- 1Department of Community Healthcare and Geriatrics, Nagoya University Graduate School of Medicine, Nagoya, Japan
- 2Center for Comprehensive Care and Research on Memory Disorders, National Center for Geriatrics and Gerontology, Obu, Japan
- 3National Center for Geriatrics and Gerontology, Obu, Japan
A growing body of evidence clearly indicates the beneficial effects of physical activity (PA) on cognition. The importance of PA is now being reevaluated due to the increase in sedentary behavior in older adults during the COVID-19 pandemic. Although many studies in humans have revealed that PA helps to preserve brain health, the underlying mechanisms have not yet been fully elucidated. In this review, which mainly focuses on studies in humans, we comprehensively summarize the mechanisms underlying the beneficial effects of PA or exercise on brain health, particularly cognition. The most intensively studied mechanisms of the beneficial effects of PA involve an increase in brain-derived neurotrophic factor (BDNF) and preservation of brain volume, especially that of the hippocampus. Nonetheless, the mutual associations between these two factors remain unclear. For example, although BDNF presumably affects brain volume by inhibiting neuronal death and/or increasing neurogenesis, human data on this issue are scarce. It also remains to be determined whether PA modulates amyloid and tau metabolism. However, recent advances in blood-based biomarkers are expected to help elucidate the beneficial effects of PA on the brain. Clinical data suggest that PA functionally modulates cognition independently of neurodegeneration, and the mechanisms involved include modulation of functional connectivity, neuronal compensation, neuronal resource allocation, and neuronal efficiency. However, these mechanisms are as yet not fully understood. A clear understanding of the mechanisms involved could help motivate inactive persons to change their behavior. More accumulation of evidence in this field is awaited.
Introduction
Cognitive decline and dementia are major health concerns worldwide. A major cause of dementia is Alzheimer’s disease (AD) and very recently pharmacological treatment with aducanumab was approved in the United States for AD. However, the effects of this drug are far from a “cure” and other therapeutics are needed.
Cumulative research results clearly indicate the beneficial effects of physical activity (PA) on brain health, and some reports have suggested that physical inactivity in older adults caused by COVID-19 pandemic-related lockdowns have had a negative impact on brain health (Fiorenzato et al., 2021; Ismail et al., 2021; Tondo et al., 2021; Znazen et al., 2021).
Physical activity has been found to have a positive impact on cognition in a wide range of individuals, from those who are cognitively normal to those who have dementia (Heyn et al., 2004; Aarsland et al., 2010; Sofi et al., 2011; Groot et al., 2016). Many basic, epidemiological, and observational cohort studies, as well as randomized controlled studies, have provided evidence of the beneficial effects of PA on cognition (Sofi et al., 2011). Various studies, mainly epidemiological, have also reported that PA reduces the risk of onset of dementia (Hamer and Chida, 2009). However, the mechanism underlying these beneficial effects of PA on brain health has not been completely elucidated. A neurodegenerative process may be accelerated with aging through pathological changes, oxidative stress, or inflammation, and PA may counteract these neurodegenerative processes. PA may prevent the development of brain pathologies, including AD-related and cerebrovascular pathologies, although some evidence indicates that the cognitive benefits of PA are independent of pathological changes in the brain (Buchman et al., 2019). PA may protect the gray and white matter structure of the brain and may also enhance physiological aspects of the brain, including cerebral blood flow and neurotrophic factor release. Functional enhancement of each neuron and the neural network as a whole may also be involved.
This review, which largely focuses on human studies, describes the various mechanisms of the brain-protective effects induced by PA. We divide the beneficial mechanisms of PA or exercise into several components and review the structural, physiological, anti-neurodegenerative, and functional effects of PA or exercise (Table 1).
Effects on Brain Structure
Brain Volume
The volume of the brain declines with aging, starting in midlife (Scahill et al., 2003). This reduction in brain volume is presumed to reflect decreases in neuron volume and number (Gogniat et al., 2021). One of the mechanisms underlying the brain-protective effects of PA is the preservation of brain volume, or even an increase in brain volume. Observational studies have found that brain volume, particularly that of the hippocampus, is preserved in more active people (Erickson et al., 2010; Flöel et al., 2010; Maasakkers et al., 2021). PA is also associated with preservation of cortical thickness (Walhovd et al., 2014; Lee et al., 2016).
Many interventional trials have also shown the positive impact of PA on brain volume. Some studies showed that PA interventions reduced the development of brain atrophy compared with controls (Best et al., 2015), and some studies even demonstrated an increase in brain volume after an exercise intervention (Colcombe et al., 2006; Tao et al., 2017) in older adults. A systematic review of the effects of exercise intervention on the hippocampus concluded that aerobic exercise helped to preserve hippocampal volume by preventing its decrease, while some of the included studies even showed an increased volume (Firth et al., 2018). The characteristics of these representative studies are summarized in Table 2.
Although the precise mechanisms remain to be elucidated, the neuroprotective effects of PA may be exerted through increased brain-derived neurotrophic factor (BDNF) and blood flow or reduced oxidative stress and amyloid accumulation (as discussed below). Recently, the MAPT study reported that more physically active individuals had lower blood concentrations of neurofilament light chain, a well-established biomarker of neurodegeneration (Raffin et al., 2021). These results suggest that PA may ameliorate neurodegeneration. Moreover, decades of research have shown that adult neurogenesis persists throughout life, although it declines with aging. Hippocampal neurogenesis is reported to be crucial in learning and memory in rodent experiments (Babcock et al., 2021). PA may accelerate neurogenesis, particularly that of the hippocampus (Leal-Galicia et al., 2019). The increased brain volume may thus be due to enhanced neurogenesis. However, human evidence, particularly that from older adults, is scarce.
White Matter Integrity
White matter plays a crucial role in cognition by connecting different brain regions to enable efficient signal transmission. White matter in adult brains exhibits plasticity involving myelin formation and remodeling (Sampaio-Baptista and Johansen-Berg, 2017). Observational studies suggest that higher PA is associated with better white matter integrity as measured by diffusion tensor imaging on magnetic resonance imaging (MRI; Buchman et al., 2018; Franchetti et al., 2020; Wolf et al., 2020). Several interventional studies have found evidence of improved white matter integrity (Voss et al., 2013; Burzynska et al., 2017; Clark et al., 2019; Colmenares et al., 2021), but another study failed to show an effect (Venkatraman et al., 2020).
Small Vessel Disease
Small vessel disease is represented by white matter lesions (WMLs). T2-weighted or fluid-attenuated MRI images visualize WMLs as diffuse high-signal areas. WMLs have been linked to cognitive impairment (Alber et al., 2019). Although the reported results are not in complete agreement, several cross-sectional and longitudinal studies have shown that PA is associated with fewer WMLs (Torres et al., 2015; Moon et al., 2018). However, no relevant interventional studies have been reported.
Stroke
A systematic review concluded that PA reduced stroke risk (Wendel-Vos et al., 2004). Therefore, PA may contribute to reducing the risk of vascular cognitive impairment.
Physiological Mechanisms
Brain-Derived Neurotrophic Factor
Brain-derived neurotrophic factor is a neurotrophin that influences neuronal survival, differentiation, synapse generation, and long-term potentiation (Phillips et al., 2014). Decreased BDNF level is associated with neuropathological conditions, including mild cognitive impairment (MCI; Shimada et al., 2014). The blood concentrations of BDNF decrease with aging, and there is a strong correlation between the brain and blood levels of BDNF (Knaepen et al., 2010). A recent systematic review concluded that PA interventions increased the plasma level of BDNF in individuals with MCI or AD dementia (Ruiz-González et al., 2021). A pair of studies also showed that exercise increased plasma BDNF levels in cognitively normal older people (Müller et al., 2017; Rehfeld et al., 2018). The representative studies are summarized in Table 3.
