- 1Plant Health Theme, International Centre of Insect Physiology and Ecology (icipe), Nairobi, Kenya
- 2Department of Zoology and Entomology, University of Pretoria, Hatfield, South Africa
- 3Forestry and Agricultural Biotechnology Institute (FABI), University of Pretoria, Hatfield, South Africa
The efficiency of an autodissemination technique in controlling adult whiteflies, Trialeurodes vaporariorum Westwood (Hemiptera: Aleyrodidae) on tomato, Solunum lycopersicum was investigated with previously identified potent fungal isolates of Metarhizium anisopliae ICIPE 18, ICIPE 62 and ICIPE 69 under screenhouse or semi-field conditions. The autodissemination device was inoculated with dry conidia of the M. anisopliae isolates, while control insects were exposed to a fungus–free device. Sampling for conidia uptake, conidial viability and persistence, and insect mortality was done at 1, 2, 3, 5 and 8 days post–exposure, and collected insects were monitored for mortality over ten days. Overall, mortality was higher in insects exposed to ICIPE 18 (62.8%) and ICIPE 69 (61.8%) than in those exposed to ICIPE 62 (42.6%), with median lethal times, (LT50) ranging between 6.73–8.54 days. The control group recorded the lowest mortality rates (18.9%). A general linear reduction in conidial viability with exposure time was observed, although this was more pronounced with M. anisopliae ICIPE 62. Insects exposed to M. anisopliae ICIPE 69 also recorded the highest conidia uptake, hence selected for further evaluation with a T. vaporariorum attractant volatile organic compound, (E)–2–hexenal. The volatile inhibited fungal germination in laboratory compatibility tests, therefore, spatial separation of M. anisopliae ICIPE 69 and (E)–2–hexenal in the autodissemination device was conducted. The inhibitory effects of the volatile were significantly reduced by spatial separation at a distance of 5 cm between the fungus and the volatile, which was found to be more suitable and chosen for the subsequent experiments. Results showed that (E)–2–hexenal did not influence conidia uptake by the insects, while fungal viability and the subsequent mortality variations were more related to duration of exposure. The fungus–volatile compatibility demonstrated with spatial separation provides a basis for the optimisation of the volatile formulation to achieve better T. vaporariorum suppression with an excellent autodissemination efficiency when used in the management of whiteflies under screenhouse conditions.
Introduction
Whiteflies (Hemiptera: Aleyrodidae) have emerged as important vectors of several plant viruses that limit crop productivity and cause substantial losses in horticultural production (1). Out of more than 1,550 species that have been described to date, the sweet–potato whitefly, Bemisia tabaci Gennadius, and the greenhouse whitefly, Trialeurodes vaporariorum Westwood, are recognised as the main vectors of viruses transmitted by whiteflies (2, 3). Trialeurodes vaporariorum is a primary pest of several horticultural crops, and a key pest of tomato, cucumber, eggplant, pumpkin and French bean (4, 5). As a polyphagous pest, when farmers practice continuous cropping with successive hosts, which is common in horticultural production systems, the T. vaporariorum population builds up rapidly, resulting in high infestations and possible outbreaks (6, 7). In addition, the polyphagous behaviour and persistent high densities predispose whiteflies to excessive applications of different classes of pesticides, resulting in pesticide resistance that makes it difficult to achieve satisfactory control with pesticides (6, 8–10). Adverse effects on both human and ecological health as a result of intensive use of synthetic pesticides against some major arthropod pest species such as whiteflies has been documented (11). These include high pesticide residues on food crops, loss of biodiversity through the elimination of natural enemies and pollinators, and pesticide resistance which subsequently leads to high production costs (12–14). In sub–Saharan Africa, significantly higher pesticide use is common in local and export market cash crops, mainly fruits and vegetables (14). Due to the growing concerns regarding the risks associated with the intensive and indiscriminate use of pesticides in crop protection, calls for the development, adoption and promotion of biological control strategies that strengthen ecological sustainability are becoming increasingly paramount.
The pathogenicity of various entomopathogenic fungi from Aschersonia, Metarhirizium, Beauveria, Isaria and Lecanicillium genera against whiteflies has been evaluated by several researchers as reviewed by Sani etal. (15). Metarhizium anisopliae (Metschnikoff) Sorokin (Hypocreales: Clavicipitaceae) is among these entomopathogenic fungi proven to cause mortality in all stages of T. vaporariorum life cycle (16, 17). However, most of the pathogenicity studies have primarily focused on the nymphal stages (9, 11, 18–21), where fungal formulations were mainly applied through leaf spraying and dipping methods (15, 21). There are very limited studies on the adult stage of T. vaporariorum where the fungus was used as dry conidia (17). In instances where pathogenicity bioassays were conducted on adults, the method of application was through foliar sprays (22, 23). Similarly, most of the commercial entomopathogenic fungal products available in the market have been formulated for inundative application (24). The majority of these formulations are wettable powders administered as foliar sprays, which remains the most common way to apply entomopathogenic fungi (25, 26). However, because of the high mobility of the pest, spraying sometimes becomes less effective as compared to combining the biopesticide with attractant in a trap through autodissemination approach.
Considering that fungal viability and persistence are crucial for the efficacy of entomopathogenic fungi (21, 27), the application method greatly influences the performance of the pathogen. Spraying poses limitations on efficacy by exposing the fungus on the foliage to abiotic factors like temperature, humidity and ultraviolet radiation, which adversely affect conidial germination, vegetative growth, virulence and pathogenicity of the fungi (28–30). In addition, given both the cryptic and flying behaviour of whiteflies, achieving effective contact by spraying is difficult (31–33). Greater efficiency with spraying may need additional considerations such as specialised sprayers that are unique to each crop’s setting (26). One of the main challenges to the adoption of biopesticides by farmers is their limitation on viability and persistence with field applications (30, 34, 35). Therefore, addressing challenges related to the application system would increase reliability for successful field applications and adoption by growers.
As highlighted by Zanchi etal. (35), adult insects have an advantage of being able to horizontally disseminate the fungus amongst themselves, hence formulating control strategies that are effective for both the adult and immature stages enhances population suppression of the pest (17). Controlling adult insects, however, requires a more targeted application system that enhances efficient delivery of the fungus to its target host. This can be achieved by using an autodissemination device where the fungus is used together with an attractant (“attract and infect/kill”); and this has previously been demonstrated for other insect species [fruit flies – Ceratitis capitata and C. rosa var. fasciventris (36); diamondback moth – Plutella xylostella (37); Western flower thrips – Frankliniella occidentalis (38); mosquitoes – Aedes aegypti (39); pea leafminer – Liriomyza huidobrensis (40); polyphagous moth – Thaumatotibia leucotreta (41) and the beet worm – Spoladea recurvalis (42)].
Research on plant volatile organic compounds in whitefly systems have established their effects on whitefly orientation responses, host seeking behaviour, predator–parasitoids interactions and stimulation of the biosynthesis of defense enzymes that induce plant systemic resistance against pests (43). Semiochemical–based approaches using attractant and repellant plant volatile organic compounds is a developing area of research that seeks to expand the non-chemical approaches that can be adapted for incorporation into various integrated pest management systems against whiteflies (13, 44, 45). From that perspective, the current study explores the application of a semiochemical and entomopathogenic fungi in an integrated biological control approach against T. vaporariorum.
Results from our previous study demonstrated the efficacy, spore acquisition and horizontal transmission of M. anisopliae ICIPE 18, ICIPE 62 and ICIPE 69 by adults of T. vaporariorum (17). The objectives of this current study were therefore to assess the compatibility between the fungal isolates and the attractant, as well as evaluate the efficiency of autodissemination device using dry conidia of the three M. anisopliae isolates, ICIPE 18, ICIPE 62 and ICIPE 69 combined with the plant volatile attractant, (E)–2–hexenal, for sustainable management of T. vaporariorum.
Materials and methods
Insects
Experiments were conducted in the laboratory and screenhouses at the International Centre of Insect Physiology and Ecology (icipe), Duduville Campus, Nairobi - Kenya (1°13ʹ14.50.ʺ S, 36°53ʹ43.823ʺ E). The whiteflies, T. vaporariorum were reared on potted tomato plants, Solanum lycopersicum L., cv. Moneymaker and kept in large Perspex cages measuring 40 cm × 60 cm × 80 cm, which were ventilated on the sides with fine netting material. Upon adult emergence, new plants were introduced weekly for oviposition, removed thereafter, and placed into a new cage where the nymphs were left to develop into adults (~ 4 weeks). After wards, a new cage was started with the newly emerged insects from the oldest cage, and new plants were introduced for oviposition. This procedure was repeated in succession for all the other cages, where newly emerged T. vaporariorum adults, ≤ 5 days old, were used for the bioassays (46).
Mass production of the fungus
Three Metarhizium anisopliae isolates, ICIPE 18, ICIPE 62 and ICIPE 69 were selected as the most potent against the adults of T. vaporariorum based on the findings of our previous study (17). The isolates obtained from the Arthropod Germplasm Centre at icipe were mass produced using whole rice as the substrate. Two kilograms of rice, in Milner bags (60 cm long × 35 cm wide) was autoclaved for 1 hour at 121°C, cooled and then inoculated with a 3–day–old culture of the fungal blastospores. The liquid culture for inoculating the rice was obtained by inoculating 50 mL sterilised liquid broth (peptone 15 g, yeast extract 30 g and glucose 30 g/L) with a loopful of spores scrapped off a 2–week–old culture previously sub–cultured on Sabouraud Dextrose Agar (SDA) and incubated in darkness at 25 ± 2°C. The broth was incubated on a rotary shaker at 100 rpm for three days. The volume of the incubated liquid culture was made up to 100 mL with sterile distilled water before inoculating the rice. The inoculated rice was incubated in a culture room for 21 days at 26 ± 1°C, 40–70% RH, and emptied into basins and left to dry for five days. Dry spores were harvested mechanically using a conidia harvester and used immediately. Viability of the fungus was confirmed through a germination test, where 100 µL of a 3 × 106 conidia/mL suspension was spread–plated on three SDA media plates and incubated for 18-20 hours at 25°C. Percentage germination was observed under four microscopic fields covered by cover slips, where 100 conidia were randomly counted under each cover slip using a compound microscope (Leica DM500) at 400× magnification. Conidia with visible germ tubes or which had twice the diameter of the conidium were recorded as viable, and the mean values of the replicates and their standard errors calculated.
The autodissemination device
The design of the autodissemination device was similar to the one described by Toledo etal. (47), with slight modifications to its dimensions and features (Figure 1A). A cylindrical container (12 cm length and 6 cm diameter) was lined with a yellow velvet material on both the inner and outer surfaces, using a contact adhesive glue. A wire ran through two holes directly opposite each other in the upper section of the device and this was used to secure a lid (12.5 cm diameter) over the cylinder. The bottom of the device was left open. Three grammes (3 g) of dry conidia were applied onto the velvet material on both sides of the device. The velvet material offers good retention capacity of the spores which adhered very well onto this material. A yellow netting, lightly dusted with the dry conidia, was then wrapped around the inoculated velvet material and fastened with pins (40). The lid was also dusted with the fungus on both sides. However, for the control treatment, a fungus-free device was used.
