- 1Department of Physiology, Yong Loo Lin School of Medicine, National University of Singapore, Singapore, Singapore
- 2Immunology Programme, Life Sciences Institute, National University of Singapore, Singapore, Singapore
Dendritic cell (DC)-based immunotherapies are being explored for over 20 years and found to be very safe. Most often, granulocyte-macrophage colony-stimulating factor (GM-CSF) and interleukin-4 (IL-4)-induced monocyte-derived DCs (moDCs) are being used, which have demonstrated some life-prolonging benefit to patients of multiple tumors. However, the limited clinical response and efficacy call for the development of more potent DCs. CD137L-DC may meet this demand. CD137L-DCs are a novel type of monocyte-derived inflammatory DCs that are induced by CD137 ligand (CD137L) agonists. CD137L is expressed on the surface of antigen-presenting cells, including monocytes, and signaling of CD137L into monocytes induces their differentiation to CD137L-DCs. CD137L-DCs preferentially induce type 1 T helper (Th1) cell polarization and strong type 1 CD8+ T cell (Tc1) responses against tumor-associated viral antigens. The in vitro T cell-stimulatory capacity of CD137L-DCs is superior to that of conventional moDCs. The transcriptomic profile of CD137L-DC is highly similar to that of in vivo DCs at sites of inflammation. The strict activation dependence of CD137 expression and its restricted expression on activated T cells, NK cells, and vascular endothelial cells at inflammatory sites make CD137 an ideally suited signal for the induction of monocyte-derived inflammatory DCs in vivo. These findings and their potency encouraged a phase I clinical trial of CD137L-DCs against Epstein–Barr virus-associated nasopharyngeal carcinoma. In this review, we introduce and summarize the history, the characteristics, and the transcriptional profile of CD137L-DC, and discuss the potential development and applications of CD137L-DC.
Introduction
The past 10 years have witnessed a renewed enthusiasm for cancer immunotherapy, prompted by the success of chimeric antigen receptor-T cells (CAR-T) and immune checkpoint inhibitors (ICI). Despite impressive therapeutic responses in some patients of certain tumors, CAR-T and ICI failed to show efficacy in most patients of the majority of cancers, especially solid cancers (1, 2). Moreover, the high frequency of severe adverse effects and the risk of breaking immune tolerance (3) by CAR-T cells or ICI emphasize that these treatments need to be carried out with caution.
A much safer immunotherapeutic approach is the use of dendritic cells (DCs). Since DCs were first described (4), substantial knowledge about the ontogeny, functions, and therapeutic applications of DCs has been accumulated, leading to the recognition that DCs exist in the form of several subsets, characterized by differences in ontogenies and functional properties (5–7). DC-based immunotherapies have been explored for two decades and are well-tolerated. Although DC-based cancer therapies has been proven to prolong the overall survival of patients, their efficacy and the clinical responses are far from satisfactory (8–10). Different strategies are being investigated to improve the efficacy, such as optimizing the tumor antigen source and loading, seeking optimal maturation methods, and the combination of DCs with ICI (11). However, first and foremost, a pivotal parameter is the source and type of DCs.
Due to the low frequency of natural DCs in blood, monocyte-derived DCs (moDCs), obtained by treating human peripheral monocytes with granulocyte-macrophage colony-stimulating factor (GM-CSF) and interleukin-4 (IL-4), are currently the most commonly used DC type in clinical trials. New methods to target DCs in vivo (12, 13), to enrich blood DCs in GMP facilities ex vivo (14, 15), or to differentiate myeloid DCs from stem cells (16, 17) have been explored. Yet the yield of DCs is limited. We have found a new type of human DC, CD137 ligand-induced DC (CD137L-DC), that is differentiated from peripheral monocytes by recombinant CD137-Fc protein or anti-CD137 ligand (CD137L) antibodies (18). Compared to the commonly used GM-CSF and IL-4-induced moDCs, CD137L-DCs have shown superior activities in inducing T cell responses (19, 20). In this review, we will give a systematic review on the development, the function, and the clinical application of this new type of DCs.
The Discovery of CD137L-DC
CD137 (TNFRSF9, 4-1BB) is an important co-stimulatory molecule expressed strictly upon activation, predominantly on T cells, NK cells, and vascular endothelial cells (21–23). Engagement of CD137 potently costimulates T cells and induces effective anti-tumor immune responses (24–27). Two agonistic anti-CD137 antibodies (urelumab and utomilumab) have shown great potency in preclinical experiments, and are currently being tested in clinical trials (28). In CAR, the intracellular domain of CD137 delivers signals for CAR-T cell persistence and delays their exhaustion (29, 30). CD137 ligand (CD137L, TNFSF9, 4-1BBL) is expressed on all types of antigen-presenting cells (APCs), and expression levels of CD137L increase upon APC activation (31). In the 1990s, several tumor necrosis factor super family (TNFSF) members were reported to trigger reverse signals into APCs (32–34). Reverse signaling is possible when a ligand is not a soluble molecule but is expressed as a transmembrane protein on the cell surface and can transmit a signal into the cell it is expressed on. Thus, functionally, it is identical to a receptor but it is referred to as a ligand (1) due to historical reasons and/or (2) because its partner molecule is also a receptor. Hence, both interacting molecules send and receive signals, i.e., act at the same time as a receptor and ligand, thereby establishing bidirectional signaling (35).
