- Universidad Tecnológica de Santiago, UTESA, Santiago de los Caballeros, Dominican Republic
Introduction: The use of biosolids from wastewater treatment plants in agriculture is considered relevant for the promotion of sustainable agricultural practices, the improvement of soil fertility, and the reduction of dependence on synthetic chemical products. The Dominican Republic is today the world's largest producer of organic bananas and the main exporter of organic and fair-trade bananas to Europe. The biosolids generated in the wastewater treatment plants in the Dominican Republic currently have no use. In other countries, biosolids are used for agriculture, although biosolids have different characteristics in each place and their potential must be analyzed. In the Dominican Republic, there is no research that analyzes biosolids and their impact on agriculture. This research aims to compare the nutritional composition of bananas in plantations fertilized with different doses of biosolids and other organic fertilizers.
Methods: For this test, five blocks were prepared with organic fertilization, another with potassium sulfate, and a control block. Laboratory analysis was used to determine the characteristics of the biosolids used and also to know the nutritional composition of bananas of the Williams variety.
Results: The results, when compared, show the impact that each of these fertilizers has or can have on the production of organic bananas in the Dominican Republic.
Discussion: The use of dose Y of the biosolid, characterized by the application of 16 kilograms of biosolid per plant, is the most recommended to fertilize the banana since the collected fruits presented the most optimal nutritional values.
1 Introduction
Organic farming is a natural system that brings together agronomic, ecological, economic and social aspects. This type of farming includes the use of organic inputs for fertilizers, such as manure and plant residues; the management of insects, pests, and diseases based on plant extracts used as repellents or biological control agents; and natural or induced biological control and crop rotation for the protection of crops and a better use of the soil (Echeverry-Navarro, 2001). As an alternative to substituting chemical fertilizers applied to crops, the use of organic fertilizers has been suggested (compost and biosolids, among others), which can supply the nutrients required by plants, increase the amount of microorganisms, and improve the physical characteristics of the soil (Ramos-Agüero et al., 2016). The indiscriminate or inappropriate use of chemicals (fertilizers and pesticides, among others) has caused a progressive deterioration of agricultural soils across the world, as well as pollution of the environment. This situation generates negative environmental effects, such as soil toxicity and infertility, increased acidity, surface and groundwater contamination, and high concentrations of nitrates causing damage to plants, as well as affecting ecosystems and human health (Sánchez-Murcia, 2021).
Sludge from wastewater treatment plants consists of a residue of organic matter that remains after processing, which looks like a stable, nutrient-rich humus in aqueous suspension (Sharma and Garg, 2019). Treated sludge suitable for use in farming is known by the name of biosolids and is an excellent organic fertilizer that can be applied to orchards and fields in a liquid state (biosolid with high moisture content) or can be dehydrated, leaving the organic material in the form of a semi-solid or solid sludge paste that is easy to stack, distribute and apply to the fields (Munoz et al., 2021). Nutrients contained in biosolids increase the biomass and yield of crop plants (Ploughe et al., 2021). However, their application on agricultural land could present, if the appropriate treatment is not applied, some negative aspects, such as the presence of heavy metals (Jordán et al., 2022) and pathogenic microorganisms, which can negatively influence both the soil and the crops (Law et al., 2021). The agricultural use of biosolids is one of the most recommended options in literature for improving soils and fertilizing crops (Sharma et al., 2017). The main components of biosolids are organic matter (OM) and nutrients beneficial to all plants, especially nitrogen (N) and potassium (P) (Latare et al., 2014).
However, given the nature and physicochemical characteristics found in biosolids, they must be analyzed at a chemical level before their application to agricultural land (Sharma et al., 2017), especially to control the presence of certain heavy metals (Türkdogan et al., 2003), pathogens (Al-Gheethi et al., 2018), or other organic contaminants (Mattana et al., 2014). This is why the safety of applying treated dewatered sludge as fertilizer has been a topic of research and discussion, since potential risks have been associated with the presence of heavy metals in biosolids (Cheng et al., 2013; Okoffo et al., 2020). The accumulation of heavy metals in the soil and their possible uptake by crops can pose risks to human health (Jordán et al., 2022). There is also a need for further assessment of environmental damage and potential contamination of agroecosystems where biosolids are applied to land as fertilizer (Judy et al., 2015; Hogue, 2018). Furthermore, the presence of contaminants such as pharmaceuticals and alkylphenol ethoxylates in biosolids has been identified as a potential concern (Örmeci, 2014). Also, the fate of regulated contaminants and antibiotic-resistant bacteria in biosolids and their potential impacts on the environment and soil degradation have been studied (Cunningham et al., 2020). But in general, biosolids can provide benefits in terms of soil fertility and plant growth (Broderick and Evans, 2017; Sun et al., 2022), however it is important to consider potential risks and ensure regulations are in place of quality monitoring and adequate treatment to mitigate any adverse effects (Okoffo et al., 2020).
The banana is a widely grown fruit and consumed worldwide because of its organoleptic properties (characteristic aroma and pleasant taste) and as a result of its high content of energy, minerals and Vitamins A, C, and B6 (Khawas et al., 2014). This tropical fruit has a high nutritional value and is consumed widely in Europe (Arvanitoyannis and Mavromatis, 2009). Soil fertility is the main factor that guarantees the sustainability of banana cultivation. Junqueira-Teixeira (2005) noted that nutrient export by the banana cluster, nutrient losses by leaching and surface runoff, and the acidification of the soil profile call for the careful management of soil fertility to maintain a high yield. An important aspect in the use of biofertilizers in banana cultivation is to evaluate the response of the crop to organic fertilization. Thus, some literature indicates that there is a direct relationship between the conditions in the physiological development or growth of the plant and the yield variables of the plant in morphological terms (Martínez-Cardozo et al., 2016; Cobeña-Loor et al., 2020). Currently, agricultural research is aimed at improving crops to increase the quantity and quality of nutrients in fruits (Welch and Graham, 2004; Aluru et al., 2008; Ploughe et al., 2021), and the nutritional quality and number of fruits per bunch are important selection indices in banana and banana improvement programs (Ndukwe et al., 2012), although the nutritional composition of bananas in response to soil fertilization with biosolids has not been widely addressed in literature.
The relationship between nutrient content in the soil and the response in banana has provided doses for different levels of soil fertility in all banana-producing countries in Latin America (Arias, 1984; Hernández, 1985; López, 1994). The banana cultivation requires adequate amounts of nutrients to be applied to the soil in the form of organic and inorganic fertilizers. These nutrients promote vegetative growth and fruit production. Part of the nutrients accumulated by the plant in the different tissues during the growth and production cycle are exported at the time of harvest (Cruzate and Casas, 2009). The amount extracted depends on the banana variety, the management, the edaphoclimatic conditions (Quintero, 1995), and the yield of the plantation (Cruzate and Casas, 2009). Bananas are relatively fast-growing plants and demand high levels of nutrients available in the soil for normal development and production. According to and Lahav (1995) and Soto Ballestero (2008), the amount of nutrients provided by the soil and soil-plant recycling system is not enough to meet the demands of the crop, so the application of fertilizers in adequate amounts is required.
Thus, the main objective of this research is to carry out a comparative evaluation of the response in the nutritional composition of bananas on fertilized cropland with different doses of biosolids and with other organic fertilizers. In this way, compared to previous studies on the subject, this research on the use of biosolids as organic fertilizers in banana cultivation offers several innovative aspects. Firstly, it focuses on evaluating the response in the nutritional composition of bananas when fertilized with different doses of biosolids and other organic fertilizers. This is important because the nutritional composition of bananas in response to soil fertilization with biosolids has not been widely addressed in the literature (Castellanos-Rozo et al., 2022). Secondly, the research aims to assess the potential risks associated with the application of biosolids to agricultural land. It acknowledges that biosolids can contain heavy metals, pathogens, and other organic contaminants, which can negatively influence both the soil and the crops (Khalid et al., 2017; Jordán et al., 2022). By analyzing the chemical level of biosolids before their application, the research aims to ensure the safety of using treated dewatered sludge as fertilizer (Shanableh and Ginige, 1999). This is crucial because the accumulation of heavy metals in the soil and their uptake by crops can pose risks to human health (Jordán et al., 2022). Thirdly, the research takes into account the specific context of organic banana production in the Dominican Republic, where a high market demand for organic bananas in Europe and environmental concerns in the banana industry favor the development of sustainable production (Gebert, 2010). Approximately 95% of organic banana exports from the Dominican Republic are sent to the European Union, accounting for almost 50% of its supply (FAO, 2023). Lastly, the research contributes to the evolving biosolids industry by evaluating the potential benefits of using biosolids as a soil improver to reduce the use of chemical fertilizers, optimize costs, and increase crop yields (Castellanos-Rozo et al., 2022). The optimization of costs through the use of biosolids is very important for banana producers, since these crops are located in the most arid region of the country, generating very high costs due to drought (Ramesh and Ostad-Ali-Askari, 2023a,b). Overall, this research provides a comprehensive and innovative approach to understanding the use of biosolids as organic fertilizers in banana cultivation, considering both the potential benefits and risks associated with their application.
