- 1Medical ICU, Paris Cité University– Bichat University Hospital, Assistance Publique – Hôpitaux de Paris, Paris, France
- 2Inserm UMR 1137 – IAME Team 5 – Decision Sciences in Infectious Diseases, Control and Care INSERM/Paris Diderot, Sorbonne Paris Cité University, Paris, France
Fever can be viewed as an adaptive response to infection. Temperature control in sepsis is aimed at preventing potential harms associated with high temperature (tachycardia, vasodilation, electrolyte and water loss) and therapeutic hypothermia may be aimed at slowing metabolic activities and protecting organs from inflammation. Although high fever (>39.5°C) control is usually performed in critically ill patients, available cohorts and randomized controlled trials do not support its use to improve sepsis prognosis. Finally, both spontaneous and therapeutic hypothermia are associated with poor outcomes in sepsis.
1. Introduction
Fever (or pyrexia) is a common feature of sepsis and septic shock. Although multiple scientific societies have defined fever as a temperature above 38.2°C (1), the actual threshold varies widely between authors (2). Fever is most often a symptom of infection but at least 25% of hyperthermia in critical care are not infectious but of tumoral, ischemic or allergic nature (3). Fever has long been considered beneficial against infection, as many germs replicate less in high-temperature environments (4). However, very high temperatures can lead to organ damage or failure.
Temperature control in sepsis, on both sides of the spectrum, has been an unresolved issue for many years (5). Recent research into fever in sepsis has isolated groups of patients with different prognoses. This, combined with a better understanding of the microbiota, may lead us to consider the correct attitude toward the treatment of fever in a more personalized way.
In this review, we will summarize recent advances on fever in sepsis from a pathophysiological and clinical point of view.
2. Physiopathology
2.1. Physiopathology and cellular changes in fever
Body temperature is tightly regulated by the hypothalamus within a range of 0.2–0.4°C, with little circadian and ovarian variation. Homeostasis is maintained by an afferent, central and efferent system. The information comes from thermal receptors, located mainly in the skin. In the event of external or central temperature change, the hypothalamus activates efferent channels to maintain temperature by shivering, sweating or redistributing blood flow. Fever is often differentiated from hyperthermia without infection due to external heating, excessive heat production or inefficient heat loss. In hyperthermia, the hypothalamus attempts to maintain normothermia, but is overwhelmed. Fever is the result of an increase in the usual hypothalamic target or set point (usually around 37°C). The extra- and intracellular signaling leading to fever is illustrated in Figure 1, but the precise locations of action of the various molecules in the hypothalamus remain unclear (4, 6–8).
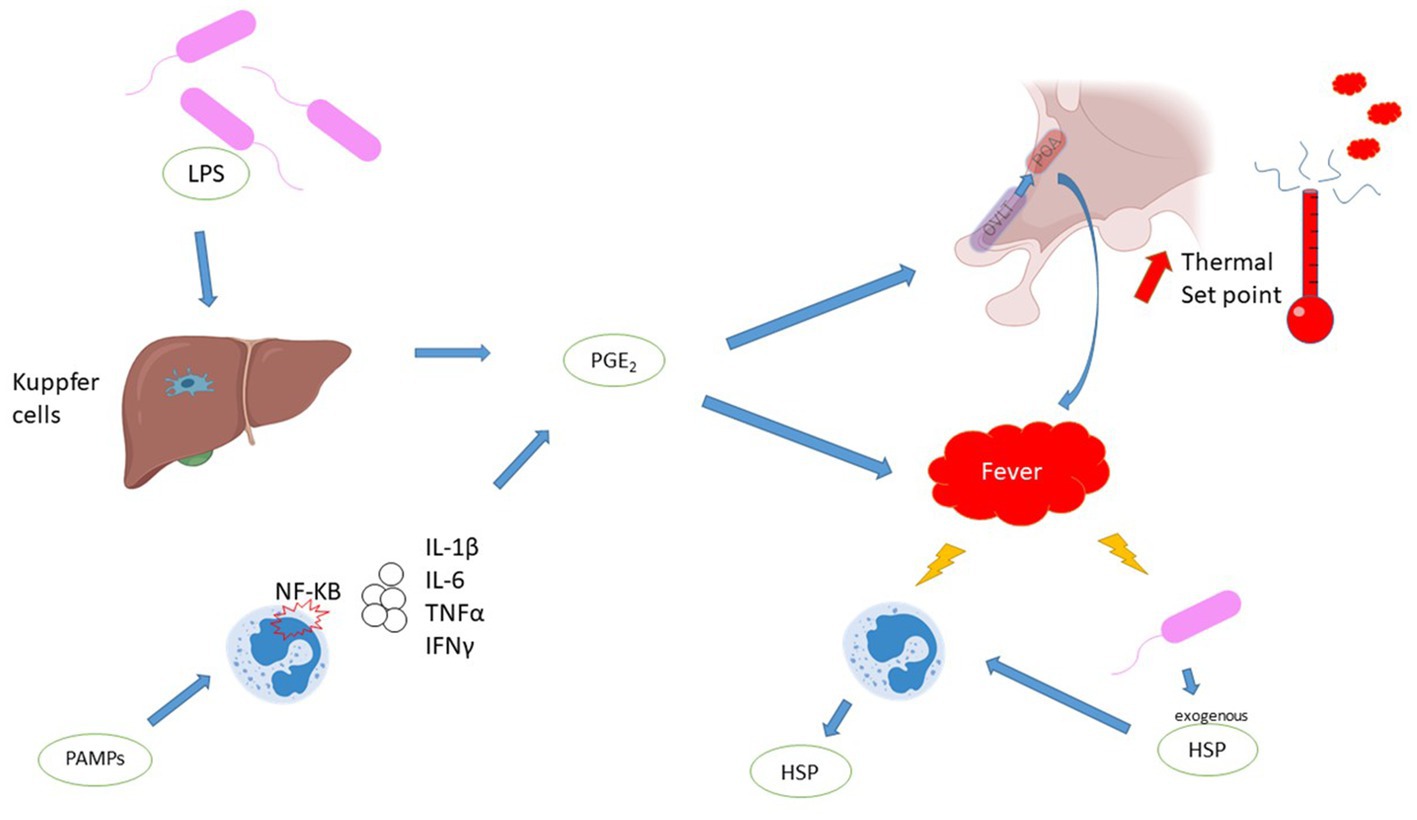
Figure 1. Mechanisms of fever. Comments: PGE2 is a major component of fever production. PGE2 induces a higher hypothalamus set point and promotes peripheral heat production. PGE2 is synthesized by two pathways: direct stimulation of Kuppfer cells by exotoxins, or stimulation of leukocytes via the Pathogen Associated Molecular Patterns /toll like receptor (PAMPs/TLR) pathway, leading to the production of endogenous pyrogens. Fever has multiple molecular consequences, Heat shock protein is a key response to fever is represented. Legends: HSP, heat shock protein; IFN, interferon; IL, interleukin; LPS, lipopolysaccharide; NF-KB, nuclear factor-kappa B; OVLT, organum vasculosum of the lamina terminalis; PAMPs, Pathogen Associated Molecular Patterns; PGE2, prostaglandin E2; POA, pre-optic area; TNF, tumor necrosis factor.
