- 1Elasmobranch Institute Indonesia, Denpasar, Bali, Indonesia
- 2Sahul Papua Program, Konservasi Indonesia, Sorong, Papua Barat Daya, Indonesia
- 3Focal Species Conservation Program, Konservasi Indonesia, Jakarta, Indonesia
- 4Badan Layanan Umum Daerah Unit Pelaksana Teknis Daerah (BLUD UPTD) Pengelolaan Kawasan Konservasi Perairan (KKP) Kepulauan Raja Ampat, Waisai, Papua Barat Daya, Indonesia
- 5Harry Butler Institute, Murdoch University, Perth, WA, Australia
- 6Conservation International Aotearoa, The University of Auckland, Auckland, New Zealand
- 7Institute of Marine Science, The University of Auckland, Auckland, New Zealand
The globally endangered oceanic manta ray Mobula birostris is believed to spend significantly more time in the open ocean than in coastal areas. Nonetheless, the Bird's Head Seascape (BHS) in eastern Indonesia hosts a large population of this species (over 850 individuals), frequently sighted at coral reef cleaning stations and forming a vital asset for marine tourism in the region. Despite this, detailed understanding of the movements and habitat use of this wide-ranging species within shallow (<30 m) coral reef ecosystems remains limited. Addressing this knowledge gap is urgently required for the sustainable management of oceanic manta ray-focused tourism in the region. Here, we report the results of an initial passive acoustic telemetry study investigating the use of coral reef cleaning stations by oceanic manta rays. Forty-one rays were acoustically tracked from February 2016 to August 2019 in an array of 28 acoustic receivers deployed at known cleaning stations and hypothesized transit points across the BHS (including the regions of Raja Ampat, Fakfak, and Kaimana). A total of 5,822 detections were recorded by 16 of the 28 receivers from all tagged individuals, and 421 visitation events were documented from 37 out of the 41 tagged individuals, providing valuable insights into their visitation patterns at cleaning stations. Tagged individuals were detected at receiver stations for durations ranging from 0.2 to 427.0 min (mean ± SD = 48 ± 64 min). Notably, 94% of detections were recorded during daylight hours (reaching a peak at noon), underscoring an apparent diurnal pattern in time spent around cleaning stations. The study documented frequent short-range movements between adjacent receivers (5–12 km apart), with two-thirds of all 191 tracked movements occurring between neighboring stations in southern Raja Ampat. Notably, tagged individuals were detected repeatedly at three cleaning stations which were previously only known for hosting reef manta rays, suggesting oceanic manta rays likely also utilize these cleaning stations. Our study provides important insights into the visitations and local movements of oceanic manta rays between coral reef cleaning stations, which will inform effective oceanic manta ray conservation efforts and tourism management in the BHS.
1 Introduction
A comprehensive understanding of the movements and habitat use of marine megafauna is critical to the success of efforts to conserve and manage them (1, 2). The oceanic manta ray Mobula birostris (Walbaum, 1792), which was recently uplisted to Endangered (EN) on the IUCN Red List of Threatened Species, is distributed circumglobally in tropical to temperate waters (3). This wide-ranging species is considered highly migratory and is known to undertake long-distance movements of up to 1,500 km in the eastern tropical Pacific (4) and southwestern Pacific (5). Despite this, a multidisciplinary study using satellite telemetry, genetic analysis, and stable isotopes suggested that at three sites in the Indo-Pacific (Sri Lanka, Mexico, and Raja Ampat in Indonesia), this species exhibits restricted movements and fine-scale population structure (6).
The majority of studies on the movement ecology of manta rays, particularly those utilizing satellite and acoustic telemetry, have focused on the reef manta ray (M. alfredi), which was only removed from taxonomic synonymy with M. birostris in 2009 (7). The oceanic manta ray tends to spend more time in oceanic environments, including offshore islands and seamounts (8, 9), making telemetry studies more logistically challenging. Although oceanic manta rays are generally thought to have less reliance on shallow coastal ecosystems than reef manta rays, both species are known to use coral reef cleaning stations (10–13), in some cases in full microsympatry (14). At cleaning stations, manta rays hover over reef patches and interact with cleaner fish species (e.g., Chaetodon kleinii, Thalassoma lunare, Labroides bicolor, and L. dimidiatus) that feed not only on ectoparasites on their bodies but may also feed on their mucus and skin, particularly around wounds (15–17). Most studies on the use of manta ray cleaning stations have focused on reef manta rays, which are known to frequent cleaning stations for a number of purposes, including removing parasites from their bodies and for social/courtship interactions to seek mating opportunities (10, 18, 19).
The Bird's Head Seascape (BHS) in West Papua (eastern Indonesia) is home to large populations of both manta ray species (11, 14), with aggregation sites distributed throughout the seascape. The majority of the known manta ray sites are located within the Raja Ampat archipelago (14), which is also known as the global epicenter of tropical marine biodiversity (20–22). Given these factors, marine tourism in the BHS has increased significantly in the last two decades (23). In Raja Ampat, the high abundance and frequent aggregations of manta rays, particularly at cleaning stations, is a major attraction for most diving tourists vising the region (24, 25). Despite the value of manta ray-based tourism being one of the primary factors leading to the protection of manta rays in Raja Ampat, controlling the rapid growth of tourism has also become one of the key challenges in manta ray conservation and management in the region (25).
Passive acoustic telemetry has been widely used across various regions to study the residency, movement patterns, and site fidelity of various marine megafauna, including hawksbill turtles Eretmochelys imbricata (26), tiger sharks Galeocerdo cuvier (27), and whale sharks Rhincodon typus (28). To date, the majority of acoustic telemetry studies on manta rays have focused on reef manta rays [e.g., (29–31)], with only one study investigating oceanic manta rays (32).
While extensive studies have been conducted on the population dynamics and movement of manta rays within the BHS, particularly in the Raja Ampat region (33), only two studies have specifically targeted oceanic manta rays. These studies have employed photographic identification (11) and satellite telemetry (6) to investigate population structure and spatial movements, respectively. There is a need for more detailed knowledge of the utilization of cleaning stations by oceanic manta rays to better understand the ecological services provided by shallow coastal ecosystems for this species. In Mexico, Domínguez-Sánchez et al. (32) demonstrated the effectiveness of passive acoustic telemetry in tracking the occupancy of oceanic manta rays. In Raja Ampat, oceanic manta rays are frequently observed aggregating in groups at cleaning stations on coral reefs and photo-identified individuals exhibited a robust resighting rate of 28% (11, 14). Given these considerations, we hypothesized that passive acoustic telemetry could enable us to monitor the presence and movements of oceanic manta rays throughout the Bird's Head Seascape in eastern Indonesia, especially at cleaning stations.
The primary aim of this study was to elucidate the residency and site fidelity of oceanic manta rays at cleaning stations situated on coral reefs, utilizing passive acoustic telemetry. Furthermore, we explored the movement patterns of oceanic manta rays and assessed the spatial connectivity among these cleaning stations. The objective was to generate insights to guide the development of effective management strategies for oceanic manta ray-focused tourism within the Raja Ampat region and the broader BHS.
2 Materials and methods
2.1 Study region
The Bird's Head Seascape (BHS) in eastern Indonesia, recognized as an important tropical marine biodiversity hotspot, is safeguarded by 26 marine protected areas (MPAs) (25). This region is home to large populations of both manta ray species, which are reported to be in a healthy state (11, 34). Importantly, the seascape encompasses over 100 manta ray sites (e.g., cleaning stations and feeding areas), where both species have been observed in varying size of aggregations or as solitary individuals (14). In the BHS, our research specifically targeted three regions: Raja Ampat, Fakfak, and Kaimana (Figure 1), where oceanic manta rays have been frequently sighted in aggregations, primarily at cleaning stations on coral reefs, or occasionally observed in the open sea (14).
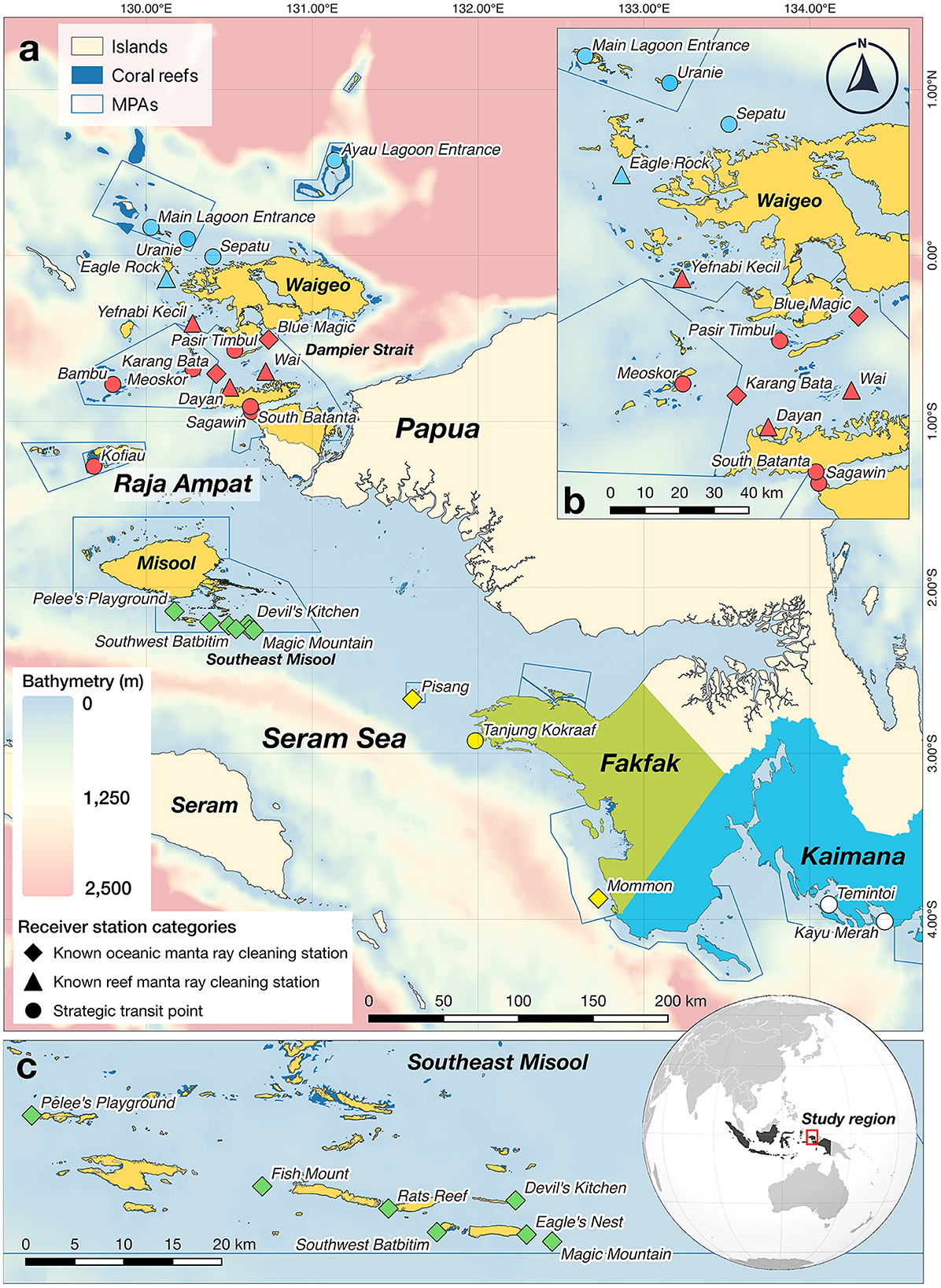
Figure 1. Locations of 28 VR2W acoustic receivers deployed in Raja Ampat, Fakfak, and Kaimana regions in the Bird's Head Seascape, eastern Indonesia. (a) Bird's Head Seascape, including three study regions: Fakfak (in green); Kaimana (in blue), and Raja Ampat (in yellow), which consisted of three subregions: northern (NRA), central (CRA), and southern Raja Ampat (SRA). Receiver locations are indicated by blue symbols in NRA, red symbols in CRA, green symbols in SRA, yellow symbols in Fakfak, and white symbols in Kaimana. Diamond symbols indicate receiver deployed at a known oceanic manta ray cleaning station; triangular symbols indicate receiver deployed at a known reef manta ray cleaning station with no prior observation of oceanic manta rays, and circular symbols indicate receiver deployed at a strategic transit point for manta rays. Blue polygons denote marine protected areas (MPAs) within the BHS MPA network; (b) Expanded view of 13 acoustic receiver stations in NRA and CRA; and (c) Expanded view of seven acoustic receiver stations in SRA.