Although the precise cause of the exercise-associated plasma BDNF increase has not yet been fully elucidated, some studies have shown increased BDNF in samples from the internal jugular vein after acute training (Rasmussen et al., 2009) and chronic regular training (Seifert et al., 2010), possibly suggesting that exercise-associated BDNF may have a central origin. Rasmussen et al. (2009) estimated that 70%–80 of circulating BDNF was derived from the brain and that the remaining levels were derived from peripheral sources. Thus, the question of whether circulating BDNF levels reflect those in the human brain warrants further study.
Insulin-Like Growth Factor 1
Circulating insulin-like growth factor 1 (IGF-1) passes through the blood–brain barrier, exerts neuroprotective effects, and induces synaptic plasticity (Sonntag et al., 2005). The upstream IGF-1 signaling pathway also induces BDNF expression (Yan et al., 2011). Interventional trials demonstrated that aerobic exercise increased plasma IGF-1 in older adults (Kang et al., 2020), including those with MCI (Baker et al., 2010) and AD (Stein et al., 2021). A systematic review concluded that resistance training also increased IGF-1 levels in participants, including older adults (Jiang et al., 2020).
Blood Flow
Cerebral blood flow decreases with aging and may be associated with cognitive decline (Tarumi and Zhang, 2018). This reduced blood flow may reflect a decreased cerebral metabolic rate (Marchal et al., 1992). Increased sympathetic nervous activity and impaired vasodilation may also contribute to the age-associated decline in cerebral blood flow. Cardiac output tends to decline with aging and may also be associated with reduced blood flow in the brain (Tarumi and Zhang, 2018). Regular exercise may help to counteract the effects of these aging-associated changes in cerebral blood flow.
Angiogenesis may also be involved in the ability of PA to increase blood flow (Swain et al., 2003). Vascular endothelial growth factor (VEGF) is an important regulator of angiogenesis, and several animal studies have shown increased VEGF levels with physical exercise. However, studies in humans have been inconclusive (Vital et al., 2014). Observational studies have found that sedentary older adults had lower cerebral blood flow than active older adults (Rogers et al., 1990; Thomas et al., 2013; Knight et al., 2021; Li et al., 2021). Recently, a small randomized controlled trial involving a 1-year aerobic exercise intervention reported increased cerebral blood flow in the exercise group (Tomoto et al., 2021).
Anti-Neurodegenerative Mechanisms
Amyloid β and Tau
Alzheimer’s disease is a major neurodegenerative disease and is the leading cause of dementia. The main pathological features of AD are accumulation of amyloid β (Aβ; senile plaques) and intracellular accumulation of hyper-phosphorylated tau (neurofibrillary tangles). PA has been associated with reduced risk of AD (Barnes and Yaffe, 2011). Extensive basic research results suggest that exercise may be associated with increased Aβ clearance (Vecchio et al., 2018), reduced Aβ production (Adlard et al., 2005), enhanced tau degeneration, and decreased tau phosphorylation (Brown et al., 2019). While animal studies have established that high PA leads to better amyloid profiles (Brown et al., 2019), human studies investigating the effects of PA on brain pathologies have been very difficult to do. However, recent advances in AD-related biomarkers are enabling research into the effects of PA on AD pathologies in human subjects.
Although cerebrospinal fluid biomarkers and amyloid PET imaging are the most frequently applied modalities in this field, the applicability of plasma biomarkers is gradually improving. Many observational studies involving measures of Aβ in cerebrospinal fluid (Liang et al., 2010; Brown et al., 2013, 2017; Law et al., 2018), plasma (Stillman et al., 2017), and PET (Head et al., 2012; Brown et al., 2013, 2017; Jeon et al., 2020; Treyer et al., 2021) have reported better profiles of amyloid-related biomarkers in active people but inconsistencies remain (de Souto Barreto et al., 2015; Jeon et al., 2020; Palta et al., 2020; Stojanovic et al., 2020). The representative studies are summarized in Table 4.
Observational studies, mainly retrospective in nature, have suggested that individuals with higher PA tend to have biomarker profiles indicative of lower Aβ deposition in the brain (Frederiksen et al., 2019). However, interventional studies with AD biomarkers are rare and have had relatively small sample sizes. Most obtained inconclusive results, although some showed favorable effects (Moniruzzaman et al., 2020). More studies are warranted.
Researchers have investigated the effects of PA on tau-related biomarkers. Some cross-sectional studies involving cerebrospinal fluid biomarkers (Liang et al., 2010) and tau PET (Brown et al., 2018) showed lower tau profiles in participants with high PA levels, but a longitudinal study did not find an interaction between PA and tau over time.
Oxidative Stress
Acute exercise increases oxidative stress, but regular PA is expected to regulate the cellular redox state of the brain, and PA-induced redox adaptation may contribute to the neuroprotective effects of PA (Radak et al., 2016). Animal studies showed that PA protected the brain from oxidative stress (Radák et al., 2001; Radak et al., 2006). A systematic review concluded that antioxidant indicators tend to increase and pro-oxidant indicators tend to decrease after resistance training in humans (de Sousa et al., 2017). However, another systematic review found no effects of resistance training on molecular oxidation and antioxidant capacity markers (Cuyul-Vásquez et al., 2020).
Nonetheless, studies of individuals with dementia or MCI are rare. Only Jensen et al. (2019) have measured the plasma level of 8-isoprostane, an oxidative stress marker, in AD participants before and after an exercise intervention and reported only non-significant changes.
Inflammation
Inflammation is one of the major mechanisms of neurodegeneration (Onyango et al., 2021). PA may have anti-inflammatory potential (Mee-Inta et al., 2019). Cross-sectional observational studies have reported lower levels of inflammatory markers (IL-6 and C-reactive protein) in active older adults (Reuben et al., 2003) and determined that active women had lower levels of the plasma inflammatory biomarker TNF-α (Castells-Sánchez et al., 2021). Another observational study also found low levels of IL-6, C-reactive protein, and TNF-α (Colbert et al., 2004).
Plasma IL-6 levels decrease in response to aerobic exercise in both MCI (Nascimento et al., 2014) and AD (Abd El-Kader and Al-Jiffri, 2016). In addition, levels of C-reactive protein, a representative inflammatory marker, decrease in response to exercise interventions (Muscari et al., 2010; Alghadir et al., 2016). A systematic review concluded that exercise was associated with a decrease in C-reactive protein levels regardless of age or sex (Fedewa et al., 2017). Another recent systematic review of exercise interventions in people with MCI or dementia reported that exercise significantly decreased the levels of IL-6 and TNF-α (Huang et al., 2021).
Functional Mechanisms
Functional Connectivity
Functional MRI enables functionally connected brain regions to be identified by measuring simultaneous activations via the blood oxygen level-dependent (BOLD) signals of spatially distinct regions. A systematic review by Li et al. (2017) suggested that aerobic exercise increases functional connectivity in the default mode network, which is associated with memory and abstract thinking. Recently, Bray et al. (2021) reviewed the effect of physical exercise on functional brain network connectivity in older adults with and without cognitive impairment and concluded that physical exercise increases functional brain network connectivity.
Allocation of Neuronal Circuits
Physical activity might somehow help to recruit new neuronal circuits that would be involved in processing tasks. An interesting fMRI study suggested that 6-week dance exercise training led to the involvement of the motor-related network during highly cognitive-demanding memory tasks, possibly as a compensatory mechanism. Exercise may accelerate the involvement of new networks in the cognitive process (Ji et al., 2018).
Event-related potentials are generated in response to specific events or stimuli such as audio sound. Studies involving the P3 component, the major focus of studies of event-related potentials, showed that exercise increases the amplitude of P3, possibly suggesting that exercise enhances the allocation of neural resources as part of a compensatory mechanism (Chang et al., 2015). However, the results are controversial (Gajewski and Falkenstein, 2018; Alatorre-Cruz et al., 2020).
