- 1Hospital de Santo Espirito da Ilha Terceira EPER, SEEBMO, Angra do Heroísmo, Portugal
- 2Comprehensive Health Research Centre, Hospital de Santo Espírito da Ilha Terceira, Lisbon, Portugal
- 3University of Algarve, Center of Marine Science (CCMAR), Faro, Portugal
- 4Hospital de Santo Espirito da Ilha Terceira EPER, Orthopedics Service, Angra do Heroísmo, Portugal
- 5Centro Hospitalar Do Medio Tejo EPE Unidade de Torres Novas, Rheumatology Department, Santarém, Portugal
- 6CHRC Campus Nova Medical School, EpiDoc Research Unit, CEDOC, Lisboa, Portugal
- 7Emek Medical Center, Rheumatology Unit, Afula, Israel
- 8Sheba Medical Center, Tel Aviv, Israel
- 9Luigi Sacco University Hospital, Rheumatology Unit, Milano, Italy
- 10Istituti Clinici Scientifici Maugeri IRCCS, Neuromotor Rehabilitation Unit of Telese Terme Institute, Pavia, Italy
- 11Universita Degli Studi di Messina, Rheumatology Unit, Clinical and Experimental Medicine, Messina, Italy
- 12University Medical Centre, Department of Orthopedics, Utrecht, Netherlands
- 13Universitair Medisch Centrum Utrecht, Utrecht, Netherlands
- 14Istituti Clinici Scientifici Maugeri IRCCS, Cardiac Rehabilitation Unit of Telese Terme Institute, Pavia, Italy
- 15Ruhr-Universitat Bochum, Rheumazentrum Ruhrgebiet, Bochum, Germany
- 16Case Western Reserve University, Cleveland, OH, United States
- 17Rappaport Faculty of Medicine, Technion, Haifa, Israel
- 18Ruhr University Bochum, Rheumazentrum Ruhrgebiet, Herne, Germany
Diffuse Idiopathic Skeletal Hyperostosis (DISH) and Ossification of the Posterior Longitudinal Ligament (OPLL) are common disorders characterized by the ossification of spinal ligaments. The cause for this ossification is currently unknown but a genetic contribution has been hypothesized. Over the last decade, many studies on the genetics of ectopic calcification disorders have been performed, mainly on OPLL. Most of these studies were based on linkage analysis and case control association studies. Animal models have provided some clues but so far, the involvement of the identified genes has not been confirmed in human cases. In the last few years, many common variants in several genes have been associated with OPLL. However, these associations have not been at definitive levels of significance and evidence of functional significance is generally modest. The current evidence suggests a multifactorial aetiopathogenesis for DISH and OPLL with a subset of cases showing a stronger genetic component.
1 Introduction
The spine is a columnar structure composed of bony vertebrae interconnected by intervertebral discs and supported by ligaments, such as the anterior and posterior longitudinal ligaments, ligament nuchae and ligamentum flavum. The spinal canal, enclosed within the foramen of the vertebrae, contains the spinal cord. In the intervertebral spaces, the canal is protected by the ligament flavum posteriorly and the posterior longitudinal ligament anteriorly. Spinal stenosis consists in the reduction of the area of the spinal canal, leading to motor neuron deficits and related neurological symptoms, depending on the location of the stenosis (Bai et al., 2022). In the elderly population, the most common cause of spinal cord impairment is the degenerative cervical myelopathy (DCM). DCM can be secondary to osteoarthritic degeneration or to ligamentous ossifications such as the Ossification of the Posterior Longitudinal Ligament (OPLL) or the ossification of the Ligament Flavum (OLF) (Nouri et al., 2015). OPLL, frequently in association with DISH, can result in various degrees of neurological complications that can range from a slowly progressive painless myelopathy to a rapid progression of a neurological deficit even after minor injury (Takayuki et al., 2021; Prabhu et al., 2022). The physical and socioeconomic burden of disability associated with DCM is expected to grow evenly, due to the ageing population (Badhiwala et al., 2020). It is thus crucial to improve the diagnosis and assessment of disorders involved in DCM for early detection and swift intervention.
This review will focus on genetic studies of the ossification of the anterior and posterior longitudinal ligaments, the Diffuse Idiopathic Skeletal Hyperostosis (DISH) [MIM: 106400] and the Ossification of the Posterior Longitudinal Ligament (OPLL) [MIM: 602475], respectively. A short outline of DISH, OPLL and OLF can be seen in Table 1. These conditions may co-occur in some patients suggesting possible common etiopathogenic factors (Nouri et al., 2015; Takayuki et al., 2021). The objective was to collect and present evidences that supports a genetic foundation, based on the following observations: 1) familial aggregation reports, 2) animal models, 3) associated genetic variants and 4) genetics of associated disorders.
2 Familial aggregation reports
2.1 DISH
Reports of familial DISH are scarce. Beardwell, A. in 1969 (Beardwell, 1969), describes a family with Ankylosing Vertebral Hyperostosis (AVH), by the third decade, with many family members also presenting tylosis (punctuate hyperkeratosis). As demonstrated by the author, the X-ray of the affected family members showed ossification of paraspinal distribution, mainly in the lower thoracic region and also some osteophytosis and marginal sclerosis of the sacroiliac joints.
Another report of familial DISH, described 2 families; one had 4 siblings showing AVH by the fourth decade and two other family members had probably AVH. The second family was dentified after hip surgery of two sisters aged 71 and 82 years. The proband had five daughters, two of them affected by AVH and other two with a mild phenotype, classified as possible AVH (Abiteboul et al., 1985). An unusual DISH-like phenotype was described in a family with severe cervical disease lacking the extensive dorsal involvement (Gorman et al., 2005).
In Azores region, twelve families were identified presenting early onset (third decade) of DISH and/or Chondrocalcinosis (CC). The affected members had a pyrophosphate arthropathy showing exuberant axial and peripheral enthesopathic calcifications, meaning calcification of the connective tissues in the attachments of tendons or ligaments to the bones, in joints other than the spine (Bruges-Armas et al., 2006). Genetic studies in these families suggest that the phenotype DISH/CC is polygenic and influenced by the interaction of several, small-effect gene variants and possibly by unidentified environmental factors (Couto et al., 2017; Parreira et al., 2020). Similar cases, of patients with CPPD and/or CC and DISH, were mentioned in other studies (Okazaki et al., 1976), also showing familial aggregation (van der Korst et al., 1974; Bruges-Armas et al., 2006).
A postmortem examination of a skeleton allowed the diagnosis of DISH and ankylosing spondylitis in the same patient (Jordana et al., 2009). An extensive radiographic survey on several members of the Medici family (15th–17th century), demonstrated that DISH, rheumatoid arthritis and uric acid gout affected several family members (Fornaciari et al., 2009; Fornaciari and Giuffra, 2013). A study of 13 royal Egyptian mummies detected ossifications at the anterior aspects of the spines in five male mummies but only four fulfilled the criteria for DISH (Saleem and Hawass, 2014).
2.2 OPLL
The cause of OPLL is unclear but people of Asian heritage, have a higher likelihood of developing this condition (Choi et al., 2011). Familial aggregation of cervical OPLL was first demonstrated in a study assessing 347 families (Terayama, 1989); the relative risk of first degree relatives came to have OPLL was five times greater than expected in the general population. Another study shows a prevalence of 27% with a relative risk seven times that of the general population (Tanikawa et al., 1986). Other OPLL familial cases included the report of familial thoracic OPLL in Caucasian siblings (Tanabe et al., 2002) (Terayama, 1989).
The mode of inheritance for OPLL is still poorly defined due to the absence of large families, late onset of the disorder, environmental effects and sex differences (Koga et al., 1998). However, segregation studies shows that OPLL have both autosomal dominant (Tanikawa et al., 1986) and autosomal recessive (Hamanishi et al., 1995) patterns of inheritance. As discussed later, ectopic ossification resembling OPLL, as seen in the tiptoe walking mouse (ttw) or also called tiptoe walking of Yoshimura (twy), is inherited as an autosomal recessive disease with complete penetrance (Ikegawa et al., 2007).
3 Animal models for Ossification of Spinal Ligaments
The study of mouse strain models and the progress of strategies to find genetic mutations, affecting the mineralization pattern, have permitted the discovery of many genes and proteins to be evaluated.
3.1 DISH
3.2 OPLL
4 Genetic variants associated with OSL in humans
4.1 Genetic studies of DISH
Some of the earliest genetic studies were performed on genes belonging to Major Histocompatibility complex, specifically Human Leucocyte Antigens (HLA) (Brewerton et al., 1973; Schlosstein et al., 1973), but this association was never confirmed.
In a small study, polymorphisms of the Collagen Type I Iα1 (COL1A1), and Vitamin D Receptor (VDR) were investigated, but the authors concluded that these genes do not seem to be related to DISH etiology (Havelka et al., 2002). One more study, investigated polymorphisms of the collagen 6A1 gene (COL6A1) in Czech and Japanese DISH patients and the polymorphism, in intron 32, was associated with the disorder in Japanese patients but failed the association test with DISH Czech patients (Table 2). However, the authors suggested that COL6A1 could be related to ectopic bone formation in spinal ligaments (Tsukahara et al., 2005). Due to the possible common aetiopathogenesis of OPLL and DISH, a genotyping study (intron 6; −4) on the COL11A2 gene was performed, and no significant difference was observed between both cohorts (Havelka et al., 2001). Jun et al (Jun and Kim, 2012) described that two polymorphisms in the FGF2 gene were associated with DISH (Table 2). Another study identified a genetic variant in the PPP2R2D gene significantly associated with a phenotype characterized by DISH and CC. It was proposed that PPP2R2D may contribute to the development of this disorder (Parreira et al., 2020). Although these variants are significantly associated with DISH, the direct evidence for pathogenicity is lacking.
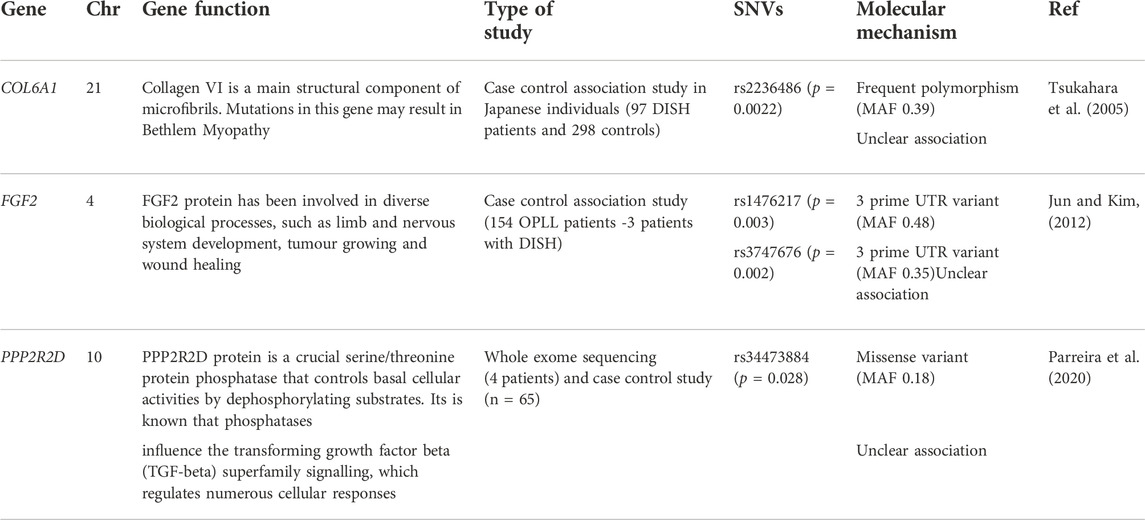
Table 2. Genes and genetic variants associated with DISH. The protein physiological function is also mentioned. Gene function was obtained from GeneCards database.
