- 1Department of Rehabilitation, The First Affiliated Hospital of Nanjing Medical University, Nanjing, China
- 2School of Rehabilitation Medicine, Nanjing Medical University, Nanjing, China
Background: Virtual reality (VR) intervention is an innovative and efficient rehabilitative tool for patients affected by stroke, Parkinson's disease, and other neurological disorders. This meta-analysis aims to evaluate the effects of VR intervention on cognition and motor function in older adults with mild cognitive impairment or dementia.
Methods: Seven databases were systematically searched for relevant articles published from inception to April 2020. Randomized controlled trials examining VR intervention in adults with mild cognitive impairment or dementia aged >60 years were included. The primary outcome of the study was cognitive function, including overall cognition, global cognition, attention, executive function, memory, and visuospatial ability. The secondary outcome was motor function, consisting of overall motor function, balance, and gait. A subgroup analysis was also performed based on study characteristics to identify the potential factors for heterogeneity.
Results: Eleven studies including 359 participants were included for final analysis. Primary analysis showed a significant moderate positive effect size (ES) of VR on overall cognition (g = 0.45; 95% confidence interval (CI) = 0.31–0.59; P < 0.001), attention/execution (g = 0.49; 95% CI = 0.26–0.72; P < 0.001), memory (g = 0.57; 95% CI = 0.29–0.85; P < 0.001), and global cognition (g = 0.32; 95% CI = 0.06–0.58; P = 0.02). Secondary analysis showed a significant small positive ES on overall motor function (g = 0.28; 95% CI = 0.05–0.51; P = 0.018). The ES on balance (g = 0.43; 95% CI = 0.06–0.80; P = 0.02) was significant and moderate. The ES on visuospatial ability and gait was not significant. In the subgroup analysis, heterogeneity was detected in type of immersion and population diagnosis.
Conclusions: VR intervention is a beneficial non-pharmacological approach to improve cognitive and motor function in older adults with mild cognitive impairment or dementia, especially in attention/execution, memory, global cognition, and balance. VR intervention does not show superiority on visuospatial ability and gait performance.
Introduction
Dementia is a collective name for a heterogeneous group of chronic neurodegenerative diseases characterized by progressive deterioration of goal-directed behaviors and cognitive function (Aruanno and Garzotto, 2019; D'Cunha et al., 2019). As the “symptomatic predementia stage” (Langa and Levine, 2014), individuals with mild cognitive impairment (MCI) display mild impairment in cognitive function with preserved independent functional abilities and with no obvious deficits in social and occupational functioning (Albert et al., 2011). However, these individuals typically have a higher risk of dementia than age-matched individuals without MCI (Petersen et al., 2018; Aruanno and Garzotto, 2019). According to the World Health Organization, ~47 million people worldwide are afflicted by dementia; this number is expected to increase to 75 million by 2030 and nearly 131 million by 2050 (Arvanitakis et al., 2019). In people aged 60 years and older, the reported prevalence of MCI ranges from 6.7 to 25.2% (Petersen et al., 2018).
Important breakthroughs in the pharmacological treatment of dementia have not been achieved, resulting in gravitation toward non-pharmacological approaches (D'Cunha et al., 2018). Updated practice guidelines have noted that exercises (Level B) and cognitive interventions (Level C) may be beneficial to improve measures of cognitive function in patients with MCI (Petersen et al., 2018) and stress that exercise training for 6 months is likely to improve cognitive outcome (moderate confidence in the evidence based on two Class II studies).
Virtual reality (VR) is a new technology for implementing rehabilitation of cognitive and motor function (Tieri et al., 2018). VR technology is defined as a “high-end computer interface that involves real time simulation and interactions through multiple sensory channels.” All VR applications possess two key features, immersion and interaction, which means that VR can bring the subject inside a virtual environment and to respond in real time to movements of the body in a naturalistic way (Tieri et al., 2018). VR can be categorized into three types according to the level of immersion: low immersion, semi-immersion, and full immersion (García-Betances et al., 2015). In a low immersive system, the patient interacts with the virtual environment using conventional graphic workstations such as PC monitor, keyboard and mouse. A semi-immersive VR system typically consists of more complicated interactive devices such as motion tracker, haptic gloves, and balance platform. On the other hand, a full immersive VR can be defined as an immersive experience delivered through a combination of more sophisticated graphic systems for example head-mounted display, surrounded screen, along with input of other sensory information such as sound, touch or even smell to let participants fully sink into virtual environment. VR intervention can combine exercises and cognitive training together making it a good option for patients with MCI or dementia.
According to recent meta-analyses, VR has significant beneficial effects on cognitive function in individuals who have sustained a stroke and evidence supports its use as an adjunct strategy for stroke rehabilitation at different stages of recovery using numerous platforms and training parameters (Aminov et al., 2018). VR can not only achieve the same effect as conventional rehabilitation training, but also improve gait and balance performance in patients with Parkinson's disease (Lei et al., 2019; Santos et al., 2019). To the best of our knowledge, there is only one systematic review regarding VR intervention in MCI or dementia (Kim et al., 2019). However, through analyzing the papers from Kim et al. (2019), we found that the quality of the included studies varied according to the Cochrane Collaboration's tool and the PEDro scale.
Due to the inclusion of many non-randomized controlled trials (RCTs) in Kim et al. (2019), the included articles are very different in study design, setting of control groups, and the quality of studies, all of which may increase the risk of bias. In this case, they only performed a sub-analysis of randomized vs. non-randomized studies. We believed this is not enough although it is difficult to analyze all potential risk of bias. Kim et al. (2019) only performed an overall effect size on cognition and physical fitness. Therefore, we perform an updated quantitative review of RCTs focusing on the efficacy of VR intervention on specific domains of cognition in older adults with MCI or dementia. In addition, knowing that MCI and dementia patients often have an increased risk of fall (Allali and Verghese, 2017), we also identify changes in motor function such as gait and balance.
The primary objective of this meta-analysis was to assess the effect of VR intervention on cognitive function, including overall cognition, global cognition, attention, executive function, memory, and visuospatial ability. The secondary objective was to identify the effect of VR on motor function, which comprises balance and gait.
Materials and Methods
The results of this meta-analysis are reported in accordance with the Preferred Reporting Items for Systematic Reviews and Meta-Analysis (PRISMA) guidelines (Moher et al., 2009).
Search Strategy
We searched the Cochrane Library, EMBASE, EBSCO, Ovid, PubMed, Scopus, and Web of Science databases from inception to April 2020 for RCTs that examined the effects of VR on one or more cognitive or behavioral outcomes in older adults with MCI or dementia without time limit. The specific search syntax, such as PubMed, can be available in the Supplementary Material.
Eligibility Criteria
Eligibility criteria were formulated based on the PICOS framework (Moher et al., 2009):
(1) Participants: The age of the participants was >60 years, with a diagnosis of MCI or dementia (of any etiology).
(2) Intervention: We included studies using VR interventions that met the following definition: “The VR intervention should be the use of interactive simulations created with computer hardware and software to present users with a virtual figure to engage in environments that appear and feel similar to real world objects and events.” We did not limit the type of VR according to level of immersion (low immersive, semi-immersive, or full immersive). Interventions needed to be implemented for ≥5 h using standardized computerized tasks or interactive video games.
(3) Control: The comparison group received either an alternative intervention or no intervention. Alternative interventions included any activity designed to be therapeutic at the impairment, activity, or participation level that did not include the use of VR, ranging from motor and/or cognitive training to health education.
(4) Outcome: The primary outcome of this study is cognitive function, including overall cognition, global cognition, attention, executive function, memory, and visuospatial ability. The secondary outcome is motor function, consisting of balance and gait. All outcome measures had to be evaluated at baseline and immediately after the intervention period. Long-term follow-up data was not included in data synthesis, as only a small number of studies reported this information.
(5) Study: Eligible studies were peer-reviewed articles reporting results from RCTs that examined the effects of VR on one or more cognitive outcomes and motor function in adults aged ≥60 years with MCI or dementia. The following types of articles were excluded: (1) prospective or retrospective cohort studies; (2) case reports; (3) conference abstracts; and (4) not written in English.
