- 1Lee Kong Chian School of Medicine, Nanyang Technological University, Singapore, Singapore
- 2Interdisciplinary Graduate Programme (IGP-Neuroscience), Nanyang Technological University, Singapore, Singapore
- 3Department of Research, National Neuroscience Institute, Singapore, Singapore
- 4Genome Institute of Singapore, Agency for Science, Technology and Research (A*STAR), Singapore, Singapore
Synaptic Vesicle Glycoprotein 2C (SV2C), characterized by its selective expression in discrete brain regions such as the midbrain, has recently emerged as a promising player in Parkinson’s Disease (PD) – a debilitating neurodegenerative disorder affecting millions worldwide. This review aims to consolidate our current understanding of SV2C’s function, its involvement in PD pathogenesis, and to evaluate its potential as a therapeutic target. Integrating previous findings of SV2C, from genetics to molecular studies, and drawing on insights from the largest East Asian genome-wide association study that highlights SV2C as a novel risk factor for PD, we explore the potential pathways through which SV2C may influence the disease. Our discussion extends to the implications of SV2C’s role in synaptic vesicle trafficking, neurotransmitter release, and α-synuclein homeostasis, thereby laying the groundwork for future investigations that could pave the way for novel therapeutic strategies in combating PD.
1 Introduction
Synaptic vesicles (SVs) are essential in neural communication, packaging and releasing neurotransmitters into the synaptic cleft to facilitate synaptic transmission. The precise regulation of SV loading, transporting and recycling is crucial for maintaining the fidelity of synaptic transmission. Disruptions in this machinery can lead to various neurological disorders, ranging from neurodevelopmental conditions such as Autism Spectrum Disorders to neurodegenerative diseases such as Alzheimer’s Disease and Parkinson’s Disease (PD) (Lepeta et al., 2016).
Within the diverse array of SV proteins, the Synaptic Vesicle Glycoprotein 2 (SV2) family includes three distinct proteins: SV2A, SV2B and SV2C. These integral synaptic membrane proteins play an important role in synaptic processes and comprehensive reviews outlining the structure and proposed functions of SV2 proteins have been detailed by Bartholome et al. (2017), Ciruelas et al. (2019), Hu et al. (2017), and Stout et al. (2019). SV2A is the most extensively-studied paralog due to its implication in epilepsy; it is the target of the anti-epileptic drug levetiracetam (Lynch et al., 2004). Studies on SV2A-deficient mice have revealed its essential role in GABAergic neurotransmission, with the loss of SV2A leading to spontaneous seizures and early post-natal mortality (Crowder et al., 1999). In contrast to SV2A, which is ubiquitous across neuronal synapses, SV2B predominantly localized to excitatory synapses (Bajjalieh et al., 1994). Interestingly, SV2B demonstrates more robust expression in the brain during early development compared to adulthood, although its specific roles in developmental processes remain unclear. While there is some evidence suggesting a potential involvement of SV2B in Alzheimer’s disease, its exact role, particularly in relation to amyloid beta toxicity, remains to be fully elucidated (Detrait et al., 2014). Conversely, our understanding of SV2C remained obscure until recent years. Emerging evidence has begun to unveil its potential involvement in PD, a progressive neurological disorder characterized by the degeneration of dopaminergic neurons in the substantia nigra pars compacta (SNpc) of the midbrain. This association is particularly noteworthy given that SV2C is predominantly expressed in the dopaminergic neurons in the midbrain, though it is also present in GABAergic and cholinergic neurons (Dardou et al., 2013). The majority of PD-linked genes are expressed rather ubiquitously. The exploration of SV2C’s function and its underlying mechanisms within the dopaminergic pathways – which are critically impacted in PD – thus presents an interesting area of research.
This review delves into the current literature surrounding SV2C, particularly its link to PD. It explores the role of SV2C in synaptic neurotransmission and evaluates its potential as a biomarker or therapeutic target. By examining recent studies and pinpointing gaps in our understanding of SV2C’s potential function in PD pathogenesis, this paper aims to highlight the importance of SV2C in neurobiology and its potential significance in advancing PD research.
2 Discovery of SV2C and its distinctive localisation in the brain
The functional role of SV2 proteins within the nervous system remains an area of active investigation. SV2 proteins play a crucial role in synaptic transmission, notably in priming SVs for exocytosis and influencing synaptotagmin trafficking (Chang and Südhof, 2009; Yao et al., 2010). They are also recognized as receptors for botulinum neurotoxin A, modulating its entry into the neurons (Dong et al., 2006).