From a cellular point of view and based on in vitro experiments, the impact of fever is controversial. Higher temperatures inhibit the growth of Streptococcus pneumonia and Haemophilus influenzae (9, 10). In a mice pneumonia model, bacterial load was significantly lower in hyperthermic group (11). Susceptibility to antimicrobial agents is increased with temperature, especially when temperature is above 38.5°C (12). Antibiotics effects on Staphylococcal and Pseudomonas aeruginosa biofilms are better at higher temperatures (13, 14). On the host side, high temperatures are known to induce heat shock proteins (HSPs) which have cytoprotective properties. Fever promotes the production of HSPs by the pathogen, which in return are powerful activators of the host’s innate defense (15). Fever appears to result in an enhanced innate immune response, with greater recruitment of innate immune cells, improved neutrophil survival, better NETosis, increased production of reactive oxygen species, and reduced secretion of pro-inflammatory cytokines by neutrophils (11, 16, 17). Other data show effects on the adaptive immune system, with changes in CD4+ T cell differentiation and adaptive immune cell recruitment (18, 19).
But deleterious effects have also been described. Hyperthermia is cytotoxic, and direct cell death is described at 41°C (6). The enhanced innate immune response is accompanied by increased tissue damage, especially alveolar epithelial or neuronal damage (11, 20–22). Moreover, higher temperatures are accompanied by greater metabolism and higher oxygen consumption, a scarce resource for cells in sepsis and shock scenarios. For example, fever induces tachycardia, which increases cardiac oxygen consumption. High temperature favors arterial vasodilatation, inducing lower arterial pressure. Hyperthermia is an effective catalyst for coagulation. Underlying mechanisms could be increased tissue factor exposure from white blood cells and microparticles (23). Neutrophils, histones and NETosis are also likely to be initiators and facilitators of coagulation factor binding (23, 24).
2.2. Gut microbiota influence
Recently, growing knowledge in the field of microbiota has led to the question of whether gut microbiota could explain temperature heterogeneity during sepsis. A recent study isolated clusters of patients according to their temperature trajectories. Their intestinal microbiota was compared at admission to intensive care unit (ICU). The composition of the intestinal bacterial community was independently associated with individual temperature trajectories, and the mouse model reinforced the hypothesis (25). Certain taxa, such as Lachnospiraceae spp. may favor lower temperatures in sepsis. The same authors also demonstrated that this specific taxon is also present in lung microbiota, where it is associated with worse outcomes in critically ill patients (26).
In patients with COVID-19, fever was associated with altered intestinal microbiota, such as an increase in Enterococcus faecalis and Saccharomyces cerevisiae (27). In neutropenic fever, alteration and depletion of the gut microbiota may be involved through the loss of protective microbiota-derived metabolites (28, 29). The taxon Akkermansia is strongly implicated in the pathogenesis of neutropenic fever through mucus thinning (28, 30).
The interaction between the gut microbiota and the organism seems to be bidirectional. In a septic mouse model, the hosts adapted to maintain the gut microbiota. After LPS injection, small intestinal epithelial cells adapted by releasing fucose. The fucose was then consumed by the microbiota to sustain it. This phenomenon was associated with improved tolerance to infection (31).
3. The clinical impact and prognosis value of fever
3.1. Insights from non-septic situations
Heatstroke is the typical example of the harmful consequences of hyperthermia. The classic heatstroke showcases neurologic symptoms, respiratory alkalosis and in more severe cases liver dysfunction, renal failure, acute respiratory distress syndrome or even death (32). Another temperature related disease model is malignant hyperthermia. It is described as an adverse reaction during general anesthesia. The symptoms are many and have an interpatient variability (33).
In acute brain injury, fever is associated with worse outcomes (34) but target temperature management has failed show improvement in patients outcome (35, 36). Hyperthermia often occurs in cardiac arrest patients and is associated with a worse neurologic prognosis (37). Cardiac arrest management guidelines include active temperature control under 36°C in the first 24 h and fever prevention in the first 72 h (38).
Among patients admitted to the ICU without sepsis, those with the highest temperatures (>39.5°C) have the poorest prognosis (39, 40). The likely explanation is that they suffer from the negative effects of high body temperature without the alleged benefit in infectious diseases.
3.2. Fever in sepsis
The definition of fever in septic ICU patients varies according to ICU center worldwide with a median threshold of 38.2°C (IQR 38–38.5°C). The use of thermometers are protocolized in two third ICUs and a wide range of methods were reportedly used, with axillary, tympanic and urinary bladder sites as the most common as primary modalities (41).
