- 1Department of Environmental Science and Policy, George Mason University, Fairfax, VA, United States
- 2Smithsonian’s National Zoo and Conservation Biology Institute, Front Royal, VA, United States
- 3Sistema Nacional de Investigación, Secretaría Nacional de Ciencia, Tecnología e Innovación (SENACYT), Panama City, Panama
- 4Department of Biological Sciences, Virginia Tech, Blacksburg, VA, United States
- 5Zoo New England, Boston, MA, United States
- 6Smithsonian Tropical Research Institute, Panama City, Panama
- 7Cheyenne Mountain Zoo, Colorado Springs, CO, United States
The endangered Limosa harlequin frog Atelopus limosus has experienced significant chytridiomycosis-related declines, but has been successfully bred in captivity as part of the Panama Amphibian Rescue and Conservation Project. We conducted the first Atelopus release trial using 83 captive-bred A. limosus, and monitored individuals as they transitioned from captivity back into the wild at a site within their historic distribution with no extant population. We acclimated 23 animals to the environment prior to release by holding them in mesocosms for 30 days (soft release) and released a further 60 animals without prior acclimation (hard release). We radio-tracked a subsample of animals in each treatment group. We used a Bayesian mark–recapture analysis to integrate known fate data from radio-tracked individuals with data from resighted individuals that had unknown fates. For this analysis, we allowed survival to differ between release treatments (hard vs. soft) and assumed detection probability would differ between tracking methods. Per-survey detection probability was about 0.02 for individuals without radio transmitters vs. 0.88 for radio-tracked frogs. We observed that hard-released animals initially dispersed further than soft-released animals, and, despite the fact that 25% of the soft-released animals were Batrachochytrium dendrobatidis (Bd)-positive on release day, their estimated 30-day survival probability was 0.46 (vs. 0.31 for the hard-released animals). The insights from this release trial can be used in an adaptive management framework to improve and refine release methods that will inform the nascent field of amphibian reintroduction ecology.
Introduction
The pathogenic amphibian chytrid fungi of the genus Batrachochytrium cause chytridiomycosis in amphibians; they are associated with the disappearance of 90 species and a >90% decline in the populations of 124 amphibian species (Scheele et al., 2019). In Latin America, Harlequin frogs (Atelopus spp.) have undergone dramatic population declines due to chytridiomycosis (La Marca et al., 2005; Scheele et al., 2019). Seven species of Atelopus from Panama are known: Atelopus chiriquiensis, A. certus, A. fronterizo, A. glyphus, A. limosus, A. varius, and A. zeteki (Lewis et al., 2019; Veseley and Batista, 2021). The Chiriquí Harlequin frog (A. chiriquiensis) has not been observed since 1996 (Lips, 1999) and the Panamanian golden frog (A. zeteki), the national symbol of Panama, has not been seen in the wild since 2009 (Lewis et al., 2019). Captive populations of the critically endangered A. certus, A. glyphus, A. limosus, A. varius, and A. zeteki have been established and maintained by the Panama Amphibian Rescue and Conservation Project (PARC), which prioritized species at risk of extinction from chytridiomycosis, with a goal to reintroduce at-risk species back into the wild (Gratwicke et al., 2016; Lewis et al., 2019).
Amphibian species from captive breeding programs used in reintroduction efforts are likely to face ongoing threats in the wild (Griffiths and Pavajeau, 2008). For instance, while chytridiomycosis is treatable in captivity (Brannelly et al., 2012; Baitchman and Pessier, 2013), it remains a significant challenge to mitigate in nature (Bosch et al., 2015; Garner et al., 2016). Some populations of Atelopus persist due to a high recruitment of offspring, but remain in a precarious state (Lampo et al., 2017; González-Maya et al., 2018). There is evidence that A. varius populations in Panama are developing resistance to Batrachochytrium dendrobatidis (Bd) (Voyles et al., 2018; Byrne et al., 2021), and rediscoveries of Atelopus populations once thought to be extinct may offer further insight into the genetic selection pressures that chytridiomycosis places on these populations (Jaynes et al., 2022).
Because the primary chytridiomycosis threat remains unsolved, the long-term goal of establishing viable populations of Atelopus in Bd-positive sites may seem out of reach. However, using captive populations in release trials may help to answer critical questions in reintroduction biology and will likely yield new observations that improve the outcomes of reintroductions and translocations for conservation purposes (Armstrong and Seddon, 2008; Jachowski et al., 2016). Optimizing release protocols can be achieved through a process of adaptive management by observing the effects of variables that may be taxon specific, such as developmental stage, location, season, group size, and acclimation (Morrison, 2015). For example, soft-release conditioning of animals is a costly but commonly deployed release protocol that can establish site fidelity and reduce mortality in some taxa, such as mammals, reptiles, and fish, but may increase stress and reduce success in other taxa, such as birds (Tetzlaff et al., 2019). Our goal was to understand how pre-release conditions (soft vs. hard release) affect dispersal and survivorship of captive-bred Atelopus. This is the first release trial conducted of any captive-bred Atelopus species, and direct observations of the animals’ transition from captive conditions to the wild provide a valuable learning opportunity to understand the challenges during this critical time.
Methods
Study site
The Mamoní Valley Preserve is located in Central Panama and is managed as part of the larger Mamoní Valley Conservation area, which has 1,500 ha of old-growth forest and 3,500 ha of watersheds that are undergoing restoration efforts following degradation from cattle and agriculture (Geoversity, 2020). A. limosus were collected here as a source population for the captive assurance colony at the Panama Amphibian Rescue and Conservation Project following the first Bd detection in the adjacent Chagres National Park in 2009 (Lewis et al., 2019). We selected a first-order perennial stream tributary to the Mamoní River for its accessibility, deep pools separated by riffles, and waterfalls in a mature secondary rainforest that does not have extant Atelopus populations. This site was about 5 km SE from the Madroño River where a small wild population of A. limosus persists.
Release trial
The aim of this study was to evaluate the movement and survival of 60 frogs that were held in the breeding facility and released directly into the wild (hard release) compared with 23 frogs that had been held for 30 days prior to release in mesocosms established in the wild (soft release). We used animals aged approximately 1 year derived from two clutches from founders collected at the Madroño River. ARC-Alim-F1-11 were descended from a male founder collected in 2010 and a female collected in 2011. ARC-Alim-F1-13 were derived from a male collected in 2010 and a female reared in captivity in 2013. The overall sex ratio was approximately even (37 female : 46 male). Frogs were individually marked with visible alphanumeric tags in accordance with the manufacturer’s instructions [VI alpha; Visible Implant (VI) Tags standard size (1.2 mm × 2.7 mm), Northwest Marine Technology] (Heard et al., 2008; Pittman et al., 2008; Osbourn et al., 2011). We used a sterilized (alcohol-flamed) injector needle to insert the tag under the skin of each frog in the tibial region of the left hind leg rather than the dorsum, because it was less pigmented. We individually photographed the frogs’ dorsal and ventral sides as a backup photographic identification reference. This proved necessary as the sub-cutaneous tags were difficult to read upon recapture, and we do not recommend them for future Atelopus studies. Soft-released animals were held individually in outdoor mesocosms (76 cm × 76 cm × 46 cm polythene mesh with 6 mm gaps) for 1 month prior to release (Estrada et al., 2022). We established three consecutive 80-m stream transects and released 7–8 “soft-release” and 20 “hard-release” A. limosus on each transect, placing one randomly selected frog every 5 m on either side of the stream at the transition from the dry to the rainy season on 17 May 2017. We conducted recapture surveys for the A. limosus and swabbed the existing frog community for Bd to estimate prevalence by conducting nocturnal and diurnal searches of the stream transects on weeks 4 and 8.
