- 1Department of Psychology, Faculty of Philosophy, University of Novi Sad, Novi Sad, Serbia
- 2John Jay College of Criminal Justice, New York, NY, United States
- 3Mount Holyoke College, South Hadley, MA, United States
- 4Faculty of Medicine, University of Novi Sad, Novi Sad, Serbia
- 5Department of Neurology, School of Medicine, New York University, New York City, NY, United States
- 6Institute of Molecular Genetics and Genetic Engineering, University of Belgrade, Belgrade, Serbia
Epigenetic modifications of the membrane bound catechol-O-methyltransferase (MB-COMT) gene may affect the enzymatic degradation of dopamine, and consequently, human behavior. This study investigated the association between membrane bound catechol-O-methyltransferase DNA methylation (DNAm) differences in 92 monozygotic (MZ) twins with phenotypic manifestations of cognitive, behavioral, and personality indicators associated with reward-related behaviors and lack of control. We used pyrosequencing to determine DNAm of the regulatory region of membrane bound catechol-O-methyltransferase in saliva DNA. Results of intrapair differences in the percentage of membrane bound catechol-O-methyltransferase DNAm at each of five CpG sites show that there are associations between phenotypic indicators of lack of control and membrane bound catechol-O-methyltransferase DNAm differences on CpG1, CpG2 and CpG4, suggesting the common epigenetic patterns for personality traits, cognitive functions, and risk behaviors.
Introduction
An important contribution to the understanding of individual differences in behavior is provided by studies of epigenetic processes, such as the methylation of DNA sequences, which actively regulate the expression of the genetic information present in the human genome (Roth, 2013). In certain genetic regions, cytosines adjacent to guanines in CpG dinucleotides can be methylated and in this way limit access of the transcription machinery to the gene sequence. A growing body of evidence suggests that environmental stimuli can modulate DNA methylation (DNAm) patterns and the corresponding levels of expression in various genes, establishing a connection between environmental factors and biological effects including behavior (Abdolmaleky et al., 2004). From a genetic and neurophysiological perspective, many psychological traits, such as impulsivity and related behavior, are linked to changes in the dopaminergic system (DeYoung, 2013). However, an important challenge for the identification of potential susceptibility genes for impulsive behaviors arises due to different results between candidate gene studies (CGAS) and genome-wide association studies (GWAS). For example, many CGAS have found associations between aggression and dopaminergic genes, such as DAT, DRD2, DRD4, COMT, while none of GWAS related to these phenomena achieved genome-wide significance (Fernàndez-Castillo & Cormand, 2016). Moreover, CGA studies have shown importance of the interaction between COMT and DRD2 genes, suggesting that high COMT activity and low D2 receptor density is associated with high impulsivity scores in smokers (Reuter et al., 2006), while such findings have not yet been confirmed in GWAS. In general, given the conservative GWAS significance threshold, they are underpowered to detect most associated SNPs. For example, the GWAS study of personality traits showed only six replicable genetic variants associated with personality, and five of which are novel (Lo et al., 2016). Although previous CGAS studies have suggested potential associations between personality traits, cognitive functions, risk behaviors, and dopamine genes (Reuter et al., 2006; Soeiro-De-Souza et al., 2013; Gurvich & Rossell, 2015; Fernàndez-Castillo & Cormand, 2016), an important research question addressed in this study relates to the association between different indicators of impulsivity and changes in DNAm in genes encoding proteins with important function in behavior.
MB-COMT gene and dopamine regulation
Genes responsible for dopamine metabolism, transport and cellular reception have an important function in regulating all reward-related behaviors (DeYoung, 2013) include the catechol-O-methyltransferase (MB-COMT) gene, located on chromosome 22q11-q12, that plays a substantial role in the enzymatic degradation of dopamine (Dickinson & Elvevåg, 2009). MB-COMT is found in high concentrations within the prefrontal cortex of the brain and it has been found to determine personality, behavior inhibition, thinking, short-term memory, and emotion regulation (Chen et al., 2004; Abdolmaleky et al., 2006). MB-COMT-regulated processes account for approximately 60% of metabolic degradation of dopamine (Karoum et al., 1994), and phenotypic features commonly associated with MB-COMT enzyme activity include personality traits, cognitive abilities, and various behavioral indicators of lack of control (Dreher et al., 2008; Wacker et al., 2012; DeYoung, 2013). Most studies investigating variation in MB-COMT activity have looked into the presence of specific polymorphisms in this gene, rs4680, which encodes the substitution of valine (Val) by methionine (Met) at codon 158 (Val158Met substitution, see Rakvåg et al., 2008). The Met158 allele is strongly associated with lower abundance, stability, and activity of the MB-COMT enzyme. Carriers of the Val variant have a higher rate of dopamine degradation and this may be associated with the urge to constantly activate the reward system (Chen et al., 2004; Dreher et al., 2008). A recent meta-analysis on the functionality of dopamine gene variants has shown that MB-COMT Val158Met polymorphism has the clearest functional associations with dopamine regulation (Tunbridge et al., 2019). In addition to genetic variation, some studies have suggested that differential DNAm around the transcription start site of MB-COMT can play an important role on this gene’s expression and ultimately, its activity levels (Schreiner et al., 2011).
MB-COMT gene and executive functions
Studying in the field of neuroepigenetics could provide valuable information regarding the specific mechanisms by which a gene is expressed or silenced in relation to neuropsychological phenotypes. Executive function refers to a set of interconnected processes required for a purposeful, goal-oriented behavior (Anderson, 2002; Hughes & Graham, 2002; Anderson et al., 2008; Miyake & Friedman, 2012; Diamond, 2013). Since this highly complex and integrated set of cognitive abilities includes many cognitive processes, such as planning, goal setting, task initiation, task monitoring, ability to inhibit or delay responses, evaluation of responses, cognitive flexibility, and selection of efficient strategies necessary for problem-solving (Luria 1966; Welsh et al., 1991; Zelazo et al., 1997; Anderson, 2002), their study relies on different methods, frequently without a clear conceptual or empirical framework. Previous research has shown an association between executive dysfunction and impulsivity (Bickel et al., 2012), suggesting that impulsivity may result from a failure or dysfunction of the executive system. Although some studies found an association between the MB-COMT promoter methylation and prefrontal activity (Ursini et al., 2011; Córdova-Palomera et al., 2014; Walton et al., 2014; Lewis et al., 2019), several others reported contradictory results (Gomes et al., 2012; Schiepers et al., 2012). More specifically, while studying healthy controls Ursini et al. (2011) found an association between DNA methylation at S-COMT and prefrontal activity during a working memory task. This study found that methylation of the high-activity Val allele is inversely related to MB-COMT expression and therefore partially compensates for its negative effects on prefrontal cognition and activity. Moreover, DNAm differences in MB-COMT, DBH, the dopamine transporter gene (DAT1) and two dopamine receptor genes (DRD1 and DRD2) were associated with twin differences in inhibitory control (Lewis et al., 2019). However, in another study of older individuals with ages between 50–70 years, the global DNAm profile of leukocytes was not associated with cognitive function in the domains of memory, sensorimotor speed, complex speed, information speed and word fluency (Schiepers et al., 2012).
MB-COMT gene and aggression
Previous research supported the hypothesis that MB-COMT genotypes modify the sensitivity of the environment that confers either risk or protective factors for aggression (Wang et al., 2018). The effect of gene-environment interaction is also highlighted in the recent meta-analysis that showed significant relationships between aggression and blood DNAm levels that were associated with expression levels of various genes previously linked to problematic behaviors (van Dongen et al., 2021). Moreover, the same DNAm levels associated with aggression were also associated with other risk behaviors (e.g., smoking, alcohol consumption and other chemical exposures, see van Dongen et al., 2021). In the comparison of blood DNAm levels within aggression-discordant MZ twin pairs, van Dongen et al. (2015) found no genome-wide significant DNAm differences, but they mapped three top ranking sites located near different genes. Importantly, these sites had a low heritability (up to 10%) and showed mean within-pair difference in DNAm percentage of 0.8%–1.8% (van Dongen et al., 2021). While this study did not identify MB-COMT as an important gene, previous genetic studies have identified associations of polymorphisms in this gene with various risk behaviors, including aggression (for a review see Fernàndez-Castillo & Cormand, 2016). This suggests it is important to further explore the role of MB-COMT DNAm on these behaviors.
