- Department of Entomology, Cornell University, Ithaca, NY, United States
Malaria is the most important parasitic disease in the world, killing over half a million people a year. Globally, we have made gains toward eliminating this disease but our progress has stalled in the last decade. Much of our control efforts and predictive models are focused to a few well understood systems such as Plasmodium falciparum transmission by species within the Anopheles gambiae complex. However, in many areas of the world, variation in the diversity of mosquito vectors significantly shape malaria transmission dynamics, seasonal persistence, and geographic range. This perspectives piece will focus on vectors of human malaria parasites and the impact of vector diversity on disease transmission by exploring how: 1) Anopheles species richness and abundance influence daily malaria transmission, and how differences in host feeding behavior and vector competence within a species-rich vector community influence local transmission, 2) diverse species contribute to persistence of malaria across seasons and spread through different ecotypes, and 3) species-specific parasite-vector interactions determine the genotypes of the malaria parasite that are successfully transmitted and shape parasite population structure. Finally, we will discuss future areas of research that should be prioritized toward understanding a more complete picture of malaria transmission dynamics in the context of diverse vector species.
Introduction
Malaria is one of the most deadly infectious disease in the world, with a third of the human population at risk of infection. Human malaria parasites are transmitted by Anopheles mosquitoes. There are around 500 different species of anophelines, about 100 of which have been implicated in malaria transmission (Kiszewski et al., 2004; Sinka et al., 2012). Research on malaria vectors is focused almost exclusively on groups of species that we consider to be primary vectors, those that we believe are responsible for transmitting the majority of infections. These primary vector species often have high vector competence, indoor resting and feeding behaviors, and a propensity to feed on humans over other hosts. In the last decade, there has been an increasing recognition of the role of understudied vector species in sustaining malaria transmission, including species that bite and rest outdoors and have more generalist feeding habits (Antonio-Nkondjio et al., 2006; Fornadel et al., 2011; Edwards et al., 2019a).
Malaria risk can change throughout the day, over seasons, and across different types of landscapes. Changes in risk of infection are largely driven by the abundance and biting behaviors of malaria vector species. Human exposure to malaria infectious mosquito bites is determined by a number of factors including densities of local vector mosquitoes, how often those mosquitoes feed on humans, and how well malaria parasites can develop in local vectors. In many endemic regions, malaria prevalence is strongly associated with seasonal conditions, like increased rainfall, that provide abundant breeding habitats for mosquito larvae and result in an increased abundance of adult Anopheles mosquitoes. Different communities of Anopheles species have specialized to exploit different types of habitats. Land use change has resulted in increasingly fragmented landscapes, bringing humans into closer contact with diverse outdoor-biting vector species in high abundance in forest and forest fringe habitats as well as those that have recently adapted to breeding in agricultural sites. In regions of rapid deforestation, particularly in South America and Southeast Asia, people involved in wood harvesting operations experience an increased risk of exposure to infectious bites (Cui et al., 2012; da Silva-Nunes et al., 2012). Exposed forest workers can also bring malaria parasites back into their communities where there are local vectors capable of transmission, initiating local outbreaks of infection (Sluydts et al., 2014; Edwards et al., 2019a). As humans move through different environments, the diversity of anophelines across these landscapes provide opportunities for malaria transmission and spread.
The life cycle of malaria parasites is complex, with multiple distinct life stages in the human and mosquito hosts. While malaria parasites build to huge population sizes in a human host, particularly during blood stages, very few mosquito-infective sexual stage gametocytes are ultimately picked up by a suitable mosquito. Malaria parasites must then persist through multiple life stages in the vector, including invasion, replicative development, and escape from multiple mosquito tissues. The most dramatic bottlenecks in parasite population size occur within the mosquito (Alavi et al., 2003), so slight differences in the success or failure of parasite development during these mosquito stages ultimately determine the parasite populations that are successfully transmitted. Different Anopheles species have been shown to be variably susceptible to infection by different populations of Plasmodium falciparum and P. vivax, the two species of human malaria that cause the greatest disease burden worldwide (Zollner et al., 2006; Molina-Cruz et al., 2012; Goupeyou-Youmsi et al., 2020). To combat malaria, we need to understand the interaction of human malaria parasites and the many different Anopheles species that transmit them.
Anopheles species richness and abundance can increase daily malaria transmission
Vector species diversity complicates our estimates of transmission as key descriptive features that enable us to predict malaria transmission risk are not well described in understudied malaria vectors (Smith and Ellis McKenzie, 2004; Lobo et al., 2015). These include measures of vector competence, the innate susceptibility of a single species or population to malaria infection, as well as measures of host preference, population density, daily survival rate, and how long it takes for a mosquito to become infectious after taking an infected bloodmeal (extrinsic incubation period). Vectors that are not as competent can be extremely abundant and biting at high enough rates to sustain transmission (Sallum et al., 2019). Studies have shown that in some environments with multiple Anopheles species, any one of those species could sustain malaria transmission (Billingsley and Sinden, 1997; Kweyamba et al., 2025). The risk of malaria exposure from multiple vector species in a single place is additive, increasing the total risk of daily exposure of one person to potentially infectious bites.