Study Selection
Two reviewers (SZ and YS) conducted the initial online search independently to avoid selection bias. After excluding duplicate studies, article titles, and abstracts were reviewed. If an abstract was considered relevant or ambiguous, the full text was reviewed and inclusion and exclusion criteria were applied. Disagreements regarding study eligibility were resolved by CG, who approved the final list of included studies.
Data Extraction and Analysis
Data regarding study characteristics (nation, year, type of study, sample size, mean age, intensity, number of sessions, session length, immersion type of VR, comparison group, and outcome measures) of each article were extracted. We also summarized the components of the intervention in the two groups and extracted differences between them based on the description of interventions and the purpose of trials which could contribute to the superiority of VR. In the control group, a blank control group was defined as passive interventions while the same total training time in the experimental group was defined as active interventions. Most data was entered as mean with standard deviation (SD) for the VR and control groups at baseline and immediately after training. When mean and SD were not available, mean changes from baseline with SD or mean differences with 95% confidence interval (CI) were extracted. In addition, we contacted the authors to request raw summary data if there was no data available. For studies that included several tests of the same domain, each domain was averaged to one pooled effect size (ES) using Comprehensive Meta-Analysis (CMA) software version 2.0 (Biostat, Inc., Englewood, NJ, USA). For example, if a study included several attention tests, then these tests were summarized to generate one pooled effect size (Li et al., 2011). CMA allows for each of these different study outcomes to be flexibly entered into the model. A random effects model was used to correct for variable effect sizes across the studies if these studies show heterogeneity in their intervention (e.g., intervention type, duration, outcome measures) (Rosenblad, 2009). We chose the Hedges' g of the ES to estimate the efficacy of VR intervention. Hedge's g estimates of <0.3 were considered as small, ≥0.3 and <0.6 as moderate, and ≥0.6 as large, respectively (Hill et al., 2017). The calculation equation (Newton et al., 1998) of hedges' g is shown as follows:
The collected data were utilized to calculate a combined effect size (Hedges' g) and 95% CI of changes in outcome measures between the experimental group (EG) and control group (CG) from pre- to post-test. Estimate variance was scaled up based on an assumed inter-correlation between the tests of 0.7 (Lampit et al., 2014; Hill et al., 2017). For Hedges' g, the direction of ES was positive if post-test performance was better than pre-test performance. In addition, a random effects model was chosen to accommodate heterogeneity for this analysis (Higgins et al., 2020). A subgroup analysis was also conducted based on study characteristics (population diagnosis, type of immersion, training time, and effectiveness of control group).
Risk of Bias and Study Quality Assessment
The Cochrane Collaboration's tool (Higgins et al., 2011) was used to assess risk of bias in each individual study. The tool contains the domains of sequence generation; allocation concealment; blinding of participants, personnel, and outcome assessors; incomplete outcome data; selective outcome reporting; and other sources of bias. We classified items as “low risk,” “high risk,” or “unclear risk” of bias. The risk of bias is presented in Table 1.
We also used the PEDro scale (Physiotherapy Evidence Database Rating Scale) to assess the quality of included studies using a score (Table 1) (Maher et al., 2003). The scale includes 11 items to rate study quality (Maher et al., 2003); the maximum score is 11. Studies that scored seven or higher were considered high quality, while those that scored six or lower were considered low quality. The scoring process was conducted by two authors (SZ and YS). CG established consensus scores and resolved any disagreements.
Publication bias was assessed by a funnel plot that displayed the relationship between sample size and ES. Small sample studies with a relatively large variance scatter at the bottom and large sample studies appear toward the top clustering around the mean ES. Studies that fall outside the funnel shape have a high risk of bias (Rosenblad, 2009).
Results
Identification of Studies
Figure 1 shows the flow diagram of study selection. The initial search yielded 1,859 articles from seven databases and three additional articles were identified through other sources. After removing duplicates, 1,160 eligible records were retrieved. After reading titles and abstracts, 1,098 articles were excluded. After readinging full text, the remaining 62 articles met the inclusion criteria. Among these 62 articles, 21 were excluded because study design was not RCT, 17 because VR was not used as the intervention, nine because participants did not meet the inclusion criteria, and four were excluded because exact outcome values were not reported and we contacted the authors, but there was no response. Finally, 11 original research articles were selected for further analysis.
Participant and Study Characteristics
Table 1 summarizes the characteristics of the included studies. All 11 studies were RCTs. Nine studies reported older adults with MCI (n = 317) and two reported Alzheimer's dementia (n = 42). Thus, the analysis involved a total of 359 participants (experimental group = 175, mean group size = 16; control group = 184, mean group size = 17). Mean patient age was 75.84 years and 71.82% of the patients were female. Three studies reported full immersive VR, seven reported semi-immersive VR, and the remaining one reported low immersive VR. The VR methodology in all studies was task-oriented training. The interactive devices included head-mounted device, stick, Kinect, depth camera, sensor motion tracker, Nintendo Wii, touchscreen, keyboard, Bio Rescue, or gamepad. Number of intervention sessions ranged from 10 to 40 sessions. Training frequency varied from 1 to 5 sessions per week and the duration per session varied from 20 to 90 min. Three interventions in the control group were passive and eight interventions were active. The brief components of interventions in the two groups are listed in Table 2.
Table 3 provides an overview of the outcome measures used in the different studies.
(1) Attention/Executive Function: Trail Making Test (Arnett and Labovitz, 1995), Stroop Color and Word Test (Scarpina and Tagini, 2017), verbal fluency test (Park et al., 2019), verbal categorical test (Serino et al., 2017), Frontal Assessment Battery (Appollonio et al., 2005), Attentional Matrices Test (Serino et al., 2017), letter fluency test (Tarnanas et al., 2014), category fluency test (Tarnanas et al., 2014), and digit symbol substitution test (Makizako et al., 2013).
(2) Memory: visual span test (Hwang and Lee, 2017), Fuld Object Memory Evaluation (Monaco et al., 2015), Multifactorial Memory Questionnaire (Troyer and Rich, 2002), Boston Naming Test (Erdodi et al., 2018), word list test (Lee et al., 2002), constructional recall (Lee et al., 2004), digit span test (Monaco et al., 2013), Corsi block test (Serino et al., 2017), and Rey Auditory Verbal Learning Test (Lezak, 1998).
(3) Visuospatial ability: constructional praxis (Park et al., 2019) and Rey complex figure copy (Shin et al., 2006).
(4) Global Cognition: Montreal Cognitive Assessment (Nasreddine et al., 2005), Mini-Mental State Examination (Folstein et al., 1975), The Computerized Assessment of MCI (Saxton et al., 2009), and Cognitive Self-Report Questionnaire (Hughes et al., 2014).
(5) Gait: Gait speed, stride time, gait system (Liao et al., 2019), Instrument Timed Up and Go (Podsiadlo and Richardson, 1991), and 8-feet Up and Go (Thapa et al., 2020).
(6) Balance: Tinetti test (Tinetti et al., 1986), Balance Limit of Stability (Hwang and Lee, 2017), Berg Balance Scale (Muir et al., 2008), and center of mass (Pai and Patton, 1997).
Primary and Secondary Analyses
Primary analysis using the random effects model showed a significant moderate positive ES of VR on overall cognition (g = 0.45; 95% CI = 0.31–0.59; P < 0.001; I2 = 0%), attention/execution (g = 0.49; 95% CI = 0.26–0.72; P < 0.001; I2 = 31.45%), memory (g = 0.57; 95% CI = 0.29–0.85;P < 0.001; I2 = 0%), and global cognition (g = 0.32; 95% CI = 0.06–0.58; P = 0.02; I2 = 0%) (Figure 2; Table 4). The ES of VR on visuospatial ability was not significant (g = 0.33; 95% CI = −0.08–0.74; P = 0.11; I2 = 0%).