Similarly, engagement of CD137L was found to cause T cell apoptosis (36) and to activate monocytes as evidenced by the induction of adherence and cytokine secretion (37). Further, immobilized CD137-Fc protein induced survival and even proliferation of monocytes, which are mainly mediated by CD137L-induced macrophage colony-stimulating factor (M-CSF) (38, 39). Reverse signaling of CD137L was further shown in monocytic cell lines (40), B cells (41), moDCs (42, 43), and myeloid DCs (44). Notably, cross-linking of CD137L matures moDCs and myeloid DCs in vitro as seen by the increased expression of costimulatory molecules and IL-12p40 (43, 44). Altogether, these findings demonstrate that CD137L, just like other TNFSF members, not only can deliver but also can receive a signal (Figure 1).
Human monocytes that were exposed to CD137L agonists adhered to cell culture dishes very rapidly and the resultant cells were morphologically different from resting or LPS-activated monocytes and from macrophages (37, 45). The cells exhibited extensions that were comparable with DCs but their morphology was different from DCs that were generated from monocytes by GM-CSF and IL-4 treatment. In 2009, it was found that an agonistic anti-CD137L antibody could replace GM-CSF in the differentiation of moDCs. The anti-CD137L antibody + IL-4-induced DCs stimulated stronger T cell proliferation and preferentially polarized naïve CD4+ T cells toward type 1 T helper (Th1) cells compared to conventional moDCs (46). However, IL-4 is not required when employing CD137-Fc protein, which alone is sufficient to induce monocyte to DC differentiation. The resulting cells, later named CD137L-DC, had an enhanced expression of the DC maturation marker CD83 and enhanced endocytosis but reduced phagocytosis and oxidative burst (18). Despite lacking the conventional moDC markers CD1a and CD209, reverse CD137L signaling-induced cells could stimulate the proliferation of naïve T cells, which is the gold standard of defining a DC, justifying their naming as a type of DC (18). The T cell-activating capability of CD137-Fc-activated monocytes is gradually acquired because during the first 24 h of differentiation, the developing CD137L-DCs induce T cell apoptosis via elevated reactive oxygen species. This unexpected finding may have its physiological significance in a process called infection-induced T cell attrition, where old T cells are eliminated in order to create space for the new ones with specificity for the new challenge antigens (47). Altogether, these data made it clear that reverse CD137L signaling induced the differentiation of monocytes to a new type of DC, namely, CD137L-DC.
Species Difference in CD137L-DC
The abovementioned reverse CD137L signaling has been reported in both human and mouse. However, murine reverse CD137L signaling is very different from human reverse CD137L signaling. In murine endothelial cells, reverse CD137L signaling leads to chemokine secretion (48). In murine macrophages, reverse CD137L signaling sustains TNF secretion induced by toll-like receptor 4 (TLR4) activation (49, 50). However, reverse CD137L signaling was reported by the same group to suppress the activation of macrophages and DCs in vivo in mice. Kang et al. (51) found that CD137−/− mice and agonistic anti-CD137 antibody-treated mice had better anti-tumor immune responses because of an increased differentiation of myeloid cells to CD103+ DCs and type 1 macrophages. They proposed that reverse CD137L signaling suppresses the generation of pro-inflammatory DCs and macrophages and thereby the induction of effective anti-tumor responses. This hypothesis is supported by immobilized CD137-Fc, which initiates reverse CD137L signaling, inhibiting the differentiation of CD103+ DCs and M1 macrophages in vitro. However, the more effective anti-tumor response was not seen in CD137L−/− or neutralizing anti-CD137L antibody-treated mice (51). Another concern is that CD137 is also expressed on and functional in macrophages (52, 53) and DCs (41, 54). It can therefore not be ruled out that CD137 deficiency may have disturbed the equilibrium and function of endogenous DCs and macrophages.
CD137L-DCs have only been differentiated from human but not from murine monocytes. Unlike other TNFSF members that generally share 60–80% homology between mouse and human, the amino acid sequence of human CD137L protein is only 36% identical to that of murine CD137L (55). While murine monocytes also proliferate and change their morphology in response to immobilized murine CD137-Fc protein, the resulting cells are not inflammatory DCs as evidenced by the absence of DC markers and their inability to induce allogenic T cell proliferation (56). Therefore, CD137L-DC specifically refers to in vitro generated human CD137L-DC in this review. At present, the functions and activities of CD137L-DC have just started to be evaluated in vivo.
The Superior Function of CD137L-DC
To achieve more effective anti-tumor immune responses, DCs must polarize naïve T cells preferentially to Th1 and type 1 CD8+ T cell (Tc1) responses. CD137L-DCs meet this requirement. CD137L-DCs enhance the subset of interferon (IFN)γ+ T cells, especially among the CD8+ cells, and no additional maturation of CD137L-DCs is required for this activity (18). CD8+ T cells activated by CD137L-DCs express more perforin and are more cytotoxic than T cells activated by conventional moDCs (18, 19). Most importantly, human TCR-redirected T cells are stronger activated and exert superior antigen-specific killing when activated by autologous peptide antigen-pulsed CD137L-DCs than by autologous moDCs or mature moDCs (19, 20). This superior function of CD137L-DCs has been observed with cytomegalovirus-, hepatitis B virus (HBV)-, and Epstein–Barr virus (EBV)-derived antigens. HBV and EBV are associated with various types of cancers (57, 58), implying that CD137L-DCs are a good candidate for virus-associated cancer immunotherapy. Classical DCs (cDCs) are also more potent than moDCs at activating Th1 and Tc1 responses. The direct comparison between CD137L-DCs and cDCs has not yet been done. What we know is that CD137L-DCs express low level of CD141 and no CD1c, markers for cDC1 and cDC2, respectively (59).