Bd swabbing
Frogs were swabbed for Bd following a standard protocol using an MW113 swab five times on each foot, 10 times on the belly, and 10 times on each thigh for a total of 40 passes (Kriger et al., 2006; Hyatt et al., 2007). Swabs were placed into 1.5-mL microcentrifuge tubes and stored in a –20°C freezer until processing. Swabs were extracted using Applied Biosystems PrepMan™ Ultra (Waltham, USA) following the manufacturer’s protocol. For Bd detection, we used a real-time PCR assay following a standard protocol for Bd (Boyle et al., 2004) with some slight modifications. We used a Roche LightCycler® 96 System and 20 μL reactions consisting of 5 μL of sample and 15 μL of a master mix (Kriger et al., 2006; Hyatt et al., 2007; Estrada et al., 2022). We tested the samples in triplicate, along with five Bd-negative and one or two Bd-positive controls on every plate (Boyle et al., 2004; Kriger and Hero, 2007). Samples with two or three positive reactions were considered Bd positive. A few samples were repeated to confirm results. We quantified the infection load of Bd-positive samples based on the estimated number of zoospore equivalents using standards prepared from the JEL 423 Bd strain (Boyle et al., 2004; Estrada et al., 2022). We averaged the quantified load values and multiplied them by 100 to compensate for the extraction and dilution of the samples.
Radio tracking and recapture
We equipped 17 female A. limosus with radio transmitters prior to release (nine soft-release and eight hard-release frogs). We used some of the smallest commercially available 0.31 g LB-2X transmitters with a 21-day battery life (Holohil Systems Ltd.) and attached transmitters to the largest females, which weighed approximately 3 g or more, to approximate the 10% radio transmitter-to-body-mass ratio traditionally observed in herpetological studies (Heyer et al., 1994; Altobelli et al., 2022). Surgical silicone tubing was used as a waistband with a fine cotton thread to mount the radio transmitters on the amphibians (Figure 1) (Bartelt and Peterson, 2000; Rowley and Alford, 2007; Pašukonis et al., 2014). The organic cotton thread eventually degrades and breaks, acting as an auto-release mechanism (Rowley and Alford, 2007; Pašukonis et al., 2014). We used a Biotracker radio receiver (Lotek, Ontario, Canada) to track A. limosus equipped with radio transmitters after release once per day during weekdays (total of 31 sessions). When an individual was located, we recorded coordinates using a Garmin GPS in WGS84. Fluorescent flagging tape with the individual’s identification number was placed on the nearest vegetation as a starting point for the next radio-tracking period. Once a week we handled radio-tracked frogs to examine the condition of their skin where the silicone tubing contacted the frog, weighed and measured SVL, and swabbed them for Bd. The standard battery life of the transmitters is 21 days, with some variation, so we replaced radio transmitters every 18 days for a total radio-tracking duration of 54 days, after which the harness was removed and the frog released. If abrasion lesions were noted during the weekly swabbing and physical evaluation (n = 5 animals), the frog was removed from the study, the harness was removed, and the frog was placed in a plastic mouse cage and fed with wingless fruit flies. Abrasions were externally treated daily with silver sulfadiazine cream for 1 week, then frogs were released without any transmitter. Any non-radio-tagged A. limosus encountered during these radio-tracking surveys were identified or photographed and swabbed for incorporation into the mark–recapture estimates. The entire site was traversed during each radio telemetry survey, representing a continuous unconstrained search for all released animals. We also conducted weekly dedicated stream transect surveys to find non-radio-tracked animals, and the animals found were identified, photographed, and swabbed for mark–recapture analysis.
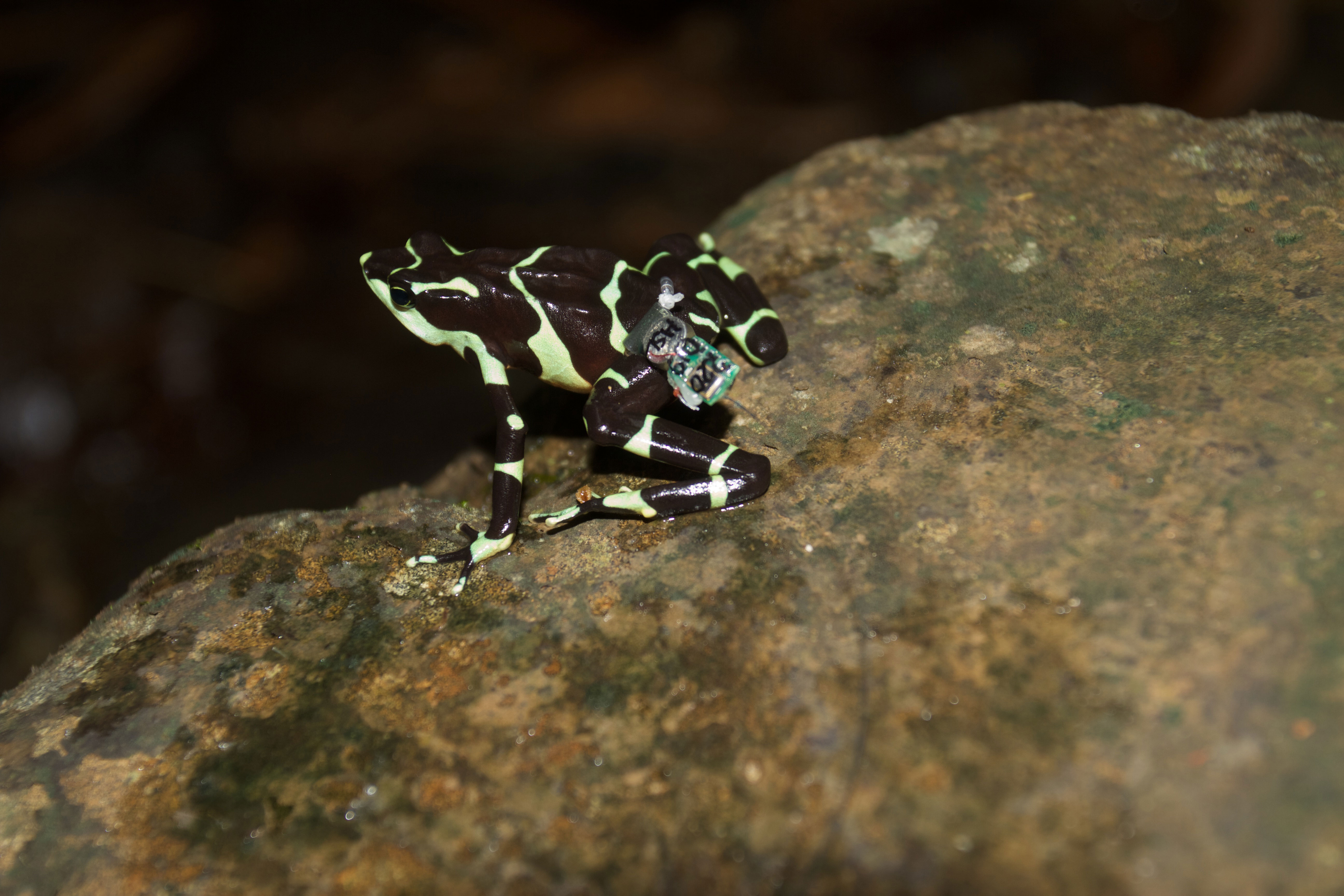
Figure 1 Atelopus limosus female fitted with a 0.31-g LB-2X transmitter with 21-day battery life attached using organic cotton threaded through a 1–2 mm silicone tubing waistband to prevent abrasion.
Statistical analysis
Movement
We used the haversine formula to calculate the daily distance that the frogs covered, for which we had consecutive day locations recorded. However, the precision of the GPS readings (within 7.6 m, SD 1.98 m) was often not sufficient to distinguish the fine-scale daily movements of the frogs. Mean weekly distances covered were therefore calculated if locations were available for individual frogs at 7-day intervals. We used weekly rainfall data collected using an automated rain gauge placed at the station to examine distance covered in relation to rainfall. We tested the hypothesis that weekly distance covered was related to week, release type, or rainfall using a GLMMPQL model in the MASS package in R (Ripley et al., 2019). We selected this approach over a regular GLM to deal with an unbalanced design, as consecutive daily or weekly locations were not collected for all individuals, and to accommodate for repeated measures using individual frog ID as a covariate (Venables and Ripley, 2002). Frog tracks were mapped in ggplot2 using R.
