- 1Department of Veterinary Sciences, University of Torino, Grugliasco, Italy
- 2Department of Life Sciences and Systems Biology, University of Turin, Turin, Italy
Introduction: The breeding of ornamental fish is increasingly widespread across the world. For this reason, assessing the welfare of ornamental fish in aquariums is becoming ever more important. This study wants to evaluate, through minimally invasive analyses, behavioral observations, and an analysis of cortisol concentrations in the water, how the absence or presence of environmental enrichment (EE) influences the welfare of guppies (Poecilia reticulata).
Methods: In total, 15 tanks, divided by EE level [without, with low (25%), and with high (75%) levels of EE], were considered. Fish were video-recorded and behavioral analyses were carried out. In addition, the cortisol concentrations in each tank's water were evaluated.
Results: In the tanks with higher EE, behaviors such as wood, flotation, and plant feeding and courting, which are indicators of a good quality of life, were significantly more expressed. Bite behavior was also expressed more in high-level EE tanks, probably as a response to the territoriality of animals. The only stereotypy behavior, a stress index, was found to be significantly more expressed in tanks with low levels of EE. Finally, cortisol concentrations were found to be significantly higher in tanks with low levels of EE.
Discussion: In light of these results, we conclude that fish in tanks with higher EE levels showed better welfare statuses. Considering the use of EE in tanks is therefore useful for enabling these fish to express their species-specific behavioral repertoire and improve their quality of life.
1 Introduction
In recent years, the breeding and trade of ornamental fish has been an increasing trend, and this industry is valued at between $15 and $30 billion per year (1, 2). Indeed, 90% of the traded ornamental fish species are freshwater (~4,500 species), and 99% of the 2 billion fish transported annually are intended for hobbyists' tanks, with the remaining 1% going to research and public aquariums (1, 3). From this perspective, the ornamental fish industry has considerable economic importance. In addition, the lack of knowledge of ornamental fish management techniques increases the risk of overexploiting the wild environment (2), and the damage from introducing non-native species into an environment is a potential risk to the native species' ecology (4). Moreover, the social importance of the ornamental fish industry should be also considered. The presence of an aquarium in the home, health care facilities, and workplaces has been shown to have a positive impact on psychophysical wellbeing. Studies have revealed that observing fish in an aquarium can reduce heart rate, increase mood and attention levels, and decrease anxiety and stress levels (5). In addition, aquariums have been found to have positive effects on older adults, particularly those with dementia, resulting in an increased interest in independent feeding and a decreased need for nutritional supplements (6, 7).
If the link between humans' welfare and aquaria ecosystems is well established (8), ornamental fish's welfare should also be taken into account, as sentient animals experience different states of welfare (1, 64). The ornamental fish breeding production chain and trade involve several stages, each with a variety of animal welfare concerns. Problems arise when welfare standards are changed at various stages of trade and are not maintained between the places of departure and arrival. Unfortunately, there are no species-specific monitoring protocols for ornamental fish welfare as there are for fish raised for food (9). In fact, there are 11 European Council (EC) Directives on aquaculture of food fish regarding the management of all productive chains [EC No 98/58, No 1099/2009, No 1007/2009, No 1/2005, No 1255/97, No 708/2007, No 1005/2008, No 1224/2009, No 1342/2008, No 1006/2008, No 1223/2009; (10)] but no species-specific directives for ornamental fish. Accordingly, for food-raised fish, the European Food Safety Authority (11) published 17 documents, between scientific opinions and reports, and ornamental fish are named in only two documents as genetically modified animals and in a podcast on animal perfect nutrition. Stress prevention is thus the only and easiest way to breed ornamental fish.
Monitoring animal welfare for ornamental fish becomes vital, considering also that maltreatment and, consequently, stress lead to a lowering of the animal's immune system and, therefore, more susceptible fish (12). Prevention is thus the cheapest and easiest way to raise healthy fish while minimizing or eliminating stress whenever possible (12). Thanks to glass tanks, monitoring ornamental fish's welfare is easier using visual and non-invasive parameters, such as behavior, color, and wounds. For this reason, behavior evaluation is one of the most promising tools for assessing fish's physical and psychological welfare. Evaluating behavior, indeed, is less invasive and can be completed by different professional figures (64). In addition to behavior, among physiological parameters, cortisol levels are one of the most used molecular markers to assess welfare. Stressful stimuli animates adaptive endocrine responses with the release of cortisol. This glucocorticoid hormone is produced by adrenocortical cells (13) and is usually measured to assess the response to stress and evaluate welfare. Generally, glucocorticoids can be extracted from different biological matrices, of which sampling may be more or less invasive: plasma, saliva, hair, feathers, milk, eggs, urine, and feces (14). In fish, it can also be estimated from the gills (15, 16) and the tank's water. Water is a very useful and easy matrix because obtaining a sample is completely non-invasive for the animals (13).
Like other animals (17), for ornamental fish, environmental enrichment (EE), together with parameters such as water quality, photoperiod, or tank size, is essential for maintaining a good welfare state (3). There are different types of EE, but the setting and choice of enrichment must be made considering the bred species (63). EE could be nutritional, occupational, physical, sensorial, and social. Knowing each species is important for properly balancing the different types of enrichment (18). When assessing welfare, it also should be considered that the genotypic profile of fish, selected for domestication, affects the stress response (3). In guppy species, for example, the density of animals in the tank impacts the fish's mental welfare (65).
The guppy, Poecilia reticulata, is one of the most widespread and popular species of ornamental fish in aquariums, private and public. Moreover, the welfare indicators and parameters considered for this species are suitable and studied for other species, such as zebrafish (65, 66). Originally from South America, guppies are now distributed almost everywhere. It is mainly bred in Southeast Asia and Central America and, from there, exported to the applicant countries (65).
From these assumptions, it is evident that, with so many fish farmed and sold, studying their biology, ecology, and behavior to ensure their welfare and increased survival is essential (18). Thus, the main objective of this study was to assess, through non-invasive tools such as behavioral analyses and cortisol concentration in water, the impact of including natural physical EE at three different levels in tanks on the welfare of guppies. The aim is therefore to increase knowledge and provide clear guidelines for effective tank management to improve guppies' quality of life.
2 Materials and methods
The study was conducted at the Aquaculture Laboratory (@acquaponica_lab) of the Department of Veterinary Sciences (University of Turin, Italy). The study was approved by the University Bioethics Committee (protocol number 0654239). All methods were carried out following the relevant guidelines and regulations.
2.1 Fish acclimation
In all, 400 guppies were purchased from the ornamental fish company Tuscia Fish Trading (Viterbo, Italy). Upon arrival, the guppies underwent a 7-day quarantine period in a 100-L aquarium to facilitate acclimatization and observe for the possible presence of pathologies.
2.2 Experimental protocol and housing
Using ImageJ software (19), 360 adult guppies were selected for homogeneous size and divided into 18 tanks (60 L/tank); 15 tanks were experimental, and three hosted the fish reserves (one for each treatment). Each tank contained 20 fish maintaining a sex ratio of 1:1 and respecting the guppy density of one fish every 3 L of water (20).
The balanced monofactorial experimental design (5 × 3) included five replication tanks for three treatments, with different percentages of EE compared to the total volume of each tank. The EEs were selected according to da Silva et al. (21). A neutral white substrate was used (Amtra Ivory white quartz 1–3 mm) in addition to wood on the bottom. Ornamental plants (Myriophyllum aquaticum and Pistia stratiotes) were selected to re-create as similarly as possible the guppies' original South American aquatic environment (22). The 15 experimental tanks were equipped according to three levels of EE:
• Absence (A), empty tank (Figure 1a).
• Low level (L); on the bottom of the tank, 30 mm of substrate (Amtra Ivory white quartz 1–3 mm) and one oak wood (25–30 cm; Figure 1b) were added.
• High level (H); the same substrate and wood on the bottom were used as the low-level tanks, plus two different ornamental plants, selected to re-create a natural environment as similar as possible to the South America one (22): Myriophyllum aquaticum (N = 4) and Pistia stratiotes (N = 6; Figure 1c).