MB-COMT gene and personality traits
The revised reinforcement sensitivity theory (rRST; Gray and McNaughton, 2000), the most prominent conceptual frameworks in the field of “personality neuroscience”, provides plausible explanations of reactions to signals of reward and punishment, as well as reactions to the estimated danger in the form of fight, flight or freeze. Within the rRST, the Behavioral Inhibition System (BIS) is defined as the basis for the processing of conflicting stimuli, corresponding to anxiety; the Behavioral Approach System (BAS) is responsible for reactions to all appetitive stimuli, corresponding to impulsivity. Fight/Flight/Freeze system (FFFS) is the fear-related, underlying, defensive reaction to present threats, in the form of reactions such as confrontation, escape, or a temporary blocking (e.g., Gray and McNaughton, 2000). The dopamine system contributes to cognitive, sensory, and social perception, as well as cognitive and emotional processing of stimuli, which are key to responding to environments, according to rRST theory. Therefore, it is reasonable to assume that personality traits which are likely to be partially regulated by the MB-COMT gene, such as impulsivity (BAS) (Smederevac et al., 2022) and reactive aggression (Fight) as a response to a perceived threat (Reuter et al., 2006), will also be associated with differences in the level of MB-COMT DNAm. Moreover, previous studies have shown evidence that associate MB-COMT DNAm with personality disorders (Dammann et al., 2011; Thomas et al., 2019).
Current study
Different research designs can be used to examine epigenetic changes, but the most significant advantage is derived from the co-twin design, which includes monozygotic twins (MZ twins). That design is based on the assumption that MZ twins share 100% of their DNA sequence (i.e., also DNA outside genes), and all the differences between them can be attributed to the influence of environmental factors. However, recent evidence points to the fact that the DNA of some MZ twins is not identical (e.g., Jonsson et al., 2021), which raises important methodological and conceptual questions for behavioral genetics. The effect of environmental factors, which can accumulate over time, resulting in significant phenotypic differences between MZ twins in adulthood (Fraga et al., 2005), as well as differences in their epigenome (Van Dongen et al., 2021). The main goal of the present study is to determine associations between differences in DNAm in the promoter region of the MB-COMT gene and differences in phenotypic manifestations associated with reward-related behaviors and lack of control between MZ twins. Previous studies have shown that impulsivity is a multidimensional construct, covering indicators such as impulsive choices, impulsive actions, and impulsive personality traits (MacKillop et al., 2016). Therefore, we used various psychological measures as cognitive and behavioral indicators of lack of control, as well as personality dimensions defined by the rRST (Gray and McNaughton, 2000) to encompass a wide range of phenotypic features. Personality traits defined by rRST represent a comprehensive measure of strategies for responding to environmental challenges, which may contribute to epigenetic changes. Indicators of cognitive aspects of control are WCST (Heaton et al., 1993) measures of executive functions, while behavioral indicators are represented by the frequency of risk behaviors, such as cigarette, alcohol, or drug abuse, as well as aggression under provocation induced by Competitive Reaction Time Task (CRTT; Warburton and Bushman, 2019). We hypothesized that differences between MZ twins on various indicators of lack of control would be associated with significant differences in MB-COMT gene DNAm. This assumption is possible for all phenotypic indicators that can be expressed as continuous measures, such as personality traits, executive functions, and aggressive reactions. However, since the study includes phenotypic characteristics that have different manifestations, we assumed that objectively measurable indicators, such as risky behaviors, can be examined by determining concordant and discordant MZ pairs, and by studying the association between intrapair phenotypic concordance/discordance and intrapair differences in DNAm on five MB-COMT gene sites.
Materials and methods
Sample
The entire procedure for recruitment, testing, and data collection within Serbian Twin Advanced Registry (STAR) is described elsewhere (Smederevac et al., 2019). From the STAR Registry which contains data on 1,654 participants (827 twin pairs), all monozygotic twin pairs with data on all relevant phenotypic measures and MB-COMT (rs4680) genotyping were selected. After excluding some cases due to failed genotyping, this sample consisted of 432 twins. To investigate whether DNAm differences were associated with behavioral phenotypic features, we selected a subsample of 92 (46 pairs) monozygotic twins (MZ) for whom buccal swab DNA was of good quality for methylation analysis. The population studied here includes 24 male and 68 female twins. The age of the participants ranged from 18 to 44 years old, with a mean age of 23.38 ± 6.28 years old. Due to incomplete data for some twins, the number of twin pairs depended on the analysis. Participation was voluntary and for all participants informed written consent have been obtained prior to the participating in the study. The research was approved by the Institutional Ethical Committees (codes: #02-374/15, #01-39/229/1, #O-EO-024/2020).
Phenotypic measures
Reinforcement sensitivity questionnaire
Reinforcement Sensitivity Questionnaire (RSQ; Smederevac et al., 2014) is based on the Revised Reinforcement Sensitivity Theory (Gray & McNaughton, 2000) and contains 29 items distributed amongst five scales: Behavioral inhibition system—BIS (7 items, α = 0.65), Behavioral activation system—BAS (6 items, α = 0.75), and Fight/Flight/Freeze system—FFS (with 5 items each, α = 0.81, 0.44, 0.61, respectively). Items are rated on a four-point Likert scale (1 = completely disagree to 4 = completely agree).
Wisconsin card sorting test
Wisconsin Card Sorting Test (WCST; Heaton et al., 1993) is the most prominent test for the assessment of set-shifting, attention, and inhibition. The test assesses the possibility of creating and changing the principles of categorization, using the task of classifying a series of cards according to one of the three classification criteria: color, form, and a number of elements. The variables used in this study were: number of categories completed, number of perseverative errors, number of non-perseverative errors, and failures to maintain set.
Competitive reaction time task
Competitive Reaction Time Task (CRTT; Warburton and Bushman, 2019) is an experimental procedure for aggression induction. Throughout the procedure, twins were led to believe they were competing with each other in the reaction time tasks. Before each task, each twin had an opportunity to set the “punishment” for his/her twin pair. The punishment consisted of the settled intensity (on a scale from 0 = no punishment, 1 = 60 db to 10 = 105 db) and duration of an aversive noise (on a scale from 1 = 0.5 s to 10 = 5 s, see Dinić et al., 2020). After the punishment was established, the researchers began the competition. The slower twin received the punishment determined by the faster, winning twin. There were four blocks in the procedure (each contained 10 trials), with the first block designed as practice in which twins only administered the punishment and did not receive it if they were slower. In blocks 2 through 4, twins received predetermined punishments, which increased during the procedure: in the second block Mintensity = 70 db (60–75) and Mduration = 0.75 s (0.5–1); in the third block Mintensity = 85 db (80–90) and Mduration = 2 s (1.5–3); and in the fourth block Mintensity = 100 db (95–105) and Mduration = 4.2 s (3.5–5). In each block, twins randomly won in 50% of the tasks. Settled punishment in the 1st block refers to unprovoked aggression and the rest of the blocks to provoked aggression, punishment intensity refers to explicit, direct physical aggression and punishment duration to implicit, indirect aggression, although these outcomes could be combined (e.g., Giancola and Chermack, 1998).
Data on risk behaviors
Risk behaviors were assessed using three self-reported questionnaires about cigarette smoking, alcohol, and drug consumption. Participants also answered whether or not they consume alcohol, use drugs, and smoke cigarettes. The answers were categorized as 1 (yes) and 0 (no).
Zygosity analysis
Zygosity was determined using Investigator 24plex GO! Kit (Qiagen®, Valencia, CA, USA). DNA swab analysis was tested by STR multiplex amplification of the CODIS and ESS loci, SE33, DYS391 and Amelogenin without prior DNA extraction. Kit detects 21 autosomal and two gender markers, Amelogenin and DYS391. Samples with partial profiles were interpreted if at least 10 loci had results. The amplified loci then underwent capillary electrophoresis in the Applied Biosystems 3,500 Genetic Analyzer. The results were analyzed using Applied Biosystems GeneMapper ID-X software.
