- 1Université Paris Cité, Paris, France
- 2APHP, Hématologie Greffe, Hôpital Saint Louis, Paris, France
- 3INSERM UMR 976, Hôpital Saint Louis, Paris, France
In the past 65 years, over 25 000 referenced articles have been published on graft-versus-host disease (GVHD). Although this included clinically orientated papers or publications on chronic GVHD, the conservative estimate of scientific publications still contains several thousands of documents on the pathophysiology of acute GVHD. Thus, summarizing what we believe are prominent publications that can be considered milestones in our knowledge of this disease is a challenging and inherently biased task. Here we review from a historical perspective what can be regarded as publications that have made the field move forward. We also included several references of reviews on aspects we could not cover in detail.
Introduction
It has been more than 65 years since the seminal description that mice exposed to lethal irradiation and received allogeneic splenocytes developed diarrhea and skin lesions and died (1, 2). Initially designated as a “secondary disease, “ this syndrome was indeed graft-versus-host disease (GVHD). Ten years later, Matheí and colleagues reported the first patient who underwent allogeneic bone marrow transplantation (BMT) who developed lethal GVHD (3). The outcomes of patients transplanted at this early age were poor [reviewed in (4)]. This mainly resulted from the poor knowledge of histocompatibility, the need for immunosuppressive drugs post-transplant, and advanced stage of the hematological malignancies.
Thousands of patients have since been transplanted, and our understanding of the disease has improved. At the time of writing this perspective, we made a PubMed search on “GVHD included in the title or the abstract.” This resulted in over 25,000 referenced publications (Figure 1). Thus, when we naively accepted to write a review on a historical perspective of GVHD, we were stuck with the tough task of choosing among these 25 000 publications what we believed could be the milestones in the understanding GVHD pathophysiology. The obvious consequences of this unattainable task are the following: 1) we must drastically limit the number of papers to be cited, and: 2) this perspective is inherently associated with our own bias on what we believe are milestones! Thus, we deeply apologize for non-citing major articles from friends and colleagues in this manuscript and refer the general audience of this Journal to excellent reviews that cover this field more extensively [reviewed in (5–10)]. Finally, to keep the field of this perspective in a narrower ambition, we decided to restrict the subject to acute GVHD. Again, readers interested in chronic GVHD will find excellent reviews elsewhere [reviewed in (6, 11)].
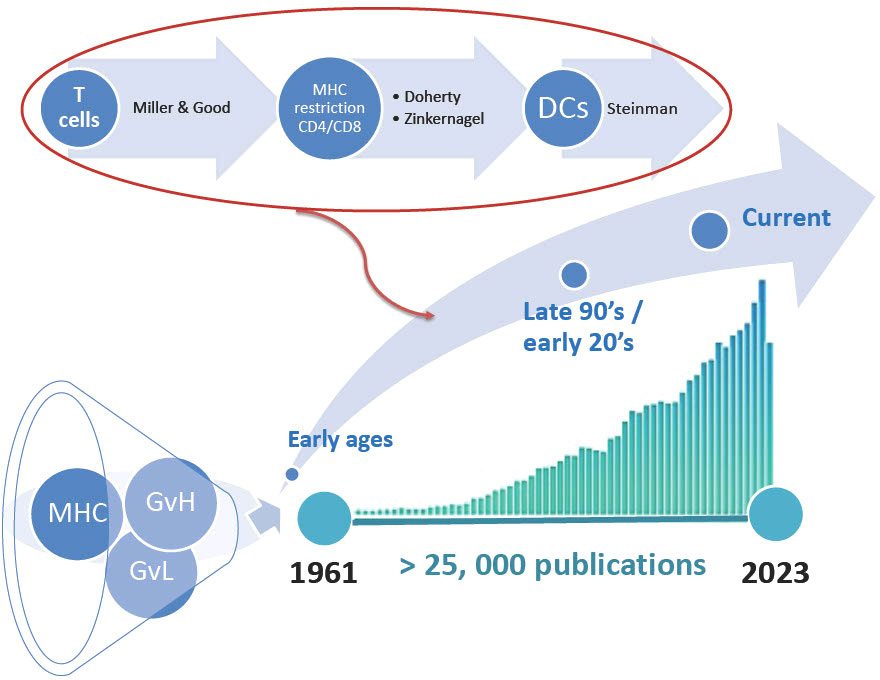
Figure 1 Referenced publications on graft-versus host disease (GVH). The diagram illustrates the number of publications referenced in PubMed as of October 2022. MHC, major histocompatibility complex; GvL, graft-versus-leukemia effect; DCs, Dendritic cells.
Classical steps of GVHD pathophysiology
Major milestones from the early ages of hematopoietic stem cell transplantation deserve first to be mentioned. Pioneers in the field included Van Bekkum, Thomas, and Santos [reviewed in (4, 12–14)]. In parallel, in this period, the major histocompatibility complexes (MHC) in the mice (15) and humans (16) were discovered. Similar advances were made in the MHC typing of dogs and later in non-human primates (17, 18). These early ages were also associated with a bloom in our knowledge of basic (and transplant) immunology that set the basis for developing our knowledge of GVHD (19, 20). But the foundation of our ongoing effort to understand GVHD should be attributed to Billingham, who described the three prerequisite mechanisms for developing GVHD (21, 22); “the graft must contain immunologically competent cells; the recipient must express antigens that are not present in the transplant donor, and the recipient must be incapable of mounting an effective response to eliminating the transplanted cells” [summarized by Ferrara in (5)].
In the late ‘70s, another piece of complexity was introduced with the advent of minor histocompatibility antigens (mi-Ha) in mice that led to GVHD but was prevented by the removal of T-lymphocytes from the graft (23). It then took 20 years before the role of mi-Ha was strongly suggested in humans (24), although the role of individual mi-Ha remains controversial (25).
In the past 60 years, our knowledge of acute GVHD has dramatically improved. Readers with interest might compare figures on the pathophysiology of acute GVHD published by Ferrara and Deeg in 1991 (26) to those published by Zeiser and Blazar (10) or Hill and coworkers (6) to get a sense of how much we improved (although much more science is still needed)!.
The biology of acute GVHD has been classically described by successive steps from conditioning-induced tissue damage to the induction phase, donor T-cell expansion, and recruitment of neutrophils and macrophages, to the effector stage and tissue damages (5). However, describing these stages from a historical perspective to describe what have been the significant milestones in acute GVHD pathophysiology poorly fit with this classical circle. In each of the following sections, we aimed to give credit to the first seminal papers and then discussed more recent reports to show how each step evolved during the time. Finally, we introduce in the last sections new aspects that help, in our view, the field to move forward.