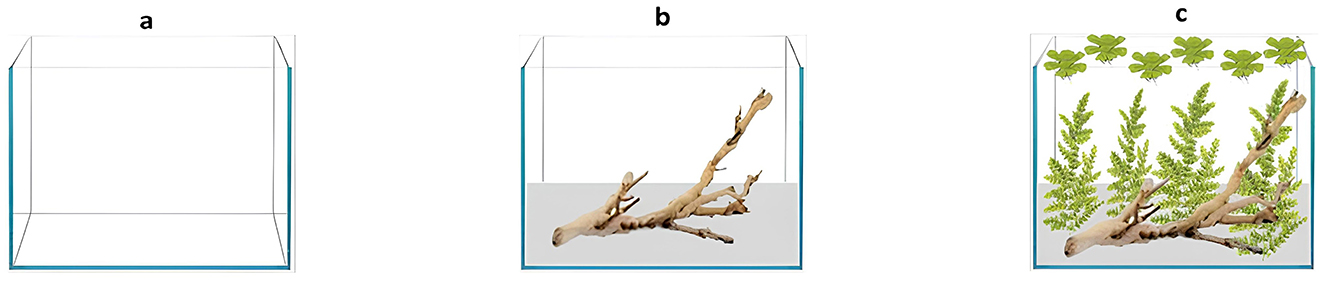
Figure 1. Different levels of enrichment in the experimental tanks. (A) Absence of environmental enrichment. (B) Low level of environmental enrichment (Amtra Ivory white quartz as substrate and one oak wood). (C) High level of environmental enrichment (Amtra Ivory white quartz as substrate, one oak wood, and two different ornamental plants, Myriophyllum aquaticum and Pistia stratiotes).
Each tank was equipped with a 100-W heater to keep the temperature at 24°C, respecting the optimal water temperature range between 22 and 26°C for guppy (20). Every tank had an individual filter containing a 600 L/h submersible water pump, with Perlon® wool as the mechanical filter and lava rock as the biological filter. Lights (Oase classic daylight) were used to have the same illumination level in each tank and were set to 12 h of light and 12 h of dark (20, 23, 24). All guppies were fed ad libitum for 5 days a week twice a day (around 9:00 a.m. and 5:00 p.m.) using a species-specific commercial food (Tetra guppy Mini Flakes® proximal composition: raw protein 44%, raw fats 8.0%, raw cellulose 2.0%, moisture content 6.0%, vit. D3 3170IU/Kg, manganese (II) sulfate monohydrate 32 mg/kg, zinc sulfate monohydrate 19 mg/kg, and iron (II) sulfate monohydrate 13 mg/kg). A partial water change (10% of the total) in the tanks was done once a week, with monthly filter cleaning.
Following Kithsiri et al. (25), the experiment lasted 55 days, from March 15 to May 9, 2023, for a total of 8 weeks. All maintenance practices and sampling were carried out between 9:00 a.m. and 1:00 p.m.
2.3 Water physical and chemical analysis
Upon fish arrival, the following water characteristics were evaluated: saturation (SAT., %), dissolved oxygen (DO, mg/L), pH, ammonium (NH4), nitrites (NO2), nitrates (NO3), and temperature (TEMP., °C).
During the trial, the physical and chemical parameters of the water were monitored weekly with a Spectroquant NOVA 60 spectrophotometer and an XS Instruments Oxy 7 Vio field oximeter. From each aquarium, 50 mL of water was taken; placed in a numbered Eppendorf conical tube to minimize disturbing the fish when taking the water sample, which was done only once; and divided among 15-mL Eppendorf conical tubes for various tests in the morning. To determine the pH, a Hach pH meter and a 3-mL sample were used. Ammonium, nitrites, and nitrates were analyzed with the required tests for reading with the Spectroquant physical and chemical parameters.
2.4 Fish behavioral observation and data collection
The recorded behaviors were sampled using the focal animal scan sampling method (26) to analyze behaviors between three groups. Behavioral data recording was preceded by preliminary observations of all the fish present in the aquariums aimed at identifying and selecting the behaviors to be included in the ethogram employed in this study, starting from the ethogram of Martins et al. (27).
Once a week, between 9:00 a.m. and 1:00 p.m. (the period of greatest motility of the guppies), the behaviors of the fish in the A, L, and H groups were recorded, and each section of the tank was sampled for 5 min (Figure 2) using a video camera (Sony Handycam FDR-AX43) positioned on a stand at 1 m from the tank.
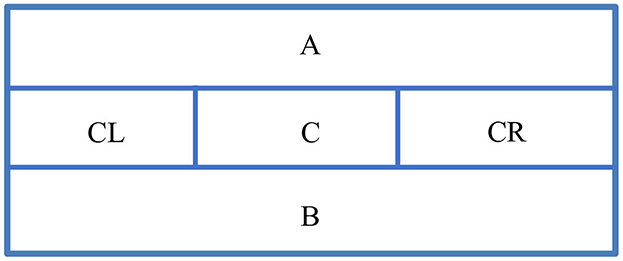
Figure 2. Tank division. (A) upper section. (B) Lower section. (C) Central section. CR, central-right section; CL, central-left section.
Each tank was recorded for 5 min once a week (for a total of 600 min). The first and last minutes of the videos were discarded, as the behavioral findings could be altered by the presence of the operator positioning, starting, and then turning off the camera after 5 min. The remaining 3 min of the videos were observed and analyzed for each section of each tank, for a total of 1,800 min analyzed.
The behaviors were divided into macro categories: motor, feeding, stereotypies, social, and aggressive (Table 1). Motor behaviors are related to moving in the surrounding environment. For feeding behaviors, wellbeing indicators include the time dedicated to feeding on different surfaces. Stereotypies are repeated behaviors according to a fixed pattern exhibited by the animal, apparently without a stimulus evoking them, that highlight a state of stress and can be aimed at reducing this state (28): hiding, standing still, remaining stopped with increasing ventilatory activity, and swimming from right to left or from above to below continuously. Social behaviors, which are typically assessed to evaluate welfare, include courtship and group movement in guppies. In contrast, aggressive behaviors, such as biting and mouth-to-mouth biting, could indicate stress within the social group (27, 29).
The interactions between fish (social or aggressive social behaviors), according to a species-specific ethogram (30), and the characterization of fish interaction with EE were recorded in an Excel spreadsheet (Microsoft Office 365, Excel version 2406). The final table used for fish behavior analyses was structured in this way: group, date, duration of observed behaviors (minutes of video starts and ends), sex, state or event behavior, and the tank number.
2.5 Cortisol analyses
When the fish arrived, a water sample was taken from each bag to evaluate the cortisol concentration. After the acclimatization period (first week), another water sample was taken for chemical-physical analyses and cortisol detection. During the sampling period, a water sample was collected once every 2 weeks (T0–T4) from each of the 15 tanks in 10-mL Falcon tubes, appropriately labeled, and stored at −20°C until analyzed.
Extracting the cortisol from the water was carried out following the protocol proposed by Ellis et al. (31). Initially, 5 mL of water was filtered using 0.20-μm filters to eliminate any impurities; then, the extraction phase was carried out by passing the filtered water drop by drop into an extraction cartridge (Oasis HLB, Water Ltd). To complete the extraction, the cartridge was eluted with 5 mL of ethyl acetate, flowed into the cartridge dropwise, and collected in a 10-mL glass tube. The ethyl acetate was evaporated under a stream of nitrogen at 45°C until complete evaporation. The tubes were finally closed with Parafilm® and stored at −20°C for subsequent analyses.