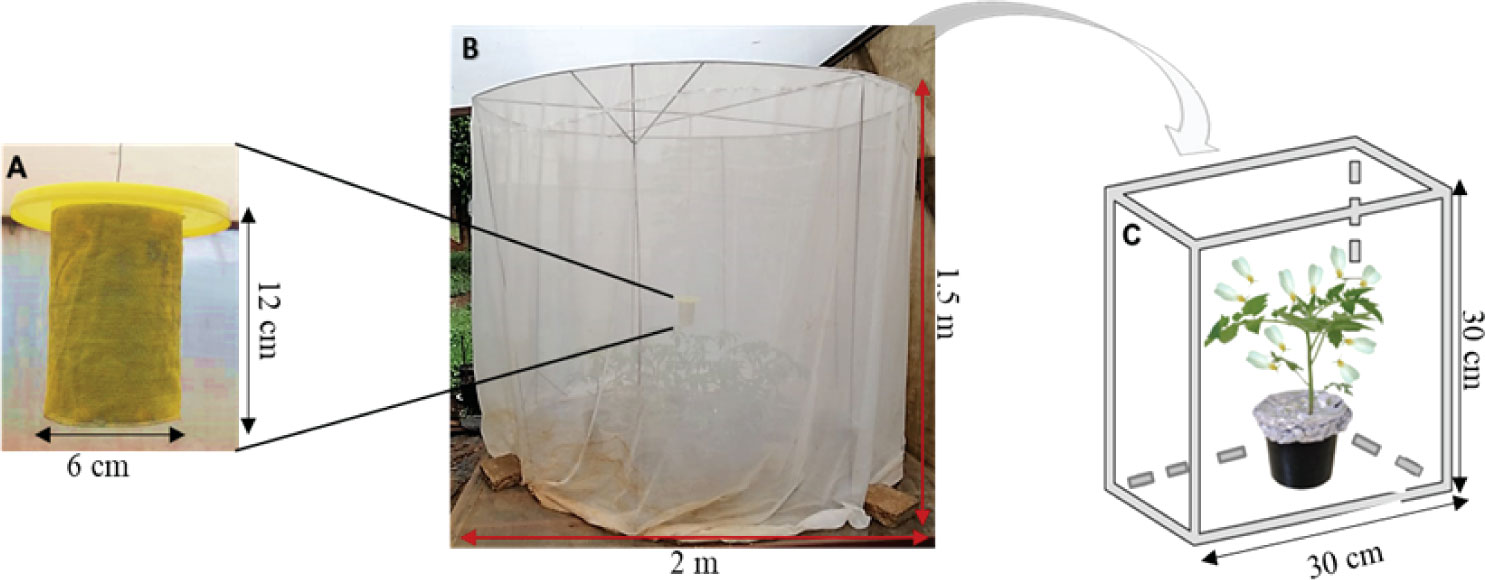
Figure 1 The autodissemination experimental set up: (A) the autodissemination device; (B) the field cage for insect exposure; (C) ventilated plexiglass cage used for mortality observation of insects sampled from the field cage after exposure.
Evaluation of the autodissemination device
The initial evaluation using only visual cues of the yellow colour of the autodissemination device was done with all the three M. anisopliae isolates, ICIPE 18, ICIPE 62 and ICIPE 69. Experiments were conducted under screenhouse conditions in field cages (1.5 m height and 2 m diameter). One caging unit was used per fungal isolate for the evaluation of the autodissemination device. Sixteen five–week–old Solanum lycopersicum L. var. Moneymaker plants were placed in a circular arrangement inside the field cage. The plants were heavily infested with approximately ten thousand newly emerged whiteflies taken from the colony. The inoculated autodissemination device was suspended in the centre-top of the cage, 30 cm above the plant canopy (48), while a fungus–free device was used for the control group.
At 1, 2, 3, 5 and 8 days post exposure to the autodissemination device in the field cage (Figure 1B), individual whiteflies were randomly sampled from the field cage using an aspirator and released onto a plant in a ventilated Plexiglas cage (30 cm × 30 cm × 30 cm) inside a screenhouse to monitor mortality for ten days (Figure 1C), with four replicates per sampling day. The dead whiteflies were surface sterilised in 1% sodium hypochlorite solution, thereafter, rinsed thrice using distilled water. Insects were placed in a Petri dish lined with a moist filter paper for mycosis examination. Percentage of the mycosis was determined by the presence/absence of fungal growth of the inoculated fungus species on the surface of the cadavers. The experiments were repeated twice for each treatment.
Assessment of conidial viability and persistence
Conidial viability and persistence were evaluated by carrying out germination tests on conidia collected from the autodissemination devices from each of the treatments at 1, 2, 3, 5 and 8 days after exposure. Four moist cotton buds were used per replicate to collect conidia from different positions of the autodissemination device. The cotton bud with the spores was placed in a universal bottle containing 10 mL of 0.05% Triton X-100 water, vortexed then spread–plated on SDA plates (38) in four replicates. The conidia germination counts were done as described for the determination of viability for the mass production of the fungus in section 2.2.
Conidia acquisition by insects
On each sampling day, ten unsexed insects were randomly picked for evaluation of conidia uptake (40). Insects were individually transferred to Eppendorf tubes containing 100µL of 0.05% Triton X-100 water and vortexed to dislodge conidia (36, 42). The concentration of the conidia was determined by counting the number of spores using an Improved Neubauer haemocytometer (VWR International, USA) as described by Inglis etal. (49).
Compatibility between the fungus and (E)–2–hexenal
The plant volatile organic compound, (E)–2–hexenal (98% purity, Sigma-Aldrich) used in this study was chosen based on results from a study conducted by Li etal. (50) which identified the volatile as a strong attractant for B. tabaci adults at 100× and 1000× dilution. For the analysis of compatibility between M. anisopliae isolates ICIPE 18, ICIPE 62 and ICIPE 69 and (E)–2–hexenal, viability of the fungus was assessed at two concentrations; 100× and 1000× dilution of (E)–2–hexenal using hexane as a solvent at three volumes; 3, 4 and 5 mL in the laboratory. Spores were filtered from a 10 mL (1 × 108 conidia/mL) fungal suspension and retained on a nitrocellulose filter membrane (diameter 47 mm, pore size 0.45 µm, Sigma Chemicals) after filtration using a vacuum filter holder (51). The nitrocellulose membranes were left to dry in a laminar flow cabinet before being transferred to a five–litre glass desiccator. For each volume and concentration, a glass vial was placed in a desiccator with five fungus treated membranes. At day 1, 2, 3, 5 and 8 post exposure, a single membrane was removed, transferred into 10 mL of sterile 0.05% Triton X-100 water and vortexed in order to dislodge conidia. A 100 µL aliquot drawn from the suspension was spread–plated on an SDA plate. Germination was examined 18-20 hours post incubation at 25 ± 2°C. Each membrane was considered a replicate for each corresponding exposure time and each treatment had three replicates/desiccator. The experiment was set up as a completely randomised design.
Spatial separation of the fungus and the volatile
The above bioassays indicated the same level of compatibility with the volatile for all the isolates at 1000× dilution, while a higher conidia uptake and mortality were recorded with M. anisopliae ICIPE 69 than the other isolates, thus it was selected for the subsequent assays with (E)–2–hexenal. Therefore, an experiment was set up to reduce the inhibitory effect on conidial germination by spatially separating the volatile and the fungus in the autodissemination device. A glass vial with (E)–2–hexenal was placed at three different positions; 0 cm (directly below the device); 5 cm and 10 cm below the autodissemination device. A device with fungus but without (E)–2–hexenal was treated as the control (42). The treatments were separated from each other by 10 m and set up in screenhouses and replicated thrice.
Evaluation of Metarhizium anisopliae ICIPE 69 with (E)–2–hexenal in the autodissemination device
Five millilitres of a 1000× dilution of (E)–2–hexenal was placed in a 8 mL glass vial (50). The vial was hung from the lid and suspended 5 cm below the autodissemination device inoculated with M. anisopliae ICIPE 69. No replacement of the volatile was done once the vial was empty. The viability/persistence of M. anisopliae ICIPE 69 was assessed at day 1, 2, 3, 5 and 8 days post–exposure, where moist cotton buds were used to collect spores from the different positions of the replicate autodissemination devices as described above. The cotton buds were then suspended in 10 mL of 0.05% Triton X-100 water and vortexed. A 100 µL aliquot of the suspension was spread–plated on an SDA plate and incubated at 25 ± 2°C for 18-20 hours. Conidial germination was determined as described in section 2.2.
Data analyses
All the conidial viability/persistence data were analysed using a logistic linear mixed model, (GLMM), with the function glmer for the binomial family, where treatments and sampling day were kept as fixed effects while subject (replicate) was treated as the random effect (42, 52). Whitefly mortality data for the different treatments were analysed using a Generalized Linear Model (GLM) assuming a binomial distribution error, and the Probit model in the MASS package was used for the estimation of median lethal time (LT50) for each sampling day. The presence/absence binary mycosis data was analysed by GLM with a binomial distribution as the response variable, while treatments and sampling day were the explanatory variables (35). Data on conidial counts based on the different sampling days were fitted into GLM using Poisson regression with treatment and sampling day being the explanatory variables. When means were significantly different among the treatments, a multiple comparison post hoc test was performed with the function emmeans at 5% significance level (53).
Comparison of treatments with and without the plant volatile were done in two ways; for individual sampling times and for the overall means across the eight days. For individual sampling times, data were first checked for normality using the Shapiro–Wilk test followed by an unpaired Student’s t-test (mortality, conidial viability, and mycosis). Where data showed a non–normal distribution (conidia uptake counts), a two-tailed Mann–Whitney U test was used (54). Data for the overall eight–day means exhibited a non–normal distribution, therefore comparisons for all the variables were analysed with a two-tailed Mann–Whitney U test. All statistical analyses were performed with R version 4.0.5 (55).
Results
Fungal viability and persistence in the autodissemination device
There was strong interaction between treatments and duration of exposure (χ2 = 141.33, df = 10, P < 0.0001) regarding conidia viability and persistence. There was a general decrease in the viability of the fungus corresponding to an increase in duration of exposure for all the M. anisopliae isolates (ICIPE 18, χ2 = 1059.4, df = 5, P < 0.0001; ICIPE 62, χ2 = 1603.3, df = 5, P < 0.0001; ICIPE 69, χ2 = 912.4, df = 5, P < 0.0001). Compared to the other sampling times, the highest decrease in fungus viability occurred 24 hours after exposure, which translated to 20.4, 36.2 and 22.3% loss in viability for M. anisopliae ICIPE 18, ICIPE 62 and ICIPE 69 respectively (Figure 2). The overall viability of M. anisopliae ICIPE 18 and M. anisopliae ICIPE 69 was similar and higher (68.2 ± 1.83 and 68.7 ± 1.56%) than M. anisopliae ICIPE 62 which significantly exhibited the lowest viability throughout the eight days evaluation period, with an overall germination mean of 53.1 ± 2.23% (Figure 2).