2.2 Passive acoustic telemetry
2.2.1 Acoustic receiver deployments
Passive acoustic telemetry involves three main components: the target animals, acoustic receivers, and acoustic transmitters (35). For this study, we utilized an array of acoustic receivers that was already in place for a previous acoustic telemetry study on Raja Ampat reef manta rays (30), and augmented the array by deploying additional acoustic receivers in the Fakfak and Kaimana regions, as well as at two known oceanic manta ray cleaning stations in Raja Ampat. In total, the acoustic array for this study was comprised of 28 VR2W acoustic receivers (Innovasea, Halifax, Canada) deployed across three regions in the BHS: Fakfak, Kaimana, and Raja Ampat (Figure 1). For the purposes of analysis, we further subdivided the large Raja Ampat archipelago into three subregions of northern Raja Ampat (NRA), central Raja Ampat (CRA), and southern Raja Ampat (SRA; Figure 1). The acoustic receivers were divided across (sub)regions as follows: NRA (n = 5); CRA (n = 11); SRA (n = 7); Fakfak (FAK; n = 3); and Kaimana (KAI; n = 2). Importantly, the receivers were deployed at all 11 known oceanic manta ray cleaning stations in the BHS (nine of which also function as cleaning stations for reef manta rays), as well as at four reef manta ray cleaning stations (but where oceanic manta rays had not been previously observed) and at 13 strategic transit points where manta rays (especially reef manta rays) had been frequently observed swimming past prominent geological features, such as promontories or reef points exposed to strong currents.
The 28 acoustic receivers were deployed at depths ranging from 10 to 40 m and operated throughout the study period from February 2016 to August 2019 (~3.5 years), adhering to the methodologies detailed by Setyawan et al. (30) and Setyawan et al. (36). Each of these acoustic receivers, deployed ~2 m above the substrate, was secured with cable ties to a buoyed mooring that was attached to the substrate with mooring lines. The 15 receivers deployed at known manta ray cleaning stations were each strategically placed within 20 m of the actual cleaning station. The acoustic receivers were periodically cleaned to remove any fouling and the acoustic data were downloaded every 6 months. The batteries of each receiver, which had an approximate lifespan of 15 months, were replaced every 12 months. To ensure the acoustic transmitters were detectable by the receivers within a practical range, a range test was conducted, revealing an estimated detection radius of 150 m, as reported by Setyawan et al. (30). Technically, a transmitter was positioned in the water alongside a drifting boat, carried by the tidal current. A handheld GPS was used to record the timestamp and location of the boat. This data was then synchronized with detections recorded by the receiver in Yefnabi Kecil (Figure 1), and the distance to the receiver from the last recorded detection was measured.
2.2.2 Acoustic transmitter deployments
To monitor the movement and residency of oceanic manta rays, 41 individuals were equipped with Innovasea V16 acoustic transmitters, which have an expected battery life of 1,358 days. The deployment of these transmitters followed methodologies from previous studies that utilized the same type of acoustic transmitters in this region (30, 36). Each transmitter, coated with ablative silicone-based coating Propspeed™ to prevent marine fouling and attached to a titanium dart tip with a 12-cm stainless steel tether, was deployed while SCUBA diving using a pole spear to insert the dart tip into the dorsal musculature between one pectoral fin and the body cavity of the animal. The transmitters were deployed on manta rays encountered at six oceanic manta ray cleaning stations: Karang Bata (n = 6) and Blue Magic (n = 3) in CRA; Magic Mountain (n = 22), Eagle's Nest (n = 7); and Devil's Kitchen (n = 2) in SRA; and Mommon seamount (n = 1) in Fakfak region (Supplementary Table 1).
Before the deployment of transmitters, identification photos were taken of each individual when feasible, the sex of the individuals was determined, and their disc width (DW) was visually estimated (14). Sex determination relied on the presence (in males) or absence (in females) of claspers. Mature males were identified by large, calcified claspers protruding beyond the posterior edge of the pelvic fins, while mature females were recognized by the presence of mating scars or a pregnancy bulge (10).
2.3 Data analyses
2.3.1 Visitations and movements of oceanic manta rays
Acoustic detection data from all tagged oceanic manta rays were downloaded and extracted using VUE software (Innovasea, Halifax, Canada) for each acoustic receiver. The dataset included transmitter IDs, timestamps of detections, and metadata from the acoustic receivers (e.g., site name), along with transmitter metadata (i.e., deployment time and location, as well as sex and estimated disc width (DW) of the animals) and additional receiver metadata (e.g., geographic coordinates).
The data were subsequently imported into the R statistical environment (37) for further processing, which involved formatting, filtering, and analysis. To ensure accuracy, the acoustic detection data were filtered to exclude false-positive detections, retaining only those from transmitters deployed for this study. Detections logged by receivers before transmitter deployments were also removed. To assess differences in the hourly mean number of acoustic detections between daytime (06.00–18.00) and night-time (18.00–06.00) across receiver stations recording acoustic detections, we first conducted a Shapiro-Wilk normality test to evaluate the distribution of the data, followed by an F-test to assess the homogeneity of variances. Subsequently, an unpaired two-sample Wilcoxon test was applied to determine statistical significances.
To analyze the visitations and movements of acoustically tagged oceanic manta rays at receiver stations, the filtered data were processed using the RunResidenceExtraction function from the “VTrack” R package version 2.11 (38). From this analysis, two metrics were extracted: residence events (visitation events) and non-residence events (movement events). A visitation event, or visit, was characterized by the detectability of an acoustically tagged oceanic manta ray within the detection range of an acoustic receiver at a receiver station. This event commenced when at least two acoustic detections from a tagged oceanic manta ray were recorded by a receiver, concluding when the ray was not detected by the receiver for a duration of 60 min, or if it was detected at another receiver station. Conversely, a movement event was identified by the detectability of an acoustically tagged oceanic manta ray at two acoustic receivers positioned at different sites, occurring when a tagged ray was detected at one receiver station and subsequently detected at another.
To evaluate the level of visitation by each acoustically tagged oceanic manta ray to and around receiver stations, the visitation index (VI), also commonly known as the Residency Index [e.g., (36)], was calculated using the following formula:
VI (in %) provides a quantitative measure of how frequently a tagged oceanic manta ray visited areas in the vicinity of the receiver stations, offering insights into their residency patterns within the study area. In our study, we opted not to use the term “Residency Index,” as this metric typically measures the residency of animals within a specific area. Given the wide-ranging, oceanic nature of the species in question, we deemed the term inappropriate for describing their patterns of movement. Instead, we used the term VI to describe this metric, as the oceanic manta rays are more appropriately described as visiting the area rather than residing within it.
We, moreover, used a Detection Index (DI; in %) to measure the detectability level and relative prevalence of each acoustic receiver within our array in recording detections from the tagged oceanic manta rays. This index indicated the frequency of use for each acoustic receiver by the tagged oceanic manta rays, showing how often it recorded detections compared to others in the array. It was calculated using the following formula as detailed in Setyawan et al. (36):
The “active monitoring period” of each receiver was defined as the timeframe from the initial deployment date of the receiver until 31 August 2019, or until the receiver was retrieved, if earlier. Importantly, this period excludes any durations when receivers at sites were inactive. Due to logistical challenges, such as replacing stolen or damaged receivers in remote locations, the deployment periods of acoustic receivers varied between sites, resulting in intermittent gaps in the monitoring periods (Supplementary Figure 1).
2.3.2 Movement network of oceanic manta rays
To analyze the movements and spatial connectivity among receiver stations of acoustically tracked oceanic manta rays, we constructed a movement network and visualized it using the “igraph” R package (39). The network comprised nodes, representing sites where VR2W receivers were deployed (receiver stations), and edges, indicating the movements of the tagged oceanic manta rays recorded between these sites (40). Edges were weighted according to the proportion of movements recorded during the tracking period, following the approach outlined by Setyawan et al. (31).
To elucidate the network's structure, we calculated metrics at two levels: network level and node level, as described in Setyawan et al. (31). Network level metrics assessed the connectivity pattern among nodes and edges (41). These metrics included seven measures: (1) the total number of nodes within the network, (2) the number of connected nodes, (3) the number of edges between two nodes, (4) the number of oceanic manta ray movements between two nodes, (5) average path length (APL), (6) edge density, and (7) network diameter. Edge density refers to the proportion of actual edges out of the total possible edges in the network (42), APL represents the average length of the shortest paths connecting all nodes in the network (41), and network diameter is the longest path between any two nodes, indicating the size of the network (43). Node level metrics, which are also known as centrality measures, detailed the relative importance and influence of individual nodes within the network's overall structure (44). These included: (1) in-degree centrality, (2) out-degree centrality, (3) degree centrality, (4) betweenness, (5) closeness, and (6) eigenvector, following Setyawan et al. (31). The in-degree and out-degree centrality represents the number of neighboring nodes connected by incoming and outgoing edges, respectively (45). Degree centrality depicts the number of nodes connected to a node, which include both the in-degree and out-degree (46). Betweenness indicates the number of shortest paths passing through a node, reflecting the extent to which a receiver station was involved in the movements of oceanic manta rays (47). Closeness measures the average distance from a node to others, indicating its centrality within the network. Eigenvector centrality assesses a node's importance by considering the centrality of nodes it is connected to (46). Both network and node level metrics were calculated using functions available in the “igraph” package.
3 Results
3.1 Passive acoustic tracking
The 41 tagged oceanic manta rays comprised 12 males (29%) and 29 females (71%; Supplementary Table 1), all of which were adults. The estimated DW of these individuals ranged from 3.2 to 5.5 m (mean ± SD = 4.0 ± 0.4). Females (mean ± SD = 4.1 ± 0.4 m) were slightly larger than males (mean ± SD = 3.8 ± 0.4 m). Between February 2016 and August 2019, a total of 5,822 acoustic detections were recorded by 16 of the 28 receivers deployed across the Bird's Head Seascape (BHS). These included 11 of the receivers deployed at known oceanic manta ray cleaning stations, three receivers deployed at known reef manta ray cleaning stations, and two receivers deployed at strategic transit points (Figure 1). The majority of these detections (93.5%) occurred at four sites (Magic Mountain, Eagle's Nest, Devil's Kitchen, Southwest Batbitim) around southeast Misool in the southern Raja Ampat (SRA) and at one site (Blue Magic) in the central Raja Ampat (CRA; Table 1, Figure 1). All the 41 deployed transmitters were successfully detected by the receiver array (Supplementary Table 1) and the number of days each of these oceanic manta rays was detected ranged from 1 to 47 days (mean ± SD: 10 ± 9). The tracking periods for the tagged manta rays ranged from 1 to 1,169 days (mean ± SD: 281 ± 299). Notably, 11 individuals (~27% of all tagged individuals) had transmitter retention times of at least 1 year, including two individuals tracked for more than 1,000 days (Supplementary Table 1).