Fever is reported in around 60% of septic patients in ICU (42). Approximately 10–30% of patients with sepsis may be hypothermic on admission (43–45). In a systematic review of 42 studies, mortality rate of septic patients with fever >38°C was 22.2% (CI, 19.2–25.5) which was higher, 31.2% (CI, 25.7–37.3), in normothermic patients, and it was the highest, 47.3% (CI, 38.9–55.7), in hypothermic patients (<36.0°C) (46). The-meta regression showed a strong negative linear correlation between temperature and mortality.
In contrast to non-septic patients, very high body temperature does not affect the prognosis of septic patients in the ICU (39, 40, 42, 45). The same applies to neutropenic patients admitted to the ICU, with or without hematological malignancy (47). Conversely, hypothermia is associated with a poor prognosis (40, 42, 44, 48) and a higher incidence of ICU-acquired infections (49). The deeper the hypothermia, the greater the effect. One reason for this could be a delay in the diagnosis and treatment of sepsis (50). Children, neonates and neutropenic populations also have higher mortality when hypothermic (47, 51). However, in elderly patients with sepsis, hypothermia is more frequent and not associated with mortality (52).
Interestingly, the temperature profile in sepsis can be related to outside temperature. In a German ICU population with sepsis, body temperature was independently related to outside temperature (42). High fever was more frequent when outside temperature was above 15°C, and hypothermia when it was below 4°C. This could lead to confounding bias in other studies when comparing fever and hypothermia, given that they can occur at unequal times of year. In support of this, one study shows that the incidence of nosocomial bacteremia increases with outside temperature (53).
By examining temperature trajectories, 4 phenotypes were described: rapid-resolution hyperthermia, slow-resolution hyperthermia, normothermia and hypothermia (43). Hypothermia was more frequently observed in older patients, while slow-resolving hyperthermic patients were the youngest and had the fewest comorbidities. Rapid-resolution hyperthermic patients had the lowest mortality (3%), while hypothermic patients had three times the mortality (10%). In neutropenic patients, slow-resolving hyperthermic patients have the highest mortality (54). Neutropenic patients with persistent hyperthermia could be affected by multi-resistant bacteria, fungal infections or associated with invasive devices, which could explain their poor prognosis.
Another use for temperature trajectories could be the prediction of hospital-acquired sepsis. In a case–control study, temperature changes (in terms of frequency, amplitude or progressive increase) prior to fever were an early marker of sepsis and could lead to earlier recognition of sepsis (55).
4. How do we control fever in sepsis? And should we?
Temperature control is variable between ICUs. A survey in the EUROBACT participants in 2012 found that temperature control management is protocolized in only 22% of the ICUs. While reported practice was to treat almost all patients with neurological impairment and most patients with acute coronary syndromes and infections, severe sepsis and septic shock, this was not the case for most patients with liver failure (41).
Target temperature management (TTM) has been a hot topic in sepsis for some time. Although there is no evidence of increased mortality in patients with very high temperatures, the authors point to the deleterious effect outside the sepsis setting as a reason to explore the effects of target temperature management in sepsis. The expected beneficial effect of TTM is a decrease in metabolism and an increase in vasomotor tone. Control of infection with antibiotics is mandatory before attempting TTM.
4.1. Means to TTM
4.1.1. Paracetamol
The effect of paracetamol is mediated by a lowering of the hypothalamic set point, although the exact mechanism is unknown. The drug and its safety are well established. The main side effect is hepatic cytolysis and, in the most severe cases, liver failure. In terms of efficacy, the “HEAT” trial involved 700 septic patients with fever (>38°C). Patients were randomized to receive either paracetamol or placebo. The study concluded that there was no effect on ICU length of stay or mortality (relative risk 0.96, p = 0.84) (56). However, the study had two shortcomings: patients were febrile for a short time, and the difference in temperature between the two groups was marginal.
4.1.2. NSAIDs
NSAIDs ultimately reduce PGE2 production via inhibition of cyclooxygenase. NSAIDs are widely used in ambulatory scenarios, benign and/or viral fevers (57). The most common side effect is gastrointestinal bleeding, but renal and hepatic side effects are also possible. The main obstacle in the case of sepsis is the risk of worsening the infection.
All attempts to demonstrate improved survival with NSAIDs have failed. Haupt et al. randomized 29 patients to ibuprofen or placebo in the setting of sepsis, although an antipyretic effect was demonstrated, no effect on mortality was found (58). They found no difference in secondary outcomes, including cytokine levels. Bernard et al. conducted a double blind randomized controlled trial, including 455 patients with sepsis. Although ibuprofen reduced prostacyclin and thromboxane and lowered fever, tachycardia, oxygen consumption and lactic acidosis, it did not prevent the development of shock or improve mortality (59). Morris et al. randomized 120 patients to ibuprofen or placebo. Ibuprofen lowered temperature, but had no effect on outcome (60). Finally, Memiş et al. compared 40 patients randomized to lornoxicam or placebo in severe sepsis (61). They found no difference in outcomes, biological parameters, including cytokine levels.
4.1.3. External cooling
Physical methods include surface cooling and endovascular devices. Surface cooling can range from simple skin exposure or ice packs to dedicated devices (62, 63). Apart from air-circulating blankets, there is no difference in the induction of normothermia (64). Endovascular devices carry their own risks associated with central vascular access (8). Physical cooling, does not influence central control, but acts by directly eliminating heat. The hypothalamus reply will result in shivering, tachycardia and peripheral vasoconstriction, all of which are sources of discomfort for patients and may paradoxically lead to increased oxygen consumption. Shivering should therefore be avoided, and can be prevented by sedation and neuromuscular blockade (65).