2. Materials and methods
2.1. Experimental area
The study was carried out at an experimental farm (19°26′41”N 70°38'09”E) located in the vicinity of the city of Santiago de los Caballeros (Dominican Republic), where five experimental blocks of 15 × 16 m each were prepared and arranged for the cultivation of bananas, and a different organic fertilizer process was applied to each of the blocks. Each block was a space of land made up of 63 banana crops, distributed in seven rows of nine banana crops. The planting distribution recommended by producers in the region was used, which consists of planting nine vines in each row, separated by 2 m. The Williams variety of banana was used. The Williams banana crop is characterized by its high productivity and resistance to common diseases in banana plantations. It requires a warm and humid climate (75–85%), with an average temperature ranging between 27 and 30°C. It adapts well to various soil types, as long as they are well-drained. Williams bananas require proper irrigation (1,000–1,500 mm of water/year) and careful management of pests and diseases to ensure optimal production. Additionally, they have a maturation time of ~9–12 months from planting to harvest (Gamez et al., 2019). The crop of this research was planted in December 2021 and its fruits were collected in September 2022.
The fertilizers used in each block were incorporated into the soil in the pre-sowing stage, in the month of November 2021. Block 2 was fertilized with 190 kilos of animal-based organic fertilizer (poultry litter); Block 4 was applied with an X-dose of biosolids, Block 5 was applied with a Y-dose of biosolids; Block 6 was applied with an X-dose of biosolids plus a crop protection compound; and Block 7 was applied with a Y-dose of biosolids plus the crop protection compound. The X-dose refers to the application of 8 kg of biosolid/plant, while, the Y-dose refers to the application of 16 kg of biosolid/plant. Therefore, for blocks 4 and 6, a total of 504 kilos of biosolids were applied to the soil, pre-sowing, and for blocks 5 and 7, 1,008 kilos of biosolids were also applied pre-sowing. The crop protection compound applied together with the biosolid in Blocks 6 and 7 consisted of a solution made with Neem fruit (25 g of milled Neem/gallon of water, mixed twice a day for 3 days) of an applied dose equivalent to 1 L of solution/hole of each plant. Block 1 (control, no fertilizer applied) and Block 3 (fertilized with 11 kilos of potassium sulfate in the growing months number 2 -February 2022-, number 5 -May 2022- and number 8 -August 2022-) did not produce bunches of bananas at the time of harvest of the other blocks mentioned, and were therefore not analyzed (Figure 1).
2.2. Soil analysis
Before sowing, a nutritional and heavy metal analysis of the soil was carried out. The soil analysis was carried out by the company A&L Greatlakes Laboratories, located in the United States. Important parameters such as pH, total nitrogen, phosphorus and potassium were evaluated. These analyses were repeated 1 week before harvest, this time by the company EQLAB Environmental Quality Laboratories, based in Puerto Rico. The soils were sampled at a depth of 0–30 cm. Three soil samples were taken from each block using an auger with an internal diameter of 4.0 cm. Subsequently, they were immediately mixed and sealed in marked plastic bags. Before analyzing the parameters, the soil samples were air-dried. Regarding the soil pH, a pH electrode was used following a soil to water ratio of 1:2.5. Total nitrogen was measured by the Kjeldahl method. Available phosphorus was extracted using 0.5 M NaHCO3, and available potassium was extracted using 1.0 M NH4OA (Bastida et al., 2008). Table 1 shows a summary of the parameters analyzed, indicating their procedure or method. Heavy metal analyzes were performed prior to planting by A&L Greatlakes Laboratories. The following heavy metals were present in the soil: sulfur, zinc, manganese, iron, copper, and boron. These were determined by the diethylene triamine pentaacetic acid extraction-inductively couple plasma method. The analyzes were made for three samples: a sample in zone 1 (experimental area of blocks 1, 2, and 3), a sample for zone 2 (experimental area of blocks 4 and 5) and a sample for zone 3 (area experimental block 6 and 7).
2.3. Biosolids analysis
The treated dehydrated sludge (biosolid) was extracted from the main Wastewater Treatment Plant (WWTP) of the city of Santiago de los Caballeros and one of the most important in the country (WWTP Rafey), and was characterized by the Puerto Rican laboratory EQLAB (Environmental Quality Laboratories, SRL), using EPA Methods. EPA Methods refer to analytical procedures developed and published by the United States Environmental Protection Agency (EPA). These methods provide standardized guidelines for the analysis of various environmental samples, including water, soil, air, and biological materials. EPA's methods ensure consistency and reliability in environmental monitoring and regulatory compliance by specifying the appropriate techniques, instrumentation, reagents, and quality control measures to be used during the testing process. A simple random sampling method was used to select the biosolids samples for analysis. Below are the chromatographic analyses:
• Herbicidal Compounds Analysis: Herbicidal compounds present in the biosolid were analyzed using gas chromatography. Gas chromatography is a technique used to separate and analyze volatile compounds. The specific method used for this analysis is EPA Method 8151A.
• Volatile and Semi-Volatile Organic Compounds Analysis: The determination of volatile and semi-volatile organic compounds in the biosolid was carried out using gas chromatography coupled with mass spectrophotometry. This technique allows for the identification and quantification of a wide range of organic compounds. The specific methods used for this analysis are EPA Method 8260B and EPA Method 8270C. The biosolid sample would be prepared and subjected to gas chromatographic separation followed by mass spectrometric detection. The instrument parameters, calibration standards, and sample preparation steps would follow the guidelines outlined in EPA Methods.
• Pesticide Analysis: The analysis of pesticides present in the biosolid was conducted using gas chromatography. Gas chromatography is used to separate and identify different pesticide compounds. The specific method used for this analysis is EPA Method 8081A.
• Heavy Metals Analysis: The determination of heavy metals in the biosolid was performed using inductively coupled plasma atomic emission spectroscopy. ICP-AES is a technique that allows for the identification and quantification of various elements, including heavy metals. The specific method used for this analysis is EPA Method 6010D.
In addition, several other chemicals analyze were conducted:
• Total Organic Carbon (TOC): The determination of total organic carbon in the biosolid was carried out using either catalytic combustion or chemical oxidation methods. Total organic carbon analysis provides information about the carbon content in the sample. The specific method used for this analysis is EPA Method 415.1.
• Total Nitrates and Nitrites Analysis: The analysis of total nitrates and nitrites (NO2 + NO3) in the biosolid was performed using an automated colorimetric method with a reduction column. This method allows for the quantification of nitrates and nitrites present in the sample. The specific method used for this analysis is EPA Method 353.2.
• Total Kjeldahl Nitrogen Analysis: The determination of total Kjeldahl nitrogen in the biosolid was conducted using semi-automated colorimetry. Total Kjeldahl nitrogen analysis provides information about the nitrogen content in the sample. The specific method used for this analysis is EPA Method 351.2.
• Total Phosphorus Analysis: The analysis of total phosphorus in the biosolid was performed using colorimetric methods after sample digestion. Total phosphorus analysis provides information about the phosphorus content in the sample. The specific methods used for this analysis are SM Methods 4500P-A, B, and E.
In addition to these chemical analyses, temperature was determined using a mercury thermometer (EPA 170.1), and pH was measured using the electrometric method with a platinum electrode (SM 4500 HB).
Microbiological analyzes performed on the biosolid included the following:
• Salmonella Analysis: The presence and quantification of the Salmonella genus were determined using the membrane filter technique followed by incubation in a selective culture medium (SM 9260D). SM is a Standard Methods for the Examination of Water and Wastewater, that provides standardized methods for the analysis of water and wastewater samples. The biosolid sample was filtered through a membrane filter, and the filter was placed on the selective culture medium. Incubation allowed for the growth of Salmonella colonies, which were then counted and reported as Most Probable Number (MPN) per 4 g of sample. MPN is a statistical estimation of the number of microorganisms present in a sample.
• Molds and Yeast Analysis: Molds and yeasts were determined using the plate counting technique. The biosolid sample was plated onto agar media suitable for the growth of molds and yeasts. After incubation, visible colonies were counted and expressed as Colony-Forming Units (CFU) per gram of sample. CFU is a measurement of viable microorganism cells capable of forming colonies under specific conditions.
• Fecal Coliform Analysis: Fecal coliforms were analyzed using the multiple tube fermentation technique (SM 9221E). Multiple fermentation tubes containing appropriate growth media were inoculated with the biosolid sample. Incubation allowed for the growth of fecal coliforms, and the tubes showing positive gas production were counted. The result was expressed as MPN per gram of sample.
• Viable Helminth Ova Analysis: The number of viable helminth ova was determined using the helminth ova concentration technique (EPA 625/R-92). The biosolid sample was centrifuged with buffered water and a surfactant. After decanting the supernatant, the resulting pellet was examined for the presence of viable helminth ova. The result was expressed as Viable Helminth Ova (VHO) per 4 g of sample. VHO is a test for the presence of viable parasitic worm eggs.