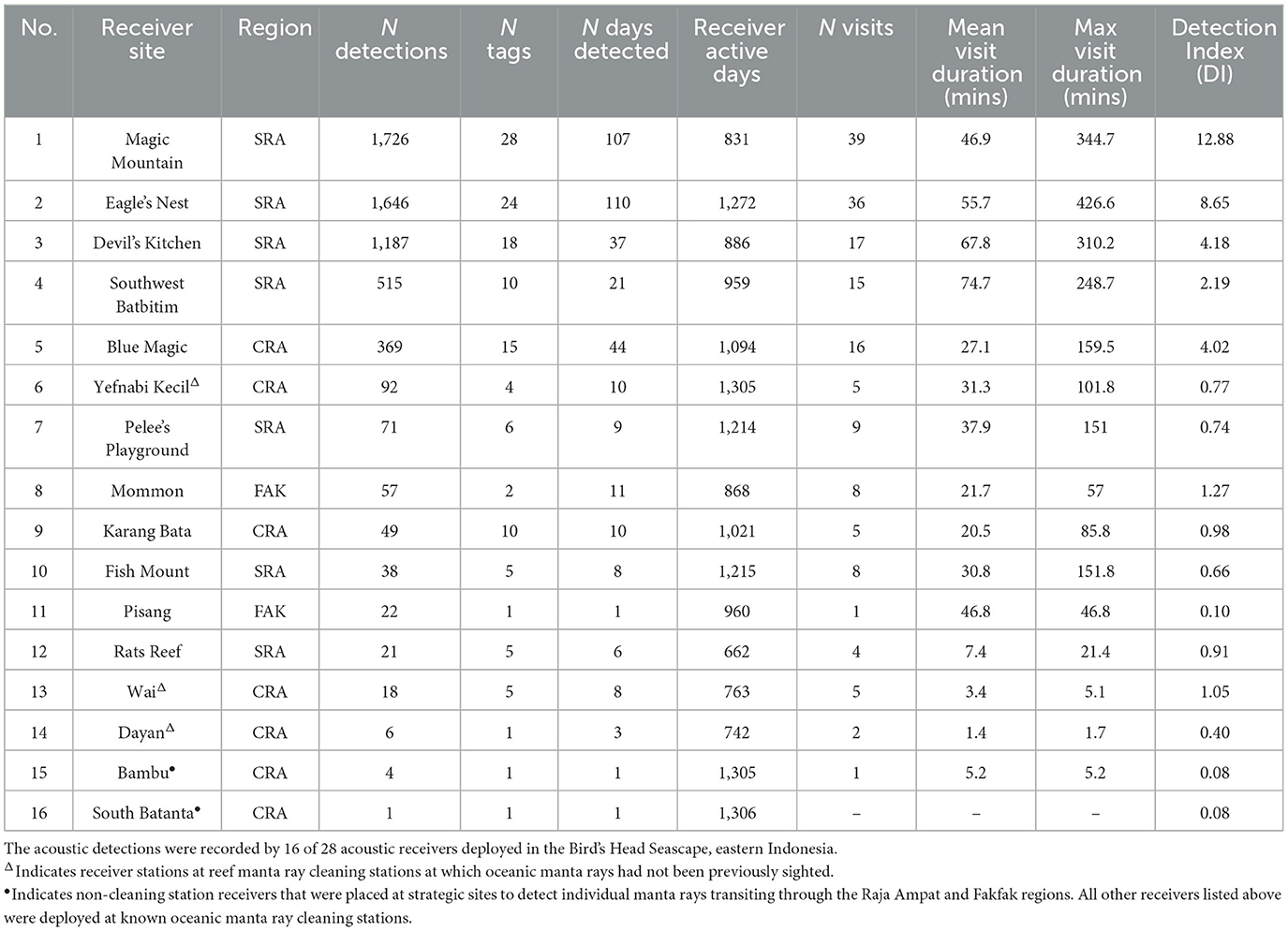
Table 1. Summary of acoustic detections and visitation by tagged oceanic manta rays recorded between February 2016 and August 2019.
3.2 Visitations and site fidelity to cleaning stations
The analysis of visitation events made by the tagged oceanic manta rays resulted in a total of 421 visitation events from 37 of the 41 tagged individuals (Supplementary Table 1). It also revealed a wide range of visitation durations at receiver stations, from as brief as 0.2 min to as long as 426.6 min (~7 h; Figure 2), with an average visitation duration of 48.1 ± 63.9 min. That longest single visitation was recorded from an individual ID#21575 at Eagle's Nest in May 2019. On an individual basis, the tagged oceanic manta rays visited receiver stations up to 50 times (by a male individual ID#55478), averaging 11 ± 11 visits, and were detected by as many as nine different receiver stations with an average of three stations (SD = 2; Supplementary Table 1). The mean duration of these visitations to receiver stations by each individual varied significantly, from 5.2 min (by ID#55469) to 121.1 min (by ID#55482; Supplementary Table 1).
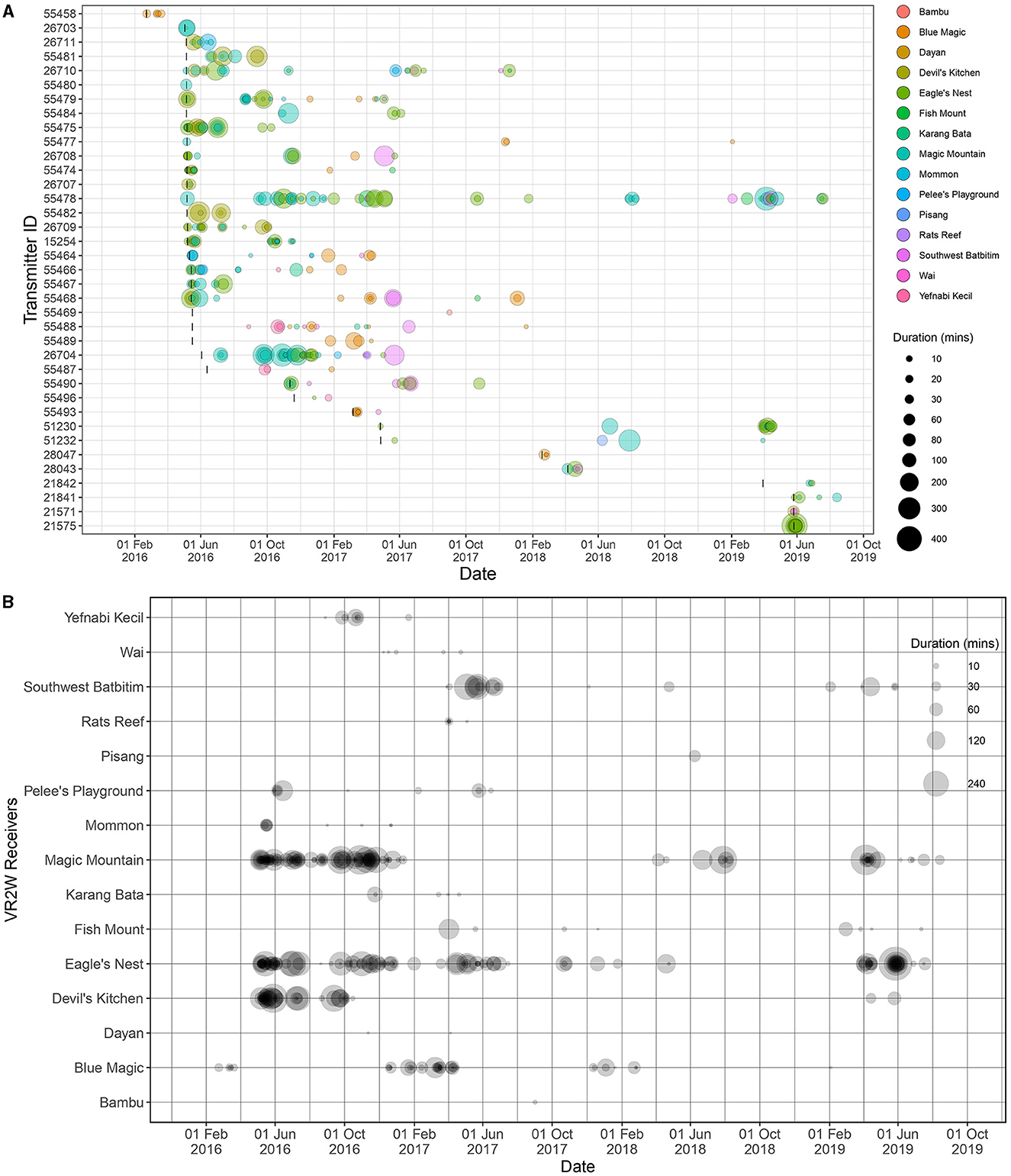
Figure 2. Visitation events of each tagged oceanic manta rays (n = 37) recorded at 15 of 28 receivers deployed across the BHS between February 2016 and August 2019. South Batanta receiver station was excluded from this figure given only one detection recorded during the study period, which was insufficient to calculate a visitation event. (A) Visitation events recorded by each receiver for each individual, and the size of the bubbles indicates the duration of visitation events; (B) visitation events recorded for each individual at each receiver station, and the size of the bubbles indicates the duration of visitation events at the stations. In (A), bubble colors correspond to receiver stations as shown in the legend, and black vertical lines represent deployment dates of each transmitter.
The VI for the 41 tagged oceanic manta rays spanned a broad range, from 0.4 to 100% (Supplementary Table 1). Notably, VIs of 100% were observed from six individuals tracked only from 1 to 4 days. For individuals tracked for more than 1 day (n = 37 individuals), the mean VI was 13.8% (SD = 24.2). In contrast, for those tracked for over 1 year (n = 11 individuals), the mean VI was only 2.05% (SD = 1.15). In addition to their visitation patterns, the tagged individuals demonstrated site fidelity to specific receiver stations (Figure 2). For example, ID#55478, tagged at Magic Mountain in May 2016, was last detected in July 2019, more than 3 years later. This individual periodically returned and was detected 49 times by six receivers in southeast Misool, southern Raja Ampat, showcasing a consistent pattern of site fidelity.
The Detection Index (DI) for acoustic receivers across various sites within the BHS demonstrated substantial variability (mean ± SD = 2.4 ± 3.6%; Table 1). Among the receivers recording detections from tagged oceanic manta rays, the station at Magic Mountain in SRA exhibited the highest DI, reaching 12.89%. This was closely followed by Eagle's Nest, also in southern Raja Ampat, with a DI of 8.65%; both of these receiver stations were placed at known cleaning stations for oceanic and reef manta rays. In contrast, the receiver stations at South Batanta and Bambu, both situated in central Raja Ampat, and both chosen as strategic transit points (rather than known cleaning stations), registered the lowest DI, each with only 0.08%. Within the CRA region, the Blue Magic receiver station (a known oceanic manta ray cleaning station) recorded the highest DI, accounting for 4.02%.
Visitation metrics, including the number of transmitters detected, the number of visitations, the number of detections, and DI, identified Magic Mountain, Eagle's Nest, Devil's Kitchen, and Southwest Batbitim as the most frequented sites in southern Raja Ampat. Blue Magic emerged as the primary site in CRA visited by the tagged oceanic manta rays (Table 1). The average visitation duration at these sites varied, with the shortest mean time being 27.1 min at Blue Magic and the longest being 74.7 min at Southwest Batbitim. Across all receiver stations, the average duration of visits to known oceanic manta ray cleaning stations (49.3 ± 64.6 min; n = 405 visits) was substantially longer than to known reef manta ray cleaning stations (18.0 ± 29.3 min; n = 15 visits) and strategic transit points (5.2 min; n = 1 visit). Importantly, the acoustic receivers placed at three cleaning stations previously known to host only reef manta rays (Dayan, Wai, and Yefnabi Kecil) recorded a total of 116 detections of tagged oceanic manta rays, with mean visit durations of 1.4, 3.4, and 31.3 min, respectively (Table 1). Both Dayan and Wai were visited each by one tagged manta ray, while Yefnabi Kecil was visited by four different oceanic manta rays.
3.3 Seasonal visitation and diel pattern
Throughout the study period, the majority of visitation events took place within the 14 months following the deployment of transmitters, particularly between May 2016 and July 2017 (Figure 2). During this period, five receivers (Magic Mountain, Eagle's Nest, Devil's Kitchen, and Southwest Batbitim in southeast Misool in SRA, and Blue Magic in the Dampier Strait in CRA) recorded a substantial amount of acoustic detections from 24 oceanic manta rays tagged in May-June 2016 (Supplementary Figure 2). Just over two-third of these manta rays frequently visited multiple receivers in SRA. Among them, five individuals subsequently visited the Blue Magic receiver station in CRA in December 2016–April 2017, following their initial visitations to receivers in SRA. In May 2017, two of these five individuals were subsequently detected by the receiver in Southwest Batbitim in SRA.
Throughout the year, the number of detections recorded by all receivers was the highest in May (36.2% of all detections; Supplementary Figure 3A). Seasonal patterns of visitation were discernable among the top five receivers (Supplementary Figure 3B) as well as between two subregions in Raja Ampat (Table 1, Supplementary Figure 4). In SRA, visitations to receiver stations predominantly took place from April to December, accounting for 96.0% of all visitation events recorded throughout the season in this southern region of Raja Ampat. In CRA, visitation events generally occurred from December to April, accounting for 82.3% of all visitation events recorded during the season in this region. However, the number of visitation events recorded in CRA (n = 62) was 82% lower than that in SRA (n = 350) during the study period.
The majority (94.2%) of detections across the study sites were recorded during daytime hours. To assess the differences between day and nighttime detections, we employed an unpaired two-sample Wilcoxon test. A Shapiro-Wilk test indicated a normal distribution of the data (p > 0.05), and an F-test revealed a significant difference in variance between daytime and nighttime detections (p < 0.05). Subsequently, the unpaired two-sample Wilcoxon test demonstrated a significant disparity in the mean hourly number of acoustic detections between daytime (mean ± SD = 456.8 ± 241.5) and nighttime (mean ± SD = 28.3 ± 8.1), with a p-value of < 0.001.
