- 1School of Plant Sciences, The University of Arizona, Tucson, AZ, United States
- 2United States Department of Agriculture-Agricultural Research Service (USDA-ARS), Yakima Agricultural Research Laboratory (YARL), Wapato, WA, United States
- 3School of Plant Sciences University of Arizona, Tucson, AZ, United States
Introduction: The potato psyllid Bactericera cockerelli is the insect vector of the fastidious bacterium ‘Candidatus Liberibacter solanacearum’. The bacterium infects both B. cockerelli and plant species, causing zebra chip (ZC) disease of potato and vein-greening disease of tomato. Temperatures are known to influence the initiation and progression of disease symptom in the host plant, and seasonal transitions from moderate to high temperatures trigger psyllid dispersal migration to facilitate survival.
Methods: ‘Ca. L. solanacearum’ -infected and uninfected psyllids were reared at previously established ‘permissible’, optimal, and ‘non-permissible’ and temperatures of 18°C, 24°C, and 30°C, respectively. Gene expression profiles for ‘Ca. L. solanacearum’-infected and -uninfected adult psyllids reared at different temperatures were characterized by Illumina RNA-Seq analysis. Bacterial genome copy number was quantified by real-time quantitative-PCR (qPCR) amplification.
Results: Relative gene expression profiles varied in psyllids reared at the three experimental temperatures. Psyllids reared at 18°C and 30°C exhibited greater fold-change increased expression of stress- and ‘Ca. L. solanacearum’ invasion-related proteins. Quantification by qPCR of bacterial genome copy number revealed that ‘Ca. L. solanacearum’ accumulation was significantly lower in psyllids reared at 18°C and 30°C, compared to 24°C.
Discussion: Temperature is a key factor in the life history of potato psyllid and multiplication/accumulation of ‘Ca. L. solanacearum’ in both the plant and psyllid host, influences the expression of genes associated with thermal stress tolerance, among others, and may have been instrumental in driving the co-evolution of the pathosystem.
1 Introduction
Zebra chip (ZC) is a recent and economically important disease of potato, occurring in Mexico, Central America, United States of America (U.S.), and New Zealand (1–5). The potato/tomato psyllid, Bactericera cockerelli (Sulc.) (so. Hemiptera: order Homoptera), is native to North America (6, 7) and occurs in Central America, the western U.S., and as far north as Canada (5). Infestations by B. cockerelli have long been associated with psyllid yellows disease of potato and tomato, an uncharacterized disorder characterized by foliar chlorosis, stunting, and yield loss (8–10). Most recently, the emergent obligate, plant pathogenic bacterium, ‘Candidatus Liberibacter solanacearum’ belonging to the Alphaproteobacteria (11), also known as ‘Ca. Liberibacter psyllaurous’ (12), has been identified as the agent associated with zebra chip disease of potato and vein-greening (13) diseases of potato and tomato crops, respectively. The bacterium infects other solanaceous crops, including eggplant, pepper, and tobacco, and weed species (3, 5, 11, 14–20) as well as non-solanaceous crops (21–23).
Insect metabolic rate is known to be highly sensitive to temperature, and the concomitant acceleration of metabolism associated with increased temperature exposure can result in higher nutrient consumption, lipogenesis, and increased growth and development rates (24–27) that in turn can lead to increased population size and downstream wide-spread disease outbreaks. Indeed, specific temperature requirements have been reported for seasonal establishment of the psyllid vector and outbreaks of Liberibacter-associated diseases of citrus, and potato and tomato (5, 28–31). Also, reduced acquisition/transmission efficiency of ‘Ca. L. asiaticus’ (CLas) in Diaphorina citri nymphs and adults was reported to occur under sub-optimal temperatures (32). Further, reduced transmission rates have been demonstrated for bacterial pathogens of humans and other mammals, including Yersinia pestis, by the Oriental rat flea Xenopsylla cheopis (33), at non-optimal temperatures (34). In contrast, in human pathosystems, increased pathogenicity and disease severity of Legionella pneumophila and Shigella sp. have been correlated with periods of elevated temperatures (35–37).
Functional “omics” characterization of insect physiological responses and tolerance to environmental stress physiology is facilitated by comparative analyses of the proteome, transcriptome, metabolome, considered the primary or core pillars of response hierarchies (38). Several hemipteran phloem-feeding insects, including psyllids (39, 40) and whiteflies (41), have a specialized alimentary structure, or filter chamber that consists of a substrate permeable membrane channel on the hydrophobic interior and size-exclusion filter on the extracellular side of the membrane that allows for rapid water transport. This structure functions in osmoregulation essential for insects with high-volume liquid diets, cryoprotection, and anhydrobiosis, the latter, required to survive thermal and other environmental stresses (42, 43). In the whitefly, Bemisia tabaci (Genn.) and potato psyllid, movement of water through opposing alimentary tract tissues within the filter chamber involves the integral transmembrane protein, aquaporin (44, 45). In whiteflies, it has been shown to be expressed in the filter chamber and hindgut of early instar nymphs and adults where it relieves osmotic stress by stabilizing water balance through recycling and excretion functions (45). Down-regulation of aquaporin homologs encoded by aphids (46), B. tabaci (45, 47), and psyllids (48) resulted in disruption of normal osmoregulation functions, and for latter two insects, increased nymphal mortality and/or reduced lifespan of adults. In D. citri, knockdown evidence of differential changes in the metabolome and increased uric acid accumulation (48), which increases free radical-scavenging activity in hemolymph (49).
Osmotic stress has been shown to be involved in regulating expression of the Ras-like GTPase Rap 1 (guanosine triphosphatase), which functions as conserved molecular switch involved in downstream regulation of effector molecules that regulate cell growth and differentiation, secretion, cell adhesion and morphogenesis. In most organisms, Rap1 associates with effectors that regulate signaling pathways governing actin cytoskeleton and adhesion molecules (50) that modulate and control critical cellular processes. Further, actin cytoskeleton dynamics is known to be altered by certain pathogens to gain cellular entry, which triggers Rap 1 and other molecules that function in pathogenesis responsive processes and in dsRNA uptake (51, 52).
Other strategies for combatting biotic stress reside within the insect innate immune system Toll and immune deficiency (IMD) pathways that are involved in the genesis of antimicrobial peptides. They regulate the host response to Gram-negative bacterial infection by recognizing diaminopimelic acid (DAP)-type peptidoglycans that in turn interact with peptidoglycan recognition proteins LC (PGRP-LC) and LE receptors. Among the best-studied downstream components of the IMD pathway are Drosophila homologs of TAK1, IKK, and JNK kinases (53). Finally, lysosomes, a type of membrane bound organelle, contain hydrolytic enzymes that digest or degrade endogenous and exogenous sub-stances, and importantly, are responsible for nutrient sensing and storage, and retrieval. In these latter functions they participate in fusion and fission events with other organelles and the plasma membrane, including pino- and micropinocytotic vesicles and phagocytic vacuoles by which exogenous substances are transported into the cell. Lysosomes also participate in core physiological and pathogen-related processes in eukaryotes, including animal species, and invertebrates such as insects [see references in 54]. Cathepsins are proteases involved in the innate immune response in insects, for example, in the ACP midgut Cath-L (but not Cath-O) has been associated with the expression of Toll pathway constituents in CLas-infected psyllids, presumably to combat CLas invasion (55).
Despite recent advancements, studies to elucidate the multi-trophic interactions of biotic and abiotic stress enhancers (i.e., temperature, pathogen infection, other) in insect vector-pathosystems at gene expression and other functional “omics” levels, are limited (56). Yet, these studies are important and are evidenced by a study that suggests the negative effects of ‘Ca. L. solanacearum’ on potato psyllid fecundity were most likely due to the direct effects of the bacteria on the insect host and not host-plant associated (57). Further, understanding the dynamics of biotic-abiotic stress interactors could lead to new biopesticidal technologies to complement IPM functional genomic insights into psyllid-Liberibacter pathosystem, since biochemical, cellular, and functional genomic processes that could help guide the exploitation of RNA interference (RNAi) technologies have been limited. The paucity of relevant information has continued to hinder the identification of targets, that if knocked down, could inhibit key steps of ‘Ca. Liberibacter’ pathogenesis and circulative or systemic infection of the psyllid vector, while also reducing the number of bacteriliferous offspring.
‘Ca. Liberibacter’-associated diseases are managed primarily by chemical pesticide applications aimed at reducing the vector population size to concomitantly lower the rate of pathogen transmission to plant hosts, a number of complementary approaches have been implemented or are under consideration. These include breeding for host plant resistance (5), augmentative biological control (58), RNA interference (RNAi) technology for knockdown gene expression (59-61), and CRISPR-Cas editing approaches (62, 63).
The goal of this study was to investigate the prospective effects of temperature on potato psyllid vector fitness in different climatic and ecological scenarios using functional genomic analysis of gene expression together with quantitative PCR detection of ‘Ca. L. solanacearum’ to monitor pathogen load in adult psyllids post-exposure to temperatures representing ambient, extreme heat, or cold conditions. The rationale is that knowledge derived from gene profiling, in relation to ‘Ca. L. solanacearum’ accumulation in potato psyllids post-temperature treatments, could guide disease control efforts that focus on disrupting ‘Ca. L. solanacearum’ gut invasion and multiplication therein, and/or downstream systemic infection processes in the circulative transmission pathway to reduce bacterial transmission by the potato psyllid vector.
2 Materials and methods
2.1 Psyllid colonies
Certified pathogen-free potato mini-tubers (derived from tissue culture) of variety Atlantic were obtained from CSS Farms Inc. (Colorado City, CO, USA). Potato psyllid colonies were initially established from individuals collected from potato fields in Texas and Washington, as previously reported (40). Individuals from each colony were tested for ‘Ca. L. solanacearum’ presence by PCR amplification of the 16S rRNA gene, using an established method (11). Psyllids and ‘Ca. L. solanacearum’ were previously identified as the “central” and ‘Ca. L. solanacearum B’ haplotypes, respectively (5, 64). Colonies of ‘Ca. L. solanacearum’-infected and -uninfected potato psyllids were established and maintained in a separate growth chamber at the USDA-ARS facility in Wapato, WA (USA).
2.2 Temperature treatments
Temperature studies were conducted in a growth chamber (Percival Inc., Boone, IA) maintained at the following three temperatures, 18°C, 24°C, and 30°C. All chambers were maintained at 70% RH and light was provided at a 12:12 (L: D) h photoperiod. Plants used in this study were housed in small, individual hoop cage as described previously (5). Briefly, metallic wires were inserted into the four corners of the pot to create a frame over the plant and the frame was covered with insect screen fabric secured to the base of the pot with a large rubber band. Each experimental replicate consisted of 10 plants per temperature. Ten female and ten male adult psyllids (infected or uninfected) were placed on each plant and placed in a growth chamber. Adult psyllids were allowed to mate and reproduce. Approximately 30 days after psyllid addition, the progeny adult psyllids (n=100) were collected, crushed in Trizol, and stored at -80°C until shipping on dry ice for RNAseq library preparation at the Institute of Biological Chemistry, Washington State University, Pullman, WA, USA.