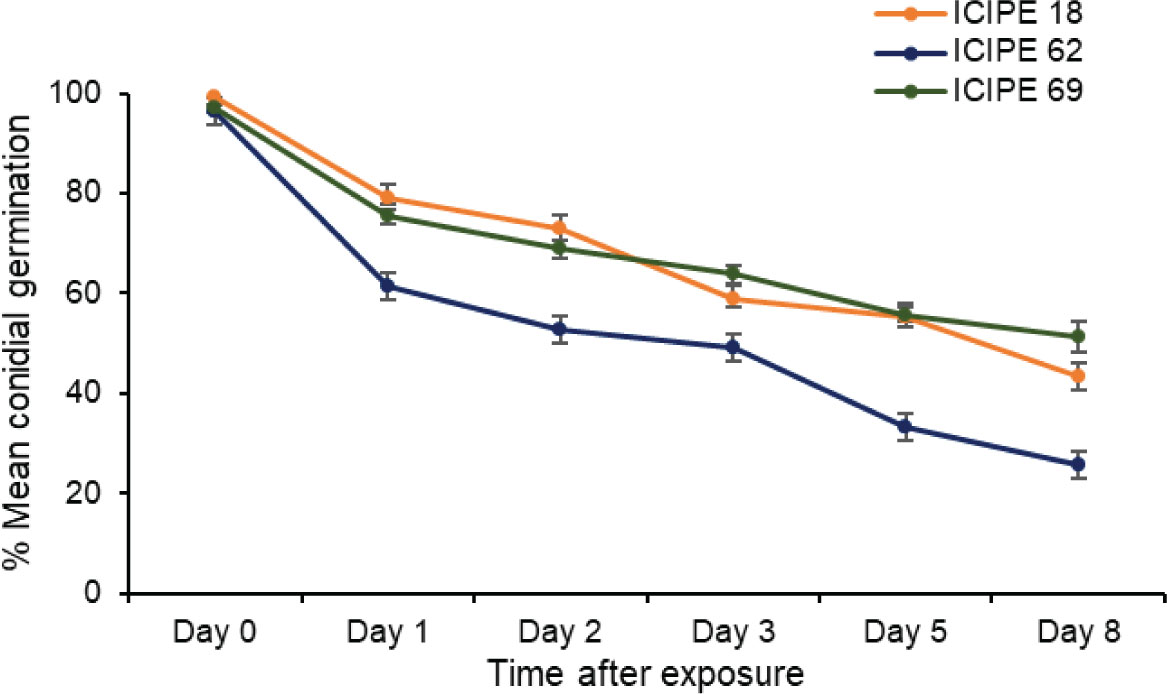
Figure 2 Mean ± standard errors conidial germination of Metarhizium anisopliae ICIPE 18, ICIPE 62 and ICIPE 69 in the autodissemination device after placement in the field cage.
Conidia acquisition after exposure to the autodissemination device
The number of conidia picked by the insects varied significantly among the three isolates (χ2 = 7.95, df = 2, P < 0.05). Other than the final day (day 8) which recorded no variations in conidia counts, conidia acquisition by insects exposed to M. anisopliae ICIPE 69 was significantly higher than those sampled from M. anisopliae ICIPE 18 and M. anisopliae ICIPE 62 inoculated autodissemination devices (Figure 3). The overall mean conidia number for the five sampling days was highest for M. anisopliae ICIPE 69 (1.37 ± 0.16 × 104 conidia/mL) and comparably similar for M. anisopliae ICIPE 18 (1 ± 0.12 × 104 conidia/mL) and M. anisopliae ICIPE 62 (0.99 ± 0.13 × 104 conidia/mL). The effect of exposure time was not significantly different for each isolate (χ2 = 3.80, df = 4, P > 0.05), with the number of fungal spores acquired by individual insects remaining generally constant throughout the sampling days for all the three M. anisopliae isolates (ICIPE 18, χ2 = 2.54, df = 4, P > 0.05; ICIPE 62, χ2 = 0.89, df = 4, P > 0.05; ICIPE 69, χ2 = 2.62, df = 4, P > 0.05) (Figure 3).
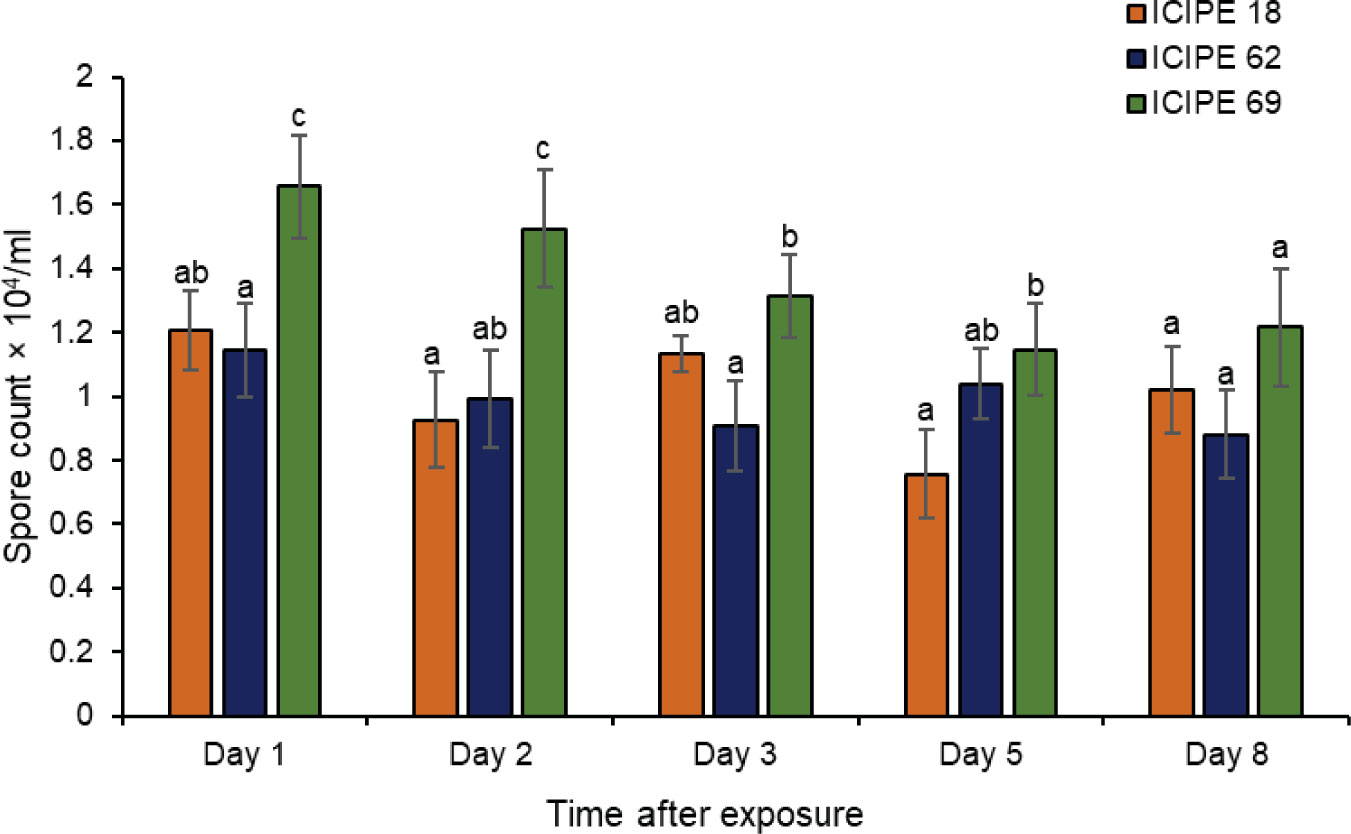
Figure 3 Mean ± standard errors number of conidia picked by individual Trialeurodes vaporariorum at different sampling intervals after exposure to autodissemination devices inoculated with Metarhizium anisopliae ICIPE 18, ICIPE 62 and ICIPE 69. Significant differences among treatments were denoted by different letters above the bars for each sampling interval (Tukey’s HSD, P < 0.05).
Insect mortality after exposure to autodissemination devices inoculated with the different Metarhizium anisopliae isolates
The exposure of insects to inoculated autodissemination devices had a significant effect on their survival or mortality rates. There was no significant interaction between the treatments and duration of exposure denoted as per sampling interval (χ2 = 492.4, df = 12, P > 0.05). However, the effects of the main independent variables were highly significant among treatments (χ2 = 3312.6, df = 3, P < 0.0001) and the duration of exposure to the fungus (χ2 = 376.4, df = 4, P < 0.01). Although an average mortality of 62.8% was recorded from M. anisopliae ICIPE 18-treated device, no significant differences (χ2 = 46.68, df = 4, P > 0.05) were observed among the various sampling intervals (Figure 4A). For the other treatments, mortality was variable across days for M. anisopliae ICIPE 62 (χ2 = 183.58, df = 4, P < 0.05), M. anisopliae ICIPE 69 (χ2 = 234.23, df = 4, P < 0.0001) and control (χ2 = 56.75, df = 4, P < 0.0001). The mortality of insects exposed to M. anisopliae ICIPE 62-treated device remained generally lower than the other two isolates for the first four sampling days with < 50%. However, for insects sampled on the eighth day post exposure, a significant increase led to a mortality of 61.3% (Figure 4B). The mortality of insects sampled from the M. anisopliae ICIPE 69-treated device showed that at day 1 and 2 post exposure, mortality was significantly lower, (49.4 and 52.1% respectively) than insects sampled at days 3, 5, and 8 (64.9–74.3%) (Figure 4C). As expected, insects in the controltreatment recorded the lowest mortality for all the sampling days (Figure 4D). In general, M. anisopliae ICIPE 18 and M. anisopliae ICIPE 69 had comparable averages for the overall mortality rates from the five sapling days (62.8 and 61.8% respectively), followed by M. anisopliae ICIPE 62 which averaged 42.6% while the control treatment had the lowest mortality rate of 18.9%.