During daytime, detections began increasing from 07:00, peaked at noon, and then declined until 17:00 (Figure 3A). At the site level, hourly detection patterns varied among the five sites with the highest number of detections (Magic Mountain, Eagle's Nest, Devil's Kitchen, Southwest Batbitim in SRA, and Blue Magic in CRA). Specifically, Blue Magic experienced a surge in detections (35% of all detections recorded at this site) early in the morning (07:00–09:00); Devil's Kitchen saw heightened activity (42% of detections) from 11:00 to 14:00; Magic Mountain had increased detections (59% of detections) from 10:00 to 15:00; both Eagle's Nest and Southwest Batbitim showed the highest number of detections from 09:00 to 15:00 with 75 and 63% of detections, respectively (Figure 3B).
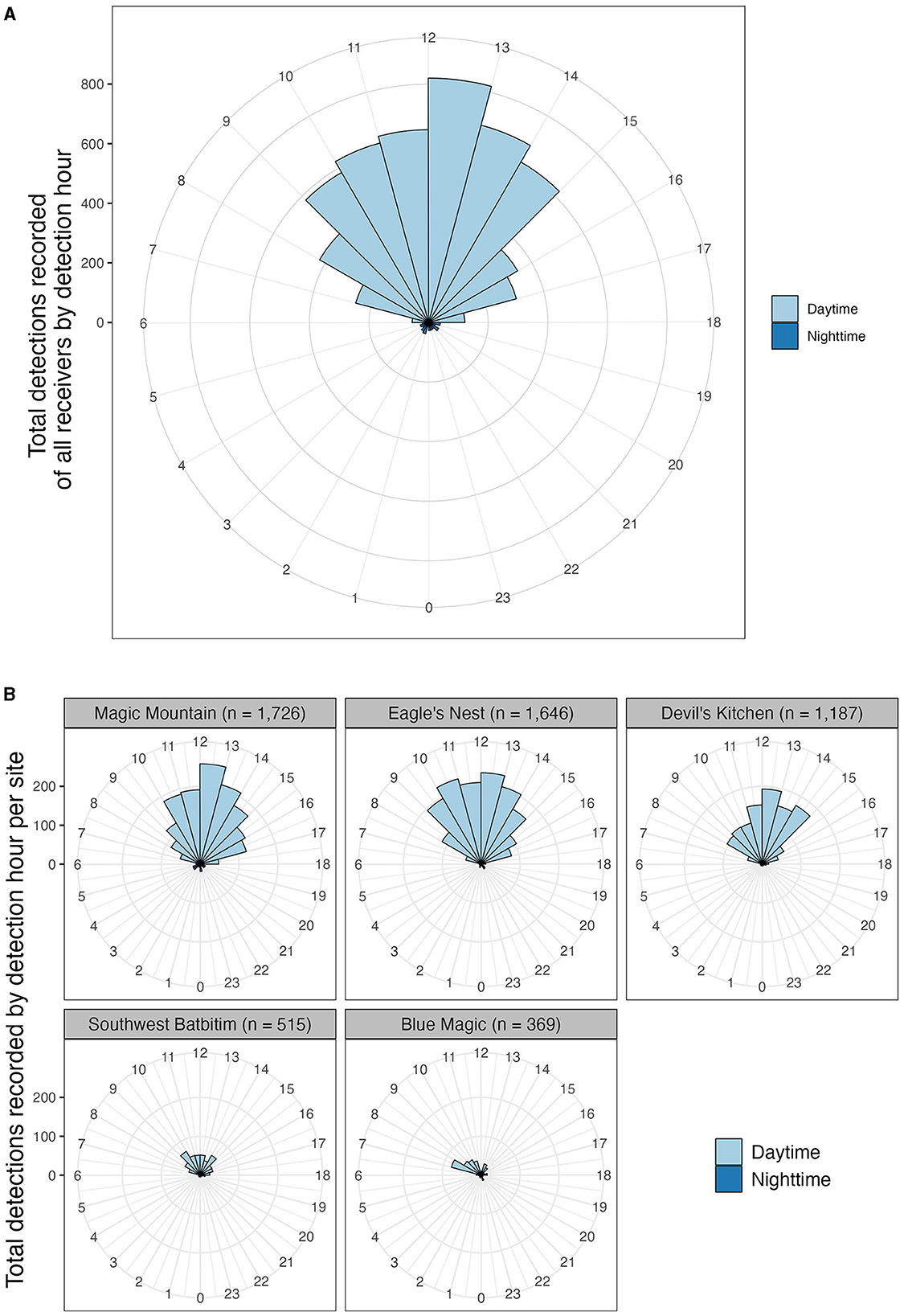
Figure 3. Detection records of oceanic manta rays at receiver stations by detection hour, between February 2016 and August 2019. Detections colored by daytime (06.00–18.00 h local time) and night-time periods (18.00–06.00 h local time). (A) Total detections of all receivers by detection hour; (B) total detections at the five sites which recorded the highest numbers of detections by detection hour. These sites included Magic Mountain, Eagle's Nest, Devil's Kitchen, and Southwest Batbitim in southeast Misool (southern Raja Ampat) and Blue Magic in Dampier Strait (central Raja Ampat).
3.4 Movement network and critical aggregation sites
The movement network of oceanic manta rays was constructed from 14 nodes and 52 edges, representing 191 movement events made by the tagged oceanic manta rays between these nodes (Figure 4). Only those receivers that were connected through movement events made by the oceanic manta rays were used to construct the movement network. This led to the exclusion of Bambu and South Batanta in constructing a network with 14 nodes (Table 2). The average path length of the network was 2.027, indicating the mean number of steps along the shortest paths for all possible pairs of network nodes. The edge density, which measures the relative density of connectivity in the network, was determined to be 0.069. Furthermore, the network's diameter—the longest of all the calculated shortest paths in the network—was 4, suggesting a moderate degree of separation between the furthest nodes.
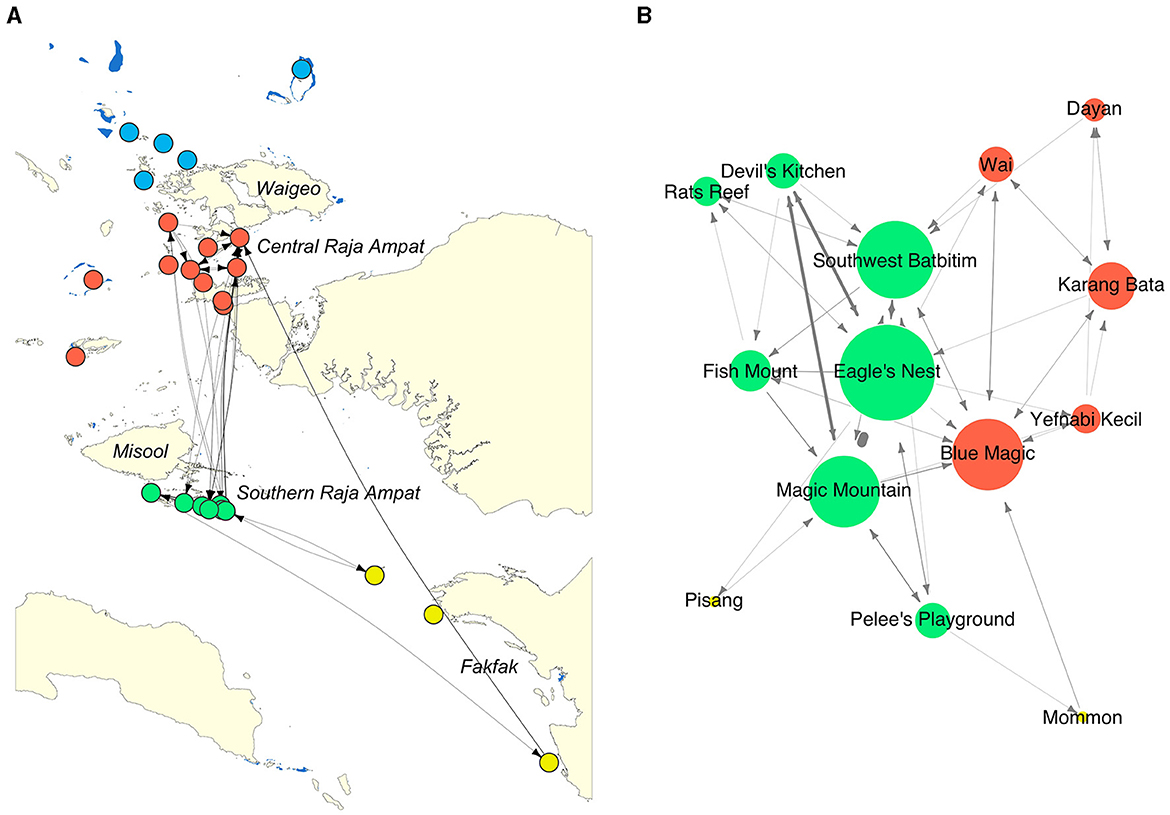
Figure 4. Movement networks of oceanic manta rays acoustically tagged in the Bird's Head Seascape between February 2016 and August 2019. Dots represent receiver stations and are colored by region and subregion within the region: northern Raja Ampat (NRA, blue), central Raja Ampat (CRA, red); southern Raja Ampat (SRA, green); Fakfak (FAK, yellow). Lines represent the movement of tagged oceanic manta rays between receiver stations. Arrow heads on lines show the direction of movements between two receiver stations. (A) Geographic coordinate layout; (B) multidimensional scale layout, showing only connected receiver stations (nodes). The size of nodes in (B) represent the degree centrality of each node (Table 2).
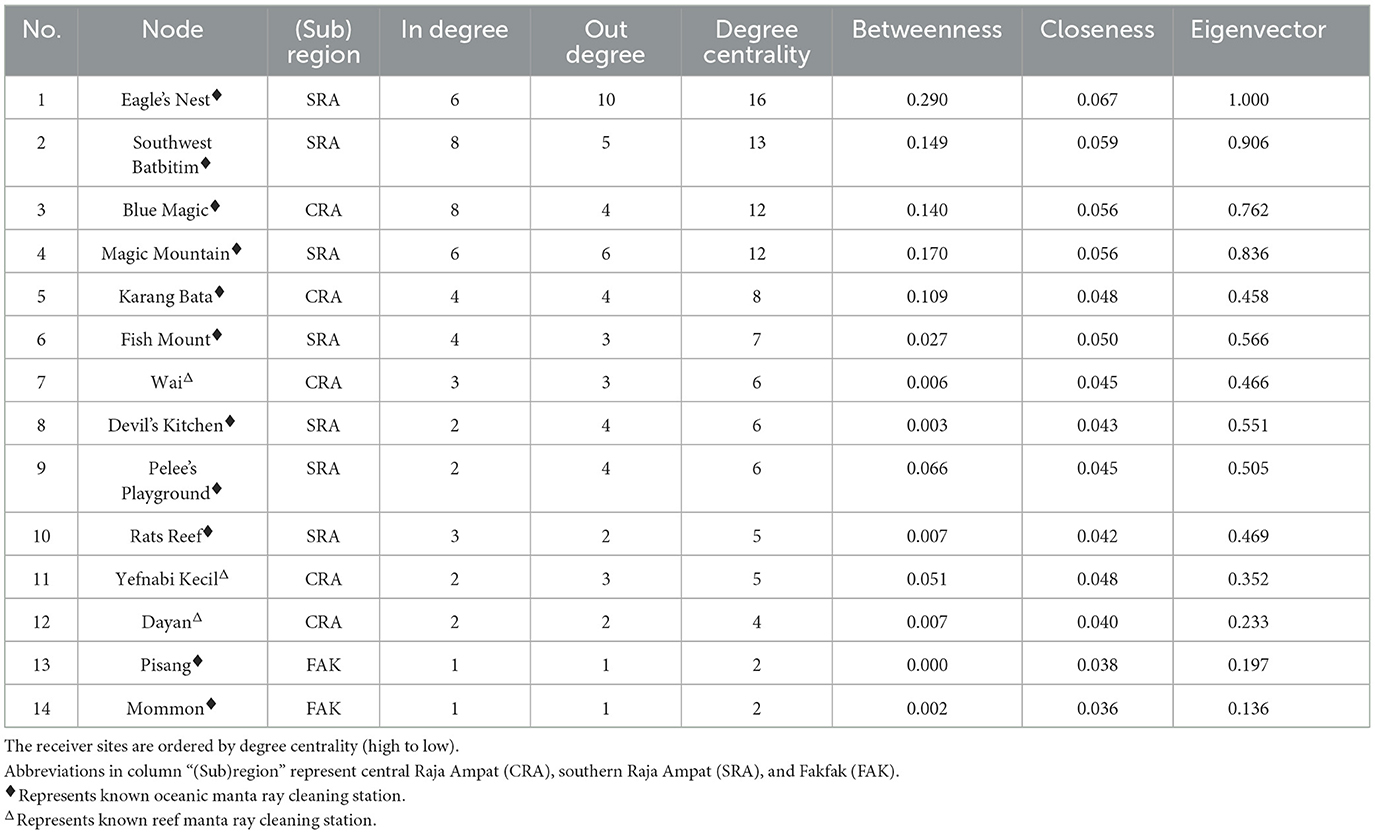
Table 2. Centrality measures (node level metrics) of tagged oceanic manta ray movement network in the Bird's Head Seascape between February 2016 and August 2019.