Mark–recapture analysis
We estimated treatment-specific (hard vs. soft) survival probabilities by fitting a Cormack–Jolly–Seber model to individual encounter history data (Cormack, 1964; Jolly, 1965; Seber, 1965). We fit this model in a hierarchical Bayesian framework by specifying (1) a sub-model for the true state of each individual over time (alive or dead) and (2) a sub-model for the observation data (observed or not) (Royle, 2008; Kéry and Schaub, 2011). Our dataset integrated both radio-tagged individuals and VI-tagged individuals recaptured by chance in each of 31 radio-tracking survey occasions and four dedicated stream recapture surveys in the 58-day study period (total of 291 recaptures). We estimated standardized 30-day survival probabilities for both the hard- and soft-release treatment groups. Field observations indicate that radio-tracked individuals may have poorer survivorship than VI-tagged animals due to transmitter lesions, but for the purposes of this analysis we assumed survival did not differ between radio-tracked and VI-tagged individuals in order to estimate general survivorship for hard- and soft-released groups. We accounted for different detection probabilities associated with radio telemetry and visual resighting of individuals marked with visual implant tags but assumed, due to similarly low numbers of recaptures, that the detection probability of VI-tagged individuals did not differ between dedicated stream surveys and incidental observations during radio telemetry surveys. In cases of known mortality events (n = 7 animals), this additional information was incorporated by supplying the alive/dead state of the individual as observed data, rather than treating the state as a parameter to be estimated for all surveys in which the individual was known to be dead. The lone exception was in the case of a single accidental mortality from a frog being stepped on, where observation data were “right-censored” by treating data as missing for all surveys after the mortality occurred to avoid biasing survival estimates. Observation data were also right-censored for four individuals from the date when radio transmitter signal was lost, with data treated as missing for all survey dates after the last detection of each individual.
We fit the model using the JAGS software run using the jagsUI package in the R program (Kellner, 2019; Plummer, 2020; R Development Core Team, 2021). We assigned uniform (0,1) priors for both detection and monthly survival probabilities. As is commonplace for most Bayesian analyses, JAGS performs model fitting using Markov chain Monte Carlo (MCMC) sampling by simulating probability distributions that are too complex to calculate mathematically. Probability distributions for model parameters were obtained by running three independent MCMC chains for 500,000 iterations each, discarding the first 250,000 iterations from each chain as “burn-in”, and thinning the remainder at a rate of 1 : 20 to obtain 37,500 samples from the joint posterior distribution (code provided in Supplementary Material 2).
Results
Release trial
Movement
After placing frogs alongside the stream, the animals mostly remained within 25 m of the stream, with a few, mostly hard-released individuals dispersing further (Figure 2). The release trial was started in May, which is considered the early rainy season in Panama, and dispersal appeared more pronounced after the onset of more frequent rainfall in June (BK, pers. obs.). However, there was no statistically significant relationship between dispersal distance and rainfall (Table 1). Released animals usually hid under cover, their aposematic colors became subdued, and they were very difficult to detect during re-surveys if they did not have radio transmitters. Just four re-encounters of VI-tagged frogs occurred on four dedicated stream transect surveys, and 23 re-encounters during 31 occasions spent searching for radio-tracked frogs (data in Supplementary Material S1). Hard-released frogs covered about 6 m further per week than soft-released animals [13.6 m (SD 9.1 m) vs. 7.7 m (SD 4.1 m), p= 0.017] (Table 1, Figure 3), and soft-released frogs appeared to settle more in the immediate stream vicinity than hard-released frogs (Figure 2). Week was not a statistically significant predictor of covered distance; weekly distances covered by hard-released frogs began to decline in week 3, approximating those of soft-released frogs by week 5 (Figure 3). Bd status was not predictive of movement distance among the soft-released radio-tracked animals. The mean weekly distance covered was 7.5 m (SD 0.53 m) for Bd-positive frogs, vs. 7.9 m (SD 1.8 m) for Bd-negative frogs, two-sample t-test p = 0.63 ns.
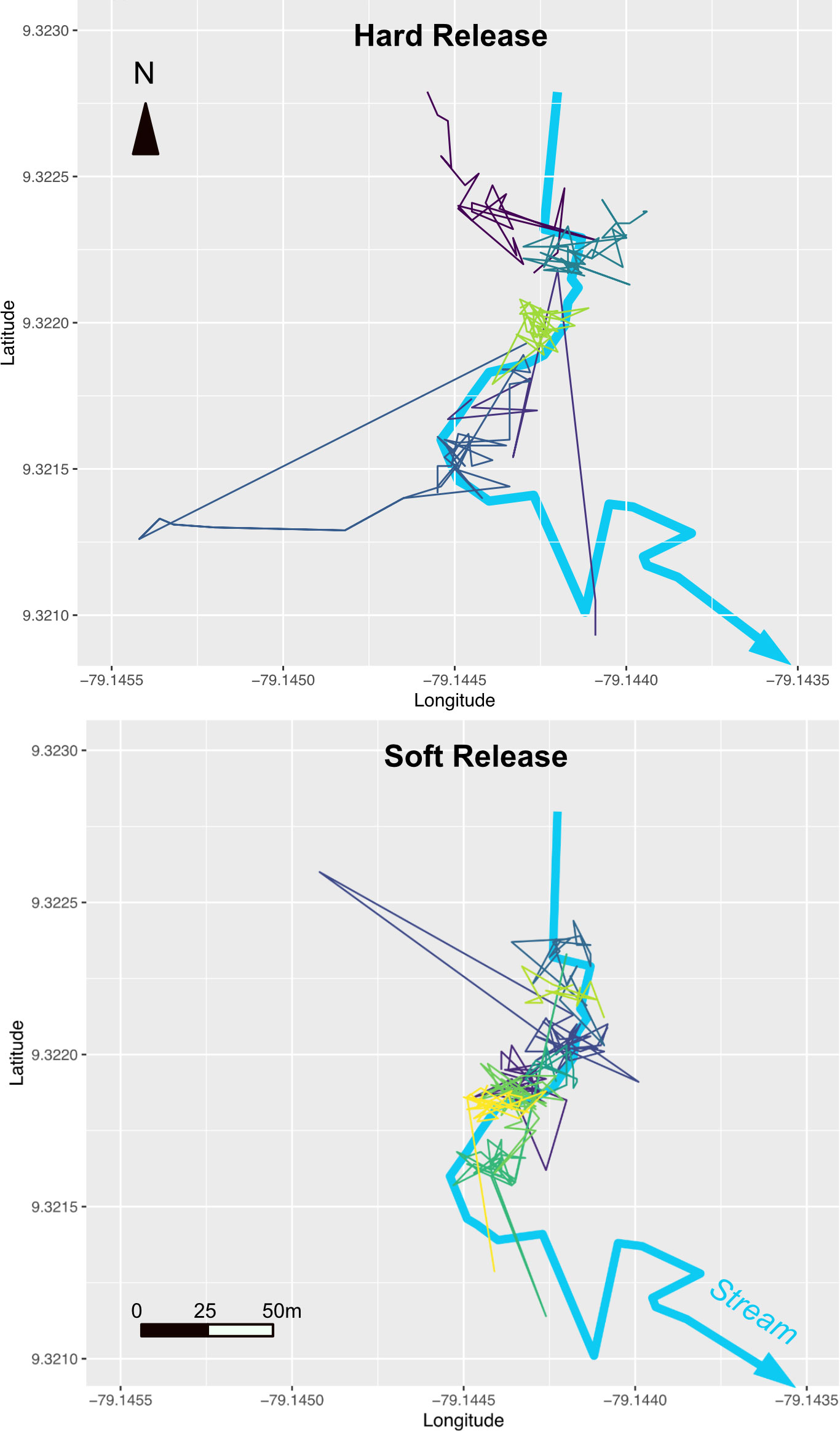
Figure 2 Tracks of individual hard-released animals (top) vs. soft-released animals (bottom) in relation to the release stream at the Mamoní Valley Preserve.