SV2C, the most recently identified member of SV2 protein family, was discovered by Janz and Südhof in 1999. Like its counterparts, SV2A and SV2B, SV2C is characterized by 12 putative transmembrane domains, a cytoplasmic N-terminal tail and a large intraluminal loop (Janz and Südhof, 1999). Each paralog is encoded on a different chromosome – SV2A on chromosome 1, SV2B on chromosome 15 and SV2C on chromosome 5. Despite sharing approximately 80% structural homology with SV2A and SV2B, SV2C’s expression in the brain is notably distinct. Unlike the widespread expression of SV2A and SV2B throughout most regions of the rat brain, SV2C shows restricted expression in phylogenetically older brain regions like the olfactory bulb (OB), the midbrain and the hindbrain. This regional specificity was corroborated by studies in mice and macaque which also demonstrated specific localisation in the striatum and the substantia nigra (Dardou et al., 2011; Dunn et al., 2017, 2019). Intriguingly, these areas are also central to the Braak staging of PD (Braak et al., 2003). Although the exact role of SV2C in PD’s pathological progression is unclear, its selective localisation in these regions hints at a unique and potentially significant role within certain neuronal circuits.
Similar to SV2A, the N-terminal tail of SV2C harbors a unique binding site for synaptotagmin-1 (SYT1), a calcium sensor essential for mediating the fusion of synaptic vesicle membrane with the plasma membrane during neurotransmitter exocytosis (Schivell et al., 2005). Previous investigations revealed that mutation of the endocytosis domain within the amino-terminal SYT1-binding domain of SV2A result in defective trafficking of SYT1 to synaptic vesicles (Haucke and De Camilli, 1999; Yao et al., 2010). While the sequence similarity between SV2A and SV2C suggests a potential role of SV2C in SYT1 trafficking, direct evidence supporting SV2C’s involvement in the trafficking of SYT1 remains elusive.
3 Pioneering investigations of SV2C in Parkinson’s disease
The initial link between SV2C polymorphisms and PD was reported by Hills-Burns and colleagues, who identified an SV2C ortholog in a genome-wide gene–environment interaction study using a nicotine-paraquat Drosophila model of PD. Although SV2C did not achieve genome-wide significance in their subsequent study, it emerged as the most notable gene. Specifically, single nucleotide polymorphisms (SNPs) within the SV2C gene, rs30196 and rs10214163, were identified as having the potential to influence the neuroprotective effects of smoking on PD risk (Hill-Burns et al., 2013). This finding underscores the intricate relationship between genetic factors and environmental exposures, such as tobacco smoking, which has been associated with a reduced PD risk, potentially through the neuroprotective properties of nicotine (Li et al., 2015). Numerous in vivo studies have demonstrated that nicotine not only decreases the degeneration of dopaminergic neurons in animal models of PD but also enhances neuronal survival, while concurrently promoting dopamine release (Quik et al., 2008; Turner, 2004). Dunn et al. (2017), suggested that SV2C may mediate nicotine’s modulation of dopamine release, as their study showed that mice lacking SV2C did not exhibit an increase in dopamine release upon nicotine stimulation. Although the relationship between SV2C and nicotine remains unclear, it is possible that SV2C is part of the neurotransmitter pathway modulated by nicotinic acetylcholine receptors on dopaminergic neurons. Nonetheless, the underlying mechanism for this relationship requires further investigation.
Genetic variations within the SV2C gene have also been implicated modulating the response to dopamine replacement therapies in PD. Notably, the presence of SV2C rs30196 C allele has been correlated with a requirement for lower doses of Levodopa, the primary treatment for managing early-stage PD motor symptoms (Altmann et al., 2016). Conversely, the rs1423099 T allele is associated with a reduced incidence of treatment-related side effects, such as nausea or vomiting (Altmann et al., 2016; Redenšek et al., 2019). While these findings have yet to be replicated in independent studies, such insights may suggest that SV2C genetic variants could play a critical role in tailoring PD management strategies, offering a framework for the stratification of PD subtypes and the selection of individualized therapeutic options that maximize efficacy and minimize adverse effects. Given the complexity of genetic influences on treatment responses, further research is essential to fully elucidate the potential of SV2C in guiding personalized PD treatment paradigms.
Additionally, it is plausible that these variants, most of which are non-coding, could influence SV2C expression or function. Non-coding variants may affect regulatory elements such as promoters, enhancers, or splice sites, thereby altering the levels of SV2C expression. Changes in SV2C levels could have an effect on synaptic vesicle trafficking and neurotransmitter release. Furthermore, alterations in SV2C function or localization could modulate its interaction with other synaptic proteins. These speculative mechanisms highlight the need for detailed molecular studies to understand the precise impact of SV2C genetic variants on its biological functions and their implications for PD therapy. SV2C is prominently expressed in nearly all dopaminergic neurons within the midbrain, including the substantia nigra and the striatum, pointing to its potential role in modulating dopamine neurotransmission (Dardou et al., 2011). SV2C-deficient mice exhibit elevated gene expression of tyrosine hydroxylase, the rate-limiting enzyme responsible for dopamine synthesis, in the SNpc and displayed diminished locomotor activity in an open field test (Dardou et al., 2013). Notably, the denervation of dopaminergic neurons, induced by MPTP and 6-OHDA lesions, led to a modest but significant upregulation of SV2C mRNA in the caudate-putamen on the lesioned side compared to the non-lesioned side using in situ hybridization techniques. This suggests SV2C’s involvement in the dopaminergic system, especially under insult. While the exact role of SV2C in dopaminergic neurotransmission and PD pathology remains to be fully elucidated, this study is pivotal in establishing the potential significance of SV2C in dopaminergic neurons and serves as a crucial foundation for future investigations aimed at unraveling the functions of SV2C and its implication for PD.