The network exhibited varying degrees of centrality across all nodes. Centrality measures at the node level identified five critical sites within the movement network. In southeast Misool (SRA), three receiver stations (Eagle's Nest, Southwest Batbitim, and Magic Mountain) were recognized as key nodes. Additionally, in the Dampier Strait area of CRA, two receiver stations (Blue Magic and Karang Bata) emerged as significant sites. These nodes exhibited considerably higher values of degree centrality, betweenness, closeness, and eigenvector compared to other nodes in the study regions, indicating their pivotal roles in the network (Table 2, Figure 4).
Movement events between receiver stations were recorded for 30 of the 37 oceanic manta rays that visited acoustic receivers (Supplementary Table 1; the other seven manta rays only visited a single station). The frequency of these movement events varied significantly among the individuals. Manta ID#55478, a male tagged at Magic Mountain in SRA, exhibited the highest activity with 22 recorded movements, distinguishing this male individual as the most mobile individual among those tagged. Furthermore, the distribution of movements across the acoustic receivers was not uniform, revealing a tendency toward local movements, particularly in SRA, where 157 movements (82.2%) were recorded. Specifically, 64% (n = 122) of all recorded movements occurred between neighboring acoustic receiver stations situated 5–12 km apart within SRA. Within CRA, only 14 movements (7.3% of all movements) were recorded among receivers in this subregion. In the Raja Ampat region, only 15 movements (7.9% of all movements) were recorded between receivers in CRA and SRA.
In addition to local and regional movements in Raja Ampat, the study also documented several long-distance movements in the BHS. Notably, two female oceanic manta rays, ID#55464 and ID#55479, tagged at Mommon seamount and Magic Mountain respectively, undertook substantial journeys between Mommon seamount in Fakfak and Blue Magic in CRA. These sites, both recognized as cleaning stations for oceanic manta rays, are separated by ~500 km over water (Figure 4).
4 Discussion
This is the first study to provide insight into the visitation and movement patterns of oceanic manta rays at cleaning stations on shallow coral reefs using passive acoustic telemetry in the BHS. The results show that our hypothesis, that passive acoustic telemetry can be used to track the presence and movements of oceanic manta rays in this region, can be accepted. Our preliminary study serves as a baseline for future research on the movement and residency patterns of this species, not only in this region, but potentially in other regions throughout its range. During ~3.5 years of passive acoustic tracking, we found that the average time spent by the tagged oceanic manta rays around receiver stations was similar to that by acoustically tracked reef manta rays in Raja Ampat, with visitation durations of 48 min (±64 SD) and 45 min (±5 SD), respectively (30). This indicates that in Raja Ampat, oceanic manta rays used cleaning stations as intensively as reef manta rays.
4.1 Visitations and site fidelity to cleaning stations
We found that the visitation index (VI = 13.8%) of acoustically tracked oceanic manta rays in Raja Ampat and Fakfak, which is similar to residency index (RI) in other studies, was markedly lower than the RI of 21% reported for reef manta rays in northern Raja Ampat (30). Our findings also revealed that the VI for oceanic manta rays in our study region, based on individuals tracked for more than 1 day (n = 35), is substantially lower than the indices recorded for reef manta rays across various regions in the Red Sea (39%) (48), Seychelle (61.9%) (49), British Indian Ocean Territory (40%) (50), and the Maldives (29.3%) (51). However, it was comparable to those recorded in southern Mozambique (14.5%) (52) and Lady Elliot Island (15.3%) (53). Comparing within the same species, the mean VI for tagged oceanic manta rays in the BHS was slightly lower than that observed in Bahia de Banderas, Mexico, which stood at 17.6% (32).
The observed low VI among tagged oceanic manta rays in our study is likely attributable to the inherent natural behavior of this species, which is characterized by a predominantly pelagic distribution, in contrast to the more residential reef manta rays. Oceanic manta rays are less residential and exhibit a larger home range than reef manta rays (18). Moreover, oceanic manta rays, being larger in size, have been documented to undertake longer distance movements (4) compared to reef manta rays (54).
The pattern of visitations of oceanic manta rays to aggregation sites in Raja Ampat and the broader BHS appears to be seasonal, likely aligned with the regional monsoon cycles. In southern Raja Ampat, the majority of detections coincided with the southeast monsoon period from June to October, whereas in central Raja Ampat, detections peaked during the northwest monsoon from November to April (23). The reef manta rays throughout the Raja Ampat archipelago showed localized movements and limited long-distance movements based on a 5-year passive acoustic tracking (31). In central and northern Raja Ampat, they displayed seasonal and local movements between Dampier Strait and the western area of Waigeo, where acoustic detections reached a peak in December-April (during the northwest monsoon) and June-October (during the southeast monsoon), respectively (30). All these suggest that unlike reef manta rays that are residents to and can be found throughout the year in Raja Ampat, the oceanic manta rays may spend considerable periods in more pelagic zones, such as the Seram Trough in the southeast of Seram Island (6), than coastal areas, when they were not detected by our receiver array.
The seasonal nature of oceanic manta ray visitations implies that they may not be permanent residents of Raja Ampat or the broader BHS, frequenting the region primarily under favorable environmental conditions. Notably, the number of oceanic manta ray sightings in Raja Ampat, as recorded through photo-identification, surged during the El Niño events of 2015–2016 (11), and then dropped off. This pattern is consistent with findings that both survival and per capita recruitment rates of reef manta rays in Raja Ampat were significantly influenced by the ENSO, particularly during El Niño phases (34). Comparable effects of ENSO on oceanic manta ray presence were reported by Fonseca-Ponce et al. (55) and Domínguez-Sánchez et al. (32) in Bahia de Banderas, Mexico, where photo-identification and passive acoustic tracking data indicated increased sightings of oceanic manta rays during La Niña phases. Conversely, in the Revillagigedo National Park, the apparent survival rate of oceanic manta rays as estimated through POPAN mark recapture models was lower during La Niña than that during El Niño in the eastern Tropical Pacific (56). Additionally, oceanic manta rays in the BHS are likely to use areas beyond the acoustic receivers' detection range. For instance, direct observations in the January–February timeframe in the Dampier Strait revealed oceanic manta rays visiting cleaning stations on coral reefs that do not have acoustic receivers. They were also observed foraging in locations distant from known cleaning stations, further underscoring the species' highly mobile nature (ES pers. obs.).
For highly oceanic species, a low VI threshold might be appropriate for indicating site fidelity. Our findings reveal that, despite a low VI, the oceanic manta rays monitored via passive acoustic telemetry in our study exhibited notable site fidelity to specific receiver stations, predominantly located at cleaning stations in both SRA and CRA, particularly within the Dampier Strait (Figure 1). This pattern of return visits, especially evident in oceanic manta rays whose transmitters were retained for over a year, underscores the importance of these sites. For example, two individuals (ID#55477 and ID#55478), with transmitter retention times exceeding 1,000 days, demonstrated return visits to the same or nearby cleaning stations in subsequent years (Figure 2A).
Site fidelity is a common behavior observed in both manta ray species [e.g., (10)]. It is often shown to cleaning stations and foraging areas [e.g., (30)], two critical habitats for the survival of manta rays. In the case of oceanic manta rays that were photo-identified in Misool (SRA), a resighting rate of 28% and an average resighting span of 285 days (11) further indicate their site fidelity to primary cleaning stations, such as Magic Mountain, where our acoustic receivers were placed. Similarly, in Isla de La Plata, Ecuador, oceanic manta rays displayed a 12.9% resighting rate, indicating fidelity to cleaning stations in the island (12).
Tagged oceanic manta rays were detected repeatedly at three cleaning stations (Wai, Dayan, and Yefnabi Kecil) in CRA which were previously only known for hosting only reef manta rays (14). This suggests that oceanic manta rays likely also utilize these cleaning stations, and in the case of Dayan, one of us (CB, pers. obs.) was able to confirm this with an observation of an oceanic manta ray cleaning on the site, subsequent to our study. Indeed, a number of the manta ray cleaning stations in Misool (e.g., Magic Mountain, Southwest Batbitim, Devil's Kitchen, Fish Mount, and Eagle's Nest) are known to host both species (14). Based upon the current finding, at least eight manta ray cleaning stations in Raja Ampat are utilized by both oceanic and reef manta rays.
4.2 Diel pattern at receiver stations
The predominance of daylight visitations to receiver stations by oceanic manta rays tagged in Raja Ampat aligns with observations from Bahia de Banderas, Mexico (32). The high number of detections recorded during daytime could be attributed to the activity of cleaner fish inhabiting cleaning stations, where the overwhelming majority of acoustic detections were recorded (5,817 of 5,822 detections were from 14 acoustic receivers installed at known cleaning stations). This diel pattern of daytime activity is consistent with findings on reef manta rays in northern Raja Ampat (30) and across the Indo-Pacific region (29, 48–53, 57).
This diel pattern of activity at cleaning station receivers might also reflect a transition from shallow coastal waters during the day to deeper or offshore waters at night to feed on vertically migrating mesopelagic zooplankton. A study on satellite-tracked oceanic manta rays in Peru by Andrzejaczek et al. (58) highlighted a reverse diel vertical migration pattern, with individuals remaining in coastal surface waters during the day and moving to deeper waters at night. Oceanic manta rays were observed feeding in deep waters in Revillagigedo archipelago, Mexico (59) and similarly in Isla de La Plata, Ecuador, stable isotope analysis demonstrated that oceanic manta rays' main source of prey came from mesopelagic habitats (60), further supporting the notion that deep-water feeding, particularly on mesopelagic prey, is a common nocturnal activity for this species. Isla de La Plata is located near the continental shelf edge (with depths exceeding 3,000 m within a few kilometers of shore, facilitating the combination of deep-water nighttime feeding and day time cleaning station visits (12).
The bathymetric conditions of the primary oceanic manta ray cleaning stations in the BHS are similar. In SRA, the oceanic manta ray cleaning stations are situated only 25 km from the Seram Sea, which reaches depths of ~2,000 m (Figure 1). Additionally, the Mommon seamount in Fakfak, located on the Seram Trough's edge, further exemplifies the strategic location of shallow cleaning stations adjacent to deep waters. In CRA, the Blue Magic cleaning station is similarly located close to the mouth of the Dampier Strait, which plunges to over 1,000 m deep. This proximity to deep waters across various sites in the BHS likely facilitates the oceanic manta rays' access to off-shelf feeding grounds during nighttime.
4.3 Movements, critical habitats, and spatial connectivity
The movement analysis revealed that the acoustically tracked oceanic manta rays in our study exhibited both local and regional movements between receiver stations (sites) across the BHS, with the majority of movements recorded between cleaning stations in SRA and CRA (Figures 1, 4). In SRA, the tracked oceanic manta rays navigated among seven key sites: Magic Mountain, Eagle's Nest, Southwest Batbitim, Fish Mount, Rat's Reef, Devil's Kitchen, and Pelee's Playground. These sites, located within ~50 km distance over water, are each recognized for their importance as cleaning station aggregation sites.
Similarly, in CRA, local movements were observed among five sites: Blue Magic, Karang Bata, Wai, Dayan, and Yefnabi Kecil, underscoring a pattern of localized movement between cleaning stations within both central and southern subregions in Raja Ampat. This localized movement pattern is similar to findings from a previous study by Setyawan et al. (31) on reef manta rays that also utilized the same acoustic array, indicating a shared use of these critical habitats by both species of manta ray. This connectivity underscores the ecological significance of these cleaning station sites as hubs of manta rays' survival and health in Raja Ampat.