The genotyping of MB-COMT polymorphisms
The genotyping of the MB-COMT gene (rs4680) was carried out using TaqMan assays (TaqMan SNP, Applied Biosystems®, Warrington, United Kingdom), as recommended by the manufacturer. The TaqMan SNP Genotyping Assays uses TaqMan 5′ -nuclease chemistry for amplifying and detecting specific polymorphisms in purified genomic DNA samples and takes advantage of minor groove-binding probes for superior allelic discrimination.
Genotyping Assays contain a VIC-dye-labelled probe, a FAM-dye-labelled probe with two target-specific primers. PCR was performed using 10 ng of genomic DNA together with 1 µL of TaqMan Genotyping assay and 12.5 µL of the genotyping master mix in the final 25 µL reaction on a 96-well plate using an ABI Prism 7,500 Fast PCR device (Applied Biosystems®, Foster City, California, USA). MB-COMT gene (rs4680) alleles with the specific fluorescence curves were detected and analyzed using the 7,500 System SDS program, integrated into the ABI Prism 7,500 Fast PCR device.
The MB-COMT gene polymorphism was defined by 3 groups (Table 1): 130 high-activity homozygotes (Met/Met carriers), 225 intermediate heterozygotes (Met/Val carriers), and 77 low-activity homozygotes (Val/Val carriers). The MB-COMT gene polymorphism was in the Hardy-Weinberg equilibrium (HWE), with no significant differences between the observed and calculated genotype frequencies (χ2 = 4.13, df = 2, p > 0.05). However, results were the same for the HWE based on one member of a MZ twin pair [χ2 (df) = .913 (2); p > 0.05]. In actual sample the distribution of polymorphisms replicates the structure of the population from which it is sampled [χ2 (df) = .900 (2); p > .05].
DNA methylation assay
Genomic DNA was extracted out of buccal swabs using QIAamp DNA Mini Kit (Qiagen®, Valencia, CA, USA) according to the manufacturer’s extraction protocol. DNA was bisulfite converted using the EZ DNA Methylation-Gold kit (Zymo Research) following the kit’s protocol. Bisulfite-converted DNA was eluted in 15 μL of water and 2.5–5 μL were used for Pyromark PCR amplification (Qiagen). The assay targets a 228-bp DNA fragment in the MB-COMT promoter region (McGregor, 2014) (Figure 1). The forward primer had the following sequence 5′-TGGGGTAGATTAGGGTTGT-3′ and the reverse primer biotinylated in the 5′ end was 5′-CCACACCCTATCCCAATATTC-3′. Amplification conditions used were as recommended by the Pyromark PCR Kit (Qiagen). Pyrosequencing was carried out in a PyroMark Q24 (Qiagen) following the manufacturer’s instructions using 5′-GGATAGGGGAGGGTTTAGTT-3′ as the sequencing primer and the following sequence to analyze 5′-TYGGGYGGGTYGTYGYGGGAGAGGTGAGAG-3′. Pyrosequencing measured DNA methylation levels of five CpG sites indicated in Figure 1. DNA methylation analysis was performed using the PyroMark Q24 Advanced 3.0.0 software. DNA methylation status was reported as the average percent methylation for the four CpG sites, or DNA methylation level at an individual CpG sites as indicated in each analysis. Each amplification and pyrosequencing run included fully methylated and unmethylated DNA (Zymo Research) as controls. No-template controls were also included in all runs.
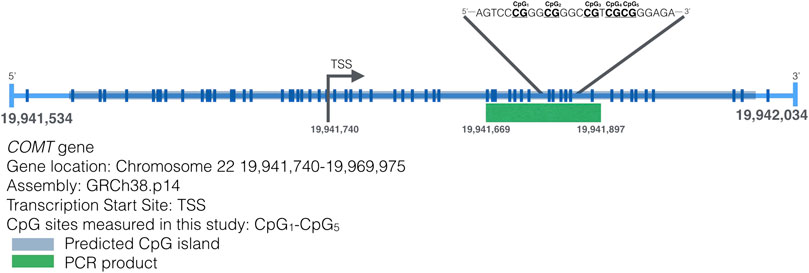
FIGURE 1. Map of the transcription start site of the MB-COMT gene and the CpG sites on that region. A 500bp sequence around the TSS of the COMT gene is presented. Lines represent CpG sites in the sequence. The inset highlights a sequence that includes the CpG sites measured in this study.
Statistical analysis
First, Friedman test was used to determine differences in DNA methylation percentage between individual CpG sites (data has marked deviations from normality).
Second, we conducted preliminary analyses to determine possible sex and age effects in phenotypic measures in order to apply appropriate correction of the scores. Phenotypes did not show a significant association with sex and age, except for three cognitive measures (percentage of responses at the conceptual level, perseverative errors, and non-perseverative errors) in which a significant sex effect was found. Thus, according to Bouchard and McGue (2003) regression technique, scores of these cognitive measures were partialized by sex and used in further analyses.
Next, given the sample size, we focused exclusively on the examination of differences between twins, avoiding analyzes that would lead to false positive findings, as they require much larger sample. Therefore, intrapair differences in the percentage of MB-COMT DNAm at each CpG site and mean CpG at all CpGs were calculated. For the analysis of associations with continuous phenotypic measures (personality and cognitive measures as well as laboratory-induced aggression), the differences in raw scores between twins in each pair were calculated. For the analysis of associations with categorical phenotypic measures (risk behaviors), we separated the twin pairs into two groups: twins that differ in the specific phenotype (discordant) versus twins that do not differ in the phenotype (concordant). For example, concordant twins are if both abuse or both do not abuse alcohol. Discordant are those in which one abuses and the other does not abuse alcohol.
Both skewness and kurtosis of these differences were positive and showed a violation of normal distribution (they are in a range from 3.88 to 6.60, i.e., outside the recommended range of ± 2 for a normal distribution, see Gravetter & Wallnau, 2017). Among intrapair differences in cognitive measures, all except non-perseverative errors showed high kurtosis (from 2.70 to 4.75), while intrapair differences in personality and aggression measures showed non-violation of normal distribution. Considering violation of normal distribution of intrapair differences in the MB-COMT DNAm and some phenotypic measures as well as that our sample is small, non-parametric tests in all analyses were applied. The associations between intrapair differences in continuous phenotypic measures and intrapair differences in DNAm levels were examined by computing Spearman rank correlation coefficients. Also, Mann-Whitney U-test was applied to determine differences between discordant and concordant twin pairs in intrapair differences in DNAm levels. To reduce Type I error, Bonferroni p-adjustment was calculated for applied 6 tests for each phenotypic measure (five CpG sites and mean CpG sites) as 0.05/6 = 0.0083. All analyses were conducted in SPSS IBM for Windows v.26 (IBM Corp, 2019).
Results
DNAm analysis and descriptive statistics
Five CpG sites were identified in the selected fragment (Chr22:19941740-19969975) of the promoter methylation region of the MB-COMT gene, as shown in Figure 1. There were significant differences across CpG sites—c2 (4, n = 92) = 159,36, p < 0.001, and in Figure 2 the mean and median of the percentage of DNA methylation for each of the five CpG sites are shown.
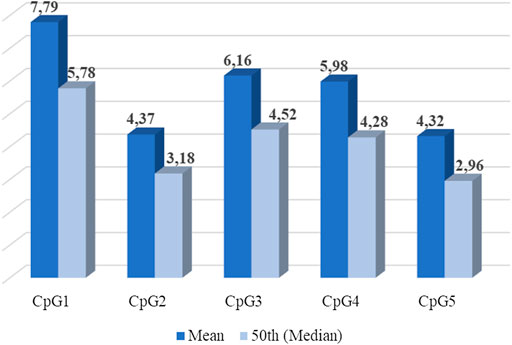
FIGURE 2. MB-COMT DNA methylation percentage among sites (Average percentage of DNA methylation for each of five CpG sites-mean and median).
CpG1 had a higher DNA methylation percentage than the other sites. Other pairwise comparisons are shown in Table 2.