Our lack of information about understudied species perpetuates itself, with scientists necessarily expending limited resources on known and well-studied vector species, ultimately dismissing vectors that we have very little data about. Locations across the tropics have diverse communities of anophelines, many of which are capable of transmitting malaria (Kiszewski et al., 2004; Antonio-Nkondjio et al., 2006; Cui et al., 2012; Sriwichai et al., 2016; Kyalo et al., 2017; Davidson et al., 2020; Lee et al., 2022; Kweyamba et al., 2025). Randomized screening of Anopheles mosquitoes collected in medium and high transmission areas has resulted in the detection of more than a dozen species in a single location having malaria parasites in their salivary glands, being potentially infectious (Lobo et al., 2015; St Laurent et al., 2016a; St Laurent et al., 2016b; Mustapha et al., 2021). Studies of diverse vector communities in the Kenya highlands and Zambia showed that species considered secondary or non-vector species had a sporozoite prevalence comparable or exceeding those in species considered primary vectors from the same collections (Lobo et al., 2015; St Laurent et al., 2016a). Due to our lack of data about natural infection rates in most Anopheles species, we cannot predict the role of diverse outdoor biting species in sustaining infection, representing a gap in our understanding of malaria transmission.
Differences in host feeding behavior and vector competence influence local transmission and enable diverse species to avoid vector control
Malaria control is often only targeted at a few malaria vector species that are regularly interacting with humans. Vector control measures that are centered around human households like long lasting insecticide-treated nets (LLINs) and indoor residual spray (IRS) only impact Anopheles species that bite humans in and around houses. Diverse species that bite humans outdoors can act as reservoirs for transmission while also avoiding common interventions centered around human habitation, such as LLINs and IRS (Cooke et al., 2015; Sherrard-Smith et al., 2019).
A diverse vector community is also more resilient to control. During trials of high coverage distribution of LLINs in Tanzania, malaria transmission shifted from one anthropophilic vector species An. gambiae transmitting near houses to several species of exophilic vectors, An. funestus and An. arabiensis, that then sustained malaria transmission outdoors (Russell et al., 2011). Shifts in vector populations in response to LLIN and ITN coverage from primary vectors biting indoors to other species biting outdoors have been observed in other high transmission areas across Africa (Reddy et al., 2011; Sherrard-Smith et al., 2019). The presence of outdoor biting species can further extend transmission risk outside of areas of human habitation (Figure 1C) (Edwards et al., 2019b), and outdoor behaviors make these species more difficult to monitor.
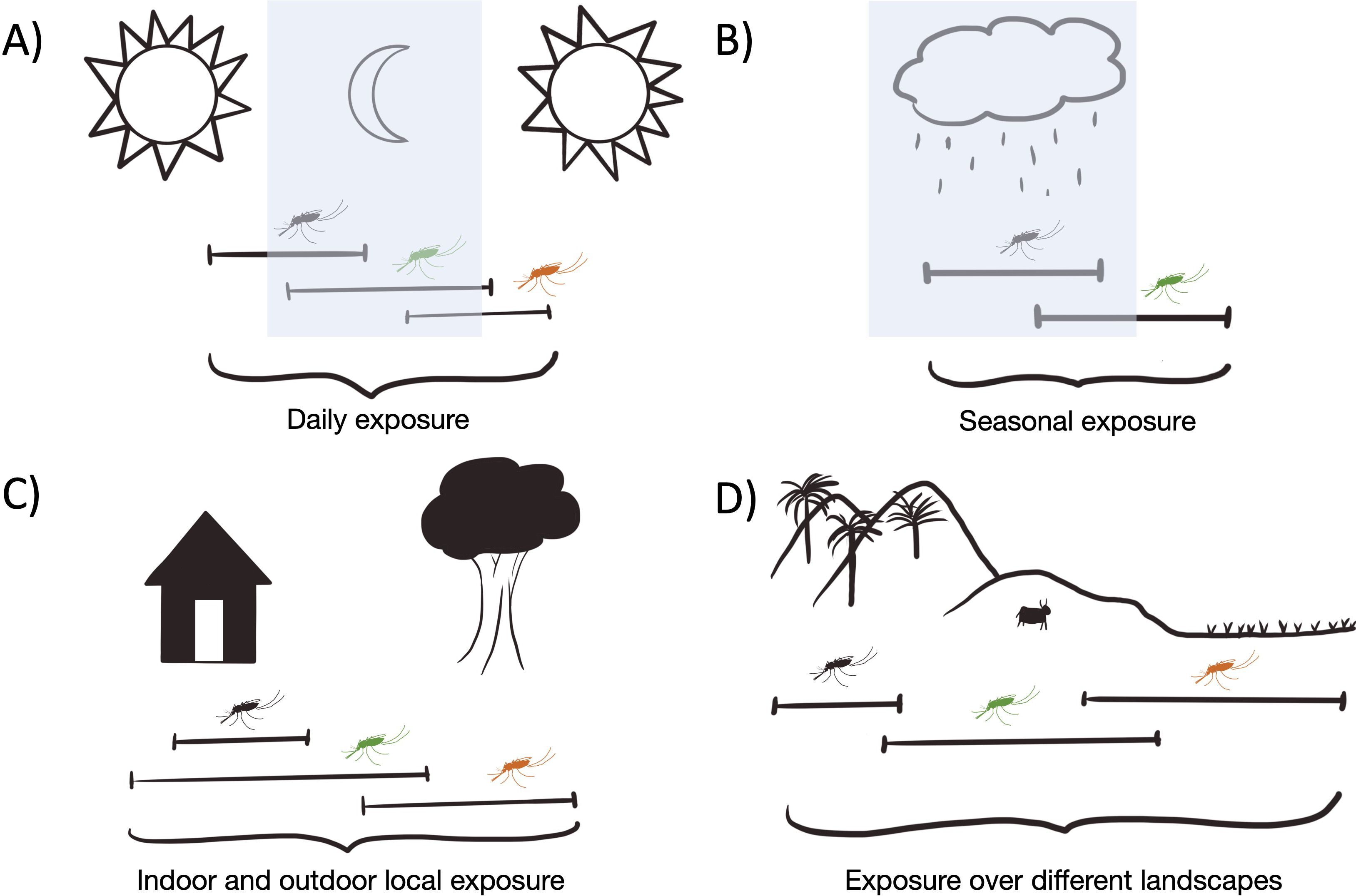
Figure 1. Diverse malaria vectors extend exposure to potentially infectious bites over (A) hours of the day, (B) seasons, (C) indoor and outdoor local areas, and (D) different landscape types.