Initiation of the allogeneic immune response
The conditioning-induced damages and their early consequences
In the clinical setting, pre-transplant conditioning regimens has long been associated with GVHD’s incidence and severity. In experimental models, Ferrara’s group also demonstrated increased severity of GVHD with increasing dose of total body irradiation (TBI). Increased GVHD was mediated by systemic increases in TNFα. TBI-induced damages increased the translocation of LPS, and LPS triggered excess TNFα alpha from macrophages primed by the GVH reaction as first described by Nestel and coworkers (27) and later confirmed by others (28, 29). T cells in irradiated allogeneic recipients lead to their rapid recruitment to nonlymphoid tissues, where they induce GVHD. But it was later elegantly demonstrated that delayed T-cell injection does not induce GVHD (30). These first pieces of evidence show that the gastrointestinal (GI) tract as a central role in the GVHD pathogenesis, which was then referred to as the “cytokine storm” (31). From a mechanistic point of view, it took another ten years to demonstrate that danger signals released upon cell damage after irradiation, namely ATP, when released from dying cells, increased CD80 and CD86 expression and initiate proinflammatory events, with STAT1, interferon-γ production, donor T cell expansion, and increased expression of the ATP’s receptorP2X (7) R (32). Finally, neutrophils increased conditioning-induced tissue damage caused, and increased GVHD-related mortality. This phenomenon was dependent on reactive oxygen species (ROS). In contrast, the transfer of neutrophils lacking Toll-like receptors led to reduced GVHD severity (33).
Cytokines and chemokines
The first cytokines associated with GVHD-associated tissue lesions were TNFα and IL-1 (34, 35). These findings and other data led Antin and Ferrara to suggest that the recruitment and activation of mononuclear effector cells, which produce inflammatory cytokines, is critical for amplifying the systemic inflammatory response (36). These first publications suggested that GVHD is initiated by alloreactive type 1 T cells that secrete gamma-interferon (IFN-γ) which induces the production of other inflammatory cytokines (TNFα and IL-1). Used polarized type-2 T-cells (secreting IL-4 but not IFN-γ) led to decreased production of both IFN-γ and TNFα despite persistent pathological changes (37). Chemokine and chemokine receptor interactions and adhesion molecules have also been shown to play roles in effector cell migration in experimental GVHD models. A review in the early 20’s discussed the role of chemokines during experimental GVHD (38). However, even to date, it is hard to decipher the actual role of chemokines/chemokine receptors in the activation phase of GVHD, most probably because of the redundant nature of these molecules that recognize different receptors with receptors able to identify different ligands. Finally, intestinal commensal bacteria and uric acid contribute to Nlrp3 inflammasome-mediated IL-1β production after conditioning. Blockade of IL-1β or IL-1-R -/- dendritic cells (DCs) and T cells improved survival. IL-1β originated from different intestinal cells and affected DCs and TH17 cells (39).
T-cell migration into lymphoid organs
The migration and homing of cells in GVHD in vivo was assessed by imaging technics using enhanced green fluorescence protein transgenic-, or luciferase-labeled, allogeneic T cells (40, 41). These in vivo experiments demonstrated an early migration of allogeneic cells first to peripheral lymphoid organs, and then to GVHD target organs. A very early expansion of T-cells in Peyer’s patches was of interest. Early in the 20s, it was demonstrated that naive T cells migrate to secondary lymphoid organs where they underwent activation by antigens and acquired the ability to home to nonlymphoid sites. Activated effector/memory T cells migrate preferentially to tissues where the antigen was first encountered. Essential receptors for intestinal homing α4β7, the receptor for the gut-associated chemokine CCL25, were described. A paper by Von Adrian’s group (outside the setting of transplantation) showed that imprinting toward the GI tract is mediated by dendritic cells (DCs) from Peyer’s patches (42). In the background of GVHD, Murai et al. (43) then demonstrated that Peyer’s patches are an essential site in initiating murine acute GVHD [although this essentiality was, after that, not confirmed (44)].
Antigen presentation and costimulation
Allogeneic T cells stimulation was mysterious till the end of the 90s. In a seminal paper, Shlomchik and Emerson elegantly demonstrated in CD8-mediated, multiple mi-Ha mismatched, models that (APCs) only host-derived antigen-presenting cells (APCs) initiated graft versus host disease (45). Subsequently, Teshima and Ferrara (46) found that, in CD4-mediated models, acute GvHD does not require alloantigen expression on host target epithelium, in a CD4-mediated model. Then, it was demonstrated that although host APCs are mandatory to initiate acute GVHD, donor APCs are required to perpetuate it (47). DCs are not only critical for GVHD induction, DCs were then shown to induce organ tropism through the induction of organ-specific homing molecules T-cells. Gut-derived DCs induced alloreactive gut-homing T-cells leading to increased GVHD severity (48). MHC class II antigen presentation by intestinal epithelial cells (IECs) was then demonstrated within the ileum at steady state. IEC-specific deletion of MHC class II prevented lethal GVHD in the GI tract, and the development of GVHD required IFNγ secretion by lamina propria lymphocytes (49). Finally, in the colon donor CD103(+)CD11b (–) DCs migrate to mesenteric lymph nodes, also leading to gut-homing signatures on donor T cells, leading to GI tract GVHD (50).
T-cell activation needs costimulation that has also been extensively studied within the context of GVHD from a therapeutical perspective. In 1994, Blazar et al. reported that blockade of the CD28/CTLA4: CD80-CD86 interaction reduces lethal GVHD in a major mismatched mice model (51). This was confirmed later in the non-human primate model (NHP) (52, 53) and had recently shown promising results in human GVHD (54). Blockade of the CD40/CD40-ligand ICOS and OX40-ligand has also been reported both in rodents and in NHP models (55–59). Increased GVHD in recipients of B7-H3(-/-) Treg-depleted grafts was also described (60). The role of ICOSL+ DCs was also recently explored both in humans and in experimental models. Increased numbers of ICOSL(+) plasmacytoid DCs (p-DCs), which correlate with CD146+CCR5+ T cell, were found in patients with GI GVHD. ICOSL-deficient mice had reduced GVHD, and prophylaxis with a dual ICOS/CD28 antagonist prevented acute GVHD (61).
Langerhans cells (LCs) act as network in the epidermis of the skin. Using an inducible depletion of host LCs experimental model, Bennet et al. found reduced GVHD when LCs were absent. However, LCs were not required either for CD8 T-cell activation or homing to the epidermis. However, LCs were necessary for licensing IFN-γ secreting donor CD8 cells in the epidermis to induce epithelial injury (62).
Effector- and regulatory-T cells
Early studies showed that both the Fas/Fas-L and perforin/granzyme cytotoxicity were important in inducing GVHD lesions (63–65). Then the development of flow cytometry allowed us to decipher which T-lymphocyte subset mediates GVHD. The landmark papers by Anderson et al. (66, 67) reported that memory CD4 cells induced neither clinical nor histologic GVHD and argued subsequently against the hypothesis that T effector memory cells are unable to generate GVHD because of inefficient trafficking to lymphoid organs. In vivo analyses of the early event in murine models also support the leading role of naïve T-cells (41). These results prompted considering naïve T-cell depletion as a clinical tool to decrease GVHD (68). Of special interest, however, is the paper by Emerson’s group in 2005 that describes a CD44(lo)CD62L(hi)CD8(+) T cell subset that generate and sustain GVHD with self-renewing capacity. These cells are Sca-1+, CD122+, and Bcl-2+ and induce GVHD in secondary recipients (69, 70). This paper is, in fact, the first description of the T-stem cell memory subset and was described in GVHD! Subsequently, with a growing focus on gastrointestinal (GI) GVHD, the role of TH17/TC17 has been the focus of research (71, 72). Finally, thanks to the development of molecular immunology, the activity of JAK 1/2 inhibition blockade was proven both in experimental studies and in humans (69), leading to a successful randomized clinical trial in steroid-resistant acute GVHD (73).