To achieve a successful DC-based immunotherapy, the maturation of DCs is of great importance. Although CD137L-DCs express the DC maturation marker CD83 and secrete pro-inflammatory cytokines, the cytokine levels are generally low. In order to increase the potency of CD137L-DCs, different combinations of cytokines and pattern recognition receptor agonists were compared regarding their ability to mature CD137L-DCs (19). Toll-like receptor 7/8 agonist R848 and IFNγ were found to generate the most potent mature CD137L-DCs. These mature CD137L-DCs significantly elevated the expression of CD40, CD70, CD80, CD86, CD137L, and HLA-DR (19, 60). Accordingly, mature CD137L-DCs more strongly enhance the proliferation and the percentage of IFNγ+ T cells (19).
The ability of DCs to migrate to the lymph nodes is another pivotal factor for a successful DC-based therapy (10, 11). To boost the expression of CCR7, and thus the migratory capacity of mature CD137L-DCs, prostaglandin E2 (PGE2) was included in the maturation cocktail (61). Even though PGE2 is known to decrease the secretion of IL-12 by DCs (62), the T cell-activating capability of mature CD137L-DCs is not significantly impaired by PGE2 (20). CD8+ T cells activated by PGE2, R848, and IFNγ-matured CD137L-DCs are more cytotoxic, less exhausted, and metabolically more active than CD8+ T cells activated by mature moDCs (20). A schematic summary of CD137L-DC functions is shown in Figure 2.
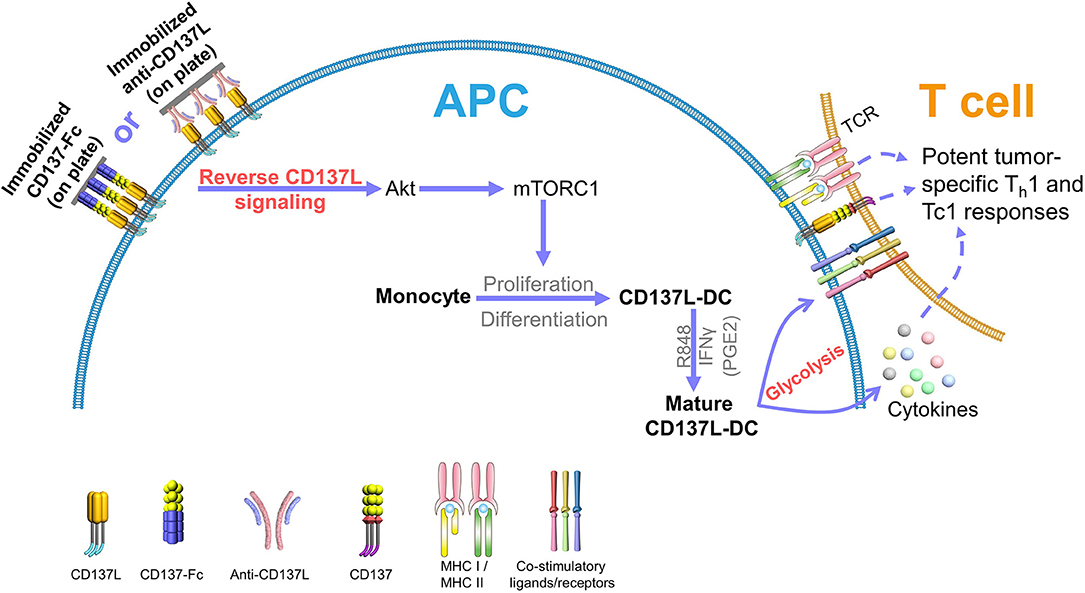
Figure 2. CD137L-DCs induce potent Th1 and Tc1 responses against tumors. Reverse CD137L signaling activates monocytes and induces the proliferation and the differentiation of monocytes to CD137L-DCs. R848 and IFNγ (and PGE2) cause maturation of CD137L-DCs. Mature CD137L-DCs are characterized by a high Akt-mediated glycolysis rate, which is important for the inflammatory properties of CD137L-DCs. Mature CD137L-DCs preferentially induce Th1 and Tc1 polarization in T cells, leading to strong immune responses against virus-associated tumor cells, making them a promising candidate for virus-associated cancer immunotherapy.
Based on these findings, we started a phase I clinical trial (NCT03282617) with CD137L-DCs to treat nasopharyngeal carcinoma (NPC). The CD137L-DCs are generated from patients' monocytes; matured with R848, IFNγ, and PGE2; and pulsed with peptide pools of EBV antigens. Currently, data are available on 10 patients and no immune-related adverse events have been observed, demonstrating an excellent safety profile of CD137L-DCs.
Characteristics and Physiological Relevance of CD137L-DC
The most commonly employed step between in vitro studies and clinical trials is the use of animal models, generally murine models. Demonstrating in vivo proof of principle of CD137L-DCs in murine tumor models was hindered by the above-described species difference in the molecular structure and in reverse CD137L signaling between mouse and human.
In order to investigate the in vivo relevance of CD137L-DC, Harfuddin et al. acquired the transcriptome of CD137L-DCs and compared it to the transcriptomes of different types of in vitro-generated human myeloid cells and in vivo inflammatory macrophages and DCs. By using hierarchical clustering and gene enrichment analysis, it was found that the gene signature of CD137L-DC is distinct from that of other myeloid cells, but is most similar to that of immature moDC and macrophages. Notably, the transcriptome of CD137L-DCs is enriched for the gene signatures of human inflammatory DCs and BDCA1+ DCs as they occur in vivo at sites of inflammation (59), indicating CD137L-DCs may exist under inflammatory conditions in vivo in man.