In our study, the greatest distance recorded between two acoustic receiver stations for oceanic manta rays in the BHS was ~500 km. This surpassed the furthest distance recorded for movement by a photo-identified reef manta ray in the BHS, which was between two sighting sites separated by 297 km within the Raja Ampat archipelago (14). It is important to note that the maximum movement distance observed for oceanic manta rays in our research was constrained by the spatial arrangement of our acoustic receiver array in the BHS. In other global locales, oceanic manta rays have been documented undertaking significant migrations. For instance, one individual traveled up to 1,500 km in the eastern Pacific Ocean (4), while another journeyed ~2,000 km from the waters of New Zealand to Fiji (5). Furthermore, a juvenile oceanic manta ray in the Red Sea was identified traveling a distance of at least 525 km, as determined through photo-identification (61).
The movement network of acoustically tagged oceanic manta rays showed high connectivity among cleaning station aggregation sites, particularly those located close to each other in SRA. However, connectivity between two subregions in Raja Ampat was comparable to connectivity within CRA, as indicated by the similar number of movements between these subregions (Figure 4). This pattern of connectivity contrasts with the movement network observed for reef manta rays in Raja Ampat, which displayed distinct clusters between Misool (SRA) and the Dampier Strait (CRA) with infrequent movements between these two subregions, suggesting differing spatial dynamics between the two species (31).
The observed connectivity among habitats in Raja Ampat and the broader BHS region implies the existence of a single, interconnected population across the BHS. In fact, genetic analyses of samples collected from across the species' range have found no evidence of population structuring, indicating a globally panmictic population (62). At the site level, several cleaning station sites in SRA (Eagle's Nest, Southwest Batbitim, and Magic Mountain) and northern Raja Ampat (Blue Magic and Karang Bata) were identified as important nodes due to their high centrality measures as shown by the high connectivity of these nodes to other nodes. This indicates that these sites were highly influential and had central roles in the local and regional movements of oceanic manta rays in the Raja Ampat and the broader BHS. Some of these key sites also correspond with receiver stations that exhibited the highest DI among all monitored sites (Table 1).
Cleaning stations, situated on shallow coral reefs, are ecologically important to the life cycle of manta rays, serving as essential sites for parasite removal through symbiotic interactions with cleaner fish (10). Cleaning stations are also pivotal for facilitating social interactions, including courtship and mating behaviors (13, 19, 63). Furthermore, access to warm waters when visiting cleaning stations in shallow coastal areas is hypothesized to enhance metabolic, digestive, and gestation rates, as observed for other marine species such as leopard sharks (64) and stingrays (65).
4.4 Limitations of the study
Despite the ability to elucidate the use of particular habitats, the use of passive acoustic telemetry only allows tracking of animals that remain or return to the same area and within the detection range of the acoustic receiver array (66). Consequently, this methodology does not capture data on the movements of acoustically tagged animals when they navigate between spatial gaps unmonitored by our receiver array. Furthermore, the finite and somewhat limited detection range of these receivers (measured at 150 m in our range test for this study) may compromise the detectability of tagged oceanic manta rays. Additionally, the pattern of detections recorded by our receivers could be affected by the detection efficiency of the acoustic array. Detection efficiency refers to the proportion of transmitted acoustic signals successfully recorded by the receivers, and it can be influenced by various factors, including environmental conditions, tag and receiver specifications, and the physical characteristics of the study site (67). In our study, the diel pattern of visitation to cleaning stations by oceanic manta rays could be influenced by extreme noise levels from coral reefs at night that dramatically reduced the receiver performance (68), decreasing detection rates. Despite this, the observed diurnal visitation pattern of oceanic manta rays to receiver stations was sufficient to infer a primarily daytime presence. This aligns with the reverse diel migration observed in the Eastern Pacific, where oceanic manta rays preferred surface habitats during the day and moved to deeper waters at night (58), suggesting their presence at shallow coral reefs during the day.
Although the tagged oceanic manta rays were detected by receivers deployed at cleaning stations, we are unable to say with certainty if the rays were cleaning or just transiting near the receiver when they were detected, as the acoustic transmitters used in our study do not have the ability to detect manta ray behaviors. However, the duration of visits, which was 48 min on average at known oceanic manta ray cleaning stations and only 5 min at strategic transit points, would strongly suggest that the manta rays were indeed cleaning. Indeed, all 41 oceanic manta rays tagged for this study were tagged at cleaning stations while they were engaged in cleaning behavior.
The scope of this study is constrained by the nature of oceanic manta ray interactions with coastal resources, which represent only a fraction of their broader life history. Although we have identified several cleaning stations, it is probable that other cleaning stations frequented by oceanic manta rays remain undiscovered. This limitation suggests that our findings may not fully describe the species' patterns of coastal area use in the BHS. Furthermore, the subset of oceanic manta rays tracked using passive acoustic telemetry in this study is significantly smaller than the total number of individuals documented in the Raja Ampat region (11). Consequently, the observed visitation and movement patterns may not fully reflect the broader behavioral trends of the species in the BHS.
In addition to limitation in spatial coverage, the variability in deployment durations and periods of the acoustic receivers across different cleaning stations introduces another layer of limitation. This variability may hinder our ability to consistently monitor and compare the movements and residency patterns of oceanic manta rays across all identified stations over uniform periods for a long period. The only period when all receivers, except for the one at Magic Mountain, were active simultaneously was between mid-February 2017 and mid-September 2017, ~7 months (Supplementary Figure 1). As such, the study's insights into the temporal dynamics of site fidelity and spatial usage by oceanic manta rays should be interpreted with caution.
4.5 Conservation and management implications
The frequent visitations of oceanic manta rays to cleaning stations are of significant interest for the management of oceanic manta ray-focused diving tourism in the Raja Ampat and Fakfak regions within the BHS. Given the critical role of cleaning stations in the lives of oceanic manta rays, strict regulation of their use for tourism is crucial. The adoption of standard operational procedures—for example, restricting the number of divers and snorkelers to a maximum of 20 individuals per site at any given time—coupled with the enforcement of a code of conduct for interactions with manta rays (69), as has been implemented at the “Manta Sandy” reef manta ray cleaning station in Raja Ampat (70), appears to mitigate overcrowding and enhance tourist satisfaction (25). Such regulatory measures and enforcement protocols should be extended to other cleaning stations frequented by oceanic manta rays. Notably, manta ray-focused diving activities have already led to significant coral reef damage around some cleaning stations such as at Blue Magic and Magic Mountain (RM, pers. obs.), making enhanced management of these sites even more important. We note that with the five cleaning stations highlighted by our study to be critically important nodes in oceanic manta ray movements in the BHS (Eagle's Nest, Southwest Batbitim, and Magic Mountain in SRA, and Blue Magic and Karang Bata in CRA), consideration should be given to seasonally or permanently prohibiting diving on at least one important cleaning station in each region to ensure oceanic manta rays have a “safe haven” for cleaning as manta tourism continues to grow.
Our research further revealed the movements of tagged oceanic manta rays through areas beyond MPA boundaries, underscoring the looming threat posed by gillnet fisheries that are still operating outside the MPA network in the BHS (25). Bycatch from gillnet fisheries has emerged as one of the foremost threats to manta ray populations globally (71). Incidental observations of manta rays with partial net entanglement within CRA and SRA (unpublished data) serve as a reminder of the dangerous interaction between this endangered species and fishing activities. Understanding the seasonal and spatial movements of oceanic manta rays beyond current MPA boundaries is essential for identifying critical areas, including foraging grounds, outside the BHS. Additionally, detailed data on fisheries operating in these areas, particularly those using gillnets or other gear that pose a threat to manta rays, is crucial. This includes assessing bycatch rates and pinpointing specific locations where interactions between oceanic manta rays and fisheries occur.
Despite being fully protected in Raja Ampat waters (72) and subsequently across Indonesian waters (73), there is an urgent need for enhanced management and conservation efforts to safeguard this globally endangered species. Importantly, all 14 known oceanic manta ray cleaning stations in Raja Ampat and Fakfak are protected from fishing activities within the existing BHS MPA network; however, a coordinated approach for oceanic manta ray conservation and management at the provincial level is nonetheless imperative. Such collaboration should involve local government entities in Raja Ampat, Fakfak, and Kaimana, in addition to the West Papua and Southwest Papua provincial governments and should be aimed at mitigating threats including tourism pressure, bycatch, and illegal fishing practices that jeopardize this highly migratory and wide-ranging species. Although none of the acoustically tagged oceanic manta rays were detected by acoustic receivers in Kaimana, the inclusion of this area in conservation strategies remains critical, as oceanic manta rays continue to be sighted sporadically in Kaimana and there are ongoing reports of occasional bycatch of this endangered species.
4.6 Future research
Future investigations should prioritize the establishment of a comprehensive survey and monitoring initiative aimed at delineating the distribution of critical habitats, including cleaning stations and foraging areas, for oceanic manta rays across Raja Ampat and the broader BHS as initially described in Setyawan et al. (14). This initiative must incorporate the continuous collection of photo IDs and sighting records to develop a robust catalog of the regional oceanic manta ray population in the BHS. Such data will facilitate assessments of population dynamics, including changes in population size and other key demographic parameters like survival and resighting rates (11, 34).
Future studies should also leverage satellite telemetry to delve into the spatial movement ecology of oceanic manta rays within the BHS. Although Stewart et al. (6) provided estimates of oceanic manta ray home ranges in Raja Ampat using data from 10 pop-up satellite archival tags, further investigation is crucial to assess potential risks associated with wide range movements beyond marine protected area (MPA) boundaries. This research is vital for evaluating the adequacy of the existing MPA network in the BHS, which is predominantly coastal, with none of these MPAs extending into oceanic habitats (25, 74). Employing satellite tags equipped with Fastloc GPS combined with state space models will facilitate the exploration of area-restricted search behaviors. This approach enables the identification of important areas where oceanic manta rays predominantly engage in activities likely related to foraging, shedding light on their behavioral ecology [e.g., (36)]. Observations of oceanic manta rays engaging in somersault feeding at the sea surface in Raja Ampat (Indonesia) and Hauraki Gulf (New Zealand; ES and ME, pers. obs.), along with the enhanced location accuracy afforded by Fastloc GPS, suggest the potential of using this type of satellite tag to identify significant near shore areas for oceanic manta rays (e.g., foraging grounds), typically situated offshore for this species.
Investigating the environmental factors influencing sightings and aggregations of oceanic manta rays at cleaning stations and foraging areas will elucidate the underlying mechanisms driving their presence at these key locations (32, 51, 55, 56). Furthermore, employing species distribution modeling approaches to predict the spatiotemporal distribution and habitat preferences of oceanic manta rays in eastern Indonesia is also essential. Such models, similar to those employed by Putra et al. (75), Lezama-Ochoa et al. (76), Ozaki (77), and Garzon et al. (2), will provide invaluable insights into the spatiotemporal distribution and conservation priorities for this species.
5 Conclusions
This study represents the first in the Indo-West Pacific to provide insights into the visitations and use of shallow coastal waters on coral reefs by oceanic manta rays, which are generally thought to spend the majority of their time in oceanic environments and deep waters. It highlights the utility of passive acoustic telemetry in tracking the presence and movements of oceanic manta rays visiting cleaning stations on coral reefs in the BHS, suggesting this methodology may also be useful in other coral reef areas which oceanic manta rays are known to frequent. The tagged oceanic manta rays exhibited site fidelity to cleaning stations, particularly those located in central and southern Raja Ampat, with acoustic detections at these stations occurring predominantly during daylight hours. Although relatively brief, these visitations and the demonstrated site fidelity to shallow coral reef cleaning stations underscore the critical importance of these cleaning stations to the health of oceanic manta rays. Furthermore, our findings on the seasonal visitation, site fidelity, and long-distance movements across areas not protected by MPAs provide essential information for improving the conservation management of oceanic manta rays in the BHS. Our study moreover revealed that three cleaning stations previously only known to host reef manta rays likely serve as cleaning stations for oceanic manta rays as well, bringing the number of known oceanic manta cleaning stations in the BHS to fourteen. Finally, our study underscores the significance of several cleaning stations in southern Raja Ampat (i.e., Eagle's Nest, Southwest Batbitim, and Magic Mountain) and central Raja Ampat within Dampier Strait (i.e., Blue Magic and Karang Bata) as pivotal nodes within the oceanic manta ray movement network in the Raja Ampat region, suggesting these are particularly critical habitats for oceanic manta rays in the BHS. The information supplied by this study will be important in managing oceanic manta diving tourism in the region and in increasing the protection of this species, especially in areas beyond existing MPA boundaries.