The allogeneic reaction is, however, subjected to cell-based regulation, primarily by regulatory T-cells (Treg). The field of Treg has been initially the subject of controversies, as nicely reviewed by Sakagushi (74). In GVHD three groups almost simultaneously published data in the early ‘20s that convincingly demonstrated that Tregs inhibit GVHD (75–78) while preserving the graft-versus-leukemia effect (79).
The GI tract and the microbiota in GVHD
Although included in the classical Ferrara’s circled schema of GVHD’s pathogenesis (31, 80), it seems us that the GI tract and its relationship with the microbiome deserve special attention in this milestones’ paper because significant recent advances in the knowledge of the dialogue between the microbiome and the intestine in the GVHD process [reviewed in (81)].
During the past ten years, more careful attention has been paid to IEC subsets (i.e., the targets) than on effectors (i.e., T-cell subsets and regulators). Somewhat arbitrary, we will first allude to IEC and second to microbiota, although, obviously, these aspects are interrelated.
As already stated GI tract damages are critical for the amplification of GVHD (31). Intestinal stem cells (ISCs) regenerate the intestinal epithelium after injury. Ten years ago, Teshima’s group demonstrated that the pretransplant conditioning regimen damaged ISCs, and GVHD markedly inhibited ISC recovery. The Wnt agonist R-spondin1 (R-Spo1) protected ISC and increased intestinal epithelium healing (82). Almost simultaneously, it was demonstrated that IL-22 deficient recipients have increased tissue damage and mortality and that ISCs expressed the IL-22 receptor. Intestinal IL-22 was produced by recipient-derived innate lymphoid cells (ILCs). This IL-22 deficiency in the recipient led to increased crypt apoptosis, depletion of ISCs, and loss of epithelial integrity (83). Outside the field of GVHD, it was then reported that Lgr5+ ISCs are the target of activated T cells while quiescent stem cells from the hair follicle were resistant to T cell killing. Immune evasion of the quiescent stem cells resulted from downregulation of the antigen presentation machinery (84). But a broad image of tissue damage was provided by 3D imaging to analyze T-cell localization by Hanash’s group (85). They nicely reported that T-cell recruitment to the basal layer of the crypt leads to direct T cell engagement with MHC+ ISCs and their subsequent loss.
Another cell subset, Paneth cells (PCs), was also studied, and it was demonstrated that GVHD disrupts the intestinal microbiome by inhibiting Paneth cell production of α-defensins (86) and restoration of the gut microbiota by R-Spo1 or recombinant α-defensin in mice (84), thus linking R-Spo1 protection of ISC damage (82, 87) with the intestinal microbiota. Recently, Zeiser’s group explored another approach that aims at protecting and regenerating Paneth cells (PCs) and intestinal stem cells (ISCs). Glucagon-like-peptide-2 (GLP-2), an enteroendocrine hormone produce by intestinal L cells, is decreased both in mice and in patients developing GVHD. Treatment with the GLP-2 agonist, teduglutide, reduced de GVHD and steroid-refractory GVHD by promoting the regeneration of PCs and ISCs (88).
Finally, after ISCs, PCs, and L cells GI-cell subsets, the role of goblet cells was explored in GI GVHD. Intestinal goblet cells form the mucus layers, which serves as a barrier between the gut microbiota and the host tissues. Goblet cell loss is one of the histologic features of GVHD. This leads to a disruption of the mucus layer and increased bacterial translocation. Pretransplant administration of interleukin-25 (IL-25) protected goblet cells from GVHD, prevented bacterial translocation, reduced IFN-γ and IL-6 plasma levels, and ameliorated GVHD. The protective role of IL-25 depended on Lypd8, an enterocyte-derived antimicrobial molecule that suppresses the motility of flagellated bacteria (89).
From the above-reviewed data, it was obvious that the IECs should be studied in the context of their environment, i.e., the microbiota, a concept already brought by Van Bekkum in the early ages of BMT when he described that there is a “Mitigation of secondary disease of allogeneic mouse radiation chimeras by modification of the intestinal microflora” (14). In our current understanding, the seminal paper was published ten years ago by Jenq and coworkers (90). In this paper, authors demonstrated in murine and human recipients that intestinal inflammation secondary to GVHD is associated with significant intestinal dysbiosis with loss of overall diversity and expansion of Lactobacillales and loss of Clostridiales. This dysbiosis early after allogeneic BMT was a risk factor for subsequent GVHD. The increased GVHD-related mortality was associated with broad-spectrum antibiotic use after BMT in humans and mice (91). This was confirmed in more than 1000 patients, where studies on more than 8000 fecal samples disclosed patterns of microbiota disruption characterized by loss of diversity and domination by single taxa. Higher diversity was associated with a lower risk of death overall and death attributable to GVHD (92). Thus, while broad-spectrum antibiotics increase the risk of intestinal GVHD, the mechanisms remained elusive. In a recent study, Jenq’s laboratory found that treatment with meropenem aggravated colonic GVHD in mice via the expansion of Bacteroides thetaiotaomicron (BT) which can degrade dietary polysaccharides and host mucin glycans. BT in meropenem-treated mice upregulated enzymes involved in the degradation of glycans within the mucus leading to thinning of the colonic mucus layer and decreased levels of xylose in the lumen. Finally, oral xylose supplementation prevented thinning of the colonic mucus layer (93).
Several studies aimed to decipher the mechanisms of the crosstalk between GVHD and the microbiota from these experimental and clinical data. In one study, recipients lacking the ability to generate or signal IL-17 develop hyper-acute intestinal GVHD. When authors cohoused wild-type (WT) with IL-17RA and IL-17R-deficient mice, they found increased GVHD severity in WT mice, and the gut microbiome of WT mice shifted toward that of the IL-17RA/C mice (94). From a microbiological point of view, a high incidence of enterococcal expansion was then described, which was associated with GVHD and mortality in humans. Enterococcus also expanded in the mouse GI tract after transplantation. Enterococcus growth depends on Lactose, and lactose depletion attenuates Enterococcus outgrowth and reduces the severity of GVHD in mice (95). To decipher the impact of intestinal microbiota on microbial metabolites in GVHD, in a major paper by Reddy’s group identified alterations in microbiota-derived short-chain fatty acids (SCFAs) and found that only one SCFA, butyrate, was decreased in the intestinal tissue. The reduced butyrate in IECs resulted in decreased histone acetylation, which was restored after the administration of butyrate. Butyrate restoration improved IEC junctional integrity, reduced apoptosis, and mitigated GVHD (96). The same group then demonstrated that the metabolite sensor G-protein-coupled receptor 43 (GPR43) was critical for the protective effects of butyrate and require GPR43-mediated ERK phosphorylation and activation of the NLRP3 inflammasome in recipients’ target tissues (97).
GVHD pathophysiology: What’s next?
From the above-summarized data, we would finally put into perspective other ways of thinking about GVHD pathophysiology.
Tissue tolerance and compartmentalization of acute GVHD
Polly Matzinger first described the concept of tissue tolerance in 2011 (98). Far outside the field of GVHD, she studied the perspective on how the immune response is regulated. Rather than describing how immune cells are turned on and off, she focused on the second significant aspect of an immune response: the control of effector class. She suggested that the immune response is “primarily tailored to fit the tissue in which the response occurs”. More recently, Wu and Reddy (99) nicely discussed tissue damages in the setting of GVHD and underlined that acute GVHD had been mostly studied through on the aspect on how immune cells generate tolerance. However, tissue-intrinsic factors might contribute to the regulation of acute GVHD severity. They thus introduced the concept of “tissue tolerance” with the aim of integrating targeted tissue repair and regeneration, which may mitigate the severity of acute GVHD. Such a concept has also been advocated by Chakraverty and Teshima more recently (100).