Schortgen et al. conducted the main trial comparing external cooling to placebo, it involved 200 patients with septic shock. The cooling method to achieve normothermia was applied according to local procedure. The primary endpoint, the number of patients with a 50% decrease in vasopressors at 48 h, was not different (72 vs. 61%; absolute difference: 11%; 95%CI, −23 to 2) but day 14 mortality was significantly lower (19 vs. 34%, p = 0.013). The mortality difference was not found at day 30 (66).
4.2. Should we control hyperthermia?
With TTM well established in the management of cardiac arrest, intensivists gradually extended its use to septic shock. In a retrospective cohort of septic shock patients, over 75% of hyperthermic patients were treated with acetaminophen, and a small proportion even received methylprednisone (43). In addition, although it is not their intended use, renal replacement therapy and any other extracorporeal circulation therapy lower the temperature. The Surviving sepsis campaign guidelines make no mention of this topic, despite the available data.
Since hyperthermic presentation of sepsis has better prognosis, one could challenge the need to lower fever in sepsis. In an original design, preventing very high fever (T > 39°C) did not prevent organ dysfunction (67). In two studies about septic patients undergoing mechanical ventilations, better temperature control did not improve hemodynamic or respiratory conditions (68, 69). But this effect could be canceled out at very high temperatures, as shown in the retrospective study by Evans et al., in which maximum temperature > 39.4°C lost its beneficial effect on survival (70). However, in the same study, antipyretic drugs did not affect survival.
A meta-analysis of the largest trials and observational studies (1,507 patients) including drug induced and external cooling, showed that antipyretic therapy did not reduce 28-day hospital mortality in the randomized studies (relative risk, 0.93; 95% CI, 0.77–1.13; I2 = 0.0%) or observational studies (odds ratio, 0.90; 95% CI, 0.54–1.51; I2 = 76.1%) (71).
In an individual patients data meta-analysis (95% of the 1,413 included patients were infected at baseline), a more active fever management did not result in a statistically significant improvement in survival time compared with less active fever management [hazard ratio 0.91; (95% CI 0.75–1.10), p = 0.32] even in the subgroup with a temperature of more than 39.5°C at baseline [hazard ratio 0.76 (0.35–1.67)] (72). In a sensitivity analysis, the metanalysis was in favor of the less active temperature management in the patients mechanically ventilated with vasopressor support [n = 595, Hazard ratio 1.21 (1.00–1.45)].
More recently another meta-analysis including 5,140 participants, 23 trials assessing antipyretic drugs, physical cooling or both methods found similar results (65). Meta-analysis and sequential analysis of the trials showed that the hypothesis that antipyretic therapy reduces the risk of death (risk ratio 1.04, 95% confidence interval 0.90 to 1.19; I2 = 0%; p = 0.62; 16 trials; high certainty evidence) and the risk of serious adverse events (risk ratio 1.02, 0.89–1.17; I2 = 0%; p = 0.78; 16 trials; high certainty evidence) could be rejected. Similar negative results were found in studies using antipyretic agents or physical cooling.
Taking this a step further, induced hypothermia (32–34°C) in septic shock has been shown to prolong the duration of acute respiratory failure and shock and to increase 30 days mortality (73).
4.3. Warming hypothermia?
Since hypothermia presentation is common in sepsis, and since is it associated with higher mortality, one could advocate for actively warming patients. Active hyperthermia could lead to better organ perfusion, antibiotics concentration, and immune system activation (74–76). The available clinical data is scarce. In a Delphi method survey, a majority of physicians actively rewarmed patients (77). In another trial, normothermic septic shock patients were to receive warming of 1.5°C vs. standard of care. Induced hyperthermia was associated with better outcome (28 day survival and hospital free days) but the trial is hampered by imbalance in baseline characteristics despite randomization (78). Further studies are needed in order to evaluate warming in hypothermic and normothermic sepsis. A pilot study is in progress to assess feasibility of warming afebrile sepsis to 39°C (Clinicaltrials.gov - NCT04961151).
4.4. What should the bedside doctor do?
As the efficacy of TTM is not widely recognized, side effects should be avoided.
In the case of normothermic sepsis, there are no data to recommend a particular temperature range.
For hyperthermia, only the highest temperatures (T > 39.5°C) justify the use of MTT. While paracetamol is common and low-risk, external cooling carries a higher risk of chills and skin lesions. We suggest treating patients with very high temperatures (T > 39.5°C) with paracetamol and external cooling if necessary to achieve <38.2°C. Shivering should be avoided at all costs by sedation or neuromuscular blockade, and TTM should be discontinued if shivering cannot be avoided or in the presence of skin lesions.
In the event of hypothermic sepsis, rewarming should be considered and carried out gradually over several hours to avoid vasoplegia.
5. Conclusion
Fever is a carefully regulated mechanism, with variable consequences. In sepsis, mortality is inversely correlated with blood temperature over a wide temperature range. However, available data suggest that extreme hypo- or hyperthermia are deleterious. Despite the scientific basis for temperature control, several trials have failed to demonstrate the benefit of induced normothermia in sepsis. The control of extremely high and extremely low temperatures is usually performed in ICU to limit patients’ discomfort without definite proof.
Author contributions
MDo: Writing – original draft, Writing – review & editing. MT: Writing – review & editing. JD: Writing – review & editing. MDl: Writing – review & editing. HR: Writing – review & editing. EC: Writing – review & editing. ME: Writing – review & editing. LB: Writing – review & editing. EM: Writing – review & editing. J-FT: Writing – original draft, Writing – review & editing.
Funding
The author(s) declare that no financial support was received for the research, authorship, and/or publication of this article.
Conflict of interest
The authors declare that the research was conducted in the absence of any commercial or financial relationships that could be construed as a potential conflict of interest.
Publisher’s note
All claims expressed in this article are solely those of the authors and do not necessarily represent those of their affiliated organizations, or those of the publisher, the editors and the reviewers. Any product that may be evaluated in this article, or claim that may be made by its manufacturer, is not guaranteed or endorsed by the publisher.