Neuronal Efficiency
Exercise may increase neuronal efficiency. An fMRI study demonstrated that an exercise group had lower BOLD signals in the hippocampus and para-hippocampal gyrus compared with a non-exercise group during a memory-encoding task. Because the BOLD signal reflects brain activity in a specific region, lower BOLD signals during a certain task mean that the task was performed with less burden, which probably reflects higher neuronal efficiency in the hippocampus and para-hippocampal gyrus in the exercise group (Friedl-Werner et al., 2020). Another trial also showed that a 12-week exercise intervention reduced neuronal activation in several brain regions, including the prefrontal cortex, during a memory task (Nishiguchi et al., 2015).
Synaptic Plasticity
Synaptic plasticity (long-term potentiation and long-term depression) is a biological model for learning and memory processes. Animal studies have demonstrated that PA controls synaptic plasticity (Bettio et al., 2019). Synaptic plasticity can be non-invasively measured in humans via a combination of transcranial magnetic stimulation and recording of motor-evoked potentials. A recent study reported that PA was associated with long-term depression-like neuroplasticity in older adults (Smith et al., 2021).
Discussion
Numerous studies have established the beneficial effects of PA on brain health. Here, we attempted to comprehensively review the potential mechanisms underlying the effects of PA on brain health. Elucidation of the mechanisms may help to establish the optimal interventional approach in terms of therapeutic effects or even lead to the development of therapeutic mimics.
Increasing PA is a relatively safe and cheap way to maintain health, including brain health. However, sedentary people often struggle to modify their behavior. Clear messages explaining the intensity and frequency of exercise required to protect brain health may help motivate them to change their behavior and lifestyle. Moreover, improved understanding of the mechanisms of the effects is expected be important for behavioral change. People may want to know how PA works, and a clearer understanding of the mechanisms involved could help encourage behavioral change.
Several mechanisms have been summarized in the current review. Each mechanism is speculated to exert its effect both independently and interrelatedly (Figure 1). However, evidence linking these mechanisms and elucidating their interrelationship is largely lacking.
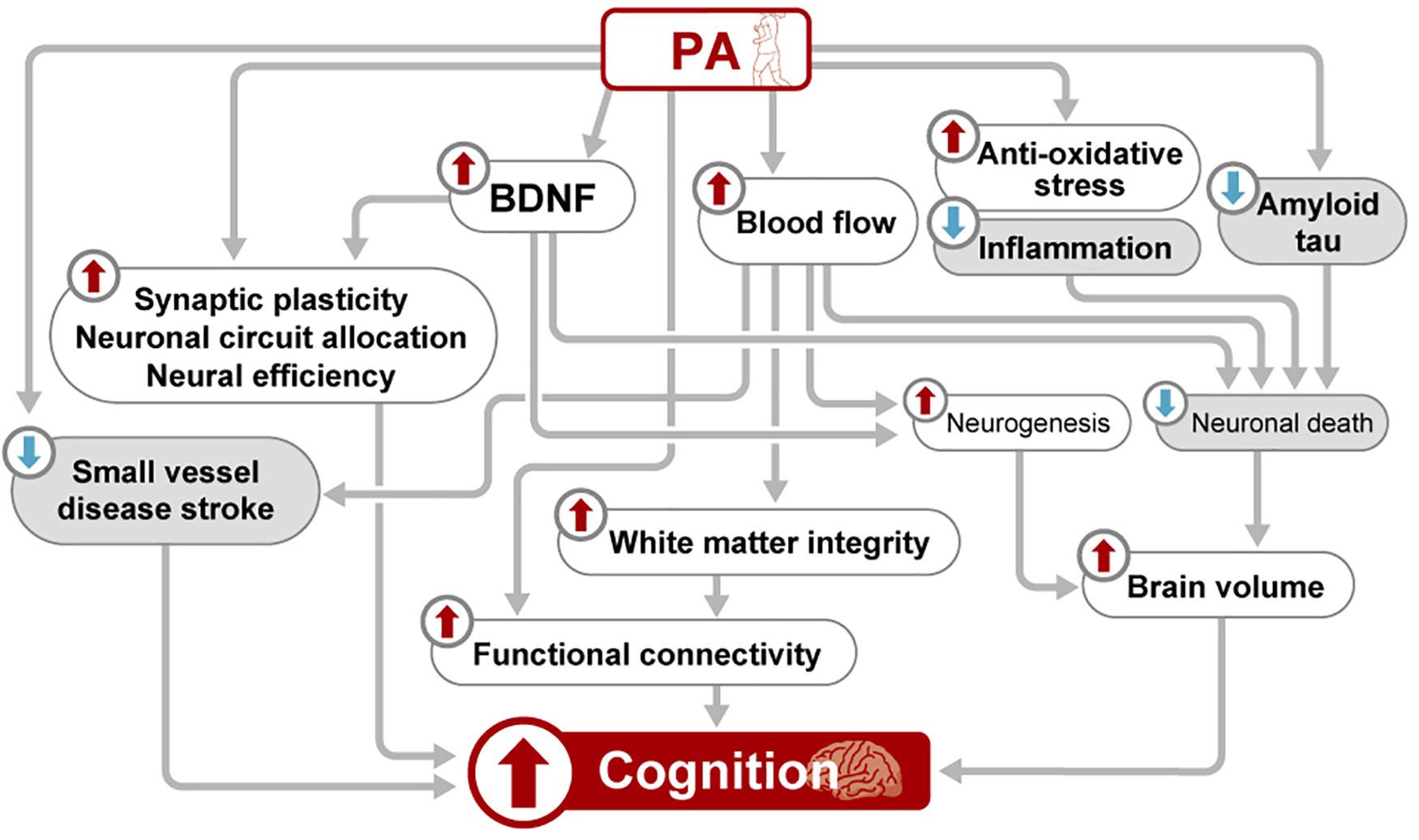
Figure 1. Schematic view of the mechanisms underlying the beneficial effects of PA on brain health. BDNF, brain-derived neurotrophic factor; PA, physical activity.
The most intensively studied topic and the one with the most accumulated evidence regarding the impact of PA on brain health is the increase in blood BDNF levels. However, the source of the BDNF production induced by PA is inconclusive, so it remains unclear whether the increase of BDNF in the blood actually reflects the increase of BDNF in the brain. Moreover, the underlying mechanisms by which increased BDNF affects human brain function have not been completely elucidated. BDNF is presumed to be associated with neuronal survival and neurogenesis, and it may also preserve white matter structure (Miyamoto et al., 2014). BDNF may play further roles in preserving brain health such as by modulating neuronal/synaptic activity and blood flow (Santhanam et al., 2010; Leal et al., 2015). From a practical point of view, more information is needed to determine the optimal exercise protocol in terms of intensity and frequency. The effects of PA on brain volume seem to be more or less established. However, whether PA merely decreases aging-related brain volume loss or even increases brain volume is still unclear, and this may be relevant to the issue of whether PA only prevents neurodegeneration or enhances neurogenesis in human brain. Also, it is largely unknown how long the effects of PA on brain volume last.
More importantly, the mechanisms by which PA affects human brain volume remain to be elucidated. BDNF may at least partly help to support brain volume preservation, possibly through neuronal survival and/or neurogenesis. However, it is not yet clear whether the preservation/increase of brain volume is due to the preservation/increase of the number of neurons in humans. There is room for further research. It also remains to be elucidated how an increase in BDNF might contribute to the preservation of brain volume in humans. The relative contribution of neurogenesis and prevention of neuronal death to the preservation of brain volume is not yet fully understood. Although BDNF has effects on neuronal preservation, PA may also counteract neurodegeneration through pathways different from those of BDNF, including antioxidative and anti-inflammatory effects.