The results of the characterization of the biosolid used in this study show that it is a safe organic fertilizer for application to the soils of banana plantations in the Dominican Republic. According to the results of microbiological analyses, the biosolid can be classified in class A of the standard (NOM-004-SEMARNAT-2002, 2002). This is because the values of fecal coliforms, Salmonella and the number of viable helminth ova were below the limits established by the official Mexican standard (NOM-004-SEMARNAT-2002, 2002) and by the USEPA (1993). Table 2 shows a summary of the parameters analyzed in the biosolids, with their procedure or method.
2.4. Banana nutritional analysis
The banana samples were obtained from a simple random sampling of bunches in each of the blocks. The harvested samples were collected on the same day, in a single sampling. In each of the five blocks, one bunch was picked and separated, from which about 300 g of fruit chosen from several single bananas (sub-samples) in the harvested bunch were extracted for analysis and removed from each block. Samples of the fruit were sent for analysis to an accredited laboratory in Madrid, Spain (AGQ-Lab). The following nutritional parameters of the bananas were determined: total sugars, ash, dietary fiber, total fats, carbohydrates, moisture, proteins, energy value, calcium, magnesium, and potassium. Below are the details of the analysis:
• Total Sugar Analysis: Total sugars were analyzed by volumetric analysis (AQ-510). The banana samples were subjected to a volumetric analysis technique to determine the total sugar content.
• Ash Analysis: Ash content was determined using a gravimetric method (PE-355). The banana samples were incinerated to remove organic matter, and the remaining ash was weighed to determine the ash content.
• Moisture Analysis: Moisture content was determined by gravimetric methods (AQ-529). The banana samples were dried and weighed before and after the drying process to determine the moisture content.
• Dietary Fiber Analysis: Dietary fiber was determined using enzymatic-gravimetric methods (PE-279). The banana samples were subjected to enzymatic treatment to remove non-dietary fiber components. The remaining dietary fiber was then measured gravimetrically.
• Total Fat Analysis: Total fats were analyzed using nuclear magnetic resonance (NMR) (PE-2117). The banana samples were subjected to NMR analysis, which allows for the quantification of the total fat content.
• Protein Analysis: Proteins were determined using the Kjeldahl method (PE-987). The banana samples were digested using the Kjeldahl method, which involves acid digestion and subsequent determination of nitrogen content to calculate protein content.
• Carbohydrates and Energy Value Analysis: Carbohydrates and energy value were derived by calculations (AQ-504). The carbohydrate and energy values were calculated based on the composition and content of other macronutrients.
• Calcium, Magnesium, and Potassium Analysis: Calcium, magnesium, and potassium parameters were analyzed by flame atomic absorption spectrophotometry (AQ-967). The banana samples were subjected to flame atomic absorption spectrophotometry, which allows for the quantification of these mineral elements.
The mentioned methods, such as AQ-510, PE-355, AQ-529, PE-279, etc., refer to specific analytical protocols or procedures used by the laboratory to determine the respective nutritional parameters. Table 3 shows a summary of the parameters analyzed in the banana, with their procedure or method.
2.5 Statistic analysis
The data analysis was performed by directly comparing the values of the nutritional parameters of the fruit harvested in the different blocks. In addition, a cluster analysis was performed to find similarities and/or differences between the nutritional composition of the banana in the different blocks tested. Cluster analysis is a multivariate statistical method that aims to search for similar clusters of individuals or sites that are clustered as homogeneously as possible based on the variables observed (Pérez-López, 2004). In the case of this research, the idea is to cluster the different experimental blocks according to the nutritional composition that the bananas presented, after the application of the organic fertilizers, including the treatments with application of biosolids. The blocks classified within the same cluster will be very similar to each other and different from the other clusters formed. For the clustering, we used the Morisita-Horn Similarity Index, which takes into account both the similarity in nutritional composition and the similarity in abundances. Values can be taken from 0 to 1, where 0 indicates no similarity and 1 total similarity. This index provides satisfactory results among quantitative similarity indices. The cluster analysis was performed using the Past 4.04 statistical program. Also, an ANOVA (Analysis of Variance) statistical analysis was performed to assess the significance of the differences between the different organic fertilizer processes applied to the banana growing blocks. The ANOVA test will determine if there are significant variations in the nutritional parameters (total sugars, ashes, dietary fiber, total fat, carbohydrates, moisture, protein, energy value, calcium, magnesium, and potassium) between the different blocks. The significance test used will be based on the F-test, which compares the variability between groups with the variability within groups. In addition, a normal distribution test, such as the Shapiro–Wilk test, will be performed to test the assumption of normality of the data before performing the ANOVA analysis. This test will examine whether the data follows a normal distribution, which is a required assumption for the validity of the ANOVA results. By carrying out the ANOVA test and normal distribution, the study aims to determine if the organic fertilizer processes significantly influence the nutritional composition of bananas and if the data meet the necessary assumptions for the analysis. This analysis was performed with the statistical program SPSS v.24.
3 Results
3.1 Characterization of soil
Nutrients and heavy metals in the soil were analyzed twice: the last week of November 2021 and the first week of September 2022. Table 4 shows the parameters analyzed in each of the analyses. The total nitrogen concentration ranged between 3.7 and 7.1 g/kg−1 in the pre-sowing period, and between 3.8 and 9.6 g/kg−1 in the analysis prior to harvesting. In the case of phosphorus, it ranged between 1.7 and 2.4 g/kg−1 in pre-sowing, and between 3.7 and 4.1 g/kg−1 in the analysis prior to harvesting. In the case of potassium, it ranged between 4.1 and 5.8 g/kg−1 in pre-sowing, and between 5.1 and 5.9 g/kg−1 in the analysis prior to harvesting. Table 5 shows the results of the heavy metals obtained in the sampling prior to planting. The results show that the highest concentration of heavy metals have been manganese and sulfur.
3.2 Characterization of the biosolid
The results of the characterization of the biosolid used in the fertilization of the experimental culture are shown in Tables 6–9. The pH value is 5.60 and the moisture value is 59%. Table 6 shows the values of concentrations of herbicides, volatile organic compounds, and pesticides were below the limit of detection of the method used (ND) and in no case exceeded the limits established in the standards of the United States environmental protection agency (USEPA, 1993).
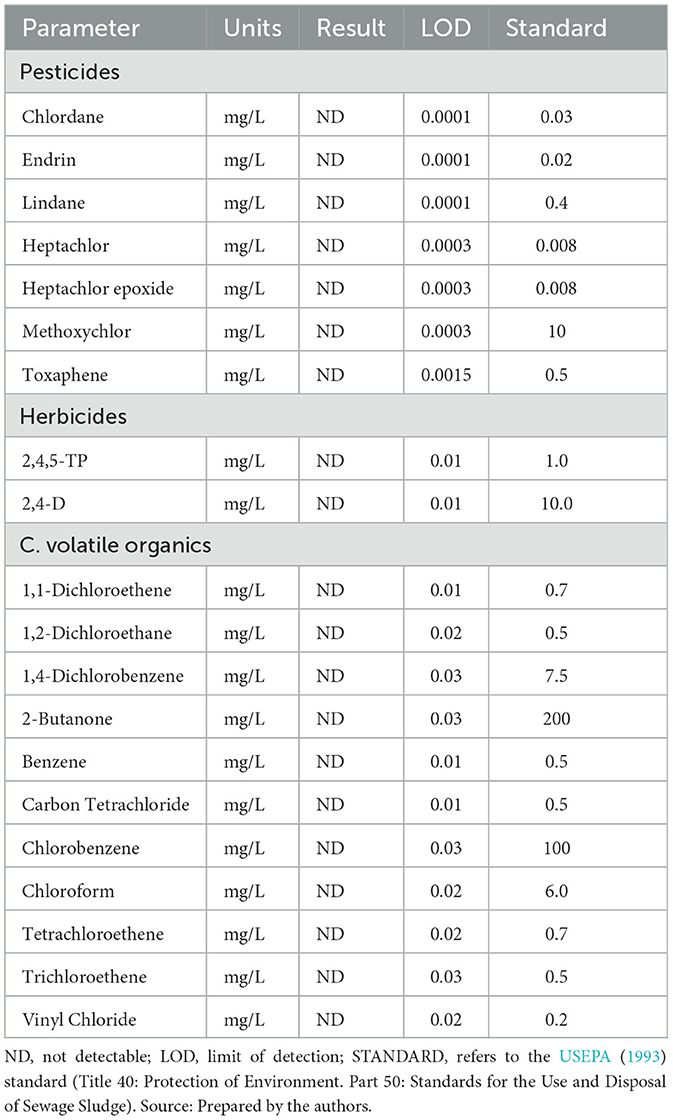
Table 6. Results of the values of pesticide, herbicide, and volatile organic compounds in the characterization of the biosolid applied in the experimental blocks in the study.