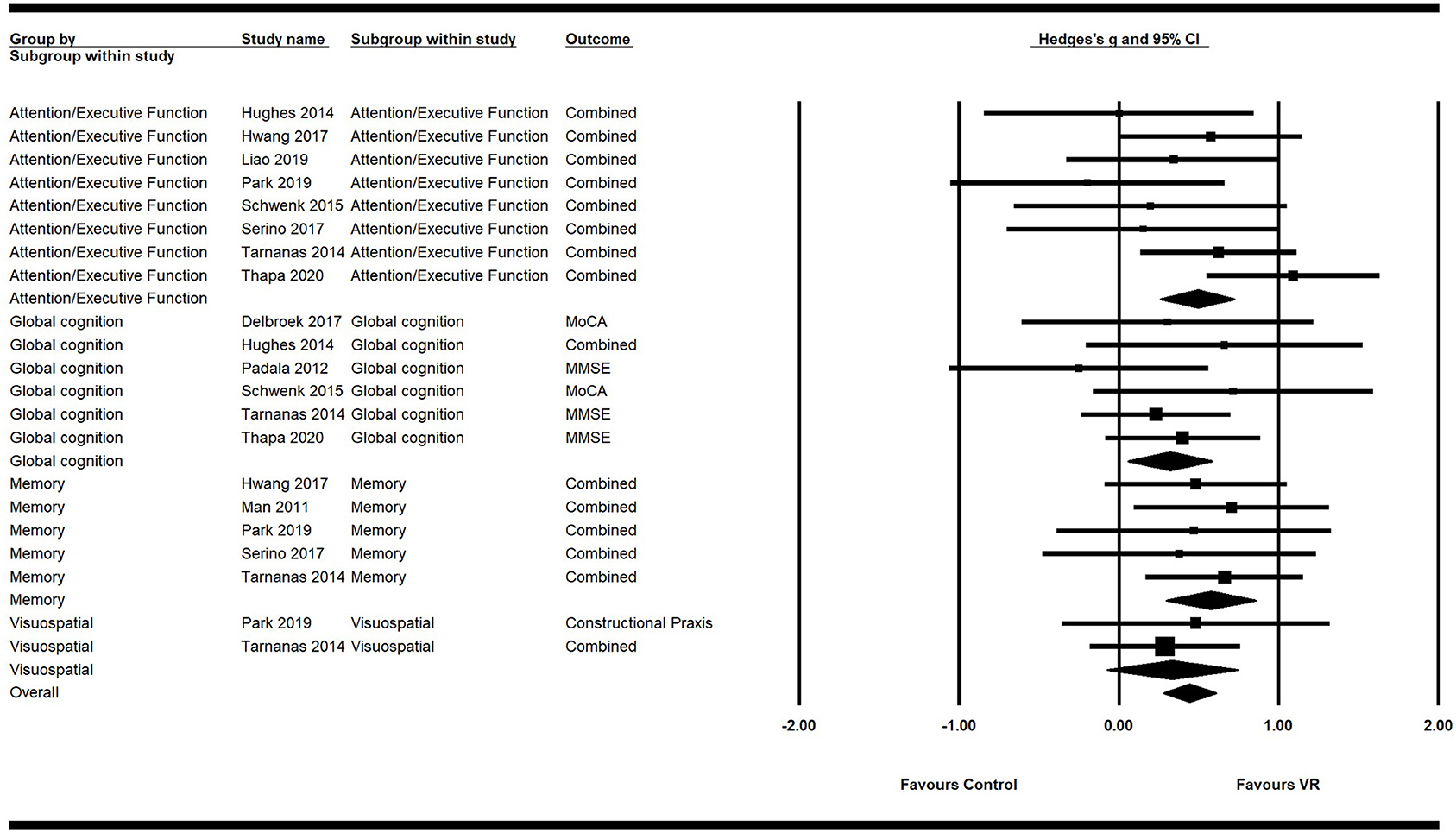
Figure 2. Forest plot for the efficacy of VR intervention on cognitive functions compared with the control group.
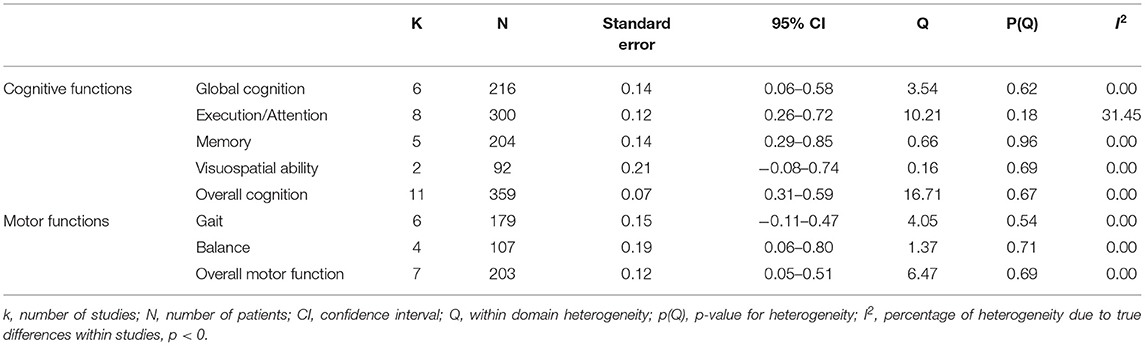
Table 4. Mean weighted effect sizes, confidence interval, and heterogeneity for primary and secondary outcome measures.
Secondary analysis showed a significant small positive ES of VR on overall motor function (g = 0.28; 95% CI = 0.05–0.51; P = 0.018; I2 = 0%). Its ES on balance (g = 0.43; 95% CI = 0.06–0.80; P = 0.02; I2 = 0%) was significant and of moderate efficacy. However, the ES of VR on gait was not significant (g = 0.18; 95% CI = −0.11–0.47; P = 0.21; I2 = 0%) (Figure 3; Table 4).
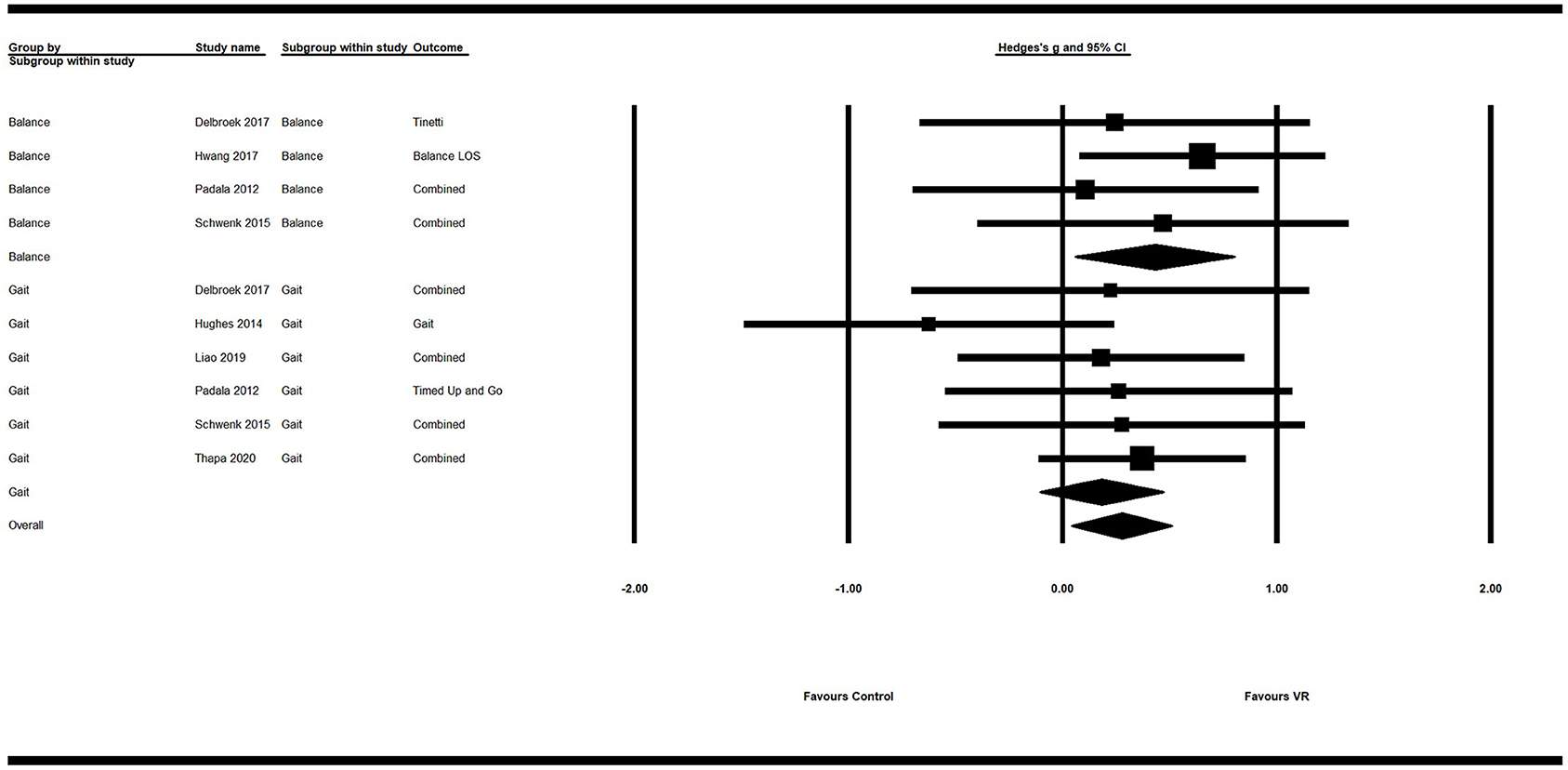
Figure 3. Forest plot for the efficacy of VR intervention on motor functions compared with the control group.