Many outdoor-biting species tend to be more generalist in their feeding behavior, exhibiting zoophilic or opportunistic feeding. Mosquitoes that are generalists only need to have bitten an infected human in their first few bloodmeals and survive long enough to bite a human again once they are infectious to transmit parasites. Occasional biting of humans may help these generalist mosquitoes avoid the killing or fitness reducing effects of insecticidal human-centered interventions, as well as increasing the risk of zoonotic disease transmission (Hawkes et al., 2019). Generalist feeding and zoophilic mosquitoes can have high vector competence. A study of East African vectors showed that species with substantially different human host preferences can have similar human-infective sporozoite loads from infections (Kweyamba et al., 2025). We currently have very little data about the vector competence of most Anopheles species.
Species-rich vector communities exhibit a range of biting behaviors. Differences in the timing of peak biting by different Anopheles species can extend transmission risk through different parts of the night, and even into daytime hours (Figure 1A). Species that bite during dawn and dusk hours when people are active present an increased risk for infection and a gap in protection from LLINs or IRS (Cooke et al., 2015; Mwema et al., 2022). In Southeast Asia, multiple forest species have been found to exhibit biting behavior during the day, unusual for Anopheles mosquitoes (Vantaux et al., 2021; Boyer et al., 2025). Daytime biting of forest mosquitoes endangers workers harvesting wood and others who work in forest-fringe areas. This diversity in the timing of biting by different species increases the risk of infectious bites per day.
Diverse species contribute to persistence of malaria across seasons and spread through different ecotypes
Malaria transmission risk can change throughout the year. The number of malaria cases is often strongly associated with seasons where Anopheles population densities are high. While populations of individual species can sustain malaria transmission, combinations of species enable an expanded transmission season, and stabilize malaria transmission across seasons, including an increased risk multiple infections for an individual per year (Bompard et al., 2020) (Figure 1B). Since anopheline species respond to environmental changes differently, a high diversity of Anopheles mosquitoes can contribute to an extended malaria transmission season for multiple human malaria parasite species. In South Asia, milder dry seasons and large stretches of forested regions sustain many different Anopheles species year-round, with continual malaria transmission (Tainchum et al., 2014; Sriwichai et al., 2016; Bourke et al., 2018; Al-Amin et al., 2023; Boyer et al., 2025). In regions with a more extreme dry seasons, diversity of vector species can extend malaria transmission through dry periods compared to areas with fewer anopheline species where transmission might be interrupted (Cross et al., 2022). In Zambia, An. arabiensis and An. funestus were found to sustain malaria transmission through extreme drought when the primary vector population of An. gambiae had been decimated (Kent et al., 2007).
Combinations of species across landscapes expand the geographic range of malaria transmission (Kiszewski et al., 2004)(Figure 1D). Variations in abundance of difference species has been associated with changes in land use (Hii et al., 1997; da Silva-Nunes et al., 2012). Classic examples include vector species that occupy specific and sometimes overlapping niches along an altitudinal or land use gradient (Overgaard et al., 2003). For example, in a single village in Northeastern Cambodia, someone would have a risk of exposure to infectious bites from highly competent An. dirus in the forest, An. barbirostris and An. maculatus in forest-fringe areas, and species like An. minimus and An. aconitus that take advantage of permanently available larval habitats in rice cultivation near human habitation (Sinka et al., 2011). Outdoor and generalist feeding anophelines that exploit rice paddies and other agricultural plots come into regular contact with humans and other hosts and can emerge in high numbers and persist across extreme dry periods.
One pressing issue of land use change is deforestation, which can create patchy forest plots and developed landscapes that also bring humans in closer regular contact with competent forest vectors that are sometimes highly abundant (Hawkes et al., 2019; Oliveira et al., 2021). In Bangladesh, vector community composition was distinct between forested and non-forested ecotypes (Hawkes et al., 2019), including differences in P. falciparum and P. knowlesi infection rates. Another study in Thailand showed that mosquito abundance and diversity was highest at forest fringe sites (Tainchum et al., 2014), with high potential for contact between humans and vector mosquitoes. Land use change in the Amazon has been a major driver of malaria transmission risk, with increases in vector species diversity and abundance associated with recently deforested areas where new larval habitats are created (da Silva-Nunes et al., 2012). In these shifting landscapes, diverse vector species can maintain malaria transmission (Cross et al., 2022).