More broadly, the compartmentalization of the allogeneic reaction may warrant further attention. Whether GVHD and GVL behave equally among different tissues remain unclear. An in vivo experimental study indeed showed that CTLs exhibited different cytotoxic function in distinct tissues after transplantation, being highest in the liver and lowest in lymph nodes. These differences came from the impact of the microenvironment, through differential PD-1 ligand expression. PD-1 blockade restored CTL sensitivity to antigens and killing in lymph nodes (101).
Rebooting immunology in GVHD pathophysiology
As we reviewed elsewhere in the issue (Michonneau & Socie) impressive bloom of new technologies has emerged in the field of biology in the past few years, including single-cell technologies that allow studying pathophysiology at the genomic, epigenetic, transcriptomic, and protein levels. These have obvious consequences for deciphering in depth the pathophysiology mechanisms of GVHD. Most of the data described here come from experimental mice models of acute GVHD. We have already described elsewhere how important these models have been operative in deciphering the mechanisms of GVHD and have been able to set the stage for (eventually) successful clinical trials (8). As nicely reviewed by Brodin and Davis (102), conceptual and technological progress in human immunology has been impressive over the past few years. In particular single-cell technologies which allow in depth analyses with small quantities of human material allow now to study in far more depth immunological mechanisms in humans, and to integrate the impact of genetic or environmental associated factors.
The first study that opened the gate in human GVHD was that of Claude Perreault’s group in 2007 (103). In this seminal paper, authors asked if transcriptional profiles may identify “dangerous” donors more prone to mount a strong allogeneic reaction and, consequently, more likely to elicit GVHD. They measured gene expression of purified CD4(+) and CD8(+) T cells from 50 donors and found that pre-transplant profiling segregates donors whose recipient suffered from GVHD or not. This phenomenon is under polygenic control and coordinated by genes that regulate transforming growth factor-beta signaling and cell proliferation. Another step forward was then achieved in the NHP model by Leslie Kean’s group which quantified gene expression profile of non-human primate (NHP) T cells during acute GVHD. They identified signatures specific for alloreactive T cells and determined the impact of both mTOR and calcineurin inhibition on GVHD and discovered a druggable target aurora kinase A (104). Using high throughput plasma metabolomic in two cohorts of genotypically HLA-identical related pairs we recently reported that the metabolomic profiles markedly differed between recipients and donors. In particular, at GvHD onset, we identified significant variation in microbiota-derived metabolites, in particular of aryl hydrocarbon receptor (AhR) ligands and bile acids. We thus suspected that lower microbiota-derived AhR ligands may limit indoleamine 2,3-dioxygenase induction and thus influence alloreactivity (105).
Most recently, 3 groups shed new light on T-resident memory (Trm) T-cells in NHP and humans. In the NHP study, authors analyzed the transcriptomic of circulating, emigrating or already existing tissue-resident T cells in aGVHD target organs. Combining intravascular staining technique with single-cell RNA sequencing they demonstrate that T cell infiltration of the GI by donor CD8+ T cells was associated with an activated/cytotoxic phenotype and provided a canonical Trm signature (106). In humans, three other studies also pointed out that skin contains a population of host derived CD69+ αβ Trm that persist and remain functionally competent for years after BMT. Single-cell RNA sequencing revealed low expression of genes encoding tissue egress by Trm in the skin. However, these Trm T-cells are characterized by the expression of molecules that favor tissue retention and display stem cell markers (107–109). Finally, most studies focused on failure (i.e., GVHD), but none paid attention to operational tolerance without GVHD. In a recent study, we studied who underwent transplantation from HLA-identical siblings. Primary tolerance was associated with a long-lasting reshaping of the recipient’s immune system toward a regulatory T cell phenotype, and decreased T cell activation, proliferation, and migration. When we compared individuals with operational tolerance and nontolerant recipients, we observed alterations centered on CD38+-activated T and B cells in nontolerant patients. In tolerant patients, cell subsets with regulatory functions were prominent and RNA sequencing demonstrated several modifications, particularly with overexpression of the ectoenzyme NT5E (encoding CD73). Metabolomic analyses suggested a central role of androgens in operational tolerance. These data were confirmed using an integrative approach including the 3 omics tools and clinical parameters providing a resource to study operational tolerance in humans (110).
It is our own bias to believe that modern tools, including single cell multi-omics, both in experimental transplantation and in the human setting open a new area in the study of the pathophysiology of acute GVHD. Furthermore, recent development in tissue imaging (including mass cytometry) and spatial transcriptomics will allow the target organ. We might thus be faced with new studies where in-depth description of affected organs in human can lead to mechanistic experiments in the mice system.
Author contributions
All authors contributed to the article and approved the submitted version.
Acknowledgments
I (GS) would like to sincerely thank my friends and worldwide recognized experts in the immune biology of GVHD. They kindly agreed to give me their own top 10 references which they believe to be the milestones in this topic: Bruce Blazar, Jamy Ferrara, Pavan Reddy, Jerry Ritz, Takanori Teshima, Marcel Van Den Brinck, Robert Zeiser. Special dedication also to Claude Perreault, whose work inspired me to study the immune biology of allogeneic transplantation in humans.
Conflict of interest
The authors declare that the research was conducted in the absence of any commercial or financial relationships that could be construed as a potential conflict of interest.
Publisher’s note
All claims expressed in this article are solely those of the authors and do not necessarily represent those of their affiliated organizations, or those of the publisher, the editors and the reviewers. Any product that may be evaluated in this article, or claim that may be made by its manufacturer, is not guaranteed or endorsed by the publisher.
References
1. Barnes DW, Corp MJ, Loutit JF, Neal FE. Treatment of murine leukaemia with X rays and homologous bone marrow; preliminary communication. Br Med J (1956) 2:626–7. doi: 10.1136/bmj.2.4993.626
2. Barnes DW, Loutit JF. Treatment of murine leukaemia with x-rays and homologous bone marrow. II. Br J Haematol (1957) 3:241–52. doi: 10.1111/j.1365-2141.1957.tb05793.x
3. Mathe G, Amiel JL, Schwarzenberg L, Cattan A, Schneider M. Haematopoietic chimera in man after allogeneic (homologous) bone-marrow transplantation (Control of the secondary syndrome. specific tolerance due to the chimerism. Br Med J (1963) 2:1633–5. doi: 10.1136/bmj.2.5373.1633
4. Teshima T, Hill GR. The pathophysiology and treatment of graft-Versus-Host disease: Lessons learnt from animal models. Front Immunol (2021) 12:715424. doi: 10.3389/fimmu.2021.715424
5. Ferrara JLM, Levine JE, Reddy P, Holler E. Graft-versus-host disease. Lancet (2009) 373:1550–61. doi: 10.1016/S0140-6736(09)60237-3
6. Hill GR, Betts BC, Tkachev V, Kean LS, Blazar BR. Current concepts and advances in graft-Versus-Host disease immunology. Annu Rev Immunol (2021) 39:19–49. doi: 10.1146/annurev-immunol-102119-073227
7. Jenq RR, van den Brink MRM. Allogeneic haematopoietic stem cell transplantation: individualized stem cell and immune therapy of cancer. Nat Rev Cancer (2010) 10:213–21. doi: 10.1038/nrc2804
8. Socié G, Kean LS, Zeiser R, Blazar BR. Insights from integrating clinical and preclinical studies advance understanding of graft-versus-host disease. J Clin Invest (2021) 131:149296. doi: 10.1172/JCI149296
9. Vincent K, Roy D-C, Perreault C. Next-generation leukemia immunotherapy. Blood (2011) 118:2951–9. doi: 10.1182/blood-2011-04-350868
10. Zeiser R, Blazar BR. Acute graft-versus-Host disease — biologic process, prevention, and therapy. N Engl J Med (2017) 377:2167–79. doi: 10.1056/NEJMra1609337
11. Zeiser R, Blazar BR. Pathophysiology of chronic graft-versus-Host disease and therapeutic targets. N Engl J Med (2017) 377:2565–79. doi: 10.1056/NEJMra1703472
12. Thomas ED. A history of haemopoietic cell transplantation. Br J Haematol (1999) 105:330–9. doi: 10.1111/j.1365-2141.1999.01337.x
13. Vriesendorp HM, Heidt PJ. History of graft-versus-host disease. Exp Hematol (2016) 44:674–88. doi: 10.1016/j.exphem.2016.05.011
14. Van Bekkum DW, Vos O, Weyzen WW. The pathogenesis of the secondary disease after foreign bone marrow transplantation in x-irradiated mice. J Natl Cancer Inst (1959) 23:75–89.