For quantifying the free cortisol concentrations, samples were reconstituted in 500 μL of Phosphate-Buffered Saline (PBS) and analyzed using a commercial immunoenzyme Enzyme-Linked Immunoorbent Assay (ELISA) kit (DRG Cortisol ELISA Kit, DRG Diagnostic International, Inc.), following the assay protocol. The DRG Cortisol ELISA kit used for the analyses is designed to determine the quantity of cortisol and has a sensitivity from 0 to 800 ng/mL. It also shows negligible cross-reactivity with other steroids of similar structure: corticosterone (45%), progesterone (9%), and deoxycortisol (2%).
2.6 Statistical analysis
In the first part of statistical elaboration, descriptive statistics were used and graphed, including histograms and box plots. In the second part, inferential statistics were used to test the differences between experimental treatments. The normality distribution of data was initially investigated using the kernel density function (KDE). This plot type estimates the probability density function using a smoothing parameter to create a continuous, smooth curve. KDE is useful for visualizing the distribution shape of the data without relying on bin-based histograms. By overlaying a normal distribution curve, one can compare the actual data distribution against the expected normal shape. Considering that the data on fish behaviors were not distributed according to normal distribution, non-parametric statistic techniques were utilized for these elaborations, in particular non-parametric analysis of variance (ANOVA; Kruskal–Wallis test). The absolute frequency and time spent exhibiting the different behaviors during the day were also measured, thus creating the time budget for the guppies in the tanks with the different experimental treatments (32, 33). Cortisol data were elaborated using ANOVA and Tukey's honestly significant difference (HSD) post-hoc test. For the statistical analysis of the cortisol data, a regression method was used, including the calculation of Pearson correlation coefficients. The statistical analysis was performed using R (version 4.3.1, “Beagle Scouts”). Program libraries for performing the Kruskal–Wallis test were taken from rgl package (34), the ggplots 2 package (35) was used to perform the multiple correlation analysis, and the programming codes in R were used for the descriptive statistics graphs and box plots.
3 Results
3.1 Water analysis
During the experimental period, mean values (±SD) of the analyzed parameters of waters were 8.23 (±0.27) pH, 0.12 (±0.07) mg/L NH4, 0.04 (±0.01) mg/L NO2, 9.51 (±4.46) mg/L NO3, 6.72 (±0.63) mg/L DO, 24.98 (±0.68) °C TEMP, and 83.16 (±8.27) % SAT. The mean values of DO (% SAT and mg/L DO), pH, NH4, NO2, NO3, and temperature (°C) of experimental tanks during the trial are reported in Table 2.
The analyzed parameters show adequate levels of welfare for fish maintained in a controlled environment, as reported by current aquariology legislation.
3.2 Behavioral evaluation
During the whole experiment, no mortality was observed in the guppies. Behavioral results are presented as time, time budget, and frequency. The analysis of the data's normal distribution was initially made to verify the assumptions for correctly applying ANOVA using kernel density plots (Figure 3). By overlapping a normal distribution curve, one can compare the actual data distribution against the expected normal shape. This analysis clearly showed that our data did not fit with normal distribution. In the next phase of statistical analysis, differences between experimental treatments across all observed behaviors were examined and results were shown through box plots, one for each behavior. To simplify interpreting the results, only those behaviors that resulted statistically different are shown in Table 3, and only box plots representing significantly different behaviors are displayed in Figures 4–8.
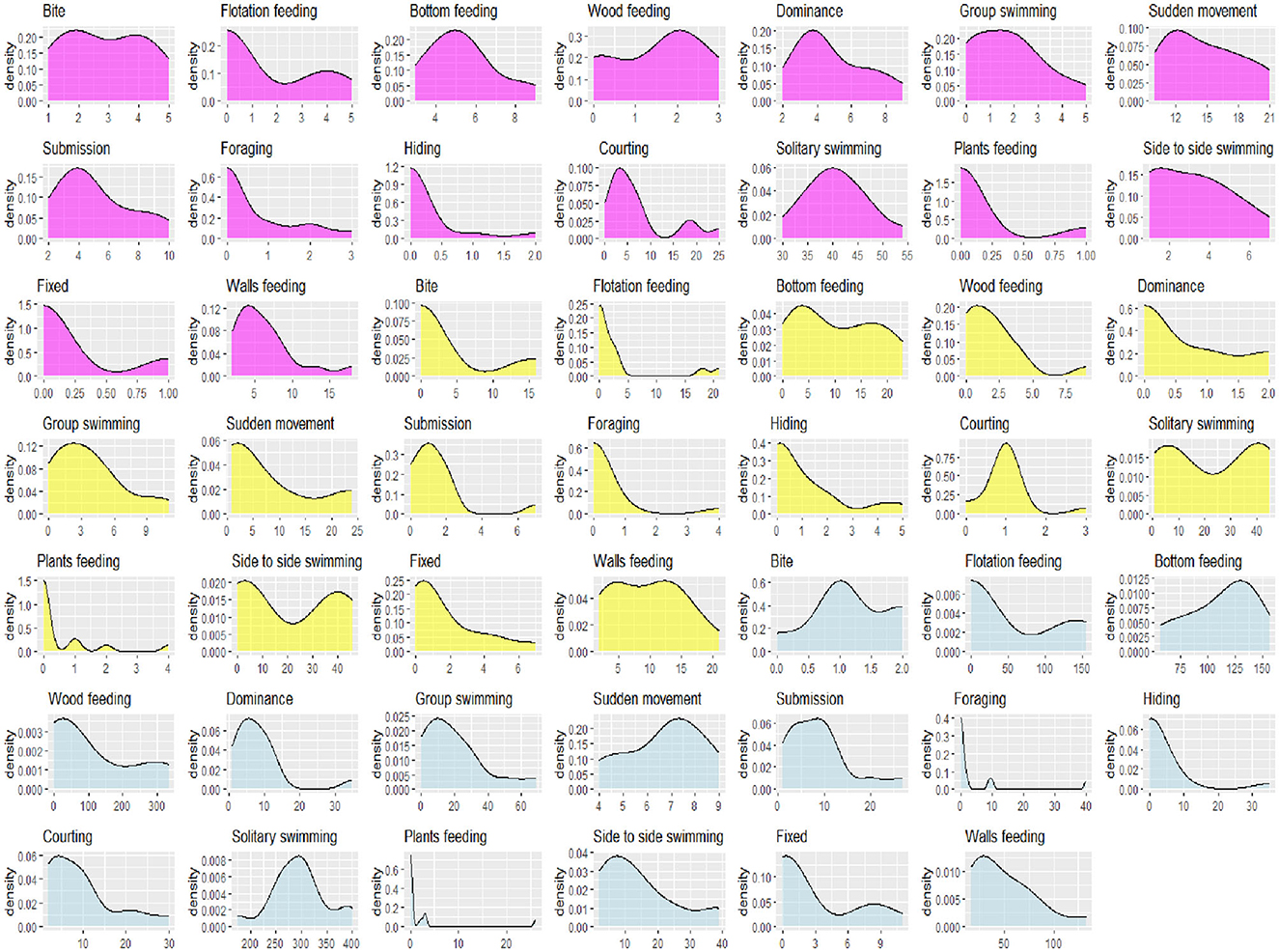
Figure 3. Data normality assessment using kernel density estimation (KDE) plots. Yellow, magenta, and light blue represent time, time budget, and frequency in each, respectively.