Species-specific vector-parasite interactions determine the genotypes of the malaria parasites that are successfully transmitted and shape parasite population structure
The most extreme population bottlenecks for the parasite occur within the mosquito (Alavi et al., 2003; Soontarawirat et al., 2017). Parasite development in mosquito tissues is largely determined by tissue-specific immune responses, some of which target Plasmodium parasites. Slight differences in parasite development at these different mosquito stages can determine whether a parasite gets successfully transmitted by that mosquito or not, and which parasites are transmitted (Figure 2). Sexual reproduction of parasites also occurs within the mosquito, which can increase the genetic diversity of the parasite population. This vector-specific filter of individual parasites shapes the population structure of parasites that are then circulating in humans (Annan et al., 2007; Das et al., 2017; Goupeyou-Youmsi et al., 2020). The selective effect of vectors on parasite populations may be more pronounced under low transmission conditions.
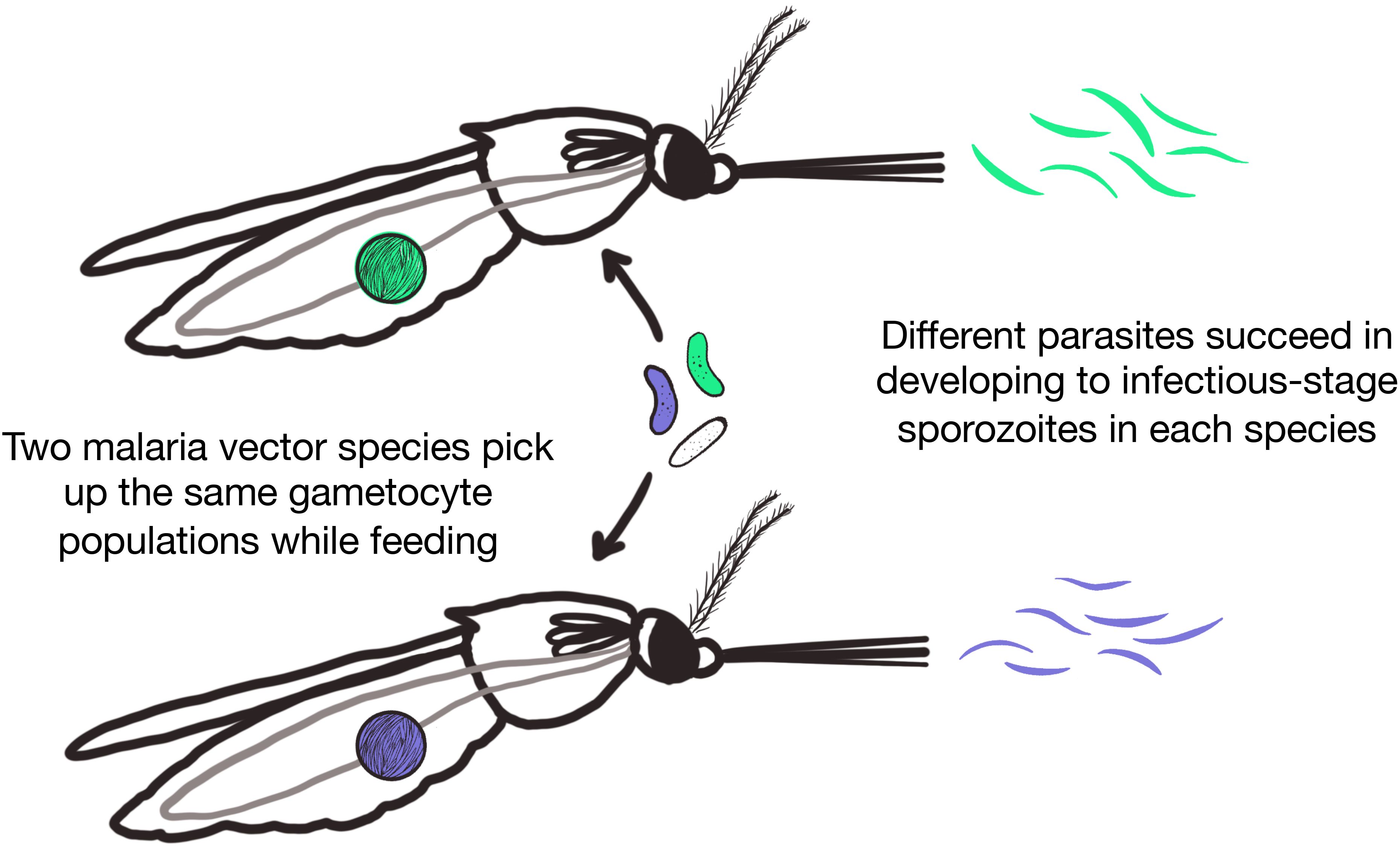
Figure 2. Diverse vector species can differentially select for the parasite species and genotypes that get transmitted.
Though species can have varying vector competence, Anopheles are often competent for multiple human malaria parasite species. Similarly, all five human Plasmodium species are able to infect a wide range of Anopheles species (Kiszewski et al., 2004; Sinka et al., 2012; Neafsey et al., 2015). Infections using patient isolates showed successful infections in multiple highly diverged species (St. Laurent et al., 2015; Sriwichai et al., 2016). Field and lab experiments have shown that different species of mosquitoes can select for human malaria parasite species and strains (Somboon et al., 1994; Joy et al., 2008; White et al., 2011; Goupeyou-Youmsi et al., 2020). In other malaria species, parasite lineages are differentially transmitted by different mosquito species, sometimes selected for through different immune mechanisms (Zollner et al., 2006; Jaramillo-Gutierrez et al., 2009; Das et al., 2017; Gutiérrez-López et al., 2020). With multiple species selecting for different parasites, multiple vectors involved in transmission can contribute to and sustain the genetic diversity of Plasmodium populations.