Descriptive data of the percentage of MB-COMT DNAm and the phenotypic measures, intrapair differences in all measures, and the twin intrapair correlations are presented in Table 3. The intrapair correlations between MZ twins in all CpG sites are not significant, indicating no association in MB-COMT DNAm levels among twins (Table 3). The mean DNAm intrapair difference between MZ twins was around 4% (in a range between 3.88% and 4.94%) for all CpG sites, except for CpG 1, where the overall intrapair difference between MZ twins was 6.20 ± 6.60%. The intrapair correlations in cognition measures were not significant, indicating that MZ twins are not similar in specific aspects of cognitive functioning; however, interpair correlations in other phenotypic measures such as personality dimensions and laboratory-induced aggression were positive, significant, and of moderate to high strength.
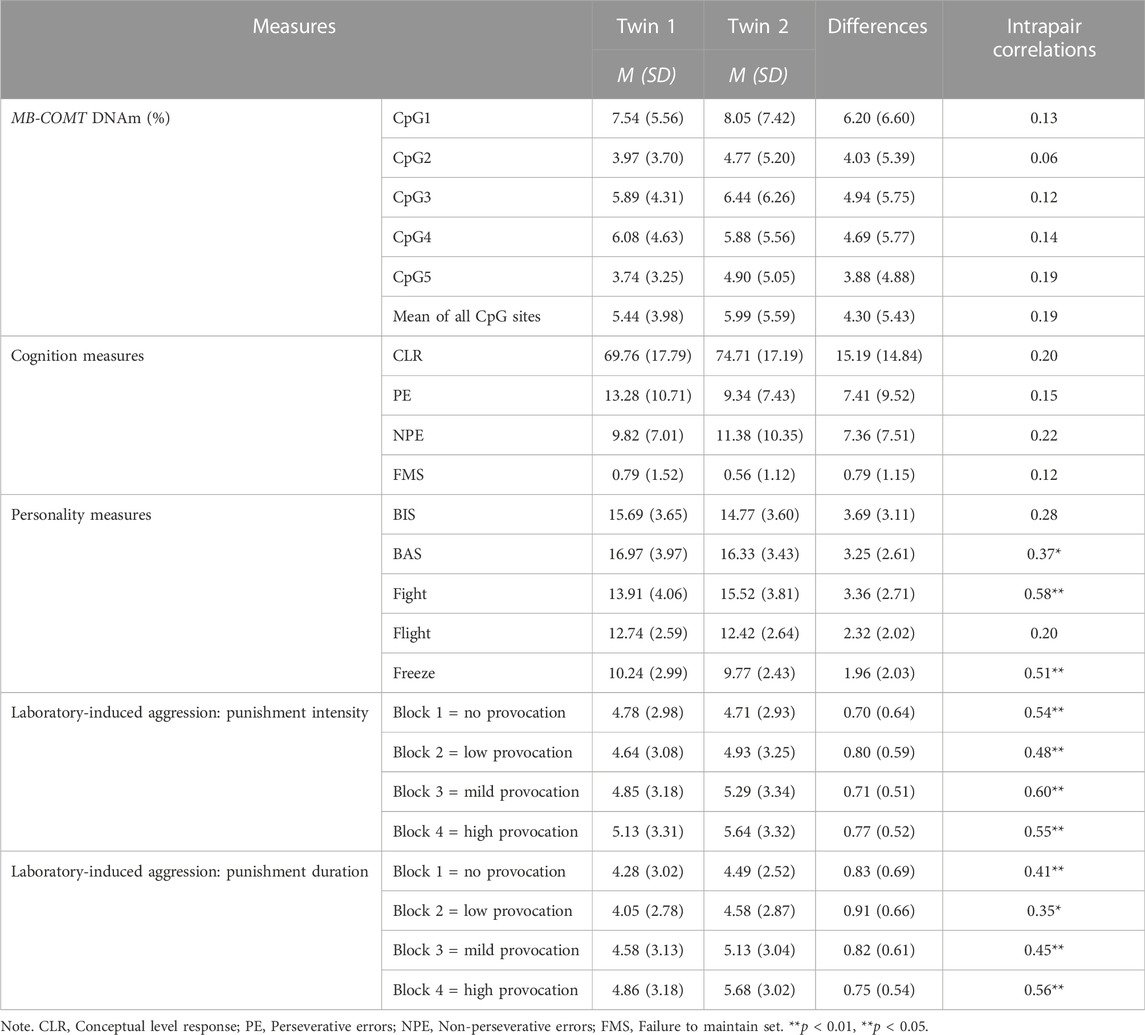
TABLE 3. Descriptive statistics and twin correlation coefficients for MB-COMT DNAm and all phenotypic measures.
Relationship between intrapair differences in MZ twins on continuous variables (cognitive measures, laboratory-induced aggression, and personality traits) and DNAm
Results of the correlations between intrapair differences in the percentage of MB-COMT DNAm and intrapair differences in cognitive measures showed that the only significant, positive and moderate correlation after the p-adjustment was obtained between differences in CpG site 1and differences in failures to maintain set (p = 0.005) (Table 4), pointing to the association between differences in CpG site 1 DNAm level and the differences in the phenotypic expression of this cognitive function. In the case of correlations with intrapair differences in aggression, two significant, positive and moderate correlations were found which remained significant after the Bonferroni p-adjustment. The significant correlations were found between intrapair differences in MB-COMT DNAm at CpG site 1 (p = 0.014) and CpG site 3 (p = 0.012) and intrapair differences in punishment intensity in block 1 of the CRTT procedure (direct unprovoked aggression). Thus, results indicated that differences in the levels of DNAm are associated with differences in the phenotypic expression of direct unprovoked aggression. Regarding correlations with personality dimensions, intrapair differences in the MB-COMT DNAm showed significant, positive and moderate to high correlations with intrapair differences in Freeze at CpG site 2 (p = 0.004), CpG site 3 (p = 0.019) and CpG site 4 (p = 0.007). However, only correlations at CpG site 2 and CpG site 4 remained significant after the p-adjustment. In addition, intrapair differences in MB-COMT DNAm at CpG site 4 showed a moderate positive correlation with intrapair differences in Fight, but this correlation was not significant after the p-adjustment (p = 0.041).
Relationship between discordances of MZ twins on categorial variables (alcohol use, cigarette use and drug abuse) and intrapair differences in DNAm.
The Mann-Whitney U-test showed significant differences in DNAm between concordant and discordant MZ twin pairs in alcohol consumption, with discordant twins had a significantly larger difference in the percentage of MB-COMT DNAm at CpG site 1 and CpG site 3 compared to concordant twin pairs (Table 5).
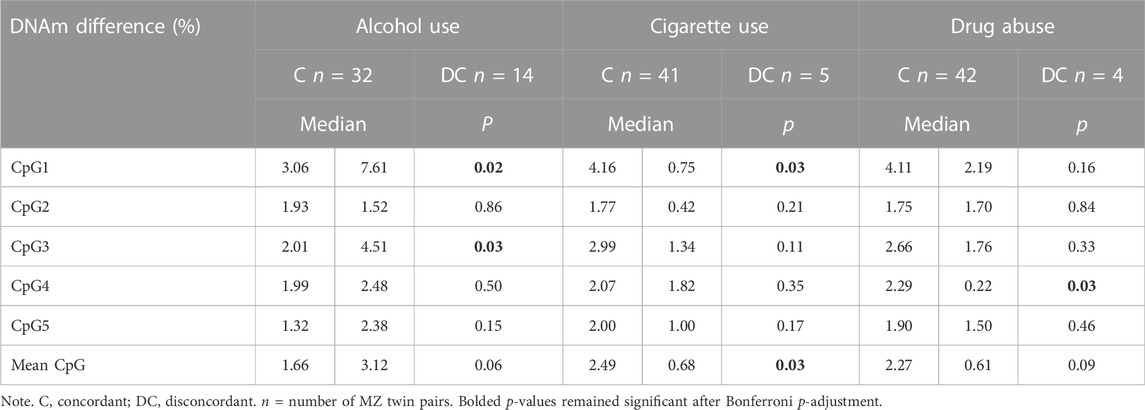
TABLE 5. Results of Mann-Whitney U-tests: Differences between concordant and discordant MZ twin pairs in risk behaviors.