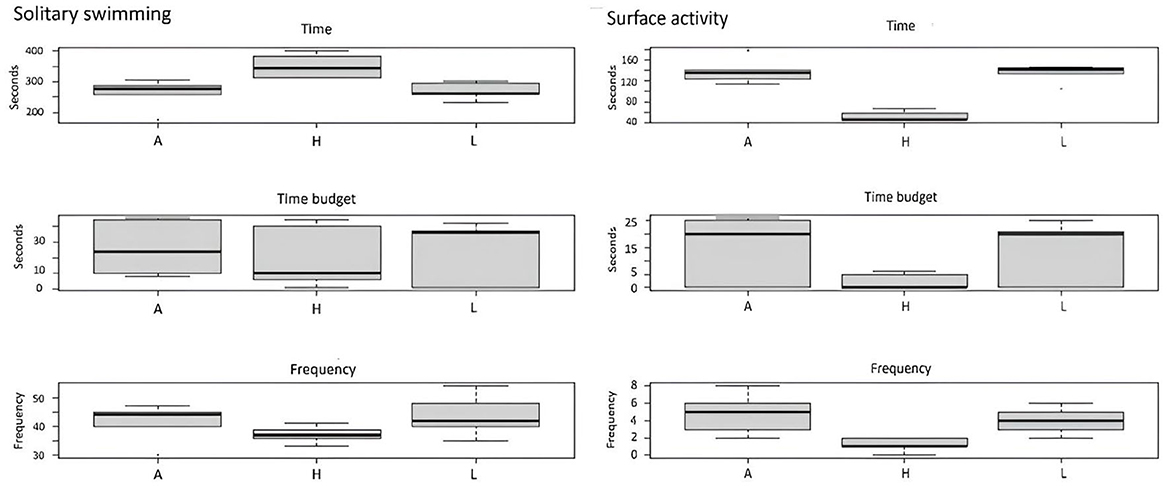
Figure 4. Effect of enrichment level (A, absence; H, high; L, low) on motor behaviors (solitary swimming and surface activity).
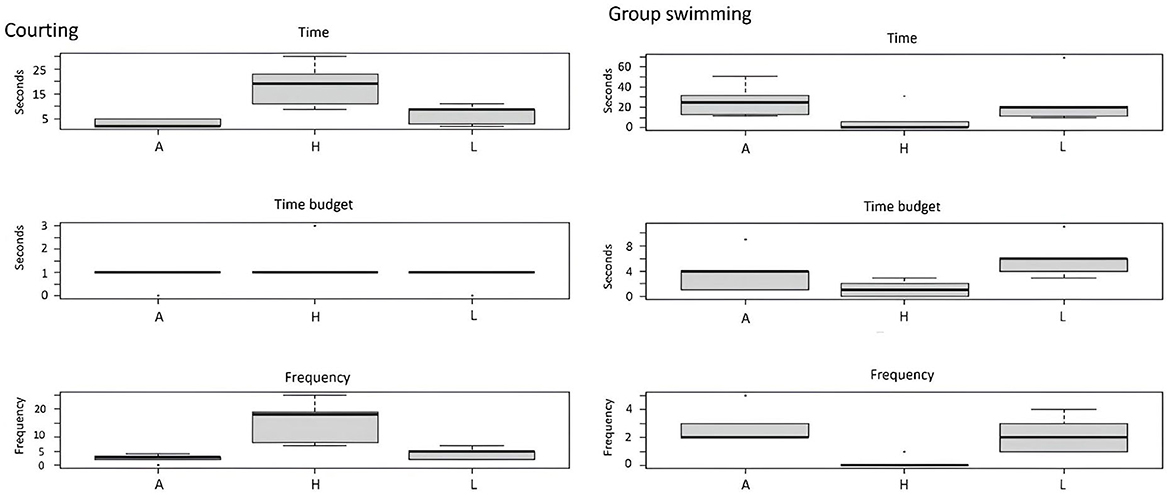
Figure 5. Effect of enrichment level (A, absence; H, high; L, low) on social behaviors (courting and group swimming).
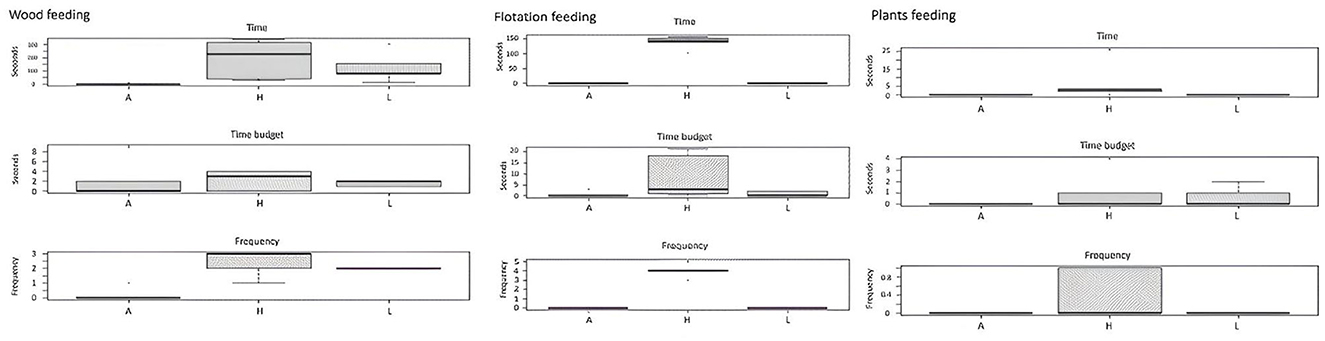
Figure 7. Effect of enrichment level (A, absence; H, high; L, low) on feeding (woods, flotation and plants) behaviors of guppy.
For motor behaviors, the difference between the H tanks and the others was found to be significant for solitary swimming time and surface activity time and frequency (Figure 4). In the aquaria with high EE, fish spent more time solitary swimming and were rarely observed near the water's surface.
For social behaviors, the time and frequency of courting were found to be significantly higher in tanks with higher levels of EE. However, regarding time budget and frequency, group swimming was lower in H tanks (Figure 5).
Regarding aggressive behaviors, they were not affected by EE; only bite was partially influenced. In fact, this behavior was more frequent in the tanks with higher levels of EE (Figure 6).
In the A group, where fish did not have EE, behaviors implying interactions with plants or wood were not detectable, but in the L and H groups, fish with higher EE (H tanks) showed greater percentages of feeding behaviors: wood feeding, flotation feeding, and, of course, plant feeding. This finding indicates that a greater variety of EE (plant species, wood, and floating objects) stimulates natural behaviors in fish, such as feeding, in the H aquaria (Figure 7).
For stereotypies, only hiding behavior resulted in a significant difference between the three experimental conditions; considering the time in particular, in the aquaria with low EE, fish spent more time hiding (Figure 8).
3.3 Cortisol evaluation
The data elaboration for the cortisol analysis was carried out following three steps: differences of cortisol during the experiment, differences of cortisol in each sampling period, and, finally, correlation between cortisol levels in the four considered sampling periods.
The analysis of the individual bags and tanks during the experiment showed a statistically significant difference (p > 0.01) for cortisol levels in the transport water bags (37.77 ± 8.0 ng/mL, n = 15) compared to the tanks' water (25.99 ± 5.8 ng/mL, n = 75) at all times, regardless of the sampling period (Figure 9). The successive statistical analysis of the cortisol data during the experiment, considering differences from the first day (T0) through the end of the experiment (T4), showed no difference in cortisol levels in the time trend.
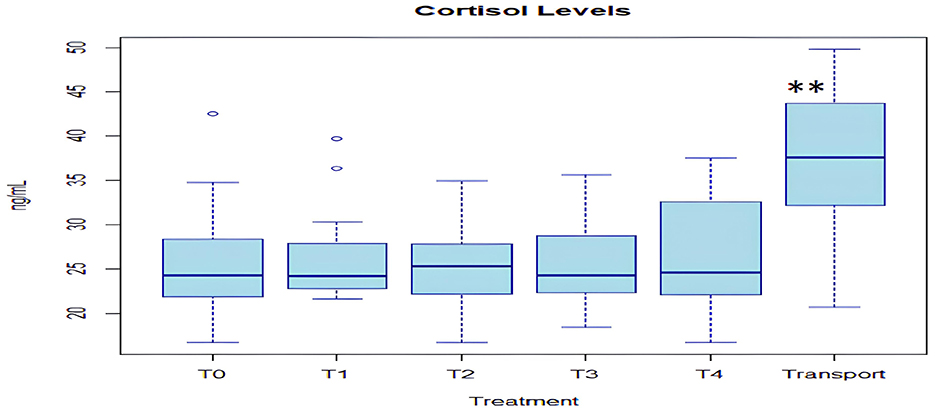
Figure 9. Cortisol levels during transport bags and experimental period (superscript asterisks correspond to analysis of variance test; p < 0.01).