4.2 Genetic studies in OPLL
Many genetic studies of OPLL have been performed and it is now well established that genetic factors are implicated in its etiology (Terayama, 1989) (Table 3). In the same way as DISH, the initial genetic studies of OPLL were performed on HLA and the possible association is much discussed in the literature (Sakou et al., 1991; Yamaguchi, 1991; Matsunaga et al., 1999). Very close to the HLA region on the chromosome 6 is COL11A2 and common variants of this gene have been associated with OPLL (Koga et al., 1998; Maeda et al., 2001a). The polymorphism in intron 6 (-4A) seems to confer protection to OPLL furthermore, it was proven that this polymorphism of COL11A2 affects the splicing of exon 6 in cells obtained from spinal ligaments from OPLL patients (Maeda et al., 2001b).

Table 3. Genes and genetic variants associated with OPLL predisposition. The protein physiological function is also mentioned. Protein function was obtained from GeneCards database.
According to Nakamura et al. (1999) the deletion of T, 11 nucleotides upstream of the splice acceptor site of intron 20 (IVS20-11delT) of ENPP1 is associated with OPLL. However, He et al. (2013) described that the polymorphism TT genotype of C973T and IVS15-14T as well as the wild type IVS20 (lack of deletion) were related with disease severity. Another study found a polymorphism (IVS15-14T-- > C) in ENPP1 gene associated with OPLL susceptibility and severity (Koshizuka et al., 2002). Interestingly, in one study the authors found that the ENPP1 variant (IVS20-11delT) and the SNP (A861G) in the leptin receptor gene (LEPR) were more frequent in OPLL patients affected in the thoracic spine compared to patients whose OPLL was restricted to cervical spine. The authors suggested that the two variants (IVS20-11delT and A861G) are associated with more extensive OPLL, but not with frequency of its occurrence (Tahara et al., 2005).
The COL6A1 gene is intensely associated to OPLL and polymorphisms in this gene are considered useful markers of OPLL (Tanaka et al., 2003; Kong et al., 2007; Wang et al., 2018a). However this association is not always confirmed in all the studies performed (Furushima et al., 2002; Liu et al., 2010). Polymorphisms in COL6A1 gene were associated with DISH in the Japanese population (Tsukahara et al., 2005) suggesting that COL6A1 may contribute in pathological ectopic ossification.
Positive associations of BMP2, an important regulator of bone metabolism, with OPLL were found with the SNPs rs3178250 (Wang et al., 2008), rs2273073 (Chen et al., 2008; Yan et al., 2013) and rs1949007 (Chen et al., 2008) (Table 3). Yan et al. (2013), confirmed that the SNP rs227373 in the BMP2 gene is associated with the higher level of Smad4 protein expression and with activity of alkaline phosphatase. On the other hand, according to Kim et al. (2014a) the SNPs rs2273073 and rs1949007, in Korean patients, are not associated with OPLL. Other study (Liu et al., 2010), performed in Chinese Han population, also failed to show association between BMP2 gene and OPLL. A genome-wide linkage study performed with 214 OPLL affected sib-pairs identified a chromosome region (20p12), linked with OPLL (Karasugi et al., 2013). This region contains 25 genes, of which two are good candidates: Jagged 1 (JAG1), which is involved in endochondral bone formation (Nobta et al., 2005) and BMP2. Furthermore, deleterious coding variants of BMP2 in peripheral blood samples was recently demonstrated (Chen et al., 2016). Three other polymorphisms (rs996544, rs965291 and rs1116867) were screened in Han Chinese subjects and the authors found that rs1116867 and rs965291 were related with the manifestation and extend of OPLL (Yan et al., 2010).
Other bone morphogenetic protein genes have been associated with OPLL; two SNPs in BMP-9 were found to be associated with OPLL: rs75024165 and rs34379100 (Ikuma et al., 2022). BMP-4 SNPs rs17563 (Mader et al., 2013; Cudrici et al., 2021), rs76335800 and a specific haplotype, TGGGCTT (Mader et al., 2013), were identified as risk factors for developing OPLL in the Chinese population. Furushima et al. (Ramos et al., 2015) also confirmed the association of BMP-4 with OPLL, in a large scale screening study, in which only BMP-4 reached criteria of suggestive evidence of linkage. In a recent study, BMP-4 has even been proposed as a new therapeutic option for treating bone diseases due to its role on a RUNX2/CHRDLI/BMP4 pathway. Several SNPs in gene RUNX2 have also been associated with OPLL (Liu et al., 2010; Chang et al., 2017).
Another important gene with contradictory results is TGFβ1, that according to Kamiya et al. (2001), is genetically associated to OPLL (869T > C; rs1982073). However, Han et al. (2013) showed that the SNP previously associated with OPLL (rs1982073) and the SNP located in the promoter region (rs1800469) are not associated with OPLL in Korean populations. Interestingly, in the chondrocytes of adjacent cartilaginous areas and in the ossified matrix of OPLL the TGF-β1 gene is overexpressed. The same authors tested the association between rs1982073 and the radiological appearance of OPLL, and they verified that SNP rs1982073 is associated to the specific area of the ossified lesion, and not to the onset of OPLL. The “C” allele could be a risk factor for patients with OPLL in cervical, thoracic, and/or lumbar spine (Kawaguchi et al., 2003).
In relation to ossification of the ligamentum flavum several genes and loci have been associated with thoracic Ossification of Ligamentum Flavum (OLF) (Kong et al., 2007; Liu et al., 2010; Qu et al., 2017; Qu et al., 2021).
5 Associated disorders
The presence of OSL has been described in association with numerous diseases of diverse etiologies. The type of disorders, the main pathways affected and the consequences, including the main anomalies identified in laboratory analysis, are outlined in Figure 1.
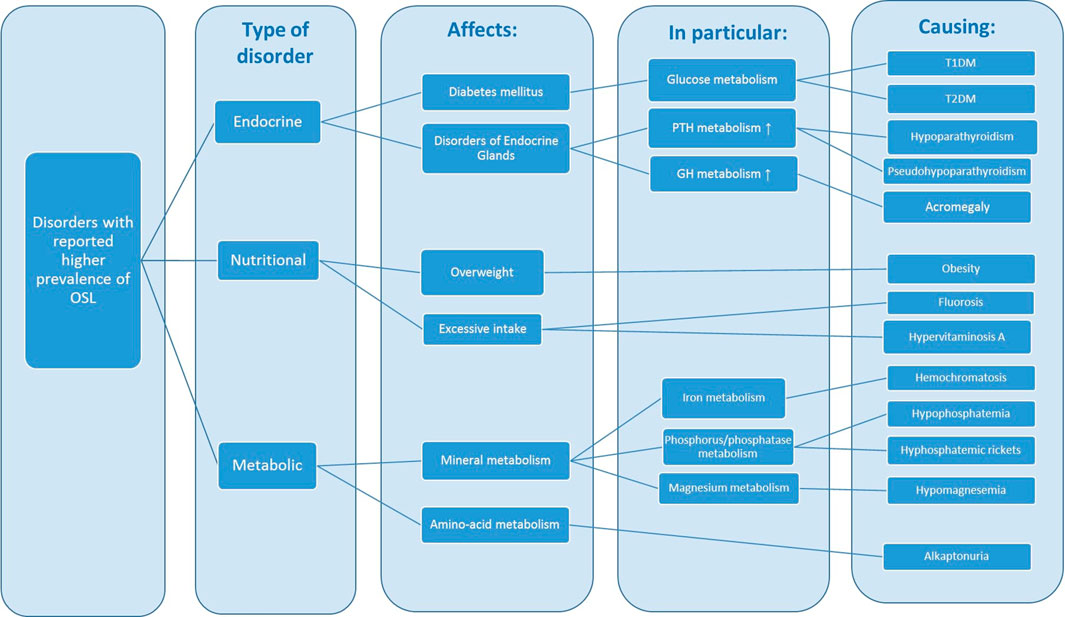
Figure 1. Disorders associated with a higher prevalence of OSL. GH: growth hormone, PTH: parathormone, IGF-A: insulin growth factor 1, HGA: homogentisic acid.
The OSL associated disorders can be of endocrine, nutritional or metabolic nature. The main endocrine associated disorders—diabetes mellitus, acromegaly and hypoparathyroidism—are characterized by disturbances in the metabolism of glucose, growth hormone (GH), and parathyroid hormone (PTH), leading to hypocalcemia, hyperphosphatemia, hyperglycemia and hyperinsulinemia. These endocrine anomalies are often linked to obesity, which can also have a strong genetic basis. The excessive intake of fluoride and vitamin A leads to OSL resembling DISH. Disturbances in mineral metabolism namely phosphorus phosphatase and calcium can also originate disorders that have been reported in association with OSL: familial hypocalciuric hypercalcemia, hypophosphatemic rickets and hypophosphatasia.
5.1 Monogenic disorders
Table 4 lists a subset of DISH and OPLL cases originated by monogenic disorders. With the exception of alkaptonuria, characterized by the levels of Homogentisic acid, all of the other disorders are directly involved in calcium and phosphate homeostasis. As expected, genes related in hypophosphatemic rickets and hypophosphatasia are directly involved in phosphate homeostasis. However, the reports of OSL are not related to all types of hypophosphatasia disorders. Saito et al. (2011), reported a case of OPLL with hypophosphatemic rickets/osteomalacia caused by a splice donor site mutation in the ENPP1 gene. Cases of hypoparathyroidism associated with changes similar to DISH are also reported in the literature (Lambert and Becker, 1989; Unverdi et al., 2009; John and Suthar, 2016). The genes GNAS, GCM2 and PTH, closely related to hypoparathryroidism, play a role in both calcium and phosphorus metabolism. According to what we know, there is only one case described of a patient with DISH and familial hypocalciuric hypercalcemia (FHH). The patient, a 45-year-old diabetic woman, have hypercalcemia secondary to FHH and developed dysphagia because of external esophageal compression from DISH. According to the authors, the relationship between FHH and DISH remains unproven (Rivas and Lado-Abeal, 2013). Acromegaly is a rare condition of high elevated somatic growth and distorted proportions arising from hypersecretion of growth hormone (GH) and insulin-like growth factor 1 (IGF-1) due to adenomas and pathogenic pituitary secretion (Ben-Shlomo and Melmed, 2008). According to Altomonte et al. (1992), GH levels may act as bone promoting factors in DISH.