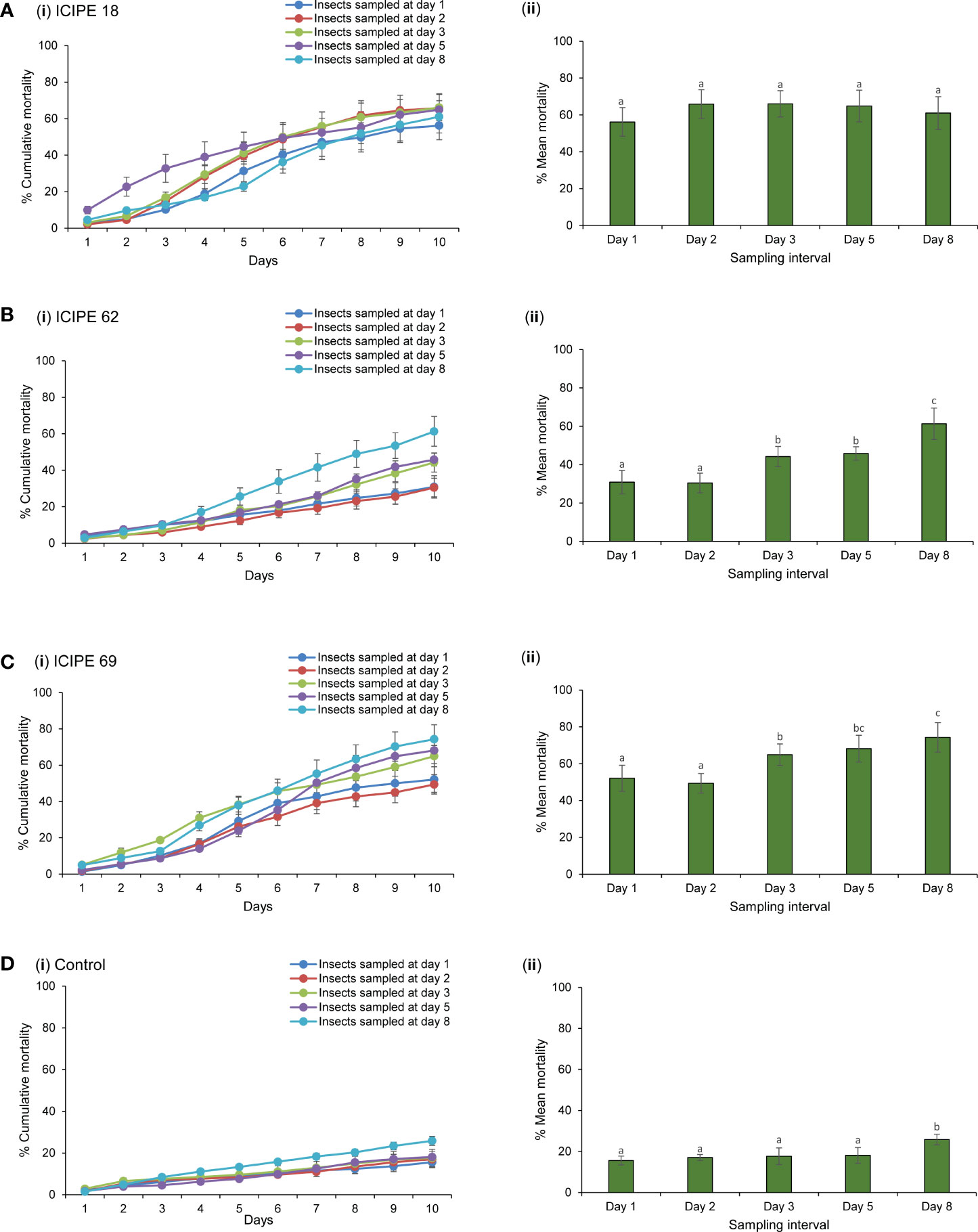
Figure 4 Mortality ± standard errors of adult Trialeurodes vaporariorum after exposure to Metarhizium anisopliae ICIPE 18, ICIPE 69, ICIPE 62 inoculated autodissemination devices and fungus-free device (control) collected on different sampling intervals. Line graphs A(і)–D(і) show the cumulative mortality across days for whiteflies sampled on different sampling intervals. Bar graphs A(іі)–D(іі) show the mean mortality for insects sampled at different time intervals and monitored for 10 days. Means followed by the same letter are not significantly different (Tukey’s HSD test, P < 0.05).
The median lethal times (LT50) were estimated for the three isolates (when mortality > 50%), where the shortest LT50 values were obtained at day 5 for M. anisopliae ICIPE 18 (6.73 ± 0.45 days) and day 8 for M. anisopliae ICIPE 69 (6.77 ± 0.21 days) (Table 1). For mycosis, sampling interval did not show any significant influence on the mycosis rates for all the isolates (χ2 = 3.07, df = 4, P > 0.05), but only treatment effects explained the differences in the level of mycosis of the cadavers (χ2 = 38.78, df = 2, P < 0.0001). There was lower incidence of mycelial growth and sporulation from the cadavers exposed to M. anisopliae ICIPE 62–treated device, in comparison to those collected from M. anisopliae ICIPE 18 and M. anisopliae ICIPE 69 cages which showed no statistical differences among the sampling intervals (Table 1). No fungal growth or sporulation was observed on the insects that were incubated from the control group.
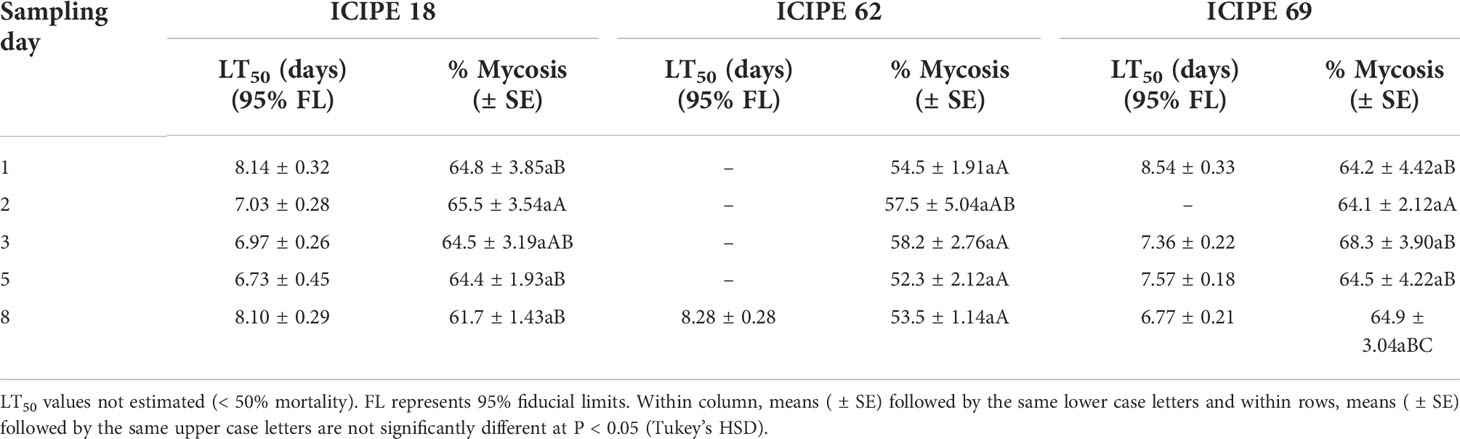
Table 1 Median lethal time (LT50) and mycosis rates of adult Trialeurodes vaporariorum collected on different sampling intervals after exposure to autodissemination devices inoculated with Metarhizium anisopliae ICIPE 18, ICIPE 62 and ICIPE 69 and monitored over ten days.
Compatibility between Metarhizium anisopliae isolates and (E)–2–hexenal in the laboratory
The laboratory compatibility experiment showed complete inhibition of fungal germination when the isolates were exposed to a 100× dilution of the volatile (E)–2–hexanal. At 1000× dilution, the volatile still exerted a pronounced inhibitory effect on conidial germination for all the three isolates compared with their respective control treatments (χ2 = 410.84, df = 11, P < 0.0001) (Figure 5). However, the amount of tested volatile caused different inhibition levels in the three isolates, with 4 mL exhibiting a stronger inhibitory effect on M. anisopliae ICIPE 18 (χ2 = 307.11, df = 2, P < 0.0001) and ICIPE 62 (χ2 = 88.07, df = 2, P < 0.001) spore germination than 3 mL and 5 mL, while the effect of volatile volume was not significant for M. anisopliae ICIPE 69 conidial germination (χ2 = 0.20, df = 2, P > 0.05). Viability of spores in the control treatments remained considerably high for all the isolates, with average conidial germination of 93.4–95.7% at 8 days post exposure, while germination counts for volatile exposed M. anisopliae treatments ranged between 45.1–59.0% (Figure 5).
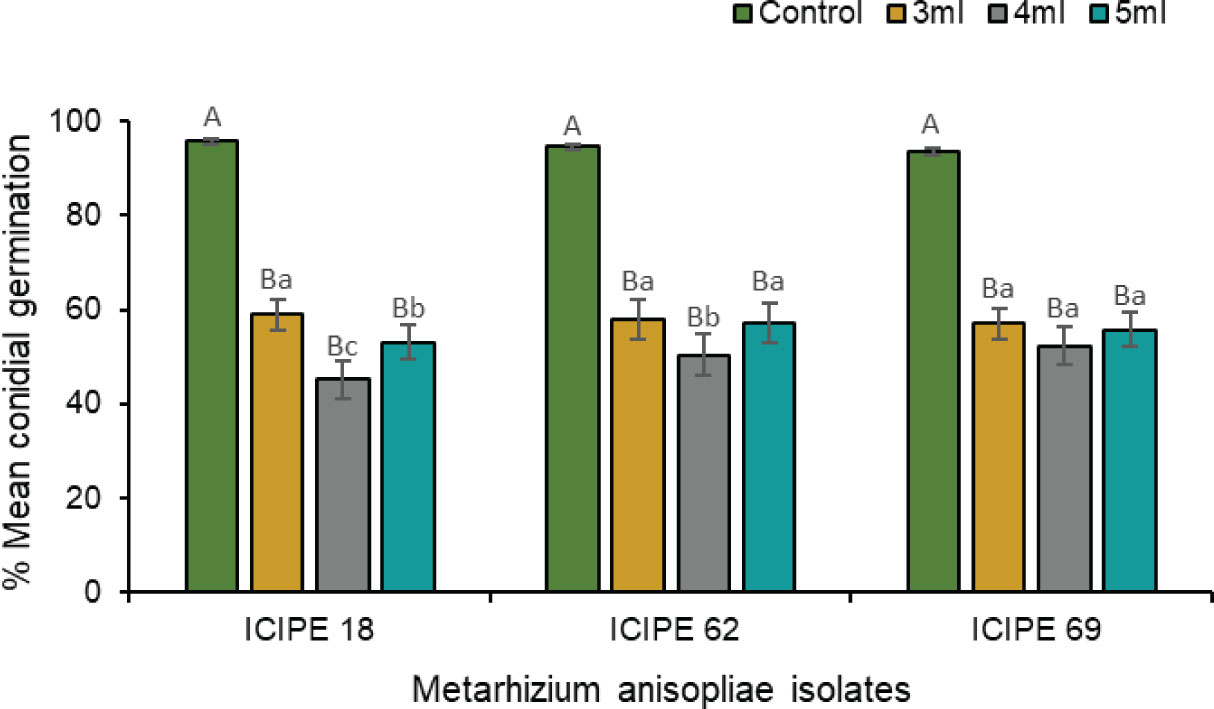
Figure 5 Effects of three volumes of 1000× dilution (E)–2–hexenal on the conidial germination of Metarhizium anisopliae ICIPE 18, ICIPE 62 and ICIPE 69 eight days post-exposure. Bars denote means ± standard errors and means followed by the same upper–case letters across treatments and lower–case letters within isolate-volume groups are not significantly different (Tukey’s HSD test, P < 0.05).