Epidemiological evidence indicates that PA reduces the risk of clinically diagnosed AD (Buchman et al., 2012). Theoretically, several possibilities, which are not mutually exclusive, may be involved in the ability of PA to reduce the risk of a clinical presentation of AD: (1) PA directly modifies amyloid and tau metabolism; (2) PA reduces neurodegeneration provoked by the amyloid cascade; and (3) PA enhances brain function.
Some studies of AD-related biomarkers suggest that PA may directly modify AD-related pathologies. However, taken together, the results of these studies are as yet inconclusive. In particular, insufficient evidence has been accumulated from randomized controlled studies (Table 4). As discussed above, PA may have antioxidative and anti-inflammatory effects, and these effects may help neurons to survive in the neurodegenerative pathway initiated by Aβ, somewhere downstream of the amyloid cascade. It should be clarified whether PA directly modifies the amyloid cascade or exerts neuroprotective effects downstream of the cascade. Moreover, some studies have suggested that PA may have effects on cognition that are independent of pathological changes. Here, we reviewed several potential mechanisms distinct from neurodegeneration modification or ischemic pathologies. An increase in blood flow may be critical to the modification of brain function, with other contributions coming from maintained white matter microstructure and connectivity. Neuronal compensation, neuronal resource allocation, and neuronal efficiency are relatively under-studied areas and warrant further research. Also, studying a combination of several types of biomarkers—amyloid-related, tau-related, and neurodegeneration-related—might help to elucidate how PA prevents AD. Prospective interventional studies involving the combination of several biomarkers would be valuable.
To conclude, numerous studies have been conducted in this field and a substantial amount of evidence has been accumulated. Two points have been clarified so far, namely, the association of PA with increased blood levels of BDNF and its association with brain volume preservation. However, much remains to be elucidated. For example, the mechanism by which circulating BDNF affects the brain as well as the association between increased BDNF levels and neurogenesis in people are unclear. Although animal studies have demonstrated that BDNF increases neurogenesis in the hippocampus, the contribution of BDNF to neurogenesis in the context of human brain health, especially in older adults, has yet to be clarified. In addition, even though the effects of PA on AD-related pathologies have been extensively studied, the research results are inconsistent, and so it remains unclear whether PA is associated with less AD-related pathologies. Many of the studies conducted in this field have been small in scale and have employed cross-sectional designs. Larger longitudinal studies or RCTs are needed to understand the associations between PA and AD-related pathologies. In this review, many other potential mechanisms were discussed. Although these mechanisms are interesting and could possibly be correct, at this point clear evidence is lacking. It would be very important for people, especially those who lead sedentary lives, to know how PA affects their brain because such knowledge has the potential to motivate them to increase their PA. Also, elucidation of the mechanisms may lead to the development of effective exercise programs as well as methods for efficiently monitoring the benefits. A more in-depth and clearer understanding of the mechanisms underlying the effects of PA on brain health is therefore needed. The advancement of this research field is eagerly expected.
Author Contributions
HU designed the review and wrote the draft. TS and HA contributed the content and edited the draft. All authors contributed to the article and approved the submitted version.
Funding
This work was financially supported by the Japan Agency for Medical Research and Development (AMED) under Grant Numbers JP20de0107002 and 17dk0207040h0001. The sponsors had no role in the design and conduct of the study.
Conflict of Interest
The authors declare that the research was conducted in the absence of any commercial or financial relationships that could be construed as a potential conflict of interest.
Publisher’s Note
All claims expressed in this article are solely those of the authors and do not necessarily represent those of their affiliated organizations, or those of the publisher, the editors and the reviewers. Any product that may be evaluated in this article, or claim that may be made by its manufacturer, is not guaranteed or endorsed by the publisher.
References
Aarsland, D., Sardahaee, F. S., Anderssen, S., and Ballard, C., Alzheimer’s Society Systematic Review group (2010). Is physical activity a potential preventive factor for vascular dementia? A systematic review. Aging Ment. Health 14, 386–395. doi: 10.1080/13607860903586136
Abd El-Kader, S. M., and Al-Jiffri, O. H. (2016). Aerobic exercise improves quality of life, psychological well-being and systemic inflammation in subjects with Alzheimer’s disease. Afr. Health Sci. 16, 1045–1055. doi: 10.4314/ahs.v16i4.22
Adlard, P. A., Perreau, V. M., Pop, V., and Cotman, C. W. (2005). Voluntary exercise decreases amyloid load in a transgenic model of Alzheimer’s disease. J. Neurosci. 25, 4217–4221. doi: 10.1523/JNEUROSCI.0496-05.2005
Alatorre-Cruz, G. C., Sanchez-Lopez, J., Silva-Pereyra, J., and Fernández, T. (2020). Effects of incidental physical activity on morphosyntactic processing in aging. PLoS One 15:e0239727. doi: 10.1371/journal.pone.0239727
Alber, J., Alladi, S., Bae, H. J., Barton, D. A., Beckett, L. A., Bell, J. M., et al. (2019). White matter hyperintensities in vascular contributions to cognitive impairment and dementia (VCID): knowledge gaps and opportunities. Alzheimers Dement. (N. Y.) 5, 107–117. doi: 10.1016/j.trci.2019.02.001
Alghadir, A. H., Gabr, S. A., and Al-Eisa, E. S. (2016). Effects of moderate aerobic exercise on cognitive abilities and redox state biomarkers in older adults. Oxid. Med. Cell. Longev. 2016:2545168. doi: 10.1155/2016/2545168
Babcock, K. R., Page, J. S., Fallon, J. R., and Webb, A. E. (2021). Adult hippocampal neurogenesis in aging and Alzheimer’s disease. Stem Cell Rep. 16, 681–693. doi: 10.1016/j.stemcr.2021.01.019
Baker, L. D., Frank, L. L., Foster-Schubert, K., Green, P. S., Wilkinson, C. W., McTiernan, A., et al. (2010). Effects of aerobic exercise on mild cognitive impairment: a controlled trial. Arch. Neurol. 67, 71–79.
Barnes, D. E., and Yaffe, K. (2011). The projected effect of risk factor reduction on Alzheimer’s disease prevalence. Lancet Neurol. 10, 819–828. doi: 10.1016/s1474-4422(11)70072-2
Best, J. R., Chiu, B. K., Liang Hsu, C., Nagamatsu, L. S., and Liu-Ambrose, T. (2015). Long-term effects of resistance exercise training on cognition and brain volume in older women: results from a randomized controlled trial. J. Int. Neuropsychol. Soc. 21, 745–756. doi: 10.1017/s1355617715000673
Bettio, L., Thacker, J. S., Hutton, C., and Christie, B. R. (2019). Modulation of synaptic plasticity by exercise. Int. Rev. Neurobiol. 147, 295–322. doi: 10.1016/bs.irn.2019.07.002
Bray, N. W., Pieruccini-Faria, F., Bartha, R., Doherty, T. J., Nagamatsu, L. S., and Montero-Odasso, M. (2021). The effect of physical exercise on functional brain network connectivity in older adults with and without cognitive impairment. A systematic review. Mech. Ageing Dev. 196:111493. doi: 10.1016/j.mad.2021.111493
Brown, B. M., Peiffer, J., and Rainey-Smith, S. R. (2019). Exploring the relationship between physical activity, beta-amyloid and tau: a narrative review. Ageing Res. Rev. 50, 9–18. doi: 10.1016/j.arr.2019.01.003
Brown, B. M., Peiffer, J. J., Taddei, K., Lui, J. K., Laws, S. M., Gupta, V. B., et al. (2013). Physical activity and amyloid-β plasma and brain levels: results from the Australian imaging, biomarkers and lifestyle study of ageing. Mol. Psychiatry 18, 875–881. doi: 10.1038/mp.2012.107
Brown, B. M., Rainey-Smith, S. R., Dore, V., Peiffer, J. J., Burnham, S. C., Laws, S. M., et al. (2018). Self-reported physical activity is associated with tau burden measured by positron emission tomography. J. Alzheimers Dis. 63, 1299–1305. doi: 10.3233/JAD-170998
Brown, B. M., Sohrabi, H. R., Taddei, K., Gardener, S. L., Rainey-Smith, S. R., Peiffer, J. J., et al. (2017). Habitual exercise levels are associated with cerebral amyloid load in presymptomatic autosomal dominant Alzheimer’s disease. Alzheimers Dement. 13, 1197–1206. doi: 10.1016/j.jalz.2017.03.008
Buchman, A. S., Boyle, P. A., Yu, L., Shah, R. C., Wilson, R. S., and Bennett, D. A. (2012). Total daily physical activity and the risk of AD and cognitive decline in older adults. Neurology 78, 1323–1329. doi: 10.1212/wnl.0b013e3182535d35
Buchman, A. S., Dawe, R. J., Yu, L., Lim, A., Wilson, R. S., Schneider, J. A., et al. (2018). Brain pathology is related to total daily physical activity in older adults. Neurology 90, e1911–e1919.