Further support for this hypothesis comes from the presence of CD137 in blood vessels at sites of inflammation and the involvement of the CD137–CD137L system in monocyte recruitment into inflamed tissues. CD137 expression is induced on vascular endothelial cells by TNF, and CD137 on the vascular endothelial cells strengthens intercellular adhesion molecule 1 and lymphocyte function-associated antigen 1-mediated adhesion of monocytes. In in vitro systems, CD137 attracts monocytes to infiltrate into spheroids and matrigels (63, 64). Therefore, circulating monocytes that are recruited to sites of inflammation via CD137 on vascular endothelial cells would receive a CD137L signal during the recruitment that may initiate their CD137L-DC differentiation. Further CD137L signaling would be induced by CD137-expressing leukocytes at the site of inflammation.
These data support the concept that reverse CD137L signaling in circulating monocytes may contribute to the physiological generation of inflammatory DCs in man. After all, CD137 expression is strictly activation-dependent and only found at sites of inflammation. Expression of CD137L is enhanced upon APC activation. This restricted expression of CD137 would confine the generation of CD137L-DCs to sites of inflammation.
The transcriptome profile of human CD137L-DC has generated additional interesting information. For example, CD137L-DCs strongly adhere to the plastic cell culture dishes. This feature finds its explanation in the gene ontology enrichment analysis. Compared to both immature and mature moDCs, CD137L-DCs express 22 genes involved in cell adhesion at more than 2-fold higher levels (59).
Another property of CD137L-DCs that was identified by the transcriptome analysis is their metabolism. Metabolic reprogramming is being increasingly appreciated as an important driving force of immune cell activation and effective immune responses (65). Our recent data demonstrate that Akt-driven glycolysis contributes to the superior function of CD137L-DCs. Compared to GM-CSF and IL-4-generated moDCs, CD137L-DCs show a significantly higher basal glycolysis rate and glycolytic capacity due to the elevated activation of the phosphoinositide 3-kinase (PI3K)–Akt–mechanistic target of rapamycin complex 1 (mTORC1) pathway. This higher rate of glycolysis is important not only for the maturation but also for the sustained activation of CD137L-DCs. The inhibition of the PI3K or Akt recapitulates the inhibition of CD137L-DC by suppressed glycolysis (Figure 2). In contrast to the flux of glycolysis intermediates into lipid synthesis in murine bone marrow-derived DCs (66), the higher glycolysis of mature CD137L-DCs leads to an increase in succinate and serine, which are known metabolites that regulate inflammation (60).
Reverse CD137L Signaling Mechanism
An interesting aspect is the signal transduction mechanism employed by CD137L. In human primary monocytes and THP-1 cells, protein tyrosine kinases, mitogen-activated protein kinase (MAPK) kinase, p38 MAPK, extracellular regulated protein kinases 1/2, PI3K-Akt-mTORC1, and protein kinase A are activated by recombinant CD137-Fc protein (60, 67). mTORC1 is critical for the differentiation of CD137L-DCs from monocytes (60). Interestingly, CD137L associates with several cell surface receptors with well-characterized signal transduction cascades. In human cells, CD137L associates with TNFRI, which is required for CD137L-induced cell adhesion, CD14 expression, and IL-8 production (68). In murine macrophages, the association of murine CD137L with TLR4 is required for TLR4-induced activation of the transcription factors cAMP response element binding protein (CREB) and CCAAT-enhancer-binding proteins (C/EBP), and for sustained TNF secretion by murine peritoneal macrophages (49). The murine TLR4–CD137L complex associates with toll–interleukin-1 receptor domain-containing adaptor protein (TIRAP), interleukin-1 receptor-associated kinase-like 2 (IRAK2), TNF receptor–associated factor 6 (TRAF6), transforming growth factor–b–activated kinase 1 (TAK1), and TAK-binding protein 1 (TAB1) to form a larger signaling complex (50). Further, CD137L associates with transmembrane protein 126A (TMEM126A), and knockdown of TMEM126A in murine macrophages prevents the induction of tyrosine phosphorylation and the secretion of M-CSF, IL-1β, and Tenascin C (69). It is currently not known whether these associations are species-specific or apply similarly to human and mouse. Nevertheless, the common theme from all these studies is that CD137L seems not to signal by itself, but to be part of a larger signaling complex.
On reverse CD137L signaling, there is an entirely different mechanism to consider. Upon cell–cell interaction of CD137- and CD137L-expressing cells, human CD137 gets transferred to the CD137L-expressing cells by trogocytosis and then forms a complex with CD137L, which gets internalized and degraded via the proteasome (70–72). Whether this process is involved in mediating reverse CD137L signaling has not yet been addressed.
Future Perspectives
CD137L-DCs are an attractive candidate for cancer immunotherapy as CD137L-DCs are more potent at inducing and strengthening Th1 and Tc1 responses than the conventional GM-CSF and IL-4-induced moDCs. CD137L-DCs are showing an excellent safety profile in NPC patients. The evaluation of their immunological efficacy and clinical usefulness is pending. Apart from NPC, other virus-associated malignancies could also be targeted by CD137L-DCs (19, 20) such as the EBV-associated Hodgkin lymphoma and HBV-associated hepatocellular carcinoma (58). How to further enhance the efficacy of CD137L-DCs will be a topic of future research. Despite the addition of PGE2 to increase the mobility of DCs, Davignon et al. have shown that most DCs die at the site of injection (73). Improving the homing of functional DCs to the lymph nodes remains a challenge. Preconditioning the injection site may be one way to improve the efficacy of CD137L-DCs (10). Additionally, the combination of CD137L-DCs with ICI could be an approach to overcome the suppressive tumor microenvironment and boost the activation of T cells (74, 75).