Effect of spatial separation of Metarhizium anisopliae ICIPE 69 and (E)–2–hexenal on conidial viability
The viability of the fungus was significantly affected by the interaction between separation distance and duration of exposure (χ2 = 157.46, df = 15, P < 0.0001; Table 2). There was general linear reduction in germination in all treatments with increasing duration of exposure (χ2 = 2958.1, df = 5, P < 0.0001). In addition, the separation distance had a pronounced effect on germination (χ2 = 699.0.38, df = 3, P < 0.0001). The overall viability was lower in all the treatments where the fungus was exposed to volatiles compared to the control, and the level of germination inhibition was dependent on the separation distance. The lowest germination was recorded in the treatment in which the volatile was closest to the fungus (0 cm) (51.0 ± 2.62%), and this effect was reduced with increasing separation distance (Table 2). A separation distance of 5 cm was used for evaluation with M. anisopliae ICIPE 69 since conidial viability at that distance was not significantly different, (P > 0.05), with that at 10 cm (61.3 ± 2.05 vs. 64.4 ± 1.97%) (Table 2).
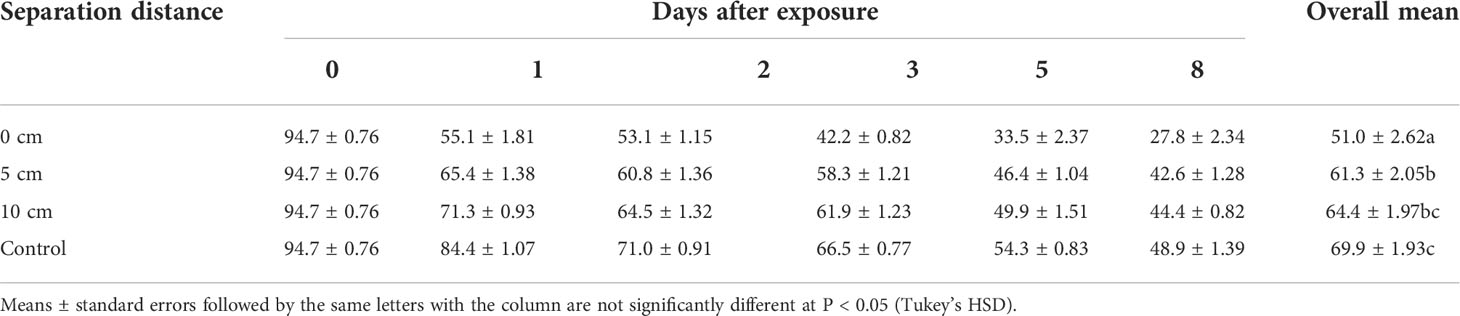
Table 2 Effect of spatial separation of (E)–2–hexenal and Metarhizium anisopliae ICIPE 69 on conidial germination in the autodissemination device.
Comparative assessment of the efficacy of Metarhizium anisopliae ICIPE 69 and (E)–2–hexenal combination
The impact of combining M. anisopliae ICIPE 69 and the volatile, (E)–2–hexenal on the various response variables, was assessed at a separation distance of 5 cm. The comparative analyses were done per sampling interval as well as on the overall means for the treatments where the autodissemination device did and did not have the volatile (Figure 6). The volatile had a significant effect on the viability of the fungus (Mann–Whitney U, W = 9231, P < 0.0001). The presence of the volatile generally lowered the viability of the fungus throughout the evaluation period (Figure 6A), leading to a significantly lower conidial germination in the treatment with the volatile (60.8 ± 1.77%) than in the volatile free device (68.6 ± 1.56%). Notably, the presence of the volatile did not affect conidia uptake by the insects over the entire sampling period and the mean spore counts for both treatments did not vary significantly (Mann–Whitney U, W = 4563.5, P > 0.05) (Figure 6B). However, despite similar conidia uptake counts by the insects from the two treatments, a significantly lower mortality was observed in insects exposed to an autodissemination device combined with the volatile than a volatile–free treatment at day 1 and day 3 post treatment (Figure 6C). The average overall mortality was 26.1% lower in the treatment with the volatile compared to (E)–2–hexenal–free treatment (Mann–Whitney U, W = 1175, P < 0.001). The shortest LT50 for both treatments were on day 8, although it was shorter for the volatile–free treatment (6.77 ± 0.21 vs. 9.05 ± 0.31 days). Similarly, the mycosis rates in insects exposed to the volatile plus the fungus were significantly lower than in volatile–free treatment, and this trend was consistent for all the days (Mann–Whitney U, W = 1462, P < 0.0001) (Figure 6D). Therefore, though considerable compatibility between the attractant and fungus was achieved through spatial separation, the application of (E)–2–hexenal still did not improve the efficacy of M. anisopliae ICIPE 69 using the autodissemination technology.
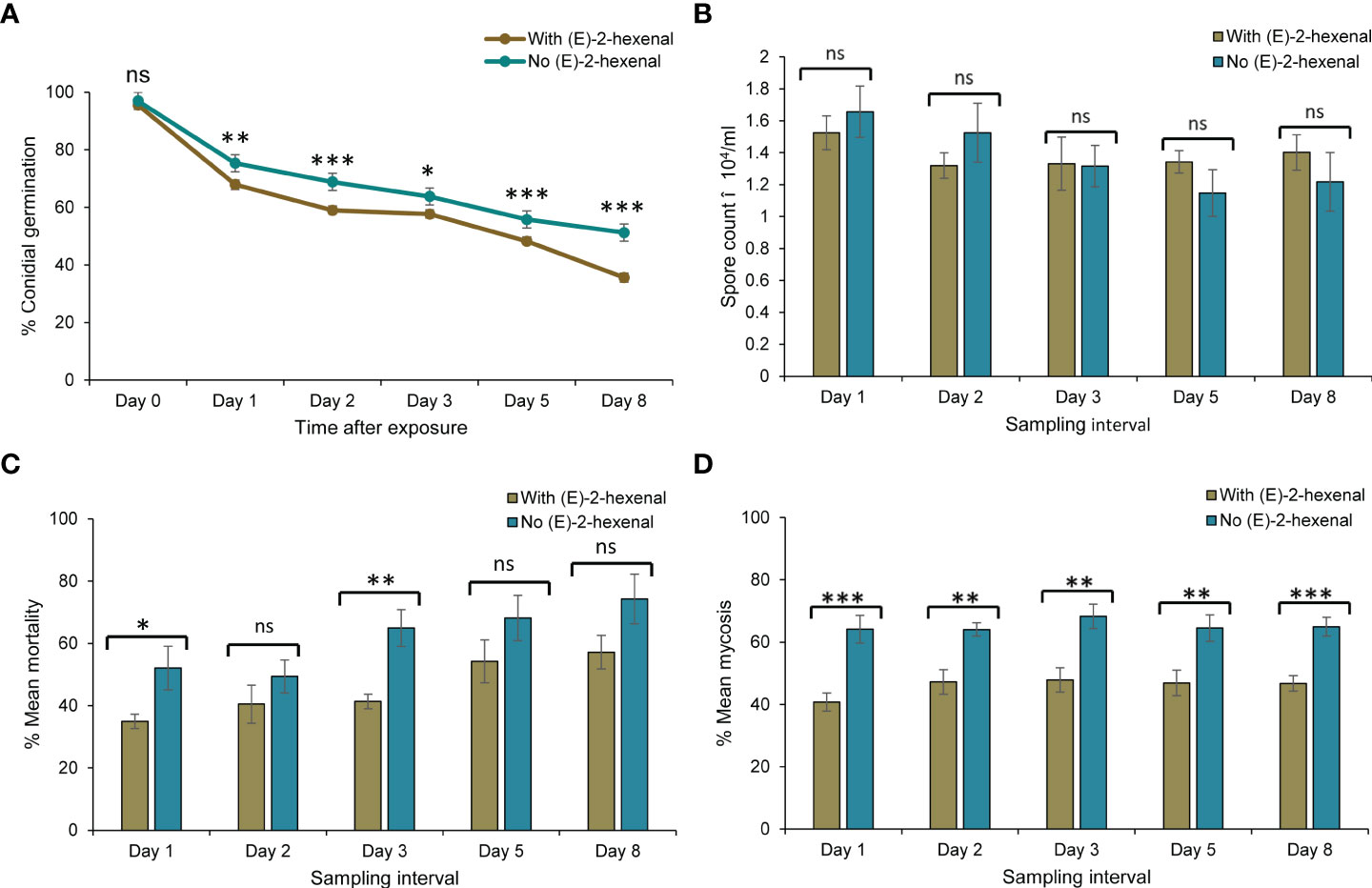
Figure 6 Comparison of response variables between autodissemination devices with and without the volatile (E)–2–hexenal, on Metarhizium anisopliae ICIPE 69 and its efficacy. Significant differences between treatments at individual sampling points are indicated on the graphs with asterisks; *P < 0.05, **P < 0.01, ***P < 0.001 and ns (non significant, P > 0.05). (A) shows conidial viability after the Student’s unpaired t-test with significant differences at Day 1 (t = 3.13, df = 37.6, P < 0.01), Day 2 (t = 4.65, df = 29.1, P < 0.001), Day 3 (t = 2.01, df = 35.0, P < 0.05), Day 5 (t = 4.35, df = 35.5, P < 0.001) and Day 8 (t = 4.64, df = 28.3, P < 0.001); (B) shows no significant treatment effect on conidia uptake by the insects on all sampling days following a two tailed Mann–Whitney U tests (P > 0.05); (C) shows significant treatment effects on insect mean mortality monitored for ten days for insects collected at Day 1 (t = -2.31, df = 8.42, P < 0.05) and Day 5 (t = -3.83, df = 9.19, P < 0.01) following a Student’s unpaired t-test; and (D) indicates significant differences observed on mycosis of the insect cadavers from the two treatments; Day 1 (t = 4.44, df = 12.0, P < 0.001), Day 2 (t = 3.75, df = 10.7, P < 0.01), Day 3 (t = 3.69, df = 14, P < 0.01), Day 5 (t = 2.99, df = 13.9, P < 0.01) and Day 8 (t = 4.65, df = 13.4, P < 0.001) after a Student’s unpaired t-test.
Discussion
The study showed the development and deployment of a simple and effective autodissemination device and its applicability as an efficient entomopathogenic fungi application method against adult whiteflies. The autodissemination device was efficient in attracting insects and allowed for conidia transfer, causing significantly higher mortality rates in the treatments in which the insects were exposed to the fungus, as compared to fungus free control insects. The findings from the study further demonstrated the influence of different factors, mainly related to pathogen virulence, viability and persistence, conidia uptake and mortality on the overall performance of the autodissemination device. However, further results showed that the presence of an attractant plant volatile (E)–2–hexenal did not enhance the performance of the autodissemination device.
An autodissemination device allows for the dispersion of conidia by the target insects (32, 56, 57). Insects are attracted towards the device (inoculated with the entomopathogenic fungus), where they pick up the conidia and subsequently spread the propagules to their conspecifics, in a “lure and infect” manner (37, 39). This delivery system presents many advantages; that includes the use of fewer conidia making it more economically viable than innundative application systems, it is simple and easy to set up and has greater persistence of the fungus as it provides some sheltering from the direct exposure to environmental conditions such as temperature/UV and rainfall (42). Additionally, high specificity is attained when the device is used in combination with an attractant lure (57–59).