Buchman, A. S., Yu, L., Wilson, R. S., Lim, A., Dawe, R. J., Gaiteri, C., et al. (2019). Physical activity, common brain pathologies, and cognition in community-dwelling older adults. Neurology 92, e811–e822.
Burzynska, A. Z., Jiao, Y., Knecht, A. M., Fanning, J., Awick, E. A., Chen, T., et al. (2017). White matter integrity declined over 6-months, but dance intervention improved integrity of the fornix of older adults. Front. Aging Neurosci. 9:59. doi: 10.3389/fnagi.2017.00059
Castells-Sánchez, A., Roig-Coll, F., Dacosta-Aguayo, R., Lamonja-Vicente, N., Sawicka, A. K., Torán-Monserrat, P., et al. (2021). Exercise and fitness neuroprotective effects: molecular, brain volume and psychological correlates and their mediating role in healthy late-middle-aged women and men. Front. Aging Neurosci. 13:615247. doi: 10.3389/fnagi.2021.615247
Chang, Y. K., Pesce, C., Chiang, Y. T., Kuo, C. Y., and Fong, D. Y. (2015). Antecedent acute cycling exercise affects attention control: an ERP study using attention network test. Front. Hum. Neurosci. 9:156. doi: 10.3389/fnhum.2015.00156
Clark, C. M., Guadagni, V., Mazerolle, E. L., Hill, M., Hogan, D. B., Pike, G. B., et al. (2019). Effect of aerobic exercise on white matter microstructure in the aging brain. Behav. Brain Res. 373:112042. doi: 10.1016/j.bbr.2019.112042
Colbert, L. H., Visser, M., Simonsick, E. M., Tracy, R. P., Newman, A. B., Kritchevsky, S. B., et al. (2004). Physical activity, exercise, and inflammatory markers in older adults: findings from the health, aging and body composition study. J. Am. Geriatr. Soc. 52, 1098–1104. doi: 10.1111/j.1532-5415.2004.52307.x
Colcombe, S. J., Erickson, K. I., Scalf, P. E., Kim, J. S., Prakash, R., McAuley, E., et al. (2006). Aerobic exercise training increases brain volume in aging humans. J. Gerontol. A Biol. Sci. Med. Sci. 61, 1166–1170. doi: 10.1093/gerona/61.11.1166
Colmenares, A. M., Voss, M. W., Fanning, J., Salerno, E. A., Gothe, N. P., Thomas, M. L., et al. (2021). White matter plasticity in healthy older adults: the effects of aerobic exercise. Neuroimage 239:118305. doi: 10.1016/j.neuroimage.2021.118305
Cuyul-Vásquez, I., Berríos-Contreras, L., Soto-Fuentes, S., Hunter-Echeverría, K., and Marzuca-Nassr, G. N. (2020). Effects of resistance exercise training on redox homeostasis in older adults. A systematic review and meta-analysis. Exp. Gerontol. 138:111012. doi: 10.1016/j.exger.2020.111012
de Sousa, C. V., Sales, M. M., Rosa, T. S., Lewis, J. E., de Andrade, R. V., and Simões, H. G. (2017). The antioxidant effect of exercise: a systematic review and meta-analysis. Sports Med. 47, 277–293.
de Souto Barreto, P., Andrieu, S., Payoux, P., Demougeot, L., Rolland, Y., Vellas, B., et al. (2015). Physical activity and amyloid-β brain levels in elderly adults with intact cognition and mild cognitive impairment. J. Am. Geriatr. Soc. 63, 1634–1639. doi: 10.1111/jgs.13530
Erickson, K. I., Raji, C. A., Lopez, O. L., Becker, J. T., Rosano, C., Newman, A. B., et al. (2010). Physical activity predicts gray matter volume in late adulthood: the cardiovascular health study. Neurology 75, 1415–1422. doi: 10.1212/WNL.0b013e3181f88359
Fedewa, M. V., Hathaway, E. D., and Ward-Ritacco, C. L. (2017). Effect of exercise training on C reactive protein: a systematic review and meta-analysis of randomised and non-randomised controlled trials. Br. J. Sports Med. 51, 670–676. doi: 10.1136/bjsports-2016-095999
Fiorenzato, E., Zabberoni, S., Costa, A., and Cona, G. (2021). Cognitive and mental health changes and their vulnerability factors related to COVID-19 lockdown in Italy. PLoS One 16:e0246204. doi: 10.1371/journal.pone.0246204
Firth, J., Stubbs, B., Vancampfort, D., Schuch, F., Lagopoulos, J., Rosenbaum, S., et al. (2018). Effect of aerobic exercise on hippocampal volume in humans: a systematic review and meta-analysis. Neuroimage 166, 230–238. doi: 10.1016/j.neuroimage.2017.11.007
Flöel, A., Ruscheweyh, R., Krüger, K., Willemer, C., Winter, B., Völker, K., et al. (2010). Physical activity and memory functions: are neurotrophins and cerebral gray matter volume the missing link? Neuroimage 49, 2756–2763. doi: 10.1016/j.neuroimage.2009.10.043
Franchetti, M. K., Bharawaj, P. K., Nguyen, L. A., Van Etten, E. J., Klimentidis, Y. C., Hishaw, G. A., et al. (2020). Interaction of age and self-reported physical sports activity on white matter hyperintensity volume in healthy older adults. Front. Aging Neurosci. 12:576025. doi: 10.3389/fnagi.2020.576025
Frederiksen, K. S., Gjerum, L., Waldemar, G., and Hasselbalch, S. G. (2019). Physical activity as a moderator of alzheimer pathology: a systematic review of observational studies. Curr. Alzheimer. Res. 16, 362–378. doi: 10.2174/1567205016666190315095151
Friedl-Werner, A., Brauns, K., Gunga, H. C., Kühn, S., and Stahn, A. C. (2020). Exercise-induced changes in brain activity during memory encoding and retrieval after long-term bed rest. Neuroimage 223:117359. doi: 10.1016/j.neuroimage.2020.117359
Gajewski, P. D., and Falkenstein, M. (2018). ERP and behavioral effects of physical and cognitive training on working memory in aging: a randomized controlled study. Neural. Plast. 2018:3454835.