Subgroup Analyses
The results of a subgroup analysis according to study characteristics are shown in Table 5. VR interventions for patients with MCI resulted in greater efficacy (g = 0.46; 95% CI = 0.25–0.68; P < 0.0001) than for patients with dementia (g = 0.11; 95% CI = −0.47–0.70; P = 0.706). Regarding type of immersion, studies using full immersive VR (g = 0.47; 95% CI = 0.10–0.83; P = 0.012) had a greater efficacy than studies using semi-immersive VR (g = 0.38; 95% CI = 0.11–0.64; P = 0.005), but did not reach statistical significance on low immersive VR (g = 0.54; 95% CI = −0.06–1.15; P = 0.077). For the training time, more than 20 h showed moderate effect size (g = 0.43; 95% CI = 0.15–0.70; P = 0.002), which were almost equal to those <20 h (g = 0.42; 95% CI = 0.12–0.72; P = 0.006). In terms of the effectiveness of interventions in the control group, active (g = 0.40; 95% CI = 0.15–0.64; P = 0.001) and passive (g = 0.55; 95% CI = 0.15–0.95; P = 0.008) interventions both showed moderate effect sizes.
Risk of Bias and Study Quality
Figure 4 and Table 6 show the risk of bias in the 11 included studies. All studies used random sequence generation (Man et al., 2012; Padala et al., 2012; Hughes et al., 2014; Tarnanas et al., 2014; Schwenk et al., 2016; Delbroek et al., 2017; Hwang and Lee, 2017; Serino et al., 2017; Liao et al., 2019; Park et al., 2019; Thapa et al., 2020). Participants and assessments were blinded in only one study (Padala et al., 2012). Four studies had adequate blinding of outcome assessment (Tarnanas et al., 2014; Delbroek et al., 2017; Liao et al., 2019; Park et al., 2019). All studies had a low risk of attrition bias on incomplete outcome data and selective reporting (Man et al., 2012; Padala et al., 2012; Hughes et al., 2014; Tarnanas et al., 2014; Schwenk et al., 2016; Delbroek et al., 2017; Hwang and Lee, 2017; Serino et al., 2017; Liao et al., 2019; Park et al., 2019; Thapa et al., 2020). Study quality is shown in Table 1. All 11 studies were high quality and the mean PEDro score was 7.67.
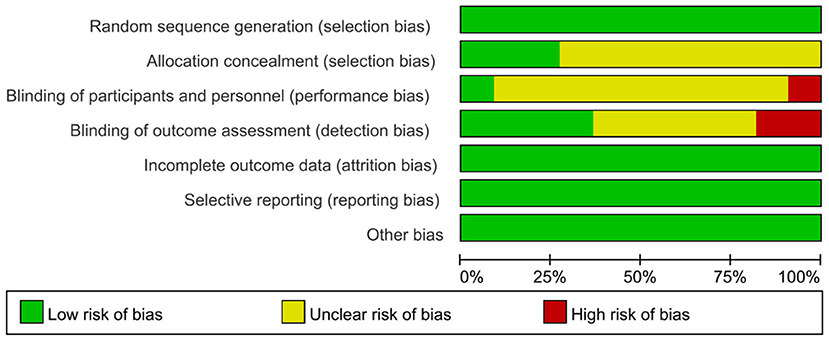
Figure 4. Risk of bias assessment per domain across studies with domains of bias on the Y-axis and % of studies having a high, unclear, or low risk of bias in each domain on the X-axis. The total score is the final author judgment of the total risk of bias.
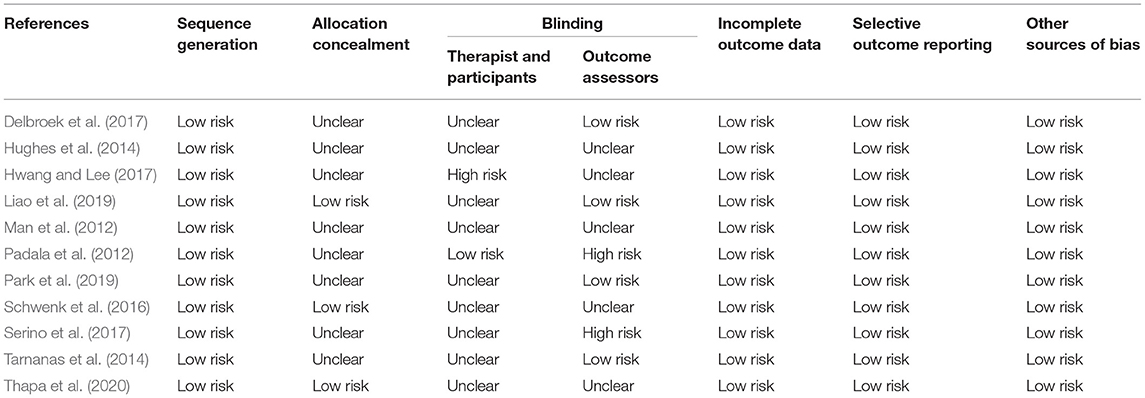
Table 6. Risk of bias assessment in included studies: the authors' judgments on each risk of bias item for all included studies.
Figure 5 shows the funnel plot of the studies. Distribution was fairly symmetric, indicating no hint of publication bias. Orwin's fail-safe N was calculated only for the measures that showed significant differences between the experimental and control groups. For overall cognition, 71 studies would be required to reduce the observed effect to an ES <0.1.
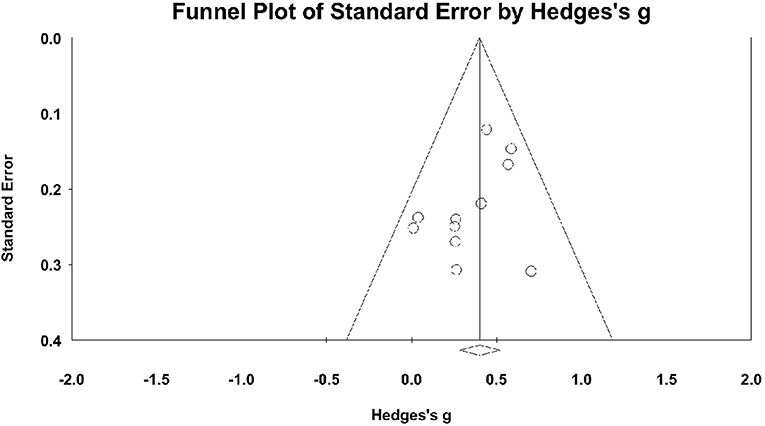
Figure 5. Funnel plot for overall cognition with Hedge's g on the X-axis and the standard error on the Y-axis.
Discussion
Based on the results from 11 high quality RCTs, this meta-analysis showed that VR intervention exhibited a moderate effect on several measures of cognitive and motor function, including attention/execution, memory, global cognition, and balance. This indicates VR is a promising effective non-pharmacological therapy for older adults with MCI or dementia. However, VR intervention did not show a significant effect on visuospatial ability or gait.
Interpretation of Results and Comparison With Previous Research
To the best of our knowledge, this is the first meta-analysis to focus on the effect of VR intervention on specific cognitive and motor function domains in older adults with MCI or dementia. Recently, Kim et al. (2019) investigated the benefits of VR intervention in older adults with MCI or dementia. In their meta-analysis, four of the 11 included studies were not RCTs. However, by including only RCTs, our meta-analysis was able to quantify the magnitude of the effect, confirming the efficacy of VR intervention in MCI or dementia patients (Karssemeijer et al., 2017). Moreover, our exploration of the effects of VR intervention on specific cognitive and motor function domains can provide a framework for future clinical practice.