Conclusions
Flexibility in vector behaviors make species rich vector communities able to adapt to our most effective vector control tools and increases potential exposure to infectious bites over the course of a day, over the course of a year, and in different types of habitats. Diverse vector species represent a black box for malaria control, with very little scientific baseline information about dozens of species that contribute to malaria transmission and very few tools available to address malaria transmission by outdoor and generalist feeding species. A diverse vector community is also more resilient to insecticide-based control tools, with a reservoir of genetic diversity, different molecular mechanisms of coping with chemical and oxidative stressors, and adaptive behaviors that avoid insecticidal interventions. These species represent a dangerous unknown in our estimations of persistence of malaria in different types of habitats and the spread of parasites and vectors to new locations.
Vector diversity presents a challenge for both established vector control and new control tools that are being developed. Species that are genetically divergent from major malaria vector species will not be effectively controlled by new developments in gene drive and other genetic control tools that typically target conserved gene sequences within a well-studied primary vector species. Diverse species should be represented in our genetic and genomic studies of the natural genetic variation of malaria vectors. Sequence data from diverse Anopheles species will allow for more accurate species identification and improved molecular monitoring. More comprehensive field studies are needed to address gaps in our understanding of malaria transmission, including crucial information about natural infection rates of different species and their distributions. The presence of multiple species and shifts in vector populations can make monitoring and evaluating the impact of control tools more complicated (Máquina et al., 2024). Utilizing multiple collection techniques that capture outdoor species and species with different feeding behaviors will capture a broader range of anophelines. A regular assessment of species composition, with random screening of all collected species for infection, should be a part of malaria control program activities where vector control tools are being evaluated.
Our understanding of malaria transmission is also limited by the strains of anophelines and Plasmodium that have been studied. Most malaria transmission experiments in the lab involve Anopheles colonies of primary vector species that have been kept in the lab for decades, undergoing constant population bottlenecks that limit their genetic diversity and any similarity to wild populations. We need to expand our studies of vector-parasite interactions to include actively circulating malaria parasites and vectors that represent current real-world populations, including understudied vector species. Vector competence is one important transmission metric that can be evaluated in the lab. Malaria transmission models currently use limited infection data from An. gambiae s.l. or An. stephensi to predict infection parameters for all other anophelines. Infection studies including diverse Anopheles species would help to disentangling the role that different vector species play in malaria transmission, providing better comparative metrics for estimating infection risk across species and across environments with different species compositions.
Diverse vector species make malaria eradication much more difficult to achieve (Fornace et al., 2021). Multiple species that are not well understood sustain malaria transmission through harsh environmental conditions and over seasons, in habitats that are not associated with humans, and through times of the day when people are not protected by bednets or other vector control interventions. These species contribute to what is known as residual malaria transmission, the transmission that remains after highly effective vector control tools have been rolled out to high coverage. It is essential that we work to better understand malaria transmission by diverse outdoor species, as these will harbor and sustain the last reservoirs of infection when we reach the endgame of global malaria eradication.
Data availability statement
The original contributions presented in the study are included in the article/supplementary material. Further inquiries can be directed to the corresponding author.
Author contributions
BS: Writing – review & editing, Writing – original draft, Visualization.
Funding
The author(s) declare that no financial support was received for the research and/or publication of this article.
Conflict of interest
The author declares that the research was conducted in the absence of any commercial or financial relationships that could be construed as a potential conflict of interest.
Generative AI statement
The author(s) declare that no Generative AI was used in the creation of this manuscript.
Publisher’s note