References
1. O’Grady, NP, Barie, PS, Bartlett, JG, Bleck, T, Carroll, K, Kalil, AC, et al. Guidelines for evaluation of new fever in critically ill adult patients: 2008 update from the American College of Critical Care Medicine and the Infectious Diseases Society of America. Crit Care Med. (2008) 36:1330–49. doi: 10.1097/CCM.0b013e318169eda9
2. Mackowiak, PA, Chervenak, FA, and Grünebaum, A. Defining fever. Open Forum Infect Dis. (2021) 8:ofab161. doi: 10.1093/ofid/ofab161
3. Kaul, DR, Flanders, SA, Beck, JM, and Saint, S. Brief report: incidence, etiology, risk factors, and outcome of hospital-acquired fever: a systematic, evidence-based review. J Gen Intern Med. (2006) 21:1184–7. doi: 10.1111/j.1525-1497.2006.00566.x
4. Mackowiak, PA. Concepts of fever. Arch Intern Med. (1998) 158:1870–81. doi: 10.1001/archinte.158.17.1870
5. Annane, D. Body temperature in sepsis: a hot topic. Lancet Respir Med. (2018) 6:162–3. doi: 10.1016/S2213-2600(18)30003-1
6. Walter, EJ, Hanna-Jumma, S, Carraretto, M, and Forni, L. The pathophysiological basis and consequences of fever. Crit Care Lond Engl. (2016) 20:200. doi: 10.1186/s13054-016-1375-5
8. Doyle, JF, and Schortgen, F. Should we treat pyrexia? And how do we do it? Crit Care Lond Engl. (2016) 20:303. doi: 10.1186/s13054-016-1467-2
9. O’Reilly, T, and Zak, O. Elevated body temperature restricts growth of Haemophilus influenzae type b during experimental meningitis. Infect Immun. (1992) 60:3448–51. doi: 10.1128/iai.60.8.3448-3451.1992
10. Small, PM, Täuber, MG, Hackbarth, CJ, and Sande, MA. Influence of body temperature on bacterial growth rates in experimental pneumococcal meningitis in rabbits. Infect Immun. (1986) 52:484–7. doi: 10.1128/iai.52.2.484-487.1986
11. Rice, P, Martin, E, He, JR, Frank, M, DeTolla, L, Hester, L, et al. Febrile-range hyperthermia augments neutrophil accumulation and enhances lung injury in experimental gram-negative bacterial pneumonia. J Immunol Baltim Md 1950. (2005) 174:3676–85. doi: 10.4049/jimmunol.174.6.3676
12. Mackowiak, PA, Marling-Cason, M, and Cohen, RL. Effects of temperature on antimicrobial susceptibility of Bacteria. J Infect Dis. (1982) 145:550–3. doi: 10.1093/infdis/145.4.550
13. Aljaafari, H, Gu, Y, Chicchelly, H, and Nuxoll, E. Thermal shock and ciprofloxacin act orthogonally on Pseudomonas aeruginosa biofilms. Antibiotics. (2021) 10:1017. doi: 10.3390/antibiotics10081017
14. Hajdu, S, Holinka, J, Reichmann, S, Hirschl, AM, Graninger, W, and Presterl, E. Increased temperature enhances the antimicrobial effects of Daptomycin, vancomycin, Tigecycline, Fosfomycin, and Cefamandole on staphylococcal biofilms. Antimicrob Agents Chemother. (2010) 54:4078–84. doi: 10.1128/AAC.00275-10
15. Hasday, JD, and Singh, IS. Fever and the heat shock response: distinct, partially overlapping processes. Cell Stress Chaperones. (2000) 5:471–80. doi: 10.1379/1466-1268(2000)005<0471:FATHSR>2.0.CO;2
16. Keitelman, IA, Sabbione, F, Shiromizu, CM, Giai, C, Fuentes, F, Rosso, D, et al. Short-term fever-range hyperthermia accelerates NETosis and reduces pro-inflammatory cytokine secretion by human neutrophils. Front Immunol. (2019) 10:2374. doi: 10.3389/fimmu.2019.02374
17. Díaz, FE, Dantas, E, Cabrera, M, Benítez, CA, Delpino, MV, Duette, G, et al. Fever-range hyperthermia improves the anti-apoptotic effect induced by low pH on human neutrophils promoting a proangiogenic profile. Cell Death Dis. (2016) 7:e2437. doi: 10.1038/cddis.2016.337
18. Umar, D, Das, A, Gupta, S, Chattopadhyay, S, Sarkar, D, Mirji, G, et al. Febrile temperature change modulates CD4 T cell differentiation via a TRPV channel-regulated notch-dependent pathway. Proc Natl Acad Sci U S A. (2020) 117:22357–66. doi: 10.1073/pnas.1922683117
19. Rubin, RL. Mice housed at elevated vivarium temperatures display enhanced T-cell response and survival to Francisella tularensis. Comp Med. (2017) 67:491–7.