The results of the metal elements are shown in Table 7. Zinc, copper, nickel, and lead had the highest values; while arsenic, chromium, cadmium, and mercury showed lower values. The values of these parameters do not exceed the limit values required by USEPA (1993) and the Mexican standard for biosolids (NOM-004-SEMARNAT-2002, 2002) placed on soils for agricultural purposes. The values indicate a concentration sequence of Zn > Cu > Ni > Pb > As > Cr > Cd > Hg. This indicates that the biosolid does not pose a risk in terms of heavy metal contamination. Likewise, the concentrations of pesticides, herbicides and volatile and non-volatile organic compounds were below the limits of detection of the methods used and below what is established in the standards.
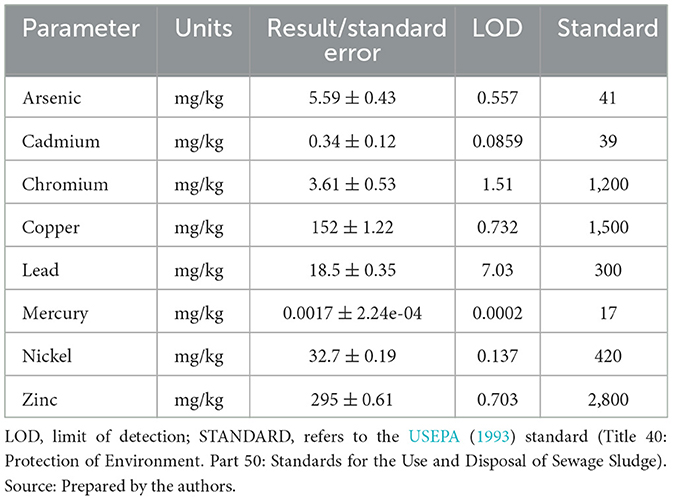
Table 7. Values of the heavy metals analyzed during the characterization of the biosolid applied in the experimental blocks during the study.
The values of the nutrients analyzed in the biosolid (total phosphorus, total nitrogen, potassium, calcium, magnesium, sodium, and iron) are shown in Table 8. The content of macro- and micronutrients in the biosolid follows the sequence: Fe > N > Ca > P > K > Mg > COT > Zn > Cu. This indicates that the biosolid is rich in essential nutrients such as nitrogen, phosphorus, potassium, calcium, and iron, which are beneficial for plant growth and development.
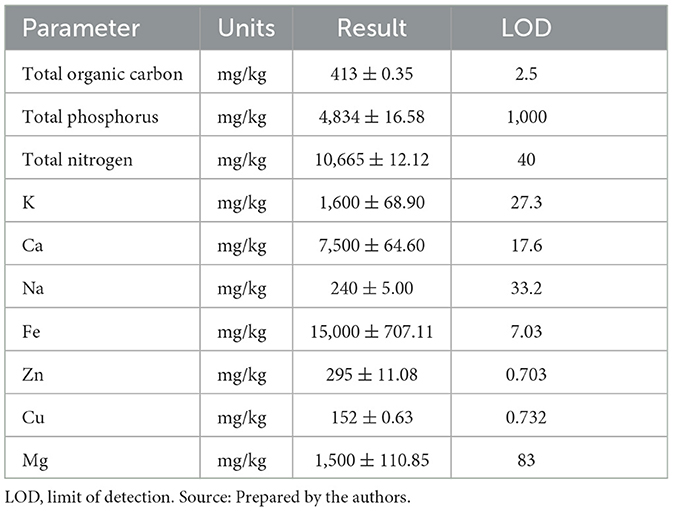
Table 8. Composition of macronutrients in the biosolid applied to the experimental blocks during the study.
The analyses of the pathogenic microorganisms are shown in Table 9. The results for salmonella were below the limit of detection of the method (<0.72 MPN/4 g) and below the values indicated in the standards (NORM-004-SERMANAT-2002). Similarly, the fecal coliforms were found to have values below the limit of detection of the method (<1.8 MPN/g) and the number of viable helminth ova (VHO) was below the limit of detection (<0.2 VHO/4 g). These results demonstrate that the biosolid is safe in terms of pathogenic microorganisms and does not pose a risk to human health.
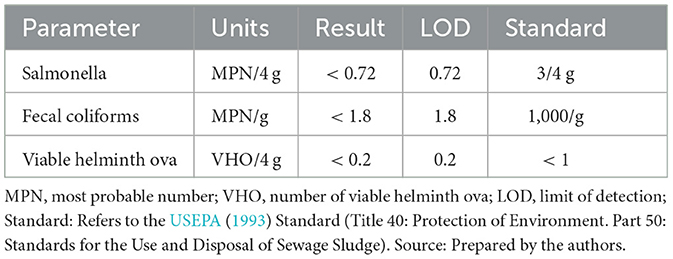
Table 9. Values obtained in the microbiological parameters salmonella, total coliforms, and viable helminth ova in the characterization of the biosolid applied in the fertilization of the experimental blocks in the study.
3.3 Nutritional composition of bananas as affected by biosolids and an organic animal fertilizer
The results of the nutritional composition of the banana fertilized with different doses of biosolid and with the organic fertilizer (poultry litter) are shown in Table 10. The highest content of total sugars, carbohydrates, magnesium and potassium was obtained in Block 5 where Y-dose fertilization of the biosolid was applied. The Y-dose of the biosolid without application of the plant protection compound (Block 5) and with crop protection compound (Block 7) showed the highest energy values of 50.8 and 54.8 Kcal/100 g, respectively. As for the protein content, it was higher in the block fertilized with poultry litter (Block 2). The moisture content was lower in the blocks where the Y-dose of the biosolid was applied (Block 5 and Block 7), but slightly higher in the blocks where the poultry litter were applied (Block 2) and in the blocks with X-dose of the biosolid (Block 4 and Block 6).
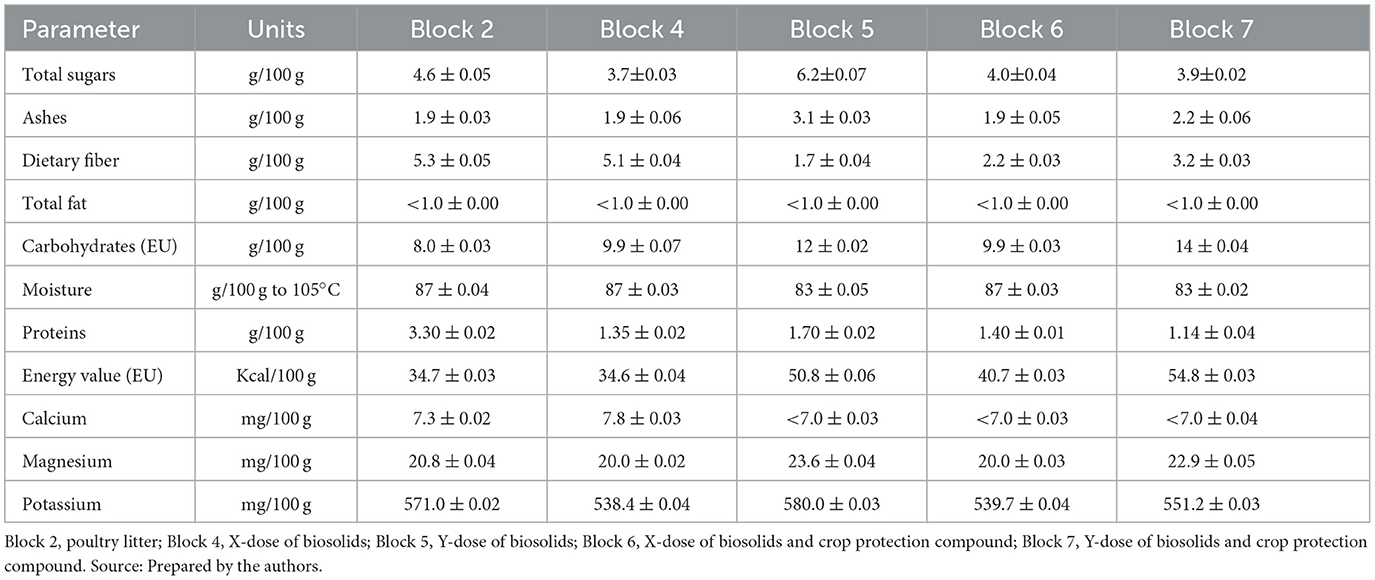
Table 10. Nutritional composition of the banana under fertilization in different experimental blocks.
An ANOVA analysis was performed in Table 11. The results indicate that there are significant differences in the nutritional parameters between the banana crop blocks for the variables of sugars, dietary fiber, total fats, carbohydrates, proteins, calcium, magnesium, and potassium, as evidenced by the significant values of p (p < 0.05) from the ANOVA test. The Shapiro–Wilk test suggests that the data for most parameters follow an approximately normal distribution, except for dietary fiber and protein, which show deviations from normality (p < 0.05). These deviations from normality must be taken into account when interpreting the ANOVA results.