Somewhat surprisingly, MZ twin pairs reporting cigarette smoking for both twins had a difference in the percentage of MB-COMT DNAm that was significantly higher at CpG site 1 and in the mean of all CpG sites. Similarly, we found that MB-COMT DNAm difference between MZ twin pairs in which both twins reported drug use was significantly higher than for those in which only one twin did at CpG site 4. Thus, these results pointed to the greater intrapair DNAm differences in phenotypically concordant, than in discordant MZ twin pairs regarding cigarettes and drugs consumption.
Discussion
In this study, a comprehensive range of indicators of impulsivity and lack of control is associated with DNAm percentages at five different CpG sites of the MB-COMT gene in MZ twins. The most important result is that the differences between MZ twins on DNAm at three of the five analyzed CpG sites in the promoter region of MB-COMT are associated with phenotypic indicators of lack of control. Although these associations are of moderate intensity, they deserve a more thorough consideration, which would facilitate the accumulation of knowledge necessary to understand the mechanisms underlying them.
The rRST, which includes stable responses to environmental stimuli, as sources of potential reward, punishment, or danger (Gray and McNaughton, 2000), is particularly important for the examination of epigenetic mechanisms. We found that phenotypic differences between MZ twins at Fight and Freeze are associated with their differences at methylation level. Twin studies consistently show that approximately half of the variance in all personality traits is genetically influenced (Takahashi et al., 2007; Kandler et al., 2010). It is plausible that the similarity of MZ in personality traits stems from the same genotype and shared environment, while all differences can be attributed to unshared environmental influences. Result that differences at Fight are associated with differences in MB-COMT DNAm levels at CpG site 4, may have important implications for etiology of aggression. It is quite possible that reactive aggression, defined by Fight, as a striking phenotypic indicator of lack of control, is influenced by environmental factors (Sypher et al., 2019), which determine epigenetic modulation on at least one DNAm marker. The association of the phenotypic differences between MZ twins at Freeze and differences in MB-COMT DNAm observed at CpG site 2, CpG site 3, and CpG site 4 is a particularly intriguing finding. Although Freeze, seemingly, cannot be easily associated with impulsive behavior, the importance of cognitive blockage in dealing with threatening stimuli should not be overlooked, since it can imply the perception of a lack of control over reactions and events (Smederevac et al., 2014). It is also possible that behavioral lack of control (Fight) includes some form of cognitive blocking (Freeze), which prevents the processing of broader information, narrowing the choice of possible reactions. Both Fight and Freeze systems underlie responses to the present threat, and although behaviorally different, both may be initiated by a similar experience. The perception of losing control can thus be manifested in a sudden and aggressive reaction to a threatening situation, but also in a more subtle way in the complete absence of reaction, i.e., freezing. Although Freeze and Fight are negatively correlated (Smederevac et al., 2014), they can have joint positive effects on certain behaviors (e.g., Sadiković et al., 2020). Since in our study both dimensions correlate positively with MB-COMT DNAm CpG site 4, the possibility of joint covariance of epigenetic changes in the domain of cognitive and behavioral reactions arises.
Besides self-report measures, differences between MZ twins in aggression was identified in specific aggressive behavior measured by experimental CRTT procedure as unprovoked direct aggression. Previous research using CRTT showed that shared and non-shared environmental factors, without genetic influences, almost equally contributed to the explanation of the initial level of aggression (Dinić et al., 2020) which could be linked to aggression in the 1st block of the CRTT scale. Thus, unprovoked aggression is partly influenced by the learned patterns developed in a shared, family environment. Significant associations were observed for MB-COMT DNAm MZ twin differences in CpG site 1 and CpG site 3 for block 1, which is in contrast to the association found with rRST Fight and CpG site 1. This implies a link to different aspects of aggression and different DNAm markers, CpG site 1 and 3 for direct unprovoked (CRTT) versus reactive (rRST Fight) aggression associated with CpG 4. If we consider that phenotypes result from specific combinations of genome composition, epigenetic components, and environmental influences (Cavalli & Heard, 2019), this result suggests that the effect of various environmental triggers could manifest in changes to DNAm at different sites associated with aggressive responses.
At WCST, as a complex measure of executive functions, MZ twins differ in Failures to maintain set and Non-perseverative errors, which are associated with different DNAm levels at CpG site 1. Failures to maintain set, as a measure of distractibility (Barceló and Knight, 2002) or cognitive flexibility (Greve et al., 2005), refers to the number of failures in sorting cards according to the sorting rule, due to a change of direction before the rules require it. Non-perseverative errors refer to a qualitative change in the search for the right strategy, which indicates the flexibility, yet ineffective, of cognitive style (Greve et al., 1996). As indicators of executive dysfunction, these measures may be associated with impulsivity and lack of control (Bickel et al., 2012). The correlation with CpG site one, which is also associated with the aggressive reactions, provides additional indirect evidence of the relationship between executive function and aggression. Therefore, Failures to maintain set may represent an aggressive response to a structured task with positive and negative feedback, an integral part of the WCST administration, which opens a new path for examining the etiology of executive dysfunctions. Namely, previous findings have shown large effect sizes related to association between failures to maintain set at WCST and aggression/violence (Burgess, 2020).
Despite the small sample size, this study identified discordant MZ twin pairs in relation to substance abuse as a form of risk behavior. Alcohol abuse is significantly associated with the level of DNAm at CpG site 1 and CpG site 3, confirming the importance of this aspect of methylation changes for dysfunctional aspects of impulsivity, which are reflected in the inability to resist temptation. Namely, this site showed the largest number of associations with different phenotypic traits, which include cognitive and behavioral indicators of lack of control. Although cigarette consumption is significantly associated with the level of DNAm at CpG site 1 and drug consumption with the level of DNAm at CpG site 4, the direction of the differences indicates an artifact due to the extremely small number of discordant twins on these phenotypic features. Also, it is possible that the epigenetic mechanisms underlying the use of alcohol and cigarettes, as more common forms of behavior, are significantly different from those of drug abuse, but these should be further investigated in future studies.
Interestingly, CpG site 2 and CpG site 5 are not relevant for any of the examined phenotypic traits. It is possible that each of the sites has a specific role in epigenetic mechanisms, which determines unique patterns of behavior. The most consistent links with different lack of control indicators exist for CpG 1 and CpG 3, while CpG 4 is related only to Fight. One of the most important implications of the results of this study is the indication that there are common epigenetic patterns for personality traits, cognitive functions, and behaviors. The highest level of methylation was registered at CpG1, associated with phenotypic differences among MZ pairs on five indicators of lack of control. Two of them belongs to measures of executive functions, one to aggressive reactions and two are behavioral measures of risky behaviors-alcohol and cigarettes abuse. In general, hypermethylation is associated with decreased gene expression, which is also related to MB-COMT (Abdolmaleky et al., 2006). Therefore, a potential risk mechanism for lack of control may lead to reduced methylation and higher levels of MB-COMT expression. In other words, increased COMT activity can lead to lower levels of dopamine in synapses. In this context, the role of CpG1 may be particularly important. In our study, it is bound to the P53 binding site CCCGGG (Yang & Festing, 2001) which protects the organism from the spread of an aberrant genetic signal (Jacobs et a., 2006) and is associated with neurological development and mental illnesses such as schizophrenia (Warburton et al., 2015). Also, CpG2 is bound to the SP1 binding dynamics (GGGCGG), which affects the expression of many genes including genes linked to mental diseases or functions (Abdolmaleky et al., 2006; Hasegawa, and Struhl, (2021).
It is plausible to assume that epigenetic modulations on the MB-COMT gene associated with phenotypic differences on impulsivity indicators in MZ twins are probably due to environmental influences. Given the similar environment experienced by MZ twins during development, differences in epigenetic events may be explained by unshared environmental factors (Wong et al., 2005). Previous research suggests that gene expression pattern can be strongly affected by environmental factors (Sutherland and Costa, 2003; Roth, 2013), so all unshared experiences can contribute to the discordance of MZ twins, through mechanisms such as DNAm. Although they cannot be viewed as a direct replication of previous studies, these results partially support the findings that indicate the importance of DNAm on the MB-COMT gene for individual differences in impulsivity and lack of control (Nielsen et al., 2012; van der Knaap et al., 2014; Lewis et al., 2019).