Our results are comparable with those of Kim et al. (Cohen' d = 0.42; 95% CI = 0.24–0.60), demonstrating a moderate effect of VR on cognitive function (Kim et al., 2019). However, they did not perform an analysis of specific cognitive domains and found that VR had no significant effect on execution (Cohen' d = 0.07; 95% CI = −0.34–0.49), in contrast to our findings. Another recent meta-analysis that evaluated the effect of combined cognitive and physical exercise training on cognitive function in older adults with MCI or dementia did not find a significant effect on the attention/execution cognitive domain (SMD = 0.38; 95% CI = −0.21–0.97) or memory (SMD = 0.02; 95% CI = −0.35–0.39) either (Karssemeijer et al., 2017), which is inconsistent with our findings. However, the combined ES data in their study were based on only two studies. Furthermore, whether VR intervention utilizes its unique features of immersion and interaction to improve these cognitive domains remains to be explored. A meta-analysis conducted by Hill et al. (2017) showed a positive effect of computerized cognitive training on working memory (Hedges' g = 0.74; 95% CI = 0.32–1.15), verbal memory (g = 0.42; 95% CI = 0.21–0.63), and attention (g = 0.44; 95% CI = 0.20–0.68) in older adults with MCI or dementia. They found no significant effect on visuospatial ability (g = 0.18; 95% CI = −0.23–0.60), which is consistent with our findings. Another recent review noted that VR was more effective in improving attention, visuospatial deficits, and motor impairment in stroke patients (Maggio et al., 2019). Of all the studies included in our meta-analysis, only two focused on MCI subjects and provided visuospatial ability data. Therefore, future studies are needed to determine if VR intervention can improve visuospatial ability in older adults with MCI or dementia. Meanwhile, tasks of VR interventions in these two studies were not especially designed for the visuospatial ability, so the effects could not be translated into the significant improvement of a general visuospatial ability compared with the interventions in the control group. Our meta-analysis showed a moderate ES of VR intervention on global cognition (SMD = 0.32; 95% CI = 0.17–0.47), which is in agreement with the results of Karssemeijer et al. (2017) and Hill et al. (2017).
Kim et al. (2019) also reported a moderate ES of VR intervention on overall motor function (Cohen' d = 0.41; 95% CI = 0.16–0.65), but did not evaluate the effect on measures of gait, balance, or falls. Balance improvement (MD = 2.99; 95% CI = 1.80–4.18) was in accordance with the efficacy of VR intervention in community-dwelling older adults found by Neri et al. (2017). Their study also showed no significant effect on gait performance (MD = −1.20; 95% CI = −1.62 to −0.77), which is consistent with our study. Indeed, in addition to cognitive impairment, patients with MCI or dementia likely have impaired motor function, which is often ignored in current studies. Poor performance in motor function is seldom seen in a single walking or motor task, since patients may sacrifice their efficiency in cognitive tasks to compensate for deficits in motor performance; however, this relationship is broken when a complex cognitive task is added to complete concurrently (Bloem et al., 2006). Therefore, in future studies, researchers should take dual tasking into consideration when designing measurements of motor outcomes.
In order to understand what makes the VR intervention efficient, we did a subgroup analysis according to study characteristics, as follows: (1) population diagnosis (e.g., MCI., AD.); (2) type of immersion (e.g., full immersion, semi-immersion, low immersion); (3) training time (e.g., less or more than 20 h); (4) effectiveness of control group (e.g., active or passive interventions). We have found positive effect of VR intervention in the MCI group while no statistical significance in AD. It may be related to the progression of MCI to dementia that MCI as the “predementia stage” is less severe compared to AD (Langa and Levine, 2014; Roberts et al., 2014). Consequently, we assumed that VR may be a beneficial intervention for patients with MCI and the intervention in the early stage of cognitive impairment is very important. With different types of immersion, a deeper sense of immersion can provide the users with more real experience. This might explain why full immersive and semi-immersive VR showed efficacy. Previous studies demonstrated that the more sophisticated VR technology used, in terms of the degree of immersion, their participants would get deeper experience of the virtual environment (Tieri et al., 2018). One thing we have noticed is that there is no statistical significance in low immersive VR type, however, it is highly accepted by the older adults, due to low “cibersikness” symptomatology (An and Park, 2018). The subgroup analysis did not show obvious differences based on training time. However, we believe that total training time may be a factor affecting the outcome. Therefore, more attention should be paid to training time in future studies. We found VR interventions had better effectiveness than traditional strategies in the control group, no matter whether the intervention in the control group was active or passive.
Mechanism and Shortcomings of VR
The mechanism behind VR technology for patients with MCI or dementia is still unclear and few studies have tried to explore it. As mentioned before, VR intervention has two unique features, immersion and interaction, which are thought to be the mechanisms behind the beneficial effect of VR technology. The feature of immersion can bring the sense of embodiment (Tieri et al., 2018), just likes “Avatar” to simulate a virtual body that substitutes the real one is able to elicit illusory sensations that virtual body itself belongs to the observer (Slater et al., 2008). According to neuroscience, to regulate and control the body in the world effectively, the brain creates an embodied simulation of the body in the world used to represent and predict actions, concepts, and emotions (Barrett, 2017), and VR just shares the similar basic mechanism to the brain to bring the simulated body into the virtual environment. The feature of immersion can elicit real physiological and psychological reactions (Meehan et al., 2005; Miller et al., 2008). For example, Salter (Slater et al., 2008) showed electromyography activity on the right arm of the participants when they observed an embodied virtual limb rotating on itself. At the same time, exposure to an immersive virtual environment having a whole hole on the floor has been shown to be able to elicit a stressful state determined by an increase in heart rate (Meehan et al., 2005). In our subgroup analysis, we have found that low immersive VR has no statistical significance in patients with MCI or dementia, but semi- and full immersive VR have, indicating that immersion plays an important role in the improvement of function in these patients.
Another unique feature is interaction which plays an important role in the immersion, feeds back the information in real time and has a reward system. By combining different external equipment such as motion tracker, headphone, haptic gloves, we can deliver the information of changes in the real body, resulting from the reactions to virtual environment, to the virtual body and easily set up the association of the virtual body with the virtual environment (Slater, 1999). In addition, the feedback system can provide the participants with the instant information that can be used to reinforce control of movement parameters and to reduce compensation movements (Subramanian et al., 2013) from judging these details. Finally, the reward system can arise the motivation of the participants to facilitate the repetition of body movement as well as improve patient compliance, treatment endurance, and happiness (Burdea et al., 2015; De Luca et al., 2018). Schmidt et al. (2012) also found that neuroplasticity process in the ventral striatum by connecting the motor and cognitive circuits can be enhanced by rewards.
However, these two features of VR technology are not independent and they influence each other. Therefore, to avoid one and explore the mechanism of the other is difficult. The above mechanism is our speculation, so future trials should try to set up a reasonable comparable group to explore these features respectively and help clarify the underlying mechanism of VR.
Furthermore, among current trials of VR interventions for patients with MCI or dementia, there are still few limitations. First, all of 11 included studies are goal-orientated that could be difficult for patients with severe cognitive impairment, like dementia, to finish such tasks. Our subgroup analysis showing no statistical significance in dementia may be the result of training difficulty. The efficacy of VR may reduce because the majority of the VR devices used are commercialized and few have adjusted their components to fit the needs of these patients. Second, although VR as a non-invasive, non-pharmacological cognitive rehabilitation intervention has gained increasing attention in recent years, health and safety must be taken into consideration, especially when intended for the use in older adults with neurodegenerative diseases. Cyber-sickness, a visually induced motion sickness reaction (Nooij et al., 2018) that can arise during or after immersion with a virtual environment depending on the level of immersion, should be a matter of concern in clinical settings (Bohil et al., 2011). Third, the conditions of the intervention between the groups are not the same among the included trials. By comparing differences between VR and control groups, we found there were more than one difference among these trials such as instant adjustment of difficulty, feedback system, and virtual environment. The question arises that whether one of them, like the element of the task, will produce the same efficacy as VR or no. The best way to answer this question is to find articles where the VR group set up a task and the control group performed the same task without VR at the same time. However, there is still a lack of such articles. The goal-oriented training has been proved to be effective in the recovery of many neurological diseases and all of the included studies used it in their VR training. At the same time, an advantage of VR is to combine such elements into their training. In future trials, it would be better to design a protocol to explore a single variable between experimental and control groups which could help us to learn more about the efficacy of that specific variable.