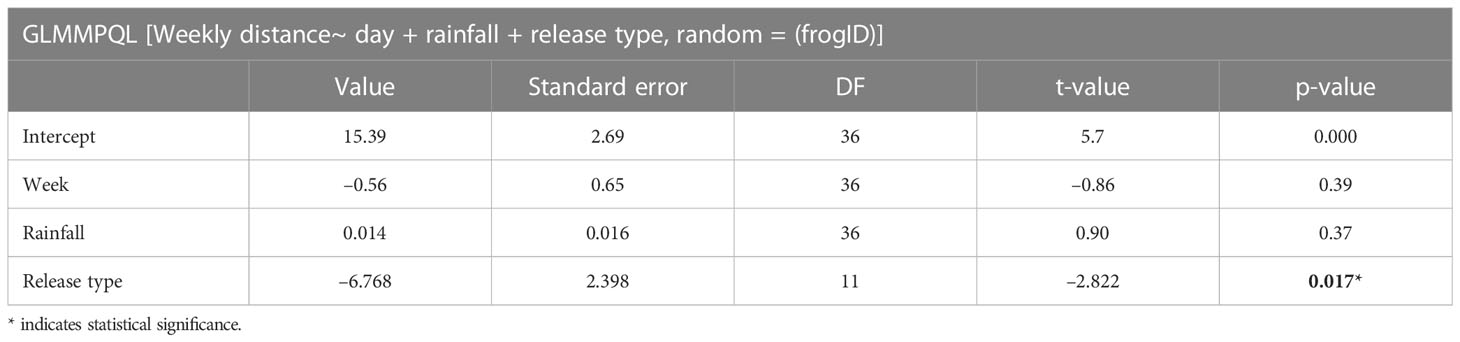
Table 1 Main effects of a GLMMPQL analysis examining the effects of time, rainfall, and release type on weekly distance covered.
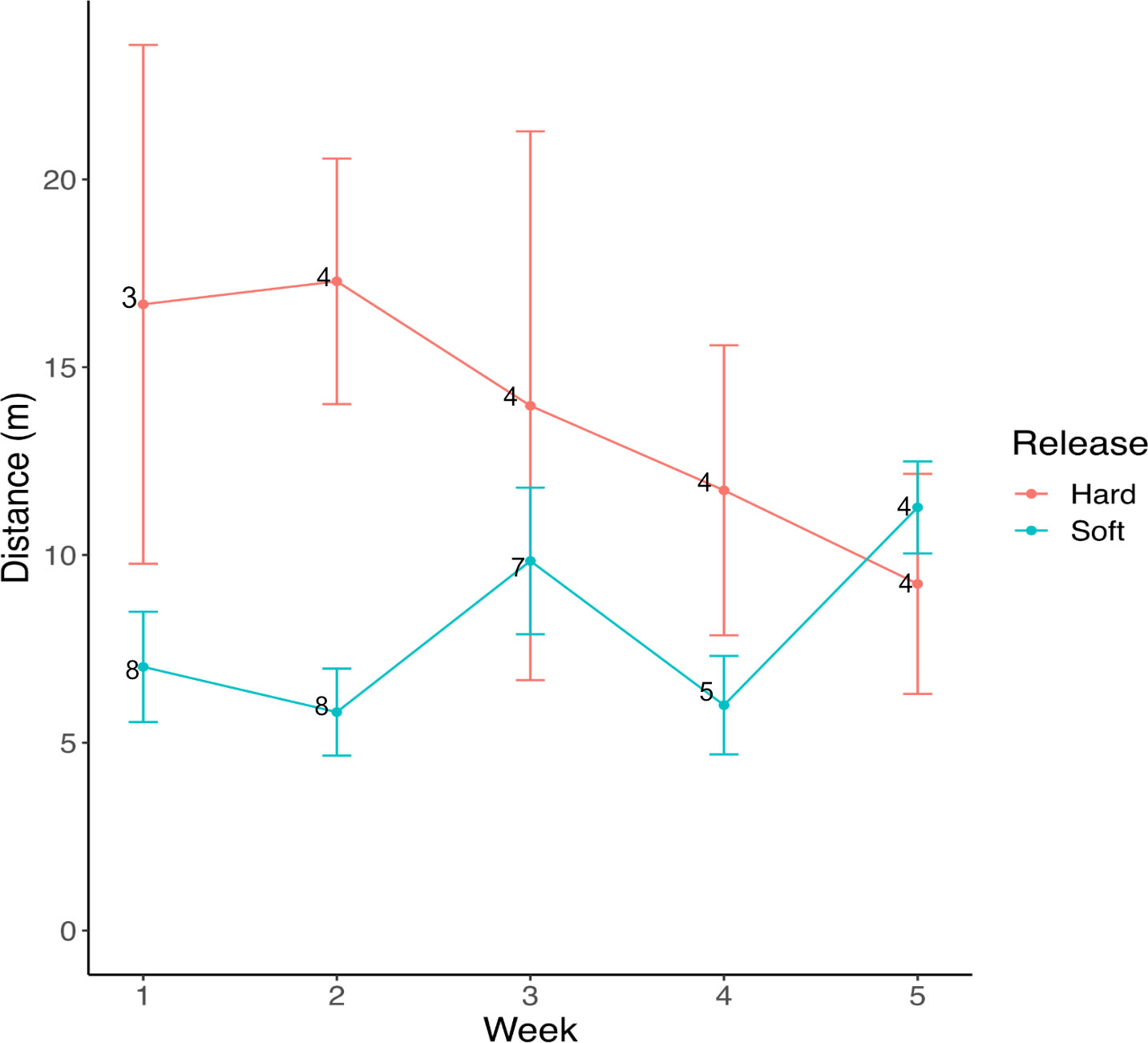
Figure 3 Mean weekly distances ± SE covered by hard- vs. soft-released frogs. Numerals opposite the plotted means indicate numbers of individual animals for which weekly movement was observed.
Survival
The individual fates of all radio-tracked animals are summarized in Table 2. Four of the frogs (25%) experienced abrasions from the radio transmitter and were removed from the experiment. All the abrasions were detected during the third consecutive transmitter placement (between days 36 and 54). Four of the transmitters (25%) failed at some point during the tracking. Two of the frogs died from predation and one likely died from Bd. Only two were released into the wild following their final recapture at the end of the 58-day tracking period (Table 2).
We estimated per-survey detection probabilities of 0.024 (95% CRI: 0.014 to 0.04) for visual resighting of marked individuals and 0.88 (95% CRI: 0.84 to 0.92) for radio tagged individuals. Our low recapture rate of marked individuals led to a small sample size and considerable uncertainty in survival estimates. Monthly survival probability was estimated at 0.31 (95% CRI: 0.13 to 0.57) for individuals in the hard-release treatment and 0.46 (95% CRI: 0.23 to 0.72) for individuals in the soft-release treatment. The estimated difference in monthly survival (soft – hard) was 0.15 (95% CRI: –0.15 to 0.44), which equated to a posterior probability of 0.85 that individuals in the soft-release treatment had higher survival rates (Figure 4).