Dopamine homeostasis is critical for neuronal health, as the accumulation of cytosolic dopamine can increase the vulnerability of dopaminergic neurons (Mosharov et al., 2009). The study by Dunn et al. (2017) identifies a crucial link between SV2C deficiency in mice and disrupted dopamine regulation, manifesting as reduced dopamine content and release, alongside mild motor deficits in locomotion (Dunn et al., 2017). Intriguingly, SV2C was found to co-immunoprecipitate with α-synuclein, although there is currently no evidence for a direct interaction between the two proteins. Furthermore, the presence of increased high molecular weight α-synuclein in SV2C-deficient mice suggests a potential modulatory role of SV2C on the functioning or aggregation processes of α-synuclein. These findings may imply that SV2C may play a crucial role in the pathological changes associated with α-synuclein, potentially influencing its propensity to aggregate. This interaction may underpin key aspects of synaptic dysfunction observed in PD, given the central role of α-synuclein in Lewy body pathology as well as its involvement at presynaptic sites (Calabresi et al., 2023). While the study provides compelling evidence for the role of SV2C in dopaminergic neurotransmission and its possible interaction with α-synuclein aggregation, the use of mouse models necessitates cautious extrapolation to human PD pathology. Future investigations should aim to elucidate the precise mechanisms by which SV2C influences synaptic vesicle dynamics, as well as α-synuclein misfolding or aggregation. This research could potentially illuminate novel therapeutic targets for mitigating synaptic dysfunction or the Lewy body pathology in PD.
4 SV2C emergence: a novel risk locus for Parkinson’s disease uncovered
The largest East Asian genome-wide association study (GWAS) conducted in 6,724 patients and 24,851 controls identified SV2C as a novel risk locus for PD, with the intronic SNP rs246814 showing genome-wide significant association with PD (p = 3.48 × 10−8; OR = 1.24). This association is replicated across both Asian and European cohorts, with differences in allele frequencies between the two populations (minor allele frequency in Asians: 9–11%; in Europeans: 7–8%) (Foo et al., 2020). This finding was further corroborated by a subsequent study in the European ancestry population by Grover et al. (2021) and observed in the Singapore population by which the variant is present at different frequencies between mild and severe PD subtypes, underscoring SV2C’s potential role in PD risk modulation and disease progression (Deng et al., 2022; Grover et al., 2021). Interestingly, the rs246814 is in complete linkage disequilibrium with the missense variant rs31244, located in exon 10 of SV2C gene, which results in the amino acid substitution p.Asp543Asn within SV2C’s luminal domain and could potentially introduce a new N-glycosylation motif (N-X-S/T). The large luminal domain of SV2C protein contains three N-glycosylation sites which are well-conserved across SV2 proteins and binds with high affinity to botulinum neurotoxin A (Dong et al., 2006; Iezzi et al., 2005; Janz and Südhof, 1999). That being said, empirical validation is essential to confirm the impact of this amino acid substitution on SV2C’s N-glycosylation. This highlights a critical gap in our understanding, as the precise role of N-glycosylation alterations in the SV2C’s function and its interaction with other synaptic proteins are yet to be elucidated. Given the crucial role of glycosylation in the luminal loop of SV2 proteins on localizing the protein to the synapse from the endoplasmic reticulum-Golgi apparatus, detailed biochemical and cellular studies are warranted to clarify the biological implications of the rs31244 variant (Nowack et al., 2010). Future investigations should aim not only to validate the functional predictions but also to investigate how glycosylation changes in SV2C might contribute to the pathophysiology of PD, potentially unveiling new disease pathways for therapeutic intervention.
5 Advancements and novel perspectives in SV2C—Parkinson’s disease research
Recent investigations have expanded our understanding of SV2C’s functions, particularly its mediation of nicotine effect on autophagy flux, as demonstrated in an α-synuclein-based Drosophila model where the effect of nicotine to decrease α-synuclein aggregates were abolished by the knockdown of SV2C (Olsen et al., 2023). Autophagy, crucial for degradation and recycling of dysfunctional proteins and organelles, is integral in maintaining protein homeostasis and neuronal health. Dysregulation of this process has been implicated in the pathogenesis of PD, potentially contributing to the synaptic degradation that precedes neuronal loss (Soukup et al., 2018). Whether SV2C influences autophagy, and the precise mechanisms by which it may do so, remain elusive, highlighting the need for future research to dissect its interactions with key players of the autophagic machinery.