Data availability statement
The original contributions presented in the study are included in Supplementary material, further inquiries can be directed to the corresponding author.
Ethics statement
The animal study was approved by the University of Auckland Animal Ethics Committee and was conducted following protocol 002228. The study was conducted in accordance with the local legislation and institutional requirements.
Author contributions
ES: Conceptualization, Data curation, Formal analysis, Investigation, Methodology, Visualization, Writing – original draft, Writing – review & editing. RM: Investigation, Writing – review & editing. AS: Investigation, Writing – review & editing, Project administration. AH: Investigation, Writing – review & editing. MP: Writing – review & editing. IM: Investigation, Writing – review & editing. CB: Investigation, Writing – review & editing. ME: Conceptualization, Funding acquisition, Project administration, Supervision, Writing – review & editing.
Funding
The author(s) declare financial support was received for the research, authorship, and/or publication of this article. The research was generously funded by Allchin Family's Sunbridge Foundation, the Wolcott Henry Foundation, Alex and Sybilla Balkanski, the Paine Family Trust, Shannon and Audrey Wong and Save the Blue, and MAC3 Impact Philanthropies.
Acknowledgments
The authors extend their deepest gratitude to the Government of Indonesia, including the Ministry of Marine Affairs and Fisheries and the Raja Ampat MPA Management Authority (BLUD UPTD Pengelolaan Kawasan Konservasi Perairan Kepulauan Raja Ampat). A special acknowledgment is due to the traditional adat communities of Raja Ampat for graciously hosting our research activities. Our sincere appreciation also goes to Conservation International and Konservasi Indonesia donors for their generous financial support of this project. Further thanks are extended to Misool Resort for facilitating our work in southern Raja Ampat. We are profoundly grateful to Meity Mongdong and the late Kristian Thebu for their invaluable support of the manta ray research and conservation program in Raja Ampat and the wider Bird's Head Seascape. A heartfelt thanks to Urias Tuhumena and the Yaswal boat crew (Demas Fiay) for their dedication and tireless efforts in assisting with data collection in the field. Additionally, we acknowledge the indispensable support of our field team, Timore Kristiani, Hanrika Leimena, Julius Thonak, Poerwanto, Marselinus Uskono, and Yakonias Thonak, who ensured the availability of boats and logistical supplies for numerous extensive trips to the study region.
Conflict of interest
The authors declare that the research was conducted in the absence of any commercial or financial relationships that could be construed as a potential conflict of interest.
Publisher's note
All claims expressed in this article are solely those of the authors and do not necessarily represent those of their affiliated organizations, or those of the publisher, the editors and the reviewers. Any product that may be evaluated in this article, or claim that may be made by its manufacturer, is not guaranteed or endorsed by the publisher.
Supplementary material
The Supplementary Material for this article can be found online at: https://www.frontiersin.org/articles/10.3389/frish.2024.1432244/full#supplementary-material
References
1. Stewart JD, Jaine FRA, Armstrong AJ, Armstrong AO, Bennett MB, Burgess KB, et al. Research priorities to support effective manta and devil ray conservation. Front Mar Sci. (2018) 5:314. doi: 10.3389/fmars.2018.00314
2. Garzon F, Seymour ZTA, Monteiro ZL, Graham RT. Spatial ecology of a newly described oceanic manta ray population in the Atlantic Ocean. Mar Biol. (2023) 170:68. doi: 10.1007/s00227-023-04219-y
3. Marshall A, Barreto R, Carlson J, Fernando D, Fordham S, Francis MP, et al. Mobula Birostris. The IUCN Red List of Threatened Species. Cambridge: International Union for Conservation of Nature (2020). p. e.T198921A68632946.
4. Hearn AR, Acuna D, Ketchum JT, Penaherrera C, Green J, Marshall A, et al. Elasmobranchs of the Galapagos marine reserve. In:Denkinger J, Vinueza L., , editors. The Galapagos Marine Reserve. Berlin: Springer (2014). p. 23–59.
5. Setyawan E, Duffy CA, Erdmann MV, Green L, Tindale S. First Insights Into the Spatial Ecology of Endangered Mobula birostris in Aotearoa. Tauranga: New Zealand Marine Sciences Society (2021).
6. Stewart JD, Beale CS, Fernando D, Sianipar AB, Burton RS, Semmens BX, et al. Spatial ecology and conservation of Manta birostris in the Indo-Pacific. Biol Conserv. (2016) 200:178–83. doi: 10.1016/j.biocon.2016.05.016
7. Marshall AD, Compagno LJ, Bennett MB. Redescription of the genus Manta with resurrection of Manta alfredi (Krefft, 1868) (Chondrichthyes; Myliobatoidei; Mobulidae). Zootaxa. (2009) 2301:1–28. doi: 10.11646/zootaxa.2301.1.1
8. Yano K, Sato F, Takahashi T. Observations of mating behavior of the manta ray, Manta birostris, at the Ogasawara Islands, Japan. Ichthyol Res. (1999) 46:289–96. doi: 10.1007/BF02678515
9. Kashiwagi T, Ito T, Sato F. Occurrences of reef manta ray, Manta alfredi, and giant manta ray, M. birostris, in Japan, examined by photographic records. Rep Jpn Soc Elasmobranch Stud. (2010) 46:20–7. doi: 10.1371/journal.pone.0088655
10. Stevens GMW. Conservation and Population Ecology of Manta Rays in the Maldives. York, United Kingdom: University of York (2016).
11. Beale CS, Stewart JD, Setyawan E, Sianipar AB, Erdmann MV. Population dynamics of oceanic manta rays (Mobula birostris) in the Raja Ampat Archipelago, West Papua, Indonesia, and the impacts of the El Niño-Southern Oscillation on their movement ecology. Divers Distrib. (2019) 25:1472–87. doi: 10.1111/ddi.12962
12. Harty K, Guerrero M, Knochel AM, Stevens GMW, Marshall A, Burgess K, et al. Demographics and dynamics of the world's largest known population of oceanic manta rays Mobula birostris in coastal Ecuador. Mar Ecol Prog Ser. (2022) 700:145–59. doi: 10.3354/meps14189
13. Setyawan E, Heinrichs S, Erdmann M. First documented courtship behavior between Mobula birostris and M. alfredi at a Coral Reef Cleaning Station in Misool, Raja Ampat. Diversity. (2024) 16:319. doi: 10.3390/d16060319
14. Setyawan E, Erdmann MV, Lewis SA, Mambrasar R, Hasan AW, Templeton S, et al. Natural history of manta rays in the Bird's Head Seascape, Indonesia, with an analysis of the demography and spatial ecology of Mobula alfredi (Elasmobranchii: Mobulidae). J Ocean Sci Found. (2020) 36:49–83. doi: 10.5281/zenodo.4396260
16. Germanov ES, Bejder L, Chabanne DB, Dharmadi D, Hendrawan IG, Marshall AD, et al. Contrasting habitat use and population dynamics of reef manta rays within the Nusa Penida marine protected area, Indonesia. Front Mar Sci. (2019) 6:215. doi: 10.3389/fmars.2019.00215
17. Armstrong AO, Armstrong AJ, Bennett MB, Richardson AJ, Townsend KA, Everett JD, et al. Mutualism promotes site selection in a large marine planktivore. Ecol Evol. (2021) 11:5606–23. doi: 10.1002/ece3.7464
18. Couturier LIE, Marshall AD, Jaine FRA, Kashiwagi T, Pierce SJ, Townsend KA, et al. Biology, ecology and conservation of the Mobulidae. J Fish Biol. (2012) 80:1075–119. doi: 10.1111/j.1095-8649.2012.03264.x
19. Stevens GMW, Hawkins JP, Roberts CM. Courtship and mating behaviour of manta rays Mobula alfredi and M. birostris in the Maldives. J Fish Biol. (2018) 93:344–59. doi: 10.1111/jfb.13768
20. Allen GR, Erdmann MV. Reef fishes of the Bird's Head Peninsula, West Papua, Indonesia. Check List. (2009) 5:587–628. doi: 10.15560/5.3.587
21. Allen GR, Erdmann MV. Reef Fishes of the East Indies. 2nd ed. Perth, WA: Tropical Reef Research (2024).
22. DeVantier L, Turak E, Allen G. Reef-scapes, reef habitats and coral communities of Raja Ampat, Bird's Head Seascape, Papua, Indonesia. Rep. Nat. Conserv. Bali Indones. (2009) 7:24.
23. Mangubhai S, Erdmann MV, Wilson JR, Huffard CL, Ballamu F, Hidayat NI, et al. Papuan bird's head seascape: emerging threats and challenges in the global center of marine biodiversity. Mar Pollut Bull. (2012) 64:2279–95. doi: 10.1016/j.marpolbul.2012.07.024
24. O'Malley MP, Lee-Brooks K, Medd HB. The global economic impact of manta ray watching tourism. PLoS ONE. (2013) 8:e65051. doi: 10.1371/journal.pone.0065051
25. Setyawan E, Erdmann MV, Gunadharma N, Gunawan T, Hasan AW, Izuan M, et al. A holistic approach to manta ray conservation in the Papuan Bird's Head Seascape: resounding success, ongoing challenges. Mar Policy. (2022) 137:104953. doi: 10.1016/j.marpol.2021.104953
26. Chevis MG, Godley BJ, Lewis JP, Jackson Lewis J, Scales KL, Graham RT. Movement patterns of juvenile hawksbill turtles Eretmochelys imbricata at a Caribbean coral atoll: long-term tracking using passive acoustic telemetry. Endanger Species Res. (2017) 32:309–19. doi: 10.3354/esr00812
27. Acuña-Marrero D, Smith ANH, Hammerschlag N, Hearn A, Anderson MJ, Calich H, et al. Residency and movement patterns of an apex predatory shark (Galeocerdo cuvier) at the Galapagos Marine Reserve. PLoS ONE. (2017) 12:e0183669. doi: 10.1371/journal.pone.0183669
28. Rohner CA, Cochran JEM, Cagua EF, Prebble CEM, Venables SK, Berumen ML, et al. No place like home? High residency and predictable seasonal movement of whale sharks off Tanzania. Front Mar Sci. (2020) 7:423. doi: 10.3389/fmars.2020.00423
29. Dewar H, Mous P, Domeier M, Muljadi A, Pet J, Whitty J. Movements and site fidelity of the giant manta ray, Manta birostris, in the Komodo Marine Park, Indonesia. Mar Biol. (2008) 155:121–33. doi: 10.1007/s00227-008-0988-x
30. Setyawan E, Sianipar AB, Erdmann MV, Fischer AM, Haddy JA, Beale CS, et al. Site fidelity and movement patterns of reef manta rays (Mobula alfredi: Mobulidae) using passive acoustic telemetry in northern Raja Ampat, Indonesia. Nat Conserv Res. (2018) 3:17–31. doi: 10.24189/ncr.2018.043
31. Setyawan E, Erdmann MV, Mambrasar R, Ambafen O, Hasan AW, Izuan M, et al. Spatial connectivity of reef manta rays across the Raja Ampat archipelago, Indonesia. R Soc Open Sci. (2024) 11:230895. doi: 10.1098/rsos.230895
32. Domínguez-Sánchez PS, Širović A, Fonseca-Ponce IA, Zavala-Jiménez AA, Rubin RD, Kumli KR, et al. Occupancy of acoustically tagged oceanic manta rays, Mobula birostris, in Bahia de Banderas, Mexico. Mar Biol. (2023) 170:128. doi: 10.1007/s00227-023-04278-1
33. Setyawan E. Movement Ecology, Population Dynamics, and Conservation of Reef Manta Rays (Mobula alfredi) in Raja Ampat, Indonesia. Auckland, New Zealand. The University of Auckland (2023). Available at: https://researchspace.auckland.ac.nz/handle/2292/67033 (accessed February 1, 2024).