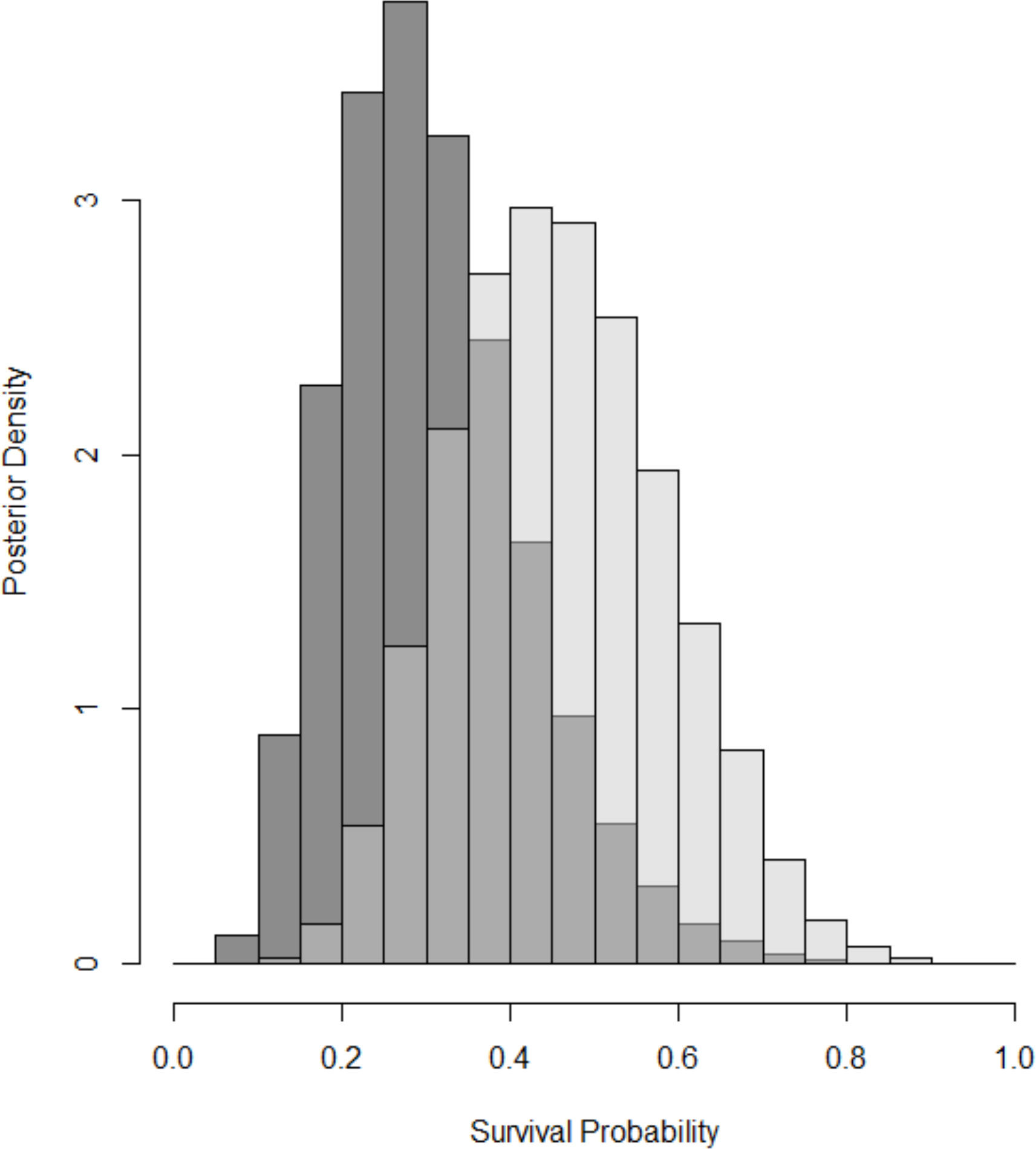
Figure 4 Comparison of 30-day survival probability between the hard-released animals (dark gray) and soft-released animals (light gray). Histograms show Bayesian posterior distributions for each treatment, which depict the relative likelihood of all possible values for survival.
Bd
After 1 month in mesocosms, about 25% of the frogs were Bd positive (Table 3, Estrada et al., 2022). This prevalence of Bd was similar to that observed in the stream frog community (24%–29%; Table 4) during our study. Smaller species of amphibians such as Dendrobatids or recent metamorphs of larger species were observed inside the mesocosms. Contaminated leaflitter potentially served as an additional source of infection. None of the hard-released animals were Bd positive at the time of release or upon subsequent recapture. One radio-tracked animal was found deceased with high Bd loads (6,950 zoospore equivalents) and two animals with transmitter-related abrasions died with high Bd loads (6,653 and 181,400 zoospore equivalents), with Bd likely contributing to the poor health and skin condition of these animals.
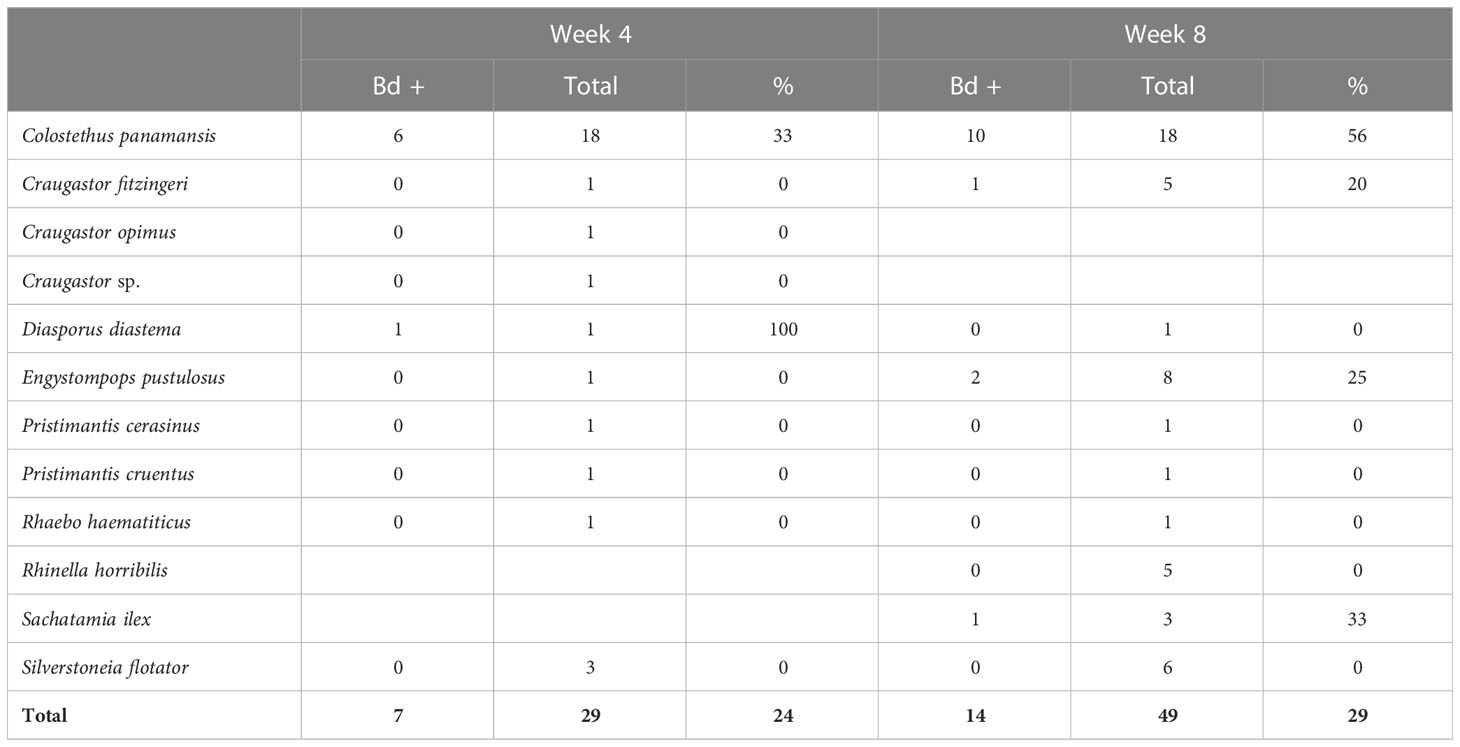
Table 4 Bd prevalence in the natural stream frog community at release stream transects during weeks 4 and 8 of the study.