2.3 Quantitative polymerase chain reaction amplification
DNA was extracted and purified from ‘Ca. L. solanacearum’-infected B. cockerelli adults transferred to potato plants maintained in a growth chamber, at 18°C, 24°C, or 30°C, respectively. Quantitative-PCR (qPCR) was carried out using Taqman (hydrolysis) probes and primers designed to detect the ‘Ca. L. solanacearum’ outer membrane protein gene (omp; GenBank accession FJ914617) and B. cockerelli cytochrome oxidase 1 (COX; GenBank accession EF372597) reference gene (PrimeTime qPCR Assay 6-FAM/ZEN/IBFQ, synthesized by Integrated DNA Technology, IDT, Iowa, USA) (65). The experiment consisted of two biological replicates and three technical replicates with two negative controls (water instead of template; uninfected B. cockerelli total DNA) and one positive experimental control that consisted of a fragment of the ‘Ca. L. solanacearum’ 16S rRNA target and the B. cockerelli reference gene, respectively, cloned into the pGemT-Easy cloning vector (Promega) (65). A ten-fold serial dilution (to 10-6) of each linearized plasmid containing the respective verified, cloned insert was used to establish the standard curve. Each qPCR reaction contained 10 μl PCR Master Mix (Applied Biosystems) and 15 ng total DNA in 20 μl, adjusted with DNase/RNase-free water for omp and COX. Reactions contained 500 nM of each primer, 250 nM of probe (in 1 μl 20X Prime Time Assay, IDT). The qPCR amplification was carried out using a StepOnePlus™ Real-Time PCR System (Applied Biosystems, CA). Cycling conditions consisted of one cycle at 50°C for 2 minutes, followed by one cycle of 95°C for 10 minutes, then 40 cycles of 95°C for 15 seconds and 60°C for 60 seconds. To verify the presence of qPCR products in reactions, amplicons were visualized on a Gelred (Biotium)-stained 1% agarose gel in Tris-Acetate-EDTA buffer, pH 8.0, post-amplification.
2.4 Total RNA extraction, library construction and Illumina sequencing of adult psyllids
Total RNA was purified from ‘Ca. L. solanacearum’-infected and uninfected B. cockerelli adults grown at different temperatures as shown, 8 replicates from each treatment, for a total of 48 samples. For RNA extraction, 0.3 ml chloroform were added to 1 ml Trizol homogenate, followed by vigorous shaking for 30s and incubation for 3min at room temperature. Tubes containing samples were centrifuged at 12,000 ×g for 15 min at 4°C to separate organic and aqueous phases. The aqueous phase (200-250μl) was transferred to a sterile, RNase-free tube. An equal volume of 100% EtOH was added to each, and the contents were gently mixed. Samples were purified using the RNeasy Mini Kit (Qiagen, Valencia, CA), according to the manufacturer’s protocol. The quality and quantity of RNA was assessed using a NanoDrop 2000 Spectrophotometer (Thermo Scientific, Wilmington, DE). The ratio of absorbance at 260 nm and 280 nm was used to assess the purity of DNA, and ratios >2.0 were considered optimal. The RNA quality was analyzed on an Agilent 2100 Bioanalyzer (Agilent Technologies Inc., Santa Clara, CA). Samples with an RNA Integrity Number (RIN) value greater than or equal to 8 were selected for preparing the sequencing libraries (Agilent Technologies Inc., Santa Clara, CA). The poly-A RNA was isolated from 2 μg of the total RNA using Dynal oligo (dT) beads (Invitrogen) according to the manufacturer’s protocol. Following purification, the mRNA was fragmented by zinc treatment at 94°C for 5 min, and reverse-transcribed for first-strand cDNA synthesis using SuperScript II reverse transcriptase (Invitrogen). Random primers were added, then second-strand cDNA synthesis was carried out. The ends were repaired, phosphorylated, and an adenine base was added to the 3’-end of the blunt phosphorylated DNA fragments. Illumina adapters with unique indexes were ligated to the fragments, according to the Illumina’s TruSeq RNA Sample Preparation Guide (Illumina). The products were purified for the section of approximately 250 bp in size using Qiaquick Gel Extraction Kit (Qiagen). The cDNA fragments were amplified following the addition of PCR Master Mix and PCR Primer Cocktail (Illumina) to ligate the adapters, for 30 s at 98°C followed by 15 cycles of 10 s at 98°C, 30 s at 60°C, 30 s at 72°C and a final elongation step of 5 min at 72°C. The products were purified using the QIAquick PCR Purification Kit (Qiagen) to create an Illumina paired-end library. Library quality was assessed using a Bioanalyzer DNA 1000 Chip Series II (Agilent).
The libraries were quantified by qPCR amplification, prior to clustering using the protocol recommended by the manufacturer (Illumina). The library was diluted to a final concentration of 10 nM in elution buffer (Qiagen). Samples were diluted to 2nM with 1M NaOH and held at 25°C for 2 min before transferring 2.5 μl into 497.5 μl of HT1 (high salt buffer supplied with cluster kit) to yield a final concentration of 10 pM. One hundred and twenty microliters were transferred to a 200 μl strip tube and applied for cluster generation in a flow cell on a cBOT (Illumina). The flow cell was loaded onto the Illumina Hiseq 2000 instrument, according to the manufacturer’s instructions. Sequencing was carried out at Macrogen with 50bp single read format according to the manufacturer’s instructions.
2.5 Bioinformatics analyses
The Illumina raw reads obtained for the ‘Ca. L. solanacearum’-infected and -uninfected adult and nymph whole body potato psyllids subjected to the three temperature treatments (64) were used to reassemble a transcriptome, to maximize quality and coverage of the de novo assembled transcript data. Briefly, three different assemblers were implemented, using the respective default settings for each: CLC Genomics Workbench v6.5.1 (http://www.clcbio.com), SOAP de novo trans v1.03 (66), and EBARDenovo v2.01 (67). The EvidentialGene algorithm was used to generate consensus sequences from the individual assemblies (68). A consensus was determined and compiled for each assembly. The resulting contigs and read counts per library were analyzed using Transcriptome Computational Workbench v 1.6.8 (TCW) software (69). The resultant 25,634 potato psyllid Illumina transcript database was consulted as the reference transcriptome for mapping reads.
Raw data was normalized, and subsequent data was processed using the ArrayStar suite of programs (DNASTAR, Madison, WI). Raw data was normalized using reads per kilobase per million mapped reads (RPKM) method. The processed reads were mapped with the Q-Seq in ArrayStar v14.0 (DNASTAR, Madison, WI) using the default settings. The analysis consisted of 8 replicates. The TCW ‘Filters Query’ was used to query the KEGG (Kyoto Encyclopedia of Genes and Genomes) assignments by exporting the translated open reading frames of selected contigs. Contigs were mapped to the respective biochemical pathways available through the KEGG Automatic Annotation Server (KASS). The bi-directional best hit (BBH) method that implements BLAST to query a set of orthologous groups in KEGG GENES, was used to identify the respective orthologs in KEGG Orthology (KO) and the Enzyme Commission (EC) distributions were determined using the online tool, available at http://www.genome.jp/kegg/kaas/ (50). Recently annotated Diaphorina citri sequences (70) were used to supplement potato psyllid transcript identifications using the database available in the Brown lab [database in ref 64].
3 Results
3.1 Altered ‘Ca. L. solanacearum’ accumulation in response to temperature
Results from qPCR analysis indicated that long term temperature exposures, e.g. ~ 30 days, comparably influenced the ‘Ca. L. solanacearum’ population size, based on the nearly equal ‘Ca. L. solanacearum’ genome copy number (based on Cq value) detected in psyllids reared at 18°C and 30°C. Based on Cq values, psyllids reared at both 18° and 30°C harbored one order of magnitude fewer ‘Ca. L. solanacearum’ genome copies, compared to PoP reared at the more ‘optimal temperature’ of 25°C (Figure 1).
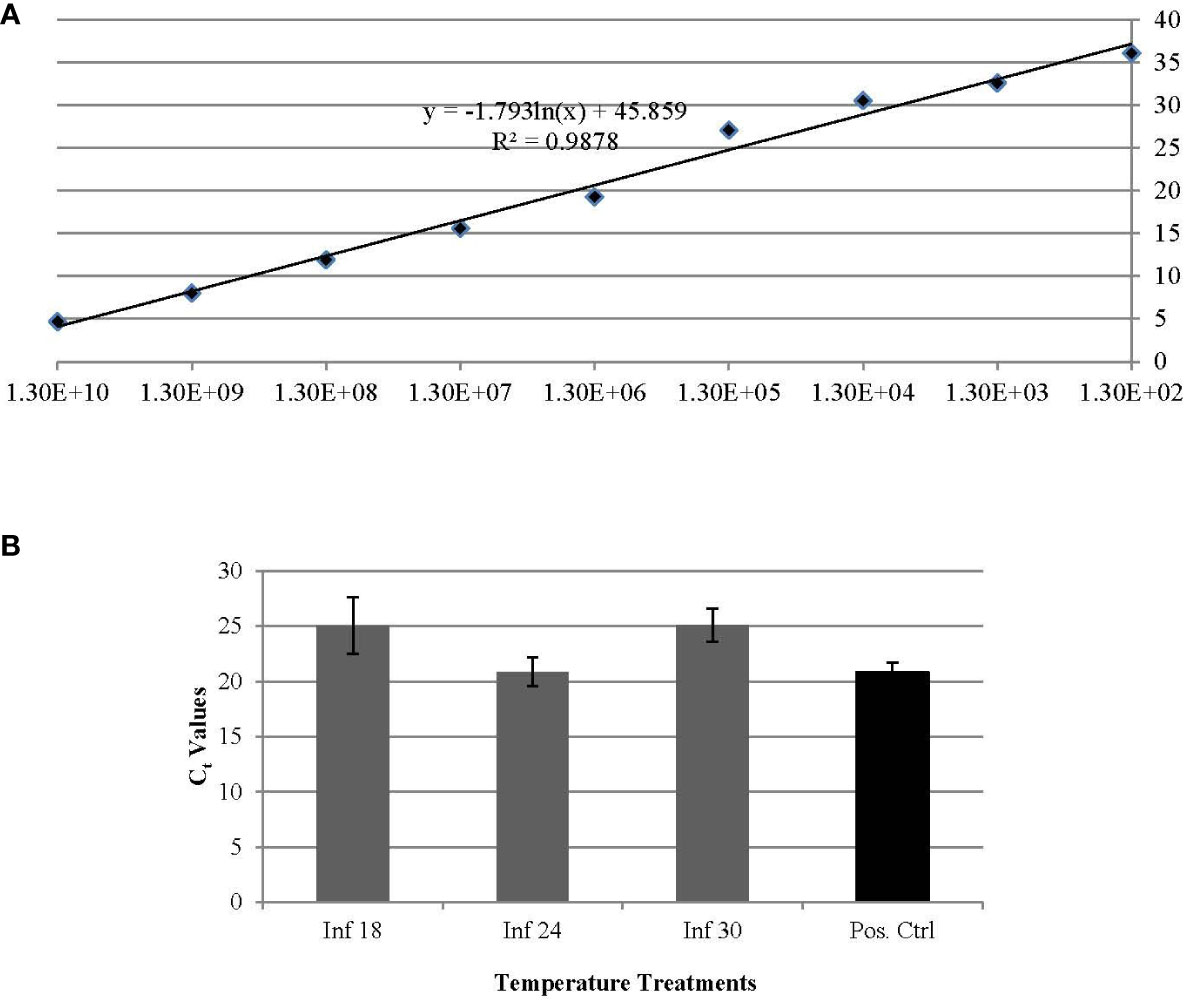
Figure 1 (A, B). Standard curve based on relationship of Ct (y-axis) and copy number (x-axis) per 25 ng of DNA established for quantification of ‘Ca. L. solanacearum’ in potato psyllids (A). The average Ct values of experimental potato psyllids reared at 18°C, 24°C, and 30°C and the average Ct value of potato psyllids maintained in laboratory colonies (B). Potato psyllids reared at the two temperature extremes, 18 and 30°C, had one order of magnitude lower number of copies of ‘Ca. L. solanacearum’ DNA, compared to those reared at the optimal temperature of 24°C.