15. Snell GD. The h-2 locus of the mouse: observations and speculations concerning its comparative genetics and its polymorphism. Folia Biol (Praha) (1968) 14:335–58.
16. Dausset J. The major histocompatibility complex in man. Science (1981) 213:1469–74. doi: 10.1126/science.6792704
17. Atkinson K, Shulman HM, Deeg HJ, Weiden PL, Graham TC, Thomas ED, et al. Acute and chronic graft-versus-host disease in dogs given hemopoietic grafts from DLA-nonidentical littermates. two distinct syndromes. Am J Pathol (1982) 108:196–205.
18. Boieri M, Shah P, Dressel R, Inngjerdingen M. The role of animal models in the study of hematopoietic stem cell transplantation and GvHD: A historical overview. Front Immunol (2016) 7:333. doi: 10.3389/fimmu.2016.00333
19. Simpson E. Medawar’s legacy to cellular immunology and clinical transplantation: a commentary on billingham, Brent and medawar (1956) “Quantitative studies on tissue transplantation immunity. III. actively acquired tolerance.” Philos Trans R Soc Lond B Biol Sci (2015) 370:20140382 doi: 10.1098/rstb.2014.0382
20. Kaufmann SHE. Immunology’s coming of age. Front Immunol (2019) 10:684. doi: 10.3389/fimmu.2019.00684
22. Wilson DB, Silvers WK, Billingham RE. Failure to transfer sensitivity to skin homografts by means of “immune” lymphoid cells in diffusion chambers. Nature (1966) 209:1359–60. doi: 10.1038/2091359a0
23. Korngold R, Sprent J. Lethal graft-versus-host disease after bone marrow transplantation across minor histocompatibility barriers in mice. prevention by removing mature T cells from marrow. J Exp Med (1978) 148:1687–98. doi: 10.1084/jem.148.6.1687
24. Goulmy E, Schipper R, Pool J, Blokland E, Falkenburg JH, Vossen J, et al. Mismatches of minor histocompatibility antigens between HLA-identical donors and recipients and the development of graft-versus-host disease after bone marrow transplantation. N Engl J Med (1996) 334:281–5. doi: 10.1056/NEJM199602013340501
25. Martin PJ, Levine DM, Storer BE, Zheng X, Jain D, Heavner B, et al. A model of minor histocompatibility antigens in allogeneic hematopoietic cell transplantation. Front Immunol (2021) 12:782152. doi: 10.3389/fimmu.2021.782152
26. Ferrara JL, Deeg HJ. Graft-versus-host disease. N Engl J Med (1991) 324:667–74. doi: 10.1056/NEJM199103073241005
27. Nestel FP, Price KS, Seemayer TA, Lapp WS. Macrophage priming and lipopolysaccharide-triggered release of tumor necrosis factor alpha during graft-versus-host disease. J Exp Med (1992) 175:405–13. doi: 10.1084/jem.175.2.405
28. Hill GR, Crawford JM, Cooke KR, Brinson YS, Pan L, Ferrara JL. Total body irradiation and acute graft-versus-host disease: The role of gastrointestinal damage and inflammatory cytokines. Blood (1997) 90:3204–13. doi: 10.1182/blood.V90.8.3204
29. Cooke KR, Hill GR, Crawford JM, Bungard D, Brinson YS, Delmonte J, et al. Tumor necrosis factor- alpha production to lipopolysaccharide stimulation by donor cells predicts the severity of experimental acute graft-versus-host disease. J Clin Invest (1998) 102:1882–91. doi: 10.1172/JCI4285
30. Chakraverty R, Côté D, Buchli J, Cotter P, Hsu R, Zhao G, et al. An inflammatory checkpoint regulates recruitment of graft-versus-host reactive T cells to peripheral tissues. J Exp Med (2006) 203:2021–31. doi: 10.1084/jem.20060376
31. Hill GR, Ferrara JL. The primacy of the gastrointestinal tract as a target organ of acute graft-versus-host disease: rationale for the use of cytokine shields in allogeneic bone marrow transplantation. Blood (2000) 95:2754–9. doi: 10.1182/blood.V95.9.2754.009k25_2754_2759
32. Wilhelm K, Ganesan J, Müller T, Dürr C, Grimm M, Beilhack A, et al. Graft-versus-host disease is enhanced by extracellular ATP activating P2X7R. Nat Med (2010) 16:1434–8. doi: 10.1038/nm.2242
33. Schwab L, Goroncy L, Palaniyandi S, Gautam S, Triantafyllopoulou A, Mocsai A, et al. Neutrophil granulocytes recruited upon translocation of intestinal bacteria enhance graft-versus-host disease via tissue damage. Nat Med (2014) 20:648–54. doi: 10.1038/nm.3517
34. Piguet PF, Grau GE, Allet B, Vassalli P. Tumor necrosis factor/cachectin is an effector of skin and gut lesions of the acute phase of graft-vs.-host disease. J Exp Med (1987) 166:1280–9. doi: 10.1084/jem.166.5.1280
35. Hill GR, Teshima T, Gerbitz A, Pan L, Cooke KR, Brinson YS, et al. Differential roles of IL-1 and TNF-alpha on graft-versus-host disease and graft versus leukemia. J Clin Invest (1999) 104:459–67. doi: 10.1172/JCI6896
36. Antin JH, Ferrara JL. Cytokine dysregulation and acute graft-versus-host disease. Blood (1992) 80:2964–8. doi: 10.1182/blood.V80.12.2964.2964
37. Krenger W, Cooke KR, Crawford JM, Sonis ST, Simmons R, Pan L, et al. Transplantation of polarized type 2 donor T cells reduces mortality caused by experimental graft-versus-host disease. Transplantation (1996) 62:1278–85. doi: 10.1097/00007890-199611150-00018
38. Wysocki CA, Panoskaltsis-Mortari A, Blazar BR, Serody JS. Leukocyte migration and graft-versus-host disease. Blood (2005) 105:4191–9. doi: 10.1182/blood-2004-12-4726
39. Jankovic D, Ganesan J, Bscheider M, Stickel N, Weber FC, Guarda G, et al. The Nlrp3 inflammasome regulates acute graft-versus-host disease. J Exp Med (2013) 210:1899–910. doi: 10.1084/jem.20130084
40. Panoskaltsis-Mortari A, Price A, Hermanson JR, Taras E, Lees C, Serody JS, et al. In vivo imaging of graft-versus-host-disease in mice. Blood (2004) 103:3590–8. doi: 10.1182/blood-2003-08-2827
41. Beilhack A, Schulz S, Baker J, Beilhack GF, Wieland CB, Herman EI, et al. In vivo analyses of early events in acute graft-versus-host disease reveal sequential infiltration of T-cell subsets. Blood (2005) 106:1113–22. doi: 10.1182/blood-2005-02-0509