Discussion
We were surprised by the low re-encounter rates of non-radio-tracked animals in stream transects. Hence, using this recapture survey method alone in our study would have been insufficient for post-release monitoring. We determined that a release of 83 animals should be sufficient, as similar transect-based mark–recapture surveys had monitored wild Atelopus populations of 60–100 animals (Tarvin et al., 2014; McCaffery et al., 2015; Lampo et al., 2017). However, newly released captive animals are unlikely to exhibit the site fidelity observed in wild Atelopus (Crump, 1986; Tarvin et al., 2014), and translocated animals may be prone to dispersal (Knox and Monks, 2014; Linhoff and Donnelly, 2022).
To counter low recapture rates, we employed a hybrid mark–recapture analysis that used both radio telemetry data and re-encounters of untracked animals, most of which were chance re-encounters during radio telemetry surveys. This approach allowed us to incorporate all redetection observations of animals into our mark–recapture model. Symptoms of heavy Bd infections include lethargy and the loss of righting reflex before death of the animal (Voyles et al., 2009); this could be a confounding influence explaining the reduced dispersal rates observed in the soft-released animals, as five out of nine soft-released radio-tracked animals were Bd positive by the end of the study and none of the hard-released animals was infected. However, there was no statistical difference in weekly distances covered between the soft-released Bd-positive and Bd-negative animals. Other studies with larger sample sizes noted that observed movement distances of Litoria pearsoniana were unrelated to Bd status (Murray et al., 2009). Although the probability of having a Bd infection in the soft-released cohort was much higher, there was likely a survival benefit from prior acclimation to the site in mesocosms. The observed reduction in survivorship of hard-released animals is likely connected to the increased dispersal distanced observed for these animals, as increased activity and movement is associated with increased predation risk in amphibians (Chovanec, 1992; Skelly, 1994). In another study, a hard-released amphibian dispersed outside of its optimal habitat and died from desiccation (Linhoff and Donnelly, 2022).
While it is possible that these observed differences in survival are due to chance, these data will be useful to future practitioners, as our data point to some important potential benefits of a delayed release program similar to those observed in other species (Tetzlaff et al., 2019). For instance, a 2-week acclimation period had marked beneficial effects in Wyoming toads (Linhoff and Donnelly, 2022). Our observation that weekly dispersal distances were greater for hard-released frogs than for soft-released frogs for the first 3–4 weeks (Figure 3) suggests that 1 month might be a suitable acclimation period for Atelopus. A separately published study of these soft-released frogs during the 28-day period in the mesocosm found that skin bacterial communities were rewilded in approximately 3 weeks and the body condition approached that of wild counterparts in 28 days (Estrada et al., 2022), providing further biological support for a 3–4-week delayed release period.
Anecdotal observations of the fate of radio-tracked animals suggest that predation, Bd, and injury from radio transmitters all contributed to the low survivorship probability. Predation in released animals is likely to be higher than in their wild counterparts in part due to the fact that wild A. limosus are known to have a powerful neurotoxin in their skin, known as chiriquitoxin (Yotsu-Yamashita and Tateki, 2010; Pearson and Tarvin, 2022). These toxins are lost in captive settings (Daly et al., 1997) and the released animals probably lacked valuable anti-predator skin defenses. Mesocosms are also known to reduce the dispersal tendency of newly released animals (Knox and Monks, 2014; Tetzlaff et al., 2019; Linhoff and Donnelly, 2022) and may therefore reduce the chances of encountering a predator, such as a whip scorpion (Phrynus sp.) or a fishing spider (Trechalea sp.), both of which were directly observed predating released animals. This reduced movement may account for the improved 30-day survivorship probability of soft-released animals.
While radio transmitters were the most reliable way of tracking and understanding the fate of these animals, they occasionally failed or were dropped, and abrasion injuries became an issue after about 3 weeks of transmitter attachment. It is important to note that the three known mortalities potentially linked to abrasions (Table 2) could cause an underestimation in the survival rates compared with a release of only non-tracked individuals under similar conditions. These mortalities would not contribute to the higher survival estimates in the soft-release treatment, as two of these three mortalities occurred in the soft-release treatment. However, any differences in survival between VI-tagged and radio-tagged individuals do have the potential to increase variability in survival within the soft- and hard-released groups and may have reduced our power to detect the effects of release treatment. Abrasions were likely due to a loose waistband placement that allowed the transmitter to flip to a ventral position, requiring regular fit checks in the field (BK, pers. obs.). It is also possible that Bd, which disrupts skin function and causes skin flaking (Baitchman and Pessier, 2013), may have compounded the severity of transmitter-related skin abrasions.
In summary, we learned that soft-release strategies of just 3–4 weeks reduce dispersal and likely improve the survival of animals. The rapid dispersal of captive-bred animals quickly reduces the densities of animals, increases predation risk, and increases the total number of animals needed in a release trial to conduct effective post-release monitoring. Recapture probabilities for marked animals are very low using conventional survey methods, and radio tracking is the most reliable way to re-encounter frogs. However, transmitters should be snugly fitted to reduce the likelihood of injury to the animal. Due to GPS error, GIS relocation has limitations for mapping fine-scale daily movements. Physical grids or mobile mapping applications to more precisely observe direct movements (McVey et al., 1981; Bartelt and Peterson, 2000; Pašukonis et al., 2018) will likely be needed to investigate territory establishment in Atelopus. This release trial did not directly seek to establish a viable population of A. limosus, but our results will inform and improve monitoring and release methods for future studies and reintroduction efforts. Further intensively monitored release trials will be needed to provide knowledge that will be key to species recovery.
Data availability statement
The original contributions presented in the study are included in the article/Supplementary Material. Further inquiries can be directed to the corresponding author.
Ethics statement
The animal study was reviewed and approved by Smithsonian Tropical Research ACUC 2016-0311-2019.
Author contributions
BK, AE, DM, BG, EB, and RI conceived the research. BK, BG, RI, DM, AE, and LB obtained research funding and permits for the field work. BK, MM, JG, ME, JB, AE, DM, BG, and RI conducted the field work. EB provided veterinary oversight. EI and RI analyzed swabs. BK, GC, and BG analyzed the data. LB, RI, EB, and BG supervised the project. BK and BG wrote the first draft of the manuscript. All authors contributed to the article and approved the submitted version.
Funding
This study was funded by the Shared Earth Foundation, Anele Kolohe Foundation, Holohil Systems Ltd grant program, and Smithsonian Women’s Committee. RI received support from the Sistema Nacional de Investigación, SENACYT. AE and DM were supported by a Smithsonian Tropical Research Institute short-term fellowship and the National Science Foundation (grant no. DEB-1136640 to LKB). This work is a product of the Panama Amphibian Rescue and Conservation Project, which is a partnership between the Cheyenne Mountain Zoo, Zoo New England, the Smithsonian’s National Zoo and Conservation Biology Institute, and the Smithsonian Tropical Research Institute.
Acknowledgments
We thank Mark Knetsch, Nico Armstrong, Kevin and Jamie Kratt, Caroline Gable, Suzanne Engel, and the Mamoní Valley Preserve for field assistance. Miambiente provided permits and field assistance. We thank Nathan Gray CoFounder Mamoní Valley Preserve & Geoversity for permission to conduct this work in the Mamoní Valley Preserve. We also thank the staff who reared the frogs at PARC: Nair Cabezón, Lanki Cheucarama, Rigoberto Díaz, and Nancy Fairchild.
Conflict of interest
This study received funding from a commercial entity, Holohil Systems Ltd. The funder was not involved in the study design; the collection, analysis, and interpretation of data; the writing of this article; or the decision to submit it for publication.