Strengths and Limitations
The strength of this meta-analysis is that only RCTs were included. Furthermore, to the best of our knowledge, this is the first meta-analysis to focus on both cognitive and motor functions as well as specific cognitive domains. However, several limitations should be addressed. First, the intervention characteristics varied between studies. The optimal frequency and duration of intervention remain to be explored to maximize intervention effects. Second, as the training sessions in each study are diverse, we only evaluated the immediate results after VR intervention to avoid bias. Therefore, the follow-up effect of VR in MCI or dementia was not analyzed so we could not estimate whether VR can prevent long-term worsening of dementia or conversion of MCI to dementia without persistent training. Third, due to the relatively small number of participants in the included studies, it is statistically inappropriate to analyze the impact of varied intervention components or different subtypes and severities of MCI or dementia.
Implications for Future Studies
Further research is needed to explore the most effective characteristics of VR intervention, specifically examining type, frequency, and duration of intervention as well as immersion. In addition, large sample studies are needed and long-term effects should be studied to gain insight into possible maintenance effects. Finally, future studies should investigate the effects of VR intervention on changes in neuroimaging findings and molecular markers.
Conclusion
Our meta-analysis shows that VR intervention is a beneficial non-pharmacological approach to improve cognitive and motor function in older adults with MCI or dementia, especially in attention/execution, memory, global cognition, and balance. Moreover, VR intervention does not show superiority on visuospatial ability and gait performance. The clinical relevance of our findings remains to be confirmed in future research.
Data Availability Statement
The raw data supporting the conclusions of this article will be made available by the authors, without undue reservation.
Author Contributions
SZ, YSu, YSh, and YZ chose the topic. SZ, YSu, and YSh performed the analysis. SZ, YSu, and YZ analyzed the data. CG, TW, and NA participated in the whole process. CG and TW made final decisions. All authors contributed to writing of this manuscript.
Funding
This work was supported by the National Key R&D Program of China [Grant No.: 2018YFC2001600 and 2018YFC2001603].
Conflict of Interest
The authors declare that the research was conducted in the absence of any commercial or financial relationships that could be construed as a potential conflict of interest.
Acknowledgments
We thank the authors of the primary studies for providing their data and other critical information. Additionally, the authors would like to thank the researchers and participants for their valuable contributions to this article.
Supplementary Material
The Supplementary Material for this article can be found online at: https://www.frontiersin.org/articles/10.3389/fnagi.2021.586999/full#supplementary-material
References
Albert, M. S., DeKosky, S. T., Dickson, D., Dubois, B., Feldman, H. H., Fox, N. C., et al. (2011). The diagnosis of mild cognitive impairment due to Alzheimer's disease: recommendations from the National Institute on Aging-Alzheimer's Association workgroups on diagnostic guidelines for Alzheimer's disease. Alzheimers Dement. 7, 270–279. doi: 10.1016/j.jalz.2011.03.008
Allali, G., and Verghese, J. (2017). Management of gait changes and fall risk in MCI and dementia. Curr. Treat. Options Neurol. 19:29. doi: 10.1007/s11940-017-0466-1
Aminov, A., Rogers, J. M., Middleton, S., Caeyenberghs, K., and Wilson, P. H. (2018). What do randomized controlled trials say about virtual rehabilitation in stroke? a systematic literature review and meta-analysis of upper-limb and cognitive outcomes. J. Neuroeng. Rehabil. 15:29. doi: 10.1186/s12984-018-0370-2
An, C.-M., and Park, Y.-H. (2018). The effects of semi-immersive virtual reality therapy on standing balance and upright mobility function in individuals with chronic incomplete spinal cord injury: a preliminary study. J. Spinal Cord Med. 41, 223–229. doi: 10.1080/10790268.2017.1369217
Appollonio, I., Leone, M., Isella, V., Piamarta, F., Consoli, T., Villa, M. L., et al. (2005). The Frontal Assessment Battery (FAB): normative values in an Italian population sample. Neurol. Sci. 26, 108–116. doi: 10.1007/s10072-005-0443-4
Arnett, J. A., and Labovitz, S. S. (1995). Effect of physical layout in performance of the Trail Making Test. Psychol. Assess. 7, 220–221. doi: 10.1037/1040-3590.7.2.220
Aruanno, B., and Garzotto, F. (2019). MemHolo: mixed reality experiences for subjects with Alzheimer's disease. Multimed. Tools Appl. 78, 13517–13537. doi: 10.1007/s11042-018-7089-8
Arvanitakis, Z., Shah, R. C., and Bennett, D. A. (2019). Diagnosis and management of dementia: review. JAMA 322, 1589–1599. doi: 10.1001/jama.2019.4782
Barrett, L. F. (2017). The theory of constructed emotion: an active inference account of interoception and categorization. Soc. Cogn. Affect. Neurosci. 12, 1–23. doi: 10.1093/scan/nsx060
Bloem, B. R., Grimbergen, Y. A., van Dijk, J. G., and Munneke, M. (2006). The “posture second” strategy: a review of wrong priorities in Parkinson's disease. J. Neurol. Sci. 248, 196–204. doi: 10.1016/j.jns.2006.05.010
Bohil, C. J., Alicea, B., and Biocca, F. A. (2011). Virtual reality in neuroscience research and therapy. Nat. Rev. Neurosci. 12, 752–762. doi: 10.1038/nrn3122
Burdea, G., Polistico, K., Liu, R., House, G., Muñiz, R., Macaro, N., . (2015). “BrightBrainer feasibility study in a medical adult day program,” in International Conference on Virtual Rehabilitation (ICVR); 2015 9-12 June 2015. (Valencia).
D'Cunha, N. M., Georgousopoulou, E. N., Dadigamuwage, L., Kellett, J., Panagiotakos, D. B., Thomas, J., et al. (2018). Effect of long-term nutraceutical and dietary supplement use on cognition in the elderly: a 10-year systematic review of randomised controlled trials. Br. J. Nutr. 119, 280–298. doi: 10.1017/S0007114517003452
D'Cunha, N. M., Nguyen, D., Naumovski, N., McKune, A. J., Kellett, J., Georgousopoulou, E. N., et al. (2019). A mini-review of virtual reality-based interventions to promote well-being for people living with dementia and mild cognitive impairment. Gerontology 65, 430–440. doi: 10.1159/000500040
De Luca, R., Russo, M., Naro, A., Tomasello, P., Leonardi, S., Santamaria, F., et al. (2018). Effects of virtual reality-based training with BTs-Nirvana on functional recovery in stroke patients: preliminary considerations. Int. J. Neurosci. 128, 791–796. doi: 10.1080/00207454.2017.1403915
Delbroek, T., Vermeylen, W., and Spildooren, J. (2017). The effect of cognitive-motor dual task training with the biorescue force platform on cognition, balance, and dual task performance in institutionalized older adults: a randomized controlled trial. J. Phys. Ther. Sci. 29, 1137–1143. doi: 10.1589/jpts.29.1137
Erdodi, L. A., Dunn, A. G., Seke, K. R., Charron, C., McDermott, A., Enache, A., et al. (2018). The boston naming test as a measure of performance validity. Psychol. Inj. Law 11, 1–8. doi: 10.1007/s12207-017-9309-3
Folstein, M. F., Folstein, S. E., and McHugh, P. R. (1975). “Mini-mental state.” a practical method for grading the cognitive state of patients for the clinician. J. Psychiatr. Res. 12, 189–198. doi: 10.1016/0022-3956(75)90026-6
García-Betances, R. I., Jiménez-Mixco, V., Arredondo, M. T., and Cabrera-Umpiérrez, M. F. (2015). Using virtual reality for cognitive training of the elderly. Am. J. Alzheimer's Dis. Other Dement. 30, 49–54. doi: 10.1177/1533317514545866
Higgins, J. P., Altman, D. G., Gøtzsche, P. C., Jüni, P., Moher, D., Oxman, A. D., et al. (2011). The Cochrane collaboration's tool for assessing risk of bias in randomised trials. BMJ 343:d5928. doi: 10.1136/bmj.d5928
Higgins, J. P. T., Thomas, J., Cumpston, M., Li, T., Page, M. J., et al. (2020). Cochrane Handbook for Systematic Reviews of Interventions. London: Cochrane. Available online at: www.trainingcochrane.org/handbook (accessed September, 2020).