An issue that a CD137L-DC therapy shares with most DC therapies and personalized therapies in general is the high cost of generating the cells. Monocytes need to be harvested and converted to CD137L-DC for each patient individually under good manufacturing practice conditions. It would be a huge advantage if an approach could be developed that enables the in vivo generation of CD137L-DC in patients, just like how inflammatory monocyte-derived DCs are generated at the site of inflammation, when and where they are needed. For instance, controlled and site-specific CD137L agonist delivery to monocytes may fulfill this requirement and mimic the in vivo generation of inflammatory monocyte-derived DCs. Practically, liposome- or exosome-mediated CD137 gene/protein delivery could be suited for this task. Nevertheless, a comprehensive understanding of the mechanism of CD137L-DC differentiation is necessary for the generation of CD137L-DCs in vivo. CD137L likely forms a complex with other signaling mediators, and this complex formation may be necessary to induce the differentiation of CD137L-DC. A more detailed investigation of the molecules and signaling pathways leading to CD137L-DC differentiation will surely augment our understanding of the possible roles of CD137L-DCs under inflammatory conditions such as infection, autoimmune disease, and antibody treatment-induced inflammation.
CD137L-DCs have been studied for 10 years. Transcriptomic data indicate them to be close in vitro counterparts to in vivo monocyte-derived inflammatory DCs. Due to their potent immune-stimulatory activity, CD137L-DCs are being developed for cancer immunotherapy.
Author Contributions
QZ, YZ, and HS organized, wrote, and edited the manuscript. Figures were drawn by QZ and edited by YZ and HS.
Funding
This study was funded by the National Medical Research Council, Singapore (NMRC/OFIRG/0022/2016).
Conflict of Interest
The authors declare that the research was conducted in the absence of any commercial or financial relationships that could be construed as a potential conflict of interest.
Abbreviations
CD137L-DC, CD137 ligand-induced DC; DC, dendritic cell; ICI, immune checkpoint inhibitors; moDC, monocyte-derived DC.
References
1. June CH, O'connor RS, Kawalekar OU, Ghassemi S, Milone MC. CAR T cell immunotherapy for human cancer. Science. (2018) 359:1361–5. doi: 10.1126/science.aar6711
2. Park YJ, Kuen DS, Chung Y. Future prospects of immune checkpoint blockade in cancer: from response prediction to overcoming resistance. Exp Mol Med. (2018) 50:109. doi: 10.1038/s12276-018-0130-1
3. Kumar P, Saini S, Prabhakar BS. Cancer immunotherapy with check point inhibitor can cause autoimmune adverse events due to loss of Treg homeostasis. Semin Cancer Biol. (2019). doi: 10.1016/j.semcancer.2019.01.006
4. Steinman RM, Cohn ZA. Identification of a novel cell type in peripheral lymphoid organs of mice. I. Morphology, quantitation, tissue distribution. J Exp Med. (1973) 137:1142–62. doi: 10.1084/jem.137.5.1142
5. Satpathy AT, Wu X, Albring JC, Murphy KM. Re(de)fining the dendritic cell lineage. Nat Immunol. (2012) 13:1145–54. doi: 10.1038/ni.2467
6. Haniffa M, Collin M, Ginhoux F. Ontogeny and functional specialization of dendritic cells in human and mouse. Adv Immunol. (2013) 120:1–49. doi: 10.1016/B978-0-12-417028-5.00001-6
7. Merad M, Sathe P, Helft J, Miller J, Mortha A. The dendritic cell lineage: ontogeny and function of dendritic cells and their subsets in the steady state and the inflamed setting. Annu Rev Immunol. (2013) 31:563–604. doi: 10.1146/annurev-immunol-020711-074950
8. Anguille S, Smits EL, Lion E, Van Tendeloo VF, Berneman ZN. Clinical use of dendritic cells for cancer therapy. Lancet Oncol. (2014) 15:e257–67. doi: 10.1016/S1470-2045(13)70585-0
9. Anguille S, Van De Velde AL, Smits EL, Van Tendeloo VF, Juliusson G, Cools N, et al. Dendritic cell vaccination as postremission treatment to prevent or delay relapse in acute myeloid leukemia. Blood. (2017) 130:1713–21. doi: 10.1182/blood-2017-04-780155
10. Sabado RL, Balan S, Bhardwaj N. Dendritic cell-based immunotherapy. Cell Res. (2017) 27:74–95. doi: 10.1038/cr.2016.157
11. Anguille S, Smits EL, Bryant C, Van Acker HH, Goossens H, Lion E, et al. Dendritic cells as pharmacological tools for cancer immunotherapy. Pharmacol Rev. (2015) 67:731–53. doi: 10.1124/pr.114.009456
12. Dhodapkar MV, Sznol M, Zhao B, Wang D, Carvajal RD, Keohan ML, et al. Induction of antigen-specific immunity with a vaccine targeting NY-ESO-1 to the dendritic cell receptor DEC-205. Sci Transl Med. (2014) 6:232ra251. doi: 10.1126/scitranslmed.3008068
13. Li J, Ahmet F, Sullivan LC, Brooks AG, Kent SJ, De Rose R, et al. Antibodies targeting Clec9A promote strong humoral immunity without adjuvant in mice and non-human primates. Eur J Immunol. (2015) 45:854–64. doi: 10.1002/eji.201445127