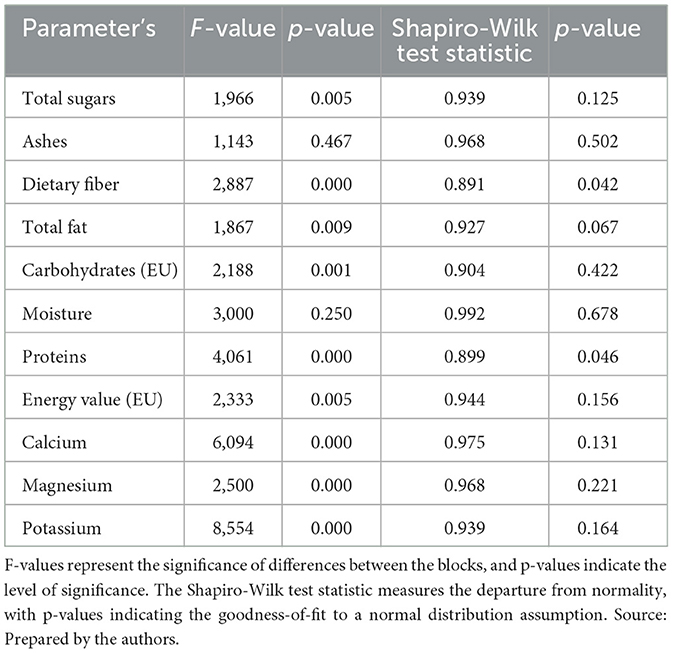
Table 11. Results of ANOVA and Shapiro-Wilk Test for Nutritional Parameters in Banana Cultivation Blocks.
When comparing by cluster analysis the nutritional composition of the banana in the different Blocks (Figure 2), it was observed that Blocks 5 and 7 containing the Y-dose without crop protection compound (Block 5) and with crop protection compound (Block 7) show nutritional compositions of the fruit more similar to each other (greater value of the Morisita similarity index), forming a more homogeneous first cluster (Cluster A) widely separated from the other blocks. Meanwhile, the analysis puts Blocks 2, 4 and 6 into a second cluster (Cluster B), which showed similar nutritional compositions. Although the nutritional composition of the blocks in this cluster is similar, some separation of Cluster 2 from the other two blocks was observed. Block 2 represents the treatment with application of fertilization with poultry litter. In summary, it can be noted that the nutritional composition of the banana plants in Blocks (5 and 7), where the Y-dose of the biosolid (with and without crop protection compound) was applied, differs significantly from the nutritional composition of the banana plants in Blocks (2, 4, and 6) where the X-dose of the biosolid (with and without crop protection compound without fertilization) was applied and where an organic animal-based fertilizer (poultry litter) was applied.
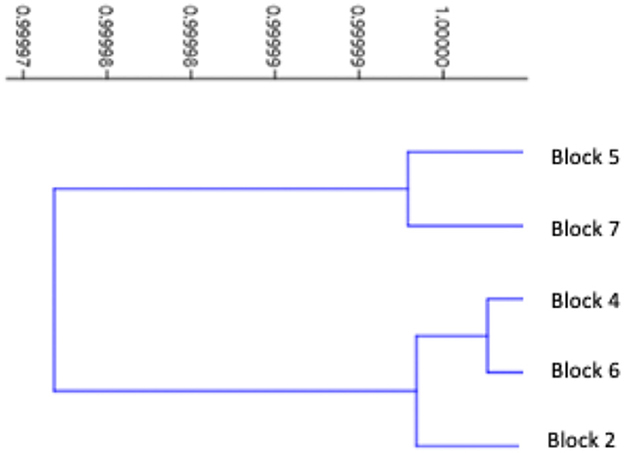
Figure 2. Dendrogram showing the cluster analysis of the blocks with application of different doses of biosolid and organic animal-based fertilizer. The clusters are formed according to the similarity in the nutritional composition of the banana plants in the different fertilization treatments of each experimental block. The Morisita index was used to estimate the similarity between the different blocks.
4 Discussion
4.1 Characterization of soil
The analysis of soil parameters revealed significant variations in the concentrations of nitrogen, phosphorus, and potassium between the pre-sowing period and the analysis prior to harvesting. Comparing the results to the control block, it was observed that the application of gallinase and biosolids significantly increased nitrogen levels by 28.9 and 12.3–32.9%, respectively. Phosphorus levels also showed a significant increase with the application of chicken manure by 25.9% and, more notably, with the application of biosolids by 42.5–51.2%. The variation in potassium levels between block 1 and the other blocks also showed an increase. These findings highlight the effectiveness of biosolids and other organic fertilizers in improving the nutrient content of the soil and subsequently enhancing the nutritional composition of bananas. The application of biosolids, in particular, demonstrated significant increases in nitrogen and phosphorus levels, which are essential for the growth and development of banana plants (Leonel et al., 2020). This suggests that the use of biosolids as an organic fertilizer can contribute to the optimization of soil fertility and nutrient availability, ultimately leading to improved crop yields. In relation to heavy metals, there are no studies in the country to be able to compare our values. However, and in comparison with the different standards for heavy metal concentrations in the soil, the levels of metals found in the soil of the experimental area were all below the global limits (Goni et al., 2014; Wang et al., 2016).
4.2 Characterization of the biosolid
The concentrations of heavy metals in the biosolid were found to be below the limits established in the relevant standards. This is significant because it ensures that potential issues such as toxicity to plants (Sidhu et al., 2019), accumulation of heavy metals in plants (Pan et al., 2021), water and environmental contamination (Mansilla et al., 2021), and non-compliance with regulations and standards are effectively controlled when using these biosolids. Furthermore, the concentrations of pesticides, herbicides, and volatile/non-volatile organic compounds were below the detection limits of the methods used and also below the limits set by the standards. The results of the characterization of the biosolid used in the fertilization of the experimental culture indicate that it meets the required standards and regulations for agricultural use. The pH value of the biosolid is 5.60, indicating a slightly acidic nature. The moisture content is 59%, suggesting a relatively high-water content in the biosolid.
The obtained macro and micronutrient concentrations in the biosolid are sufficient to meet the required doses for banana plantations. Specifically, the high levels of nitrogen and potassium in the biosolid adequately fulfill the crop's nutrient requirements without the need for additional inorganic fertilizers. Compared to other crops, banana plants have relatively high nutrient demands, necessitating the application of substantial amounts of potassium and nitrogen to replenish exported nutrients per fruit harvest (average: N 1.66 kg Mg−1, P2O5 0.36 kg Mg−1, and K2O 4.33 kg Mg−1; Ratke et al., 2012; Nomura et al., 2016). According to studies conducted in Latin America, recommended fertilization doses for banana plants based on soil nutrient levels are approximately 600–700 kg K2O/ha/year for soils with low potassium content, 100 kg P2O5/ha/year for phosphorus, and 350–400 kg/ha/year for nitrogen (López and y Espinoza, 1995).
Therefore, the results indicate that the biosolids exhibit highly favorable characteristics for their utilization in banana cultivation in the Dominican Republic. They provide essential nutrients that are vital for the optimal growth and development of banana plants. Moreover, their application can enhance soil structure, improving water retention capacity and aeration (Adhikari et al., 2022). This, in turn, promotes root growth, enhances plant resilience against diseases, and adverse weather conditions (Sorinolu et al., 2021), and ultimately contributes to the production of fruits with optimal nutritional levels (Eid et al., 2021). Furthermore, employing biosolids in banana cultivation aligns with the principles of sustainable agriculture by responsibly utilizing and recycling resources.
4.3 Comparative analysis of banana nutrients through fertilization with biosolids
An important factor in the study of banana composition is moisture (Fernández-Cruz et al., 2021). The moisture content of banana fruit can be influenced by factors such as the level of ripeness and the banana variety (Ogbonna et al., 2016). Ripe bananas, which contain ~80% moisture, are highly susceptible to post-harvest losses and weight loss during transport and storage (Abano and Sam-Amoah, 2011). Worldwide, moisture values can range between 58.27 and 85%, depending on the banana variety and ripeness level (Arvanitoyannis and Mavromatis, 2009; Hapsari and Lestari, 2016). In our study, the moisture values ranged between 83 and 87%, slightly above the average (76.0%) reported for fruits from the Canary Islands (Spain), Tenerife (Spain), Ecuador, and Colombia (Arvanitoyannis and Mavromatis, 2009). It is worth noting that Blocks 5 and 7 in our study correspond to the application of the Y-dose of biosolid.
The ash content was higher in Block 5 (3.1 g/100 g), which received the Y-dose fertilization of biosolid. The ash content tends to increase in the early stages of ripening, particularly in fruits intended for cooking and in certain varieties, with values ranging between 16.9 and 27.1% (Arvanitoyannis and Mavromatis, 2009). Our results exceed the range (0.66–1.45 g/100 g) reported for 15 banana varieties (Dotto et al., 2019) and the average ash content in bananas from the Canary Islands (1%; Fernández-Cruz et al., 2021). Other studies have reported ash content values ranging from 2.89 to 3.75 g/100 g (Pragati et al., 2014). The variations in ash content among different banana varieties are attributed to their differential mineral-absorbing abilities. The higher ash content observed in bananas fertilized with the Y-dose of biosolid suggests a higher mineral content compared to bananas fertilized with poultry litter or the X-dose of biosolid. This observation is consistent with the higher concentrations of potassium and magnesium found in Block 5 with the Y-dose application of biosolid.