42. Mora JR, Bono MR, Manjunath N, Weninger W, Cavanagh LL, Rosemblatt M, et al. Selective imprinting of gut-homing T cells by peyer’s patch dendritic cells. Nature (2003) 424:88–93. doi: 10.1038/nature01726
43. Murai M, Yoneyama H, Ezaki T, Suematsu M, Terashima Y, Harada A, et al. Peyer’s patch is the essential site in initiating murine acute and lethal graft-versus-host reaction. Nat Immunol (2003) 4:154–60. doi: 10.1038/ni879
44. Welniak LA, Kuprash DV, Tumanov AV, Panoskaltsis-Mortari A, Blazar BR, Sun K, et al. Peyer patches are not required for acute graft-versus-host disease after myeloablative conditioning and murine allogeneic bone marrow transplantation. Blood (2006) 107:410–2. doi: 10.1182/blood-2004-11-4565
45. Shlomchik WD, Couzens MS, Tang CB, McNiff J, Robert ME, Liu J, et al. Prevention of graft versus host disease by inactivation of host antigen-presenting cells. Science (1999) 285:412–5. doi: 10.1126/science.285.5426.412
46. Teshima T, Ordemann R, Reddy P, Gagin S, Liu C, Cooke KR, et al. Acute graft-versus-host disease does not require alloantigen expression on host epithelium. Nat Med (2002) 8:575–81. doi: 10.1038/nm0602-575
47. Matte CC, Liu J, Cormier J, Anderson BE, Athanasiadis I, Jain D, et al. Donor APCs are required for maximal GVHD but not for GVL. Nat Med (2004) 10:987–92. doi: 10.1038/nm1089
48. Kim TD, Terwey TH, Zakrzewski JL, Suh D, Kochman AA, Chen ME, et al. Organ-derived dendritic cells have differential effects on alloreactive T cells. Blood (2008) 111:2929–40. doi: 10.1182/blood-2007-06-096602
49. Koyama M, Mukhopadhyay P, Schuster IS, Henden AS, Hülsdünker J, Varelias A, et al. MHC class II antigen presentation by the intestinal epithelium initiates graft-versus-Host disease and is influenced by the microbiota. Immunity (2019) 51:885–898.e7. doi: 10.1016/j.immuni.2019.08.011
50. Koyama M, Cheong M, Markey KA, Gartlan KH, Kuns RD, Locke KR, et al. Donor colonic CD103+ dendritic cells determine the severity of acute graft-versus-host disease. J Exp Med (2015) 212:1303–21. doi: 10.1084/jem.20150329
51. Blazar BR, Taylor PA, Linsley PS, Vallera DA. In vivo blockade of CD28/CTLA4: B7/BB1 interaction with CTLA4-ig reduces lethal murine graft-versus-host disease across the major histocompatibility complex barrier in mice. Blood (1994) 83:3815–25. doi: 10.1182/blood.V83.12.3815.3815
52. Miller WP, Srinivasan S, Panoskaltsis-Mortari A, Singh K, Sen S, Hamby K, et al. GVHD after haploidentical transplantation: a novel, MHC-defined rhesus macaque model identifies CD28- CD8+ T cells as a reservoir of breakthrough T-cell proliferation during costimulation blockade and sirolimus-based immunosuppression. Blood (2010) 116:5403–18. doi: 10.1182/blood-2010-06-289272
53. Watkins BK, Tkachev V, Furlan SN, Hunt DJ, Betz K, Yu A, et al. CD28 blockade controls T cell activation to prevent graft-versus-host disease in primates. J Clin Invest (2018) 128:3991–4007. doi: 10.1172/JCI98793
54. Watkins B, Qayed M, McCracken C, Bratrude B, Betz K, Suessmuth Y, et al. Phase II trial of costimulation blockade with abatacept for prevention of acute GVHD. J Clin Oncol (2021) 39:1865-77. doi: 10.1200/JCO.20.01086
55. Blazar BR, Taylor PA, Panoskaltsis-Mortari A, Buhlman J, Xu J, Flavell RA, et al. Blockade of CD40 ligand-CD40 interaction impairs CD4+ T cell-mediated alloreactivity by inhibiting mature donor T cell expansion and function after bone marrow transplantation. J Immunol (1997) 158:29–39.
56. Blazar BR, Sharpe AH, Chen AI, Panoskaltsis-Mortari A, Lees C, Akiba H, et al. Ligation of OX40 (CD134) regulates graft-versus-host disease (GVHD) and graft rejection in allogeneic bone marrow transplant recipients. Blood (2003) 101:3741–8. doi: 10.1182/blood-2002-10-3048
57. Taylor PA, Panoskaltsis-Mortari A, Freeman GJ, Sharpe AH, Noelle RJ, Rudensky AY, et al. Targeting of inducible costimulator (ICOS) expressed on alloreactive T cells down-regulates graft-versus-host disease (GVHD) and facilitates engraftment of allogeneic bone marrow (BM). Blood (2005) 105:3372–80. doi: 10.1182/blood-2004-10-3869
58. Taylor PA, Panoskaltsis-Mortari A, Noelle RJ, Blazar BR. Analysis of the requirements for the induction of CD4+ T cell alloantigen hyporesponsiveness by ex vivo anti-CD40 ligand antibody. J Immunol (2000) 164:612–22. doi: 10.4049/jimmunol.164.2.612
59. Tkachev V, Furlan SN, Watkins B, Hunt DJ, Zheng HB, Panoskaltsis-Mortari A, et al. Combined OX40L and mTOR blockade controls effector T cell activation while preserving treg reconstitution after transplant. Sci Transl Med (2017) 9. doi: 10.1126/scitranslmed.aan3085
60. Veenstra RG, Flynn R, Kreymborg K, McDonald-Hyman C, Saha A, Taylor PA, et al. B7-H3 expression in donor T cells and host cells negatively regulates acute graft-versus-host disease lethality. Blood (2015) 125:3335–46. doi: 10.1182/blood-2014-09-603357
61. Adom D, Dillon SR, Yang J, Liu H, Ramadan A, Kushekhar K, et al. ICOSL+ plasmacytoid dendritic cells as inducer of graft-versus-host disease, responsive to a dual ICOS/CD28 antagonist. Sci Transl Med (2020) 12. doi: 10.1126/scitranslmed.aay4799
62. Bennett CL, Fallah-Arani F, Conlan T, Trouillet C, Goold H, Chorro L, et al. Langerhans cells regulate cutaneous injury by licensing CD8 effector cells recruited to the skin. Blood (2011) 117:7063–9. doi: 10.1182/blood-2011-01-329185
63. Graubert TA, DiPersio JF, Russell JH, Ley TJ. Perforin/granzyme-dependent and independent mechanisms are both important for the development of graft-versus-host disease after murine bone marrow transplantation. J Clin Invest (1997) 100:904–11. doi: 10.1172/JCI119606
64. Jiang Z, Podack E, Levy RB. Major histocompatibility complex-mismatched allogeneic bone marrow transplantation using perforin and/or fas ligand double-defective CD4(+) donor T cells: involvement of cytotoxic function by donor lymphocytes prior to graft-versus-host disease pathogenesis. Blood (2001) 98:390–7. doi: 10.1182/blood.v98.2.390