14. Prue RL, Vari F, Radford KJ, Tong H, Hardy MY, D'rozario R, et al. A phase I clinical trial of CD1c (BDCA-1)+ dendritic cells pulsed with HLA-A*0201 peptides for immunotherapy of metastatic hormone refractory prostate cancer. J Immunother. (2015) 38:71–6. doi: 10.1097/CJI.0000000000000063
15. Schreibelt G, Bol KF, Westdorp H, Wimmers F, Aarntzen EH, Duiveman-De Boer T, et al. Effective clinical responses in metastatic melanoma patients after vaccination with primary myeloid dendritic cells. Clin Cancer Res. (2016) 22:2155–66. doi: 10.1158/1078-0432.CCR-15-2205
16. Balan S, Arnold-Schrauf C, Abbas A, Couespel N, Savoret J, Imperatore F, et al. Large-scale human dendritic cell differentiation revealing notch-dependent lineage bifurcation and heterogeneity. Cell Rep. (2018) 24:1902–1915.e6. doi: 10.1016/j.celrep.2018.07.033
17. Kirkling ME, Cytlak U, Lau CM, Lewis KL, Resteu A, Khodadadi-Jamayran A, et al. Notch signaling facilitates in vitro generation of cross-presenting classical dendritic cells. Cell Rep. (2018) 23:3658–72.e6. doi: 10.1016/j.celrep.2018.05.068
18. Kwajah MMS, Schwarz H. CD137 ligand signaling induces human monocyte to dendritic cell differentiation. Eur J Immunol. (2010) 40:1938–49. doi: 10.1002/eji.200940105
19. Harfuddin Z, Kwajah S, Chong Nyi Sim A, Macary PA, Schwarz H. CD137L-stimulated dendritic cells are more potent than conventional dendritic cells at eliciting cytotoxic T-cell responses. Oncoimmunology. (2013) 2:e26859. doi: 10.4161/onci.26859
20. Dharmadhikari B, Nickles E, Harfuddin Z, Ishak NDB, Zeng Q, Bertoletti A, et al. CD137L dendritic cells induce potent response against cancer-associated viruses and polarize human CD8(+) T cells to Tc1 phenotype. Cancer Immunol Immunother. (2018) 67:893–905. doi: 10.1007/s00262-018-2144-x
21. Schwarz H, Valbracht J, Tuckwell J, Von KJ, Lotz M. ILA, the human 4-1BB homologue, is inducible in lymphoid and other cell lineages. Blood. (1995) 85:1043–52.
22. Melero I, Johnston JV, Shufford WW, Mittler RS, Chen L. NK1.1 cells express 4-1BB (CDw137) costimulatory molecule and are required for tumor immunity elicited by anti-4-1BB monoclonal antibodies. Cell Immunol. (1998) 190:167–72. doi: 10.1006/cimm.1998.1396
23. Broll K, Richter G, Pauly S, Hofstaedter F, Schwarz H. CD137 expression in tumor vessel walls. High correlation with malignant tumors. Am J Clin Pathol. (2001) 115:543–9. doi: 10.1309/E343-KMYX-W3Y2-10KY
24. Lee SW, Croft M. 4-1BB as a therapeutic target for human disease. Adv Exp Med Biol. (2009) 647:120–9. doi: 10.1007/978-0-387-89520-8_8
25. Thum E, Shao Z, Schwarz H. CD137, implications in immunity and potential for therapy. Front Biosci. (2009) 14:4173–88. doi: 10.2741/3521
26. Wang S, Chen L. Immunobiology of cancer therapies targeting CD137 and B7-H1/PD-1 cosignal pathways. Curr Top Microbiol Immunol. (2011) 344:245–67. doi: 10.1007/82_2010_81
27. Vinay DS, Kwon BS. 4-1BB (CD137), an inducible costimulatory receptor, as a specific target for cancer therapy. BMB Rep. (2014) 47:122–9. doi: 10.5483/BMBRep.2014.47.3.283
28. Chester C, Sanmamed MF, Wang J, Melero I. Immunotherapy targeting 4-1BB: mechanistic rationale, clinical results, and future strategies. Blood. (2018) 131:49–57. doi: 10.1182/blood-2017-06-741041
29. Milone MC, Fish JD, Carpenito C, Carroll RG, Binder GK, Teachey D, et al. Chimeric receptors containing CD137 signal transduction domains mediate enhanced survival of T cells and increased antileukemic efficacy in vivo. Mol Ther. (2009) 17:1453–64. doi: 10.1038/mt.2009.83
30. Long AH, Haso WM, Shern JF, Wanhainen KM, Murgai M, Ingaramo M, et al. 4-1BB costimulation ameliorates T cell exhaustion induced by tonic signaling of chimeric antigen receptors. Nat Med. (2015) 21:581–90. doi: 10.1038/nm.3838
31. Croft M. The role of TNF superfamily members in T-cell function and diseases. Nat Rev. Immunol. (2009) 9:271–85. doi: 10.1038/nri2526
32. Grewal IS, Xu J, Flavell RA. Impairment of antigen-specific T-cell priming in mice lacking CD40 ligand. Nature. (1995) 378:617–20. doi: 10.1038/378617a0
33. Stuber E, Neurath M, Calderhead D, Fell HP, Strober W. Cross-linking of OX40 ligand, a member of the TNF/NGF cytokine family, induces proliferation and differentiation in murine splenic B cells. Immunity. (1995) 2:507–21. doi: 10.1016/1074-7613(95)90031-4
34. Wiley SR, Goodwin RG, Smith CA. Reverse signaling via CD30 ligand. J Immunol. (1996) 157:3635–9.
35. Shao Z, Schwarz H. CD137 ligand, a member of the tumor necrosis factor family, regulates immune responses via reverse signal transduction. J Leukoc Biol. (2011) 89:21–9. doi: 10.1189/jlb.0510315
36. Schwarz H, Blanco FJ, Von Kempis J, Valbracht J, Lotz M. ILA, a member of the human nerve growth factor/tumor necrosis factor receptor family, regulates T-lymphocyte proliferation and survival. Blood. (1996) 87:2839–45.
37. Langstein J, Michel J, Fritsche J, Kreutz M, Andreesen R, Schwarz H. CD137 (ILA/4-1BB), a member of the TNF receptor family, induces monocyte activation via bidirectional signaling. J Immunol. (1998) 160:2488–94.