The autodissemination device in the study was designed with features to enhance both visual and olfactory stimuli since they are major factors in whitefly behaviour. Visual cues in whiteflies are important in long distance responses while plant volatiles facilitate short distance orientation (60, 61). The designed autodissemination device was yellow, based on the colour preference of T. vaporariorum adults (62–64). Pinto-Zevallos and Vänninen (48) reported that using cylindrical yellow sticky traps resulted to higher whitefly catches compared to other shapes, hence the same shape was adopted. A yellow velvet material was selected for inoculation because of its high spore retention capacity (40). However, due to the darker pigmentation of the M. anisopliae conidia (dark green), a yellow netting material was used over the inoculated velvet material to bring out the desired yellow colour.
Combining the entomopathogenic fungus with a plant volatile organic compound was intended to improve the device’s efficiency by adding the odour stimuli. However, a series of optimisations were necessary since combining (E)–2–hexenal and M. anisopliae ICIPE 69 in an autodissemination device has not been reported elsewhere. Li etal. (50) showed that attraction of B. tabaci adults by (E)–2–hexenal was concentration dependant. A 100× and 1000× dilution caused 76 and 70% attraction respectively, indicating a stronger response at a higher concentration. For our bioassays, however, a working concentration of 1000× dilution was adopted following laboratory results that showed complete inhibition of conidial germination by (E)–2–hexenal at 100× dilution. Although the fungus exhibited a volume–dependant germination rate for M. anisopliae ICIPE 18 and ICIPE 62, there were no differences on the overall germination scores over eight days for M. anisopliae ICIPE 69, hence the volume that was adopted for bioassays was five millilitres. Inhibition of M. anisopliae conidial germination by (E)–2–hexenal via both direct contact and fumigation has previously been reported (65). To reduce the fungicidal effects of the volatile, spatial separation of the volatile from the fungus was initially assessed at three separation distances of 0, 5 and 10 cm. Based on the results, the evaluation of the compatibility efficacy of the fungus was then conducted using a separation distance of 5 cm since conidial viability at that distance did not differ significantly from that at 10 cm. Since the aim was to have the volatile attract insects towards the inoculated part of the autodissemination device, a shorter distance between the fungus and the volatile also presented an advantage. Although the spatial separation did not eliminate the antifungal effects of the volatile completely, there was significant improvement in fungal viability to levels comparable to the control treatment, similar to findings by Mfuti et al. (66) and Opisa etal. (42).
In consideration of results reported by Li etal. (50) that showed the efficacy of (E)–2–hexenal as a strong attractant volatile of B. tabaci, there was an expectation that combining the autodissemination device with the volatile would be more effective in attracting whiteflies, effect higher conidia uptake and lead to higher mortality rates. Contrary to this expectation, however, conidia uptake by insects in the presence or absence of the volatile was similar. It is therefore, possible that visits to the autodissemination device by the insects were predominantly influenced by visual cues, the yellow colour of the device. It has been shown that visual cues override volatile/odour cues in whitefly behavioural responses (6, 45, 67). There is also a possibility that the variation in the response to the volatile could be due to species variation, B. tabaci used by Li etal. (50) versus T. vaporariorum used in the current study. However, both species are recognised as extreme generalists, with a preference for diverse volatile blends and are capable of detecting different volatiles specific to many plants (44, 68). For this reason, we could speculate that species variation may have played a minor role.
There was marked differences in conidial viability of the three different M. anisopliae isolates. Exposure of entomopathogenic fungi to the natural environment negatively affects viability due to the differential effects of various abiotic factors. Temperature affects the germination and growth of the fungus, humidity is important for the germination rate of the fungus on the insect’s body and subsequent infection, while direct sunlight exposes the fungus to ultra-violet (UV) radicals that cause alteration in its DNA structure (29, 69). Different fungal species and isolates of the same species have been shown to have variable UV tolerance and thermotolerance levels (70), and this has also been reported for different M. anisopliae isolates (71–73). Metarhizium anisopliae ICIPE 18 and M. anisopliae ICIPE 69 exhibited better and similar environmental fitness with respect to fungal viability and persistence than M. anisopliae ICIPE 62. The resulting mortality observed with the two isolates, likewise, were higher than with M. anisopliae ICIPE 62.
Similarly, in a follow up experiment with M. anisopliae ICIPE 69 and (E)–2–hexenal, mortality was higher in a volatile–free treatment than when the autodissemination device was used in combination with the volatile at a 5 cm separation distance. Similar results were reported for bed bugs, Cimex lectularius L. (Hemiptera: Cimicidae) where lower mortality was recorded in insects treated with M. anisopliae previously exposed to (E)–2–octenal (a compound structurally related to (E)–2–hexenal), than in treatments where conidia without the aldehyde were used (65). The inhibitory effects of (E)–2–hexenal on conidial germination and vegetative growth have also been previously demonstrated by da Silva et al. (74) in M. anisopliae, B. bassiana (75) and some bacterial strains (76). The fungistatic effects of (E)–2–hexenal were reported to be a result of the reactions between the aldehyde’s highly reactive side chains with the nucleophile, sulfhydryl, amino and hydroxyl groups of the fungus through addition and condensation reactions (76). These reactions induce cell damage which impairs the functions of the membrane associated proteins and alters cell permeability resulting in reduced viability. In our current study, in both scenarios, lower mortality rates seemed related to lower fungal viability. Based on this observation, we hypothesised that the lower mortality was as a result of lower fungal viability, since only viable propagules can warrant effective infection which leads to mortality.
In reference to conidia uptake, there was a consistent presence of conidia on the insects that were randomly sampled throughout the evaluation period for all the isolates. There are different possible ways through which conidia acquisition by insects could have occurred; spore transfer following visits to the autodissemination device, horizontal transmission between contaminated and clean individuals or conidia retained by the insects after conidia acquisition. Spore retention and horizontal transmission by the adults of T. vaporariorum for up to 72 hours was reported in our previous study (17). Considering that the autodissemination device was the first source of inoculum, the evidence of the presence of conidia on the insects is important as it validates the efficiency of the device in delivering the pathogen. As pointed out by Tol etal. (77), an efficient autodissemination device allows for easy conidia acquisition by insects upon contact and the contaminated adults then disperse the infective propagules to their conspecifics (39). The relatively long median lethal times (LT50) recorded (6.73–8.54 days) still present an advantage for the role of the insects as dispersal agents; because a longer survival time for an infected insect gives ample time for horizontal transmission (47, 78).
In conclusion, our findings demonstrated the effective control of adult T. vaporariorum using autodissemination device with M. anisopliae ICIPE 69 under a screenhouse environment. Using both visual and olfactory stimuli present a good approach in devising an efficient control strategy against whiteflies. Although the results demonstrated that application of the attractant, (E)–2–hexenal, did not enhance the efficacy of M. anisopliae ICIPE 69 and improve the efficiency of the autodissemination device for the management of the adult greenhouse whitefly, the study still provides important baseline information on the volatile’s effects on both the insect and the fungus, and consequently lays a foundation for comparison with future studies. In addition, the results give a basis for the optimisation of this volatile formulation and, therefore, could guide in designing further experiments to improve the overall efficiency of an integrated system where entomopathogenic fungus and an attractant volatile can be combined (through spatial separation) to achieve better T. vaporariorum suppression. This information would also compliment ongoing research approaches that seek to exploit the practical application of semiochemicals in crop protection.
Data availability statement
The original contributions presented in the study are included in the article/Supplementary Material. Further inquiries can be directed to the corresponding author.
Author contributions
VP, FK, AY, SS and KA: conceptualisation of the research. FK, SS and KA: funding acquisition and resources. VP: investigation, statistical analyses and writing first draft. VP, FK, AY, SS and KA: validation, data interpretation and writing (reviewing and editing). All authors read and approved the submitted manuscript.
Funding
This research was funded by BioInnovate Africa Phase I project “Promoting smallholder access to fungal biopesticides through Public Private Partnerships in East Africa” (BA/CI/2017-02/PROSAFE), and UK’s Foreign, Commonwealth and Development Office (FCDO) (FCDO Biopesticide Project, B2291A - FCDO - BIOPESTICIDE) through the International Centre of Insect Physiology and Ecology (icipe). We thank the German Academic Exchange Service (DAAD) In-Region Postgraduate Scholarship for the financial assistance provided to the first author through African Regional Postgraduate Programme in Insect Science (ARPPIS). The authors gratefully acknowledge the icipe core funding provided by UK’s Foreign, Commonwealth and Development Office (FCDO); Swedish International Development Cooperation Agency (Sida); the Swiss Agency for Development and Cooperation (SDC); the Federal Democratic Republic of Ethiopia; and the Government of the Republic of Kenya.
Acknowledgments
The authors would like to thank Dr. Daisy Salifu for her statistical advice, and Sospeter Wafula, Jane Kimemia and Levi Ombura for their technical assistance.
Conflict of interest
The authors declare that the research was conducted in the absence of any commercial or financial relationships that could be construed as a potential conflict of interest.
Publisher’s note
All claims expressed in this article are solely those of the authors and do not necessarily represent those of their affiliated organizations, or those of the publisher, the editors and the reviewers. Any product that may be evaluated in this article, or claim that may be made by its manufacturer, is not guaranteed or endorsed by the publisher.
References
1. Wintermantel WM. Emergence of greenhouse whitefly (Trialeurodes vaporariorum) transmitted criniviruses as threats to vegetable and fruit production in north America (2004). APSnet Features (Accessed 19 July 2021). Available at: https://www.apsnet.org/edcenter/apsnetfeatures/Pages/GreenhouseWhitefly.aspx.
2. Can-Vargas X, Barboza N, Fuchs EJ, Hernández EJ. Spatial distribution of whitefly species (Hemiptera: Aleyrodidae) and identification of secondary bacterial endosymbionts in tomato fields in Costa Rica. J Econ Entomol (2020) 113:2900–10. doi: 10.1093/jee/toaa215
3. Fiallo-Olivé E, Pan L-L, Liu S-S, Navas-Castillo J. Transmission of begomoviruses and other whitefly-borne viruses: Dependence on the vector species. Phytopathology (2020) 110:10–7. doi: 10.1094/PHYTO-07-19-0273-FI
4. Campos OR, Crocomo WB, Labinas AM. Comparative biology of the whitefly Trialeurodes vaporariorum (West.) (Hemiptera - homoptera: Aleyrodidae) on soybean and bean cultivars. Neotrop. Entomol (2003) 32:133–8. doi: 10.1590/S1519-566X2003000100020
5. Sood AK, Sood S, Devi A. Morphometrics and annual life cycle of greenhouse whitefly [Trialeurodes vaporariorum (Westwood)] in Himachal Pradesh. Himachal J Agric Res (2017) 40:50–7.