Publisher’s note
All claims expressed in this article are solely those of the authors and do not necessarily represent those of their affiliated organizations, or those of the publisher, the editors and the reviewers. Any product that may be evaluated in this article, or claim that may be made by its manufacturer, is not guaranteed or endorsed by the publisher.
Supplementary material
The Supplementary Material for this article can be found online at: https://www.frontiersin.org/articles/10.3389/famrs.2023.1205938/full#supplementary-material
Supplementary material S1 | (1) Atelopus radiotelemetry data, (2) recapture data, and (3) Bd data.
Supplementary material S2 | R code for the Cormack–Jolly–Seber model.
References
Altobelli J. T., Dickinson K. J. M., Godfrey S. S., Bishop P. J. (2022). Methods in amphibian biotelemetry: two decades in review. Austral Ecol. 47, 1382–1395. doi: 10.1111/aec.13227
Armstrong D. P., Seddon P. J. (2008). Directions in reintroduction biology. Trends Ecol. Evol. 23, 20–25. doi: 10.1016/J.TREE.2007.10.003
Baitchman E. J., Pessier A. (2013). Pathogenesis, diagnosis and treatment of amphibian chytridiomycosis. Vet. Clin. Exot Anim. 16, 669–685. doi: 10.1016/j.cvex.2013.05.009
Bartelt P. E., Peterson C. R. (2000). A description and evaluation of a plastic belt for attaching radio transmitters to Western toads (Bufo boreas). Northwest. Nat. 81, 122. doi: 10.2307/3536823
Bosch J., Sanchez-Tome E., Fernandez-Loras A., Oliver J. A., Fisher M. C., Garner T. W. J. (2015). Successful elimination of a lethal wildlife infectious disease in nature. Biol. Lett. 11, 20150874–20150874. doi: 10.1098/rsbl.2015.0874
Boyle D. G., Boyle D. B., Olsen V., Morgan J. T., Hyatt A. D. (2004). Rapid quantitative detection of chytridiomycosis (Batrachochytrium dendrobatidis) in amphibian samples using real-time taqman PCR assay. Dis. Aquat. Organ. 60, 141–148. doi: 10.3354/dao060141
Brannelly L. A., Richards-Zawacki C. L., Pessier A. P. (2012). Clinical trials with itraconazole as a treatment for chytrid fungal infections in amphibians. Dis. Aquat. Organ. 101, 95–104. doi: 10.3354/dao02521
Byrne A. Q., Richards-Zawacki C. L., Voyles J., Bi K., Ibáñez R., Rosenblum E. B. (2021). Whole exome sequencing identifies the potential for genetic rescue in iconic and critically endangered Panamanian harlequin frogs. Glob. Change Biol. 27, 50–70. doi: 10.1111/gcb.15405
Chovanec A. (1992). The influence of tadpole swimming behaviour on predation by dragonfly nymphs. Amphibia-Reptilia 13 (4), 341–349. doi: 10.1163/156853892X00049
Cormack R. M. (1964). Estimates of survival from the sighting of marked animals. Biometrika 51, 429. doi: 10.2307/2334149
Crump M. L. (1986). Homing and site fidelity in a Neotropical frog, Atelopus varius (Bufonidae ). Copeia 1986, 438–444. doi: 10.2307/1445001
Daly J. W., Padgett W. L., Saunders R. L., Cover J. F. (1997). Absence of tetrodotoxins in a captive-raised riparian frog, Atelopus varius. Toxicon 35, 705–709. doi: 10.1016/S0041-0101(96)00165-1
Estrada A., Medina D., Gratwicke B., Ibáñez R., Belden L. K. (2022). Body condition, skin bacterial communities and disease status: insights from the first release trial of the Limosa harlequin frog, Atelopus limosus. Proc. R. Soc B Biol. Sci. 289, 20220586. doi: 10.1098/rspb.2022.0586
Garner T. W. J., Schmidt B. R., Martel A., Pasmans F., Muths E., Cunningham A. A., et al. (2016). Mitigating amphibian chytridiomycoses in nature. Philos. Trans. R. Soc Lond. B. Biol. Sci. 371, 20160207. doi: 10.1098/rstb.2016.0207
Geoversity (2020)Mamoni valley preserve. In: Mamoni val. preserv. Available at: http://mamonivalleypreserve.org/#valley (Accessed September 16, 2020).
González-Maya J. F., Gómez-Hoyos D. A., Cruz-Lizano I., Schipper J. (2018). From hope to alert: demography of a remnant population of the critically endangered Atelopus varius from Costa Rica. Stud. Neotrop. Fauna Environ. 53, 194–200. doi: 10.1080/01650521.2018.1460931
Gratwicke B., Ross H., Batista A., Chaves G., Crawford A. J., Elizondo L., et al. (2016). Evaluating the probability of avoiding disease-related extinctions of Panamanian amphibians through captive breeding programs. Anim. Conserv. 19, 324–336. doi: 10.1111/acv.12249
Griffiths R. A., Pavajeau L. (2008). Captive breeding, reintroduction, and the conservation of amphibians. Conserv. Biol. 22, 852–861. doi: 10.1111/j.1523-1739.2008.00967.x
Heard G. W., Scroggie M. P., Malone B. (2008). Visible implant alphanumeric tags as an alternative to toe-clipping for marking amphibians – a case study. Wildl. Res. 35, 747. doi: 10.1071/WR08060
Heyer R., Donnelly M. A., Foster M., McDiarmid R. W. (1994) Measuring and monitoring biological diversity: standard methods for amphibians. Available at: https://books.google.com/books?hl=en&lr=&id=mqdqBgAAQBAJ&pgis=1 (Accessed January 22, 2016).
Hyatt A. D., Boyle D. G., Olsen V., Boyle D. B., Berger L., Obendorf D., et al. (2007). Diagnostic assays and sampling protocols for the detection of batrachochytrium dendrobatidis. Dis. Aquat. Org. 73, 175–192.
Jachowski D., Millspaugh J. J., Angermeier P. L., Slotow R. H. (2016). Reintroduction of fish and wildlife populations (Oakland, CA, USA.: University of California Press Oakland).
Jaynes K. E., Páez-Vacas M. I., Salazar-Valenzuela D., Guayasamin J. M., Terán-Valdez A., Siavichay F. R., et al. (2022). Harlequin frog rediscoveries provide insights into species persistence in the face of drastic amphibian declines. Biol. Conserv. 276, 109784. doi: 10.1016/j.biocon.2022.109784
Jolly G. M. (1965). Explicit estimates from capture-recapture data with both death and immigration-stochastic model. Biometrika 52, 225–247. doi: 10.2307/2333826
Kellner K. (2019) jagsUI: a wrapper around “rjags” to streamline “JAGS” analyses. Available at: https://CRAN.R-project.org/package=jagsUI.