38. Langstein J, Michel J, Schwarz H. CD137 induces proliferation and endomitosis in monocytes. Blood. (1999) 94:3161–8.
39. Langstein J, Schwarz H. Identification of CD137 as a potent monocyte survival factor. J Leukoc Biol. (1999) 65:829–33. doi: 10.1002/jlb.65.6.829
40. Choi J-W, Lee H-W, Roh G-S, Kim H-H, Kwack K. CD137 induces adhesion and cytokine production in human monocytic THP-1 cells. Exp Mol Med. (2005) 37:78–85. doi: 10.1038/emm.2005.11
41. Pauly S, Broll K, Wittmann M, Giegerich G, Schwarz H. CD137 is expressed by follicular dendritic cells and costimulates B lymphocyte activation in germinal centers. J Leukoc Biol. (2002) 72:35–42. doi: 10.1189/jlb.72.1.35
42. Laderach D, Wesa A, Galy A. 4-1BB-ligand is regulated on human dendritic cells and induces the production of IL-12. Cell Immunol. (2003) 226:37–44. doi: 10.1016/j.cellimm.2003.11.003
43. Lippert U, Zachmann K, Ferrari DM, Schwarz H, Brunner E, Mahbub-Ul Latif AH, et al. CD137 ligand reverse signaling has multiple functions in human dendritic cells during an adaptive immune response. Eur J Immunol. (2008) 38:1024–32. doi: 10.1002/eji.200737800
44. Kim Y-J, Li G, Broxmeyer HE. 4-1BB ligand stimulation enhances myeloid dendritic cell maturation from human umbilical cord blood CD34+ progenitor cells. J Hematother Stem Cell Res. (2002) 11:895–903. doi: 10.1089/152581602321080556
45. Langstein J, Becke FM, Sollner L, Krause G, Brockhoff G, Kreutz M, et al. Comparative analysis of CD137 and LPS effects on monocyte activation, survival, and proliferation. Biochem Biophys Res Commun. (2000) 273:117–22. doi: 10.1006/bbrc.2000.2889
46. Ju S, Ju S, Ge Y, Qiu H, Lu B, Qiu Y, et al. A novel approach to induce human DCs from monocytes by triggering 4-1BBL reverse signaling. Int Immunol. (2009) 21:1135–44. doi: 10.1093/intimm/dxp077
47. Kwajah MMS, Mustafa N, Holme AL, Pervaiz S, Schwarz H. Biphasic activity of CD137 ligand-stimulated monocytes on T cell apoptosis and proliferation. J Leukoc Biol. (2011) 89:707–20. doi: 10.1189/jlb.1010569
48. Kim HJ, Lee JS, Kim JD, Cha HJ, Kim A, Lee SK, et al. Reverse signaling through the costimulatory ligand CD137L in epithelial cells is essential for natural killer cell-mediated acute tissue inflammation. Proc Natl Acad Sci USA. (2012) 109:E13–22. doi: 10.1073/pnas.1112256109
49. Kang YJ, Kim SO, Shimada S, Otsuka M, Seit-Nebi A, Kwon BS, et al. Cell surface 4-1BBL mediates sequential signaling pathways ‘downstream' of TLR and is required for sustained TNF production in macrophages. Nat Immunol. (2007) 8:601–9. doi: 10.1038/ni1471
50. Ma J, Bang B-R, Lu J, Eun S-Y, Otsuka M, Croft M, et al. The TNF family member 4-1BBL sustains inflammation by interacting with TLR signaling components during late-phase activation. Sci Signal. (2013) 6:ra87–7. doi: 10.1126/scisignal.2004431
51. Kang SW, Lee SC, Park SH, Kim J, Kim HH, Lee H-W, et al. Anti-CD137 suppresses tumor growth by blocking reverse signaling by CD137 ligand. Cancer Res. (2017) 77:5989–6000. doi: 10.1158/0008-5472.CAN-17-0610
52. Bartkowiak T, Jaiswal AR, Ager CR, Chin R, Chen CH, Budhani P, et al. Activation of 4-1BB on liver myeloid cells triggers hepatitis via an interleukin-27-dependent pathway. Clin Cancer Res. (2018) 24:1138–51. doi: 10.1158/1078-0432.CCR-17-1847
53. Lu Y, Li C, Du S, Chen X, Zeng X, Liu F, et al. 4-1BB signaling promotes alveolar macrophages-mediated pro-fibrotic responses and crystalline silica-induced pulmonary fibrosis in mice. Front Immunol. (2018) 9:1848–8. doi: 10.3389/fimmu.2018.01848
54. Futagawa T, Akiba H, Kodama T, Takeda K, Hosoda Y, Yagita H, et al. Expression and function of 4-1BB and 4-1BB ligand on murine dendritic cells. Int Immunol. (2002) 14:275–86. doi: 10.1093/intimm/14.3.275
55. Alderson MR, Smith CA, Tough TW, Davis-Smith T, Armitage RJ, Falk B, et al. Molecular and biological characterization of human 4-1BB and its ligand. Eur J Immunol. (1994) 24:2219–27. doi: 10.1002/eji.1830240943
56. Tang Q, Jiang D, Shao Z, Martinez Gomez JM, Schwarz H. Species difference of CD137 ligand signaling in human and murine monocytes. PLoS ONE. (2011) 6:e16129. doi: 10.1371/journal.pone.0016129
57. Michaelis M, Doerr HW, Cinatl J. The story of human cytomegalovirus and cancer: increasing evidence and open questions. Neoplasia. (2009) 11:1–9. doi: 10.1593/neo.81178
58. Tashiro H, Brenner MK. Immunotherapy against cancer-related viruses. Cell Res. (2017) 27:59–73. doi: 10.1038/cr.2016.153
