- 1Earth and Environmental Sciences, Lawrence Berkeley National Laboratory, Berkeley, CA, United States
- 2Institute of Hydrobiology and Aquatic Ecosystems Management, BOKU University, Vienna, Austria
- 3Department of Geosciences, Colorado State University, Fort Collins, CO, United States
Editorial on the Research Topic
Investigating connectivity to advance the predictive understanding of watershed processes and the Earth's critical zone
Hydrologic connectivity shapes watershed processes by regulating water, sediment, and nutrient movement, integrating the Earth's critical zone processes, and linking the hydrosphere with other physical and ecological “landscape” compartments. However, connectivity has traditionally been studied independently in hydrology, biogeochemistry, and geomorphology; as a result, these efforts have lacked a unified framework. Recognizing this challenge, the 2015 Geomorphology Symposium on Connectivity (Wohl et al., 2017) was not just another discussion on connectivity, but also one of the pivotal moments fostering interdisciplinary dialogue that emphasized the need for a more integrated approach. The 2015 Geomorphology Symposium on Connectivity highlighted key themes such as sediment, hydrologic, geochemical, riverine, and landscape connectivity as well as connectivity modeling—concepts that have often remained siloed within their respective disciplines. Since this symposium, significant progress in modeling, observations, and technologies has expanded the scope and depth of hydrologic connectivity research across scales. However, its full potential remains underutilized in catchment science, emphasizing the need to synthesize existing research and bridge critical knowledge gaps (see Wohl et al., 2019 and references therein). This Research Topic in Frontiers in Water addresses challenges in hydrologic connectivity by integrating key developments, theoretical innovations, data-driven methods, and interdisciplinary perspectives. The contributions span four key themes, as outlined in the following paragraphs.
Conceptual and theoretical frameworks
The studies presented here examine the conceptual framework of hydrologic connectivity by integrating interdisciplinary perspectives, refining models, and incorporating emerging technologies to enhance predictability and management strategies. Dwivedi et al. synthesized current knowledge from hydrology, ecology, biogeochemistry, and geomorphology, offering a more comprehensive framework for connectivity research. Their study categorized connectivity according to spatial domains (surface, subsurface, and surface-subsurface) and connectivity dimensions (longitudinal, lateral, and vertical). They argued that, although analyzing connectivity by spatial domains, connectivity dimensions, or temporal scale is practical, its full impact on catchment dynamics is best understood holistically, yet many resource management strategies fail to incorporate this integrated perspective. They further emphasized that incorporating emerging technologies such as artificial intelligence, graph theory, and entropy-based metrics into improved measurement and modeling approaches can address critical gaps in understanding connectivity dynamics, particularly the inadequate spatial and temporal coverage.
These researchers proposed an integrated, system-based approach to hydrologic connectivity, linking key processes across disciplines. They suggested that simple analogies, such as the electric circuit analogy, can help interdisciplinary integration, illustrating how water flow, resistance, and storage interact within a system. In this analogy, gravitational potential drives water movement like voltage in an electrical circuit (Figure 1). At the same time, obstacles such as vegetation and rocks act as resistors; meanwhile, wetlands function as capacitors for temporary water storage, and groundwater flow represents inductance, highlighting subsurface interactions. Such simplified representations provide intuitive ways to bridge disciplinary perspectives and enhance integrated water management approaches. Table 1 expands on this analogy by contextualizing them within the effects of disturbances (e.g., wildfire, drought) and management practices (e.g., thinning, prescribed fire). While this analogy is intended to inspire a unified framework for understanding hydrologic connectivity, further research is necessary to enable its practical implementation in watershed management. Ultimately, the researchers recommended better interdisciplinary collaboration and adaptive management approaches to incorporate hydrologic connectivity into ecosystem restoration and resource management.