Kéry M., Schaub M. (2011). Bayesian Population analysis using WinBUGS: a hierarchical perspective. (Cambridge, MA, USA: Academic Press). doi: 10.1016/C2010-0-68368-4
Knox C. D., Monks J. M. (2014). Penning prior to release decreases post-translocation dispersal of jewelled geckos. Anim. Conserv. 17, 18–26. doi: 10.1111/ACV.12149
Kriger K. M., Hines H. B., Hyatt A. D., Boyle D. G., Hero J. M. (2006). Techniques for detecting chytridiomycosis in wild frogs: comparing histology with real-time taqman PCR. Dis. Aquat. Organ. 71, 141–148. doi: 10.3354/dao071141
Kriger K. M., Hero J.-M. (2007). Large-scale seasonal variation in the prevalence and severity of chytridiomycosis. J. Zool. 271, 352–359. doi: 10.1111/j.1469-7998.2006.00220.x
La Marca E., Lips K. R., Lötters S., Puschendorf R., Ibáñez R., Rueda-Almonacid J. V., et al. (2005). Catastrophic population declines and extinctions in neotropical harlequin frogs (Bufonidae: Atelopus). Biotropica 37, 190–201. doi: 10.1111/j.1744-7429.2005.00026.x
Lampo M., Señaris C., García C. Z. (2017). Population dynamics of the critically endangered toad Atelopus cruciger and the fungal disease chytridiomycosis. PloS One 12, e0179007. doi: 10.1371/journal.pone.0179007
Lewis C. H. R., Richards-Zawacki C. L., Ibáñez R., Luedtke J., Voyles J., Houser P., et al. (2019). Conserving Panamanian harlequin frogs by integrating captive-breeding and research programs. Biol. Conserv. 236, 180–187. doi: 10.1016/j.biocon.2019.05.029
Linhoff L. J., Donnelly M. A. (2022). Assessing release strategies for reintroductions of endangered Wyoming toads. Wildl. Soc Bull. 46, e1341. doi: 10.1002/wsb.1341
Lips K. R. (1999). Mass mortality and population declines of anurans at an upland site in Western Panama. Conserv. Biol. 13, 117–125. doi: 10.1046/j.1523-1739.1999.97185.x
McCaffery R., Richards-Zawacki C. L., Lips K. R. (2015). The demography of Atelopus decline: harlequin frog survival and abundance in central Panama prior to and during a disease outbreak. Glob. Ecol. Conserv. 4, 232–242. doi: 10.1016/j.gecco.2015.07.003
McVey M. E., Zahary R. G., Perry D., MacDougal J. (1981). Territoriality and homing behavior in the poison dart frog (Dendrobates pumilio). Copeia 1981, 1–8. doi: 10.2307/1444035
Morrison M. L. (2015). Advances in reintroduction biology of Australian and New Zealand Fauna. Restor. Ecol. 23, 721–722. doi: 10.1111/rec.12266
Murray K. A., Skerratt L. F., Speare R., McCallum H. (2009). Impact and dynamics of disease in species threatened by the amphibian chytrid fungus, Batrachochytrium dendrobatidis. Conserv. Biol. 23 (5), 1242–1252. doi: 10.1111/j.1523-1739.2009.01211.x
Osbourn M. S., Hocking D. J., Conner C. A., Peterman W. E., Semlitsch R. D. (2011). Use of fluorescent visible implant alphanumeric tags to individually mark juvenile ambystomatid salamanders. Herpetol. Rev. 42, 43–47.
Pašukonis A., Loretto M. C., Hodl W. (2018). Map-like navigation from distances exceeding routine movements in the three-striped poison frog (Ameerega trivittata). J. Exp. Biol. 221, 169714. doi: 10.1242/JEB.169714/262662/AM/MAP-LIKE-NAVIGATION-FROM-DISTANCES-EXCEEDING
Pašukonis A., Loretto M. C., Landler L., Ringler M., Hödl W. (2014). Homing trajectories and initial orientation in a Neotropical territorial frog, allobates femoralis (Dendrobatidae). Front. Zool. 11. doi: 10.1186/1742-9994-11-29
Pearson K. C., Tarvin R. D. (2022). A review of chemical defense in harlequin toads (Bufonidae: Atelopus). Toxicon X 13, 100092. doi: 10.1016/J.TOXCX.2022.100092
Pittman S. E., Jendrek A. L., Price S. J., Dorcas M. E. (2008). Habitat selection and site fidelity of cope’s Gray treefrog (Hyla chrysoscelis) at the aquatic-terrestrial ecotone. J. Herpetol. 42, 378–385. doi: 10.1670/07-1702.1
Plummer M. (2020) JAGS: just another Gibbs sampler download | SourceForge.net. JAGS softw. Available at: https://sourceforge.net/projects/mcmc-jags/files/ (Accessed September 17, 2020).
R Development Core Team (2021). R: a language and environment for statistical computing. (Vienna, Austria: R Foundation for Statistical Computing). ISBNISBN 3-900051-07-0.
Ripley B., Venables B., Bates D. M., Hornik K., Gebhardt A., Firth D. (2019). MASS 7.3-51.5 support functions and datasets for Venables and ripley’s MASS “Modern applied statistics with s”, 4th edition. (Oxford, UK: CRAN).
Rowley J. J. L., Alford R. A. (2007). Techniques for tracking amphibians: the effects of tag attachment, and harmonic direction finding versus radio telemetry. Amphibia-Reptilia 28, 367–376. doi: 10.1163/156853807781374755
Royle J. A. (2008). Modeling individual effects in the cormack-Jolly-Seber model: a state-space formulation. Biometrics 64, 364–370. doi: 10.1111/j.1541-0420.2007.00891.x
Scheele B. C., Pasmans F., Skerratt L. F., Berger L., Martel A., Beukema W., et al. (2019). Amphibian fungal panzootic causes catastrophic and ongoing loss of biodiversity. Sci. (80-. ). 363, 1459–1463. doi: 10.1126/science.aav0379
Seber G. A. (1965). A note on the multiple-recapture census. Biometrika 52, 249–259. doi: 10.2307/2333827
Skelly D. K. (1994). Activity level and the susceptibility of anuran larvae to predation. Anim. Behaviour. 47, 465–468. doi: 10.1006/anbe.1994.1063
Tarvin R. D., Peña P., Ron S. R. (2014). Changes in population size and survival in Atelopus spumarius (Anura: Bufonidae) are not correlated with chytrid prevalence. J. Herpetol. 48, 291–297. doi: 10.1670/11-269
Tetzlaff S. J., Sperry J. H., DeGregorio B. A. (2019). Effects of antipredator training, environmental enrichment, and soft release on wildlife translocations: a review and meta-analysis. Biol. Conserv. 236, 324–331. doi: 10.1016/J.BIOCON.2019.05.054
Veseley M., Batista A. (2021). A new species of Atelopus (Amphibia, Bufonidae) from antioquia, Colombia. Zool. Res. 42, 272–279. doi: 10.24272/j.issn.2095-8137.2020.319
Voyles J., Woodhams D. C., Saenz V., Byrne A. Q., Perez R., Rios-Sotelo G., et al. (2018). Shifts in disease dynamics in a tropical amphibian assemblage are not due to pathogen attenuation. Science 359, 1517–1519. doi: 10.1126/science.aao4806
Voyles J., Young S., Berger L., Campbell C., Voyles W. F., Dinudom A., et al. (2009). Pathogenesis of chytridiomycosis, a cause of catastrophic amphibian declines. Science 326 (5952), 582–585. doi: 10.1126/science.1176765
Keywords: captive-breeding, amphibian, mesocosm, release, radio telemetry, translocation
Citation: Klocke B, Estrada A, Mataya M, Medina D, Baitchman E, Belden L, Guerrel J, Evans M, Baughman J, Connette G, Illueca E, Ibáñez R and Gratwicke B (2023) Movement and survival of captive-bred Limosa harlequin frogs (Atelopus limosus) released into the wild. Front. Amphib. Reptile Sci. 1:1205938. doi: 10.3389/famrs.2023.1205938
Received: 14 April 2023; Accepted: 22 June 2023;
Published: 26 July 2023.
Edited by:
Trenton Garner, Zoological Society of London, United KingdomReviewed by:
Franco Andreone, Museo Regionale di Scienze Naturali, ItalyDavid Lesbarreres, Environment and Climate Change Canada (ECCC), Canada
Copyright © 2023 Klocke, Estrada, Mataya, Medina, Baitchman, Belden, Guerrel, Evans, Baughman, Connette, Illueca, Ibáñez and Gratwicke. This is an open-access article distributed under the terms of the Creative Commons Attribution License (CC BY). The use, distribution or reproduction in other forums is permitted, provided the original author(s) and the copyright owner(s) are credited and that the original publication in this journal is cited, in accordance with accepted academic practice. No use, distribution or reproduction is permitted which does not comply with these terms.
*Correspondence: Brian Gratwicke, Z3JhdHdpY2tlYkBzaS5lZHU=