Carbohydrates are considered the most important nutrients in bananas due to their abundance, with concentrations ranging between 10.2 and 26.5 g/100 g (Fernández-Cruz et al., 2021). In our study, the highest carbohydrate values were observed in bananas from Block 5 (Y-dose fertilization of biosolid) and Block 7 (Y-dose fertilization of biosolid plus the addition of a crop protection compound), with 12 and 14 g/100 g, respectively. These values fall within the range reported for the nutrient composition of different banana varieties mentioned earlier. Although an average value of 20 g/100 g of carbohydrates has been reported in many varieties (Aurore et al., 2009), our values are lower than the average. It is known that bananas in the initial ripening stages, grade 1 or 2, tend to have a high starch content, which decreases significantly as the ripening process progresses (Fernández-Cruz et al., 2021). This may explain the low carbohydrate values obtained in our study.
Our results indicate that the total sugar content in bananas is higher (6.2 g/100 g) in treatments with the Y-dose of biosolid (Block 5). Similar to our study, other research shows that the total sugar content is below 16 g (11.92–15.91 g; Fernández-Cruz et al., 2021). This suggests that total sugar levels depend on the specific banana variety analyzed.
Dietary fiber content was higher (5.3 g/100 g) in Block 2, which received poultry litter fertilization, while it was lower (1.7 g/100 g) in Block 5 with the Y-dose of biosolid. In a study conducted in the Canary Islands, the dietary fiber content of six banana varieties ranged from 2 to 5.3 g/100 g (Fernández-Cruz et al., 2021), and our value falls within this reported range. Fats were not detectable in all blocks and were similar to the values reported for other banana varieties, which are below 1 g/100 g. Protein concentrations were higher (3.30 g/100 g) in Block 2 (poultry litter fertilization) and lower (1.14–1.70 g/100 g) in the blocks with the application of biosolid as fertilizer (Blocks 4, 5, 6, and 7). It has been reported that protein concentrations in bananas generally do not exceed 2 g (1.09–1.92 g/100 g; Fernández-Cruz et al., 2021). In our case, only the treatment of poultry litter fertilization slightly exceeds the reported value. Overall, protein levels in bananas fertilized with biosolids are similar to those reported in literature (Arvanitoyannis and Mavromatis, 2009; Aurore et al., 2009; Fernández-Cruz et al., 2021).
The energy value of bananas was considerably higher (58–54.8 kcal/100 g) in the blocks with the application of the Y-dose of biosolid compared to other blocks (Block 2, Block 4, and Block 6). The range reported in literature for the energy value of different banana varieties is from 89 to 105.3 kcal/100 g (Arvanitoyannis and Mavromatis, 2009; Aurore et al., 2009). The highest values obtained in our study are below the reported range, which aligns with the low values of total sugars and carbohydrates observed in the bananas analyzed. As mentioned earlier, this may be attributed to the dependence of total sugars and carbohydrates on the specific banana variety analyzed and the ripeness stage of the fruit.
Mineral content in bananas was relatively high across all blocks, particularly for magnesium and potassium. The calcium concentration in our study (7–7.3 g/100 g) falls within the reported range (4–20 mg/100 g) and is very close to the calcium value (6.2 mg/100 g) reported for the Gran Enana variety from Ecuador (Arvanitoyannis and Mavromatis, 2009). Magnesium is also present in significant amounts in bananas (Fernández-Cruz et al., 2021). In our study, the magnesium values in the different blocks were very similar (20.0–23.6 g/100 g). However, the highest values were obtained in Blocks 5 (23.6 mg/100 g) and 7 (22.9 mg/100 g), where the Y-dose of the biosolid was applied. Nevertheless, the magnesium values in our study were slightly lower than the values reported in literature (27−41.2 mg/100 g; Arvanitoyannis and Mavromatis, 2009; Fernández-Cruz et al., 2021). Notably, potassium content was quite high (538.4–580 mg/100 g) in all the blocks studied, with Block 5 (fertilization with the Y-dose of the biosolid) resulting in the highest potassium values (580 mg/100 g) in the bananas. These potassium values exceed the range reported for other varieties (275–534 mg/100 g; Fernández-Cruz et al., 2021). Potassium is one of the essential minerals with the highest concentration in the biosolid, and the concentrations of other important minerals (Ca, Mg, Cu, Fe, Na, Zn, Cu) suggest that the biosolid can adequately meet the nutritional requirements of banana plantations.
5. Conclusions
The application of biosolids as organic fertilizer in banana cultivation has significant benefits for soil fertility and nutrient availability. The analysis of soil parameters revealed that the use of biosolids increased nitrogen, phosphorus, and potassium levels in the soil, which are essential for the growth and development of banana plants. Biosolids demonstrated particularly effective results in increasing nitrogen and phosphorus concentrations. The biosolid used in the study met the required standards and regulations for agricultural use, with low concentrations of heavy metals, pesticides, herbicides, and organic compounds.
When comparing the nutrient composition of bananas grown with biosolids to other treatments, several observations were made. The bananas fertilized with biosolids showed higher ash content, indicating a higher mineral content, especially potassium and magnesium. Carbohydrate levels were within the reported range, while total sugar levels varied depending on the banana variety analyzed. Dietary fiber content varied among treatments, and fats were not detected in any blocks. Protein levels in bananas fertilized with biosolids were similar to reported values, while the energy value was lower compared to other banana varieties. Mineral content, particularly magnesium and potassium, was relatively high in all blocks, with the highest potassium values observed in bananas grown with biosolids. The use of dose Y of the biosolid, characterized by the application of 16 kilograms of biosolid/plant, is the most recommended to fertilize the banana, since the collected fruits presented the most optimal nutritional values.
One of the limitations of the research is the collection of data only once, in the first harvest. Furthermore, it is necessary to investigate the accumulation of heavy metals after using biosoils. In this context, for future lines of research, it is recommended to analyse the behavior of heavy metals in the soil after applying biosolids generated in the Dominican Republic.
Data availability statement
The raw data supporting the conclusions of this article will be made available by the authors, without undue reservation.
Author contributions
AM designed the survey, was responsible for writing the introduction and literature review, and was in charge of reviewing the structure and final content of the article. VR was in charge of applying the questionnaire to the sample, writing the methodology, and tabulating and analyzing the data. JC wrote the results and conclusions. All authors validated the results and the final paper. All authors contributed to the article and approved the submitted version.
Funding
This research was funded by National Fund for Innovation and Scientific and Technological Development (FONDOCyT) 2018–2019 (Ministry of Higher Education, Science and Technology, MESCyT, of the Dominican Republic). Grant number 2018-2019-2A1-026/077-2019. The APC was funded by FONDOCyT.
Acknowledgments
The authors of this work want to thank the Ministry of Higher Education, Science and Technology (MESCyT) of the Dominican Republic, who supported the research project Comparative model of the behavior of banana varieties with a vocation for export using biosolids as fertilizer, to through the National Fund for Innovation and Scientific and Technological Development (FONDOCyT) 2018-2019.
Conflict of interest
The authors declare that the research was conducted in the absence of any commercial or financial relationships that could be construed as a potential conflict of interest.
Publisher's note
All claims expressed in this article are solely those of the authors and do not necessarily represent those of their affiliated organizations, or those of the publisher, the editors and the reviewers. Any product that may be evaluated in this article, or claim that may be made by its manufacturer, is not guaranteed or endorsed by the publisher.
References
Abano, E. E., and Sam-Amoah, L. K. (2011). Effects of different pretreatments on drying characteristics of banana slices. ARPN J. Eng. Appl. Sci. 6, 121–129.
Adhikari, S., Timms, W., and Mahmud, M. P. (2022). Optimising water holding capacity and hydrophobicity of biochar for soil amendment–A review. Sci. Tot. Environ. 2022:158043. doi: 10.1016/j.scitotenv.2022.158043
Al-Gheethi, A. A., Efaq, A. N., Bala, J. D., Norli, I., Abdel-Monem, M. O., and Kadir, M. A. (2018). Removal of pathogenic bacteria from sewage-treated effluent and biosolids for agricultural purposes. Appl. Water Sci. 8, 74–99. doi: 10.1007/s13201-018-0698-6
Aluru, M., Xu, Y., Guo, R., Wang, Z., Li, S., White, W., et al. (2008). Generation of transgenic maize withenhanced provitamin A content. J. Exp. Bot. 59, 3551–3562. doi: 10.1093/jxb/ern212
Arias, H. (1984). Respuesta del Banano (Musa AAA) Subgrupo Cavendish “Gran Enano” a Dosis Creciente de Sulfato de Potasio en un Suelo Oxyc Dystropets de Río Jimenez, Provincia de Limon. Tesis Ingeneniería Agronómica. Facultad de Agronomía, Universidad de Costa Rica, 89.