One important limitation of this study is the small sample size, which limits the relevance of our findings. However, this limitation is partially compensated by many impulsivity indicators and epigenetic analyzes, generated on one sample of MZ twins. We also observed some smaller effects that were no longer statistically significant after the correction for multiple comparisons; however, these can provide a base for future studies. Previous research has shown that small effects could have substantial consequences on the identification and understanding of the actual determinants of complex psychological phenomena (Götz et al., 2022). Also, one of the major limitations of this study is lack of concurrent gene expression analysis to portray functional impacts of DNA methylation alterations, which should certainly be included in future replication studies. The epigenetic effects of the MB-COMT gene on personality, risk behaviors, and cognitive processes suggest its pleiotropic role in the regulation of various phenotypic features. Namely, associations between phenotypic differences and differences in methylation level represent a contribution to the accumulation of evidence on the influence of the environment on the structure of the epigenome. The current results highlight the importance of studying gene methylation in the context of their genetic environment and the impact on phenotypic traits associated with lack of control. However, it is still difficult to determine how these changes affect gene expression and thus modulate phenotypes, since functional analyzes of these CpG sites are not yet associated with a wider spectrum of phenotypic characteristics.
Data availability statement
The data that support the findings of this study are openly available in the OSF repository: https://osf.io/694yn/.
Ethics statement
The studies involving human participants were reviewed and approved by the Institutional Ethics Committee of the Faculty of Philosophy (#02-374/15), the Committee for Ethics of Clinical Trials at the Faculty of Medicine (#01-39/229/1) at the University of Novi Sad and Research Ethics Committee of the Institute of Molecular Genetics and Genetical Engineering, University of Belgrade (#O-EO-024/2020). The patients/participants provided their written informed consent to participate in this study.
Author contributions
SSm: Conceptualization, Methodology, Investigation, Writing—Original Draft, Funding acquisition; LD-C: Resources, Investigation, Methodology, Supervision, Writing—Review and Editing; DM; Writing—Original Draft, Investigation; BD; Investigation, Writing—Original Draft, Data Curation; T-AB: Investigation, Writing—Review and Editing; MD: Investigation, Writing—Review and Editing; VB; Writing—Original Draft, Investigation; SSa; Investigation, Writing—Original Draft, Data Curation; IM; Investigation, Writing—Original Draft, Data Curation; NV; Investigation, Writing—Original Draft, Data Curation; BB: Investigation, Data Curation; MP: Resources, Investigation; ZB: Resource; JK-T: Writing—Review and Editing; and ZN; Methodology, Software, Formal analysis, Data Curation, Writing—Original Draft. All authors provided critical feedback, contributed to manuscript editing, and approved the final version of the manuscript.
Funding
This research was fully supported by the Science Fund of the Republic of Serbia (#7744418, Genetic and environmental influences on psychological adaptation of children and adults—GENIUS).
Acknowledgments
We would like to thank students volunteers from the STAR Center of Excellence for Behavioral Research in Psychology at the Faculty of Philosophy, University of Novi Sad for their help in requirement participants and collecting the data.
Conflict of interest
The authors declare that the research was conducted in the absence of any commercial or financial relationships that could be construed as a potential conflict of interest.
Publisher’s note
All claims expressed in this article are solely those of the authors and do not necessarily represent those of their affiliated organizations, or those of the publisher, the editors and the reviewers. Any product that may be evaluated in this article, or claim that may be made by its manufacturer, is not guaranteed or endorsed by the publisher.
References
Abdolmaleky, H. M., Cheng, K., Faraone, S. V., Wilcox, M., Glatt, S. J., Gao, F., et al. (2006). Hypomethylation of MB-COMT promoter is a major risk factor for schizophrenia and bipolar disorder. Hum. Mol. Genet. 15 (21), 3132–3145. doi:10.1093/hmg/ddl253
Abdolmaleky, H. M., Smith, C. L., Faraone, S. V., Shafa, R., Stone, W., Glatt, S. J., et al. (2004). Methylomics in psychiatry: Modulation of gene-environment interactions may be through DNA methylation. Am. J. Med. Genet. B Neuropsychiatr. Genet. 127B (1), 51–59. doi:10.1002/ajmg.b.20142
Anderson, P. (2002). Assessment and development of executive function (EF) during childhood. Child. Neuropsychol. 8, 71–82. doi:10.1076/chin.8.2.71.8724
Anderson, V., Jacobs, R., and Anderson, P. J. (2008). Executive functions and the frontal lobes: A lifespan perspective. Milton Park: Taylor & Francis Group.
Barceló, F., and Knight, R. T. (2002). Both random and perseverative errors underlie WCST deficits in prefrontal patients. Neuropsychologia 40, 349–356. doi:10.1016/s0028-3932(01)00110-5
Bickel, W. K., Jarmolowicz, D. P., Mueller, E. T., Gatchalian, K. M., and McClure, S. M. (2012). Are executive function and impulsivity antipodes? A conceptual reconstruction with special reference to addiction. Psychopharmacology 221 (3), 361–387. doi:10.1007/s00213-012-2689-x
Bouchard, T. J., and McGue, M. (2003). Genetic and environmental influences on human psychological differences. J. Neurobiol. 54, 4–45. doi:10.1002/neu.10160
Burgess, J. (2020). A brief review of the relationship of executive function assessment and violence. Aggress. Violent Behav. 54, 101414. doi:10.1016/j.avb.2020.101414
Cavalli, G., and Heard, E. (2019). Advances in epigenetics link genetics to the environment and disease. Nature 571 (7766), 489–499. doi:10.1038/s41586-019-1411-0
Chen, J., Lipska, B. K., Halim, N., Ma, Q. D., Matsumoto, M., Melhem, S., et al. (2004). Functional analysis of genetic variation in catechol-O-methyltransferase (COMT): Effects on mRNA, protein, and enzyme activity in postmortem human brain. Am. J. Hum. Genet. 75 (5), 807–821. doi:10.1086/425589
Córdova-Palomera, A., Alemany, S., Fatjó-Vilas, M., Goldberg, X., Leza, J. C., González-Pinto, A., et al. (2014). Birth weight, working memory and epigenetic signatures in IGF2 and related genes: A MZ twin study. PloS one 9 (8), e103639. doi:10.1371/journal.pone.0103639
Dammann, G., Teschler, S., Haag, T., Altmüller, F., Tuczek, F., and Dammann, R. H. (2011). Increased DNA methylation of neuropsychiatric genes occurs in borderline personality disorder. Epigenetics 6 (12), 1454–1462. doi:10.4161/epi.6.12.18363
DeYoung, C. G. (2013). The neuromodulator of exploration: A unifying theory of the role of dopamine in personality. Front. Hum. Neurosci. 7, 762. doi:10.3389/fnhum.2013.00762
Diamond, A. (2013). Executive functions. Annu. Rev. Psychol. 64, 135–168. doi:10.1146/annurev-psych-113011-143750
Dickinson, D., and Elvevåg, B. (2009). Genes, cognition and brain through a COMT lens. Neuroscience 164, 72–87. doi:10.1016/j.neuroscience.2009.05.014
Dinić, B. M., Smederevac, S., Sadiković, S., Oljača, M., Vučinić, N., Prinz, M., et al. (2020). Twin study of laboratory-induced aggression. Aggress. Behav. 46, 489–497. doi:10.1002/ab.21916
Dreher, J., Kohn, P., Kolachana, B., Weinberger, D. R., and Berman, K. F. (2008). Variation in dopamine genes influences responsivity of the human reward system. Proc. Natl. Acad. Sci. U. S. A. 106, 617–622. doi:10.1073/pnas.0805517106
Fernàndez-Castillo, N., and Cormand, B. (2016). Aggressive behavior in humans: Genes and pathways identified through association studies. Am. J. Med. Genet. B Neuropsychiatr. Genet. 171 (5), 676–696. doi:10.1002/ajmg.b.32419
Fraga, M. F., Ballestar, E., Paz, M. F., Ropero, S., Setien, F., Ballestar, M. L., et al. (2005). Epigenetic differences arise during the lifetime of monozygotic twins. Proc. Natl. Acad. Sci. U. S. A. 109, 10604–10609. doi:10.1073/pnas.0500398102
Giancola, P. R., and Chermack, S. T. (1998). Construct validity of laboratory aggression paradigms: A response to tedeschi and quigley (1996). Aggress. Violent Behav. 3 (3), 237–253. doi:10.1016/S1359-1789(97)00004-9
Gomes, M. V., Toffoli, L. V., Arruda, D. W., Soldera, L. M., Pelosi, G. G., Neves-Souza, R. D., et al. (2012). Age-related changes in the global DNA methylation profile of leukocytes are linked to nutrition but are not associated with the MTHFR C677T genotype or to functional capacities. PloS one 7 (12), e52570. doi:10.1371/journal.pone.0052570
Götz, F. M., Gosling, S. D., and Rentfrow, J. (2022). Small effects: The indispensable foundation for a cumulative psychological science. Perspect. Psychol. Sci. 17 (1), 205–215. doi:10.1177/1745691620984483
Gravetter, F., and Wallnau, L. (2017). Essentials of statistics for the behavioral sciences. 10th Edition. Boston: Cengage Learning.