3.2 Altered gene expression in response to temperature in ‘Ca. L. solanacearum’-infected and –uninfected potato psyllid
The HiSeq 200 sequencing of psyllid RNA yielded approximately 1070 million pair-end high quality reads of 50 bp in length (Table 1), which were analyzed using the Q-Seq program in ArrayStar v14.0 (DNASTAR, Madison, WI), with default settings. The 25,634 potato psyllid transcripts (100%) were mapped. Among them, 3,142 transcripts (12%) exhibited a 2-fold change (r2 = 0.9591) or greater in gene expression at 18°C, compared to 24°C for the ‘Ca. L. solanacearum’-free (uninfected) psyllids (Figure 2). In particular, expression of transcript BcAN_04148; 60S ribosomal) was significantly differentially expressed (Figure 3; Supplementary Table 1). Comparisons of B. cockerelli gene expression profiles at 30°C and 24°C, indicated that 5,547 transcripts (21%) exhibited at least a 2-fold change (r2 = 0.8833) for ‘Ca. L. solanacearum’-free (uninfected) psyllids (Figure 2). Though the overall number of significantly expressed genes was reduced after the Bonferroni statistical analysis (Figure 3), a robust number of genes, or 858, showed at least a 2-fold change in gene expression due to temperature-related affects (95% confidence level) (Supplementary Table 2).
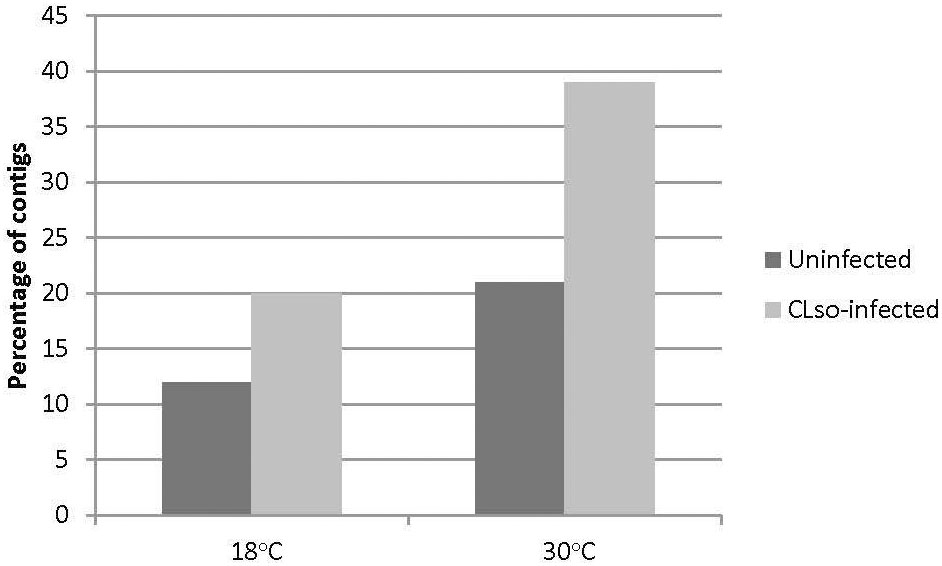
Figure 2 Percentage of transcripts with 2-fold or greater change in gene expression at 18°C or 30°C, to the moderate, herein considered ‘optimal’ temperature of 24°C, and 18°C to 30°C comparisons. The results indicate that more transcripts were differentially expressed at 30°C, but not at 18°C, when compared to 24°C, for the uninfected psyllids (black bars), and the ‘Ca. L. solanacearum’-infected psyllids (gray bars). The direct comparisons of 18°C to 30°C showed a trend similar to that observed for the 30°C and 24°C temperatures.
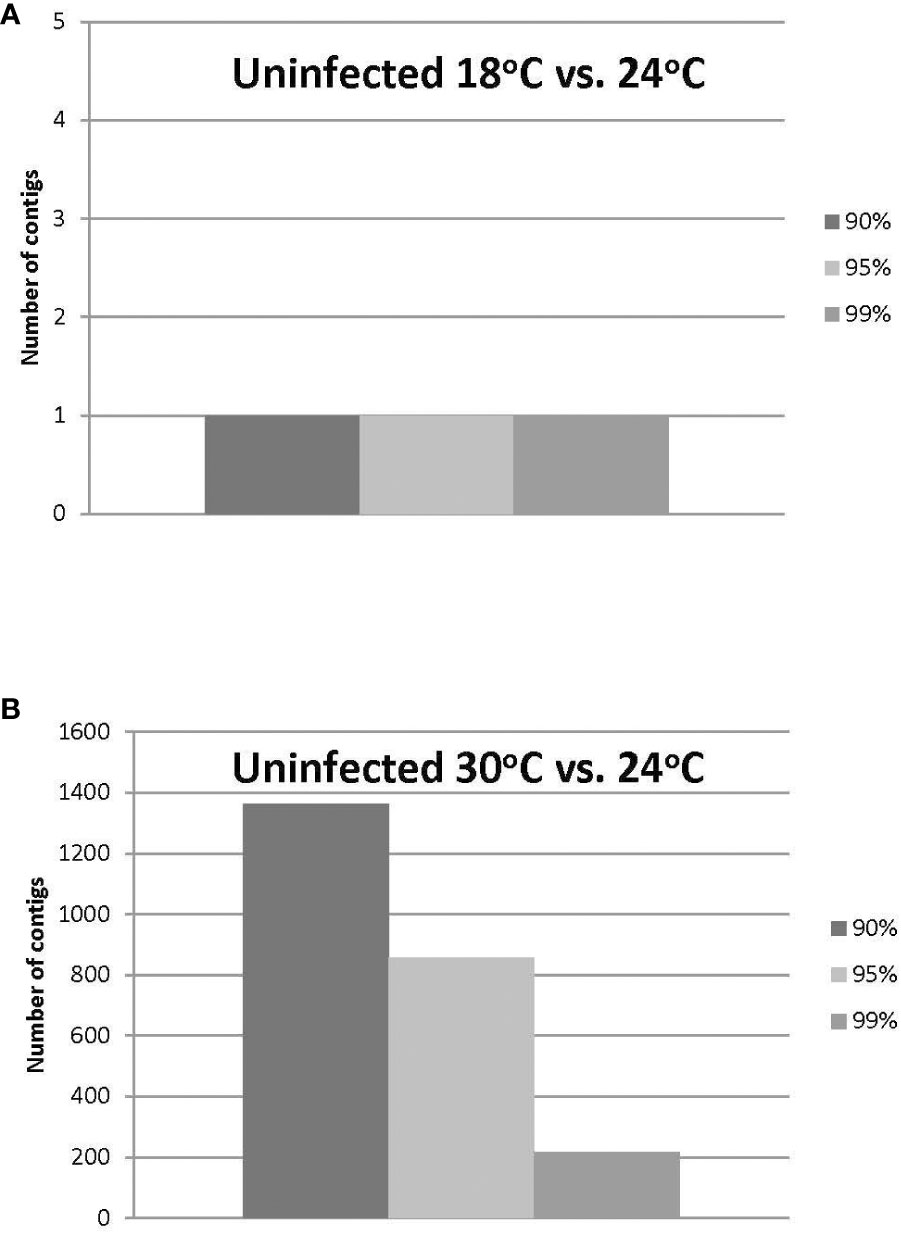
Figure 3 (A, B). Number of uninfected potato psyllid transcripts with significant changes in gene expression at 18°C or 30°C, compared to the optimal temperature of 24°C at the different Boneferroni confidence intervals. More transcripts were affected by the highest (B) compared to the lowest temperature (A), and fewer transcripts exhibited significant differential expression, with increasing confidence (A, B).
Approximately 20% of the transcripts expressed in the ‘Ca. L. solanacearum’-infected psyllids showed at least 2-fold change in expression at 18°C compared to 24°C (r2 = 0.8778), and nearly 40% at 30°C compared to 24°C (r2 = 0.8382) temperature comparisons (Figure 2). Of these, 165 and 368 transcripts were significantly differentially expressed (95% confidence; Bonferroni) at 18°C or 30°C treatments, compared to the 24°C temperature, respectively (Figure 4; Supplementary Tables 3, 4). Further, approximately 87% (1,218) of the 1,392 differentially expressed genes exhibiting altered expression following low and high temperature treatments, respectively (Supplementary Tables 1-4), however, expression of certain genes was often found to apply exclusively to a specific temperature treatment over others (Figure 5). Regardless of the ‘Ca. L. solanacearum’-infection status, fewer genes were differentially expressed at the lowest temperature (18°C). However, based on the differential expression of 23 genes compared to one unique gene, respectively, the ‘Ca. L. solanacearum’-infected psyllids appeared to exhibit a greater sensitivity to heat stress than their Ca. L. solanacearum-free (uninfected) counterparts, (Figure 5). The 23 uniquely and significantly differentially expressed genes of ‘Ca. L. solanacearum’-infected psyllids reared at 18°C were identified (annotated) as mostly ribosome synthesis-related genes to increase protein synthesis. Another notable gene in this group was Acetyl-CoA (BcAN_14104), which is known to be involved in lipogenesis. The differential expression of this gene was associated with high and low temperature regimes and is consistent with the increased need for cuticular hydrocarbons during stress (26, 71). This observation is supported by a previous study in which ‘Ca. L. asiaticus’-infected D. citri exhibited mitochondrial dysfunction associated with changes in gene expression of D. citri genes involved in the citric acid cycle (TCA) (72). Strikingly, quite the opposite outcome was documented for D. citri adults exposed to the high temperature regime (30°C) when compared to 24°C treatment. Finally, based on the significantly greater number of differentially expressed and unique genes, e.g., 824 and 197, respectively, the expression profiles indicated that the ‘Ca. L. solanacearum’-free psyllids were much more sensitive to thermal stress than their ‘Ca. L. solanacearum’-infected counterparts (Figure 5).