In more recent investigations, cutting-edge single-nuclei RNA sequencing analysis on post-mortem substantia nigra from PD patients and healthy controls has revealed a subpopulation of dopaminergic neurons exhibiting a 0.5-fold decrease in SV2C expression in the PD cases (Wang et al., 2024). This data aligns with Wu et al.’s (2024) discovery of reduced levels of SV2C in the plasma of PD patients and identified SV2C as a potential biomarker using machine learning algorithms (Wu et al., 2024). The convergence of these findings marks a promising direction for future research, particularly in validating SV2C as a biomarker and clarifying its role in the molecular cascade that characterizes PD progression.
To further investigate the role of SV2C in modulating synaptic vesicle dynamics, recent research found that SV2C promotes the uptake and retention of dopamine in the synaptic vesicles (Bucher et al., 2024). Mice treated with MPTP and deficient in SV2C showed increased vulnerability to the toxin’s effect, suggesting a neuroprotective role for SV2C, potentially by sequestering the toxic compounds from the cytosol. However, no transporter activity has been reported for SV2C till date. It is possible that SV2C mediates its effect of substrate uptake through VMAT2, a monoamine transporter that transports monoamine neurotransmitters into synaptic vesicles. It is intriguing to consider whether SV2C may have a similar effect on cytosolic dopamine, as high levels of cytosolic dopamine have been reported to be neurotoxic (Bucher et al., 2020; Mosharov et al., 2009).
6 Discussion and future directions
In summary, the literature reviewed herein underscores the potential significance of SV2C in the pathogenesis of PD, particularly in the context of synaptic vesicle trafficking, dopamine neurotransmission and α-synuclein homeostasis (Figure 1). The potential pathways modulated by SV2C are beginning to be charted, yet the mechanisms by which SV2C exert its influence in PD pathology remain elusive. The precise stage at which SV2C influences the synaptic trafficking pathway is also unclear. Furthermore, the phenotypic impact of SV2C SNPs identified in genetic studies may differ from those observed in knockout models, indicating a call for comprehensive investigations in both the function of SV2C and the effects of these SNPs.
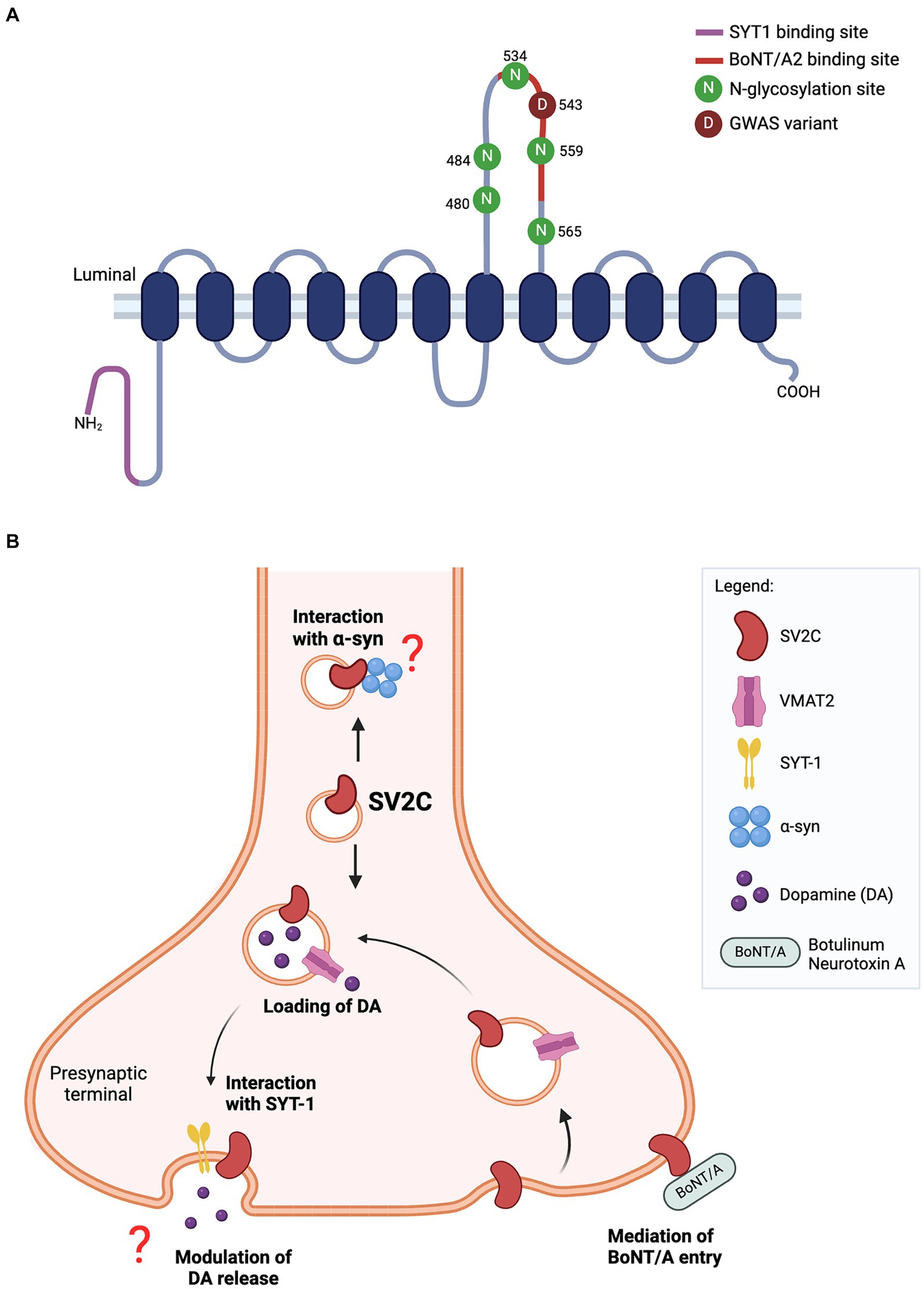
Figure 1. (A) Predicted structure of SV2C protein. The luminal domain contains five N-glycosylation sites while N-terminal tail harbors a binding site for synaptotamin-1 (SYT1). The missense variant (p.Asp543Asn) of SV2C reported in GWAS lies within the binding site for botulinum neurotoxin A2 (BoNT/A2). (B) Schematic of SV2C’s putative functions at the presynaptic terminal. SV2C is expressed on synaptic vesicles (SVs), where it may play a role in dopamine neurotransmission. SVs are loaded with dopamine through its transporter vesicular monoamine transporter 2 (VMAT2), and trafficked to the presynaptic membrane where dopamine is released through exocytosis. A key player mediating the exocytosis process is synaptotagmin-1 (SYT1) and SV2C has a unique binding site for SYT1. However, the functional significance of this interaction remains to be elucidated. Studies have reported the loss of SV2C decreased dopamine release, but the exact role of SV2C mediating this process is unclear. The highly-glycosylated luminal domain of SV2C faces the extracellular space and serves as a receptor for botulinum neurotoxin A (BoNT/A) entry into the neuron. Previous work also showed the direct interaction of SV2C with α-synuclein, details of which are yet to be fully understood (Schematics were built on BioRender).