6. Albajes R, Gullino ML, Van Lenteren JC, Elad Y. Integrated pest and disease management in greenhouse crops. Netherlands: Springer (1999). doi: 10.1007/978-3-030-22304-5
7. Gamarra H, Sporleder M, Carhuapoma P, Kroschel J, Kreuze J. A temperature-dependent phenology model for the greenhouse whitefly Trialeurodes vaporariorum (Hemiptera: Aleyrodidae). Virus Res (2020) 289:198107. doi: 10.1016/j.virusres.2020.198107
8. Pappas ML, Migkou F, Broufas GD. Incidence of resistance to neonicotinoid insecticides in greenhouse populations of the whitefly, Trialeurodes vaporariorum (Hemiptera: Aleyrodidae) from Greece. Appl Entomol Zool (2013) 48:373–8. doi: 10.1007/s13355-013-0197-z
9. Pineda S, Alatorre R, Schneider M, Martinez A-M. Pathogenicity of two entomopathogenic fungi on Trialeurodes vaporariorum and field evaluation of a Paecilomyces fumosoroseus isolate. Southwest Entomol (2007) 32:43–52. doi: 10.3958/0147-1724-32.1.43
10. Reid MC, McKenzie FE. The contribution of agricultural insecticide use to increasing insecticide resistance in African malaria vectors. Malar J (2016) 15:1–8. doi: 10.1186/s12936-016-1162-4
11. Silva J, do N, Mascarin GM, de Paula Vieira de Castro, Castilho LR, Freire DM. Novel combination of a biosurfactant with entomopathogenic fungi enhances efficacy against Bemisia whitefly. Pest Manage Sci (2019) 75:2882–91. doi: 10.1002/ps.5458
12. Bale JS, van Lenteren JC, Bigler F. Biological control and sustainable food production. Philos Trans R Soc B Biol Sci (2008) 363:761–76. doi: 10.1098/rstb.2007.2182
13. Conboy NJA, McDaniel T, George D, Ormerod A, Edwards M, Donohoe P, et al. Volatile organic compounds as insect repellents and plant elicitors: An integrated pest management (IPM) strategy for glasshouse whitefly (Trialeurodes vaporariorum). J Chem Ecol (2020) 46:1090–104. doi: 10.1007/s10886-020-01229-8
14. De Bon H, Huat J, Parrot L, Sinzogan A, Martin T, Malézieux E, et al. Pesticide risks from fruit and vegetable pest management by small farmers in sub-Saharan africa. a review. Agron Sustain Dev (2014) 34:723–36. doi: 10.1007/s13593-014-0216-7
15. Sani I, Ismail SI, Abdullah S, Jalinas J, Jamian S, Saad N. A review of the biology and control of whitefly, Bemisia tabaci (Hemiptera: Aleyrodidae), with special reference to biological control using entomopathogenic fungi. Insects (2020) 11:619. doi: 10.3390/insects11090619
16. Malsam O, Kilian M, Oerke E-C, Dehne H-W. Oils for increased efficacy of Metarhizium anisopliae to control whiteflies. Biocontrol Sci Technol (2002) 12:337–48. doi: 10.1080/09583150220128121
17. Paradza VM, Khamis FM, Yusuf AA, Subramanian S, Akutse KS. Virulence and horizontal transmission of Metarhizium anisopliae by the adults of the greenhouse whitefly, Trialeurodes vaporariorum (Hemiptera: Aleyrodidae) and the efficacy of oil formulations against its nymphs. Heliyon (2021) 7:e08277. doi: 10.1016/j.heliyon.2021.e08277
18. Malekan N, Hatami B, Ebadi R, Akhavan A, Abdul AB. Effect of entomopathogenic fungi Beauveria bassiana (bals.) and Lecanicillium muscarium (petch) on Trialeurodes vaporariorum Westwood. Indian J Entomol (2013) 75:95–8.
19. Negasi A, Parker BL, Brownbridge M. Screening and bioassay of entomopathogenic fungi for the control of silverleaf whitefly, bemisia argentifolli. Int J Trop Insect Sci (1998) 18:37–44. doi: 10.1017/S174275840000744X
20. Scorsetti AC, Humber RA, De Gregorio C, López Lastra CC. New records of entomopathogenic fungi infecting Bemisia tabaci and Trialeurodes vaporariorum, pests of horticultural crops, in Argentina. BioControl (2007) 53:787. doi: 10.1007/s10526-007-9118-9
21. Spence EL, Chandler D, Edgington S, Berry SD, Martin G, O’Sullivan C, et al. A standardised bioassay method using a bench-top spray tower to evaluate entomopathogenic fungi for control of the greenhouse whitefly, Trialeurodes vaporariorum. Pest Manage Sci (2020) 76:2513–24. doi: 10.1002/ps.5794
22. Aiuchi D, Moyo D, Ishikura S, Tani M, Kinoshita R, Rakotondrafara AM, et al. Virulence of lecanicillium spp. (Hypocreales: Cordycipitaceae) hybrid strains against various biological stages of the greenhouse whitefly, Trialeurodes vaporariorum (Hemiptera: Aleyroidae). Biocontrol Sci Technol (2020) 30:1006–17. doi: 10.1080/09583157.2020.1771281
23. Boopathi T, Karuppuchamy P, Singh SB, Kalyanasundaram M, Mohankumar S, Ravi M. Microbial control of the invasive spiraling whitefly on cassava with entomopathogenic fungi. Braz J Microbiol (2015) 46:1077–85. doi: 10.1590/S1517-838246420141067
24. Sánchez-Peña SR, Lara JS-J, Medina RF. Occurrence of entomopathogenic fungi from agricultural and natural ecosystems in saltillo, méxico, and their virulence towards thrips and whiteflies. J Insect Sci (2011) 11:1. doi: 10.1673/031.011.0101
25. Maina U, Galadima I, Gambo F, Zakaria D. A review on the use of entomopathogenic fungi in the management of insect pests of field crops. J Entomol Zool Stud (2018) 6:27–32.
26. Skinner M, Parker BL, Kim JS. Role of entomopathogenic fungi in integrated pest management. In: Integrated pest management. New York: Academic Press (2014). p. 169–91. doi: 10.1016/B978-0-12-398529-3.00011-7
27. Kirubakaran SA, Sathish-Narayanan S, Revathi K, Chandrasekaran R, Senthil-Nathan S. Effect of oil-formulated Metarhizium anisopliae and Beauveria bassiana against the rice leaffolder Cnaphalocrocis medinalis guenée (Lepidoptera: Pyralidae). Arch Phytopathol Plant Prot (2014) 47:977–92. doi: 10.1080/03235408.2013.828388
28. Daoust RA, Pereirn RM. Stability of entomopathogenic fungi Beauveria bassiana and Metarhizium anisopliae on beetle-attracting tubers and cowpea foliage in Brazil. Environ Entomol (1986) 15:1237–43. doi: 10.1093/ee/15.6.1237
29. Ignoffo CM. Environmental factors affecting persistence of entomopathogens. Fla. Entomol (1992) 75:516–25. doi: 10.2307/3496133
30. Rai D, Updhyay V, Mehra P, Rana M, Pandey AK. Potential of entomopathogenic fungi as biopesticides. Indian J Sci Res Technol (2014) 2:7–13.
31. Bleeker PM, Diergaarde PJ, Ament K, Guerra J, Weidner M, Schütz S, et al. The role of specific tomato volatiles in tomato-whitefly interaction. Plant Physiol (2009) 151:925–35. doi: 10.1104/pp.109.142661
32. Gonzalez F, Tkaczuk C, Dinu MM, Fiedler Ż., Vidal S, Zchori-Fein E, et al. New opportunities for the integration of microorganisms into biological pest control systems in greenhouse crops. J Pest Sci (2016) 89:295–311. doi: 10.1007/s10340-016-0751-x
33. Wraight SP, Ramos ME. Effects of inoculation method on efficacy of wettable powder and oil dispersion formulations of Beauveria bassiana against Colorado potato beetle larvae under low-humidity conditions. Biocontrol Sci Technol (2017) 27:348–63. doi: 10.1080/09583157.2017.1291904
34. Constantine KL, Kansiime MK, Mugambi I, Nunda W, Chacha D, Rware H, et al. Why don’t smallholder farmers in Kenya use more biopesticides? Pest Manage Sci (2020) 76:3615–25. doi: 10.1002/ps.5896
35. Zanchi C, Lo LK, Reshma R, Moritz I, Kurtz J, Müller C. Survival of the sawfly Athalia rosae upon infection by an entomopathogenic fungus and in relation to clerodanoid uptake. Front Physiol (2021) 12:637617. doi: 10.3389/fphys.2021.637617
36. Dimbi S, Maniania NK, Lux SA, Ekesi S, Mueke JK. Pathogenicity of Metarhizium anisopliae (Metsch.) sorokin and Beauveria bassiana (Balsamo) vuillemin, to three adult fruit fly species: Ceratitis capitata (Weidemann), C. rosa var. fasciventris karsch and C. cosyra (Walker) (Diptera:Tephritidae). Mycopathologia (2003) 156:375–82. doi: 10.1023/B:MYCO.0000003579.48647.16
37. Vega FE, Dowd PF, Lacey LA, Pell JK, Jackson DM, Klein MG. Dissemination of beneficial microbial agent by insects. In: Lacey LA, Kaya HK, editors. Field manual of techniques in invertebrate pathology: Application and evaluation of pathogens for control of insects and other invertebrate pests. The Netherlands: Kluwer Academic Publishers (2007). p. 127–46. doi: 10.1007/978-94-017-1547-8_6
38. Niassy S, Maniania NK, Subramanian S, Gitonga LM, Ekesi S. Performance of a semiochemical-baited autoinoculation device treated with Metarhizium anisopliae for control of Frankliniella occidentalis on French bean in field cages: Use of autoinoculation device for thrips control. Entomol Exp Appl (2012) 142:97–103. doi: 10.1111/j.1570-7458.2011.01203.x
39. Snetselaar J, Andriessen R, Suer RA, Osinga AJ, Knols BG, Farenhorst M. Development and evaluation of a novel contamination device that targets multiple life-stages of Aedes aegypti. Parasitol Vectors (2014) 7:200. doi: 10.1186/1756-3305-7-200
40. Migiro LN, Maniania NK, Chabi-Olaye A, Vandenberg J. Pathogenicity of entomopathogenic fungi Metarhizium anisopliae and Beauveria bassiana (Hypocreales: Clavicipitaceae) isolates to the adult pea leafminer (Diptera: Agromyzidae) and prospects of an autoinoculation device for infection in the field. Environ Entomol (2010) 39:468–75. doi: 10.1603/EN09359
41. Mkiga AM, Mohamed SA, du Plessis H, Khamis FM, Akutse KS, Ekesi S. Metarhizium anisopliae and Beauveria bassiana: Pathogenicity, horizontal transmission, and their effects on reproductive potential of Thaumatotibia leucotreta (Lepidoptera: Tortricidae). J Econ Entomol (2020) 113:660–8. doi: 10.1093/jee/toz342
42. Opisa S, du Plessis H, Akutse KS, Fiaboe KKM, Ekesi S. Horizontal transmission of Metarhizium anisopliae between Spoladea recurvalis (Lepidoptera: Crambidae) adults and compatibility of the fungus with the attractant phenylacetaldehyde. Microb Pathog (2019) 131:197–204. doi: 10.1016/j.micpath.2019.04.010
43. Ángeles López YI, Martínez-Gallardo NA, Ramírez-Romero R, López MG, Sánchez-Hernández C, Délano-Frier JP. Cross-kingdom effects of plant-plant signaling via volatile organic compounds emitted by tomato (Solanum lycopersicum) plants infested by the greenhouse whitefly (Trialeurodes vaporariorum). J Chem Ecol (2012) 38:1376–86. doi: 10.1007/s10886-012-0201-z
44. Darshanee HLC, Ren H, Ahmed N, Zhang Z-F, Liu Y-H, Liu T-X. Volatile-mediated attraction of greenhouse whitefly Trialeurodes vaporariorum to tomato and eggplant. Front Plant Sci (2017) 8:1285. doi: 10.3389/fpls.2017.01285
45. Togni PHB, Laumann RA, Medeiros MA, Sujii ER. Odour masking of tomato volatiles by coriander volatiles in host plant selection of Bemisia tabaci biotype b. Entomol Exp Appl (2010) 136:164–73. doi: 10.1111/j.1570-7458.2010.01010.x
46. Pakkianathan BC, Kontsedalov S, Lebedev G, Mahadav A, Zeidan M, Czosnek H, et al. Replication of tomato yellow leaf curl virus in its whitefly vector, bemisia tabaci. J Virol (2015) 89:9791–803. doi: 10.1128/JVI.00779-15
47. Toledo J, Flores S, Campos S, Villaseñor A, Enkerlin W, Liedo P, et al. Pathogenicity of three formulations of Beauveria bassiana and efficacy of autoinoculation devices and sterile fruit fly males for dissemination of conidia for the control of Ceratitis capitata. Entomol Exp Appl (2017) 164:340–9. doi: 10.1111/eea.12608
48. Pinto-Zevallos DM, Vänninen I. Yellow sticky traps for decision-making in whitefly management: What has been achieved? Crop Prot (2013) 47:74–84. doi: 10.1016/j.cropro.2013.01.009
49. Inglis GD, Enkerli J, Goettel MS. Laboratory techniques used for entomopathogenic fungi: Hypocreales. In: Manual of techniques in invertebrate pathology. Amsterdam: Academic Press (2012). p. 18–53.