Gray, J. A., and McNaughton, N. (2000). The neuropsychology of anxiety: An enquiry into the functions of the septo-hippocampal system. 2nd ed. United Kingdom: Oxford University Press.
Greve, K. W., Stickle, T. R., Love, J. M., Bianchini, K. J., and Stanford, M. S. (2005). Latent structure of the Wisconsin card sorting test: A confirmatory factor analytic study. Arch. Clin. Neuropsychol. 20, 355–364. doi:10.1016/j.acn.2004.09.004
Greve, K. W., Williams, M. C., Haas, W. G., Littell, R. R., and Reinoso, C. (1996). The role of attention in Wisconsin card sorting test performance. Arch. Clin. Neuropsychol. 11 (3), 215–222. doi:10.1093/arclin/11.3.215
Gurvich, C., and Rossell, S. L. (2015). Dopamine and cognitive control: Sex-by-genotype interactions influence the capacity to switch attention. Behav. Brain Res. 281, 96–101. doi:10.1016/j.bbr.2014.11.045
Hasegawa, Y., and Struhl, K. (2021). Different SP1 binding dynamics at individual genomic loci in human cells. Proc. Natl. Acad. Sci. U. S. A. 118 (46), e2113579118. doi:10.1073/pnas.2113579118
Heaton, R. K., Chelune, G. J., Talley, J. L., Kay, G. G., and Curtiss, G. (1993). Wis. Card Sorting Test Man. Revis. Expand. Psychol. Assess. Resour.
Hughes, C., and Graham, A. (2002). Measuring executive functions in childhood: Problems and solutions? Child. Adolesc. Ment. Health 7, 131–142. doi:10.1111/1475-3588.00024
Jacobs, W. B., Kaplan, D. R., and Miller, F. D. (2006). The p53 family in nervous system development and disease. J. Neurochem. 97 (6), 1571–1584. doi:10.1111/j.1471-4159.2006.03980.x
Jonsson, H., Magnusdottir, E., Eggertsson, H. P., Stefansson, O. A., Arnadottir, G. A., Eiriksson, O., et al. (2021). Differences between germline genomes of monozygotic twins. Nat. Genet. 53 (1), 27–34. doi:10.1038/s41588-020-00755-1
Kandler, C., Riemann, R., Spinath, F. M., and Angleitner, A. (2010). Sources of variance in personality facets: A multiple-rater twin study of self-peer, peer-peer, and self-self (dis) agreement. J. Pers. 78 (5), 1565–1594. doi:10.1111/j.1467-6494.2010.00661.x
Karoum, F., Chrapusta, S. J., and Egan, M. F. (1994). 3-Methoxytyramine is the major metabolite of released dopamine in the rat frontal cortex: Reassessment of the effects of antipsychotics on the dynamics of dopamine release and metabolism in the frontal cortex, nucleus accumbens, and striatum by a simple two pool model. J. Neurochem. 63, 972–979. doi:10.1046/j.1471-4159.1994.63030972.x
Lewis, C. R., Henderson-Smith, A., Breitenstein, R. S., Sowards, H. A., Piras, I. S., Huentelman, M. J., et al. (2019). Dopaminergic gene methylation is associated with cognitive performance in a childhood monozygotic twin study. Epigenetics 14 (3), 310–323. doi:10.1080/15592294.2019.1583032
Lo, M.-T., Hinds, D. A., Tung, J. Y., Franz, C., Fan, C.-C., Wang, Y., et al. (2016). Genome-wide analyses for personality traits identify six genomic loci and show correlations with psychiatric disorders. Nat. Genet. 49 (1), 152–156. doi:10.1038/ng.3736
MacKillop, J., Weafer, J., Gray, J. C., Oshri, A., Palmer, A., and de Wit, H. (2016). The latent structure of impulsivity: Impulsive choice, impulsive action, and impulsive personality traits. Psychopharmacology 233 (18), 3361–3370. doi:10.1007/s00213-016-4372-0
McGregor, N. R. (2014). Catechol O-methyltransferase: A review of the gene and enzyme. J. Dent. Clin. Res. 1:007.
Miyake, A., and Friedman, N. P. (2012). The nature and organization of individual differences in executive functions: Four general conclusions. Curr. Dir. Psychol. Sci. 21, 8–14. doi:10.1177/0963721411429458
Nielsen, D. A., Utrankar, A., Reyes, J. A., Simons, D. D., and Kosten, T. R. (2012). Epigenetics of drug abuse: Predisposition or response. Pharmacogenomics 13 (10), 1149–1160. doi:10.2217/pgs.12.94
Rakvåg, T. T., Ross, J. R., Sato, H., Skorpen, F., Kaasa, S., and Klepstad, P. (2008). Genetic variation in the catechol-O-methyltransferase (COMT) gene and morphine requirements in cancer patients with pain. Mol. Pain 4, 64. doi:10.1186/1744-8069-4-64
Reuter, M., Schmitz, A., Corr, P., and Hennig, J. (2006). Molecular genetics support Gray’s personality theory: The interaction of COMT and DRD2 polymorphisms predicts the behavioural approach system. Int. J. Neuropsychopharmacol. 9, 155–166. doi:10.1017/s1461145705005419
Roth, T. L. (2013). Epigenetic mechanisms in the development of behavior: Advances, challenges, and future promises of a new field. Dev. Psychopathol. 25, 1279–1291. doi:10.1017/s0954579413000618
Sadiković, S., Branovački, B., Oljača, M., Mitrović, D., Pajić, D., and Smederevac, S. (2020). Daily monitoring of emotional responses to the coronavirus pandemic in Serbia: A citizen science approach. Front. Psychol. 11, 2133. doi:10.3389/fpsyg.2020.02133
Schiepers, O. J., Van Boxtel, M. P., de Groot, R. H., Jolles, J., Kok, F. J., Verhoef, P., et al. (2012). DNA methylation and cognitive functioning in healthy older adults. Br. J. Nutr. 107 (5), 744–748. doi:10.1017/S0007114511003576
Schreiner, F., El-Maarri, O., Gohlke, B., Stutte, S., Nuesgen, N., Mattheisen, M., et al. (2011). Association of COMT genotypes with S-COMT promoter methylation in growth-discordant monozygotic twins and healthy adults. BMC Med. Genet. 12, 115. doi:10.1186/1471-2350-12-115
Smederevac, S., Mitrović, D., Čolović, P., and Nikolašević, Ž. (2014). Validation of the measure of revised reinforcement sensitivity theory constructs. J. Individ. Differ. 35, 12–21. doi:10.1027/1614-0001/a000121
Smederevac, S., Mitrović, D., Sadiković, S., Milutinović, A., Branovacki, B., Dinic, B. M., et al. (2019). Serbian twin Registry. Twin Res. Hum. Genet. 22, 660–666. doi:10.1017/thg.2019.114
Smederevac, S., Sadiković, S., Čolović, P., Vučinić, N., Milutinović, A., Riemann, R., et al. (2022). Quantitative behavioral genetic and molecular genetic foundations of the approach and avoidance strategies. Curr. Psychol., 1–15. doi:10.1007/s12144-022-02724-9
Soeiro-De-Souza, M. G., Stanford, M. S., Bio, D. S., Machado-Vieira, R., and Moeno, R. A. (2013). Association of the COMT Met¹⁵⁸ allele with trait impulsivity in healthy young adults. Mol. Med. Rep. 7 (4), 1067–1072. doi:10.3892/mmr.2013.1336