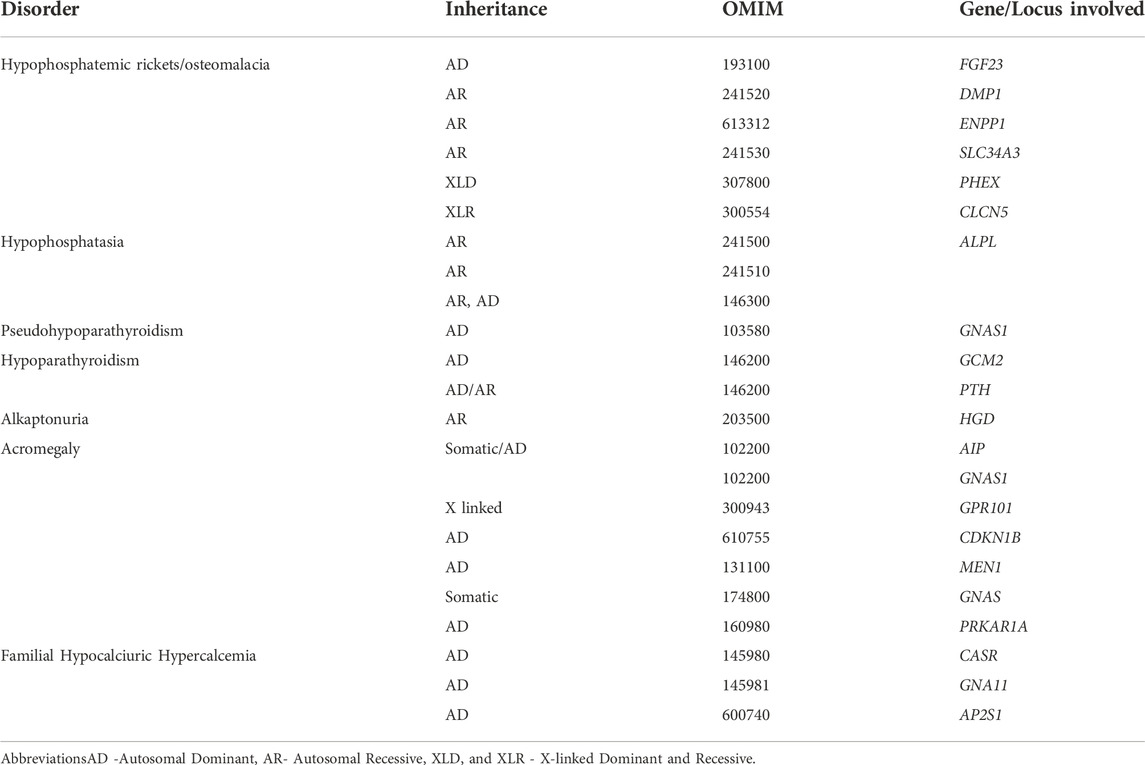
Table 4. Monogenic disorders previously associated with OSL. Lack of inheritance means that it is still unconfirmed.
5.2 “Risk-factor” complex disorders
The etiology of “risk-factor” OSL disorders is complex, and determined by the interaction of inherited and environmental factors, such as age, smoking, alcohol consumption, diet and physical inactivity. These factors, as already know, effect type 2 diabetes mellitus (T2D) and obesity, two of the known risk factor for developing DISH. Even though heterogeneous, there are some monogenic forms of these OSL disorders; see Table 5 for more details. Diabetes mellitus is considered to be a heterogeneous group of disorders having as a main characteristic persistent hyperglycemia (Pillai and Littlejohn, 2014). Obesity is considered a complex and a multifactorial disease, however there are monogenic cases reported that are related to mutations in genes of the leptin/melanocortin system involved in food intake regulation (Huvenne et al., 2016). It is interesting to see that genetic variants in LEPR gene, as occurs in the ZFR murine model, can cause obesity, hypercholesterolemia, hyperinsulinemia, hyperlipidemia and also ossification of spinal ligaments, similar to human OPLL (Okano et al., 1997). Furthermore, there are studies reporting increased levels of serum leptin in female patients with OPLL (Ikeda et al., 2011) (Feng et al., 2018) as well as in DISH patients (Tenti et al., 2017). The osteogenic effects of leptin/leptin receptor (LepR) in conjunction with mechanical stress, on the ossification of the posterior ligament, through its interaction with osteogenic markers such as osteopontin, osteocalcin and RUNX2, were also recently shown (Chen et al., 2018). It is also pertinent to mention that ENPP1 is a predisposition gene for both obesity and type 2 diabetes. The importance of leptin/LEPR in disorders such as DISH, with an important metabolic association, remain to be revealed.
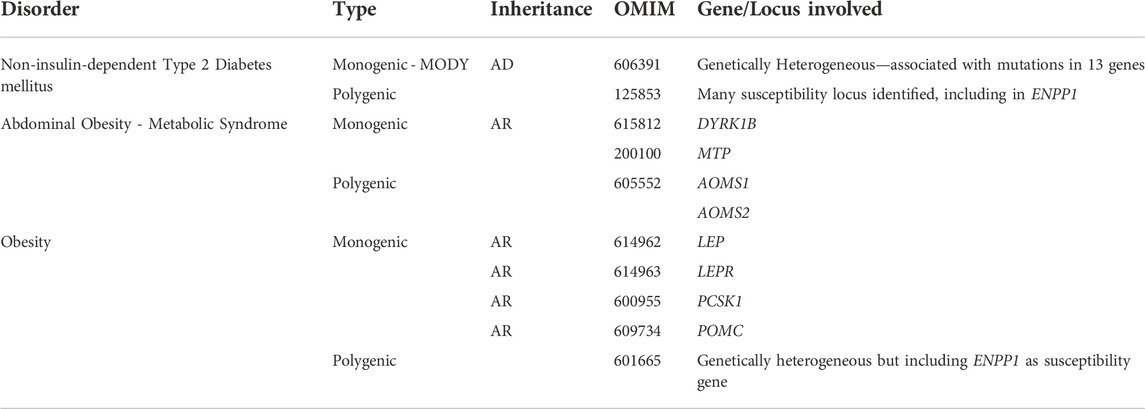
Table 5. Complex disorders previously associated with OSL. AD stands for Autosomal Dominant, AR for Autosomal Recessive. Lack of inheritance means that it is not confirmed.
5.3 Other rheumatic disorders coexisting with Ossification of Spinal Ligaments
The co-existence of DISH with other rheumatic disorders was first reported in 1950 by Forestier and Rotes Querol (Forrestier, 1950). Subsequent studies indicate, in some cases that up to 50% of DISH patients also have OPLL proposing that they share common etiopathogenic factors. Simultaneous OPLL and OLF are also very common in the literature (Li et al., 2012; Onishi et al., 2016). In addition, the co-existence of the three OSL disorders—DISH; OPLL and OLF has also been described in the literature (Guo et al., 2011). The association of DISH with psoriatic arthritis in the literature (Ben-Shlomo and Melmed, 2008) is common but studies concluded that is a side effect of retinoids treatment (Bologna et al., 1991). Other rheumatic diseases co-existing with DISH include: hyperostosis frontalis interna (Arlet et al., 1978; Mazières et al., 1978; Ciocci et al., 1985; Fukunishi et al., 1987; Fukunishi and Hosokawa, 1988), CPPD and/or CC (Resnick et al., 1978a; Bruges-Armas et al., 2006), gout (Resnick et al., 1978a; Littlejohn and Hall, 1982; Constantz, 1983; Fornaciari et al., 2009), rheumatoid arthritis (Resnick et al., 1978a; Resnick et al., 1978b; Forster et al., 1981; Mata et al., 1995), osteoarthritis (Resnick et al., 1978a), Heberden and Bouchard nodes (Schlapbach et al., 1992) and Paget’s disease (Mazières et al., 1978; Morales et al., 1993).
DISH and Ankylosing Spondylitis (AS) generally have a distinct radiographic appearance but sometimes, possibly in the early disease stages, they are difficult to distinguish radiologically (Williamson and Reginato, 1984; Olivieri et al., 1987; Olivieri et al., 1989; Rillo et al., 1989; Troise Rioda and Ferraccioli, 1990; Olivieri, 1991; Passiu et al., 1991; Maertens et al., 1992; Tishler and Yaron, 1992; Jattiot et al., 1995; Moreno et al., 1996; Kozanoglu et al., 2002; Jordana et al., 2009; Wooten, 2009; Macia-Villa et al., 2016; Kuperus et al., 2018). OPLL has also been observed in patients with AS but this coexistence is probably coincidental (Kim et al., 2007). Chondrocalcinosis, is characterized by the deposition of calcium containing crystals in synovial membranes, articular cartilage and, sometimes it can also affect periarticular soft tissues. Curiously, in some patients, the deposition of calcium crystals—hydroxyapatite or CPPD—can also occur in the spinal ligaments (Resnick and Pineda, 1984; Muthukumar et al., 2000) but this is usually difficult to differentiate from ossification (Ehara et al., 1998). ANKH is the only monogenic cause identified for CC (Table 6); a recent study described a gain-of-function mutation in the gene TNFRSF11B, which resulted in early-onset osteoarthritis and CC (Ramos et al., 2015). A recently described hereditary autosomal recessive ectopic mineralization syndrome in patients with arterial Calcification due to deficiency of CD73 (ACDC), was the result of a loss of function mutations in the 5′-nucleoside Ecto (NT5E) gene. These patients had erosive peripheral arthropathy and axial enthesopathic calcifications, resembling DISH although with decreased disc space height and the presence of large intervertebral disk calcifications (Cudrici et al., 2021). The similarities to both DISH and AS of the outcome of spine imaging of ACDC patients are noteworthy.
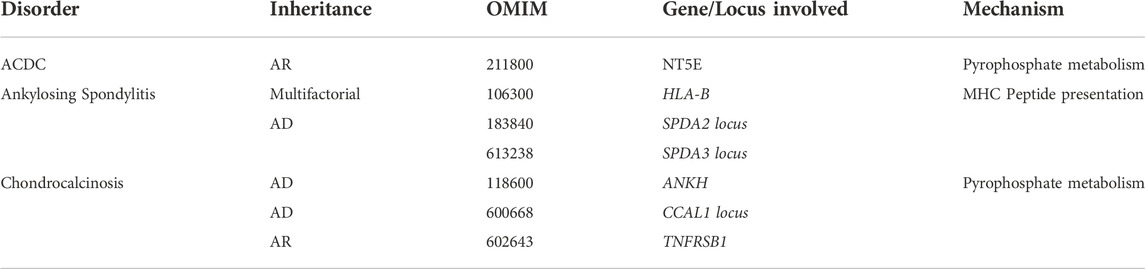
Table 6. Rheumatic disorders previously seen coexisting with OSL. AD stands for Autosomal Dominant, AR for Autosomal Recessive. Lack of inheritance means that it is not confirmed.
6 Discussion
6.1 Familial aggregation reports
The existence of a small number of family reports, with early-onset and exuberant phenotypes, in which the genetic cause was not identified and most of the times was not even investigated, raises the possibility that there are some cases of monogenic DISH and OPLL. There are possibly three main types of OSL: A sporadic form, a type that is secondary to associated metabolic disorders and a hereditary type. It is now clear that most OSL cases do not follow a simple, single gene Mendelian inheritance pattern, but instead are multifactorial disorders developing in individuals with a genetic predisposition from a variety of genetic variants in different genes.