Gogniat, M. A., Robinson, T. L., and Miller, L. S. (2021). Exercise interventions do not impact brain volume change in older adults: a systematic review and meta-analysis. Neurobiol. Aging 101, 230–246. doi: 10.1016/j.neurobiolaging.2021.01.025
Groot, C., Hooghiemstra, A. M., Raijmakers, P. G., van Berckel, B. N., Scheltens, P., Scherder, E. J., et al. (2016). The effect of physical activity on cognitive function in patients with dementia: a meta-analysis of randomized control trials. Ageing Res. Rev. 25, 13–23. doi: 10.1016/j.arr.2015.11.005
Hamer, M., and Chida, Y. (2009). Physical activity and risk of neurodegenerative disease: a systematic review of prospective evidence. Psychol. Med. 39, 3–11. doi: 10.1017/s0033291708003681
Head, D., Bugg, J. M., Goate, A. M., Fagan, A. M., Mintun, M. A., Benzinger, T., et al. (2012). Exercise engagement as a moderator of the effects of APOE genotype on amyloid deposition. Arch. Neurol. 69, 636–643. doi: 10.1001/archneurol.2011.845
Heyn, P., Abreu, B. C., and Ottenbacher, K. J. (2004). The effects of exercise training on elderly persons with cognitive impairment and dementia: a meta-analysis. Arch. Phys. Med. Rehabil. 85, 1694–1704. doi: 10.1016/j.apmr.2004.03.019
Huang, X., Zhao, X., Li, B., Cai, Y., Zhang, S., Yu, F., et al. (2021). Biomarkers for evaluating the effects of exercise interventions in patients with MCI or dementia: a systematic review and meta-analysis. Exp. Gerontol. 151:111424. doi: 10.1016/j.exger.2021.111424
Ismail, I. I., Kamel, W. A., and Al-Hashel, J. Y. (2021). Association of COVID-19 pandemic and rate of cognitive decline in patients with dementia and mild cognitive impairment: a cross-sectional study. Gerontol. Geriatr. Med. 7:23337214211005223. doi: 10.1177/23337214211005223
Jensen, C. S., Bahl, J. M., Østergaard, L. B., Høgh, P., Wermuth, L., Heslegrave, A., et al. (2019). Exercise as a potential modulator of inflammation in patients with Alzheimer’s disease measured in cerebrospinal fluid and plasma. Exp. Gerontol. 121, 91–98. doi: 10.1016/j.exger.2019.04.003
Jeon, S. Y., Byun, M. S., Yi, D., Lee, J. H., Ko, K., Sohn, B. K., et al. (2020). Midlife lifestyle activities moderate APOE ε4 effect on in vivo Alzheimer’s disease pathologies. Front. Aging Neurosci. 12:42. doi: 10.3389/fnagi.2020.00042
Ji, L., Pearlson, G. D., Zhang, X., Steffens, D. C., Ji, X., Guo, H., et al. (2018). Physical exercise increases involvement of motor networks as a compensatory mechanism during a cognitively challenging task. Int. J. Geriatr. Psychiatry 33, 1153–1159. doi: 10.1002/gps.4909
Jiang, Q., Lou, K., Hou, L., Lu, Y., Sun, L., Tan, S. C., et al. (2020). The effect of resistance training on serum insulin-like growth factor 1(IGF-1): a systematic review and meta-analysis. Complement. Ther. Med. 50:102360. doi: 10.1016/j.ctim.2020.102360
Kang, D. W., Bressel, E., and Kim, D. Y. (2020). Effects of aquatic exercise on insulin-like growth factor-1, brain-derived neurotrophic factor, vascular endothelial growth factor, and cognitive function in elderly women. Exp. Gerontol. 132:110842. doi: 10.1016/j.exger.2020.110842
Knaepen, K., Goekint, M., Heyman, E. M., and Meeusen, R. (2010). Neuroplasticity – exercise-induced response of peripheral brain-derived neurotrophic factor: a systematic review of experimental studies in human subjects. Sports Med. 40, 765–801. doi: 10.2165/11534530-000000000-00000
Knight, S. P., Laird, E., Williamson, W., O’Connor, J., Newman, L., Carey, D., et al. (2021). Obesity is associated with reduced cerebral blood flow – modified by physical activity. Neurobiol. Aging 105, 35–47. doi: 10.1016/j.neurobiolaging.2021.04.008
Law, L. L., Rol, R. N., Schultz, S. A., Dougherty, R. J., Edwards, D. F., Koscik, R. L., et al. (2018). Moderate intensity physical activity associates with CSF biomarkers in a cohort at risk for Alzheimer’s disease. Alzheimers Dement. (Amst.) 10, 188–195. doi: 10.1016/j.dadm.2018.01.001
Leal, G., Afonso, P. M., Salazar, I. L., and Duarte, C. B. (2015). Regulation of hippocampal synaptic plasticity by BDNF. Brain Res. 1621, 82–101. doi: 10.1016/j.brainres.2014.10.019
Leal-Galicia, P., Romo-Parra, H., Rodríguez-Serrano, L. M., and Buenrostro-Jáuregui, M. (2019). Regulation of adult hippocampal neurogenesis exerted by sexual, cognitive and physical activity: an update. J. Chem. Neuroanat. 101:101667. doi: 10.1016/j.jchemneu.2019.101667
Lee, J. S., Shin, H. Y., Kim, H. J., Jang, Y. K., Jung, N. Y., Lee, J., et al. (2016). Combined effects of physical exercise and education on age-related cortical thinning in cognitively normal individuals. Sci. Rep. 6:24284. doi: 10.1038/srep24284
Li, L., Wang, J., Guo, S., Xing, Y., Ke, X., Chen, Y., et al. (2021). Tai Chi exercise improves age-associated decline in cerebrovascular function: a cross-sectional study. BMC Geriatr. 21:293. doi: 10.1186/s12877-021-02196-9
Li, M. Y., Huang, M. M., Li, S. Z., Tao, J., Zheng, G. H., and Chen, L. D. (2017). The effects of aerobic exercise on the structure and function of DMN-related brain regions: a systematic review. Int. J. Neurosci. 127, 634–649. doi: 10.1080/00207454.2016.1212855
Liang, K. Y., Mintun, M. A., Fagan, A. M., Goate, A. M., Bugg, J. M., Holtzman, D. M., et al. (2010). Exercise and Alzheimer’s disease biomarkers in cognitively normal older adults. Ann. Neurol. 68, 311–318. doi: 10.1002/ana.22096
Maasakkers, C. M., Thijssen, D. H., Knight, S. P., Newman, L., O’Connor, J. D., Scarlett, S., et al. (2021). Hemodynamic and structural brain measures in high and low sedentary older adults. J. Cereb. Blood Flow Metab. 41, 2607–2616. doi: 10.1177/0271678X211009382
Marchal, G., Rioux, P., Petit-Taboué, M. C., Sette, G., Travère, J. M., Le Poec, C., et al. (1992). Regional cerebral oxygen consumption, blood flow, and blood volume in healthy human aging. Arch. Neurol. 49, 1013–1020. doi: 10.1001/archneur.1992.00530340029014
Mee-Inta, O., Zhao, Z. W., and Kuo, Y. M. (2019). Physical exercise inhibits inflammation and microglial activation. Cells 8:691. doi: 10.3390/cells8070691
Miyamoto, N., Pham, L. D., Seo, J. H., Kim, K. W., Lo, E. H., and Arai, K. (2014). Crosstalk between cerebral endothelium and oligodendrocyte. Cell. Mol. Life Sci. 71, 1055–1066. doi: 10.1007/s00018-013-1488-9
Moniruzzaman, M., Kadota, A., Akash, M. S., Pruitt, P. J., Miura, K., Albin, R., et al. (2020). Effects of physical activities on dementia-related biomarkers: a systematic review of randomized controlled trials. Alzheimers Dement. (N. Y.) 6:e12109. doi: 10.1002/trc2.12109
Moon, S. Y., de Souto Barreto, P., Cesari, M., Chupin, M., Mangin, J. F., Bouyahia, A., et al. (2018). Physical activity and changes in white matter hyperintensities over three years. J. Nutr. Health Aging 22, 425–430. doi: 10.1007/s12603-017-0959-3
Müller, P., Rehfeld, K., Schmicker, M., Hökelmann, A., Dordevic, M., Lessmann, V., et al. (2017). Evolution of neuroplasticity in response to physical activity in old age: the case for dancing. Front. Aging Neurosci 9:56. doi: 10.3389/fnagi.2017.00056
Muscari, A., Giannoni, C., Pierpaoli, L., Berzigotti, A., Maietta, P., Foschi, E., et al. (2010). Chronic endurance exercise training prevents aging-related cognitive decline in healthy older adults: a randomized controlled trial. Int. J. Geriatr. Psychiatry 25, 1055–1064. doi: 10.1002/gps.2462
Nascimento, C. M., Pereira, J. R., de Andrade, L. P., Garuffi, M., Talib, L. L., Forlenza, O. V., et al. (2014). Physical exercise in MCI elderly promotes reduction of pro-inflammatory cytokines and improvements on cognition and BDNF peripheral levels. Curr. Alzheimer Res. 11, 799–805. doi: 10.2174/156720501108140910122849
Nishiguchi, S., Yamada, M., Tanigawa, T., Sekiyama, K., Kawagoe, T., Suzuki, M., et al. (2015). A 12-week physical and cognitive exercise program can improve cognitive function and neural efficiency in community-dwelling older adults: a randomized controlled trial. J. Am. Geriatr. Soc. 63, 1355–1363. doi: 10.1111/jgs.13481
Onyango, I. G., Jauregui, G. V., Čarná, M., Bennett, J. P. Jr., and Stokin, G. B. (2021). Neuroinflammation in Alzheimer’s disease. Biomedicines 9:524.