65. van Den Brink MR, Moore E, Horndasch KJ, Crawford JM, Hoffman J, Murphy GF, et al. Fas-deficient lpr mice are more susceptible to graft-versus-host disease. J Immunol (2000) 164:469–80. doi: 10.4049/jimmunol.164.1.469
66. Anderson BE, McNiff J, Yan J, Doyle H, Mamula M, Shlomchik MJ, et al. Memory CD4+ T cells do not induce graft-versus-host disease. J Clin Invest (2003) 112:101–8. doi: 10.1172/JCI17601
67. Anderson BE, Taylor PA, McNiff JM, Jain D, Demetris AJ, Panoskaltsis-Mortari A, et al. Effects of donor T-cell trafficking and priming site on graft-versus-host disease induction by naive and memory phenotype CD4 T cells. Blood (2008) 111:5242–51. doi: 10.1182/blood-2007-09-107953
68. Bleakley M, Heimfeld S, Loeb KR, Jones LA, Chaney C, Seropian S, et al. Outcomes of acute leukemia patients transplanted with naive T cell-depleted stem cell grafts. J Clin Invest (2015) 125:2677–89. doi: 10.1172/JCI81229
69. Zhang Y, Joe G, Hexner E, Zhu J, Emerson SG. Host-reactive CD8+ memory stem cells in graft-versus-host disease. Nat Med (2005) 11:1299–305. doi: 10.1038/nm1326
70. Spoerl S, Mathew NR, Bscheider M, Schmitt-Graeff A, Chen S, Mueller T, et al. Activity of therapeutic JAK 1/2 blockade in graft-versus-host disease. Blood (2014) 123:3832–42. doi: 10.1182/blood-2013-12-543736
71. Furlan SN, Watkins B, Tkachev V, Cooley S, Panoskaltsis-Mortari A, Betz K, et al. Systems analysis uncovers inflammatory Th/Tc17-driven modules during acute GVHD in monkey and human T cells. Blood (2016) 128:2568–79. doi: 10.1182/blood-2016-07-726547
72. Gartlan KH, Markey KA, Varelias A, Bunting MD, Koyama M, Kuns RD, et al. Tc17 cells are a proinflammatory, plastic lineage of pathogenic CD8+ T cells that induce GVHD without antileukemic effects. Blood (2015) 126:1609–20. doi: 10.1182/blood-2015-01-622662
73. Zeiser R, von Bubnoff N, Butler J, Mohty M, Niederwieser D, Or R, et al. Ruxolitinib for glucocorticoid-refractory acute graft-versus-Host disease. N Engl J Med (2020) 382:1800–10. doi: 10.1056/NEJMoa1917635
74. Sakaguchi S, Wing K, Miyara M. Regulatory T cells - a brief history and perspective. Eur J Immunol (2007) 37 Suppl 1:S116–123. doi: 10.1002/eji.200737593
75. Cohen JL, Trenado A, Vasey D, Klatzmann D, Salomon BL. CD4(+)CD25(+) immunoregulatory T cells: new therapeutics for graft-versus-host disease. J Exp Med (2002) 196:401–6. doi: 10.1084/jem.20020090
76. Hoffmann P, Ermann J, Edinger M, Fathman CG, Strober S. Donor-type CD4(+)CD25(+) regulatory T cells suppress lethal acute graft-versus-host disease after allogeneic bone marrow transplantation. J Exp Med (2002) 196:389–99. doi: 10.1084/jem.20020399
77. Taylor PA, Noelle RJ, Blazar BR. CD4(+)CD25(+) immune regulatory cells are required for induction of tolerance to alloantigen via costimulatory blockade. J Exp Med (2001) 193:1311–8. doi: 10.1084/jem.193.11.1311
78. Taylor PA, Lees CJ, Blazar BR. The infusion of ex vivo activated and expanded CD4(+)CD25(+) immune regulatory cells inhibits graft-versus-host disease lethality. Blood (2002) 99:3493–9. doi: 10.1182/blood.v99.10.3493
79. Edinger M, Hoffmann P, Ermann J, Drago K, Fathman CG, Strober S, et al. CD4+CD25+ regulatory T cells preserve graft-versus-tumor activity while inhibiting graft-versus-host disease after bone marrow transplantation. Nat Med (2003) 9:1144–50. doi: 10.1038/nm915
80. Koyama M, Hill GR. The primacy of gastrointestinal tract antigen-presenting cells in lethal graft-versus-host disease. Blood (2019) 134:2139–48. doi: 10.1182/blood.2019000823
81. Rafei H, Jenq RR. Microbiome-intestine cross talk during acute graft-versus-host disease. Blood (2020) 136:401–9. doi: 10.1182/blood.2019000950
82. Takashima S, Kadowaki M, Aoyama K, Koyama M, Oshima T, Tomizuka K, et al. The wnt agonist r-spondin1 regulates systemic graft-versus-host disease by protecting intestinal stem cells. J Exp Med (2011) 208:285–94. doi: 10.1084/jem.20101559
83. Hanash AM, Dudakov JA, Hua G, O’Connor MH, Young LF, Singer NV, et al. Interleukin-22 protects intestinal stem cells from immune-mediated tissue damage and regulates sensitivity to graft versus host disease. Immunity (2012) 37:339–50. doi: 10.1016/j.immuni.2012.05.028
84. Agudo J, Park ES, Rose SA, Alibo E, Sweeney R, Dhainaut M, et al. Quiescent tissue stem cells evade immune surveillance. Immunity (2018) 48:271–285.e5. doi: 10.1016/j.immuni.2018.02.001
85. Fu Y-Y, Egorova A, Sobieski C, Kuttiyara J, Calafiore M, Takashima S, et al. T Cell recruitment to the intestinal stem cell compartment drives immune-mediated intestinal damage after allogeneic transplantation. Immunity (2019) 51:90–103.e3. doi: 10.1016/j.immuni.2019.06.003
86. Eriguchi Y, Takashima S, Oka H, Shimoji S, Nakamura K, Uryu H, et al. Graft-versus-host disease disrupts intestinal microbial ecology by inhibiting paneth cell production of α-defensins. Blood (2012) 120:223–31. doi: 10.1182/blood-2011-12-401166
87. Hayase E, Hashimoto D, Nakamura K, Noizat C, Ogasawara R, Takahashi S, et al. R-Spondin1 expands paneth cells and prevents dysbiosis induced by graft-versus-host disease. J Exp Med (2017) 214:3507–18. doi: 10.1084/jem.20170418
88. Norona J, Apostolova P, Schmidt D, Ihlemann R, Reischmann N, Taylor G, et al. Glucagon-like peptide 2 for intestinal stem cell and paneth cell repair during graft-versus-host disease in mice and humans. Blood (2020) 136:1442–55. doi: 10.1182/blood.2020005957
89. Ara T, Hashimoto D, Hayase E, Noizat C, Kikuchi R, Hasegawa Y, et al. Intestinal goblet cells protect against GVHD after allogeneic stem cell transplantation via Lypd8. Sci Transl Med (2020) 12:eaaw0720. doi: 10.1126/scitranslmed.aaw0720