20. Lipke, AB, Matute-Bello, G, Herrero, R, Kurahashi, K, Wong, VA, Mongovin, SM, et al. Febrile-range hyperthermia augments lipopolysaccharide-induced lung injury by a mechanism of enhanced alveolar epithelial apoptosis. J Immunol Baltim Md 1950. (2010) 184:3801–13. doi: 10.4049/jimmunol.0903191
21. Hasday, JD, Garrison, A, Singh, IS, Standiford, T, Ellis, GS, Rao, S, et al. Febrile-range hyperthermia augments pulmonary neutrophil recruitment and amplifies pulmonary oxygen toxicity. Am J Pathol. (2003) 162:2005–17. doi: 10.1016/S0002-9440(10)64333-7
22. White, MG, Luca, LE, Nonner, D, Saleh, O, Hu, B, Barrett, EF, et al. Cellular mechanisms of neuronal damage from hyperthermia. Prog Brain Res. (2007) 162:347–71. doi: 10.1016/S0079-6123(06)62017-7
23. Levi, M. Hemostasis and thrombosis in extreme temperatures (hypo- and hyperthermia). Semin Thromb Hemost. (2018) 44:651–5. doi: 10.1055/s-0038-1648231
24. Kannemeier, C, Shibamiya, A, Nakazawa, F, Trusheim, H, Ruppert, C, Markart, P, et al. Extracellular RNA constitutes a natural procoagulant cofactor in blood coagulation. Proc Natl Acad Sci. (2007) 104:6388–93. doi: 10.1073/pnas.0608647104
25. Bongers, KS, Chanderraj, R, Woods, RJ, McDonald, RA, Adame, MD, Falkowski, NR, et al. The gut microbiome modulates body temperature both in Sepsis and health. Am J Respir Crit Care Med. (2023) 207:1030–41. doi: 10.1164/rccm.202201-0161OC
26. Dickson, RP, Schultz, MJ, van der Poll, T, Schouten, LR, Falkowski, NR, Luth, JE, et al. Lung microbiota predict clinical outcomes in critically ill patients. Am J Respir Crit Care Med. (2020) 201:555–63. doi: 10.1164/rccm.201907-1487OC
27. Zhou, Y, Shi, X, Fu, W, Xiang, F, He, X, Yang, B, et al. Gut microbiota Dysbiosis correlates with abnormal immune response in moderate COVID-19 patients with fever. J Inflamm Res. (2021) 14:2619–31. doi: 10.2147/JIR.S311518
28. Rashidi, A, Ebadi, M, Rehman, TU, Elhusseini, H, Nalluri, H, Kaiser, T, et al. Altered microbiota-host metabolic cross talk preceding neutropenic fever in patients with acute leukemia. Blood Adv. (2021) 5:3937–50. doi: 10.1182/bloodadvances.2021004973
29. Rashidi, A, Ebadi, M, Rehman, TU, Elhusseini, H, Halaweish, H, Holtan, SG, et al. Loss of microbiota-derived protective metabolites after neutropenic fever. Sci Rep. (2022) 12:6244. doi: 10.1038/s41598-022-10282-0
30. Schwabkey, ZI, Wiesnoski, DH, Chang, CC, Tsai, WB, Pham, D, Ahmed, SS, et al. Diet-derived metabolites and mucus link the gut microbiome to fever after cytotoxic cancer treatment. Sci Transl Med. (2022) 14:eabo3445. doi: 10.1126/scitranslmed.abo3445
31. Pickard, JM, Maurice, CF, Kinnebrew, MA, Abt, MC, Schenten, D, Golovkina, TV, et al. Rapid fucosylation of intestinal epithelium sustains host–commensal symbiosis in sickness. Nature. (2014) 514:638–41. doi: 10.1038/nature13823
32. Epstein, Y, and Yanovich, R. Heatstroke. N Engl J Med. (2019) 380:2449–59. doi: 10.1056/NEJMra1810762
33. Hopkins, PM, Girard, T, Dalay, S, Jenkins, B, Thacker, A, Patteril, M, et al. Malignant hyperthermia 2020. Anaesthesia. (2021) 76:655–64. doi: 10.1111/anae.15317
34. Greer, DM, Funk, SE, Reaven, NL, Ouzounelli, M, and Uman, GC. Impact of fever on outcome in patients with stroke and neurologic injury. Stroke. (2008) 39:3029–35. doi: 10.1161/STROKEAHA.108.521583
35. Gowda, R, Jaffa, M, and Badjatia, N. Thermoregulation in brain injury. Handb Clin Neurol. (2018) 157:789–97. doi: 10.1016/B978-0-444-64074-1.00049-5
36. Bogossian, EG, and Taccone, FS. Fever management in acute brain injury. Curr Opin Crit Care. (2022) 28:130–7. doi: 10.1097/MCC.0000000000000918
37. Leary, M, Grossestreuer, AV, Iannacone, S, Gonzalez, M, Shofer, FS, Povey, C, et al. Pyrexia and neurologic outcomes after therapeutic hypothermia for cardiac arrest. Resuscitation. (2013) 84:1056–61. doi: 10.1016/j.resuscitation.2012.11.003
38. Sandroni, C, Nolan, JP, Andersen, LW, Böttiger, BW, Cariou, A, Cronberg, T, et al. ERC-ESICM guidelines on temperature control after cardiac arrest in adults. Intensive Care Med. (2022) 48:261–9. doi: 10.1007/s00134-022-06620-5
39. Lee, BH, Inui, D, Suh, GY, Kim, JY, Kwon, JY, Park, J, et al. Association of body temperature and antipyretic treatments with mortality of critically ill patients with and without sepsis: multi-centered prospective observational study. Crit Care Lond Engl. (2012) 16:R33. doi: 10.1186/cc11211
40. Young, PJ, Saxena, M, Beasley, R, Bellomo, R, Bailey, M, Pilcher, D, et al. Early peak temperature and mortality in critically ill patients with or without infection. Intensive Care Med. (2012) 38:437–44. doi: 10.1007/s00134-012-2478-3
41. Niven, DJ, Laupland, KB, Tabah, A, Vesin, A, Rello, J, Koulenti, D, et al. Diagnosis and management of temperature abnormality in ICUs: a EUROBACT investigators’ survey. Crit Care. (2013) 17:R289–8. doi: 10.1186/cc13153
42. Thomas-Rüddel, DO, Hoffmann, P, Schwarzkopf, D, Scheer, C, Bach, F, Komann, M, et al. Fever and hypothermia represent two populations of sepsis patients and are associated with outside temperature. Crit Care. (2021) 25:368. doi: 10.1186/s13054-021-03776-2