The analysis of the cortisol levels in tanks in relation to enrichment condition in each sampling period shows that the cortisol concentration in the aquaria absent EE (A tanks) was systematically higher (ANOVA, p < 0.01) than in the aquaria with EE (H and L tanks), regardless of the enrichment level at T1, T3, and T4 (Figure 10). Moreover, at T0 and T2, the cortisol levels are different between all three treatments, but treatment A still had the highest concentration of cortisol, although it was not significant. Finally, the Tukey post-hoc test showed that the cortisol levels in the aquaria with EE were often comparable. This finding clearly shows EE's beneficial effect in managing guppy.
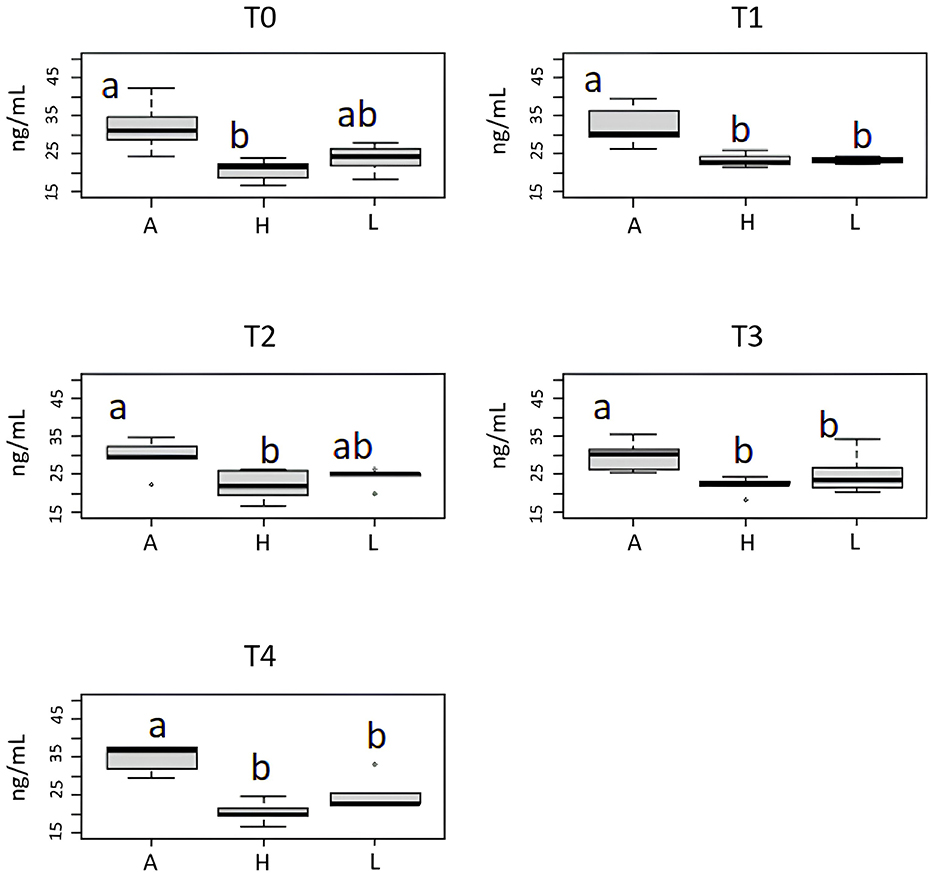
Figure 10. Effect of enrichment level (A, absence; H, high; L, low) on cortisol levels in tank's water of guppy within any sampling period (T0, T1, T2, T3, T4). Superscript letters on box plots correspond to the Tukey post-hoc test.
The results within the sampling periods provide interesting information from this study, and multiple correlation, together with scatterplots, is an optimal descriptive method for this (36). Therefore, to better investigate the cortisol dynamics throughout the experiment, a multiple correlation analysis was performed, considering the four sampling periods (Figure 11). Observing the scatterplots, it is evident that cortisol levels in the tanks without treatments (A tanks) are consistently higher than in the others (blue dots in Figure 11, which are always between 30 and 35 ng/mL), along all the experiment duration, while in the aquaria with EE, the cortisol levels are systematically lower (Figure 11: L tanks, red dots, and H tanks, yellow dots, that are both between 20 and 25 ng/mL). The variability in the experiment progressively increases during the experiment, as visible by the scatterplots and by the decreasing regression coefficients. Specifically, this variability is caused by the cortisol levels in the aquaria equipped with EE. This condition, measured only in aquaria with EE, shows that the fish's physiological reaction to EE is affected by other environmental factors. The frequency histograms in the five sampling periods basically show a constant positive skewness that confirms the existence of outlier values, which correspond to the aquaria without EE. The frequency histogram in the last period (T4) with a bimodal pattern confirms the separation between aquaria without EE from other aquaria. The progressive reduction in Pearson correlation coefficients of cortisol values analyzed between different periods (Figure 11) indicates the previously described change in the physiological reaction of fish along with the duration of the experiment.
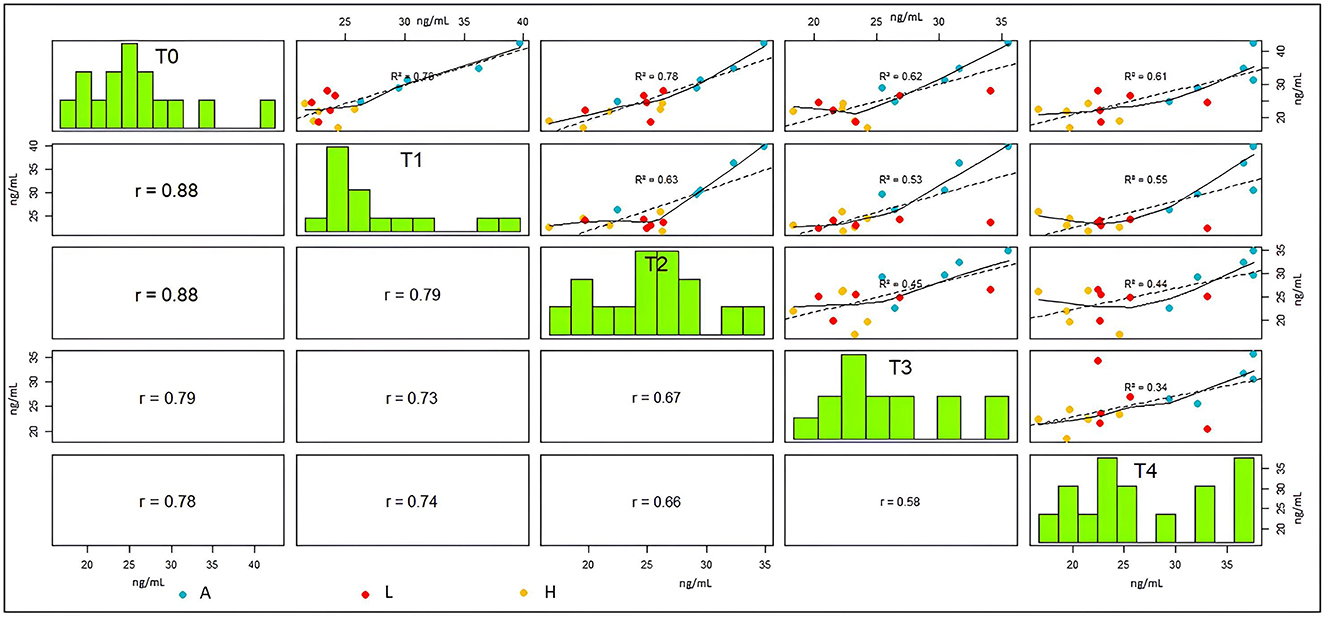
Figure 11. Cortisol analysis, multiple correlation, and frequency distribution in the sampling periods. In the upper part of the figure, linear regression was calculated and regression coefficients; red dots indicate cortisol levels in the low-level environmental enrichment (L) tanks, blue dots for the tanks absent (A) EE, and yellow dots for the high-level environmental enhancement (H) tanks. On the diagonal, the frequency histogram of cortisol levels is shown in each period. In the lower part of the figure, Pearson correlation coefficients are shown, with the size of the character proportional to the Pearson value (Pearson test significant in all plots).