90. Jenq RR, Ubeda C, Taur Y, Menezes CC, Khanin R, Dudakov JA, et al. Regulation of intestinal inflammation by microbiota following allogeneic bone marrow transplantation. J Exp Med (2012) 209:903–11. doi: 10.1084/jem.20112408
91. Shono Y, Docampo MD, Peled JU, Perobelli SM, Velardi E, Tsai JJ, et al. Increased GVHD-related mortality with broad-spectrum antibiotic use after allogeneic hematopoietic stem cell transplantation in human patients and mice. Sci Transl Med (2016) 8:339ra71. doi: 10.1126/scitranslmed.aaf2311
92. Peled JU, Gomes ALC, Devlin SM, Littmann ER, Taur Y, Sung AD, et al. Microbiota as predictor of mortality in allogeneic hematopoietic-cell transplantation. N Engl J Med (2020) 382:822–34. doi: 10.1056/NEJMoa1900623
93. Hayase E, Hayase T, Jamal MA, Miyama T, Chang C-C, Ortega MR, et al. Mucus-degrading bacteroides link carbapenems to aggravated graft-versus-host disease. Cell (2022) 185:3705–3719.e14. doi: 10.1016/j.cell.2022.09.007
94. Varelias A, Ormerod KL, Bunting MD, Koyama M, Gartlan KH, Kuns RD, et al. Acute graft-versus-host disease is regulated by an IL-17-sensitive microbiome. Blood (2017) 129:2172–85. doi: 10.1182/blood-2016-08-732628
95. Stein-Thoeringer CK, Nichols KB, Lazrak A, Docampo MD, Slingerland AE, Slingerland JB, et al. Lactose drives enterococcus expansion to promote graft-versus-host disease. Science (2019) 366:1143–9. doi: 10.1126/science.aax3760
96. Mathewson ND, Jenq R, Mathew AV, Koenigsknecht M, Hanash A, Toubai T, et al. Gut microbiome-derived metabolites modulate intestinal epithelial cell damage and mitigate graft-versus-host disease. Nat Immunol (2016) 17:505–13. doi: 10.1038/ni.3400
97. Fujiwara H, Docampo MD, Riwes M, Peltier D, Toubai T, Henig I, et al. Microbial metabolite sensor GPR43 controls severity of experimental GVHD. Nat Commun (2018) 9:3674. doi: 10.1038/s41467-018-06048-w
98. Matzinger P, Kamala T. Tissue-based class control: the other side of tolerance. Nat Rev Immunol (2011) 11:221–30. doi: 10.1038/nri2940
99. Wu S-R, Reddy P. Tissue tolerance: a distinct concept to control acute GVHD severity. Blood (2017) 129:1747–52. doi: 10.1182/blood-2016-09-740431
100. Chakraverty R, Teshima T. Graft-versus-host disease: a disorder of tissue regeneration and repair. Blood (2021) 138:1657–65. doi: 10.1182/blood.2021011867
101. Michonneau D, Sagoo P, Breart B, Garcia Z, Celli S, Bousso P. The PD-1 axis enforces an anatomical segregation of CTL activity that creates tumor niches after allogeneic hematopoietic stem cell transplantation. Immunity (2016) 44:143–54. doi: 10.1016/j.immuni.2015.12.008
102. Davis MM, Brodin P. Rebooting human immunology. Annu Rev Immunol (2018) 36:843–64. doi: 10.1146/annurev-immunol-042617-053206
103. Baron C, Somogyi R, Greller LD, Rineau V, Wilkinson P, Cho CR, et al. Prediction of graft-versus-host disease in humans by donor gene-expression profiling. PloS Med (2007) 4:e23. doi: 10.1371/journal.pmed.0040023
104. Furlan SN, Watkins B, Tkachev V, Flynn R, Cooley S, Ramakrishnan S, et al. Transcriptome analysis of GVHD reveals aurora kinase a as a targetable pathway for disease prevention. Sci Transl Med (2015) 7:315ra191. doi: 10.1126/scitranslmed.aad3231
105. Michonneau D, Latis E, Curis E, Dubouchet L, Ramamoorthy S, Ingram B, et al. Metabolomics analysis of human acute graft-versus-host disease reveals changes in host and microbiota-derived metabolites. Nat Commun (2019) 10:5695. doi: 10.1038/s41467-019-13498-3
106. Tkachev V, Kaminski J, Potter EL, Furlan SN, Yu A, Hunt DJ, et al. Spatiotemporal single-cell profiling reveals that invasive and tissue-resident memory donor CD8+ T cells drive gastrointestinal acute graft-versus-host disease. Sci Transl Med (2021) 13. doi: 10.1126/scitranslmed.abc0227
107. Strobl J, Pandey RV, Krausgruber T, Bayer N, Kleissl L, Reininger B, et al. Long-term skin-resident memory T cells proliferate in situ and are involved in human graft-versus-host disease. Sci Transl Med (2020) 12. doi: 10.1126/scitranslmed.abb7028
108. Strobl J, Gail LM, Kleissl L, Pandey RV, Smejkal V, Huber J, et al. Human resident memory T cells exit the skin and mediate systemic Th2-driven inflammation. J Exp Med (2021) 218:e20210417. doi: 10.1084/jem.20210417
109. Divito SJ, Aasebø AT, Matos TR, Hsieh P-C, Collin M, Elco CP, et al. Peripheral host T cells survive hematopoietic stem cell transplantation and promote graft-versus-host disease. J Clin Invest (2020) 130:4624–36. doi: 10.1172/JCI129965
110. Dubouchet L, Todorov H, Seurinck R, Vallet N, Van Gassen S, Corneau A, et al. Operational tolerance after hematopoietic stem cell transplantation is characterized by distinct transcriptional, phenotypic, and metabolic signatures. Sci Transl Med (2022) 14:eabg3083. doi: 10.1126/scitranslmed.abg3083
Keywords: GvHD, history, acute, pathophysiology, milestones
Citation: Socie G and Michonneau D (2022) Milestones in acute GVHD pathophysiology. Front. Immunol. 13:1079708. doi: 10.3389/fimmu.2022.1079708
Received: 25 October 2022; Accepted: 22 November 2022;
Published: 05 December 2022.
Edited by:
José Laurent Cohen, Université Paris-Est Créteil Val de Marne, FranceReviewed by:
Paul Joseph Martin, Fred Hutchinson Cancer Research Center, United StatesDaniel Peltier, Michigan Medicine, University of Michigan, United States
Copyright © 2022 Socie and Michonneau. This is an open-access article distributed under the terms of the Creative Commons Attribution License (CC BY). The use, distribution or reproduction in other forums is permitted, provided the original author(s) and the copyright owner(s) are credited and that the original publication in this journal is cited, in accordance with accepted academic practice. No use, distribution or reproduction is permitted which does not comply with these terms.
*Correspondence: Gerard Socie, gerard.socie@aphp.fr