59. Harfuddin Z, Dharmadhikari B, Wong SC, Duan K, Poidinger M, Kwajah S, et al. Transcriptional and functional characterization of CD137L-dendritic cells identifies a novel dendritic cell phenotype. Sci Rep. (2016) 6:29712. doi: 10.1038/srep29712
60. Zeng Q, Mallilankaraman K, Schwarz H. Increased Akt-driven glycolysis is the basis for the higher potency of CD137L-DCs. Front Immunol. (2019) 10:868. doi: 10.3389/fimmu.2019.00868
61. Scandella E, Men Y, Gillessen S, Forster R, Groettrup M. Prostaglandin E2 is a key factor for CCR7 surface expression and migration of monocyte-derived dendritic cells. Blood. (2002) 100:1354–61. doi: 10.1182/blood-2001-11-0017
62. Van Der Pouw Kraan TC, Boeije LC, Smeenk RJ, Wijdenes J, Aarden LA. Prostaglandin-E2 is a potent inhibitor of human interleukin 12 production. J Exp Med. (1995) 181:775–9. doi: 10.1084/jem.181.2.775
63. Drenkard D, Becke FM, Langstein J, Spruss T, Kunz-Schughart LA, Tan TE, et al. CD137 is expressed on blood vessel walls at sites of inflammation and enhances monocyte migratory activity. FASEB J. (2007) 21:456–63. doi: 10.1096/fj.05-4739com
64. Quek BZ, Lim YC, Lin JH, Tan TE, Chan J, Biswas A, et al. CD137 enhances monocyte-ICAM-1 interactions in an E-selectin-dependent manner under flow conditions. Mol Immunol. (2010) 47:1839–47. doi: 10.1016/j.molimm.2009.11.010
65. Pearce EL, Pearce EJ. Metabolic pathways in immune cell activation and quiescence. Immunity. (2013) 38:633–43. doi: 10.1016/j.immuni.2013.04.005
66. Everts B, Amiel E, Huang SC, Smith AM, Chang CH, Lam WY, et al. TLR-driven early glycolytic reprogramming via the kinases TBK1-IKKvarepsilon supports the anabolic demands of dendritic cell activation. Nat Immunol. (2014) 15:323–32. doi: 10.1038/ni.2833
67. Sollner L, Kwajah MMSDO, Wu JT, Schwarz H. Signal transduction mechanisms of CD137 ligand in human monocytes. Cell Signal. (2007) 19:1899–908. doi: 10.1016/j.cellsig.2007.04.014
68. Moh MC, Lorenzini PA, Gullo C, Schwarz H. Tumor necrosis factor receptor 1 associates with CD137 ligand and mediates its reverse signaling. FASEB J. (2013) 27:2957–66. doi: 10.1096/fj.12-225250
69. Bae JS, Choi JK, Moon JH, Kim EC, Croft M, Lee HW. Novel transmembrane protein 126A (TMEM126A) couples with CD137L reverse signals in myeloid cells. Cell Signal. (2012) 24:2227–36. doi: 10.1016/j.cellsig.2012.07.021
70. Ho WT, Pang WL, Chong SM, Castella A, Al-Salam S, Tan TE, et al. Expression of CD137 on hodgkin and reed-sternberg cells inhibits T-cell activation by eliminating CD137 ligand expression. Cancer Res. (2013) 73:652–61. doi: 10.1158/0008-5472.CAN-12-3849
71. Shao Z, Harfuddin Z, Pang WL, Nickles E, Koh LK, Schwarz H. Trogocytic CD137 transfer causes an internalization of CD137 ligand on murine APCs leading to reduced T cell costimulation. J Leukoc Biol. (2015) 97:909–19. doi: 10.1189/jlb.3A0213-079RRR
72. Wu M, Wong HY, Lin JL, Moliner A, Schwarz H. Induction of CD137 expression by viral genes reduces T cell costimulation. J Cell Physiol. (2019) 234:21076–88. doi: 10.1002/jcp.28710
73. Davignon JL, Hayder M, Baron M, Boyer JF, Constantin A, Apparailly F, et al. Targeting monocytes/macrophages in the treatment of rheumatoid arthritis. Rheumatology. (2013) 52:590–8. doi: 10.1093/rheumatology/kes304
74. Lin H, Wei S, Hurt EM, Green MD, Zhao L, Vatan L, et al. Host expression of PD-L1 determines efficacy of PD-L1 pathway blockade-mediated tumor regression. J Clin Invest. (2018) 128:1708. doi: 10.1172/JCI120803
Keywords: CD137L-DC, reverse CD137L siganling, moDC, Th1, Tc1, tumor immunotherapy
Citation: Zeng Q, Zhou Y and Schwarz H (2019) CD137L-DCs, Potent Immune-Stimulators—History, Characteristics, and Perspectives. Front. Immunol. 10:2216. doi: 10.3389/fimmu.2019.02216
Received: 07 May 2019; Accepted: 02 September 2019;
Published: 02 October 2019.
Edited by:
Riccardo Dolcetti, University of Queensland, AustraliaReviewed by:
Zwi Berneman, University of Antwerp, BelgiumLai Guan Ng, Singapore Immunology Network (A*STAR), Singapore
Copyright © 2019 Zeng, Zhou and Schwarz. This is an open-access article distributed under the terms of the Creative Commons Attribution License (CC BY). The use, distribution or reproduction in other forums is permitted, provided the original author(s) and the copyright owner(s) are credited and that the original publication in this journal is cited, in accordance with accepted academic practice. No use, distribution or reproduction is permitted which does not comply with these terms.
*Correspondence: Herbert Schwarz, phssh@nus.edu.sg