4 Discussion
The present study aimed to investigate the role and importance of the environment on the welfare and quality of life of ornamental fish. The study showed that EE plays a crucial role in this context. Behavioral assessment, combined with the analysis of cortisol concentrations in water, can be an accurate tool for assessing the welfare of ornamental fish.
Stress is defined as an organism's adaptive response to a stressor, such as an environmental condition (37). The effects of the stressful event (stressor) can lead to an adaptive response and, therefore, to a positive stress response (eustress), but if the stressor persists or the animal fails to adapt, the response becomes negative (distress). Behavior reflects the adaptive response to stressors, and therefore, its assessment may be crucial for detecting poor animal welfare early. In farmed fish, considering both the individual and the group is important because group indicators can mask stress and individual animal discomfort (27, 38). In our assessment, we included both individual and group behaviors. Our findings demonstrate that tank enrichment does not impact all behaviors. Most of them differed significantly in highly enriched tanks across all the categories considered.
Studies on fish welfare have found that stressful events lead to an increase in aggressive behaviors, which subsequently alter the fish group's social dynamics. In zebrafish (Danio rerio), Powell et al. (39) highlighted more aggressive behaviors and less cohesion and coordination within the school during and after cleaning procedures. The density of animals in tanks can also affect aggressive behaviors. Sen Sarma et al. (40) found that zebrafish kept at a density of 1 fish/L are more aggressive compared to those kept at densities of 3 or 6 fish/L. In this study, aggression decreased after 5 weeks in all tanks, once the hierarchy was stabilized.
Moreover, EE could be a stressor that leads to behavioral changes. The EE type and level affect fish's welfare (41). Graham et al. (42) showed that introducing enrichments modifies the social dynamics of zebrafish. Indeed, providing animals the opportunity to explore new environments and new enrichments reduces aggression and increases shoaling and coordination within the group. The same study showed that several days after introducing novelties into the environment, social dynamics return to their original state. In our study, we found a significant increase in solitary swimming and a significant decrease in group swimming in tanks with high EE levels. This modification of behaviors may be due to the physical spatial size of enrichments inside the tank, which can be an obstacle to group swimming. The literature reports that introducing EE reduces the overall activity of fish, with varying effects on aggressive behaviors (43).
Other behaviors that delineate welfare are those related to feeding. In our study, wood feeding, floatation feeding, and plant feeding were significantly more expressed in high-level EE tanks. Using aquatic vegetation as EE can provide food resources and safety for fish during foraging activities (44), increasing these activity types, allowing fish to express their natural behaviors, and enhancing their welfare status (21). Finally, an increase in these behaviors can lead to a decrease in surface activity, as we found in our study.
Aggressive bite behavior was expressed more in H tanks. This result coincides with the literature, and it can be attributed to different dynamics. In fact, some studies have demonstrated that introducing EE affects the aggressiveness of fish. In black rockfish (Sebastes melanops), an increase in EE leads to a decrease in aggressive behavior. For fat greenling, aggression increases at both low and intermediate levels of enrichment, while at high levels of enrichment, aggression decreases (41).
These data indicate that the complexity of enrichment influences the aggressive response of fish. If the density of enrichment in the tank is high, the objects and structures divide the space (45), reduce the frequency of encounters (46), obstruct vision (47), and provide places to hide (43). This “shelter effect,” therefore, reduces aggressiveness in fish (41). However, if the enrichment is limited in relation to animal density and space, territorial fish compete for resources, increasing aggressive behaviors accordingly (43, 48).
In addition, the physiological phase of fish also affects their interaction with the environment, such as during courtship when the male butterfly splitfins (Ameca splendens) increase their aggressiveness in the presence of EE (49). In line with this research, in our study, fish exhibited significantly higher levels of courting behaviors in highly enriched tanks, and bite behavior was associated with males fighting for females. Accordingly, at T4, some fries were present only in the H group. The increase in mating behavior and fry survival is a good indicator of animal welfare (50).
These findings suggest that fish in tanks with high levels of EE have better welfare standards. The significant increase in hiding, a stereotypied behavior, in tanks without EE confirms this hypothesis. Indeed, stereotypies are maladaptive behaviors that are expressed in high-stress environments (51).
Regarding water cortisol concentrations, the analyses revealed that the transport bags had greater hormone levels than any of the examined tanks. In fish, as in other animals, an increase in corticosteroid concentration is the primary physiological response to stress conditions (52). In studies on stress, plasma cortisol is the most common and widely utilized marker (53). It has been observed that plasma cortisol increases in fish being transported (54–58). Moreover, cortisol levels not only increase during the initial phase of transport but also remain high throughout the transport period (59, 60). The cortisol from the plasma is released into the water, allowing for a less invasive measurement of this parameter under stress conditions (13). In light of this research and our findings, we can conclude that transport is a stressful event.
Among all the tanks, the cortisol levels were significantly higher in those without enrichment throughout the study period, decreasing in tanks with low enrichment and, to a greater extent, in those with high enrichment levels. This result is consistent with the findings of Zhang et al. (48) and confirms that using EE can improve fish's welfare, as presented in the literature (61, 62).
To conclude, multiple indicators of stress, such as behavioral, physiological (cortisol concentrations in water), and overall fitness (reproductive activity success), can be used as an accurate tool for assessing the welfare of ornamental fish; accordingly, our findings demonstrate that tank enrichment levels affected fish behavior and water cortisol levels.
This study showed how using adequate (level and quality, such as natural origin) EE can improve the wellbeing of guppies. In the future, analyzing the use of EE in relation to reproductive performance, which is an index of welfare, may be interesting for improving the local production of this fish and contributing to the natural exploitation of ornamental fish for home aquariums.
Data availability statement
The original contributions presented in the study are included in the article/supplementary material, further inquiries can be directed to the corresponding author.
Ethics statement
The animal study was approved by Comitato di Bioetica d'Ateneo—Università di Torino. The study was conducted in accordance with the local legislation and institutional requirements.
Author contributions
IM: Investigation, Validation, Visualization, Writing – original draft, Writing – review & editing. BS: Conceptualization, Data curation, Formal analysis, Methodology, Project administration, Resources, Supervision, Visualization, Writing – review & editing. EM: Formal analysis, Methodology, Writing – review & editing. PP: Resources, Writing – review & editing. RC: Investigation, Validation, Writing – original draft. SC: Investigation, Validation, Writing – original draft. MT: Resources, Writing – review & editing. CM: Formal analysis, Methodology, Writing – original draft.
Funding
The author(s) declare that no financial support was received for the research and/or publication of this article.
Conflict of interest
The authors declare that the research was conducted in the absence of any commercial or financial relationships that could be construed as a potential conflict of interest.
Generative AI statement
The author(s) declare that no Gen AI was used in the creation of this manuscript.
Publisher's note
All claims expressed in this article are solely those of the authors and do not necessarily represent those of their affiliated organizations, or those of the publisher, the editors and the reviewers. Any product that may be evaluated in this article, or claim that may be made by its manufacturer, is not guaranteed or endorsed by the publisher.