Palta, P., Heiss, G., Sharrett, A. R., Gabriel, K. P., Walker, K., Evenson, K. R., et al. (2020). Mid- and late-life leisure-time physical activity and global brain amyloid burden: the Atherosclerosis Risk in Communities (ARIC)-PET Study. J. Alzheimers Dis. 76, 139–147. doi: 10.3233/JAD-200152
Phillips, C., Bakir, M. A., Srivatsan, M., and Salehi, A. (2014). Neuroprotective effects of physical activity on the brain: a closer look at trophic factor signaling. Front. Cell Neurosci. 8:170. doi: 10.3389/fncel.2014.00170
Radák, Z., Kaneko, T., Tahara, S., Nakamoto, H., Pucsok, J., Sasvári, M., et al. (2001). Regular exercise improves cognitive function and decreases oxidative damage in rat brain. Neurochem. Int. 38, 17–23. doi: 10.1016/s0197-0186(00)00063-2
Radak, Z., Suzuki, K., Higuchi, M., Balogh, L., Boldogh, I., and Koltai, E. (2016). Physical exercise, reactive oxygen species and neuroprotection. Free Radic. Biol. Med. 98, 187–196. doi: 10.1016/j.freeradbiomed.2016.01.024
Radak, Z., Toldy, A., Szabo, Z., Siamilis, S., Nyakas, C., Silye, G., et al. (2006). The effects of training and detraining on memory, neurotrophins and oxidative stress markers in rat brain. Neurochem. Int. 49, 387–392. doi: 10.1016/j.neuint.2006.02.004
Raffin, J., Rolland, Y., Aggarwal, G., Nguyen, A. D., Morley, J. E., Li, Y., et al. (2021). Associations between physical activity, blood-based biomarkers of neurodegeneration, and cognition in healthy older adults: the MAPT study. J. Gerontol. A Biol. Sci. Med. Sci. 76, 1382–1390.
Rasmussen, P., Brassard, P., Adser, H., Pedersen, M. V., Leick, L., Hart, E., et al. (2009). Evidence for a release of brain-derived neurotrophic factor from the brain during exercise. Exp. Physiol. 94, 1062–1069. doi: 10.1113/expphysiol.2009.048512
Rehfeld, K., Lüders, A., Hökelmann, A., Lessmann, V., Kaufmann, J., Brigadski, T., et al. (2018). Dance training is superior to repetitive physical exercise in inducing brain plasticity in the elderly. PLoS One 13:e0196636. doi: 10.1371/journal.pone.0196636
Reuben, D. B., Judd-Hamilton, L., Harris, T. B., Seeman, T. E., and MacArthur Studies of Successful Aging (2003). The associations between physical activity and inflammatory markers in high-functioning older persons: MacArthur Studies of Successful Aging. J. Am. Geriatr. Soc. 51, 1125–1130. doi: 10.1046/j.1532-5415.2003.51380.x
Rogers, R. L., Meyer, J. S., and Mortel, K. F. (1990). After reaching retirement age physical activity sustains cerebral perfusion and cognition. J. Am. Geriatr. Soc. 38, 123–128. doi: 10.1111/j.1532-5415.1990.tb03472.x
Ruiz-González, D., Hernández-Martínez, A., Valenzuela, P. L., Morales, J. S., and Soriano-Maldonado, A. (2021). Effects of physical exercise on plasma brain-derived neurotrophic factor in neurodegenerative disorders: a systematic review and meta-analysis of randomized controlled trials. Neurosci. Biobehav. Rev. 128, 394–405. doi: 10.1016/j.neubiorev.2021.05.025
Sampaio-Baptista, C., and Johansen-Berg, H. (2017). White matter plasticity in the adult brain. Neuron 96, 1239–1251. doi: 10.1016/j.neuron.2017.11.026
Santhanam, A. V., Smith, L. A., and Katusic, Z. S. (2010). Brain-derived neurotrophic factor stimulates production of prostacyclin in cerebral arteries. Stroke 41, 350–356. doi: 10.1161/STROKEAHA.109.564492
Scahill, R. I., Frost, C., Jenkins, R., Whitwell, J. L., Rossor, M. N., and Fox, N. C. (2003). A longitudinal study of brain volume changes in normal aging using serial registered magnetic resonance imaging. Arch. Neurol. 60, 989–994. doi: 10.1001/archneur.60.7.989
Seifert, T., Brassard, P., Wissenberg, M., Rasmussen, P., Nordby, P., Stallknecht, B., et al. (2010). Endurance training enhances BDNF release from the human brain. Am. J. Physiol. Regul. Integr. Comp. Physiol. 298, R372–R377.