All claims expressed in this article are solely those of the authors and do not necessarily represent those of their affiliated organizations, or those of the publisher, the editors and the reviewers. Any product that may be evaluated in this article, or claim that may be made by its manufacturer, is not guaranteed or endorsed by the publisher.
References
Al-Amin H. M., Rodriguez I., Phru C. S., Khan W. A., Haque R., Nahlen B. L., et al. (2023). Composition of Anopheles species and bionomic characteristics over the peak malaria transmission season in Bandarban, Bangladesh. Malaria J. 22, 176. doi: 10.1186/s12936-023-04614-2
Alavi Y., Arai M., Mendoza J., Tufet-Bayona M., Sinha R., Fowler K., et al. (2003). The dynamics of interactions between Plasmodium and the mosquito: a study of the infectivity of Plasmodium berghei and Plasmodium gallinaceum, and their transmission by Anopheles stephensi, Anopheles Gambiae and Aedes aEgypti. Int. J. parasitol 33, 933–943. doi: 10.1016/S0020-7519(03)00112-7
Annan Z., Durand P., Ayala F. J., Arnathau C., Awono-Ambene P., Simard F., et al. (2007). Population genetic structure of Plasmodium falciparum in the two main African vectors, Anopheles Gambiae and Anopheles funestus. Proc. Natl. Acad. Sci. United States America 104, 7987–7992. doi: 10.1073/pnas.0702715104
Antonio-Nkondjio C., Kerah C. H., Simard F., Awono-Ambene P., Chouaibou M., Tchuinkam T., et al. (2006). Complexity of the malaria vectorial system in Cameroon: contribution of secondary vectors to malaria transmission. J. Med. entomol 43, 1215–1221. doi: 10.1093/jmedent/43.6.1215
Billingsley P. and Sinden R. (1997). Determinants of malaria-mosquito specificity. Parasitol Today 13, 297–301. doi: 10.1016/S0169-4758(97)01094-6
Bompard A., Da D. F., Yerbanga S. R., Morlais I., Awono-Ambéné P. H., Dabiré R. K., et al. (2020). High Plasmodium infection intensity in naturally infected malaria vectors in Africa. Int. J. parasitol 50, 985–996. doi: 10.1016/j.ijpara.2020.05.012
Bourke B. P., Conn J. E., de Oliveira T. M., Chaves L. S., Bergo E. S., Laporta G. Z., et al. (2018). Exploring malaria vector diversity on the Amazon Frontier. Malaria J. 17, 1–17. doi: 10.1186/s12936-018-2483-2
Boyer S., Doeurk B., Rakotonirina A., Chy S., Vong C., Piv E., et al. (2025). Anopheles mosquitoes in Mondulkiri forest, Cambodia: abundance, distribution, seasonal patterns and Plasmodium prevalence. Malaria J. 24, 6. doi: 10.1186/s12936-024-05166-9
Cooke M. K., Kahindi S. C., Oriango R. M., Owaga C., Ayoma E., Mabuka D., et al. (2015). ‘A bite before bed’: exposure to malaria vectors outside the times of net use in the highlands of western Kenya. Malaria J. 14, 259. doi: 10.1186/s12936-015-0766-4
Cross D. E., Healey A. J. E., McKeown N. J., Thomas C. J., Macarie N. A., Siaziyu V., et al. (2022). Temporally consistent predominance and distribution of secondary malaria vectors in the Anopheles community of the upper Zambezi floodplain. Sci. reports 12, 240. doi: 10.1038/s41598-021-04314-4
Cui L., Yan G., Sattabongkot J., Cao Y., Chen B., Chen X., et al. (2012). Malaria in the Greater Mekong Subregion: heterogeneity and complexity. Acta tropica 121, 227–239. doi: 10.1016/j.actatropica.2011.02.016
Das S., Muleba M., Stevenson J. C., Pringle J. C., and Norris D. E. (2017). Beyond the entomological inoculation rate: characterizing multiple blood feeding behavior and Plasmodium falciparum multiplicity of infection in Anopheles mosquitoes in northern Zambia. Parasites vectors 10, 45. doi: 10.1186/s13071-017-1993-z
da Silva-Nunes M., Moreno M., Conn J. E., Gamboa D., Abeles S., Vinetz J. M., et al. (2012). Amazonian malaria: asymptomatic human reservoirs, diagnostic challenges, environmentally driven changes in mosquito vector populations, and the mandate for sustainable control strategies. Acta tropica 121, 281–291. doi: 10.1016/j.actatropica.2011.10.001
Davidson J. R., Wahid I., Sudirman R., Small S. T., Hendershot A. L., Baskin R. N., et al. (2020). Molecular analysis reveals a high diversity of Anopheles species in Karama, West Sulawesi, Indonesia. Parasites vectors 13, 1–11. doi: 10.1186/s13071-020-04252-6
Edwards H. M., Chinh V. D., Le Duy B., Thanh P. V., Thang N. D., Trang D. M., et al. (2019a). Characterising residual malaria transmission in forested areas with low coverage of core vector control in central Viet Nam. Parasites vectors 12, 454. doi: 10.1186/s13071-019-3695-1
Edwards H. M., Sriwichai P., Kirabittir K., Prachumsri J., Chavez I. F., and Hii J. (2019b). Transmission risk beyond the village: entomological and human factors contributing to residual malaria transmission in an area approaching malaria elimination on the Thailand-Myanmar border. Malaria J. 18, 221. doi: 10.1186/s12936-019-2852-5
Fornace K. M., Diaz A. V., Lines J., and Drakeley C. J. (2021). Achieving global malaria eradication in changing landscapes. Malaria J. 20, 69. doi: 10.1186/s12936-021-03599-0
Fornadel C. M., Norris L. C., Franco V., and Norris D. E. (2011). Unexpected anthropophily in the potential secondary malaria vectors Anopheles coustani s.l. and Anopheles squamosus in Macha, Zambia. Vector Borne Zoonotic Dis. 11, 1173–1179. doi: 10.1089/vbz.2010.0082