References
1. Jones M, Alexander ME, Snellgrove D, Smith P, Bramhall S, Carey P, et al. How should we monitor welfare in the ornamental fish trade? Rev Aquac. (2021) 14:770–90. doi: 10.1111/raq.12624
2. Peh JH, Azra MN. A global review of ornamental fish and shellfish research. Aquaculture. (2024) 596:741719. doi: 10.1016/j.aquaculture.2024.741719
3. Stevens CH, Croft DP, Paull GC, Tyler CR. Stress and welfare in ornamental fishes: what can be learned from aquaculture? J Fish Biol. (2017) 91:409–28. doi: 10.1111/jfb.13377
4. Bernery C, Bellard C, Courchamp F, Brosse S, Leroy B. A global analysis of the introduction pathways and characteristics associated with non-native fish species introduction, establishment, and impacts. Ecol Process. (2024) 13:22. doi: 10.1186/s13717-024-00495-8
5. Bowler DE, Buyung-Ali LM, Knight TM, Pullin AS. A systematic review of evidence for the added benefits to health of exposure to natural environments. BMC Public Health. (2010) 10:456. doi: 10.1186/1471-2458-10-456
6. Edwards NE, Beck AM. Animal-assisted therapy and nutrition in Alzheimer's disease. West J Nurs Res. (2002) 24:697–712. doi: 10.1177/019394502320555430
7. Edwards NE, Beck AM. The influence of aquariums on weight in individuals with dementia. Alzheimer Dis Assoc Disord. (2013) 27:379–83. doi: 10.1097/WAD.0b013e3182769b34
8. Cracknell D, White MP, Pahl S, Nichols WJ, Depledge MH. Marine biota and psychological well-being: a preliminary examination of dose-response effects in an aquarium setting. Environ Behav. (2016) 48:1242–69. doi: 10.1177/0013916515597512
9. Dara M, Carbonara P, La Corte C, Parrinello D, Cammarata M, Parisi MG. Fish welfare in aquaculture: Physiological and immunological activities for diets, social and spatial stress on Mediterranean aqua cultured species. Fishes. (2023) 8:414. doi: 10.3390/fishes8080414
10. EUR-Lex (2024). Available online at: https://eur-lex.europa.eu/homepage.html (accessed November 16, 2024).
11. EFSA (2024). Available online at: https://www.efsa.europa.eu/it/publications/corporate (accessed November 16, 2024).
12. Magda S, Mercy TVA. “Health management in ornamental fish farming.” In: Jain AK, Saini VP, Kaur VI, , editors. Best Management Practices for Freshwater Ornamental Fish Production, National Fisheries Development Board. Ministry of Agriculture and Farmer's Welfare, GOI: Hyderabad, India (2016). p. 94–106.
13. Sadoul B, Geffroy B. Measuring cortisol, the major stress hormone in fishes. J Fish Biol. (2019) 94:540–55. doi: 10.1111/jfb.13904
14. Palme R. Monitoring stress hormone metabolites as a useful, non-invasive tool for welfare assessment in farm animals. Anim Wel. (2012) 21:331–7. doi: 10.7120/09627286.21.3.331
15. Secci G, Parisi G, Meneguz M, Iaconisi V, Cornale P, Macchi E, et al. Effects of a carbon monoxide stunning method on rigor mortis development, fillet quality and oxidative stability of tench (Tinca tinca). Aquaculture. (2018) 493:233–9. doi: 10.1016/j.aquaculture.2018.05.002
16. Gai F, Cusimano GM, Maricchiolo G, Caccamo L, Caimi C, Macchi E, et al. Defatted black soldier fly meal in diet for grow-out gilthead seabream (Sparus aurata L. 1758): effects on growth performance, gill cortisol level, digestive enzyme activities, and intestinal histological structure. Aquac Res. (2023) 1:1−18. doi: 10.1155/2023/3465335
17. Zentall TR. Effect of environmental enrichment on the brain and on learning and cognition by animals. Animals. (2021) 11:973. doi: 10.3390/ani11040973
18. Stevens CH, Reed BT, Hawkins P. Enrichment for laboratory zebrafish—a review of the evidence and the challenges. Animals. (2021) 11:698. doi: 10.3390/ani11030698
19. Kowalska J, Rawski M, Homska N, Mikolajczak Z, Kieronczyk B, Swiatkiewicz S, et al. The first insight into full-fat superworm (Zophobas morio) meal in guppy (Poecilia reticulata) diets: a study on multiple-choice feeding preferences and growth performance. Ann Anim Sci. (2022) 22:371–84. doi: 10.2478/aoas-2021-0072
20. Görelşahin S, Yanar M, Kumlu M. The effects of stocking density, tubifex feeding and monosex culture on growth performance of guppy (Poecilia reticulata) in a closed indoor recirculation system. Aquaculture. (2018) 493:153–7. doi: 10.1016/j.aquaculture.2018.05.004
21. da Silva A, Lima MR, Meletti PC, Jerep FC. Impact of environmental enrichment and social group size in the aggressiveness and foraging activity of Serrapinnus notomelas. Appl Anim Behav Sci. (2020) 224:104943. doi: 10.1016/j.applanim.2020.104943
22. Günther A. Catalogue of the Fishes in the British Museum. Vol. 6. London: Taylor and Francis (1866). p. 353.
23. Gasparini C, Marino IAM, Boschetto C, Pilastro A. Effect of male age on sperm traits and sperm competition success in the guppy (Poecilia reticulata). J Evol Biol. (2010) 23:124–35. doi: 10.1111/j.1420-9101.2009.01889.x
24. Portabales FV. Estudio de la influencia del fotoperiodo en la natación de los guppies (Poecilia reticulata). Anales Universitarios de Etologìa. (2013) 7:19–25.
25. Kithsiri HMP, Sharma P, Zaidi SGS, Pal AK, Venkateshwarlu G. Growth and reproductive performance of female guppy, Poecilia reticulata (Peters) fed diets with different nutrient levels. Indian J Fish. (2010) 57:65–71.
26. Altmann J. Observational study of behavior: sampling methods. Brill. (1974) 49:227–67. doi: 10.1163/156853974X00534
27. Martins CIM, Galhardo L, Noble C, Damsgård B, Spedicato MT, Zupa W, et al. Behavioural indicators of welfare in farmed fish. Fish Physiol Biochem. (2012) 38:17–41. doi: 10.1007/s10695-011-9518-8
28. Sevillano V, Fiske S. Stereotypes, emotions, and behaviors associated with animals: a causal test of the stereotype content model and BIAS map. Group Process Intergroup Rel. (2019) 22:879–900. doi: 10.1177/1368430219851560
29. Sneddon LU, Wolfenden DCC, Thomson JS. 12-stress management and welfare. Fish Physiol. (2016) 35:463–539. doi: 10.1016/B978-0-12-802728-8.00012-6
30. Crews J, Braude S, Stephenson C, Clardy T. The Ethogram and Animal Behavior Research. Washington University, St Louis (2002). p. 1–33.