6.2 Animal models
The existence of spontaneous and manipulated animal models for both DISH and OPLL could facilitate the identification of causal human genetic factors. It seems probable that the human phenotype of OPLL and DISH are likely to be caused by mutations in genes that underlie the animal models for these disorders. As far as we know, there are no reports of SLC29A1 (ENT1 mice model for DISH) human gene mutations in association with DISH. The association of ENPP1 with OPLL susceptibility (31, 47–49) is still unsubstantiated (50). Interestingly, in one study the authors found that the combination of variants in ENPP1 and LEPR genes was associated with the location and extension of OPLL (51). An interesting report about hypophosphatemic rickets in an OPLL patient due to a homozygous mutation in the ENPP1 gene (53), substantiates the likely importance of this gene in the etiopathogenic mechanism of OSL.
The case of the ank mouse has been quite different. In humans, analysis in the ANKH gene has identified several mutations that segregate with CC phenotype but only in a very limited subset of pedigrees. The co-coexistence of spinal ossification with CC is well supported in the literature (10, 12, 13), indicating a strong genetic link between these disorders. The genetic confirmation between spinal ossification and CC comes from two animal models—twy and ankh mice—the mouse models for OPLL and CC, develop spinal ossification and hydroxyapatite arthropathy. Both genes, ENPP1 and ANKH, regulate PPi levels thus having an essential role in bone mineralization and soft tissue calcification. The association of ENPP1 variants with Chondrocalcinosis, is considered a minor determinant of the disease (58, 59).
6.3 Genetic variants association
Three different genetic variants in COL6A1 have been associated with both DISH and OPLL. Results from these studies are inconsistent due to the type of variant associated, the lack of explanation of the pathogenic mechanism and the low numbers of individuals studied. Further progress in investigation of DISH requires a concerted approach, similar to the ones used to target the genetic basis of OPLL. In the latter case linkage studies, candidate gene association studies and even genome wide association studies were performed and revealed that OPLL is genetically heterogenous. Despite all the studies, and the large number of genes that have been associated with OSL, most of the associations are still inconsistent because genetic variants were localized in non-coding regions. Several genes involved many potential low risk effects in OSL inheritance, so there is insufficient power and analysis for their detection.
6.4 Genetics of associated disorders
The higher prevalence of OSL in patients with endocrine, nutritional and metabolic disorders made us wonder if the known genetic cause for these associated disorders could help to clarify the putative genetic pathways involved in the etiology of OSL. The ectopic calcification occurring is most probably predisposed by the balance between the expression of specific genes that act directly or indirectly on the phosphorus to calcium ratio. The crucial role of angiogenesis in DISH etiology has also been suggested, as it might be the common pathogenic background of some conditions included in metabolic syndrome. Nonetheless, there are several case reports of patients with monogenic metabolic disorders with the occurrence of DISH and OPLL.
7 Conclusion
A validated set of classification criteria for diseases characterized by ectopic mineralization of spinal tissues is of utmost importance for genetic studies so homogeneous phenotype groups can be established for investigation. This is particularly important in DISH because this disease is characterized by the ossification of the anterior spinal ligaments and generalized symmetrical enthesopathic calcifications, which may well be among the first manifestations of the disease or the main evidence of the disease in a subset of patients. At this time, DISH disease is requiring a validated set of criteria to robustly describe and establish homogeneous cohorts of patients. A more comprehensive designation of DISH, including patients with early phase disease, are clearly indispensable for genetic studies (Mader et al., 2013). On the other hand, great advances have been made in understanding the presentation of different types of OPLL.
Taken together the collected evidence suggests OSL has a heterogeneous genetic basis. The rapid advance in methods for genetic studies has brought new and interesting insights into ectopic calcification, and is providing confirmation about the importance of genes for the regulation of Pi/PPi levels, which control mineralization. Future genome-scale approaches will contribute to pinpoint susceptibility genes. However, to provide sufficient analytical power, the number of patients needs to be enlarged and the clinical/radiological disease classification, especially in DISH patients, needs substantial improvement. International collaborations are essential to increase sample size and overcome analytical challenges caused by the genetic heterogeneity of these complex diseases of calcification.
Author contributions
BP and AC wrote the manuscript; all the authors provided critical revision and contributed to the final version of the manuscript.
Funding
The present publication was funded by Fundação Ciência e Tecnologia, IP national support through CHRC (UIDP/04923/2020).
Conflict of interest
The authors declare that the research was conducted in the absence of any commercial or financial relationships that could be construed as a potential conflict of interest.
Publisher’s note
All claims expressed in this article are solely those of the authors and do not necessarily represent those of their affiliated organizations, or those of the publisher, the editors and the reviewers. Any product that may be evaluated in this article, or claim that may be made by its manufacturer, is not guaranteed or endorsed by the publisher.
References
Abiteboul, M., Mazières, B., and Ménard, H. (1985). [2 new familial cases of ankylosing vertebral hyperostosis]. Rev. Rhum. Mal. Osteoartic. 52, 645–647.
Altomonte, L., Zoli, A., Mirone, L., Marchese, G., Scolieri, P., Barini, A., et al. (1992). Growth hormone secretion in diffuse idiopathic skeletal hyperostosis. Ann. Ital. Med. Int. 7 (1), 30–33.
Arlet, P., Le Tallec, Y., Mazieres, B., Arlet, J., Timbal, X., and Boulard, C. (1978). Association between ankylosing vertebral hyperostosis and frontalis interna hyperostosis. Nouv. Presse Med. 7 (33), 2968.
Badhiwala, J. H., Ahuja, C. S., Akbar, M. A., Witiw, C. D., Nassiri, F., Furlan, J. C., et al. (2020). Degenerative cervical myelopathy - update and future directions. Nat. Rev. Neurol. 16 (2), 108–124. doi:10.1038/s41582-019-0303-0
Bai, Q., Wang, Y., Zhai, J., Wu, J., Zhang, Y., and Zhao, Y. (2022). Current understanding of tandem spinal stenosis: Epidemiology, diagnosis, and surgical strategy. EFORT Open Rev. 7 (8), 587–598. doi:10.1530/EOR-22-0016
Beardwell, A. (1969). Familial ankylosing vertebral hyperostosis with tylosis. Ann. Rheum. Dis. 28, 518–523. doi:10.1136/ard.28.5.518
Ben-Shlomo, A., and Melmed, S. (2008). Endocrinol. Metab. Clin. North Am. 37 (1), 101–122. doi:10.1016/j.ecl.2007.10.002
Bicket, A., Mehrabi, P., Naydenova, Z., Wong, V., Donaldson, L., Stagljar, I., et al. (2016). Novel regulation of equlibrative nucleoside transporter 1 (ENT1) by receptor-stimulated Ca2+-dependent calmodulin binding. Am. J. Physiol. Cell Physiol. 310 (10), C808–C820. doi:10.1152/ajpcell.00243.2015
Bologna, C., Poirier, J. L., Herisson, C., and Simon, L. (1991). Hip and spinal ossification enthesopathies induced by etretinate therapy in peripheral psoriatic arthritis. Rev. Rhum. Mal. Osteoartic. 58 (9), 595–599.
Bossens, K., Bhatti, S., Van Soens, I., Gielen, I., and Van Ham, L. (2016). Diffuse idiopathic skeletal hyperostosis of the spine in a nine-year-old cat. J. Small Anim. Pract. 57 (1), 33–35. doi:10.1111/jsap.12371
Brewerton, D. A., Hart, F. D., Nicholls, A., Caffrey, M., James, D. C., and Sturrock, R. D. (1973). Ankylosing spondylitis and HL-A 27. Lancet 1, 904–907. doi:10.1016/s0140-6736(73)91360-3
Brown, T. R., Quinn, S. F., and D'Agostino, A. N. (1991). Deposition of calcium pyrophosphate dihydrate crystals in the ligamentum flavum: Evaluation with MR imaging and CT. Radiology 178 (3), 871–873. doi:10.1148/radiology.178.3.1994435
Bruges-Armas, J., Couto, A. R., Timms, A., Santos, M. R., Bettencourt, B. F., Peixoto, M. J., et al. (2006). Ectopic calcification among families in the Azores: Clinical and radiologic manifestations in families with diffuse idiopathic skeletal hyperostosis and chondrocalcinosis. Arthritis Rheum. 54 (4), 1340–1349. doi:10.1002/art.21727
Buckley, M. F., Loveland, K. A., McKinstry, W. J., Garson, O. M., and Goding, J. W. (1990). Plasma cell membrane glycoprotein PC-1. cDNA cloning of the human molecule, amino acid sequence, and chromosomal location. J. Biol. Chem. 265 (29), 17506–17511. doi:10.1016/s0021-9258(18)38193-6
Carroll, S. H., Wigner, N. A., Kulkarni, N., Johnston-Cox, H., Gerstenfeld, L. C., and Ravid, K. (2012). A2B adenosine receptor promotes mesenchymal stem cell differentiation to osteoblasts and bone formation in vivo. J. Biol. Chem. 287 (19), 15718–15727. doi:10.1074/jbc.M112.344994
Caswell, A. M., Ali, S. Y., and Russell, R. G. (1987). Nucleoside triphosphate pyrophosphatase of rabbit matrix vesicles, a mechanism for the generation of inorganic pyrophosphate in epiphyseal cartilage. Biochim. Biophys. Acta 924 (2), 276–283. doi:10.1016/0304-4165(87)90023-7
Caswell, A. M., and Russell, R. G. (1988). Evidence that ecto-nucleoside-triphosphate pyrophosphatase serves in the generation of extracellular inorganic pyrophosphate in human bone and articular cartilage. Biochim. Biophys. Acta 966 (3), 310–317. doi:10.1016/0304-4165(88)90080-3
Chang, F., Li, L., Gao, G., Ding, S., Yang, J., Zhang, T., et al. (2017). Role of Runx2 polymorphisms in risk and prognosis of ossification of posterior longitudinal ligament. J. Clin. Lab. Anal. 31 (4), e22068. doi:10.1002/jcla.22068
Chen, S., Zhu, H., Wang, G., Xie, Z., Wang, J., and Chen, J. (2018). Combined use of leptin and mechanical stress has osteogenic effects on ossification of the posterior longitudinal ligament. Eur. Spine J. 27 (8), 1757–1766. doi:10.1007/s00586-018-5663-4
Chen, X., Guo, J., Cai, T., Zhang, F., Pan, S., Zhang, L., et al. (2016). Targeted next-generation sequencing reveals multiple deleterious variants in OPLL-associated genes. Sci. Rep. 6, 26962. doi:10.1038/srep26962
Chen, Z., Meng, H., Xing, G., Yuan, H., Zhao, F., Liu, R., et al. (2008). Age-related differences in pulmonary and cardiovascular responses to SiO2 nanoparticle inhalation: Nanotoxicity has susceptible population. Environ. Sci. Technol. 42 (23), 8985–8992. doi:10.1021/es800975u
Chin, D. K., Han, I. B., Ropper, A. E., Jeon, Y. J., Kim, D. H., Kim, Y. S., et al. (2013). Association of VKORC1-1639g>A polymorphism with susceptibility to ossification of the posterior longitudinal ligament of the spine: A Korean study. Acta Neurochir. 155 (10), 1937–1942. doi:10.1007/s00701-013-1747-4
Choi, B. W., Song, K. J., and Chang, H. (2011). Ossification of the posterior longitudinal ligament: A review of literature. Asian Spine J. 5 (4), 267–276. doi:10.4184/asj.2011.5.4.267
Chon, J., Hong, J. H., Kim, J., Han, Y. J., Lee, B. W., Kim, S. C., et al. (2014). Association between BH3 interacting domain death agonist (BID) gene polymorphism and ossification of the posterior longitudinal ligament in Korean population. Mol. Biol. Rep. 41 (2), 895–899. doi:10.1007/s11033-013-2933-4
Ciocci, A., Buratti, L., and Maurelli, G. (1985). Vertebral hyperostosis and hyperostosis frontalis interna. Rev. Rhum. Mal. Osteoartic. 52 (4), 227–230.