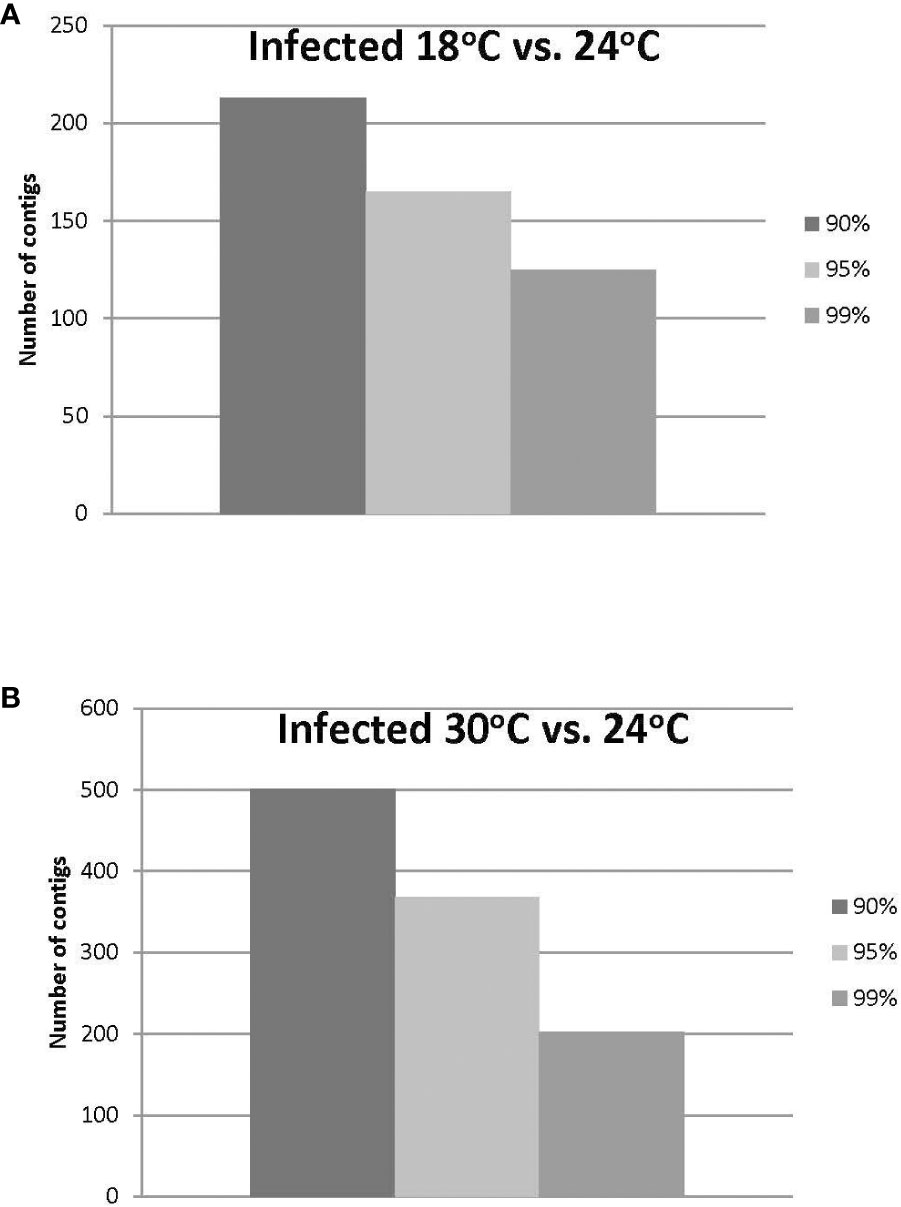
Figure 4 (A, B). Number of ‘Ca. L. solanacearum’-infected potato psyllid transcripts with significant changes in gene expression at 18°C or 30°C, compared to the optimal temperature of 24°C at the different Boneferroni confidence intervals. More transcripts were affected by high temperature (B) than lower temperature (A), and fewer transcripts exhibited significant differential expression, with increasing confidence (A, B).
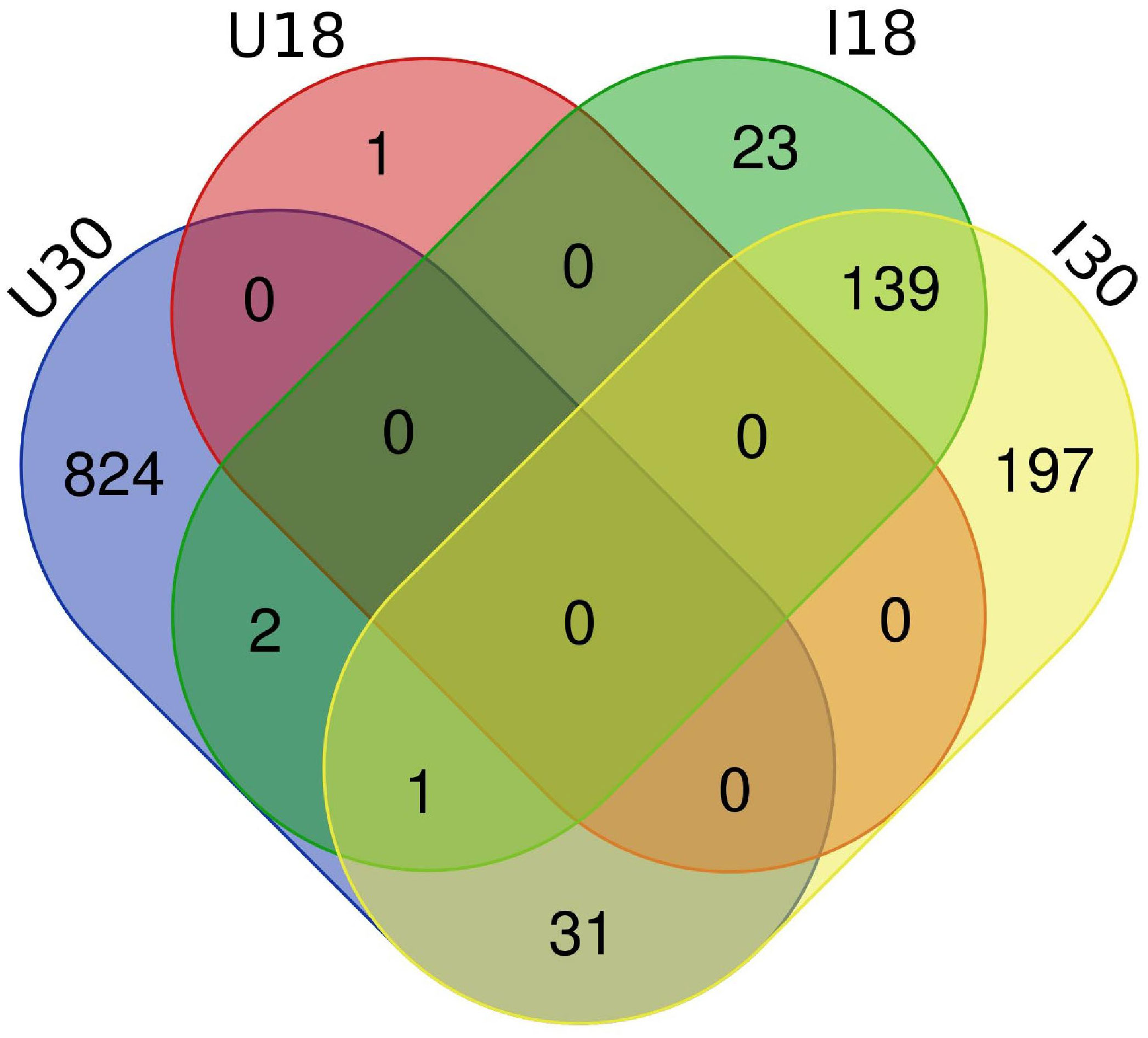
Figure 5 Venn diagram depicting the unique and overlapping genes between ‘Ca. L. solanacearum’ -infected and uninfected potato psyllids which had significant expressional differences (2-fold; 95% Bonferroni confidence interval). ‘Ca. L. solanacearum’-free psyllids had more differentially expressed transcripts at 30°C than ‘Ca. L. solanacearum’-infected psyllids. ‘Ca. L. solanacearum’-infected psyllids had more differentially expressed transcripts at 18°C than uninfected potato psyllids.
3.3 Kyoto Encyclopedia of Genes and Genomes (KEGG) analyses of temperature-sensitive potato psyllid transcripts
The KEGG analysis and gene function prediction revealed the context of specific pathways involved in physiological responses to temperature treatments. The 1,218 unique genes that showed altered expression of at least 2-fold (95% CI; Bonnferroni) in response to both low and high temperature treatments (Figure 5) were subjected to further analysis. The gene expression profiles showed that the greatest effect occurred at the highest temperature examined, 30°C, based on total number of differentially expressed psyllid genes. Subsequently, the KEGG functional assignments were compared for the ‘Ca. L. solanacearum’-infected and -uninfected adult psyllids reared at the putative optimal (24°C) and high (30°C) temperature treatments (Figure 6). Results showed that functions previously observed to be associated with pathogen invasion (65, 73–75) were over-expressed, particularly those with predicted involvement in endocytosis, Rap 1 signaling, and regulation of the actin cytoskeleton. Notably, these latter predicted functions were not observed among the transcripts of psyllids reared at 30°C.