31. Ellis T, James JD, Stewart C, Scott AP. A non-invasive stress assay based upon measurement of free cortisol released into the water by rainbow trout. J Fish Biol. (2004) 65:1233–52. doi: 10.1111/j.0022-1112.2004.00499.x
32. Marriner LM, Drickamer LC. Factors influencing stereotyped behavior of primates in a zoo. Zoo Biology. (1994) 13:267–75. doi: 10.1002/zoo.1430130308
33. Mallapur A, Chellam R. Environmental influences on stereotypy and the activity budget of Indian leopards (Panthera pardus) in four zoos in Southern India. Zool Biol. (2002) 21:585–95. doi: 10.1002/zoo.10063
35. Wickham H. (2016). Getting Started with ggplot2. In ggplot2: Elegant graphics for data analysis. Cham: Springer International Publishing. p. 11–31 doi: 10.1007/978-3-319-24277-4_2
36. Perperoglou A, Sauerbrei W, Abrahamowicz M, Schmid M. A review of spline function procedures in R. BMC Med Res Methodol. (2019) 19:1–16. doi: 10.1186/s12874-019-0666-3
37. Levine S. “A definition of stress?.” In: Animal Stress, , ed. Springer New York: New York, NY (1985). p. 51–69. doi: 10.1007/978-1-4614-7544-6_4
38. Herbert NA, Steffensen JF. The response of Atlantic cod, Gadus morhua, to progressive hypoxia: fish swimming speed and physiological stress. Mar Biol. (2005) 147:1403–12. doi: 10.1007/s00227-005-0003-8
39. Powell C, von Keyserlingk MA, Franks B. Tank cleaning temporarily increases stress and decreases affiliative behavior in zebrafish. App Anim Behav Sci. (2021) 242:105414. doi: 10.1016/j.applanim.2021.105414
40. Sen Sarma O, Frymus N, Axling F, Thörnqvist PO, Roman E, Winberg, S. Optimizing zebrafish rearing–effects of fish density and environmental enrichment. Front Behav Neurosci. (2023) 17:1204021. doi: 10.3389/fnbeh.2023.1204021
41. Zhang Z, Fu Y, Zhang Z, Zhang X, Chen S. A comparative study on two territorial fishes: the influence of physical enrichment on aggressive behavior. Animals. (2021) 11:1868. doi: 10.3390/ani11071868
42. Graham C, von Keyserlingk MA, Franks B. Free-choice exploration increases affiliative behaviour in zebrafish. Appl Anim Behav Sci. (2018) 203:103–10. doi: 10.1016/j.applanim.2018.02.005
43. Näslund J, Johnsson JI. Environmental enrichment for fish in captive environments: effects of physical structures and substrates. Fish Fish. (2016) 17:1–30. doi: 10.1111/faf.12088
44. Casatti L, Mendes HF, Ferreira KM. Aquatic macrophytes as feeding site for small fishes in the Rosana Reservoir, Paranapanema River, Southeastern Brazil. Braz J Biol. (2003) 63:213–22. doi: 10.1590/S1519-69842003000200006
45. Bilhete C, Grant JWA. Short-term costs and benefits of habitat complexity for a territorial fish. Ethology. (2016) 122:151–7. doi: 10.1111/eth.12456
46. Myhre LC, Forsgren E, Amundsen T. Effects of habitat complexity on mating behavior and mating success in a marine fish. Behav Ecol. (2013) 24:553–63. doi: 10.1093/beheco/ars197
47. Kadry VO, Barreto RE. Environmental enrichment reduces aggression of pearl cichlid, Geophagus brasiliensis, during resident-intruder interactions. Neotrop Ichthyol. (2010) 8:329–32. doi: 10.1590/S1679-62252010000200011
48. Zhang Z, Xu X, Wang Y, Zhang X. Effects of environmental enrichment on growth performance, aggressive behavior and stress-induced changes in cortisol release and neurogenesis of black rockfish Sebastes schlegelii. Acquaculture. (2020) 528:735483. doi: 10.1016/j.aquaculture.2020.735483
49. Kelley JL, Magurran AE, García CM. Captive breeding promotes aggression in an endangered Mexican fish. Biol Conserv. (2006) 133:69–177. doi: 10.1016/j.biocon.2006.06.002
50. Conte FS. Stress and the welfare of cultured fish. Appl Anim Behav Sci. (2004) 86:205–23. doi: 10.1016/j.applanim.2004.02.003
51. Mason GJ, Latham N. Can't stop, won't stop: is stereotypy a reliable animal welfare indicator? Anim Wel. (2004) 13:S57–59. doi: 10.1017/S096272860001438X
52. Wells RMG, Pankhurst NW. Evaluation of simple instruments for the measurement of blood glucose and lactate, and plasma protein as stress indicators in fish. J World Aquac Soc. (1999) 30:276–84. doi: 10.1111/j.1749-7345.1999.tb00876.x
53. Iwama GK, Afonso LOB, Vijayan MM. “Stress in fishes.” In: Evans, DH, Claiborne, JB, , editor. The Physiology of Fishes. CRC Press, Boca Raton, FL (2006). p. 319–42.
54. Gomes LC, Roubach R, Araujo-Lima CARM, Chippari-Gomes AR, Lopes NP, Urbinati EC. Effect of fish density during transportation on stress and mortality of juvenile tambaqui Colossoma macropomum. J World Aquac Soc. (2003) 34:76–84. doi: 10.1111/j.1749-7345.2003.tb00041.x
55. Acerete L, Balasch JC, Espinosa E, Josa A, Tort L. Physiological responses in Eurasian perch (Perca fluviatilis, L.) subjected to stress by transport and handling. Aquaculture. (2004) 237:167–78. doi: 10.1016/j.aquaculture.2004.03.018
56. Urbinati EC, Carneiro PCF. Sodium chloride added to transport water and physiological responses of matrinxã brycon amazonicus (teleost: characidae). Acta Amazon. (2006) 36:569–72. doi: 10.1590/S0044-59672006000400020
57. Dhanasiri AKS, Fernandes JMO, Kiron V. Liver transcriptome changes in zebrafish during acclimation to transport-associated stress. PLoS ONE. (2013) 8:e65028. doi: 10.1371/journal.pone.0065028
58. Jones M, Alexander ME, Lightbody S, Snellgrove D, Smith P, Bramhall S, et al. Influence of social enrichment on transport stress in fish: a behavioural approach. App Anim Behav Sci. (2023) 262:9. doi: 10.1016/j.applanim.2023.105920
59. Aupérin B, Baroiller JF. “Teleost fish handling and transport under reduced stress conditions.” In: Ozouf-Costaz C, Pisano E, Foresti F, Foresti LAT, , editors. Fish Cytogenetic Techniques Foresti. CRC Press: New York (2015). p. 1–10. doi: 10.1201/b18534-2
60. Sampaio FD, Freire CA. An overview of stress physiology of fish transport: changes in water quality as a function of transport duration. Fish Fish. (2016) 17:1055–72. doi: 10.1111/faf.12158
61. Arechavala-Lopez P, Cabrera-Álvarez MJ, Maia CM, Saraiva JL. Environmental enrichment in fish aquaculture: a review of fundamental and practical aspects. Rev Aquac. (2022) 14:704–28. doi: 10.1111/raq.12620
62. Marcon M, Mocelin R, Benvenutti R, Costa T, Herrmann AP, de Oliveira DL, et al. Environmental enrichment modulates the response to chronic stress in zebrafish. J Exp Biol. (2018) 221:jeb176735. doi: 10.1242/jeb.176735
63. Zhang Z, Gao L, Zhang X. Environmental enrichment increases aquatic animal welfare: a systematic review and meta-analysis. Rev Aquac. (2022) 14:1120–35. doi: 10.1111/raq.12641
64. Stevens C, Fiddes M, Rose P. The behavioural biology of freshwater fishes. In: Rose P, , editor. The Behavioural Biology of Zoo Animals. Boca Raton, FL: CRC Press (2022). p. 237–52.
65. García LN, Giraldo-Gongora PA. The effect of aquarium size/volume on the reproduction of the guppy fish Poecilia reticulata (Peters, 1859). Aquac Aquar Conserv Legis. (2023) 16:1483–87.
Keywords: guppy, welfare, environmental enrichment, behavior, cortisol
Citation: Manenti I, Sicuro B, Macchi E, Ponzio P, Crosetto R, Cavallari S, Tarantola M and Mugnai C (2025) Effect of different levels of environmental enrichment on the welfare of guppy (Poecilia reticulata). Front. Fish Sci. 3:1581493. doi: 10.3389/frish.2025.1581493
Received: 22 February 2025; Accepted: 25 March 2025;
Published: 09 April 2025.
Edited by:
Jose Luis Muñoz, University of Los Lagos, ChileReviewed by:
Patrick Astruch, GIS Posidonie, FranceCristina Velasco Rubial, University of Vigo, Spain
Copyright © 2025 Manenti, Sicuro, Macchi, Ponzio, Crosetto, Cavallari, Tarantola and Mugnai. This is an open-access article distributed under the terms of the Creative Commons Attribution License (CC BY). The use, distribution or reproduction in other forums is permitted, provided the original author(s) and the copyright owner(s) are credited and that the original publication in this journal is cited, in accordance with accepted academic practice. No use, distribution or reproduction is permitted which does not comply with these terms.
*Correspondence: Isabella Manenti, aXNhYmVsbGEubWFuZW50aUB1bml0by5pdA==