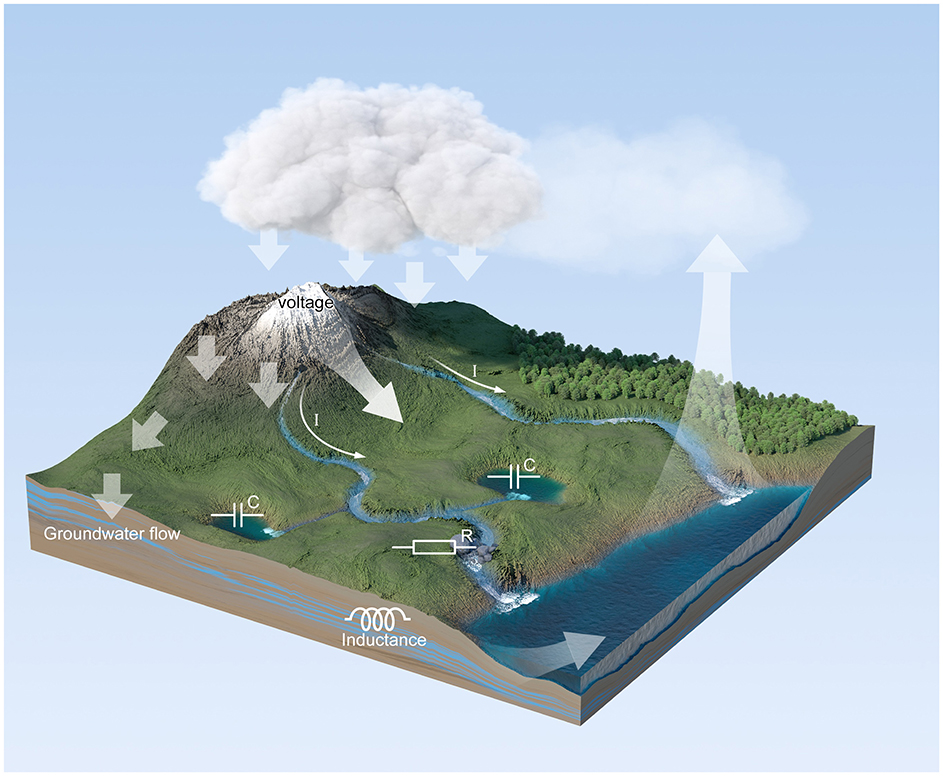
Figure 1. Conceptual analogy of hydrologic connectivity as an electric circuit. In this analogy, Voltage (V) represents the driving force, analogous to the gravitational potential that propels water flow; Current (I) corresponds to the rate of water flux through surface and subsurface pathways; Capacitor (C) symbolizes temporary water storage, such as wetlands, soil moisture, or aquifers that buffer and release water over time; Resistor (R) represents the resistance to flow imposed by features like vegetation, soil texture, compaction, or bedrock; and Inductor (L) captures system memory or lag effects, reflecting delayed hydrologic responses due to subsurface processes. While this illustration emphasizes surface processes for clarity, groundwater plays a critical role in shaping hydrologic connectivity. Specifically, aquifers act as capacitors by storing and slowly releasing water; confining layers or low-permeability zones function as resistors by limiting flow; and the slow movement and delayed discharge of groundwater contribute to the system's inductance, introducing lags in hydrologic response to climate or management interventions.