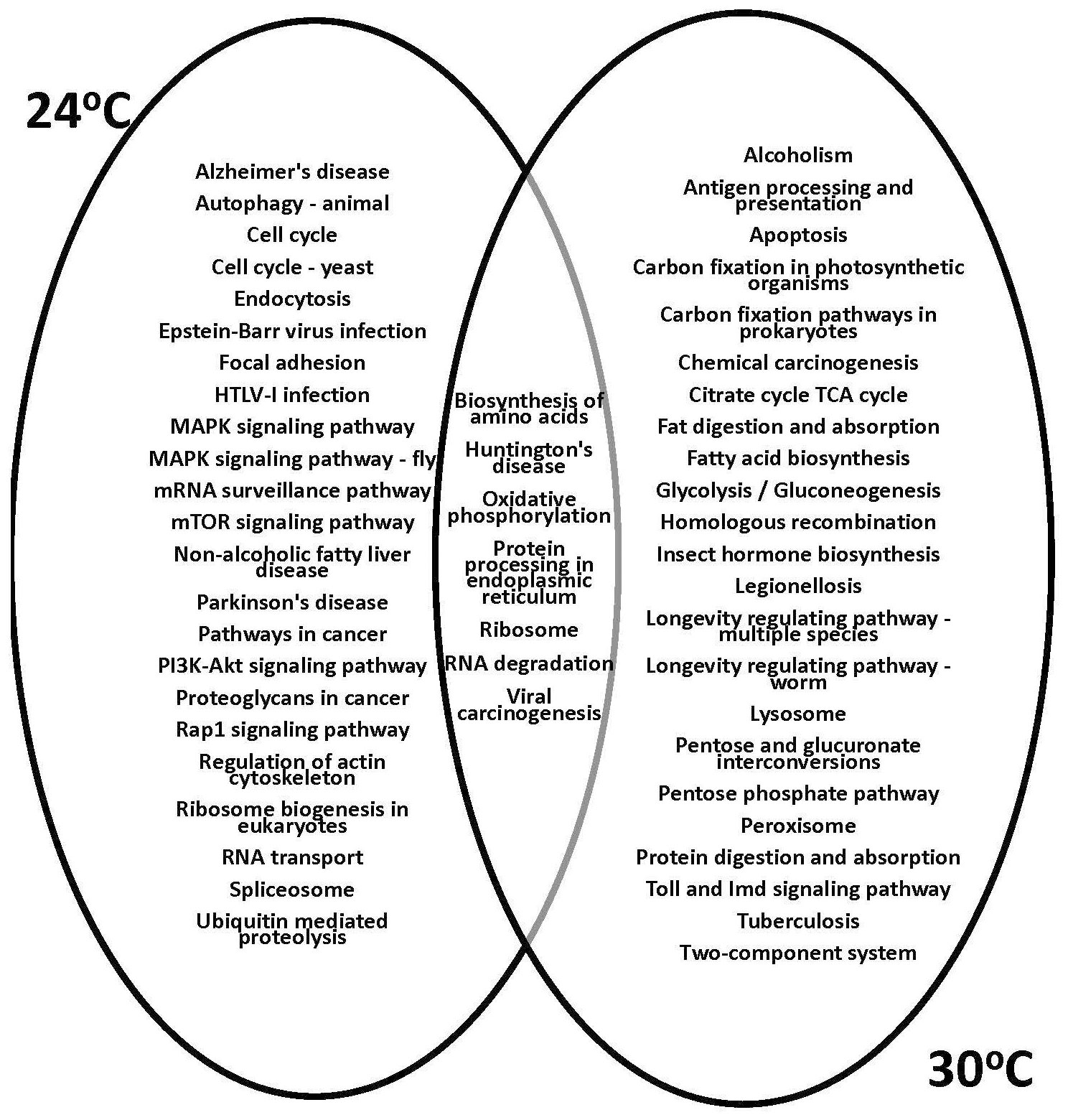
Figure 6 Kyoto Encyclopedia of Genes and Genomes (KEGG) analysis of genes significantly differentially expressed (2-fold; 95% Bonferroni confidence interval) at the 30°C temperature regime for the ‘Ca. L. solanacearum’-infected and uninfected potato psyllids. Venn diagrams show the shared and unique assigned pathways (among the top 30 non-metabolic KEGG pathways) between psyllids reared at 24°C and 30°C.
4 Discussion
Host-pathogen dynamics are complex and not well-studied for the B. cockerelli-’Ca. L. solanacearum’ pathosystem. However, environmental factors such as temperature and light are well-known to influence insect vector behavior. Recently, size and morphometric variation in psyllids associated with temperature change have been investigated (76). The effects of temperature on B. cockerelli-mediated ‘Ca. L. solanacearum’ transmission efficiency are not well-studied, nor are the cellular and tissue processes that underlie ‘Ca. L. solanacearum’ pathogenesis. Also of interest is how changes in B. cockerelli gene expression is manifest in extreme seasonal weather that may modulate ‘Ca. L. solanacearum’ multiplication/accumulation in the B. cockerelli host relative to vector competency. Further, understanding the relationship between ‘Ca. L. solanacearum’ load (accumulation), transmission efficiency, disease incidence, and disease severity could inform disease risk and knowledge-driven disease management.
The results for ‘Ca. L. solanacearum’ accumulation for B. cockerelli from qPCR analysis (Figure 1) were consistent with findings showing the significantly higher ‘Ca. L. asiaticus’ genome copy number in D. citri adults reared on citrus plants maintained at 25°C, compared to those reared on citrus maintained at 15°C or 35°C (32). However, for B. cockerelli the ‘Ca. L. solanacearum’ genome copy number at 15°C was significantly higher than for psyllids reared at 35°C. These results further support the differences seen in transmission efficiency, reported previously (5), were more likely due to increased, decreased, or neutral changes in psyllid gene expression as the result of exposure to changes in temperature. In a previous study, it was suggested that differences in transmission efficiency in the vector might be associated with differences in accumulation of ‘Ca. L. solanacearum’ in the individual psyllids and/or populations, however, it is expected that ‘Ca. L. solanacearum’ accumulation in psyllids would remain relatively constant within the B. cockerelli population collected from the same colony until they were exposed to differential temperature regimes, respectively. A greater understanding of differential gene expression in B. cockerelli experiencing temperature stress with respect to the dynamics associated with psyllid metabolism, reproduction, and transmission efficiency is needed. These parameters are the most influential in pest and disease risk models, particularly for potato psyllid, which are integral to determining the extent of ‘Ca. L. solanacearum’ spread that will occur in crops and overwinter in wild host plants.
A combination of biotic and abiotic stress may have shaped psyllid susceptibility or tolerance to ‘Ca. L. solanacearum’ infection, and perhaps benefitted, to manifest the initial invasion of solanaceous crops and wild host species by ‘Ca. L. solanacearum’-infected B. cockerelli, which was observed for the first time in the mid-1980’s (14). Previously, the potato psyllid has been known to migrate annually from possibly as far south as Central America and southern Mexico, and to disperse north and northwesterly in the continental U.S. and Canada. Since the mid-1900’s the potato psyllid was reported colonizing potato crops and to cause a disorder known as psyllid yellows, which resulted in the development of symptoms that are distinct from that presently associated with ‘Ca. L. solanacearum’ infection of potato. Because host plants recovered from symptoms when psyllid numbers were diminished on plants following insecticide application, a psyllid-transmissible pathogen could be ruled out as the causal agent of the disorder, which led to the hypothesis that psyllid yellows was caused by feeding damage, possibly associated with a salivary toxin. Thus ‘Ca. L. solanacearum’ is a relatively new pathogen that has been only recently associated with migrating B. cockerelli adults. This putative new ‘Ca. L. solanacearum’-psyllid pathosystem could well have emerged as a consequence of positive selection. This hypothesis is consistent with pathosystem that was first reported in ~2000 to the present, or at about the time estimated for the earliest temperature shifts that were experienced at equatorial/tropical and subtropical latitudes with the onset of climate change (3, 4, 14). In other scenarios, ‘Ca. Liberibacter’ spp. have been associated with the trade-offs of increased fecundity with minimal effects on longevity (77).
Only minimal statistically significant changes in gene expression were observed for ‘Ca. L. solanacearum’-free B. cockerelli reared at the sub-optimal temperature of 18°C, compared to expression profiles at 24°C, the proxy used herein for the predicted optimal temperature range of 22-26°C. Thus, psyllids experienced little to no discernable biological effects evident based on gene expression, at the low to mid-temperature ranges studied here. In contrast, at 30°C, ‘Ca. L. solanacearum’-free adults exhibited statistically significant changes in gene expression, particularly among genes involved in abiotic and biotic stress responses. Interestingly, increased gene expression by ‘Ca. L. solanacearum’-free B. cockerelli was greater than ‘Ca. L. solanacearum’-infected psyllids for which twice the number of genes had altered expression profiles. Consequently, gene expression modulation associated with the physiological responsiveness of B. cockerelli is affected more by high temperature-induced stress than by lower than optimal temperatures. These observations suggest that ‘Ca. L. solanacearum’ infection and spread in B. cockerelli populations occurred and/or can shift from once potentially rare levels to apparently near fixation. In turn, the establishment of ‘Ca. L. solanacearum’ (A and B haplotypes) has provided an adaptive mechanism for combatting thermal stress, resulting in increased survival of B. cockerelli and widespread distribution of ‘Ca. L. solanacearum’ haplotypes among B. cockerelli populations since the emergence of zebra chip disease in Mexico and the western and central U.S. beginning in the late1990’s-2000 (5).
The predicted functions of the differentially expressed genes reported in this study are consistent with previous reports that have implicated abiotic stress genes, as well as aquaporin, which showed 2.8-fold increased expression in ‘Ca. L. solanacearum’-infected psyllids reared at 30°C, compared to 24°C (48). In addition, several cathepsin-like genes (5- to 18-fold) and a phenol oxidase gene (3-fold) showed greater expression in ‘Ca. L. solanacearum’-infected psyllids reared at 30°C compared to the simulated optimal temperature of 24°C. This is consistent with previous reports in which innate immune responses have been attributed to ‘Ca. L. solanacearum’-B. cockerelli interactions associated with the circulative, propagative mode of transmission (65, 73, 78). Also, a cadherin-like gene, with predicted involvement in cell-to-cell interactions known in other pathosystems involving pathogen infections with host gut surfaces (79, 80) showed 3-fold increased expression in ‘Ca. L. solanacearum’-infected psyllids reared at 30°C, compared 24°C. These observations suggest that the B. cockerelli innate immune system is more responsive to temperature stress in ‘Ca. L. solanacearum’ infected psyllids, to afford greater protection to ‘Ca. L. solanacearum’-infected over ‘Ca. L. solanacearum’-uninfected psyllids, which could result in a higher percentage of ‘Ca. L. solanacearum’-infected potato psyllid in each population, under thermal stress. It also suggests that ‘Ca. L. solanacearum’ infection provides a protective effect to ensure survival of itself and the psyllid host and vector. Consistent with the differential transcript profiles, the predicted KEGG pathway gene functions have also been implicated in circulative, propagative, transmission of ‘Ca. L. solanacearum’, namely, proteins involved in ribosome synthesis, endocytosis, and actin cytoskeleton regulation (81, 82). Specifically, the reduction or absence of expression of these transcripts in the potato psyllid vector maintained at 30°C, and the significantly lower transmission efficiency reported in previously published experiments conducted at 30°C (5), are taken together as evidence that Rap1 signaling, actin cytoskeleton remodeling, and endocytosis are critical for host-’Ca. L. solanacearum’ interactions, particularly during the early to mid-instar stage infection processes, that corresponds to the timeframe prior to profuse systemic infection and ‘acquisition’ or entry of viable ‘Ca. L. solanacearum’ into the potato psyllid salivary glands. These results point to these and other pathway-associated genes as potentially lucrative targets for therapies aimed at disrupting optimal ‘Ca. L. solanacearum’ gut invasion and systemic infection of the psyllid host, and result in lowered rates of pathogen transmission in the psyllid population as well as reducing tree-to-tree ‘Ca. L. solanacearum’ spread. Notably, KEGG pathway predictions for potato psyllids reared at the most extreme temperature, 30°C support results of previously published studies that reported increased expression of genes in the Lysosome and Toll and Imd signaling pathway (53), a pathway responsible for modulating early responses that might be anticipated in an insect host/vector to counter invasion and systemic infection of ‘Ca. L. solanacearum’.