Hill, N. T., Mowszowski, L., Naismith, S. L., Chadwick, V. L., Valenzuela, M., and Lampit, A. (2017). Computerized cognitive training in older adults with mild cognitive impairment or dementia: a systematic review and meta-analysis. Am. J. Psychiatry 174, 329–340. doi: 10.1176/appi.ajp.2016.16030360
Hughes, T. F., Flatt, J. D., Fu, B., Butters, M. A., Chang, C. C., and Ganguli, M. (2014). Interactive video gaming compared with health education in older adults with mild cognitive impairment: a feasibility study. Int. J. Geriatr. Psychiatry 29, 890–898. doi: 10.1002/gps.4075
Hwang, J., and Lee, S. (2017). The effect of virtual reality program on the cognitive function and balance of the people with mild cognitive impairment. J. Phys. Ther. Sci. 29, 1283–1286. doi: 10.1589/jpts.29.1283
Karssemeijer, E. G. A., Aaronson, J. A., Bossers, W. J., Smits, T., Olde Rikkert, M. G. M., and Kessels, R. P. C. (2017). Positive effects of combined cognitive and physical exercise training on cognitive function in older adults with mild cognitive impairment or dementia: a meta-analysis. Ageing Res. Rev. 40, 75–83. doi: 10.1016/j.arr.2017.09.003
Kim, O., Pang, Y., and Kim, J. H. (2019). The effectiveness of virtual reality for people with mild cognitive impairment or dementia: a meta-analysis. BMC Psychiatry 19:219. doi: 10.1186/s12888-019-2180-x
Lampit, A., Hallock, H., and Valenzuela, M. (2014). Computerized cognitive training in cognitively healthy older adults: a systematic review and meta-analysis of effect modifiers. PLoS Med. 11:e1001756. doi: 10.1371/journal.pmed.1001756
Langa, K. M., and Levine, D. A. (2014). The diagnosis and management of mild cognitive impairment: a clinical review. JAMA 312, 2551–2561. doi: 10.1001/jama.2014.13806
Lee, D. Y., Lee, K. U., Lee, J. H., Kim, K. W., Jhoo, J. H., Kim, S. Y., et al. (2004). A normative study of the CERAD neuropsychological assessment battery in the Korean elderly. J. Int. Neuropsychol. Soc. 10, 72–81. doi: 10.1017/S1355617704101094
Lee, J. H., Lee, K. U., Lee, D. Y., Kim, K. W., Jhoo, J. H., Kim, J. H., et al. (2002). Development of the Korean version of the Consortium to Establish a Registry for Alzheimer's Disease Assessment Packet (CERAD-K): clinical and neuropsychological assessment batteries. J Gerontol B Psychol Sci Soc Sci 57(1):P47-P53. PubMed PMID: 11773223.
Lei, C., Sunzi, K., Dai, F., Liu, X., Wang, Y., Zhang, B., et al. (2019). Effects of virtual reality rehabilitation training on gait and balance in patients with Parkinson's disease: a systematic review. PLoS ONE 14:e0224819. doi: 10.1371/journal.pone.0224819
Lezak, M. D. (1998). Tests: One Old, One New (Mostly). Rey auditory and verbal learning test. A Handbook, by Michael Schmidt. 1996. Los Angeles, CA: Western Psychological Services. 137 pp., $49.50. The camden memory tests, by Elizabeth K. Warrington. 1996. Hove, U.K.: Psychology Press (Erlbaum, Taylor andamp; Francis). Manual. 16 pp., $9.95. ISBN: 0-86377-379-6. short recognition memory test for words, $30.00. ISBN: 0-86377-429-6; short recognition memory test for faces, $35.00. ISBN: 0-86377-430-X; paired associate learning test, $35.00. ISBN: 0-86377-428-8; topographical recognition memory test, $85.00. ISBN: 0-86377-427-X; pictorial recognition test, $85.00. ISBN: 0-86377-426-1. J. Int. Neuropsychol. Soc. 4, 410–414. doi: 10.1017/S1355617798224106
Li, H., Li, J., Li, N., Li, B., Wang, P., and Zhou, T. (2011). Cognitive intervention for persons with mild cognitive impairment: a meta-analysis. Ageing Res. Rev. 10, 285–296. doi: 10.1016/j.arr.2010.11.003
Liao, Y.-Y., Chen, I. H., Lin, Y.-J., Chen, Y., and Hsu, W.-C. (2019). Effects of virtual reality-based physical and cognitive training on executive function and dual-task gait performance in older adults with mild cognitive impairment: a randomized control trial. Front. Aging Neurosci. 11:162. doi: 10.3389/fnagi.2019.00162
Maggio, M. G., Latella, D., Maresca, G., Sciarrone, F., Manuli, A., Naro, A., et al. (2019). Virtual reality and cognitive rehabilitation in people with stroke: an overview. J. Neurosci. Nurs. 51, 101–105. doi: 10.1097/JNN.0000000000000423
Maher, C. G., Sherrington, C., Herbert, R. D., Moseley, A. M., and Elkins, M. (2003). Reliability of the PEDro scale for rating quality of randomized controlled trials. Phys. Ther. 83, 713–721. PubMed PMID: 12882612. doi: 10.1093/ptj/83.8.713
Makizako, H., Shimada, H., Park, H., Doi, T., Yoshida, D., Uemura, K., et al. (2013). Evaluation of multidimensional neurocognitive function using a tablet personal computer: test–retest reliability and validity in community-dwelling older adults. Geriatr. Gerontol. Int. 13, 860–866. doi: 10.1111/ggi.12014
Man, D. W., Chung, J. C., and Lee, G. Y. (2012). Evaluation of a virtual reality-based memory training programme for Hong Kong Chinese older adults with questionable dementia: a pilot study. Int. J. Geriatr. Psychiatry 27, 513–520. doi: 10.1002/gps.2746
Meehan, M., Razzaque, S., Insko, B., Whitton, M., and Brooks, F. P. Jr. (2005). Review of four studies on the use of physiological reaction as a measure of presence in stressful virtual environments. Appl. Psychophysiol. Biofeedback 30, 239–258. doi: 10.1007/s10484-005-6381-3
Miller, W. L., Maffei, V., Bosco, G., Iosa, M., Zago, M., Macaluso, E., et al. (2008). Vestibular nuclei and cerebellum put visual gravitational motion in context. J. Neurophysiol. 99, 1969–1982. doi: 10.1152/jn.00889.2007
Moher, D., Liberati, A., Tetzlaff, J., and Altman, D. G. (2009). Preferred reporting items for systematic reviews and meta-analyses: the PRISMA statement. BMJ Clin. Res. Ed. 339:b2535. doi: 10.1136/bmj.b2535
Monaco, M., Costa, A., Caltagirone, C., and Carlesimo, G. A. (2013). Forward and backward span for verbal and visuo-spatial data: standardization and normative data from an Italian adult population. Neurol. Sci. 34, 749–754. doi: 10.1007/s10072-012-1130-x
Monaco, M., Costa, A., Caltagirone, C., and Carlesimo, G. A. (2015). Erratum to: forward and backward span for verbal and visuo-spatial data: standardization and normative data from an Italian adult population. Neurol. Sci. 36, 345–347. doi: 10.1007/s10072-014-2019-7
Muir, S. W., Berg, K., Chesworth, B., and Speechley, M. (2008). Use of the berg balance scale for predicting multiple falls in community-dwelling elderly people: a prospective study. Phys. Ther. 88, 449–459. doi: 10.2522/ptj.20070251
Nasreddine, Z. S., Phillips, N. A., Bédirian, V., Charbonneau, S., Whitehead, V., Collin, I., et al. (2005). The montreal cognitive assessment, MoCA: a brief screening tool for mild cognitive impairment. J. Am. Geriatr. Soc. 53, 695–699. doi: 10.1111/j.1532-5415.2005.53221.x
Neri, S. G., Cardoso, J. R., Cruz, L., Lima, R. M., de Oliveira, R. J., Iversen, M. D., et al. (2017). Do virtual reality games improve mobility skills and balance measurements in community-dwelling older adults? Systematic review and meta-analysis. Clin. Rehabil. 31, 1292–12304. doi: 10.1177/0269215517694677