Constantz, R., and B, R. (1983). Case report 237: Gout associated with diffuse idiopathic skeletal hyperostosis (DISH). Skelet. Radiol. 10 (2), 117–120. doi:10.1007/BF00360796
Couto, A. R., Parreira, B., Thomson, R., Soares, M., Power, D. M., Stankovich, J., et al. (2017). Combined approach for finding susceptibility genes in DISH/chondrocalcinosis families: Whole-genome-wide linkage and IBS/IBD studies. Hum. Genome Var. 4, 17041. doi:10.1038/hgv.2017.41
Cudrici, C. D., Newman, K. A., Ferrante, E. A., Huffstutler, R., Carney, K., Betancourt, B., et al. (2021). Multifocal calcific periarthritis with distinctive clinical and radiological features in patients with CD73 deficiency. Rheumatology 61, 163–173. doi:10.1093/rheumatology/keab270
Ehara, S., Shimamura, T., NakamuRa, R., and YamazaKi, K. (1998). Paravertebral ligamentous ossification: DISH, OPLL and OLF. Eur. J. Radiol. 27 (3), 196–205. doi:10.1016/s0720-048x(97)00164-2
Elefteriou, F., Takeda, S., Ebihara, K., Magre, J., PataNoN., , Kim, C. A., et al. (2004). Serum leptin level is a regulator of bone mass. Proc. Natl. Acad. Sci. U. S. A. 101 (9), 3258–3263. doi:10.1073/pnas.0308744101
Ellman, M. H., Vazquez, T., Ferguson, L., and MaNdelN., (1978). Calcium pyrophosphate deposition in ligamentum flavum. Arthritis Rheum. 21 (5), 611–613. doi:10.1002/art.1780210512
Eytan, O., Morice-Picard, F., Sarig, O., Ezzedine, K., Isakov, O., Li, Q., et al. (2013). Cole disease results from mutations in ENPP1. Am. J. Hum. Genet. 93 (4), 752–757. doi:10.1016/j.ajhg.2013.08.007
Feng, B., Cao, S., Zhai, J., Ren, Y., Hu, J., Tian, Y., et al. (2018). Roles and mechanisms of leptin in osteogenic stimulation in cervical ossification of the posterior longitudinal ligament. J. Orthop. Surg. Res. 13 (1), 165. doi:10.1186/s13018-018-0864-4
Fornaciari, G., Giuffra, V., Giusiani, S., Fornaciari, A., Villari, N., and Vitiello, A. (2009). The 'gout' of the Medici, grand dukes of florence: A palaeopathological study. Rheumatol. Oxf. 48 (4), 375–377. doi:10.1093/rheumatology/ken493
Fornaciari, G., and Giuffra, V. (2013). The "gout of the Medici": Making the modern diagnosis using paleopathology. Gene 528 (1), 46–50. doi:10.1016/j.gene.2013.04.056
Forrestier, J., and R.-Q, J. (1950). Senile ankylosing hyperostosis of the spine. Ann. Rheum. Dis. 9, 321–330. doi:10.1136/ard.9.4.321
Forster, T. J., Leek, J. C., and Robbins, D. L. (1981). Peripheral hyperostosis in rheumatoid arthritis in the absence of axial involvement. J. Rheumatol. 8 (4), 653–657.
Fukunishi, I., Hayabara, T., and Hosokawa, K. (1987). A case of diffuse idiopathic skeletal hyperostosis (DISH) with hyperostosis frontalis interna. Rinsho Shinkeigaku 27 (8), 1013–1016.
Fukunishi, I., and Hosokawa, K. (1988). Schizophrenic psychosis with a tendency of systemic ossification. Jpn. J. Psychiatry Neurol. 42 (2), 217–222. doi:10.1111/j.1440-1819.1988.tb01973.x
Furushima, K., Shimo-Onoda, K., Maeda, S., Nobukuni, T., Ikari, K., Koga, H., et al. (2002). Large-scale screening for candidate genes of ossification of the posterior longitudinal ligament of the spine. J. Bone Min. Res. 17 (1), 128–137. doi:10.1359/jbmr.2002.17.1.128
Gorman, C., Jawad, A. S. M., and Chikanza, I. (2005). A family with diffuse idiopathic skeletal hyperostosis. Ann. Rheum. Dis. 64, 1794–1795. doi:10.1136/ard.2004.033852
Guo, Q., Lv, S. Z., Wu, S. W., Tian, X., and Li, Z. Y. (2014). Association between single nucleotide polymorphism of IL15RA gene with susceptibility to ossification of the posterior longitudinal ligament of the spine. J. Orthop. Surg. Res. 9, 103. doi:10.1186/s13018-014-0103-6
Guo, Q., Ni, B., Yang, J., Zhu, Z., and Yang, J. (2011). Simultaneous ossification of the posterior longitudinal ligament and ossification of the ligamentum flavum causing upper thoracic myelopathy in DISH: Case report and literature review. Eur. Spine J. 20 (2), S195–S201. doi:10.1007/s00586-010-1538-z
Hajjawi, M. O., MacRae, V. E., Huesa, C., Boyde, A., Millan, J. L., Arnett, T. R., et al. (2014). Mineralisation of collagen rich soft tissues and osteocyte lacunae in Enpp1(-/-) mice. Bone 69, 139–147. doi:10.1016/j.bone.2014.09.016
Hamanishi, C., Tan, A., Yamane, T., ToMiharaM., , FuKuda, K., and Tanaka, S. (1995). Ossification of the posterior longitudinal ligament. Autosomal recessive trait. Spine (Phila Pa 1976) 20 (2), 205–207. doi:10.1097/00007632-199501150-00014
Han, I. B., Ropper, A. E., Jeon, Y. J., Park, H. S., Shin, D. A., Teng, Y. D., et al. (2013). Association of transforming growth factor-beta 1 gene polymorphism with genetic susceptibility to ossification of the posterior longitudinal ligament in Korean patients. Genet. Mol. Res. 12 (4), 4807–4816. doi:10.4238/2013.February.28.26
Havelka, S., Uitterlinden, A. G., Fang, Y., Arp, P. P., Pavelkova, A., VeselaM., , et al. (2002). Collagen type I(alpha 1) and vitamin D receptor polymorphisms in diffuse idiopathic skeletal hyperostosis. Clin. Rheumatol. 21 (4), 347–348. doi:10.1007/s100670200093
Havelka, S., VeselaM., , Pavelkova, A., Ruzickova, S., Koga, H., Maeda, S., et al. (2001). Are DISH and OPLL genetically related? Ann. Rheum. Dis. 60 (9), 902–903.
He, Z., Zhu, H., Ding, L., Xiao, H., Chen, D., and XueF., (2013). Association of NPP1 polymorphism with postoperative progression of ossification of the posterior longitudinal ligament in Chinese patients. Genet. Mol. Res. 12 (4), 4648–4655. doi:10.4238/2013.October.18.3
Hinton, D. J., McGee-Lawrence, M. E., Lee, M. R., Kwong, H. K., Westendorf, J. J., and Choi, D. S. (2014). Aberrant bone density in aging mice lacking the adenosine transporter ENT1. PLoS One 9 (2), e88818. doi:10.1371/journal.pone.0088818
Horikoshi, T., Maeda, K., Kawaguchi, Y., Chiba, K., Mori, K., Koshizuka, Y., et al. (2006). A large-scale genetic association study of ossification of the posterior longitudinal ligament of the spine. Hum. Genet. 119 (6), 611–616. doi:10.1007/s00439-006-0170-9
Huvenne, H., Dubern, B., Clement, K., and Poitou, C. (2016). Rare genetic forms of obesity: Clinical approach and current treatments in 2016. Obes. Facts 9 (3), 158–173. doi:10.1159/000445061
Ikeda, Y., Nakajima, A., Aiba, A., Koda, M., Okawa, A., Takahashi, K., et al. (2011). Association between serum leptin and bone metabolic markers, and the development of heterotopic ossification of the spinal ligament in female patients with ossification of the posterior longitudinal ligament. Eur. Spine J. 20 (9), 1450–1458. doi:10.1007/s00586-011-1688-7
Ikegawa, S. (2007). in Tiptoe walking (ttw) mouse in OPLL: Ossification of the posterior longitundinal ligament. Editors K. Yonenobu, K. Nakamura, and Y. Toyama (Hicom, Japan: Springer), 71–76.
Ikuma, H., Hirose, T., Nakamura, D., Yamashita, K., Ueda, M., Sasaki, K., et al. (2022). The prevalence and characteristics of diffuse idiopathic skeletal hyperostosis (DISH): A cross-sectional study of 1519 Japanese individuals. Diagn. (Basel) 12 (5), 1088. doi:10.3390/diagnostics12051088
Jattiot, F., Goupille, P., and Valat, J. P. (1995). Coexistence of diffuse idiopathic skeletal hyperostosis and ankylosing spondylitis in two patients. Clin. Exp. Rheumatol. 13 (3), 405–406.