The majority of functional studies on SV2C have utilized animal models such as Drosophila and mice. While these models are indispensable for disease modeling, differences in genetic backgrounds between species, the physiological differences in the manifestation of PD symptoms and the disparity in complexity between human and mouse brains may limit the efficacy of animal models in fully comprehending the disease’s intricacies and translating potential therapeutic discoveries. This prompts the need for research within human cellular models and advances in stem cell technology, especially the use of patient-derived induced pluripotent stem cells, now provide us the unique opportunity to utilize midbrain dopaminergic-like neurons as an in vitro disease modeling tool (Kirkeby et al., 2012; Kriks et al., 2011). Elucidating the mechanisms of PD pathogenesis modulated by SV2C in both human and animal models will be crucial for a comprehensive understanding of the disease.
Genetic studies have pinpointed SV2C as a risk factor for PD, uncovering polymorphisms within the SV2C gene associated with PD risk and PD subtypes (Table 1). While GWAS has identified numerous risk loci, the effect sizes of these SNPs are often modest, posing challenges for functional validation (Blauwendraat et al., 2020). Yet, with the advent of precise gene-editing tools such as Clustered Regularly Interspaced Short Palindromic Repeats (CRISPR), we can now model genetic SNPs with remarkable accuracy in cellular models. Given that the rs246814 SNP is linked with a missense variant in the luminal domain of SV2C, with the latter potentially introducing new N-glycosylation, its functional validation is imperative. This will not only shed light on the role of SV2C in PD pathogenesis but may also position SV2C as a biomarker, potentially facilitating the stratification of PD subtypes for clinical trials and intervention. Furthermore, a recent structural analysis conducted by UCB (Union Chimique Belge) on SV2 ligands has unveiled new insights into the structural details for drug development aimed at targeting SV2C (Li et al., 2024). Together, these insights lay the groundwork for novel therapeutic strategies targeting SV2C in the management of PD.
As we continue to unravel the complexities of SV2C, the prospect of more precise diagnostic tools and targeted treatment approaches for PD, tailored to the molecular signature of individual patients, becomes increasingly tangible. In essence, advancing our understanding of SV2C’s role in PD not only promises to improve patient outcomes but also serves as a critical step toward mastering one of the most challenging neurodegenerative disorders of our time.
Author contributions
CC: Data curation, Formal analysis, Investigation, Methodology, Visualization, Writing – original draft. KL: Conceptualization, Supervision, Writing – review & editing, Funding acquisition, Resources. JF: Conceptualization, Funding acquisition, Resources, Supervision, Writing – review & editing.
Funding
The author(s) declare that financial support was received for the research, authorship, and/or publication of this article. This work is supported by the Singapore Ministry of Health’s National Medical Research Council Open Fund Individual Research Grant (OF-IRG; MOH-000559), NMRC Open Fund—Large Collaborative Grant (OF-LCG; MOH-000207), the Singapore Ministry of Education Academic Research Fund Tier 2 (MOE-T2EP30220–0005) and Tier 3 (MOE-MOET32020-0004).