These proteins are hypothesized to be important mediators of the initial gut invasion stages of the B. cockerelli host by ‘Ca. L. solanacearum’ (65, 83). Indeed, ‘Ca. L. solanacearum’ invasion of B. cockerelli exploits host endo-exocytic processes and cytoskeletal remodeling (84) as well as other cellular processes negatively affected by high temperatures that could ultimately result in a lowered accumulation of Liberibacter in the psyllid host which could feasibly be detrimental to ‘Ca. L. solanacearum’ survivability in the long term. Thus, blocking or disruption of these processes by RNAi for example, may result in reduced ‘Ca. L. solanacearum’ transmission frequency, while also lessening the protective effects to thermal stress afforded by ‘Ca. L. solanacearum’ to the psyllid host.
Finally, the abundance of differentially expressed genes, at 824 compared to 197, and the over-expression of genes encoding stress-responsive proteins in ‘Ca. L. solanacearum’-infected compared to ‘Ca. L. solanacearum’-free B. cockerelli indicated ‘Ca. L. solanacearum’ infection affords protection against thermal stress, effectively ensuring survival of the pathosystem and ‘Ca. L. solanacearum’ transmission to the plant host. A recent study ‘Ca. L. solanacearum’ of the A and B haplotypes of B. cockerelli showed that infection by each haplotype resulted in the differential expression of a unique suite of gut genes predicted to be involved in innate immunity. Further, the transcriptional responses in ‘Ca. L. solanacearum’ B infected B. cockerelli guts exhibited a more robust response than in psyllid guts infected with ‘Ca. L. solanacearum’ A (85).
The recent emergence of ‘Ca. L. solanacearum’-B. cockerelli pathosystems approximately 20 years ago appears to have coincided with early effects of global warming in subtropical and mild temperate climes. This observation suggests that ‘Ca. L. solanacearum’-infected B. cockerelli populations emerged in parallel with increasingly higher temperatures occurring in Central America ~1000 miles from the equator, where the earliest B. cockerelli outbreaks were observed (3, 86). Recent studies have shown that ‘Ca. L. solanacearum’-infected psyllids are endemic in potato-growing areas of Mexico and Central America from where they migrate annually to the southwest/northwest and central parts of the U.S. and Canada. Until recent outbreaks of zebra chip and tomato vein-greening diseases emerged, B. cockerelli was thought to overwinter and migrate into the western U.S. and Canada south of the Rio Grande Valley, Texas (14). Apparently, the ‘Ca. L. solanacearum’-free B. cockerelli populations previously associated with psyllids yellows disorder have only recently been replaced by the ‘Ca. L. solanacearum’- B. cockerelli pathosystem, predominating in the western U.S. and Canada since the late 1990s (4, 14).
5 Conclusions
This study documents for the first time the effects of thermal stress on the gene expression of ‘Ca. L. solanacearum’-infected compared to ‘Ca. L. solanacearum’-free (uninfected) potato psyllids from a functional genomic perspective. Less than optimal temperatures negatively influenced ‘Ca. L. solanacearum’ invasion, multiplication (genome copy number), and potentially exocytosis from the gut to the blood, ultimately reducing the circulating ‘Ca. L. solanacearum’ cells available for entry into the salivary glands and/or oral cavity (40, 87, 88) from where the bacteria are transmitted to the psyllid host. The putative high temperature-associated reduced in ‘Ca. L. solanacearum’ accumulation in the psyllid vector is consistent with previously reported in planta observations. Indeed, symptom development was reported to be delayed in ‘Ca. L. solanacearum’-infected plants maintained at low temperatures, while symptom development was accelerated in ‘Ca. L. solanacearum’-infected plants maintained at higher than optimal temperatures. In silico expression and functional analyses pointed to several B. cockerelli genes that function naturally in cytoskeletal remodeling and are likely utilized by ‘Ca. L. solanacearum’ for invasion, and in psyllid immunity and other defenses. Expression of some of the proteins identified here during rearing of psyllids under prolonged conditions of thermal stress, can be modulated by ‘Ca. L. solanacearum’-infection (65, 73, 89, 90) to enhance ‘Ca. L. solanacearum’ invasion of and pathogenesis in young psyllid instars, and ‘Ca. L. solanacearum’ multiplication and systemic infection of B. cockerelli. Other B. cockerelli gene products are implicated as ‘Ca. L. solanacearum’-effector interactors with predicted functions in attachment/invasion/exocytosis, and potentially salivary glands entry (65, 73, 84, 91, 92). The RNAseq differential expression profiles reported here provide the first snapshots into host-pathogen thermal adaptation mechanisms and highlight key biochemical pathways in its insect host, apparently essential for ‘Ca. L. solanacearum’ survival outside of the plant, and for psyllid-to-plant transmission. Whether naturally occurring extreme temperatures would be expected to result in a shift or curtailment of extant B. cockerelli-’Ca. L. solanacearum’ habitats remain to be seen. The results of this study are expected to provide useful insights into risk assessment and strategies for psyllid vector-’Ca. L. solanacearum’ control pertaining to the potential for the different B. cockerelli/’Ca. L. solanacearum’ haplotype combinations to adapt to changing temperature and weather/climatic conditions.
Data availability statement
The raw data reads used in this study are deposited in the NCBI SRA repository under Bioproject PRJNA252003.
Author contributions
TF: Formal analysis, Investigation, Validation, Writing – original draft, Writing – review & editing. JM: Conceptualization, Funding acquisition, Project administration, Supervision, Writing – review & editing. JB: Conceptualization, Project administration, Supervision, Visualization, Writing – review & editing.
Funding
The author(s) declare financial support was received for the research, authorship, and/or publication of this article. This research was funded by USDA-NIFA SRCRI Subcontract from the Citrus Research and Development Foundation, Inc. Grant no. 13-005NU-784. The funder was not involved in the study design, collection, analysis, interpretation of data, the writing of this article or the decision to submit it for publication.
Acknowledgments
The authors would like to thank Dr. James Crosslin for helpful discussions and contributions to the experimental design. We also thank Drs. David Gang and Ruifeng He for their invaluable assistance with library preparation and sequencing.
Conflict of interest
The authors declare that the research was conducted in the absence of any commercial or financial relationships that could be construed as a potential conflict of interest.
The author JB declared that they were an editorial board member of Frontiers at the time of submission. This had no impact on the peer review process and the final decision.
Publisher’s note
All claims expressed in this article are solely those of the authors and do not necessarily represent those of their affiliated organizations, or those of the publisher, the editors and the reviewers. Any product that may be evaluated in this article, or claim that may be made by its manufacturer, is not guaranteed or endorsed by the publisher.
Supplementary material
The Supplementary Material for this article can be found online at: https://www.frontiersin.org/articles/10.3389/finsc.2023.1279365/full#supplementary-material
Supplementary Table 1 | List of differentially expressed contigs between ‘Ca. L. solanacearum’-uninfected psyllids reared at 18° and 24°C.
Supplementary Table 2 | ‘Ca. L. solanacearum’-uninfected psyllids reared at 30° and 24°C.
Supplementary Table 3 | ‘Ca. L. solanacearum’-infected psyllids reared at 18° and 24°C.
Supplementary Table 4 | ‘Ca. L. solanacearum’-infected psyllids reared at 30° and 24°C.
References
1. Munyaneza JE, Crosslin JM, Upton JE. Association of Bactericera cockerelli (Homoptera: Psyllidae) with “zebra chip,” a new potato disease in southwestern United States and Mexico. J Economic Entomology (2007) 100(3):656–63. doi: 10.1603/0022-0493(2007)100[656:AOBCHP]2.0.CO;2
2. Secor GA, Rivera VV, Abad JA, Lee IM, Clover GRG, Liefting LW, et al. Association of ‘Candidatus Liberibacter solanacearum’ with zebra chip disease of potato established by graft and psyllid transmission, electron microscopy, and PCR. Plant Dis (2009) 93(6):574–83. doi: 10.1094/PDIS-93-6-0574
3. Rehman M, Melgar JC, Rivera C JM, Idris AM, Brown JK. First report of “Candidatus Liberibacter psyllaurous” or “Ca. Liberibacter solanacearum” associated with severe foliar chlorosis, curling, and necrosis and tuber discoloration of potato plants in Honduras. Plant Dis (2010) 94(3):376–6.
4. Sengoda VG, Munyaneza JE, Crosslin JM, Buchman JL, Pappu HR. Phenotypic and etiological differences between psyllid yellows and zebra chip diseases of potato. Am J Potato Res (2010) 87(1):41–9. doi: 10.1007/s12230-009-9115-x
5. Munyaneza JE. Zebra chip disease of potato: biology, epidemiology, and management. Am J Potato Res (2012) 89:329–50. doi: 10.1007/s12230-012-9262-3
6. Pletsch DJ. The potato psyllid, Paratrioza cockerelli (Sulc), its biology and control. Montana Agric Experiment Station Bull (1947) 446.
7. Wallis RL. Ecological studies on the potato psyllid as a pest of potatoes. Tech Bull US Dep Agri (1955) 1107:1–24.
8. Richards BL. Further studies with psyllid yellows of the potato. Phytopathology (1931) 21(1):103.
9. Eyer JR, Crawford RF. Observations on the feeding habits of the potato psyllid (Paratrioza cockerelli Sulc.) and the pathological history of the" psyllid yellows" which it produces. J Economic Entomology (1933) 26(4):846–50.
10. Cranshaw WS. Diseases caused by insect toxin: psyllid yellows. In Compendium of Potato Diseases (2nd Ed.), ed. Stevenson W. R., Loria R., Franc G. D., Weingartner D. P. St. Paul, MN: APS Press, pp. 73–74.
11. Liefting LW, Sutherland PW, Ward LI, Paice KL, Weir BS, Clover GR. A new ‘Candidatus Liberibacter’species associated with diseases of solanaceous crops. Plant Dis (2009) 93(3):208–14. doi: 10.1094/PDIS-93-3-0208
12. Hansen AK, Trumble JT, Stouthamer R, Paine TD. A new huanglongbing species, “Candidatus Liberibacter psyllaurous” found to infect tomato and potato, is vectored by the psyllid Bactericera cockerelli (Sulc). Appl Environ Microbiol (2008) 74(18):5862–5. doi: 10.1128/AEM.01268-08
13. Brown JK, Rehman M, Rogan D, Martin RR, Idris AM. First report of “Candidatus Liberibacter psyllaurous” (synonym “Ca. L. solanacearum”) associated with ‘tomato vein-greening’ and ‘tomato psyllid yellows’ diseases in commercial greenhouses in Arizona. Plant Dis (2010) 94(3):376–6.