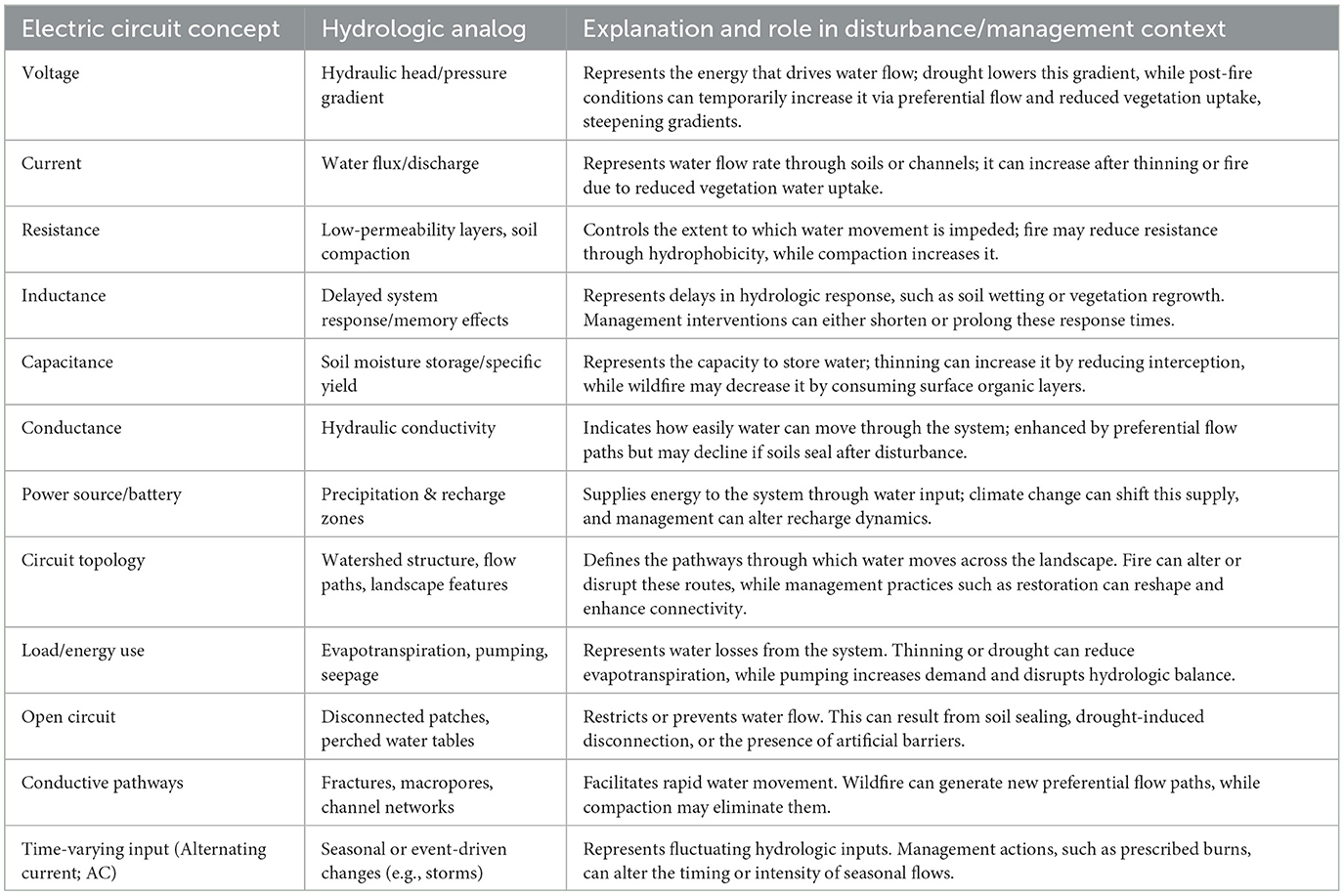
Table 1. Comparison of electric circuit concepts and hydrologic analogs, including implications for disturbance and watershed management.
Cho et al. expanded this conceptual foundation by introducing a sediment connectivity model that links hydrologic pathways and geomorphic processes. Their framework emphasized the role of spatial-temporal feedback between hydrologic processes (e.g., runoff, infiltration, return flow, percolation, and groundwater flow) and geomorphic drivers (e.g., runoff depth, soil conditions) in sediment transport, storage, and connectivity at multiple scales. Although to date their work remains theoretical and requires empirical validation for practical application, it demonstrated the value of incorporating geomorphic drivers into hydrologic connectivity studies to predict sediment flux and inform watershed management strategies. Considered together, the studies discussed in this section strengthen the conceptual foundation of hydrologic connectivity by integrating interdisciplinary perspectives, refining models, utilizing real-time data, and standardizing approaches to enhance predictability and inform sustainable water management in catchment systems.
Drivers and mechanisms of hydrologic connectivity
Several studies have explored key aspects of hydrologic connectivity—namely, topographic influence, wood accumulation, and tectonic impacts—that shape water movement, sediment transport, and landscape evolution across different timescales. Tull et al. investigated how topographic bluffs influence river–floodplain connectivity and residence times. According to their findings, bluff topography directs flow from the floodplain to the river. In contrast, levee-channels (i.e., the portion of a river confined between levees and engineered embankments) flow to the floodplain, with bluffs altering inundation patterns, creating exchange zones, and affecting residence times, nutrient transport, and sediment dynamics. Tull et al. ultimately emphasized the importance of integrating topographic features into floodplain restoration and management efforts, bolstering ecosystem functions such as solute sequestration and nutrient cycling.