Jekarl, D. W., Paek, C. M., An, Y. J., Kim, Y. J., Kim, M., Kim, Y., et al. (2013). TGFBR2 gene polymorphism is associated with ossification of the posterior longitudinal ligament. J. Clin. Neurosci. 20 (3), 453–456. doi:10.1016/j.jocn.2012.05.031
John, D. R., and Suthar, P. P. (2016). Radiological features of long-standing hypoparathyroidism. Pol. J. Radiol. 81, 42–45. doi:10.12659/PJR.896104
Jordana, X., Galtes, I., Couto, A. R., Gales, L., Damas, M., Lima, M., et al. (2009). The coexistence of ankylosing spondylitis and diffuse idiopathic skeletal hyperostosis--a postmortem diagnosis. Clin. Rheumatol. 28 (3), 353–356. doi:10.1007/s10067-008-1068-9
Jun, J. K., and Kim, S. M. (2012). Association study of fibroblast growth factor 2 and fibroblast growth factor receptors gene polymorphism in Korean ossification of the posterior longitudinal ligament patients. J. Korean Neurosurg. Soc. 52 (1), 7–13. doi:10.3340/jkns.2012.52.1.7
Kamiya, M., HarAdA, A., MizunoM., , Iwata, H., and Yamada, Y. (2001). Association between a polymorphism of the transforming growth factor-beta1 gene and genetic susceptibility to ossification of the posterior longitudinal ligament in Japanese patients. Spine 1976 26 (11), 1264–1266. doi:10.1097/00007632-200106010-00017
Karasugi, T., Nakajima, M., Ikari, K., Tsuji, T., Matsumoto, M., and Chiba, K. (2013). A genome-wide sib-pair linkage analysis of ossification of the posterior longitudinal ligament of the spine. J. Bone Min. Metab. 31 (2), 136–143. doi:10.1007/s00774-012-0404-y
Kato, K., Nishimasu, H., Okudaira, S., Mihara, E., Ishitani, R., Takagi, J., et al. (2012). Crystal structure of Enpp1, an extracellular glycoprotein involved in bone mineralization and insulin signaling. Proc. Natl. Acad. Sci. U. S. A. 109 (42), 16876–16881. doi:10.1073/pnas.1208017109
Kawaguchi, Y., Furushima, K., Sugimori, K., Inoue, I., and Kimura, T. (2003). Association between polymorphism of the transforming growth factor-beta1 gene with the radiologic characteristic and ossification of the posterior longitudinal ligament. Spine 1976 28 (13), 1424–1426. doi:10.1097/01.BRS.0000068245.27017.9F
Kim, D. H., Jeong, Y. S., Chon, J., Yoo, S. D., Kim, H. S., Kang, S. W., et al. (2011). Association between interleukin 15 receptor, alpha (IL15RA) polymorphism and Korean patients with ossification of the posterior longitudinal ligament. Cytokine 55 (3), 343–346. doi:10.1016/j.cyto.2011.05.016
Kim, D. H., Yun, D. H., Kim, H. S., Min, S. K., Yoo, S. D., Lee, K. H., et al. (2014). The insertion/deletion polymorphism of Angiotensin I converting Enzyme gene is associated with ossification of the posterior longitudinal ligament in the Korean population. Ann. Rehabil. Med. 38 (1), 1–5. doi:10.5535/arm.2014.38.1.1
Kim, K. H., Kuh, S. U., Park, J. Y., Lee, S. J., Park, H. S., Chin, D. K., et al. (2014). Association between BMP-2 and COL6A1 gene polymorphisms with susceptibility to ossification of the posterior longitudinal ligament of the cervical spine in Korean patients and family members. Genet. Mol. Res. 13 (1), 2240–2247. doi:10.4238/2014.March.31.4
Kim, K. T., Kim, D. H., Chung, J. Y., Lee, S., Joo, J., Nah, S. S., et al. (2012). Association of interferon gamma polymorphism with ossification of the posterior longitudinal ligament in the Korean population. Immunol. Invest. 41 (8), 876–887. doi:10.3109/08820139.2012.714437
Kim, T. J., Jun, J. B., Joo, K. B., and Uhm, W. S. (2007). Prevalence of ossification of posterior longitudinal ligament in patients with ankylosing spondylitis. J. Rheumatol. 34 (12), 2460–2462.
Kobashi, G., Ohta, K., Washio, M., Okamoto, K., Sasaki, S., Yokoyama, T., et al. (2008). FokI variant of vitamin D receptor gene and factors related to atherosclerosis associated with ossification of the posterior longitudinal ligament of the spine: A multi-hospital case-control study. Spine 1976 33 (16), E553–E558. doi:10.1097/BRS.0b013e31817e9de2
Koga, H., Sakou, T., TakEtomi, E., Hayashi, K., Numasawa, T., Harata, S., et al. (1998). Genetic mapping of ossification of the posterior longitudinal ligament of the spine. Am. J. Hum. Genet. 62 (6), 1460–1467. doi:10.1086/301868
Kong, Q., Ma, X., Li, F., Guo, Z., Qi, Q., Li, W., et al. (2007). COL6A1 polymorphisms associated with ossification of the ligamentum flavum and ossification of the posterior longitudinal ligament. Spine 1976 32 (25), 2834–2838. doi:10.1097/BRS.0b013e31815b761c
Koshizuka, Y., Kawaguchi, H., Ogata, N., Ikeda, T., Mabuchi, A., Seichi, A., et al. (2002). Nucleotide pyrophosphatase gene polymorphism associated with ossification of the posterior longitudinal ligament of the spine. J. Bone Min. Res. 17 (1), 138–144. doi:10.1359/jbmr.2002.17.1.138
Kozanoglu, E., Guzel, R., Guler-UysalF., , and Goncu, K. (2002). Coexistence of diffuse idiopathic skeletal hyperostosis and ankylosing spondylitis: A case report. Clin. Rheumatol. 21 (3), 258–260. doi:10.1007/pl00011224
Kranenburg, H. C., Westerveld, L. A., Verlaan, J. J., Oner, F. C., Dhert, W. J. A., Voorhout, G., et al. (2010). The dog as an animal model for DISH? Eur. Spine J. 19 (8), 1325–1329. doi:10.1007/s00586-010-1280-6
Kranenburg, H. J., Hazewinkel, H. A., and Meij, B. P. (2014). Naturally occurring spinal hyperostosis in dogs as a model for human spinal disorders. ILAR J. 55 (1), 150–163. doi:10.1093/ilar/ilu012
Kuperus, J. S., Waalwijk, J. F., Regan, E. A., van der Horst-Bruinsma, I. E., Oner, F. C., de Jong, P. A., et al. (2018). Simultaneous occurrence of ankylosing spondylitis and diffuse idiopathic skeletal hyperostosis: A systematic review. Rheumatol. Oxf. 57 (12), 2120–2128. doi:10.1093/rheumatology/key211
Lambert, R. G., and Becker, E. J. (1989). Diffuse skeletal hyperostosis in idiopathic hypoparathyroidism. Clin. Radiol. 40 (2), 212–215. doi:10.1016/s0009-9260(89)80101-1
Levy-Litan, V., Hershkovitz, E., Avizov, L., Leventhal, N., Bercovich, D., Chalifa-Caspi, V., et al. (2010). Autosomal-recessive hypophosphatemic rickets is associated with an inactivation mutation in the ENPP1 gene. Am. J. Hum. Genet. 86 (2), 273–278. doi:10.1016/j.ajhg.2010.01.010
Li, M., Meng, H., Du, J., Tao, H., Luo, Z., and Wang, Z. (2012). Management of thoracic myelopathy caused by ossification of the posterior longitudinal ligament combined with ossification of the ligamentum flavum-a retrospective study. Spine J. 12 (12), 1093–1102. doi:10.1016/j.spinee.2012.10.022
Lim, J. J., Shin, D. A., Jeon, Y. J., Kumar, H., Sohn, S., Min, H. S., et al. (2016). Association of miR-146a, miR-149, miR-196a2, and miR-499 polymorphisms with ossification of the posterior longitudinal ligament of the cervical spine. PLoS One 11 (7), e0159756. doi:10.1371/journal.pone.0159756
Littlejohn, G. O., and Hall, S. (1982). Diffuse idiopathic skeletal hyperostosis and new bone formation in male gouty subjects. A radiologic study. Rheumatol. Int. 2 (2), 83–86. doi:10.1007/BF00541250
Liu, G. Y., Liang, Q. H., Cui, R. R., Liu, Y., Wu, S. S., Shan, P. F., et al. (2014). Leptin promotes the osteoblastic differentiation of vascular smooth muscle cells from female mice by increasing RANKL expression. Endocrinology 155 (2), 558–567. doi:10.1210/en.2013-1298
Liu, Y., Zhao, Y., Chen, Y., Shi, G., and Yuan, W. (2010). RUNX2 polymorphisms associated with OPLL and OLF in the Han population. Clin. Orthop. Relat. Res. 468 (12), 3333–3341. doi:10.1007/s11999-010-1511-5
Macia-Villa, C. C., Sifuentes-Giraldo, W. A., and Medina-Luezas, J. (2016). Simultaneous occurrence of ankylosing spondylitis and diffuse idiopathic skeletal hyperostosis (Forestier-Rotes-Querol disease). Reumatol. Clin. 57, 2120–2128. doi:10.1093/rheumatology/key211
Mader, R., Verlaan, J. J., and Buskila, D. (2013). Diffuse idiopathic skeletal hyperostosis: Clinical features and pathogenic mechanisms. Nat. Rev. Rheumatol. 9 (12), 741–750. doi:10.1038/nrrheum.2013.165
Maeda, S., Ishidou, Y., Koga, H., TakEtomi, E., Ikari, K., Komiya, S., et al. (2001). Functional impact of human collagen alpha2(XI) gene polymorphism in pathogenesis of ossification of the posterior longitudinal ligament of the spine. J. Bone Min. Res. 16 (5), 948–957. doi:10.1359/jbmr.2001.16.5.948
Maeda, S., Koga, H., MatSunaga, S., Numasawa, T., Ikari, K., Furushima, K., et al. (2001). Gender-specific haplotype association of collagen alpha2 (XI) gene in ossification of the posterior longitudinal ligament of the spine. J. Hum. Genet. 46 (1), 1–4. doi:10.1007/s100380170117
Maertens, M., Mielants, H., Verstraete, K., and Veys, E. M. (1992). Simultaneous occurrence of diffuse idiopathic skeletal hyperostosis and ankylosing spondylitis in the same patient. J. Rheumatol. 19 (12), 1978–1983.
Mata, S., WolFeF., , Joseph, L., and Esdaile, J. M. (1995). Absence of an association of rheumatoid arthritis and diffuse idiopathic skeletal hyperostosis: A case-control study. J. Rheumatol. 22 (11), 2062–2064.
Matsunaga, S., and Sakou, T. (2006). in Opll: Disease entity, incidence, literature search, and prognosis. Editors K. Y. OPLL, K. Nakamura, and Y. Toyama (Tokyo: Springer).
Matsunaga, S., YaMaguchiM., , Hayashi, K., and Sakou, T. (1999). Genetic analysis of ossification of the posterior longitudinal ligament. Spine (Phila Pa 1976) 24 (10), 937–938. doi:10.1097/00007632-199905150-00002
Mazières, B., Jung-Rozenfarb, M., and Arlet, J. (1978). Paget's disease, ankylosing vertebral hyperostosis and hyperostosis frontalis interna. Sem. Hop. 54 (13-16), 521–525.
Meng, X. L., Wang, H., Yang, H., Hai, Y., Tian, B. p., and Lin, X. (2010). T allele at site 6007 of bone morphogenetic protein-4 gene increases genetic susceptibility to ossification of the posterior longitudinal ligament in male Chinese Han population. Chin. Med. J. 123 (18), 2537–2542.