14. Crosslin JM, Munyaneza JE, Brown JK, Liefting LW. Potato zebra chip disease: a phytopathological tale. Plant Health Prog (2010) 11(1):33. doi: 10.1094/PHP-2010-0317-01-RV
15. Aguilar E, Sengoda VG, Bextine B, McCue KF, Munyaneza JE. First report of “Candidatus Liberibacter solanacearum” on tomato in Honduras. Plant Dis (2013) 97(10):1375–5. doi: 10.1094/PDIS-04-13-0354-PDN
16. Aguilar E, Sengoda VG, Bextine B, McCue KF, Munyaneza JE. First report of “Candidatus Liberibacter solanacearum” on tobacco in Honduras. Plant Dis (2013) 97(10):1376–6. doi: 10.1094/PDIS-04-13-0453-PDN
17. Bextine B, Aguilar E, Rueda A, Caceres O, Sengoda VG, McCue KF, et al. First report of “Candidatus Liberibacter solanacearum” on tomato in El Salvador. Plant Dis (2013) 97(9):1245–5. doi: 10.1094/PDIS-03-13-0248-PDN
18. Munyaneza JE, Sengoda VG, Aguilar E, Bextine BR, McCue KF. First report of ‘Candidatus Liberibacter solanacearum’ infecting eggplant in Honduras. Plant Dis (2013) 97(12):1654–4. doi: 10.1094/PDIS-06-13-0641-PDN
19. Munyaneza JE, Sengoda VG, Aguilar E, Bextine B, McCue KF. First report of “Candidatus Liberibacter solanacearum” associated with psyllid-infested tobacco in Nicaragua. Plant Dis (2013) 97(9):1244–4. doi: 10.1094/PDIS-03-13-0247-PDN
20. Munyaneza JE, Sengoda VG, Aguilar E, Bextine B, McCue KF. First report of ‘Candidatus Liberibacter solanacearum’ on pepper in Honduras. Plant Dis (2014) 98(1):154–4. doi: 10.1094/PDIS-06-13-0598-PDN
21. Munyaneza JE. Psyllids as vectors of emerging bacterial diseases of annual crops. Southwestern Entomologist (2010) 35(3):471–7. doi: 10.3958/059.035.0335
22. Munyaneza JE. Zebra chip disease, Candidatus Liberibacter, and potato psyllid: A global threat to the potato industry. Am J Potato Res (2015) 92:230–5. doi: 10.1007/s12230-015-9448-6
23. Munyaneza JE, Mustafa T, Fisher TW, Sengoda VG, Horton DR. Assessing the likelihood of transmission of Candidatus Liberibacter solanacearum to carrot by potato psyllid, Bactericera cockerelli (Hemiptera: Triozidae). PloS One (2016) 11(8):e0161016. doi: 10.1371/journal.pone.0161016
24. Bale JS, Masters GJ, Hodkinson ID, Awmack C, Bezemer TM, Brown VK, et al. Herbivory in global climate change research: direct effects of rising temperature on insect herbivores. Global Change Biol (2002) 8(1):1–16. doi: 10.1046/j.1365-2486.2002.00451.x
25. Berggren A, Björkman C, Bylund H, Ayres MP. The distribution and abundance of animal populations in a climate of uncertainty. Oikos (2009) 118(8):1121–6. doi: 10.1111/j.1600-0706.2009.17558.x
26. Etges WJ, de Oliveira CC, Rajpurohit S, Gibbs AG. Effects of temperature on transcriptome and cuticular hydrocarbon expression in ecologically differentiated populations of desert Drosophila. Ecol Evol (2017) 7(2):619–37. doi: 10.1002/ece3.2653
27. Jamieson MA, Trowbridge AM, Raffa KF, Lindroth RL. Consequences of climate warming and altered precipitation patterns for plant-insect and multitrophic interactions. Plant Physiol (2012) 160(4):1719–27. doi: 10.1104/pp.112.206524
28. Bové JM. Huanglongbing: a destructive, newly emerging, century-old disease of citrus. J Plant Pathol (2006) 88:7–37.
29. Lopes SA, Frare GF, Bertolini E, Cambra M, Fernandes NG, Ayres AJ, et al. Liberibacters associated with citrus huanglongbing in Brazil: ‘Candidatus Liberibacter asiaticus’ is heat tolerant, ‘Ca. L. americanus’ is heat sensitive. Plant Dis (2009) 93(3):257–62.
30. Razi MF, Keremane ML, Ramadugu C, Roose M, Khan IA, Lee RF. Detection of citrus huanglongbing-associated ‘Candidatus Liberibacter asiaticus’ in citrus and Diaphorina citri in Pakistan, seasonal variability, and implications for disease management. Phytopathology (2014) 104(3):257–68. doi: 10.1094/PHYTO-08-13-0224-R
31. Liu X, Fan Y, Zhang C, Dai M, Wang X, Li W. Nuclear import of a secreted “Candidatus Liberibacter asiaticus” protein is temperature dependent and contributes to pathogenicity in Nicotiana benthamiana. Front Microbiol (2019) 10:1684. doi: 10.3389/fmicb.2019.01684
32. Wu F, Huang J, Xu M, Fox EG, Beattie GAC, Holford P, et al. Host and environmental factors influencing ‘Candidatus Liberibacter asiaticus’ acquisition in Diaphorina citri. Pest Manage Sci (2018) 74(12):2738–46. doi: 10.1002/ps.5060
33. Cross CL, Williams JL, Lucky A. Oriental rat flea xenopsylla cheopsis (Rothschild, 1903) (Insecta: siphonaptera: pulicidae): EENY-775/IN1330, 8/2021. EDIS (2021) 2021(5):1–5. doi: 10.32473/edis-IN1330-2021
34. Schotthoefer AM, Bearden SW, Holmes JL, Vetter SM, Montenieri JA, Williams SK, et al. Effects of temperature on the transmission of Yersinia Pestis by the flea, Xenopsylla Cheopis, in the late phase period. Parasites Vectors (2011) 4:1–11. doi: 10.1186/1756-3305-4-191
35. Maurelli AT, Blackmon B, Curtiss 3R. Temperature-dependent expression of virulence genes in Shigella species. Infection Immun (1984) 43(1):195–201. doi: 10.1128/iai.43.1.195-201.1984
36. Mauchline WS, James BW, Fitzgeorge RB, Dennis PJ, Keevil CW. Growth temperature reversibly modulates the virulence of Legionella pneumophila. Infection Immun (1994) 62(7):2995–7. doi: 10.1128/iai.62.7.2995-2997.1994
37. Friman VP, Hiltunen T, Jalasvuori M, Lindstedt C, Laanto E, Örmälä AM, et al. High temperature and bacteriophages can indirectly select for bacterial pathogenicity in environmental reservoirs. PloS One (2011) 6(3):e17651. doi: 10.1371/journal.pone.0017651
38. Hayward SA. Application of functional ‘Omics’ in environmental stress physiology: insights, limitations, and future challenges. Curr Opin Insect Sci (2014) 4:35–41. doi: 10.1016/j.cois.2014.08.005
39. Cicero JM, Brown JK, Roberts PD, Stansly PA. The digestive system of Diaphorina citri and Bactericera cockerelli (Hemiptera: Psyllidae). Ann Entomological Soc America (2009) 102(4):650–65. doi: 10.1603/008.102.0410
40. Cicero JM, Stansly PA, Brown JK. Functional anatomy of the oral region of the potato psyllid (Hemiptera: Triozidae). Ann Entomological Soc America (2015) 108:743–61. doi: 10.1093/aesa/sav059
41. Cicero JM, Hiebert E, Webb SE. The alimentary canal of Bemisia tabaci and Trialeurodes abutilonea (Homoptera, Sternorrhyncha) – histology, ultrastructure and correlations to function. Zoomorphology (1995) 115(1):31–9. doi: 10.1007/BF00397932
42. Campbell EM, Ball A, Hoppler S, Bowman AS. Invertebrate aquaporins: a review. J Comp Physiol B (2008) 178(8):935–55. doi: 10.1007/s00360-008-0288-2
43. Cohen S, Evans GW, Stokols D, Krantz DS. Behavior, health, and environmental stress. New York, NY: Springer Science & Business Media (2013).
44. Ibanez F, Hancock J, Tamborindeguy C. Identification and expression analysis of aquaporins in the potato psyllid, Bactericera cockerelli. PloS One (2014) 9:e111745. doi: 10.1371/journal.pone.0111745
45. Mathew LG, Campbell EM, Yool AJ, Fabrick JA. Identification and characterization of functional aquaporin water channel protein from alimentary tract of whitefly, Bemisia tabaci. Insect Biochem Mol Biol (2011) 41:178–90. doi: 10.1016/j.ibmb.2010.12.002
46. Shakesby AJ, Wallace IS, Isaacs HV, Pritchard J, Roberts DM, Douglas AE. A water-specific aquaporin involved in aphid osmoregulation. Insect Biochem Mol Biol (2009) 39:1–10. doi: 10.1016/j.ibmb.2008.08.008
47. Vyas M, Raza A, Ali AM, Ashraf MA, Mansoor S, Ahmad AS, et al. Knock down of whitefly gut gene expression and mortality by orally delivered gut gene-specific dsRNA. PloS One (2017) 12(1):e0168921. doi: 10.1371/journal.pone.016892
48. Santos-Ortega Y, Killiny N. Silencing of aquaporin homologue accumulates uric acid and decreases the lifespan of the Asian Citrus Psyllid, Diaphorina citri (Hemiptera: Liviidae). Insects (2021) 12(10):931. doi: 10.3390/insects12100931
49. Wigglesworth VB. Histochemical studies of uric acid in some insects. 2. Uric acid and polyphenols in the fat body. Tissue Cell (1987) 19(1):93–100.
50. Bos JL, de Rooij J, Reedquist KA. Rap1 signaling - adhering to new models. Nat Rev Mol Cell Biol (2001) 2(5):369–77. doi: 10.1038/35073073
51. Cappelle K, de Oliveira CFR, Van Eynde B, Christiaens O, Smagghe G. The involvement of clathrin-mediated endocytosis and two Sid-1-like transmembrane proteins in double-stranded RNA uptake in the Colorado potato beetle midgut. Insect Mol Biol (2016) 25(3):315–23. doi: 10.1111/imb.12222
52. Jaśkiewicz A, Pająk B, Orzechowski. A. The many faces of rap1 GTPase. Int J Mol Sci (2018) 19(10):2848. doi: 10.3390/ijms19102848
53. Tanji T, Hu X, Weber AN, Ip YT. Toll and IMD pathways synergistically activate an innate immune response in Drosophila melanogaster. Mol Cell Biol (2007) 27(12):4578–88. doi: 10.1128/MCB.01814-06
54. Van Houten B, Kong M, Bradshaw RA, Stahl PD. Eukaryotic Nucleotide Excision Repair. in: Bradshaw RA, Stahl PD (Eds.) Encyclopedia of Cell Biology. Waltham, MA: Academic Press (2016) pp. 435–41.