Marshall and Wohl focused on the role of wood accumulation in driving channel bifurcations and altering flow paths within river systems. Their study challenged traditional bifurcation classifications by proposing a continuum model that links the ratio of erosive force to erosional resistance (F/R) with bifurcation type. The authors demonstrated that higher F/R values lead to lateral bifurcations and increased channel avulsion whereas lower values result in more stable banks and longitudinal bifurcations. These findings have significant implications for river restoration and sediment transport modeling.
Similarly, Han and Wilson examined the long-term evolution of hydrologic connectivity under tectonic and climatic influences in rift basins. Employing simulation models, they assessed how tectonic extension and climate change shape water storage, connectivity, and flow dynamics over geological timescales. Their work emphasized the need to integrate tectonic processes into hydrologic models to improve predictions of landscape evolution and promote sustainable water resource management, particularly in tectonically active regions. These two studies demonstrate how topography, wood accumulation, and tectonic forces shape hydrologic connectivity across scales, providing insights for predictive modeling and sustainable watershed management.
Hydrologic connectivity and nutrient cycling
Research has also examined storm-driven, sediment-mediated nitrogen connectivity and the geomorphic control of coarse particulate organic matter (CPOM), highlighting their combined influence on nutrient fluxes and sustainable stream management. Joshi et al. investigated the dual role of suspended sediments as nitrogen exporters and reactors for denitrification and assimilatory nitrogen uptake during storms while quantifying the proportions of assimilatory nitrogen uptake and denitrification losses relative to the suspended-sediment-bound nitrogen load and examining how these vary across drainage areas, storm sizes, and the rising and falling limbs of the storm hydrograph. Their study demonstrated how storm-driven sediment transport enhances nitrogen connectivity by increasing denitrification rates and nutrient uptake, significantly altering watershed nutrient cycling. By linking sediment transport to nitrogen removal processes, the findings inform watershed management strategies to mitigate nitrogen pollution and improve water quality, particularly in response to extreme hydrological events.
Fogel and Lininger examined how geomorphic complexity influences CPOM transport and storage in headwater streams. Their study revealed that stream reaches with more retentive features, such as wood and cobbles, store greater amounts of CPOM while valley geometry influences transport at broader spatial scales. These findings underscore the need to consider geomorphic complexity in stream management and habitat restoration, as both direct alterations (e.g., dam construction, water diversions, wood removal, logging) and indirect changes (e.g., shifts in precipitation patterns and snowpack conditions) can substantially modify peak flow magnitude and frequency, valley bottom geometry, lateral connectivity, and in-stream wood and woody CPOM loads. Taken together, these studies emphasize how hydrologic and geomorphic processes regulate nutrient and organic matter fluxes in stream ecosystems, with storm-driven sediment transport enhancing nitrogen removal and geomorphic complexity shaping nutrient and CPOM retention.
Hydrologic connectivity and catchment dynamics
Understanding key aspects of climate-driven river evolution–investigating drainage system changes over geological timescales, evaluating discrepancies between predicted and observed network development, and identifying additional geomorphic and hydrologic feedbacks–can inform the refinement of predictive models for river network evolution. Hunt et al. developed a predictive model for river network evolution under climatic influences, assessing drainage system changes over geological timescales. Although some rivers align with theoretical expectations, such as groundwater–river interactions following a non-linear spatio-temporal scaling relationship, others deviate, like the Rio Grande and Pecos, suggesting additional geomorphic and hydrologic feedbacks. Importantly, the findings from this study revealed that connectivity patterns within river networks critically influence these dynamics, underscoring the need to refine climate-driven river evolution models by incorporating a broader range of hydrologic, geomorphic, and climatic variables while addressing theoretical assumptions and limited long-term data through expanded geographic coverage and improved modeling approaches.