Goupeyou-Youmsi J., Rakotondranaivo T., Puchot N., Peterson I., Girod R., Vigan-Womas I., et al. (2020). Differential contribution of Anopheles coustani and Anopheles arabiensis to the transmission of Plasmodium falciparum and Plasmodium vivax in two neighbouring villages of Madagascar. Parasites vectors 13, 430. doi: 10.1186/s13071-020-04282-0
Gutiérrez-López R., Martínez-de la Puente J., Gangoso L., Soriguer R., and Figuerola J. (2020). Plasmodium transmission differs between mosquito species and parasite lineages. Parasitology 147, 441–447. doi: 10.1017/S0031182020000062
Hawkes F. M., Manin B. O., Cooper A., Daim S., Homathevi R., Jelip J., et al. (2019). Vector compositions change across forested to deforested ecotones in emerging areas of zoonotic malaria transmission in Malaysia. Sci. reports 9, 13312. doi: 10.1038/s41598-019-49842-2
Hii J. L., Smith T., Mai A., Mellor S., Lewis D., Alexander N., et al. (1997). Spatial and temporal variation in abundance of Anopheles (Diptera: Culicidae) in a malaria endemic area in Papua New Guinea. J. Med. entomol 34, 193–205. doi: 10.1093/jmedent/34.2.193
Jaramillo-Gutierrez G., Rodrigues J., Ndikuyeze G., Povelones M., Molina-Cruz A., and Barillas-Mury C. (2009). Mosquito immune responses and compatibility between Plasmodium parasites and anopheline mosquitoes. BMC microbiol 9, 154. doi: 10.1186/1471-2180-9-154
Joy D. A., Gonzalez-Ceron L., Carlton J. M., Gueye A., Fay M., McCutchan T. F., et al. (2008). Local adaptation and vector-mediated population structure in plasmodium vivax malaria. Mol. Biol. evolution 25, 1245–1252. doi: 10.1093/molbev/msn073
Kent R. J., Thuma P. E., Mharakurwa S., and Norris D. E. (2007). Seasonality, blood feeding behavior, and transmission of Plasmodium falciparum by Anopheles arabiensis after an extended drought in southern Zambia. Am. J. Trop. Med. hygiene 76, 267–274. doi: 10.4269/ajtmh.2007.76.267
Kiszewski A., Mellinger A., Spielman A., Malaney P., Sachs S. E., and Sachs J. (2004). A global index representing the stability of malaria transmission. Am. J. Trop. Med. hygiene 70, 486–498. doi: 10.4269/ajtmh.2004.70.486
Kweyamba P. A., Hofer L. M., Kibondo U. A., Mwanga R. Y., Sayi R. M., Matwewe F., et al. (2025). Contrasting vector competence of three main East African Anopheles malaria vector mosquitoes for Plasmodium falciparum. Sci. reports 15, 2286. doi: 10.1038/s41598-025-86409-w
Kyalo D., Amratia P., Mundia C. W., Mbogo C. M., and Coetzee M. (2017). Snow RW. A geo-coded inventory of anophelines in the Afrotropical Region south of the Sahara: 1898-2016. Wellcome Open Res. 2. doi: 10.12688/wellcomeopenres.12187.1.
Lee S. Y., Kim H. C., Klein T. A., Ryu J., Won M. H., Choi J. W., et al. (2022). Species diversity of Anopheles mosquitoes and Plasmodium vivax infection rates, Gyeonggi Province, Republic of Korea during 2020. J. Med. entomol 59, 1778–1786. doi: 10.1093/jme/tjac086
Lobo N. F., St. Laurent B., Sikaala C. H., Hamainza B., Chanda J., Chinula D., et al. (2015). Unexpected diversity of Anopheles species in Eastern Zambia: implications for evaluating vector behavior and interventions using molecular tools. Sci. reports 5, 17952. doi: 10.1038/srep17952
Máquina M., Opiyo M. A., Cuamba N., Marrenjo D., Rodrigues M., Armando S., et al. (2024). Multiple Anopheles species complicate downstream analysis and decision-making in a malaria pre-elimination area in southern Mozambique. Malaria J. 23, 23. doi: 10.1186/s12936-024-04842-0
Molina-Cruz A., DeJong R. J., Ortega C., Haile A., Abban E., Rodrigues J., et al. (2012). Some strains of Plasmodium falciparum, a human malaria parasite, evade the complement-like system of Anopheles Gambiae mosquitoes. Proc. Natl. Acad. Sci. 109, E1957–E1E62. doi: 10.1073/pnas.1121183109
Mustapha A. M., Musembi S., Nyamache A. K., Machani M. G., Kosgei J., Wamuyu L., et al. (2021). Secondary malaria vectors in western Kenya include novel species with unexpectedly high densities and parasite infection rates. Parasites vectors 14, 252. doi: 10.1186/s13071-021-04748-9
Mwema T., Lukubwe O., Joseph R., Maliti D., Iitula I., Katokele S., et al. (2022). Human and vector behaviors determine exposure to Anopheles in Namibia. Parasites vectors 15, 436. doi: 10.1186/s13071-022-05563-6
Neafsey D. E., Waterhouse R. M., Abai M. R., Aganezov S. S., Alekseyev M. A., Allen J. E., et al. (2015). Mosquito genomics. Highly evolvable malaria vectors: the genomes of 16 Anopheles mosquitoes. Science 347, 1258522. doi: 10.1126/science.1258522
Oliveira T. M., Laporta G. Z., Bergo E. S., Chaves L. S. M., Antunes J. L. F., Bickersmith S. A., et al. (2021). Vector role and human biting activity of Anophelinae mosquitoes in different landscapes in the Brazilian Amazon. Parasites vectors 14, 236. doi: 10.1186/s13071-021-04725-2
Overgaard H. J., Ekbom B., Suwonkerd W., and Takagi M. (2003). Effect of landscape structure on anopheline mosquito density and diversity in northern Thailand: Implications for malaria transmission and control. Landscape ecol 18, 605–619. doi: 10.1023/A:1026074910038
Reddy M. R., Overgaard H. J., Abaga S., Reddy V. P., Caccone A., Kiszewski A. E., et al. (2011). Outdoor host seeking behaviour of Anopheles Gambiae mosquitoes following initiation of malaria vector control on Bioko Island, Equatorial Guinea. Malaria J. 10, 184. doi: 10.1186/1475-2875-10-184