Arvanitoyannis, I. S., and Mavromatis, A. (2009). Banana cultivars, cultivation practices, and physicochemical properties. Crit. Rev. Food Sci. Nutr. 49, 113–135. doi: 10.1080/10408390701764344
Aurore, G., Parfait, B., and Fahrasmane, L. (2009). Bananas, raw materials for making processed food products. Trends Food Sci. Technol. 20, 78–91. doi: 10.1016/j.tifs.2008.10.003
Bastida, F., Kandeler, E., Hernández, T., and García, C. (2008). Long-term effect of municipal solid waste amendment on microbial abundance and humus-associated enzyme activities under semiarid conditions. Microb. Ecol. 55, 651–661. doi: 10.1007/s00248-007-9308-0
Broderick, S. R., and Evans, W. B. (2017). Biosolids promote similar plant growth and quality responses as conventional and slow-release fertilizers. HortTechnology 27, 794–804. doi: 10.21273/HORTTECH03639-17
Castellanos-Rozo, J., Galvis, J., Manjarres, E., and Merchán-Castellanos, N. (2022). Biosolids as fertilizer in the tomato crop. Rev. Facult. Agron. 2:e223931. doi: 10.47280/RevFacAgron(LUZ).v39.n2.09
Cheng, S. F., Chen, J. W., Yen, H. M., Huang, C. Y., Cheng, T. C., Chen, J. R., et al. (2013). Characteristics and reuse potential as fertilizer based on heavy metal concentrations in domestic wastewater biosolids (Taiwan). Adv. Mater. Res. 647, 374–380. doi: 10.4028/www.scientific.net/AMR.647.374
Cobeña-Loor, N. V., Espinosa-Marroquin, J. A., Avellán-Vásquez, L. E., Cedeño-Zambrano, J. R., Vaca-Sotelo, D. A., Chica-Chica, D. M., et al (2020). Nutrición Vegetal, Exportación y Eficiencia del uso de Nutrientes en Plátano. Alicante, España, Ciencias, Editorial Área de Innovación y Desarrollo. Available online at: https://www.3ciencias.com/wp-content/uploads/2020/03/Nutrici%C3%B3n-vegetal-exportaci%C3%B3n-y-eficiencia-del-uso-de-nutrientes-en-pl%C3%A1tano.pdf doi: 10.17993/IngyTec.2020.60
Cruzate, G. A., and Casas, R. (2009). Extracción de nutrientes en la agricultura argentina. Inform. Agron. Cono Sur IPNI 44, 21–26.
Cunningham, C.J., Kuyukina, M.S., Ivshina, I.B., Konev, A.I., Peskur, T.A., and Knapp, C.W. (2020). Potential risks of antibiotic resistant bacteria and genes in bioremediation of petroleum hydrocarbon contaminated soils. Environ. Sci. Process Impacts. 22, 1110–1124. doi: 10.1039/C9EM00606K
Dotto, J., Matemu, A. O., and Ndakidemi, P. A. (2019). Nutrient composition and selected physicochemical properties of fifteen Mchare cooking bananas: a study conducted in northern Tanzania. Sci. Afr. 6:e00150. doi: 10.1016/j.sciaf.2019.e00150
Echeverry-Navarro, E. (2001). Fertilización orgánica vs fertilización inorgánica de plátano Cachaco común en Colombia. Infomusa 10, 7–10.
Eid, E., Shaltout, K., Alamri, S., Alrumman, S., Hussain, A., Sewelam, N., et al. (2021). Sewage sludge enhances tomato growth and improves fruit-yield quality by restoring soil fertility. Plant Soil Environ. 67, 514–523. doi: 10.17221/205/2021-PSE
Fernández-Cruz, E., López Plaza, B., Santurino, C., and Gómez Candela, C. (2021). Composición nutricional y declaraciones nutricionales del plátano de Canarias. Nutr. Hospit. 38, 1248–1256. doi: 10.20960/nh.03614
Gamez, R., Cardinale, M., Montes, M., Ramirez, S., Schnell, S., and Rodriguez, F. (2019). Screening, plant growth promotion and root colonization pattern of two rhizobacteria (Pseudomonas fluorescens Ps006 and Bacillus amyloliquefaciens Bs006) on banana cv. Williams (Musa acuminata Colla). Microbiol. Res. 220, 12–20. doi: 10.1016/j.micres.2018.11.006
Gebert, S. (2010). Assessing ecological impacts of land-applied municipal biosolids: effects of run-off and tile drainage on the aquatic organisms daphnia magna, hyalella azteca, and lemna minor (Master's thesis). Ryerson University, Ontario, ON, Canada.
Goni, M. A., Ahmad, J. U., Halim, M. A., Mottalib, M. A., and Chowdhury, D. A. (2014). Uptake and translocation of metals in different parts of crop plants irrigated with contaminated water from DEPZ area of Bangladesh. Bull. Environ. Contam. Toxicol. 92, 726–732. doi: 10.1007/s00128-014-1264-z
Hapsari, L., and Lestari, D. A. (2016). Fruit characteristic and nutrient values of four Indonesian banana cultivars (Musa spp.) at different genomic groups. Agrivita 38, 303–311. doi: 10.17503/agrivita.v38i3.696
Hernández, M. (1985). Respuesta del Banana Clon “Gran Enano” a la Fertilización Potásica en un suelo Typic Dystropets de Cariari, Cantón Pococí. Tesis de Ingeniería Agronómica. Facultad de Agronomía, Universidad de Costa Rica.
Hogue, C. (2018). Data lacking on safety of treated sewage sludge. Chem. Eng. News 96:17. doi: 10.1021/cen-09648-polcon2
Jordán, M. M., Almendro-Candel, M. B., García-Sánchez, E., and Navarro-Pedreño, J. (2022). The lixiviation of metals when amending agricultural soil of the mediterranean basin with biosolids: trials in leaching columns. Int. J. Environ. Res. Public Health 19:13736. doi: 10.3390/ijerph192113736
Judy, J. D., McNear, D. H. Jr, Chen, C., Lewis, R. W., Tsyusko, O. V., Bertsch, P. M., et al. (2015). Nanomaterials in biosolids inhibit nodulation, shift microbial community composition, and result in increased metal uptake relative to bulk/dissolved metals. Environ. Sci. Technol. 49, 8751–8758. doi: 10.1021/acs.est.5b01208
Junqueira-Teixeira, L. A. J. (2005). Topicos de Nutriçao e Adubaçao de Bananeira, En Reuniao Itinerante de Fitossanidade do Instituto Biológico, XIII, Registro, SP. Cultura da Banana. Sao Paulo: Instituto Biológico, 79–94.
Khalid, S., Shahid, M., Niazi, N. K., Murtaza, B., Bibi, I., and Dumat, C. (2017). A comparison of technologies for remediation of heavy metal contaminated soils. J. Geochem. Explor. 182, 247–268. doi: 10.1016/j.gexplo.2016.11.021
Khawas, P., Das, A. J., Sit, N., Badwaik, L. S., and Deka, S. C. (2014). Nutritional composition of culinary Musa ABB at different stages of development. Am. J. Food Sci. Technol. 3, 80–87. doi: 10.12691/ajfst-2-3-1
Lahav, E. (1995). “Banana nutrition,” in Bananas and Plantains, World Crop Series, ed S. En Gowen (Dordrecht: Chapman and Hall), 258–316. doi: 10.1007/978-94-011-0737-2_11
Latare, A. M., Kumar, O., Singh, S. K., and Gupta, A. (2014). Direct and residual effect of sewage sludge on yield, heavy metals content and soil fertility under rice–wheat system. Ecol. Eng. 69, 17–24. doi: 10.1016/j.ecoleng.2014.03.066
Law, A., Solano, O., Brown, C. J., Hunter, S. S., Fagnan, M., Top, E. M., et al. (2021). Biosolids as a source of antibiotic resistance plasmids for commensal and pathogenic bacteria. Front. Microbiol. 12:606409. doi: 10.3389/fmicb.2021.606409
Leonel, M., Bolfarini, A. C. B., da Silva, M. J. R., Souza, J. M. A., and Leonel, S. (2020). Banana fruits with high content of resistant starch: effect of genotypes and phosphorus fertilization. Int. J. Biol. Macromol. 150, 1020–1026. doi: 10.1016/j.ijbiomac.2019.10.217
López, A. (1994). Interpretación de los Análisis Químicos de Suelos y Foliares en el Cultivo del Banano (Musa AAA, Cv Valery) en Costa Rica. Análisis de un Caso y Factores Involucrados. En Resúmenes del XI ACORBAT. San José, Costa Rica.
López, A., and y Espinoza, J. (1995). Manual de Nutrición y Fertilización del Banano. INFOPOS. Quito-Ecuador.