Acknowledgments
We thank Miao Cao, Yun Xia, George J Augustine and Elaine GY Chew for helpful discussions.
Conflict of interest
The authors declare that the research was conducted in the absence of any commercial or financial relationships that could be construed as a potential conflict of interest.
The author(s) declared that they were an editorial board member of Frontiers, at the time of submission. This had no impact on the peer review process and the final decision.
Publisher’s note
All claims expressed in this article are solely those of the authors and do not necessarily represent those of their affiliated organizations, or those of the publisher, the editors and the reviewers. Any product that may be evaluated in this article, or claim that may be made by its manufacturer, is not guaranteed or endorsed by the publisher.
References
Altmann, V., Schumacher-Schuh, A. F., Rieck, M., Callegari-Jacques, S. M., Rieder, C. R. M., and Hutz, M. H. (2016). Influence of genetic, biological and pharmacological factors on levodopa dose in Parkinson’s disease. Pharmacogenomics 17, 481–488. doi: 10.2217/pgs.15.183
Bajjalieh, S. M., Frantz, G. D., Weimann, J. M., McConnell, S. K., and Scheller, R. H. (1994). Differential expression of synaptic vesicle protein 2 (SV2) isoforms. J. Neurosci. 14:5223. doi: 10.1523/JNEUROSCI.14-09-05223.1994
Bartholome, O., Van den Ackerveken, P., Sánchez Gil, J., de la Brassinne Bonardeaux, O., Leprince, P., Franzen, R., et al. (2017). Puzzling out synaptic vesicle 2 family members functions. Front. Mol. Neurosci. 10:148. doi: 10.3389/fnmol.2017.00148
Blauwendraat, C., Nalls, M. A., and Singleton, A. B. (2020). The genetic architecture of Parkinson’s disease. Lancet Neurol. 19, 170–178. doi: 10.1016/S1474-4422(19)30287-X
Braak, H., Tredici, K.Del, Rüb, U., de Vos, R. A. I., Jansen Steur, E. N. H., and Braak, E. (2003). Staging of brain pathology related to sporadic Parkinson’s disease. Neurobiol. Aging, 24, 197–211. doi: 10.1016/s0197-4580(02)00065-9
Bucher, M. L., Barrett, C. W., Moon, C. J., Mortimer, A. D., Burton, E. A., Greenamyre, J. T., et al. (2020). Acquired dysregulation of dopamine homeostasis reproduces features of Parkinson’s disease. NPJ Parkinsons Dis. 6:34. doi: 10.1038/s41531-020-00134-x
Bucher, M. L., Dunn, A. R., Bradner, J. M., Egerton, K. S., Burkett, J. P., Johnson, M. A., et al. (2024). Synaptic vesicle glycoprotein 2C enhances vesicular storage of dopamine and counters dopaminergic toxicity. Eur. J. Neurosci. 59, 2483–2501. doi: 10.1111/ejn.16311
Calabresi, P., Mechelli, A., Natale, G., Volpicelli-Daley, L., Di Lazzaro, G., and Ghiglieri, V. (2023). Alpha-synuclein in Parkinson’s disease and other synucleinopathies: from overt neurodegeneration back to early synaptic dysfunction. Cell Death Dis. 14:176. doi: 10.1038/s41419-023-05672-9
Chang, W.-P., and Südhof, T. C. (2009). SV2 renders primed synaptic vesicles competent for ca<sup>2+</sup>-induced exocytosis. J. Neurosci. 29, 883–897. doi: 10.1523/JNEUROSCI.4521-08.2009
Ciruelas, K., Marcotulli, D., and Bajjalieh, S. M. (2019). Synaptic vesicle protein 2: a multi-faceted regulator of secretion. Semin. Cell Dev. Biol. 95, 130–141. doi: 10.1016/j.semcdb.2019.02.003
Crowder, K. M., Gunther, J. M., Jones, T. A., Hale, B. D., Zhang, H. Z., Peterson, M. R., et al. (1999). Abnormal neurotransmission in mice lacking synaptic vesicle protein 2A (SV2A). Proc. Natl. Acad. Sci. 96, 15268–15273. doi: 10.1073/pnas.96.26.15268
Dardou, D., Dassesse, D., Cuvelier, L., Deprez, T., De Ryck, M., and Schiffmann, S. N. (2011). Distribution of SV2C mRNA and protein expression in the mouse brain with a particular emphasis on the basal ganglia system. Brain Res. 1367, 130–145. doi: 10.1016/j.brainres.2010.09.063
Dardou, D., Monlezun, S., Foerch, P., Courade, J.-P., Cuvelier, L., De Ryck, M., et al. (2013). A role for Sv2c in basal ganglia functions. Brain Res. 1507, 61–73. doi: 10.1016/j.brainres.2013.02.041