43. Bhavani, SV, Carey, KA, Gilbert, ER, Afshar, M, Verhoef, PA, and Churpek, MM. Identifying novel Sepsis subphenotypes using temperature trajectories. Am J Respir Crit Care Med. (2019) 200:327–35. doi: 10.1164/rccm.201806-1197OC
44. Peres Bota, D, Lopes Ferreira, F, Mélot, C, and Vincent, JL. Body temperature alterations in the critically ill. Intensive Care Med. (2004) 30:811–6. doi: 10.1007/s00134-004-2166-z
45. Laupland, KB, Zahar, JR, Adrie, C, Schwebel, C, Goldgran-Toledano, D, Azoulay, E, et al. Determinants of temperature abnormalities and influence on outcome of critical illness. Crit Care Med. (2012) 40:145–51. doi: 10.1097/CCM.0b013e31822f061d
46. Rumbus, Z, Matics, R, Hegyi, P, Zsiboras, C, Szabo, I, Illes, A, et al. Fever is associated with reduced, hypothermia with increased mortality in septic patients: a Meta-analysis of clinical trials. PLoS One. (2017) 12:e0170152. doi: 10.1371/journal.pone.0170152
47. Weinkove, R, Bailey, M, Bellomo, R, Saxena, MK, Tam, CS, Pilcher, DV, et al. Association between early peak temperature and mortality in neutropenic sepsis. Ann Hematol. (2015) 94:857–64. doi: 10.1007/s00277-014-2273-z
48. Arons, MM, Wheeler, AP, Bernard, GR, Christman, BW, Russell, JA, Schein, R, et al. Effects of ibuprofen on the physiology and survival of hypothermic sepsis. Crit Care Med. (1999) 27:699–707. doi: 10.1097/00003246-199904000-00020
49. Laupland, KB, Zahar, JR, Adrie, C, Minet, C, Vésin, A, Goldgran-Toledano, D, et al. Severe hypothermia increases the risk for intensive care unit-acquired infection. Clin Infect Dis Off Publ Infect Dis Soc Am. (2012) 54:1064–70. doi: 10.1093/cid/cir1033
50. Kushimoto, S, Abe, T, Ogura, H, Shiraishi, A, Saitoh, D, Fujishima, S, et al. Impact of body temperature abnormalities on the implementation of Sepsis bundles and outcomes in patients with severe Sepsis: a retrospective sub-analysis of the focused outcome research on emergency Care for Acute Respiratory Distress Syndrome, Sepsis and trauma study. Crit Care Med. (2019) 47:691–9. doi: 10.1097/CCM.0000000000003688
51. Ahmad, MS, Ali, N, Mehboob, N, Mehmood, R, Ahmad, M, and Wahid, A. Temperature on admission among cases of neonatal sepsis and its association with mortality. JPMA J Pak Med Assoc. (2016) 66:1303–6.
52. Shimazui, T, Nakada, TA, Walley, KR, Oshima, T, Abe, T, Ogura, H, et al. Significance of body temperature in elderly patients with sepsis. Crit Care Lond Engl. (2020) 24:387. doi: 10.1186/s13054-020-02976-6
53. Schwab, F, Gastmeier, P, Hoffmann, P, and Meyer, E. Summer, sun and sepsis-the influence of outside temperature on nosocomial bloodstream infections: a cohort study and review of the literature. PLoS One. (2020) 15:e0234656. doi: 10.1371/journal.pone.0234656
54. Benzoni, NS, Carey, KA, Bewley, AF, Klaus, J, Fuller, BM, Edelson, DP, et al. Temperature trajectory subphenotypes in oncology patients with neutropenia and suspected infection. Am J Respir Crit Care Med. (2023) 207:1300–9. doi: 10.1164/rccm.202205-0920OC
55. Drewry, AM, Fuller, BM, Bailey, TC, and Hotchkiss, RS. Body temperature patterns as a predictor of hospital-acquired sepsis in afebrile adult intensive care unit patients: a case-control study. Crit Care Lond Engl. (2013) 17:R200. doi: 10.1186/cc12894
56. Young, P, Saxena, M, Bellomo, R, Freebairn, R, Hammond, N, van Haren, F, et al. Acetaminophen for fever in critically ill patients with suspected infection. N Engl J Med. (2015) 373:2215–24. doi: 10.1056/NEJMoa1508375
57. Azh, N, Barzkar, F, Motamed-Gorji, N, Pourvali-Talatappeh, P, Moradi, Y, Vesal Azad, R, et al. Nonsteroidal anti-inflammatory drugs in acute viral respiratory tract infections: an updated systematic review. Pharmacol Res Perspect. (2022) 10:e00925. doi: 10.1002/prp2.925
58. Haupt, MT, Jastremski, MS, Clemmer, TP, Metz, CA, and Goris, GB. Effect of ibuprofen in patients with severe sepsis: a randomized, double-blind, multicenter study. The ibuprofen study group. Crit Care Med. (1991) 19:1339–47. doi: 10.1097/00003246-199111000-00006
59. Bernard, GR, Wheeler, AP, Russell, JA, Schein, R, Summer, WR, Steinberg, KP, et al. The effects of ibuprofen on the physiology and survival of patients with sepsis. The ibuprofen in Sepsis study group. N Engl J Med. (1997) 336:912–8. doi: 10.1056/NEJM199703273361303
60. Morris, PE, Promes, JT, Guntupalli, KK, Wright, PE, and Arons, MM. A multi-center, randomized, double-blind, parallel, placebo-controlled trial to evaluate the efficacy, safety, and pharmacokinetics of intravenous ibuprofen for the treatment of fever in critically ill and non-critically ill adults. Crit Care Lond Engl. (2010) 14:R125. doi: 10.1186/cc9089
61. Memiş, D, Karamanlioğlu, B, Turan, A, Koyuncu, O, and Pamukçu, Z. Effects of lornoxicam on the physiology of severe sepsis. Crit Care Lond Engl. (2004) 8:R474–82. doi: 10.1186/cc2969
62. Polderman, KH. How to stay cool in the intensive care unit? Circulation. (2015) 132:152–7. doi: 10.1161/CIRCULATIONAHA.115.017350
63. Wasserman, DD, Creech, JA, and Healy, M. Cooling techniques for hyperthermia. In: StatPearls [Internet]. Treasure Island, FL: StatPearls Publishing; (2023). Available at: http://www.ncbi.nlm.nih.gov/books/NBK459311/ (Accessed 18 July 2023).