Russell T. L., Govella N. J., Azizi S., Drakeley C. J., Kachur S. P., and Killeen G. F. (2011). Increased proportions of outdoor feeding among residual malaria vector populations following increased use of insecticide-treated nets in rural Tanzania. Malaria J. 10, 80. doi: 10.1186/1475-2875-10-80
Sallum M. A. M., Conn J. E., Bergo E. S., Laporta G. Z., Chaves L. S. M., Bickersmith S. A., et al. (2019). Vector competence, vectorial capacity of Nyssorhynchus darlingi and the basic reproduction number of Plasmodium vivax in agricultural settlements in the Amazonian Region of Brazil. Malaria J. 18, 117. doi: 10.1186/s12936-019-2753-7
Sherrard-Smith E., Skarp J. E., Beale A. D., Fornadel C., Norris L. C., Moore S. J., et al. (2019). Mosquito feeding behavior and how it influences residual malaria transmission across Africa. Proc. Natl. Acad. Sci. United States America 116, 15086–15095. doi: 10.1073/pnas.1820646116
Sinka M. E., Bangs M. J., Manguin S., Chareonviriyaphap T., Patil A. P., Temperley W. H., et al. (2011). The dominant Anopheles vectors of human malaria in the Asia-Pacific region: occurrence data, distribution maps and bionomic precis. Parasites vectors 4, 89. doi: 10.1186/1756-3305-4-89
Sinka M. E., Bangs M. J., Manguin S., Rubio-Palis Y., Chareonviriyaphap T., Coetzee M., et al. (2012). A global map of dominant malaria vectors. Parasites vectors 5, 69. doi: 10.1186/1756-3305-5-69
Sluydts V., Heng S., Coosemans M., Van Roey K., Gryseels C., Canier L., et al. (2014). Spatial clustering and risk factors of malaria infections in Ratanakiri Province, Cambodia. Malaria J. 13, 387. doi: 10.1186/1475-2875-13-387
Smith D. L. and Ellis McKenzie F. (2004). Statics and dynamics of malaria infection in Anopheles mosquitoes. Malaria J. 3, 1–14. doi: 10.1186/1475-2875-3-13
Somboon P., Suwonkerd W., and Lines J. D. (1994). Susceptibility of Thai zoophilic Anophelines and suspected malaria vectors to local strains of human malaria parasites. Southeast Asian J. Trop. Med. Public Health 25, 766–770.
Soontarawirat I., Andolina C., Paul R., Day N. P. J., Nosten F., Woodrow C. J., et al. (2017). Plasmodium vivax genetic diversity and heterozygosity in blood samples and resulting oocysts at the Thai–Myanmar border. Malaria J. 16, 355. doi: 10.1186/s12936-017-2002-x
Sriwichai P., Samung Y., Sumruayphol S., Kiattibutr K., Kumpitak C., Payakkapol A., et al. (2016). Natural human Plasmodium infections in major Anopheles mosquitoes in western Thailand. Parasites vectors 9, 17. doi: 10.1186/s13071-016-1295-x
St Laurent B., Cooke M., Krishnankutty S. M., Asih P., Mueller J. D., Kahindi S., et al. (2016a). Molecular characterization reveals diverse and unknown malaria vectors in the western Kenyan highlands. Am. J. Trop. Med. hygiene 94, 327–335. doi: 10.4269/ajtmh.15-0562
St. Laurent B., Miller B., Burton T. A., Amaratunga C., Men S., Sovannaroth S., et al. (2015). Artemisinin-resistant Plasmodium falciparum clinical isolates can infect diverse mosquito vectors of Southeast Asia and Africa. Nat. Commun. 6. doi: 10.1038/ncomms9614
St Laurent B., Oy K., Miller B., Gasteiger E. B., Lee E., Sovannaroth S., et al. (2016b). Cow-baited tents are highly effective in sampling diverse Anopheles malaria vectors in Cambodia. Malaria J. 15, 440. doi: 10.1186/s12936-016-1488-y
Tainchum K., Ritthison W., Chuaycharoensuk T., Bangs M. J., Manguin S., and Chareonviriyaphap T. (2014). Diversity of Anopheles species and trophic behavior of putative malaria vectors in two malaria endemic areas of northwestern Thailand. J. Vector Ecol. 39, 424–436. doi: 10.1111/jvec.12118
Vantaux A., Riehle M. M., Piv E., Farley E. J., Chy S., Kim S., et al. (2021). Anopheles ecology, genetics and malaria transmission in northern Cambodia. Sci. reports 11, 6458. doi: 10.1038/s41598-021-85628-1
White B. J., Lawniczak M. K., Cheng C., Coulibaly M. B., Wilson M. D., Sagnon N. F., et al. (2011). Adaptive divergence between incipient species of Anopheles Gambiae increases resistance to Plasmodium. Proc. Natl. Acad. Sci. 108, 244–249. doi: 10.1073/pnas.1013648108
Keywords: malaria, vector diversity, Plasmodium, Anopheles, transmission
Citation: St. Laurent B (2025) Mosquito vector diversity and malaria transmission. Front. Malar. 3:1600850. doi: 10.3389/fmala.2025.1600850
Received: 27 March 2025; Accepted: 25 April 2025;
Published: 15 May 2025.
Edited by:
Alena Pance, University of Hertfordshire, United KingdomReviewed by:
Colin Malcolm, University of Hertfordshire, United KingdomCopyright © 2025 St. Laurent. This is an open-access article distributed under the terms of the Creative Commons Attribution License (CC BY). The use, distribution or reproduction in other forums is permitted, provided the original author(s) and the copyright owner(s) are credited and that the original publication in this journal is cited, in accordance with accepted academic practice. No use, distribution or reproduction is permitted which does not comply with these terms.
*Correspondence: Brandy St. Laurent, YnM3NDRAY29ybmVsbC5lZHU=