Deng, X., Saffari, S. E., Liu, N., Xiao, B., Allen, J. C., Ng, S. Y. E., et al. (2022). Biomarker characterization of clinical subtypes of Parkinson disease. NPJ Parkinson’s Dis. 8:109. doi: 10.1038/s41531-022-00375-y
Detrait, E., Maurice, T., Hanon, E., Leclercq, K., and Lamberty, Y. (2014). Lack of synaptic vesicle protein SV2B protects against amyloid-β25–35-induced oxidative stress, cholinergic deficit and cognitive impairment in mice. Behav. Brain Res. 271, 277–285. doi: 10.1016/j.bbr.2014.06.013
Dong, M., Yeh, F., Tepp, W. H., Dean, C., Johnson, E. A., Janz, R., et al. (2006). SV2 is the protein receptor for botulinum neurotoxin a. Science 312, 592–596. doi: 10.1126/science.1123654
Dunn, A. R., Hoffman, C. A., Stout, K. A., Ozawa, M., Dhamsania, R. K., and Miller, G. W. (2019). Immunochemical analysis of the expression of SV2C in mouse, macaque and human brain. Brain Res. 1702, 85–95. doi: 10.1016/j.brainres.2017.12.029
Dunn, A. R., Stout, K. A., Ozawa, M., Lohr, K. M., Hoffman, C. A., Bernstein, A. I., et al. (2017). Synaptic vesicle glycoprotein 2C (SV2C) modulates dopamine release and is disrupted in Parkinson disease. Proc. Natl. Acad. Sci. 114:E2253 LP-E2262. doi: 10.1073/pnas.1616892114
Foo, J. N., Chew, E. G. Y., Chung, S. J., Peng, R., Blauwendraat, C., Nalls, M. A., et al. (2020). Identification of risk loci for Parkinson disease in Asians and comparison of risk between Asians and Europeans: a genome-wide association study. JAMA Neurol. 77, 746–754. doi: 10.1001/jamaneurol.2020.0428
Grover, S., Kumar-Sreelatha, A. A., Bobbili, D. R., May, P., Domenighetti, C., Sugier, P.-E., et al. (2021). Replication of a novel Parkinson’s locus in a European ancestry population. Mov. Disord. 36, 1689–1695. doi: 10.1002/mds.28546
Haucke, V., and De Camilli, P. (1999). AP-2 recruitment to Synaptotagmin stimulated by tyrosine-based endocytic motifs. Science, 285, 1268–1271. doi: 10.1126/science.285.5431.1268
Hill-Burns, E. M., Singh, N., Ganguly, P., Hamza, T. H., Montimurro, J., Kay, D. M., et al. (2013). A genetic basis for the variable effect of smoking/nicotine on Parkinson’s disease. Pharmacogenomics J. 13, 530–537. doi: 10.1038/tpj.2012.38
Hu, Y.-W., Xiao, L., Zheng, L., and Wang, Q. (2017). Synaptic vesicle 2C and its synaptic-related function. Clin. Chim. Acta 472, 112–117. doi: 10.1016/j.cca.2017.07.029
Iezzi, M., Theander, S., Janz, R., Loze, C., and Wollheim, C. B. (2005). SV2A and SV2C are not vesicular Ca2+ transporters but control glucose-evoked granule recruitment. J. Cell Sci. 118, 5647–5660. doi: 10.1242/jcs.02658
Janz, R., and Südhof, T. C. (1999). SV2C is a synaptic vesicle protein with an unusually restricted localization: anatomy of a synaptic vesicle protein family. Neuroscience 94, 1279–1290. doi: 10.1016/S0306-4522(99)00370-X
Kirkeby, A., Grealish, S., Wolf, D. A., Nelander, J., Wood, J., Lundblad, M., et al. (2012). Generation of regionally specified neural progenitors and functional neurons from human embryonic stem cells under defined conditions. Cell Rep. 1, 703–714. doi: 10.1016/j.celrep.2012.04.009
Kriks, S., Shim, J.-W., Piao, J., Ganat, Y. M., Wakeman, D. R., Xie, Z., et al. (2011). Dopamine neurons derived from human ES cells efficiently engraft in animal models of Parkinson’s disease. Nature 480, 547–551. doi: 10.1038/nature10648
Lepeta, K., Lourenco, M. V., Schweitzer, B. C., Martino Adami, P. V., Banerjee, P., Catuara-Solarz, S., et al. (2016). Synaptopathies: synaptic dysfunction in neurological disorders – a review from students to students. J. Neurochem. 138, 785–805. doi: 10.1111/jnc.13713
Li, J., Zou, R., Varrone, A., Nag, S., Halldin, C., and Ågren, H. (2024). Exploring the interactions between two ligands, UCB-J and UCB-F, and synaptic vesicle glycoprotein 2 isoforms. ACS Chem. Neurosci. 15, 2018–2027. doi: 10.1021/acschemneuro.4c00029
Li, X., Li, W., Liu, G., Shen, X., and Tang, Y. (2015). Association between cigarette smoking and Parkinson’s disease: a meta-analysis. Arch. Gerontol. Geriatr. 61, 510–516. doi: 10.1016/j.archger.2015.08.004