Miyasaka, K., Kaneda, K., Ito, T., Takei, H., Sugimoto, S., and TsuruM., (1982). Ossification of spinal ligaments causing thoracic radiculomyelopathy. Radiology 143 (2), 463–468. doi:10.1148/radiology.143.2.7071348
Montague, C. T., Farooqi, I. S., Whitehead, J. P., Soos, M. A., Rau, H., Wareham, N. J., et al. (1997). Congenital leptin deficiency is associated with severe early-onset obesity in humans. Nature 387 (6636), 903–908. doi:10.1038/43185
Morales, A. A., Valdazo, P., Corres, J., Talbot, J. R., PerezF., , and Baylink, D. J. (1993). Coexistence of Paget's bone disease and diffuse idiopathic skeletal hyperostosis in males. Clin. Exp. Rheumatol. 11 (4), 361–365.
Moreno, A. C., Gonzalez, M. L., DuffinM., , Lopez-Longo, F. J., Carreno, L., and Forrester, D. M. (1996). Simultaneous occurrence of diffuse idiopathic skeletal hyperostosis and ankylosing spondylitis. Rev. Rhum. Engl. Ed. 63 (4), 292–295.
Muthukumar, N., Karuppaswamy, U., and Sankarasubbu, B. (2000). Calcium pyrophosphate dihydrate deposition disease causing thoracic cord compression: Case report. Neurosurgery 46 (1), 222–225. doi:10.1097/00006123-200001000-00047
Nakajima, M., Kou, I., Ohashi, H., and Ikegawa, S. (2016). Identification and functional characterization of RSPO2 as a susceptibility gene for ossification of the posterior longitudinal ligament of the spine. Am. J. Hum. Genet. 99 (1), 202–207. doi:10.1016/j.ajhg.2016.05.018
Nakajima, M., Takahashi, A., Tsuji, T., Karasugi, T., Baba, H., Uchida, K., et al. (2014). A genome-wide association study identifies susceptibility loci for ossification of the posterior longitudinal ligament of the spine. Nat. Genet. 46 (9), 1012–1016. doi:10.1038/ng.3045
Nakamura, I., Ikegawa, S., OkAwA, A., Okuda, S., Koshizuka, Y., KawagucHi, H., et al. (1999). Association of the human NPPS gene with ossification of the posterior longitudinal ligament of the spine (OPLL). Hum. Genet. 104 (6), 492–497. doi:10.1007/s004390050993
Nitschke, Y., and Rutsch, F. (2012). Generalized arterial calcification of infancy and pseudoxanthoma elasticum: Two sides of the same coin. Front. Genet. 3, 302. doi:10.3389/fgene.2012.00302
Nobta, M., Tsukazaki, T., Shibata, Y., Xin, C., Moriishi, T., Sakano, S., et al. (2005). Critical regulation of bone morphogenetic protein-induced osteoblastic differentiation by Delta1/Jagged1-activated Notch1 signaling. J. Biol. Chem. 280 (16), 15842–15848. doi:10.1074/jbc.M412891200
Nouri, A., Tetreault, L., Singh, A., Karadimas, S. K., and Fehlings, M. G. (2015). Degenerative cervical myelopathy: Epidemiology, genetics, and pathogenesis. Spine (Phila Pa 1976) 40 (12), E675–E693. doi:10.1097/BRS.0000000000000913
Numasawa, T., Koga, H., Ueyama, K., Maeda, S., Sakou, T., Harata, S., et al. (1999). Human retinoic X receptor beta: Complete genomic sequence and mutation search for ossification of posterior longitudinal ligament of the spine. J. Bone Min. Res. 14 (4), 500–508. doi:10.1359/jbmr.1999.14.4.500
Ogata, N., Koshizuka, Y., Miura, T., Iwasaki, M., Hosoi, T., Shiraki, M., et al. (2002). Association of bone metabolism regulatory factor gene polymorphisms with susceptibility to ossification of the posterior longitudinal ligament of the spine and its severity. Spine 1976 27 (16), 1765–1771. doi:10.1097/00007632-200208150-00015
Okano, T., Ishidou, Y., KatoM., , Imamura, T., Yonemori, K., OriguchiN., , et al. (1997). Orthotopic ossification of the spinal ligaments of zucker fatty rats: A possible animal model for ossification of the human posterior longitudinal ligament. J. Orthop. Res. 15 (6), 820–829. doi:10.1002/jor.1100150606
Okawa, A., Goto, S., Moriya, H., Nakamura, Y., and Ikegawa, S. (1998). Mutation in Npps in a mouse model of ossification of the posterior longitudinal ligament of the spine. Nat. Genet. 19 (3), 271–273. doi:10.1038/956
Okazaki, T., SaiTo, T., MiTomo, T., and Siota, Y. (1976). Pseudogout: Clinical observations and chemical analyses of deposits. Arthritis Rheum. 19 (3), 293–305. doi:10.1002/1529-0131(197605/06)19:3+<293:aid-art1780190704>3.0.co;2-r
Olivieri, I. (1991). Coexisting diffuse idiopathic skeletal hyperostosis and ankylosing spondylitis. Clin. Rheumatol. 10 (1), 95. doi:10.1007/BF02208043
Olivieri, I., Trippi, D., Gherardi, S., and Pasero, G. (1987). Coexistence of ankylosing spondylitis and diffuse idiopathic skeletal hyperostosis: Another report. J. Rheumatol. 14 (5), 1058–1060.
Olivieri, I., Vitali, C., GemiGnani, G., and Pasero, G. (1989). Concomitant ankylosing spondylitis and DISH. J. Rheumatol. 16 (8), 1170–1172.
Onishi, E., Sano, H., and Matsushita, M. (2016). Surgical treatment for thoracic myelopathy due to simultaneous ossification of the posterior longitudinal ligament and ligamentum flavum at the same level. Clin. Spine Surg. 29, E389–E395. doi:10.1097/BSD.0000000000000059
Ostrander, E. A., Galibert, F., and Patterson, D. F. (2000). Canine genetics comes of age. Trends Genet. 16 (3), 117–124. doi:10.1016/s0168-9525(99)01958-7
Parreira, B., Couto, A. R., Rocha, F., Sousa, M., Faustino, V., Power, D. M., et al. (2020). Whole exome sequencing of patients with diffuse idiopathic skeletal hyperostosis and calcium pyrophosphate crystal chondrocalcinosis. Acta Reumatol. Port. 45 (2), 116–126.
Passiu, G., Sebastiani, G. D., and Tuveri, M. A. (1991). Diffuse idiopathic skeletal hyperostosis of the cervical spine in a patient with ankylosing spondylitis. Description of a personal case. Ann. Ital. Med. Int. 6 (1), 61–64.
Phillips, M. S., Liu, Q., Hammond, H. A., Dugan, V., Hey, P. J., Caskey, C. J., et al. (1996). Leptin receptor missense mutation in the fatty Zucker rat. Nat. Genet. 13 (1), 18–19. doi:10.1038/ng0596-18
Pillai, S., and Littlejohn, G. (2014). Metabolic factors in diffuse idiopathic skeletal hyperostosis - a review of clinical data. Open Rheumatol. J. 8, 116–128. doi:10.2174/1874312901408010116
Prabhu, R. M., Rathod, T. N., Mohanty, S. S., Hadole, B. S., Marathe, N. A., and Rai, A. K. (2022). A case of cervical OPLL and DISH mimicking stroke. Surg. Neurol. Int. 13, 158. doi:10.25259/SNI_247_2022
Qu, X., Chen, Z., Fan, D., Xiang, S., Sun, C., Zeng, Y., et al. (2017). Two novel BMP-2 variants identified in patients with thoracic ossification of the ligamentum flavum. Eur. J. Hum. Genet. 25 (5), 565–571. doi:10.1038/ejhg.2017.2
Qu, X., Hou, X., Chen, Z., Chen, G., Fan, T., and Yang, X. (2021). Association analysis and functional study of COL6A1 single nucleotide polymorphisms in thoracic ossification of the ligamentum flavum in the Chinese Han population. Eur. Spine J. 30 (10), 2782–2790. doi:10.1007/s00586-021-06932-y
Ramos, Y. F., Bos, S. D., van der Breggen, R., Kloppenburg, M., Ye, K., Lameijer, E. W. E. M. W., et al. (2015). A gain of function mutation in TNFRSF11B encoding osteoprotegerin causes osteoarthritis with chondrocalcinosis. Ann. Rheum. Dis. 74 (9), 1756–1762. doi:10.1136/annrheumdis-2013-205149
Ren, Y., Feng, J., Liu, Z. z., Wan, H., Li, J. h., and Lin, X. (2012). A new haplotype in BMP4 implicated in ossification of the posterior longitudinal ligament (OPLL) in a Chinese population. J. Orthop. Res. 30 (5), 748–756. doi:10.1002/jor.21586
Ren, Y., Liu, Z. z., Feng, J., Wan, H., Li, J. h., Wang, H., et al. (2012). Association of a BMP9 haplotype with ossification of the posterior longitudinal ligament (OPLL) in a Chinese population. PLoS One 7 (7), e40587. doi:10.1371/journal.pone.0040587
Resnick, D., Curd, J., Shapiro, R. F., and Wiesner, K. B. (1978). Radiographic abnormalities of rheumatoid arthritis in patients with diffuse idiopathic skeletal hyperostosis. Arthritis Rheum. 21 (1), 1–5. doi:10.1002/art.1780210101
Resnick, D., and Pineda, C. (1984). Vertebral involvement in calcium pyrophosphate dihydrate crystal deposition disease. Radiographic-pathological correlation. Radiology 153 (1), 55–60. doi:10.1148/radiology.153.1.6089266
Resnick, D., Shapiro, R. F., Wiesner, K. B., Niwayama, G., Utsinger, P. D., and Shaul, S. R. (1978). Diffuse idiopathic skeletal hyperostosis (DISH) [ankylosing hyperostosis of Forestier and Rotes-Querol]. Semin. Arthritis Rheum. 7 (3), 153–187. doi:10.1016/0049-0172(78)90036-7
Rillo, O. L., Scheines, E. J., Moreno, C., Barreira, J. C., Porrini, A. A., and Maldonado Cocco, J. A. (1989). Coexistence of diffuse idiopathic skeletal hyperostosis and ankylosing spondylitis. Clin. Rheumatol. 8 (4), 499–503. doi:10.1007/BF02032103
Rivas, A. M., and Lado-Abeal, J. (2013). Diffuse idiopathic skeletal hyperostosis and familial hypocalciuric hypercalcemia: A unique association in a young female. Am. J. Med. Sci. 346 (3), 247–249. doi:10.1097/MAJ.0b013e31828b663b
Rutsch, F., Ruf, N., Vaingankar, S., Toliat, M. R., Suk, A., Hohne, W., et al. (2003). Mutations in ENPP1 are associated with 'idiopathic' infantile arterial calcification. Nat. Genet. 34 (4), 379–381. doi:10.1038/ng1221
Saito, T., Shimizu, Y., Hori, M., Taguchi, M., Igarashi, T., Fukumoto, S., et al. (2011). A patient with hypophosphatemic rickets and ossification of posterior longitudinal ligament caused by a novel homozygous mutation in ENPP1 gene. Bone 49 (4), 913–916. doi:10.1016/j.bone.2011.06.029
Sakou, T., TakEtomi, E., MatSunaga, S., YaMaguchiM., , Sonoda, S., and YaShiki, S. (1991). Genetic study of ossification of the posterior longitudinal ligament in the cervical spine with human leukocyte antigen haplotype. Spine (Phila Pa 1976) 16 (11), 1249–1252. doi:10.1097/00007632-199111000-00001
Saleem, S. N., and Hawass, Z. (2014). Ankylosing spondylitis or diffuse idiopathic skeletal hyperostosis in royal Egyptian mummies of 18th -20th dynasties? CT and archaeology studies. Arthritis Rheumatol. 66 (12), 3311–3316. doi:10.1002/art.38864
Schlapbach, P., Beyeler, C., Gerber, N. J., van der Linden, S., Burgi, U., Fuchs, W. A., et al. (1992). The prevalence of palpable finger joint nodules in diffuse idiopathic skeletal hyperostosis (DISH). A controlled study. Br. J. Rheumatol. 31 (8), 531–534. doi:10.1093/rheumatology/31.8.531
Schlosstein, L., Terasaki, P. I., Bluestone, R., and Pearson, C. M. (1973). High association of an HL-A antigen, W27, with ankylosing spondylitis. N. Engl. J. Med. 288 (14), 704–706. doi:10.1056/NEJM197304052881403
Stefan, C., Jansen, S., and Bollen, M. (2005). NPP-Type ectophosphodiesterases: Unity in diversity. Trends biochem. Sci. 30 (10), 542–550. doi:10.1016/j.tibs.2005.08.005
Tahara, M., Aiba, A., Yamazaki, M., Ikeda, Y., Goto, S., Moriya, H., et al. (2005). The extent of ossification of posterior longitudinal ligament of the spine associated with nucleotide pyrophosphatase gene and leptin receptor gene polymorphisms. Spine (Phila Pa 1976) 30 (8), 877–880. doi:10.1097/01.brs.0000160686.18321.ad
Takayuki, M., Takashi, H., Toshitaka, Y., Hiroyuki, I., Yu, M., Satoru, E., et al. (2021). Association between diffuse idiopathic skeletal hyperostosis and thoracic kyphosis in patients with cervical myelopathy: A retrospective observational study. BMC Musculoskelet. Disord. 22 (1), 964. doi:10.1186/s12891-021-04851-z
Tanabe, J., Sarin, H., and Kindt, G. (2002). Familial ossification of the posterior longitudinal ligament (OPLL) in the thoracic spine: Case report. Surg. Neurol. 58 (6), 403–405. doi:10.1016/s0090-3019(02)00927-8
Tanaka, T., Ikari, K., Furushima, K., Okada, A., Tanaka, H., Furukawa, K. I., et al. (2003). Genomewide linkage and linkage disequilibrium analyses identify COL6A1, on chromosome 21, as the locus for ossification of the posterior longitudinal ligament of the spine. Am. J. Hum. Genet. 73 (4), 812–822. doi:10.1086/378593
Tanikawa, E., Furuya, K., and Nakajima, H. (1986). Genetic study on ossification of posterior longitudinal ligament. Bull. Tokyo Med. Dent. Univ. 33 (3), 117–128.