64. Hoedemaekers, CW, Ezzahti, M, Gerritsen, A, and van der Hoeven, JG. Comparison of cooling methods to induce and maintain normo- and hypothermia in intensive care unit patients: a prospective intervention study. Crit Care. (2007) 11:R91. doi: 10.1186/cc6104
65. Holgersson, J, Ceric, A, Sethi, N, Nielsen, N, and Jakobsen, JC. Fever therapy in febrile adults: systematic review with meta-analyses and trial sequential analyses. BMJ. (2022) 378:e069620. doi: 10.1136/bmj-2021-069620
66. Schortgen, F, Clabault, K, Katsahian, S, Devaquet, J, Mercat, A, Deye, N, et al. Fever control using external cooling in septic shock: a randomized controlled trial. Am J Respir Crit Care Med. (2012) 185:1088–95. doi: 10.1164/rccm.201110-1820OC
67. Toledo-Salinas, O, Sánchez-Hurtado, LA, and Rodríguez-Silverio, J. Association of fever and antipyretic treatment with progressive organ dysfunction in sepsis: prospective cohort. Gac Med Mex. (2021) 157:377–83. doi: 10.24875/GMM.M21000578
68. Ye, S, Xu, D, Zhang, C, Li, M, and Zhang, Y. Effect of antipyretic therapy on mortality in critically ill patients with Sepsis receiving mechanical ventilation treatment. Can Respir J. (2017) 2017:1–7. doi: 10.1155/2017/3087505
69. Markota, A, Skok, K, Kalamar, Ž, Fluher, J, and Gorenjak, M. Better control of body temperature is not associated with improved hemodynamic and respiratory parameters in mechanically ventilated patients with Sepsis. J Clin Med. (2022) 11:1211. doi: 10.3390/jcm11051211
70. Evans, EM, Doctor, RJ, Gage, BF, Hotchkiss, RS, Fuller, BM, and Drewry, AM. The association of fever and antipyretic medication with outcomes in mechanically ventilated patients: a cohort study. Shock. (2019) 52:152–9. doi: 10.1097/SHK.0000000000001368
71. Drewry, AM, Ablordeppey, EA, Murray, ET, Stoll, CRT, Izadi, SR, Dalton, CM, et al. Antipyretic therapy in critically ill septic patients: a systematic review and Meta-analysis. Crit Care Med. (2017) 45:806–13. doi: 10.1097/CCM.0000000000002285
72. Young, PJ, Bellomo, R, Bernard, GR, Niven, DJ, Schortgen, F, Saxena, M, et al. Fever control in critically ill adults. An individual patient data meta-analysis of randomised controlled trials. Intensive Care Med. (2019) 45:468–76. doi: 10.1007/s00134-019-05553-w
73. Itenov, TS, Johansen, ME, Bestle, M, Thormar, K, Hein, L, Gyldensted, L, et al. Induced hypothermia in patients with septic shock and respiratory failure (CASS): a randomised, controlled, open-label trial. Lancet Respir Med. (2018) 6:183–92. doi: 10.1016/S2213-2600(18)30004-3
74. Boulamery, A, Marouani, H, Guilhaumou, R, Rocher, E, Simon, N, and Bruguerolle, B. Effects of hyperthermia on pharmacokinetics of ertapenem in rats. Fundam Clin Pharmacol. (2008) 22:285–9. doi: 10.1111/j.1472-8206.2008.00586.x
75. Beovic, B, Mrhar, A, Karba, R, Zupancic, T, Grabnar, I, Belic, A, et al. Influence of fever on the pharmacokinetics of ciprofloxacin. Int J Antimicrob Agents. (1999) 11:81–5. doi: 10.1016/S0924-8579(98)00061-2
76. Markota, A, Kalamar, Ž, Fluher, J, and Pirkmajer, S. Therapeutic hyperthermia for the treatment of infection-a narrative review. Front Physiol. (2023) 14:1215686. doi: 10.3389/fphys.2023.1215686
77. Harmon, MBA, Pelleboer, I, Steiner, AA, Wiewel, M, Schultz, MJ, Horn, J, et al. Opinions and Management of Hypothermic Sepsis: results from an online survey. Ther Hypothermia Temp Manag. (2020) 10:102–5. doi: 10.1089/ther.2019.0002
Keywords: body temperature, fever, sepsis, septic shock, antipyretics, prognosis
Citation: Doman M, Thy M, Dessajan J, Dlela M, Do Rego H, Cariou E, Ejzenberg M, Bouadma L, de Montmollin E and Timsit J-F (2023) Temperature control in sepsis. Front. Med. 10:1292468. doi: 10.3389/fmed.2023.1292468
Edited by:
Alessandro Russo, Magna Græcia University, ItalyReviewed by:
Yuetian Yu, Shanghai Jiao Tong University, ChinaCopyright © 2023 Doman, Thy, Dessajan, Dlela, Do Rego, Cariou, Ejzenberg, Bouadma, de Montmollin and Timsit. This is an open-access article distributed under the terms of the Creative Commons Attribution License (CC BY). The use, distribution or reproduction in other forums is permitted, provided the original author(s) and the copyright owner(s) are credited and that the original publication in this journal is cited, in accordance with accepted academic practice. No use, distribution or reproduction is permitted which does not comply with these terms.
*Correspondence: Jean-François Timsit, SmVhbi1mcmFuJiN4MDAwRTc7b2lzLnRpbXNpdEBhcGhwLmZy