34. Setyawan E, Stevenson BC, Erdmann MV, Hasan AW, Sianipar AB, Mofu I, et al. Population estimates of photo-identified individuals using a modified POPAN model reveal that Raja Ampat's reef manta rays are thriving. Front Mar Sci. (2022) 9:1014791. doi: 10.3389/fmars.2022.1014791
35. Kraft S, Gandra M, Lennox RJ, Mourier J, Winkler AC, Abecasis D. Residency and space use estimation methods based on passive acoustic telemetry data. Mov Ecol. (2023) 11:12. doi: 10.1186/s40462-022-00364-z
36. Setyawan E, Erdmann MV, Mambrasar R, Hasan AW, Sianipar AB, Constantine R, et al. Residency and use of an important nursery habitat, Raja Ampat's Wayag Lagoon, by juvenile reef manta rays (Mobula alfredi). Front Mar Sci. (2022) 9:815094. doi: 10.3389/fmars.2022.815094
37. R Core Team. R: A Language and Environment for Statistical Computing. (2023). Available at: http://www.R-project.org/
38. Campbell HA, Watts ME, Dwyer RG, Franklin CE. V-Track: software for analysing and visualising animal movement from acoustic telemetry detections. Mar Freshw Res. (2012) 63:815–20. doi: 10.1071/MF12194
39. Csardi G, Nepusz T. The igraph software package for complex network research. Inter J. Compl. Syst. (2006) 2006:1695.
40. Jacoby DMP, Freeman R. Emerging network-based tools in movement ecology. Trends Ecol Evol. (2016) 31:301–14. doi: 10.1016/j.tree.2016.01.011
41. Rayfield B, Fortin M-J, Fall A. Connectivity for conservation: a framework to classify network measures. Ecology. (2011) 92:847–58. doi: 10.1890/09-2190.1
42. Brodie S, Lédée EJI, Heupel MR, Babcock RC, Campbell HA, Gledhill DC, et al. Continental-scale animal tracking reveals functional movement classes across marine taxa. Sci Rep. (2018) 8:3717. doi: 10.1038/s41598-018-21988-5
43. Urban D, Keitt T. Landscape connectivity: a graph-theoretic perspective. Ecol Durh. (2001) 82:1205–18. doi: 10.1890/0012-9658(2001)082(1205:LCAGTP)2.0.CO;2
44. Jacoby DMP, Brooks EJ, Croft DP, Sims DW. Developing a deeper understanding of animal movements and spatial dynamics through novel application of network analyses. Methods Ecol Evol. (2012) 3:574–83. doi: 10.1111/j.2041-210X.2012.00187.x
45. Urban DL, Minor ES, Treml EA, Schick RS. Graph models of habitat mosaics. Ecol Lett. (2009) 12:260–73. doi: 10.1111/j.1461-0248.2008.01271.x
46. Newman M. Measures and metrics. In: Networks. Oxford: Oxford University Press. (2018). p. 158–217.
47. Minor ES, Urban DL. Graph theory as a proxy for spatially explicit population models in conservation planning. Ecol Appl. (2007) 17:1771–82. doi: 10.1890/06-1073.1
48. Knochel AM, Hussey NE, Kessel ST, Braun CD, Cochran JEM, Hill G, et al. Home sweet home: spatiotemporal distribution and site fidelity of the reef manta ray (Mobula alfredi) in Dungonab Bay, Sudan. Mov Ecol. (2022) 10:22. doi: 10.1186/s40462-022-00314-9
49. Peel LR, Stevens GMW, Daly R, Daly CAK, Lea JSE, Clarke CR, et al. Movement and residency patterns of reef manta rays Mobula alfredi in the Amirante Islands, Seychelles. Mar Ecol Prog Ser. (2019) 621:169–84. doi: 10.3354/meps12995
50. Andrzejaczek S, Chapple TK, Curnick DJ, Carlisle AB, Castleton M, Jacoby DM, et al. Individual variation in residency and regional movements of reef manta rays Mobula alfredi in a large marine protected area. Mar Ecol Prog Ser. (2020) 639:137–53. doi: 10.3354/meps13270
51. Harris JL, Stevens GM. Environmental drivers of reef manta ray (Mobula alfredi) visitation patterns to key aggregation habitats in the Maldives. PLoS ONE. (2021) 16:e0252470. doi: 10.1371/journal.pone.0252470
52. Venables SK, Duinkerken DI, Rohner C, Marshall AD. Habitat use and movement patterns of reef manta rays Mobula alfredi in southern Mozambique. Mar Ecol Prog Ser. (2020) 634:99–114. doi: 10.3354/meps13178
53. Couturier LIE, Newman P, Jaine FRA, Bennett MB, Venables WN, Cagua EF, et al. Variation in occupancy and habitat use of Mobula alfredi at a major aggregation site. Mar Ecol Prog Ser. (2018) 599:125–45. doi: 10.3354/meps12610
54. Armstrong AO, Armstrong AJ, Bennett MB, Richardson AJ, Townsend KA, Dudgeon CL. Photographic identification and citizen science combine to reveal long distance movements of individual reef manta rays Mobula alfredi along Australia's east coast. Mar Biodivers Rec. (2019) 12:14. doi: 10.1186/s41200-019-0173-6
55. Fonseca-Ponce IA, Zavala-Jiménez AA, Aburto-Oropeza O, Maldonado-Gasca A, Galván-Magaña F, González-Armas R, et al. Physical and environmental drivers of oceanic manta ray Mobula birostris sightings at an aggregation site in Bahía de Banderas, Mexico. Mar Ecol Prog Ser. (2022) 694:133–48. doi: 10.3354/meps14106
56. Cabral MMP, Stewart JD, Marques TA, Ketchum JT, Ayala-Bocos A, Hoyos-Padilla EM, et al. The influence of El Niño Southern Oscillation on the population dynamics of oceanic manta rays in the Mexican Pacific. Hydrobiologia. (2022) 22:9. doi: 10.1007/s10750-022-05047-9
57. Clark TB. Abundance, Home Range, and Movement Patterns of Manta Rays (Manta alfredi, M. birostris) in Hawai'i. Honolulu, HI: University of Hawai'i at Manoa (2010).
58. Andrzejaczek S, Schallert RJ, Forsberg K, Arnoldi NS, Cabanillas-Torpoco M, Purizaca W, et al. Reverse diel vertical movements of oceanic manta rays off the northern coast of Peru and implications for conservation. Ecol Solut Evid. (2021) 2:e12051. doi: 10.1002/2688-8319.12051
59. Stewart JD, Hoyos-Padilla EM, Kumli KR, Rubin RD. Deep-water feeding and behavioral plasticity in Manta birostris revealed by archival tags and submersible observations. Zoology. (2016) 119:406–13. doi: 10.1016/j.zool.2016.05.010
60. Burgess KB, Couturier LIE, Marshall AD, Richardson AJ, Weeks SJ, Bennett MB. Manta birostris, predator of the deep? Insight into the diet of the giant manta ray through stable isotope analysis. R Soc Open Sci. (2016) 3:160717. doi: 10.1098/rsos.160717
61. Knochel AM, Cochran JEM, Kattan A, Stevens GMW, Bojanowksi E, Berumen ML. Crowdsourced data reveal multinational connectivity, population demographics, and possible nursery ground of endangered oceanic manta rays in the Red Sea. Aquat Conserv Mar Freshw Ecosyst. (2022) 2022:aqc.3883. doi: 10.1002/aqc.3883
62. Hosegood J, Humble E, Ogden R, de Bruyn M, Creer S, Stevens GM, et al. Phylogenomics and species delimitation for effective conservation of manta and devil rays. Mol. Ecol. (2020) 2020:15683. doi: 10.1111/mec.15683
63. Perryman RJ, Venables SK, Tapilatu RF, Marshall AD, Brown C, Franks DW. Social preferences and network structure in a population of reef manta rays. Behav Ecol Sociobiol. (2019) 73:114. doi: 10.1007/s00265-019-2720-x
64. Hight BV, Lowe CG. Elevated body temperatures of adult female leopard sharks, Triakis semifasciata, while aggregating in shallow nearshore embayments: evidence for behavioral thermoregulation? J. Exp Mar Biol Ecol. (2007) 352:114–28. doi: 10.1016/j.jembe.2007.07.021
65. Jirik KE, Lowe CG. An elasmobranch maternity ward: female round stingrays Urobatis halleri use warm, restored estuarine habitat during gestation. J Fish Biol. (2012) 80:1227–45. doi: 10.1111/j.1095-8649.2011.03208.x
66. Hussey NE, Kessel ST, Aarestrup K, Cooke SJ, Cowley PD, Fisk AT, et al. Aquatic animal telemetry: a panoramic window into the underwater world. Science. (2015) 348:1255642. doi: 10.1126/science.1255642
67. Brownscombe JW, Griffin LP, Chapman JM, Morley D, Acosta A, Crossin GT, et al. A practical method to account for variation in detection range in acoustic telemetry arrays to accurately quantify the spatial ecology of aquatic animals. Methods Ecol Evol. (2020) 11:82–94. doi: 10.1111/2041-210X.13322
68. Swadling DS, Knott NA, Rees MJ, Pederson H, Adams KR, Taylor MD, et al. Seagrass canopies and the performance of acoustic telemetry: implications for the interpretation of fish movements. Anim Biotelemetry. (2020) 8:8. doi: 10.1186/s40317-020-00197-w
69. Manta Trust. How to Swim With Manta Rays. Swim Manta Rays. (2024). Available at: https://swimwithmantas.org (accessed May 12, 2024).
70. Kasmidi M, Gunadharma NA. Diving in Manta Sandy? Here's What You Need to Know!. (2017). Available at: https://birdsheadseascape.com/diving/diving-manta-sandy-heres-need-know-meidiarti-kasmidi-nikka-amandra-gunadharma/ (accessed September 20, 2021).
71. Fernando D, Stewart JD. High bycatch rates of manta and devil rays in the “small-scale” artisanal fisheries of Sri Lanka. PeerJ. (2021) 9:e11994. doi: 10.7717/peerj.11994
72. Government of Raja Ampat. Regency Law 9/2012 on Fishing Ban on Sharks, Manta Rays, and Certain Fish Species in Raja Ampat Waters. Waisai: Government of Raja Ampat (2012).
73. Indonesian Ministry of Marine Affairs and Fisheries. Ministerial Decree 4/2014 on the Full Protection of Manta Rays in Indonesian Waters. Jakarta (2014).
74. Ceccarelli DM, Lestari AP, Rudyanto A, White AT. Emerging marine protected areas of eastern Indonesia: coral reef trends and priorities for management. Mar Policy. (2022) 141:105091. doi: 10.1016/j.marpol.2022.105091
75. Putra MIH, Setyawan E, Laglbauer BJL, Lewis S, Dharmadi D, Sianipar A, et al. Predicting mobulid ray distribution in coastal areas of Lesser Sunda Seascape: implication for spatial and fisheries management. Ocean Coast Manag. (2020) 198:105328. doi: 10.1016/j.ocecoaman.2020.105328
76. Lezama-Ochoa N, Pennino MG, Hall MA, Lopez J, Murua H. Using a Bayesian modelling approach (INLA-SPDE) to predict the occurrence of the Spinetail Devil Ray (Mobula mobular). Sci Rep. (2020) 10:18822. doi: 10.1038/s41598-020-73879-3
Keywords: spatial connectivity, passive acoustic telemetry, marine megafauna, site fidelity, marine protected areas (MPAs), endangered species (EN), network analysis
Citation: Setyawan E, Mambrasar R, Sianipar AB, Hasan AW, Putra MIH, Mofu I, Beale CS and Erdmann MV (2024) Insights into the visitations of oceanic manta rays at cleaning stations on coral reefs in the Bird's Head Seascape, eastern Indonesia. Front. Fish Sci. 2:1432244. doi: 10.3389/frish.2024.1432244
Received: 13 May 2024; Accepted: 29 July 2024;
Published: 27 August 2024.
Edited by:
Julia L. Y. Spaet, University of Cambridge, United KingdomReviewed by:
David M. Tickler, University of Western Australia, AustraliaJessica Pate, Marine Megafauna Foundation, United States
Charles Bangley, Dalhousie University, Canada
Copyright © 2024 Setyawan, Mambrasar, Sianipar, Hasan, Putra, Mofu, Beale and Erdmann. This is an open-access article distributed under the terms of the Creative Commons Attribution License (CC BY). The use, distribution or reproduction in other forums is permitted, provided the original author(s) and the copyright owner(s) are credited and that the original publication in this journal is cited, in accordance with accepted academic practice. No use, distribution or reproduction is permitted which does not comply with these terms.
*Correspondence: Edy Setyawan, ZWR5c2V0eWF3YW5AZ21haWwuY29t