Mansilla, S., Portugal, J., Bayona, J. M., Matamoros, V., Leiva, A. M., Vidal, G., et al. (2021). Compounds of emerging concern as new plant stressors linked to water reuse and biosolid application in agriculture. J. Environ. Chem. Eng. 9:105198. doi: 10.1016/j.jece.2021.105198
Martínez-Cardozo, C., Cayón-Salinas, G., and Ligarreto-Moreno, G. (2016). Composición química y distribución de materia seca del fruto en genotipos de plátano y banano. Corp. Cienc. Tecnol. Agropec. 17, 217–227. doi: 10.21930/rcta.vol17_num2_art:491
Mattana, S., Petrovičová, B., Landi, L., Gelsomino, A., Cortés, P., Ortiz, O., et al. (2014). Sewage sludge processing determines its impact on soil microbial community structure and function. Appl. Soil Ecol. 75, 150–161. doi: 10.1016/j.apsoil.2013.11.007
Munoz, G., Michaud, A. M., Liu, M., Vo Duy, S., Montenach, D., Resseguier, C., et al. (2021). Target and nontarget screening of PFAS in biosolids, composts, and other organic waste products for land application in France. Environ. Sci. Technol. 56, 6056–6068. doi: 10.1021/acs.est.1c03697
Ndukwe, O. O., Muoneke, C. O., Baiyeri, K. P., and Tenkouano, A. (2012). Effect of organic and inorganic fertilizer on nutrient concentrations in plantain (Musa spp.) fruit pup. Afr. J. Biotechnol. 11, 1651–1658. doi: 10.5897/AJB11.2278
Nomura, E. S., Cuquel, F. L., Damatto, E. R., Fuzitani, E. J., Borges, A. L., and Saes, L. A. (2016). Nitrogen and potassium fertilization on ‘Caipira' and ‘BRS Princesa' bananas in the Ribeira Valley. R. Bras. Eng. Agric. Ambiental. 20, 702–708. doi: 10.1590/1807-1929/agriambi.v20n8p702-708
Ogbonna, O. A., Ohia, G. U., Ikeyi, A. P., and Okoye, N. H. (2016). Glycoside, moisture and dry matter compositions of fruits of three Musa species at three stages of development. J. Pharm. Biol. Sci. 11, 60–67.
Okoffo, E. D., Tscharke, B. J., O'Brien, J. W., O'Brien, S., Ribeiro, F., Burrows, S. D., et al. (2020). Release of plastics to Australian land from biosolids end-use. Environ. Sci. Technol. 54, 15132–15141. doi: 10.1021/acs.est.0c05867
Örmeci, B. (2014). Comment on “Determining the ecological impacts of organic contaminants in biosolids using a high-throughput colorimetric denitrification assay: a case study with antimicrobial agents”. Environ. Sci. Technol. 48, 12469–12469. doi: 10.1021/es503333m
Pan, M., Yau, P. C., Lee, K. C., Zhang, H., Lee, V., Lai, C. Y., et al. (2021). Nutrient accumulation and environmental risks of biosolids and different fertilizers on horticultural plants. Water Air Soil Pollut. 232:480. doi: 10.1007/s11270-021-05424-5
Pérez-López, C. (2004). Técnicas de Análisis Multivariante de Datos. Madrid: Pearson Educación, S.A., 672.
Ploughe, L. W., Akin-Fajiye, M., Gagnon, A., Gardner, W. C., and Fraser, L. H. (2021). Revegetation of degraded ecosystems into grasslands using biosolids as an organic amendment: a meta-analysis. Appl. Vegetat. Sci. 24:e12558. doi: 10.1111/avsc.12558
Pragati, S., Genitha, I., and Ravish, K. (2014). Comparative study of ripe and unripe banana flour during storage. J. Food Process. Technol. 5, 1–6. doi: 10.4172/2157-7110.1000384
Quintero, R. (1995). “Fertilización y nutrición,” in El Cultivo de la Caña en la Zona Azucarera de Colombia, eds D. En Cassalett, A. Torres, and E. Isaacs (Cali: CENICAÑA), 153–177.
Ramesh, A., and Ostad-Ali-Askari, K. (2023a). Effects of magnetized municipal effluent on some physical properties of soil in furrow irrigation. Appl. Water Sci. 13:26. doi: 10.1007/s13201-022-01811-3
Ramesh, A., and Ostad-Ali-Askari, K. (2023b). Effect of effluent and magnetized effluent on Manning roughness coefficient in furrow irrigation. Appl. Water Sci. 13:21. doi: 10.1007/s13201-022-01818-w
Ramos-Agüero, D., Terry-Alfonso, E. T., Soto-Carreño, F., Adriano Cabrera-Rodríguez, A., Gloria, M., Martín-Alonso, G. M., et al. (2016). Respuesta del cultivo del plátano a diferentes proporciones de suelo y bocashi, complementadas con fertilizante mineral en etapa de vivero. Cult. Trop. 37, 165–174.
Ratke, R. F., Santos, S. C., Pereira, H. S., Souza, E. D., and Carneiro, M. A. C. (2012). Desenvolvimento e produção de bananeiras Thap Maeo e Prata-Anã com diferentes níveis de adubação nitrogenada e potássica. Rev. Bras. Frutic. 34, 277–288. doi: 10.1590/S0100-29452012000100037
Sánchez-Murcia L. Ñañez-Muñoz Y. L. (2021). Evaluación Físico–Química de Frutos de Banano (Musa paradisiaca), Variedad Banana Gros Michel (Musa acuminata, AAA Group) con Fertilización Orgánica vs Química en la Finca Australia, Vereda la Honda Municipio de Pitalito – Huila. (Tesis de Grado). Universidad Nacional Abierta y a Distancia UNAD, Escuela de Ciencias Agrícolas, Pecuarias y del Medio Ambiente, Programa de Agronomía. Huila: Recuperado de Available online at: https://repository.unad.edu.co/handle/10596/41394
Shanableh, A., and Ginige, P. (1999). Impact of metals bioleaching on the nutrient value of biological nutrient removal biosolids. Water Sci. Technol. 39, 175–181. doi: 10.2166/wst.1999.0292
Sharma, B., Sarkar, A., Singh, P., and Singh, R. P. (2017). Agricultural utilization of biosolids: a review on potential effects on soil and plant grown. Waste Manage. 64, 117–132. doi: 10.1016/j.wasman.2017.03.002
Sharma, K., and Garg, V. K. (2019). “Vermicomposting of waste: a zero-waste approach for waste management,” in Sustainable Resource Recovery and Zero Waste Approaches, eds M. J. Taherzadeh, K. Bolton, J. Wong, and A, Pandey (St. Louis, MO: Elsevier), 133–164. doi: 10.1016/B978-0-444-64200-4.00010-4
Sidhu, H., O'Connor, G., and Kruse, J. (2019). Plant toxicity and accumulation of biosolids-borne ciprofloxacin and azithromycin. Sci. Tot. Environ. 648, 1219–1226. doi: 10.1016/j.scitotenv.2018.08.218
Sorinolu, A. J., Tyagi, N., Kumar, A., and Munir, M. (2021). Antibiotic resistance development and human health risks during wastewater reuse and biosolids application in agriculture. Chemosphere 265:129032. doi: 10.1016/j.chemosphere.2020.129032
Sun, B., Kallenbach, C. M., Boh, M. Y., Clark, O. G., and Whalen, J. K. (2022). Enzyme activity after applying alkaline biosolids to agricultural soil. Can. J. Soil Sci. 103, 372–376. doi: 10.1139/cjss-2022-0056
Türkdogan, M. K., Kilicel, F., Kara, K., Tuncer, I., and Uygan, I. (2003). Heavy metals in soil, vegetables and fruits in the endemic upper gastrointestinal cancer region of Turkey. Environ. Toxicol. Pharmacol. 13, 175–179. doi: 10.1016/S1382-6689(02)00156-4
Wang, F., Wang, Z., Kou, C., Ma, Z., and Zhao, D. (2016). Responses of wheat yield, macro-and micro-nutrients, and heavy metals in soil and wheat following the application of manure compost on the North China Plain. PLoS ONE 11:e0146453. doi: 10.1371/journal.pone.0146453
Keywords: banana, biofertilizer, biosolids, nutrients, organic fertilization
Citation: Martínez Durán AJ, Rodríguez Núñez VA and Castillo Jáquez JdC (2023) Use of biosolids from wastewater treatment plants and other organic fertilizers in agriculture—a preliminary results of a case study in banana cultivation in the Dominican Republic. Front. Water 5:1236924. doi: 10.3389/frwa.2023.1236924
Received: 08 June 2023; Accepted: 24 July 2023;
Published: 07 August 2023.
Edited by:
Abdelazim Negm, Zagazig University, EgyptReviewed by:
Vineet Kumar, Central University of Rajasthan, IndiaYonglan Tian, North China Electric Power University, China
Kaveh Ostad-Ali-Askari, American University in Dubai, United Arab Emirates
Copyright © 2023 Martínez Durán, Rodríguez Núñez and Castillo Jáquez. This is an open-access article distributed under the terms of the Creative Commons Attribution License (CC BY). The use, distribution or reproduction in other forums is permitted, provided the original author(s) and the copyright owner(s) are credited and that the original publication in this journal is cited, in accordance with accepted academic practice. No use, distribution or reproduction is permitted which does not comply with these terms.
*Correspondence: José del C. Castillo Jáquez, amNhc3RpbGxvQHV0ZXNhLmVkdQ==