55. Yu H-Z, Huang Y-L, Li N-Y, Xie Y-X, Zhou G-H, Lu Z-J. Potential roles of two Cathepsin genes, DcCath-L and DcCath-O in the innate immune response of Diaphorina citri. J Asia-Pacific Entomology (2019) 22(4):1060–9. doi: 10.1016/j.aspen.2019.05.010
56. Todgham AE, Stillman JH. Physiological responses to shifts in multiple environmental stressors: relevance in a changing world. Integr Comp Biol (2013) 53(4):539–44. doi: 10.1093/icb/ict086
57. Nachappa P, Levy J, Pierson E, Tamborindeguy C. Correlation between “Candidatus Liberibacter solanacearum” infection levels and fecundity in its psyllid vector. J Invertebrate Pathol (2014) 115:55–61. doi: 10.1016/j.jip.2013.10.008
58. Lacey LA, Liu TX, Buchman JL, Munyaneza JE, Goolsby JA, Horton DR. Entomopathogenic fungi (Hypocreales) for control of potato psyllid, Bactericera cockerelli (Šulc) (Hemiptera: Triozidae) in an area endemic for zebra chip disease of potato. Biol Control (2011) 56(3):271–8. doi: 10.1016/j.biocontrol.2010.11.012
59. Mondal M, Carver M, Brown JK. Characteristics of environmental RNAi in potato psyllid, Bactericera cockerelli (Sulc)(Hemiptera: Psylloidea: Triozidae). Front Physiol (2022) 13:931951. doi: 10.3389/fphys.2022.931951
60. Paredes-Montero JR, Arif U, Brown JK. RNA interference-mediated knockdown of genes implicated in the synthesis of ecdysteroids, impairs molting in the potato psyllid, Bactericera cockerelli (Insecta: Hemiptera). Pest Manage Sci (2022) 78 (6):2204–14. doi: 10.1002/ps.6848
61. Paredes-Montero JR, Arif U, Brown JK. Knockdown of ecdysteroid synthesis genes results in impaired molting and high mortality in Bactericera cockerelli (Hemiptera: Triozidae). Pest Manage Sci (2022) 78(6):2204–14. doi: 10.1002/ps.6848
62. Goulin EH, Galdeano DM, Granato LM, Matsumura EE, Dalio RJD, MaChado MA. RNA interference and CRISPR: Promising approaches to better understand and control citrus pathogens. Microbiological Res (2019) 226:1–9. doi: 10.1016/j.micres.2019.03.006
63. Hunter WB, Gonzalez MT, Tomich J. BAPC-assisted CRISPR/Cas9 system: targeted delivery into adult ovaries for heritable germline gene editing (Arthropoda: Hemiptera). BioRxiv (2018) 1:478743.
64. Fisher TW, Vyas M, He R, Nelson W, Cicero JM, Willer M, et al. Comparison of potato and Asian citrus psyllid adult and nymph transcriptomes identified vector transcripts with potential involvement in circulative, propagative Liberibacter transmission. Pathogens (2014) 3:875–907. doi: 10.3390/pathogens3040875
65. Crosslin JM, Lin H, Munyaneza JE. Detection of ‘Candidatus Liberibacter Solanacearum’ in the potato psyllid, Bactericera cockerelli (Sulc) 1, by conventional and real-time PCR. Southwestern Entomologist (2011) 36(2):125–35. doi: 10.3958/059.036.0202
66. Li R, Yu C, Li Y, Lam TW, Yiu SM, Kristiansen K, et al. SOAP2: an improved ultrafast tool for short read alignment. Bioinformatics (2009) 25(15):1966–7. doi: 10.1093/bioinformatics/btp336
67. Chu HT, Hsiao WW, Chen JC, Yeh TJ, Tsai MH, Lin H, et al. EBARDenovo: highly accurate de novo assembly of RNA-Seq with efficient chimera-detection. Bioinformatics (2013) 29(8):1004–10. doi: 10.1093/bioinformatics/btt092
68. Gilbert D. Gene-omes built from mRNA seq not genome DNA. In: 7th Annual Arthropod Genomics Symposium (2013). p. 47405.
69. Soderlund C, Nelson W, Willer M, Gang DR. TCW: transcriptome computational workbench. PloS One (2013) 8(7):e69401. doi: 10.1371/journal.pone.0069401
70. Kanehisa M, Sato Y, Kawashima M, Furumichi M, Tanabe M. KEGG as a reference resource for gene and protein annotation. Nucleic Acids Res (2016) 44(D1):D457–62. doi: 10.1093/nar/gkv1070
71. Hosmani PS, Flores-Gonzalez M, Shippy T, Vosburg C, Massimino C, Tank W, et al. Chromosomal length reference assembly for Diaphorina citri using single-molecule sequencing and Hi-C proximity ligation with manually curated genes in developmental, structural, and immune pathways. Biorxiv (2019) 10:869685.
72. Hall DR, Wenninger EJ, Hentz MG. Temperature studies with the Asian citrus psyllid, Diaphorina citri: Cold hardiness and temperature thresholds for oviposition. J Insect Sci (2010) 11:83.
73. Kruse A, Fattah-Hosseini S, Saha S, Johnson R, Warwick E, Sturgeon K, et al. Combining 'omics and microscopy to visualize interactions between the Asian citrus psyllid vector and the Huanglongbing pathogen Candidatus Liberibacter asiaticus in the insect gut. PloS One (2017) 12(6):e0179531. doi: 10.1371/journal.pone.0179531
74. Vyas M, Fisher T, He R, Nelson WH, Yin G, Cicero JM, et al. Asian citrus psyllid expression profiles suggest Candidatus Liberibacter asiaticus-mediated alteration of adult nutrition and metabolism, and of nymphal development and immunity. PloS One (2015) 10(6):e0130328. doi: 10.1371/journal.pone.0130328
75. Yu H-Z, Li NY, Zeng XD, Song JC, Yu XD, Su HN, et al. Transcriptome analyses of Diaphorina citri midgut responses to “Candidatus” Liberibacter asiaticus infection. Insects (2020) 11(3):171.
76. Sarkar P, Kontsedalov S, Lebedev G, Ghanim M. The actin cytoskeleton mediates transmission of “Candidatus Liberibacter solanacearum” by the carrot psyllid. Appl Environ Microbiol (2020) 87(3):e02393–20.
77. Pelz-Stelinski KS, Killiny N. Better together: Association with 'Candidatus Liberibacter Asiaticus' increases the reproductive fitness of its insect vector, Diaphorina citri (Hemiptera: Liviidae). Ann Entomological Soc America (20016) 109(3):371–37. doi: 10.1093/aesa/saw007
78. Paris TM, Allan SA, Udell BJ, Stansly PA. Evidence of behavior-based utilization by the Asian citrus psyllid of a combination of UV and green or yellow wavelengths. PloS One (2017) 12(12):e0189228. doi: 10.1371/journal.pone.0189228
79. Jaiswal D, Sidharthan VK, Sharma SK, Rai R, Choudhary N, Ghosh A, et al. Candidatus Liberibacter asiaticus manipulates the expression of vitellogenin, cytoskeleton, and endocytotic pathway-related genes to become circulative in its vector, Diaphorina citri (Hemiptera: Psyllidae). 3. Biotech (2021) 11(2):1–12. doi: 10.1007/s13205-021-02641-x
80. Tavares CS, Mishra R, Ghobrial PN, Bonning BC. Composition and abundance of midgut surface proteins in the Asian citrus psyllid, Diaphorina citri. J Proteomics (2022) 261:104580. doi: 10.1016/j.jprot.2022.104580
81. Mishra S, Ghanim M. Interactions of Liberibacter species with their psyllid vectors: molecular, biological, and behavioral mechanisms. Int J Mol Sci (2022) 23(7):4029. doi: 10.3390/ijms23074029
82. Cheng X, Chen K, Dong B, Yang M, Filbrun SL, Myoung Y, et al. Dynamin-dependent vesicle twist at the final stage of clathrin-mediated endocytosis. Nat Cell Biol (2021) 23(8):859–69. doi: 10.1038/s41556-021-00713-x
83. Mann M, Saha S, Cicero JM, Pitino M, Moulton K, Hunter WB, et al. Lessons learned about the biology and genomics of Diaphorina citri infection with “Candidatus Liberibacter asiaticus” by integrating new and archived organ-specific transcriptome data. GigaScience (2022) 11:giac035.
84. Cicero JM, Fisher TW, Qureshi JA, Stansly PA, Brown JK. Colonization and intrusive invasion of potato psyllid by ‘Ca. Liberibacter solanacearum’. Phytopathology (2017) 107:36–49. doi: 10.1094/PHYTO-03-16-0149-R
85. Tang X-T, Levy J, Tamborindeguy C. Potato psyllids mount distinct gut responses against two different ‘Candidatus Liberibacter solanacearum’ haplotypes. PloS One (2023) 18(6):e0287396.
86. Espinoza-R. HR, Rivera-C. JM, Brown JK, Weller S. Manejo integrado De plagas de papa en Honduras. La Lima, Cortés, Honduras, C.A./USAID: Serie Protección Vegetal Conociendo y combatiendo los enemigos de los cultivos Fundación Hondureña De Investigación Agrícola (2014).
87. Cicero JM, Fisher TW, Brown JK. Localization of ‘Ca. Liberibacter solanacearum’ and evidence for surface appendages in the potato psyllid vector. Phytopathology (2016) 106:142–54. doi: 10.1094/PHYTO-04-15-0088-R
88. De Francesco A, Lovelace AH, Shaw D, Qiu M, Wang Y, Gurung F, et al. Transcriptome profiling of ‘Candidatus Liberibacter asiaticus’ in citrus and psyllids. Phytopathology (2022) 112(1):116–30. doi: 10.1094/PHYTO-08-21-0327-FI
89. Nachappa P, Levy J, Tamborindeguy C. Transcriptome analyses of Bactericera cockerelli adults in response to “Candidatus Liberibacter solanacearum” infection. Mol Genet Genomics (2012) 287(10):803–17. doi: 10.1007/s00438-012-0713-9
90. Ammar ED, Shatters RG Jr., Hall DG. Localization of Candidatus Liberibacter asiaticus, associated with citrus huanglongbing disease, in its psyllid vector using fluorescence in situ hybridization. J Phytopathol (2011) 159(11-12):726–34. doi: 10.1111/j.1439-0434.2011.01836.x
91. Ammar ED, Shatters RG Jr., Lynch C, Hall DG. Detection and relative titer of Candidatus Liberibacter asiaticus in the salivary glands and alimentary canal of Diaphorina citri (Hemiptera: Psyllidae) vector of citrus huanglongbing disease. Ann Entomological Soc America (2011) 104(3):526–33. doi: 10.1603/AN10134
Keywords: Bactericera cockerelli, ‘Candidatus Liberibacter solanacearum’, RNAseq, thermal stress, transcriptome
Citation: Fisher TW, Munyaneza JE and Brown JK (2024) Sub-optimal temperatures lead to altered expression of stress-related genes and increased ‘Candidatus Liberibacter solanacearum’ accumulation in potato psyllid. Front. Insect Sci. 3:1279365. doi: 10.3389/finsc.2023.1279365
Received: 17 August 2023; Accepted: 23 November 2023;
Published: 12 January 2024.
Edited by:
Sudeshna Mazumdar-Leighton, University of Delhi, IndiaReviewed by:
Assunta Bertaccini, University of Bologna, ItalySuresh Nair, International Centre for Genetic Engineering and Biotechnology, India
Copyright © 2024 Fisher, Munyaneza and Brown. This is an open-access article distributed under the terms of the Creative Commons Attribution License (CC BY). The use, distribution or reproduction in other forums is permitted, provided the original author(s) and the copyright owner(s) are credited and that the original publication in this journal is cited, in accordance with accepted academic practice. No use, distribution or reproduction is permitted which does not comply with these terms.
*Correspondence: Judith K. Brown, SkJyb3duQGFnLmFyaXpvbmEuZWR1