Tenti, S., Palmitesta, P., GiordaNoN., , GaleazziM., , and FiorAvAnti, A. (2017). Increased serum leptin and visfatin levels in patients with diffuse idiopathic skeletal hyperostosis: A comparative study. Scand. J. Rheumatol. 46 (2), 156–158. doi:10.1080/03009742.2016.1188981
Terayama, K. (1989). Genetic studies on ossification of the posterior longitudinal ligament of the spine. Spine (Phila Pa 1976) 14 (11), 1184–1191. doi:10.1097/00007632-198911000-00009
Tishler, M., and Yaron, M. (1992). Two cases of diffuse idiopathic skeletal hyperostosis and ankylosing spondylitis. Br. J. Rheumatol. 31 (8), 569–571. doi:10.1093/rheumatology/31.8.569-b
Togni, A., Morgan, J. P., and SteFFenF., (2014). Radiographic and MRI characteristics of lumbar disseminated idiopathic spinal hyperostosis and spondylosis deformans in dogs. J. Small Anim. Pract. 55 (7), 343–349. doi:10.1111/jsap.12218
Troise Rioda, W., and Ferraccioli, G. F. (1990). DISH and ankylosing spondylitis. Case report and review of the literature. Clin. Exp. Rheumatol. 8 (6), 591–593.
Tsukahara, S., Miyazawa, N., Akagawa, H., Forejtova, S., Pavelka, K., Tanaka, T., et al. (2005). COL6A1, the candidate gene for ossification of the posterior longitudinal ligament, is associated with diffuse idiopathic skeletal hyperostosis in Japanese. Spine 30 (20), 2321–2324. doi:10.1097/01.brs.0000182318.47343.6d
Unverdi, S., Ozturk, M. A., Inal, S., Selek, H., Goker, B., Haznedaroglu, C., et al. (2009). Idiopathic hypoparathyroidism mimicking diffuse idiopathic skeletal hyperostosis. J. Clin. Rheumatol. 15 (7), 361–362. doi:10.1097/RHU.0b013e3181bb9865
van der Korst, J. K., Geerards, J., and Driessens, F. C. (1974). A hereditary type of idiopathic articular chondrocalcinosis. Survey of a pedigree. Am. J. Med. 56 (3), 307–314. doi:10.1016/0002-9343(74)90612-3
Wang, H., Jin, W., and Li, H. (2018). Genetic polymorphisms in bone morphogenetic protein receptor type IA gene predisposes individuals to ossification of the posterior longitudinal ligament of the cervical spine via the smad signaling pathway. BMC Musculoskelet. Disord. 19 (1), 61. doi:10.1186/s12891-018-1966-1
Wang, H., Yang, Z. h., Liu, D. m., Wang, L., Meng, X. l., and Tian, B. p. (2008). Association between two polymorphisms of the bone morpho-genetic protein-2 gene with genetic susceptibility to ossification of the posterior longitudinal ligament of the cervical spine and its severity. Chin. Med. J. 121 (18), 1806–1810. doi:10.1097/00029330-200809020-00010
Wang, P., Liu, X., Zhu, B., Ma, Y., Yong, L., Teng, Z., et al. (2018). Association of IL17RC and COL6A1 genetic polymorphisms with susceptibility to ossification of the thoracic posterior longitudinal ligament in Chinese patients. J. Orthop. Surg. Res. 13 (1), 109. doi:10.1186/s13018-018-0817-y
Wang, P., Liu, X., Zhu, B., Ma, Y., Yong, L., Teng, Z., et al. (2018). Identification of susceptibility loci for thoracic ossification of the posterior longitudinal ligament by whole-genome sequencing. Mol. Med. Rep. 17 (2), 2557–2564. doi:10.3892/mmr.2017.8171
Warraich, S., Bone, D. B. J., Quinonez, D., Ii, H., Choi, D. S., Holdsworth, D. W., et al. (2013). Loss of equilibrative nucleoside transporter 1 in mice leads to progressive ectopic mineralization of spinal tissues resembling diffuse idiopathic skeletal hyperostosis in humans. J. Bone Min. Res. 28 (5), 1135–1149. doi:10.1002/jbmr.1826
Wei, W., He, H. L., Chen, C. Y., Zhao, Y., Jiang, H. L., Liu, W. T., et al. (2014). Whole exome sequencing implicates PTCH1 and COL17A1 genes in ossification of the posterior longitudinal ligament of the cervical spine in Chinese patients. Genet. Mol. Res. 13 (1), 1794–1804. doi:10.4238/2014.March.17.7
Williamson, P. K., and Reginato, A. J. (1984). Diffuse idiopathic skeletal hyperostosis of the cervical spine in a patient with ankylosing spondylitis. Arthritis Rheum. 27 (5), 570–573. doi:10.1002/art.1780270514
Wooten, M. (2009). The coexistence of ankylosing spondylitis and diffuse idiopathic skeletal hyperostosis. Clin. Rheumatol. 28 (5), 619. doi:10.1007/s10067-009-1111-5
Yamagami, T., Kawano, N., and Nakano, H. (2000). Calcification of the cervical ligamentum flavum--case report. Neurol. Med. Chir. 40 (4), 234–238. doi:10.2176/nmc.40.234
Yamaguchi, M. (1991). Genetic study on OPLL in the cervical spine with HLA haplotype. Nihon Seikeigeka Gakkai Zasshi 65 (8), 527–535.
Yamazaki, M., Moriya, H., Goto, S., Saitoh, Y., Arai, K., and Nagai, Y. (1991). Increased type XI collagen expression in the spinal hyperostotic mouse (TWY/TWY). Calcif. Tissue Int. 48, 182–189. doi:10.1007/bf02570553
Yan, L., Chang, Z., Liu, Y., Li, Y. B., He, B. R., and Hao, D. J. (2013). A single nucleotide polymorphism in the human bone morphogenetic protein-2 gene (109T > G) affects the Smad signaling pathway and the predisposition to ossification of the posterior longitudinal ligament of the spine. Chin. Med. J. 126 (6), 1112–1118.
Yan, L., Zhao, W. g., Li, J. j., Yang, H., Wang, H., and Lin, X. (2010). Linkage of three polymorphisms on chromosome 20p12 to ossification of the posterior longitudinal ligament of spine and its severity in Han Chinese patients. Chin. Med. J. 123 (17), 2341–2346.
Keywords: ossification, genetics, ectopic calcification, diffuse idiopathic skeletal hyperostosis, ossification of posterior longitudinal ligament
Citation: Couto AR, Parreira B, Power DM, Pinheiro L, Madruga Dias J, Novofastovski I, Eshed I, Sarzi-Puttini P, Pappone N, Atzeni F, Verlaan J-J, Kuperus J, Bieber A, Ambrosino P, Kiefer D, Khan MA, Mader R, Baraliakos X and Bruges-Armas J (2022) Evidence for a genetic contribution to the ossification of spinal ligaments in Ossification of Posterior Longitudinal Ligament and Diffuse idiopathic skeletal hyperostosis: A narrative review. Front. Genet. 13:987867. doi: 10.3389/fgene.2022.987867
Received: 06 July 2022; Accepted: 16 September 2022;
Published: 07 October 2022.
Edited by:
Jordi Pérez-Tur, Institute of Biomedicine of Valencia (CSIC), SpainReviewed by:
Michael G. Fehlings, Toronto Western Hospital, CanadaCaterina Licini, Università Politecnica delle Marche, Italy
Copyright © 2022 Couto, Parreira, Power, Pinheiro, Madruga Dias, Novofastovski, Eshed, Sarzi-Puttini, Pappone, Atzeni, Verlaan, Kuperus, Bieber, Ambrosino, Kiefer, Khan, Mader, Baraliakos and Bruges-Armas. This is an open-access article distributed under the terms of the Creative Commons Attribution License (CC BY). The use, distribution or reproduction in other forums is permitted, provided the original author(s) and the copyright owner(s) are credited and that the original publication in this journal is cited, in accordance with accepted academic practice. No use, distribution or reproduction is permitted which does not comply with these terms.
*Correspondence: Jácome Bruges-Armas, YnJ1Z2VzYXJtYXNAZ21haWwuY29t
†These authors have contributed equally to this work