Conclusion: bridging hydrologic connectivity research with practical applications
This Research Topic advances hydrologic connectivity by integrating interdisciplinary frameworks, refining sediment connectivity models, and examining key drivers such as topography, wood accumulation, and tectonic forces across different timescales. Biogeochemical processes and nutrient cycling underscore the importance of connectivity for sustainable water management, particularly through storm-driven sediment transport in nitrogen connectivity and the influence of geomorphic complexity on CPOM retention. In addition, research on climate-driven river evolution provides critical insights for refining predictive models. Future research should address critical gaps by advancing process-based and percolation theory-based models, graph theory, and entropy-based metrics to improve the precision of connectivity dynamics analysis (e.g., Matheus Carnevali et al., 2021; Dwivedi and Mohanty, 2016; Arora et al., 2019; Dwivedi et al., 2022; Dewey et al., 2022; Arora et al., 2022; Wohl et al., 2019; Wohl, 2019; Pöppl et al., 2024) to enhance the analysis of connectivity dynamics and improve model precision. Concentration–discharge (C–Q) relationships also offer valuable insights into hydrologic connectivity by linking critical zone structure, biogeochemical processes, and landscape heterogeneity (Herndon et al., 2015; Ackerer et al., 2020; Arora et al., 2020). These metrics can be leveraged to infer various dimensions of hydrologic connectivity–including vertical, lateral, and horizontal linkages–and warrant further exploration to uncover additional spatial and temporal patterns across watershed systems. Furthermore, addressing data limitations, implementing modeling approaches, and integrating machine learning techniques will be critical for strengthening hydrologic connectivity applications in environmental management (e.g., Varadharajan et al., 2019; Faybishenko et al., 2022). Ultimately, interdisciplinary collaboration and adaptive management strategies are vital for effectively integrating hydrologic connectivity into ecosystem restoration and resource management.
Author contributions
DD: Writing – original draft, Writing – review & editing. RP: Writing – review & editing. EW: Writing – review & editing.
Funding
The author(s) declare that financial support was received for the research and/or publication of this article. This research was supported by the U.S. Department of Energy (DOE), Office of Biological and Environmental Research (BER), as part of BER's Environmental System Science (ESS) Program through the Watershed Function Scientific Focus Area (SFA) at Lawrence Berkeley National Laboratory (LBNL). The Watershed Function SFA specifically supported DD's work at LBNL, funded by the DOE Office of Science, BER, under Award No. DE-AC02-05CH11231.
Conflict of interest
The authors declare that the research was conducted in the absence of any commercial or financial relationships that could be construed as a potential conflict of interest.
The author(s) declared that they were an editorial board member of Frontiers, at the time of submission. This had no impact on the peer review process and the final decision.
Generative AI statement
The author(s) declare that Gen AI was used in the creation of this manuscript. This work utilized generative AI tools solely for minor editorial refinements and formatting assistance. No original content was generated using AI.
Publisher's note
All claims expressed in this article are solely those of the authors and do not necessarily represent those of their affiliated organizations, or those of the publisher, the editors and the reviewers. Any product that may be evaluated in this article, or claim that may be made by its manufacturer, is not guaranteed or endorsed by the publisher.