Newton, H. J., Cox, N. J., Diebold, F. X., Garrett, J. M., Pagano, M., and Royston, J. P. (1998). A Publication to Promote Communication Among Stata Users. Stata Corporation. Available online at: https://www.stata.com/products/stb/journals/stb44.pdf
Nooij, S. A. E., Pretto, P., and Bülthoff, H. H. (2018). More vection means more velocity storage activity: a factor in visually induced motion sickness? Exp. Brain Res. 236, 3031–3041. doi: 10.1007/s00221-018-5340-1
Padala, K. P., Padala, P. R., Malloy, T. R., Geske, J. A., Dubbert, P. M., Dennis, R. A., et al. (2012). Wii-fit for improving gait and balance in an assisted living facility: a pilot study. J. Aging Res. 2012:597573. doi: 10.1155/2012/597573
Pai, Y. C., and Patton, J. (1997). Center of mass velocity-position predictions for balance control. J. Biomech. 30, 347–354. doi: 10.1016/S0021-9290(96)00165-0
Park, E., Yun, B. J., Min, Y. S., Lee, Y. S., Moon, S. J., Huh, J. W., et al. (2019). Effects of a mixed reality-based cognitive training system compared to a conventional computer-assisted cognitive training system on mild cognitive impairment: a pilot study. Cogn. Behav. Neurol. 32, 172–178. doi: 10.1097/WNN.0000000000000197
Petersen, R. C., Lopez, O., Armstrong, M. J., Getchius, T. S. D., Ganguli, M., Gloss, D., et al. (2018). Author response: practice guideline update summary: mild cognitive impairment: report of the guideline development, dissemination, and implementation subcommittee of the American Academy of Neurology. Neurology 91, 373–374. doi: 10.1212/WNL.0000000000006038
Podsiadlo, D., and Richardson, S. (1991). The timed “Up and Go”: a test of basic functional mobility for frail elderly persons. J. Am. Geriatr. Soc. 39, 142–148. doi: 10.1111/j.1532-5415.1991.tb01616.x
Roberts, R. O., Knopman, D. S., Mielke, M. M., Cha, R. H., Pankratz, V. S., Christianson, T. J., et al. (2014). Higher risk of progression to dementia in mild cognitive impairment cases who revert to normal. Neurology 82, 317–325. doi: 10.1212/WNL.0000000000000055
Rosenblad, A. (2009). Introduction to meta-analysis by Michael Borenstein, Larry V. Hedges, Julian P.T. Higgins, Hannah R. Rothstein. Int. Stat. Rev. 77, 478–479. doi: 10.1111/j.1751-5823.2009.00095_15.x
Santos, P., Scaldaferri, G., Santos, L., Ribeiro, N., Neto, M., and Melo, A. (2019). Effects of the Nintendo Wii training on balance rehabilitation and quality of life of patients with Parkinson's disease: a systematic review and meta-analysis. NeuroRehabilitation 44, 569–577. doi: 10.3233/NRE-192700
Saxton, J., Morrow, L., Eschman, A., Archer, G., Luther, J., and Zuccolotto, A. (2009). Computer assessment of mild cognitive impairment. Postgrad. Med. 121, 177–185. doi: 10.3810/pgm.2009.03.1990
Scarpina, F., and Tagini, S. (2017). The stroop color and word test. Front. Psychol. 8:557. doi: 10.3389/fpsyg.2017.00557
Schmidt, L., Lebreton, M., Cléry-Melin, M.-L., Daunizeau, J., and Pessiglione, M. (2012). Neural mechanisms underlying motivation of mental versus physical effort. PLoS Biol. 10:e1001266. doi: 10.1371/journal.pbio.1001266
Schwenk, M., Sabbagh, M., Lin, I., Morgan, P., Grewal, G. S., Mohler, J., et al. (2016). Sensor-based balance training with motion feedback in people with mild cognitive impairment. J. Rehabil. Res. Dev. 53, 945–958. doi: 10.1682/JRRD.2015.05.0089
Serino, S., Pedroli, E., Tuena, C., De Leo, G., Stramba-Badiale, M., Goulene, K., et al. (2017). A novel virtual reality-based training protocol for the enhancement of the “mental frame syncing” in individuals with Alzheimer's disease: a development-of-concept trial. Front. Aging Neurosci. 9:240. doi: 10.3389/fnagi.2017.00240
Shin, M.-S., Park, S.-Y., Park, S.-R., Seol, S.-H., and Kwon, J. S. (2006). Clinical and empirical applications of the Rey-Osterrieth Complex Figure Test. Nat. Protoc. 1, 892–899. doi: 10.1038/nprot.2006.115
Slater, M. (1999). Measuring presence: a response to the witmer and singer presence questionnaire. Presence Teleop. Virtual Environ. 8, 560–565. doi: 10.1162/105474699566477
Slater, M., Pérez Marcos, D., Ehrsson, H., and Sanchez-Vives, M. (2008). Towards a digital body: the virtual arm illusion. Front. Hum. Neurosci. 2:6. doi: 10.3389/neuro.09.006.2008
Subramanian, S. K., Lourenço, C. B., Chilingaryan, G., Sveistrup, H., and Levin, M. F. (2013). Arm motor recovery using a virtual reality intervention in chronic stroke: randomized control trial. Neurorehabil. Neural Repair 27, 13–23. doi: 10.1177/1545968312449695
Tarnanas, I., Tsolakis, A., and Tsolaki, M. (2014). Assessing Virtual Reality Environments as Cognitive Stimulation Method for Patients With MCI. Technologies of Inclusive Well-Being. Studies in Computational Intelligence. Berlin Heidelberg: Springer, 39–74.
Thapa, N., Park, H. J., Yang, J. G., Son, H., Jang, M., Lee, J., et al. (2020). The effect of a virtual reality-based intervention program on cognition in older adults with mild cognitive impairment: a randomized control trial. J. Clin. Med. 9:1283.doi: 10.3390/jcm9051283
Tieri, G., Morone, G., Paolucci, S., and Iosa, M. (2018). Virtual reality in cognitive and motor rehabilitation: facts, fiction, and fallacies. Expert Rev. Med. Devices 15, 107–117. doi: 10.1080/17434440.2018.1425613
Tinetti, M. E., Williams, T. F., and Mayewski, R. (1986). Fall risk index for elderly patients based on number of chronic disabilities. Am. J. Med. 80, 429–434. doi: 10.1016/0002-9343(86)90717-5
Keywords: meta-analysis, cognition, motor, virtual reality, mild cognitive impairment, dementia
Citation: Zhu S, Sui Y, Shen Y, Zhu Y, Ali N, Guo C and Wang T (2021) Effects of Virtual Reality Intervention on Cognition and Motor Function in Older Adults With Mild Cognitive Impairment or Dementia: A Systematic Review and Meta-Analysis. Front. Aging Neurosci. 13:586999. doi: 10.3389/fnagi.2021.586999
Received: 24 July 2020; Accepted: 06 April 2021;
Published: 05 May 2021.
Edited by:
Elizabeta Blagoja Mukaetova-Ladinska, University of Leicester, United KingdomReviewed by:
Rocco Salvatore Calabrò, Centro Neurolesi Bonino Pulejo (IRCCS), ItalyValérie Gyselinck, Institut Français des Sciences et Technologies des Transports, de l'Aménagement et des Réseaux (IFSTTAR), France
Copyright © 2021 Zhu, Sui, Shen, Zhu, Ali, Guo and Wang. This is an open-access article distributed under the terms of the Creative Commons Attribution License (CC BY). The use, distribution or reproduction in other forums is permitted, provided the original author(s) and the copyright owner(s) are credited and that the original publication in this journal is cited, in accordance with accepted academic practice. No use, distribution or reproduction is permitted which does not comply with these terms.
*Correspondence: Tong Wang, d2FuZ3Rvbmc2MDYyMUAxNjMuY29t; Chuan Guo, Z3VvY2h1YW5yZWhhYkAxMjYuY29t
†These authors have contributed equally to this work and share first authorship