Shimada, H., Makizako, H., Doi, T., Yoshida, D., Tsutsumimoto, K., Anan, Y., et al. (2014). A large, cross-sectional observational study of serum BDNF, cognitive function, and mild cognitive impairment in the elderly. Front. Aging Neurosci. 6:69. doi: 10.3389/fnagi.2014.00069
Smith, A. E., Dumuid, D., Goldsworthy, M. R., Graetz, L., Hodyl, N., Thornton, N. L. R., et al. (2021). Daily activities are associated with non-invasive measures of neuroplasticity in older adults. Clin. Neurophysiol. 132, 984–992. doi: 10.1016/j.clinph.2021.01.016
Sofi, F., Valecchi, D., Bacci, D., Abbate, R., Gensini, G. F., Casini, A., et al. (2011). Physical activity and risk of cognitive decline: a meta-analysis of prospective studies. J. Intern. Med. 269, 107–117. doi: 10.1111/j.1365-2796.2010.02281.x
Sonntag, W. E., Ramsey, M., and Carter, C. S. (2005). Growth hormone and insulin-like growth factor-1 (IGF-1) and their influence on cognitive aging. Ageing Res. Rev. 4, 195–212. doi: 10.1016/j.arr.2005.02.001
Stein, A. M., da Silva, T. M. V., Coelho, F. G. M., Rueda, A. V., Camarini, R., and Galduróz, R. F. S. (2021). Acute exercise increases circulating IGF-1 in Alzheimer’s disease patients, but not in older adults without dementia. Behav. Brain Res. 396:112903. doi: 10.1016/j.bbr.2020.112903
Stillman, C. M., Lopez, O. L., Becker, J. T., Kuller, L. H., Mehta, P. D., Tracy, R. P., et al. (2017). Physical activity predicts reduced plasma β amyloid in the cardiovascular health study. Ann. Clin. Transl. Neurol. 4, 284–291. doi: 10.1002/acn3.397
Stojanovic, M., Jin, Y., Fagan, A. M., Benzinger, T. L., Hassenstab, J., Cruchaga, C., et al. (2020). Physical exercise and longitudinal trajectories in alzheimer disease biomarkers and cognitive functioning. Alzheimer. Dis. Assoc. Disord. 34, 212–219. doi: 10.1097/wad.0000000000000385
Swain, R. A., Harris, A. B., Wiener, E. C., Dutka, M. V., Morris, H. D., Theien, B. E., et al. (2003). Prolonged exercise induces angiogenesis and increases cerebral blood volume in primary motor cortex of the rat. Neuroscience 117, 1037–1046. doi: 10.1016/s0306-4522(02)00664-4
Tao, J., Liu, J., Liu, W., Huang, J., Xue, X., Chen, X., et al. (2017). Tai Chi Chuan and Baduanjin increase grey matter volume in older adults: a brain imaging study. J. Alzheimers Dis. 60, 389–400. doi: 10.3233/JAD-170477
Tarumi, T., and Zhang, R. (2018). Cerebral blood flow in normal aging adults: cardiovascular determinants, clinical implications, and aerobic fitness. J. Neurochem. 144, 595–608. doi: 10.1111/jnc.14234
Thomas, B. P., Yezhuvath, U. S., Tseng, B. Y., Liu, P., Levine, B. D., Zhang, R., et al. (2013). Life-long aerobic exercise preserved baseline cerebral blood flow but reduced vascular reactivity to CO2. J. Magn. Reson. Imaging 38, 1177–1183. doi: 10.1002/jmri.24090
Tomoto, T., Liu, J., Tseng, B. Y., Pasha, E. P., Cardim, D., Tarumi, T., et al. (2021). One-year aerobic exercise reduced carotid arterial stiffness and increased cerebral blood flow in amnestic mild cognitive impairment. J. Alzheimers Dis. 80, 841–853. doi: 10.3233/JAD-201456
Tondo, G., Sarasso, B., Serra, P., Tesser, F., and Comi, C. (2021). The impact of the COVID-19 pandemic on the cognition of people with dementia. Int. J. Environ. Res. Public Health 18:4285. doi: 10.3390/ijerph18084285
Torres, E. R., Strack, E. F., Fernandez, C. E., Tumey, T. A., and Hitchcock, M. E. (2015). Physical activity and white matter hyperintensities: a systematic review of quantitative studies. Prev. Med. Rep. 2, 319–325. doi: 10.1016/j.pmedr.2015.04.013
Treyer, V., Meyer, R. S., Buchmann, A., Crameri, G. A. G., Studer, S., Saake, A., et al. (2021). Physical activity is associated with lower cerebral beta-amyloid and cognitive function benefits from lifetime experience-a study in exceptional aging. PLoS One 16:e0247225. doi: 10.1371/journal.pone.0247225
Vecchio, L. M., Meng, Y., Xhima, K., Lipsman, N., Hamani, C., and Aubert, I. (2018). The neuroprotective effects of exercise: maintaining a healthy brain throughout aging. Brain Plast. 4, 17–52. doi: 10.3233/BPL-180069
Venkatraman, V. K., Sanderson, A., Cox, K. L., Ellis, K. A., Steward, C., Phal, P. M., et al. (2020). Effect of a 24-month physical activity program on brain changes in older adults at risk of Alzheimer’s disease: the AIBL active trial. Neurobiol. Aging 89, 132–141. doi: 10.1016/j.neurobiolaging.2019.02.030
Vital, T. M., Stein, A. M., de Melo Coelho, F. G., Arantes, F. J., Teodorov, E., and Santos-Galduróz, R. F. (2014). Physical exercise and vascular endothelial growth factor (VEGF) in elderly: a systematic review. Arch. Gerontol. Geriatr. 59, 234–239. doi: 10.1016/j.archger.2014.04.011
Voss, M. W., Heo, S., Prakash, R. S., Erickson, K. I., Alves, H., Chaddock, L., et al. (2013). The influence of aerobic fitness on cerebral white matter integrity and cognitive function in older adults: results of a one-year exercise intervention. Hum. Brain Mapp. 34, 2972–2985. doi: 10.1002/hbm.22119
Walhovd, K. B., Storsve, A. B., Westlye, L. T., Drevon, C. A., and Fjell, A. M. (2014). Blood markers of fatty acids and vitamin D, cardiovascular measures, body mass index, and physical activity relate to longitudinal cortical thinning in normal aging. Neurobiol. Aging 35, 1055–1064. doi: 10.1016/j.neurobiolaging.2013.11.011
Wendel-Vos, G. C., Schuit, A. J., Feskens, E. J., Boshuizen, H. C., Verschuren, W. M., Saris, W. H., et al. (2004). Physical activity and stroke. A meta-analysis of observational data. Int. J. Epidemiol. 33, 787–798. doi: 10.1093/ije/dyh168
Wolf, D., Fischer, F. U., Riedel, D., Knaepen, K., Kollmann, B., Kocabayoglu, M., et al. (2020). The Impact of age on the association between physical activity and white matter integrity in cognitively healthy older adults. Front. Aging Neurosci. 12:579470. doi: 10.3389/fnagi.2020.579470
Yan, H., Mitschelen, M., Bixler, G. V., Brucklacher, R. M., Farley, J. A., Han, S., et al. (2011). Circulating IGF1 regulates hippocampal IGF1 levels and brain gene expression during adolescence. J. Endocrinol. 211, 27–37. doi: 10.1530/JOE-11-0200
Znazen, H., Slimani, M., Bragazzi, N. L., and Tod, D. (2021). The relationship between cognitive function, lifestyle behaviours and perception of stress during the COVID-19 induced confinement: insights from correlational and mediation analyses. Int. J. Environ. Res. Public Health 18:3194. doi: 10.3390/ijerph18063194
Keywords: Alzheimer’s disease, brain-derived neurotrophic factor, exercise, dementia, neurodegeneration, physical activity, white matter
Citation: Umegaki H, Sakurai T and Arai H (2021) Active Life for Brain Health: A Narrative Review of the Mechanism Underlying the Protective Effects of Physical Activity on the Brain. Front. Aging Neurosci. 13:761674. doi: 10.3389/fnagi.2021.761674
Received: 20 August 2021; Accepted: 08 October 2021;
Published: 30 November 2021.
Edited by:
Ignacio Torres-Aleman, Achucarro Basque Center for Neuroscience, SpainReviewed by:
Vanessa Castelli, University of L’Aquila, ItalyHailong Song, University of Pennsylvania, United States
Copyright © 2021 Umegaki, Sakurai and Arai. This is an open-access article distributed under the terms of the Creative Commons Attribution License (CC BY). The use, distribution or reproduction in other forums is permitted, provided the original author(s) and the copyright owner(s) are credited and that the original publication in this journal is cited, in accordance with accepted academic practice. No use, distribution or reproduction is permitted which does not comply with these terms.
*Correspondence: Hiroyuki Umegaki, dW1lZ2FraUBtZWQubmFnb3lhLXUuYWMuanA=