References
Ackerer, J., Steefel, C., Liu, F., Bart, R., Safeeq, M., O'Geen, A., et al. (2020). Determining how critical zone structure constrains hydrogeochemical behavior of watersheds: learning from an elevation gradient in california's sierra nevada. Front. Water 2:23. doi: 10.3389/frwa.2020.00023
Arora, B., Briggs, M. A., Zarnetske, J. P., Stegen, J., Gomez-Velez, J. D., Dwivedi, D., et al. (2022). “Hot spots and hot moments in the critical zone: identification of and incorporation into reactive transport models,” in Biogeochemistry of the Critical Zone (Springer), 9–47. doi: 10.1007/978-3-030-95921-0_2
Arora, B., Burrus, M., Newcomer, M., Steefel, C. I., Carroll, R. W., Dwivedi, D., et al. (2020). Differential cq analysis: a new approach to inferring lateral transport and hydrologic transients within multiple reaches of a mountainous headwater catchment. Front. Water 2:24. doi: 10.3389/frwa.2020.00024
Arora, B., Wainwright, H. M., Dwivedi, D., Vaughn, L. J., Curtis, J. B., Torn, M. S., et al. (2019). Evaluating temporal controls on greenhouse gas (ghg) fluxes in an arctic tundra environment: An entropy-based approach. Sci. Total Environ. 649, 284–299. doi: 10.1016/j.scitotenv.2018.08.251
Dewey, C., Fox, P. M., Bouskill, N. J., Dwivedi, D., Nico, P., and Fendorf, S. (2022). Beaver dams overshadow climate extremes in controlling riparian hydrology and water quality. Nat. Commun. 13:6509. doi: 10.1038/s41467-022-34022-0
Dwivedi, D., and Mohanty, B. P. (2016). Hot spots and persistence of nitrate in aquifers across scales. Entropy 18:25. doi: 10.3390/e18010025
Dwivedi, D., Steefel, C. I., Arora, B., Banfield, J., Bargar, J., Boyanov, M. I., et al. (2022). From legacy contamination to watershed systems science: a review of scientific insights and technologies developed through doe-supported research in water and energy security. Environ. Res. Lett. 17:043004. doi: 10.1088/1748-9326/ac59a9
Faybishenko, B., Versteeg, R., Pastorello, G., Dwivedi, D., Varadharajan, C., and Agarwal, D. (2022). Challenging problems of quality assurance and quality control (QA/QC) of meteorological time series data. Stoch. Environ. Res. Risk Assess. 36, 1049–1062. doi: 10.1007/s00477-021-02106-w
Herndon, E. M., Dere, A. L., Sullivan, P. L., Norris, D., Reynolds, B., and Brantley, S. L. (2015). Landscape heterogeneity drives contrasting concentration-discharge relationships in shale headwater catchments. Hydrol. Earth Syst. Sci. 19, 3333–3347. doi: 10.5194/hess-19-3333-2015
Matheus Carnevali, P. B., Lavy, A., Thomas, A. D., Crits-Christoph, A., Diamond, S., Méheust, R., et al. (2021). Meanders as a scaling motif for understanding of floodplain soil microbiome and biogeochemical potential at the watershed scale. Microbiome 9:121. doi: 10.1186/s40168-020-00957-z
Pöppl, R. E., Parsons, A. J., and Keesstra, S. D. (2024). Connectivity in Geomorphology. Cambridge, New York: Cambridge University Press.
Varadharajan, C., Agarwal, D. A., Brown, W., Burrus, M., Carroll, R. W., Christianson, D. S., et al. (2019). Challenges in building an end-to-end system for acquisition, management, and integration of diverse data from sensor networks in watersheds: lessons from a mountainous community observatory in east river, colorado. IEEE Access 7, 182796–182813. doi: 10.1109/ACCESS.2019.2957793
Wohl, E. (2019). Forgotten legacies: Understanding and mitigating historical human alterations of river corridors. Water Resour. Res. 55, 5181–5201. doi: 10.1029/2018WR024433
Wohl, E., Brierley, G., Cadol, D., Coulthard, T. J., Covino, T., Fryirs, K., et al. (2019). A connectivity as an emergent property of geomorphic systems. Earth Surface Proc. Landf. 44, 4–26. doi: 10.1002/esp.4434
Keywords: hydrologic connectivity, watershed processes, critical zone, wildfire, drought, integrated water resources management, electric circuit analogy
Citation: Dwivedi D, Pöppl RE and Wohl E (2025) Editorial: Investigating connectivity to advance the predictive understanding of watershed processes and the Earth's critical zone. Front. Water 7:1589792. doi: 10.3389/frwa.2025.1589792
Received: 08 March 2025; Accepted: 15 April 2025;
Published: 09 May 2025.
Edited by:
Mohammad Safeeq, University of California, Merced, United StatesReviewed by:
Julien Ackerer, University of California, Merced, United StatesYang Yang, Western Carolina University, United States
Copyright © 2025 Dwivedi, Pöppl and Wohl. This is an open-access article distributed under the terms of the Creative Commons Attribution License (CC BY). The use, distribution or reproduction in other forums is permitted, provided the original author(s) and the copyright owner(s) are credited and that the original publication in this journal is cited, in accordance with accepted academic practice. No use, distribution or reproduction is permitted which does not comply with these terms.
*Correspondence: Dipankar Dwivedi, RER3aXZlZGlAbGJsLmdvdg==