections based on turbidity measurements. Limnol. Oceanography. 15, 408–416. doi: 10.1002/lom3.10175
Sham, C. H., Tuccillo, M. E., and Rooke, J. (2013). Effects of Wildfire on Drinking Water Utilities and Best Practices for Wildfire Risk Reduction and Mitigation. Denver, Colorado: Water Research Foundation.
Shuman, J. K., Balch, J. K., Barnes, R. T., Higuera, P. E., Roos, C. I., Schwilk, D. W., et al. (2022). Reimagine fire science for the anthropocene. PNAS Nexus. 1, 3. doi: 10.1093/pnasnexus/pgac115
Silins, U., Stone, M., Emelko, M. B., and Bladon, K. D. (2009). Sediment production following severe wildfire and post-fire salvage logging in the Rocky Mountain headwaters of the Oldman River Basin, Alberta. CATENA. 79, 189–197. doi: 10.1016/j.catena.2009.04.001
Smith, H. G., Sheridan, G. J., Lane, P. N., Nyman, P., and Haydon, S. (2011). Wildfire effects on water quality in forest catchments: a review with implications for water supply. J. Hydrol. 396, 170–192. doi: 10.1016/j.jhydrol.2010.10.043
Steblin, P. F., Loehman, R. A., Miller, M. P., Holomuzki, J. R., Soileau, S. C., Brooks, M. L., et al. (2021). U.S. Geological Survey Wildland Fire Science Strategic Plan, 2021-26, Circular 1471. U.S. Geological Survey, Reston, VA, United States. p. 70. doi: 10.3133/cir1471
Stevens-Rumann, C. S., Kemp, K. B., Higuera, P. E., Harvey, B. J., Rother, M. T., Donato, D. C., et al. (2018). Evidence for declining forest resilience to wildfires under climate change. Ecol. Lett. 21, 243–252. doi: 10.1111/ele.12889
Tang, W., Llort, J., Weis, J., Perron, M. M. G., Basart, S., Li, Z., et al. (2021). Widespread phytoplankton blooms triggered by 2019–2020 Australian wildfires. Nature. 597, 370–375. doi: 10.1038/s41586-021-03805-8
Thornton, P. E., Thornton, M. M., Mayer, B. W., Wilhelmi, N., Wei, Y., Devarakonda, R., et al. (2012). Daymet: Daily Surface Weather on a 1 km Grid for North America, 1980-2008. Oak Ridge, TN: Oak Ridge National Laboratory (ORNL) Distributed Active Archive Center for Biogeochemical Dynamics (DAAC).
Touma, D., Stevenson, S., Swain, D. L., Singh, D., Kalashnikov, D. A., and Huang, X. (2022). Climate change increases risk of extreme rainfall following wildfire in the western United States. Sci. Adv. 8, eabm0320. doi: 10.1126/sciadv.abm0320
U.S. Geological Survey. (2018). General Introduction for the National Field Manual for the Collection of Water-Quality Data (ver. 1.1, June 2018). U.S. Geological Survey Techniques and Methods, Reston, VA, United States. doi: 10.3133/tm9A0
Wang, J.-J., Dahlgren, R. A., Erşan, M. S., Karanfil, T., and Chow, A. T. (2015). Wildfire altering terrestrial precursors of disinfection byproducts in forest detritus. Environ. Sci. Technol. 49, 5921–5929. doi: 10.1021/es505836m
Williamson, C. E., Overholt, E. P., Brentrup, J. A., Pilla, R. M., Leach, T. H., Schladow, S. G., et al. (2016). Sentinel responses to droughts, wildfires, and floods: effects of UV radiation on lakes and their ecosystem services. Front. Ecol. Environ. 14, 102–109. doi: 10.1002/fee.1228
Wine, M. L., and Cadol, D. (2016). Hydrologic effects of large southwestern USA wildfires significantly increase regional water supply: fact or fiction? Environ. Res. Letters. 11, 085006. doi: 10.1088/1748-9326/11/8/085006
Wondzell, S. M., and King, J. G. (2003). Postfire erosional processes in the Pacific Northwest and Rocky Mountain regions. For. Ecol. Manage. 178, 75–87. doi: 10.1016/S0378-1127(03)00054-9
Keywords: wildfire, wildland fire, post-wildfire, burned area, water supplies, water availability, disturbance, water quality
Citation: Murphy SF, Alpers CN, Anderson CW, Banta JR, Blake JM, Carpenter KD, Clark GD, Clow DW, Hempel LA, Martin DA, Meador MR, Mendez GO, Mueller-Solger AB, Stewart MA, Payne SE, Peterman CL and Ebel BA (2023) A call for strategic water-quality monitoring to advance assessment and prediction of wildfire impacts on water supplies. Front. Water 5:1144225. doi: 10.3389/frwa.2023.1144225
Received: 14 January 2023; Accepted: 23 February 2023;
Published: 13 March 2023.
Edited by:
Qian Zhang, University of Maryland, United StatesReviewed by:
Amanda Hohner, Montana State University, United StatesCopyright © 2023 Murphy, Alpers, Anderson, Banta, Blake, Carpenter, Clark, Clow, Hempel, Martin, Meador, Mendez, Mueller-Solger, Stewart, Payne, Peterman and Ebel. This is an open-access article distributed under the terms of the Creative Commons Attribution License (CC BY). The use, distribution or reproduction in other forums is permitted, provided the original author(s) and the copyright owner(s) are credited and that the original publication in this journal is cited, in accordance with accepted academic practice. No use, distribution or reproduction is permitted which does not comply with these terms.
*Correspondence: Sheila F. Murphy, c2ZtdXJwaHlAdXNncy5nb3Y=