50. Li Y, Zhong S, Qin Y, Zhang S, Gao Z, Dang Z, et al. Identification of plant chemicals attracting and repelling whiteflies. Arthropod-Plant Interact (2014) 8:183–90. doi: 10.1007/s11829-014-9302-7
51. Maniania NK. A laboratory technique for infecting adult tsetse with a fungal pathogen. Int J Trop Insect Sci (1994) 15:421–6. doi: 10.1017/S1742758400015769
52. Sigala JA, Oliet JA, Uscola M. Assessing growth, frost tolerance, and acclimation of pine seedlings with contrasted dormancy strategies as influenced by organic nitrogen supply. Physiol Plant (2021) 173:1105–19. doi: 10.1111/ppl.13506
53. Chaieb G, Wang X, Abdelly C, Michalet R. Shift from short-term competition to facilitation with drought stress is due to a decrease in long-term facilitation. Oikos (2021) 130:29–40. doi: 10.1111/oik.07528
54. Herren JK, Mbaisi L, Mararo E, Makhulu EE, Mobegi VA, Butungi H, et al. A microsporidian impairs Plasmodium falciparum transmission in Anopheles arabiensis mosquitoes. Nat Commun (2020) 11:2187. doi: 10.1038/s41467-020-16121-y
55. R Core Team. R: A language and environment for statistical computing. Vienna, Austria: R Foundation for Statistical Computing (2021). Available at: https://www.R-project.org/.
56. Inglis GD, Goettel MS, Butt TM, Strasser H. Use of hyphomycetous fungi for managing insect pests. In: Butt TM, Jackson C, Magan N, editors. Fungi as biocontrol agents: Progress, problems and potential. Wallingford: CABI (2001). p. 23–69. doi: 10.1079/9780851993560.0023
57. Baverstock J, Roy HE, Pell JK. Entomopathogenic fungi and insect behaviour: From unsuspecting hosts to targeted vectors. BioControl (2010) 55:89–102. doi: 10.1007/s10526-009-9238-5
58. Navarro-Llopis V, Ayala I, Sanchis J, Primo J, Moya P. Field efficacy of a Metarhizium anisopliae -based attractant–contaminant device to control Ceratitis capitata (Diptera: Tephritidae). J Econ Entomol (2015) 108:1570–8. doi: 10.1093/jee/tov157
59. Reddy GVP. Integrated management of insect pests on canola and other brassica oilseed crops. UK: CABI (2017) p. 193–221.
60. Moreau TL, Isman MB. Trapping whiteflies? a comparison of greenhouse whitefly (Trialeurodes vaporariorum) responses to trap crops and yellow sticky traps. Pest Manage Sci (2011) 67:408–13. doi: 10.1002/ps.2078
61. Zhang J, Li H, Liu M, Zhang H, Sun H, Wang H, et al. A greenhouse test to explore and evaluate light-emitting diode (LED) insect traps in the monitoring and control of Trialeurodes vaporariorum. Insects (2020) 11:94. doi: 10.3390/insects11020094
62. Gillespie DR, Quiring D. Yellow sticky traps for detecting and monitoring greenhouse whitefly (Homoptera: Aleyrodidae) adults on greenhouse tomato crops. J Econ Entomol (1987) 80:675–9. doi: 10.1093/jee/80.3.675
63. Sampson C, Covaci AD, Hamilton JGC, Hassan N, Al-Zaidi S, Kirk WDJ. Reduced translucency and the addition of black patterns increase the catch of the greenhouse whitefly, Trialeurodes vaporariorum, on yellow sticky traps. PloS One (2018) 13:e0193064. doi: 10.1371/journal.pone.0193064
64. van Lenteren J, Noldus L. Whitefly-plant relationships: Behavioural and ecological aspects. In: Gerling D, editor. Whiteflies: Their bionomics, pest status and management. UK: Intercept (1990). p. 47–89.
65. Ulrich KR, Feldlaufer MF, Kramer M, St. Leger RJ. Inhibition of the entomopathogenic fungus Metarhizium anisopliae sensu lato in vitro by the bed bug defensive secretions (E)-2-hexenal and (E)-2-octenal. BioControl (2015) 60:517–26. doi: 10.1007/s10526-015-9667-2
66. Mfuti DK, Subramanian S, van Tol RW, Wiegers GL, de Kogel WJ, Niassy S, et al. Spatial separation of semiochemical lurem-TR and entomopathogenic fungi to enhance their compatibility and infectivity in an autoinoculation system for thrips management. Pest Manage Sci (2016) 72:131–9. doi: 10.1002/ps.3979
67. Johnston N, Martini X. The influence of visual and olfactory cues in host selection for Bemisia tabaci biotype b in the presence or absence of tomato yellow leaf curl virus. Insects (2020) 11:115. doi: 10.3390/insects11020115
68. Schlaeger S, Pickett JA, Birkett MA. Prospects for management of whitefly using plant semiochemicals, compared with related pests. Pest Manage Sci (2018) 74:2405–11. doi: 10.1002/ps.5058
69. Zimmermann G. Review on safety of the entomopathogenic fungus Metarhizium anisopliae. Biocontrol Sci Technol (2007) 17:879–920. doi: 10.1080/09583150701593963
70. Lee WW, Shin TY, Bae SM, Woo SD. Screening and evaluation of entomopathogenic fungi against the green peach aphid, Myzus persicae, using multiple tools. J Asia-Pac. Entomol (2015) 18:607–15. doi: 10.1016/j.aspen.2015.07.012
71. Agbessenou A, Akutse KS, Yusuf AA, Wekesa SW, Khamis FM. Temperature-dependent modelling and spatial prediction reveal suitable geographical areas for deployment of two Metarhizium anisopliae isolates for Tuta absoluta management. Sci Rep (2021) 11:1–16. doi: 10.1038/s41598-021-02718-w
72. Mesquita E, Marciano AF, Corval ARC, Fiorotti J, Corrêa TA, Quinelato S, et al. Efficacy of a native isolate of the entomopathogenic fungus Metarhizium anisopliae against larval tick outbreaks under semifield conditions. BioControl (2020) 65:353–62. doi: 10.1007/s10526-020-10006-1
73. Rangel DEN, Braga GUL, Anderson AJ, Roberts DW. Influence of growth environment on tolerance to UV-b radiation, germination speed, and morphology of Metarhizium anisopliae var. Acridum conidia. J Invertebr. Pathol (2005) 90:55–8. doi: 10.1016/j.jip.2005.05.005
74. da Silva RA, Quintela ED, Mascarin GM, Pedrini N, Lião LM, Ferri PH. Unveiling chemical defense in the rice stalk stink bug against the entomopathogenic fungus Metarhizium anisopliae. J Invertebr. Pathol (2015) 127:93–100. doi: 10.1016/j.jip.2015.03.009
75. Ugine TA, Thaler JS. Insect predator odors protect herbivore from fungal infection. Biol Control (2020) 143:104186. doi: 10.1016/j.biocontrol.2020.104186
76. Bisignano G, Laganà MG, Trombetta D, Arena S, Nostro A, Uccella N, et al. In vitro antibacterial activity of some aliphatic aldehydes from Olea europaea l. FEMS Microbiol Lett (2001) 198:9–13. doi: 10.1111/j.1574-6968.2001.tb10611.x
77. Tol RWHM, Davidson MM, Butler RC, Teulon DAJ, Kogel WJ. Visually and olfactorily enhanced attractive devices for thrips management. Entomol Exp Appl (2020) 168:665–77. doi: 10.1111/eea.12969
Keywords: autodissemination device, entomopathogenic fungi, fungus–volatile compatibility, lure and infect, biopesticide
Citation: Paradza VM, Khamis FM, Yusuf AA, Subramanian S and Akutse KS (2022) Efficacy of Metarhizium anisopliae and (E)–2–hexenal combination using autodissemination technology for the management of the adult greenhouse whitefly, Trialeurodes vaporariorum Westwood (Hemiptera: Aleyrodidae). Front. Insect Sci. 2:991336. doi: 10.3389/finsc.2022.991336
Received: 11 July 2022; Accepted: 24 August 2022;
Published: 12 September 2022.
Edited by:
Arash Rashed, Virginia Tech, United StatesReviewed by:
Shaohui Wu, The University of Georgia, United StatesAi Xia, Nanjing Agricultural University, China
Copyright © 2022 Paradza, Khamis, Yusuf, Subramanian and Akutse. This is an open-access article distributed under the terms of the Creative Commons Attribution License (CC BY). The use, distribution or reproduction in other forums is permitted, provided the original author(s) and the copyright owner(s) are credited and that the original publication in this journal is cited, in accordance with accepted academic practice. No use, distribution or reproduction is permitted which does not comply with these terms.
*Correspondence: Komivi S. Akutse, a2FrdXRzZUBpY2lwZS5vcmc=