Sutherlend, J. E., and Costa, M. (2003). Epigenetics and the environment. Ann. N. Y. Acad. Sci. 983 (1), 151–160. doi:10.1111/j.1749-6632.2003.tb05970.x
Sypher, I., Hyde, L. W., Peckins, M. K., Waller, R., Klump, K., and Alexandra, B. S. (2019). Effects of parenting and community violence on aggression-related social goals: A monozygotic twin differences study. J. Abnorm. Child. Psychol. 47 (6), 1001–1012. doi:10.1007/s10802-018-0506-7
Takahashi, Y., Yamagata, S., Kijima, N., Shigemasu, K., Ono, Y., and Ando, J. (2007). Continuity and change in behavioral inhibition and activation systems: A longitudinal behavioral genetic study. Personality Individ. Differ. 43, 1616–1625. doi:10.1016/j.paid.2007.04.030
Thomas, M., Banet, N., Wallisch, A., Glowacz, K., Becker-Sadzio, J., Gundel, F., et al. (2019). Differential COMT DNA methylation in patients with borderline personality disorder: Genotype matters. Eur. Neuropsychopharmacol. 29 (11), 1295–1300. doi:10.1016/j.euroneuro.2019.09.011
Tunbridge, E. M., Narajos, M., Harrison, C. H., Beresford, C., Cipriani, A., and Harrison, P. J. (2019). Which dopamine polymorphisms are functional? Systematic review and meta-analysis of comt, dat, dbh, DDC, drd1–5, MAOA, MAOB, Th, VMAT1, and VMAT2. Biol. Psychiatry 86, 608–620. doi:10.1016/j.biopsych.2019.05.014
Ursini, G., Bollati, V., Fazio, L., Porcelli, A., Iacovelli, L., Catalani, A., et al. (2011). Stress-related methylation of the catechol-O-methyltransferase Val158 allele predicts human prefrontal cognition and activity. J. Neurosci. 31 (18), 6692–6698. doi:10.1523/JNEUROSCI.6631-10.2011
Van der Knaap, L. J., Schaefer, J. M., Franken, I. H., Verhulst, F. C., van Oort, F. V., and Riese, H. (2014). Catechol-O-methyltransferase gene methylation and substance use in adolescents: The TRAILS study. Genes Brain Behav. 13, 618–625. doi:10.1111/gbb.12147
Van Dongen, J., Gordon, S. D., McRae, A. F., Odintsova, V. V., Mbarek, H., Breeze, C. E., et al. (2021). Identical twins carry a persistent epigenetic signature of early genome programming. Nat. Commun. 12 (1), 5618. doi:10.1038/s41467-021-25583-7
Van Dongen, J., Hagenbeek, F. A., Suderman, M., Roetman, P., Sugden, K., Chioccetti, A. G., et al. (2021). DNA methylation signatures of aggression and closely related constructs: A meta-analysis of epigenome-wide studies across the lifespan. Mol. Psychiatry 26, 2148–2162. doi:10.1038/s41380-020-00987-x
Van Dongen, J., Nivard, M. G., Baselmans, B. M., Zilhão, N. R., Ligthart, L., Heijmans, B. T., et al. (2015). Epigenome-wide association study of aggressive behavior. Twin Res. Hum. Genet. 186, 686–698. doi:10.1017/thg.2015.74
Wacker, J., Mueller, E. M., Hennig, J., and Stemmler, G. (2012). How to consistently link extraversion and intelligence to the catechol-O-methyltransferase (COMT) gene: On defining and measuring psychological phenotypes in neurogenetic research. J. Pers. Soc. Psychol. 102, 427–444. doi:10.1037/a0026544
Walton, E., Liu, J., Hass, J., White, T., Scholz, M., Roessner, V., et al. (2014). MB-COMT promoter DNA methylation is associated with working-memory processing in schizophrenia patients and healthy controls. Epigenetics 9 (8), 1101–1107. doi:10.4161/epi.29223
Wang, M., Chen, P., Li, H., Kemp, A. H., and Zhang, W. (2018). Catechol-O-Methyltransferase gene Val158Met polymorphism moderates the effect of social exclusion and inclusion on aggression in men: Findings from a mixed experimental design. Front. Psychol. 11, 622914. doi:10.3389/fpsyg.2020.622914
Warburton, A., Breen, G., Bubb, V. J., and Quinn, J. P. (2015). A GWAS SNP for schizophrenia is linked to the internal MIR137 promoter and supports differential allele-specific expression. Schizophr. Bull. 42 (4), 1003–1008. doi:10.1093/schbul/sbv144
Warburton, W. A., and Bushman, B. J. (2019). The competitive reaction time task: The development and scientific utility of a flexible laboratory aggression paradigm. Aggress. Behav. 45 (4), 389–396. doi:10.1002/ab.21829
Welsh, M. C., Pennington, B. F., and Groisser, D. B. (1991). A normative–developmental study of executive function: A window on prefrontal function in children. Dev. Neuropsychol. 7, 131–149. doi:10.1080/87565649109540483
Wong, A. H., Gottesman, I. I., and Petronis, A. (2005). Phenotypic differences in genetically identical organisms: The epigenetic perspective. Hum. Mol. Genet. 14, R11–R18. doi:10.1093/hmg/ddi116
Yang, A. L., and Festing, M. F. (2001). A promoter function of the CCCGGG sma I recognition sequence and its specific role in determining p53 status and identifying DNA damaging agents. Biochem. Biophys. Res. Commun. 281 (2), 506–510. doi:10.1006/bbrc.2001.4368
Keywords: MB-COMT, DNA methylation, monozygotic twins, risk behavior, aggression, personality, executive functions (EFs), impulsivity
Citation: Smederevac S, Delgado-Cruzata L, Mitrović D, Dinić BM, Bravo T-AT, Delgado M, Bugarski Ignjatović V, Sadiković S, Milovanović I, Vučinić N, Branovački B, Prinz M, Budimlija Z, Kušić-Tišma J and Nikolašević Ž (2023) Differences in MB-COMT DNA methylation in monozygotic twins on phenotypic indicators of impulsivity. Front. Genet. 13:1067276. doi: 10.3389/fgene.2022.1067276
Received: 12 October 2022; Accepted: 28 November 2022;
Published: 06 January 2023.
Edited by:
Dennis Robert Grayson, University of Illinois at Chicago, United StatesReviewed by:
Dorret I. Boomsma, VU Amsterdam, NetherlandsHamid Abdolmaleky, Boston University, United States
Copyright © 2023 Smederevac, Delgado-Cruzata, Mitrović, Dinić, Bravo, Delgado, Bugarski Ignjatović, Sadiković, Milovanović, Vučinić, Branovački, Prinz, Budimlija, Kušić-Tišma and Nikolašević. This is an open-access article distributed under the terms of the Creative Commons Attribution License (CC BY). The use, distribution or reproduction in other forums is permitted, provided the original author(s) and the copyright owner(s) are credited and that the original publication in this journal is cited, in accordance with accepted academic practice. No use, distribution or reproduction is permitted which does not comply with these terms.
*Correspondence: Selka Sadiković, c2Vsa2Euc2FkaWtvdmljQGZmLnVucy5hYy5ycw==; Snežana Smederevac, c25lemFuYS5zbWVkZXJldmFjQHVucy5hYy5ycw==