Lynch, B. A., Lambeng, N., Nocka, K., Kensel-Hammes, P., Bajjalieh, S. M., Matagne, A., et al. (2004). The synaptic vesicle protein SV2A is the binding site for the antiepileptic drug levetiracetam. Proc. Natl. Acad. Sci. 101, 9861–9866. doi: 10.1073/pnas.0308208101
Mosharov, E. V., Larsen, K. E., Kanter, E., Phillips, K. A., Wilson, K., Schmitz, Y., et al. (2009). Interplay between cytosolic dopamine, calcium, and α-Synuclein causes selective death of substantia Nigra neurons. Neuron 62, 218–229. doi: 10.1016/j.neuron.2009.01.033
Nowack, A., Yao, J., Custer, K. L., and Bajjalieh, S. M. (2010). SV2 regulates neurotransmitter release via multiple mechanisms. Am. J. Phys. Cell Phys. 299, C960–C967. doi: 10.1152/ajpcell.00259.2010
Olsen, A. L., Clemens, S. G., and Feany, M. B. (2023). Nicotine-mediated rescue of α-Synuclein toxicity requires synaptic vesicle glycoprotein 2 in Drosophila. Mov. Disord. 38, 244–255. doi: 10.1002/mds.29283
Quik, M., O’Leary, K., and Tanner, C. M. (2008). Nicotine and Parkinson’s disease: implications for therapy. Mov. Disord. 23, 1641–1652. doi: 10.1002/mds.21900
Redenšek, S., Flisar, D., Kojović, M., Gregorič Kramberger, M., Georgiev, D., Pirtošek, Z., et al. (2019). Dopaminergic pathway genes influence adverse events related to dopaminergic treatment in Parkinson’s disease. Front. Pharmacol. 10. doi: 10.3389/fphar.2019.00008
Schivell, A. E., Mochida, S., Kensel-Hammes, P., Custer, K. L., and Bajjalieh, S. M. (2005). SV2A and SV2C contain a unique synaptotagmin-binding site. Mol. Cell. Neurosci. 29, 56–64. doi: 10.1016/j.mcn.2004.12.011
Soukup, S., Vanhauwaert, R., and Verstreken, P. (2018). Parkinson’s disease: convergence on synaptic homeostasis. EMBO J. 37:e98960. doi: 10.15252/embj.201898960
Stout, K. A., Dunn, A. R., Hoffman, C., and Miller, G. W. (2019). The synaptic vesicle glycoprotein 2: structure, function, and disease relevance. ACS Chem. Neurosci. 10, 3927–3938. doi: 10.1021/acschemneuro.9b00351
Tan, Z., Lin, Y., Zhou, M., Guo, W., Qiu, J., Ding, L., et al. (2023). Correlation of SV2C rs1423099 single nucleotide polymorphism with sporadic Parkinson’s disease in Han population in southern China. Neurosci. Lett. 813:137426. doi: 10.1016/j.neulet.2023.137426
Turner, T. J. (2004). Nicotine enhancement of dopamine release by a calcium-dependent increase in the size of the readily releasable Pool of synaptic vesicles. J. Neurosci. 24, 11328–11336. doi: 10.1523/JNEUROSCI.1559-04.2004
Wang, Q., Wang, M., Choi, I., Sarrafha, L., Liang, M., Ho, L., et al. (2024). Molecular profiling of human substantia nigra identifies diverse neuron types associated with vulnerability in Parkinson’s disease. Science. Advances 10:eadi8287. doi: 10.1126/sciadv.adi8287
Wu, J., Wu, W., Jiang, P., Xu, Y., and Yu, M. (2024). Identification of SV2C and DENR as key biomarkers for Parkinson’s disease based on bioinformatics, machine learning, and experimental verification. J. Mol. Neurosci. 74:6. doi: 10.1007/s12031-023-02182-3
Keywords: synaptic vesicle, Parkinson’s disease, dopamine, synaptic trafficking, Synaptic Vesicle Glycoprotein
Citation: Chang CH, Lim KL and Foo JN (2024) Synaptic Vesicle Glycoprotein 2C: a role in Parkinson’s disease. Front. Cell. Neurosci. 18:1437144. doi: 10.3389/fncel.2024.1437144
Edited by:
David Matuskey, Yale University, United StatesReviewed by:
Amy R. Dunn, Jackson Laboratory, United StatesSandy Bajjalieh, University of Washington, United States
Gary W. Miller, Columbia University, United States
Copyright © 2024 Chang, Lim and Foo. This is an open-access article distributed under the terms of the Creative Commons Attribution License (CC BY). The use, distribution or reproduction in other forums is permitted, provided the original author(s) and the copyright owner(s) are credited and that the original publication in this journal is cited, in accordance with accepted academic practice. No use, distribution or reproduction is permitted which does not comply with these terms.
*Correspondence: Jia Nee Foo, amlhbmVlLmZvb0BudHUuZWR1LnNn