- 1Department of Urology, Xiang’an Hospital of Xiamen University, School of Medicine, Xiamen University, Xiamen, China
- 2Central Laboratory, Xiang’an Hospital of Xiamen University, School of Medicine, Xiamen University, Xiamen, China
The sudden outbreak of coronavirus disease 2019 (COVID-19) in early 2020 posed a massive threat to human life and caused an economic upheaval worldwide. Kidney transplant recipients (KTRs) became susceptible to infection during the COVID-19 pandemic owing to their use of immunosuppressants, resulting in increased hospitalization and mortality rates. Although the current epidemic situation is alleviated, the long-term existence of COVID-19 still seriously threatens the life and health of KTRs with low immunity. The Omicron variant, a highly infectious but less-pathogenic strain of severe acute respiratory syndrome coronavirus 2 (SARS-CoV-2), has raised concerns among transplant physicians regarding managing KTRs diagnosed with this variant. However, currently, there are no clear and unified guidelines for caring for KTRs infected with this variant. Therefore, we aimed to summarize the ongoing research on drugs that can treat Omicron variant infections in KTRs and explore the potential of adjusting immunotherapy strategies to enhance their responsiveness to vaccines. Herein, we discuss the situation of KTRs since the emergence of COVID-19 and focus on various prevention and treatment strategies for KTRs since the Omicron variant outbreak. We hope to assist physicians in managing KTRs in the presence of long-term COVID-19 variants.
Highlights
• Monoclonal antibodies help kidney transplant recipients resist Omicron infection;
• Antiviral drugs can be used for the treatment of kidney transplant recipients diagnosed with Omicron;
• Alternative vaccination strategies can optimize the vaccination response of kidney transplant recipients;
• Regulating immunosuppressive strategies to optimize vaccine response in kidney transplant recipients.
1 Introduction
Organ transplantation is recognized as the most efficacious therapeutic approach for individuals afflicted by end-stage organ failure (1). Among all organ transplantations, kidney transplantation is the earliest, most common, and most widely used with the best outcomes (2). Chronic kidney disease (CKD) afflicts an estimated 700 million individuals worldwide and constitutes a formidable peril to patients’ lives when it advances to end-stage kidney disease (ESKD), resulting in the annual mortality of roughly 5–10 million individuals worldwide (3). Kidney transplantation is regarded as the optimal therapeutic intervention for ESKD patients, remarkably enhancing their long-term survival prospects, affording an improved quality of life, and yielding greater cost-effectiveness compared with dialysis therapy (4, 5).
Coronavirus disease 2019 (COVID-19) was brought about by severe acute respiratory syndrome coronavirus 2 (SARS-CoV-2), also known as novel coronavirus, rapidly evolving into a worldwide pandemic in early 2020, with its impact extending to a minimum of 222 countries and territories worldwide (6). Due to pre-existing CKD and long-term immunosuppressive therapy, kidney transplant recipients (KTRs) were found to be highly vulnerable to COVID-19 infection (7). Following the initial report of a KTR with COVID-19 pneumonia in March 2020, the incidence of COVID-19 in KTRs and its various strains has been on the rise.
The Omicron variant boasts the highest number of mutations among all the SARS-CoV-2 variants. The variant XBB and its descendant lineage EG.5(Eris) are important reasons for the current pandemic. Due to its heightened transmissibility and capacity to evade the immune response, Omicron has swiftly overridden the Delta variant and emerged as the prevailing variant in numerous regions worldwide (8). Managing KTRs during the Omicron variant epidemic is a significant concern for urologists. Although some monoclonal antibodies and antiviral drugs have been approved for use in KTRs during SARS-CoV-2 and other subtype outbreaks, their effectiveness against the Omicron variant in KTRs remains to be ascertained. Moreover, owing to the unique immunosuppressive state of KTRs, the impact of prior vaccination on protecting KTRs from Omicron variant attacks is unclear. A more comprehensive vaccine system is required to help immunocompromised patients fight the Omicron variant. This review aims to offer guidance and a point of reference for the care and management of KTRs in the ongoing presence of COVID-19 and its different variants. This is achieved by consolidating pertinent clinical research and treatment strategies related to KTRs amidst the prevalence of the Omicron variant.
2 Kidney transplantation and COVID-19
Since the onset of the COVID-19 pandemic, the rate of SARS-CoV-2 infection in KTRs has consistently exceeded that observed in the general population (9). Allograft rejection indicates that KTRs must use long-term immunosuppressants such as prednisone and mycophenolate mofetil (MMF) to prevent rejection risk. However, extended use of immunosuppressants, along with comorbid conditions such as hypertension and diabetes, and the reduced vaccine response in KTRs, considerably increase their susceptibility to COVID-19 (10). In addition to increasing the difficulty of treatment and care for KTRs with underlying COVID-19 infection, the virus can cause damage to the transplanted kidney (11). Furthermore, compared with non-transplant patients, KTRs have a higher frequency of acute kidney injury (AKI; 27.5% vs. 13.3%) and a more frequent need for renal replacement therapy (15.4% vs. 3.3%) (7, 12, 13). The mortality rate of patients with kidney failure and KTRs was observed to be particularly high after COVID-19 infection (14). This conclusion is consistent with a data report in the United States, according to which, after accounting for comorbidities, mortality risk for transplant recipients was almost twice as high as that for non-transplant patients (15).
The kidney stands as one of the specific organs susceptible to SARS-CoV-2 infection (Figure 1). After a patient is diagnosed with SARS-CoV-2 lung infection, the virus can enter the kidney through blood circulation and accumulate, damaging resident cells and causing kidney injury (16). SARS-CoV-2 can directly infect the human kidneys, leading to tubulopathy and AKI (17). SARS-CoV-2 pathogenesis closely resembles that of SARS and hinges on the interaction between the viral spike protein and the angiotensin-converting enzyme 2 (ACE2) receptor, with cell entry facilitated by the cellular protease TMPRSS2 (18). ACE2 receptors are widely distributed in multiple organs in the human body, with high expression in the kidney (19), particularly in the proximal tubules (20). After SARS-CoV-2 enters kidney cells, viral RNA is translated, inducing innate immune responses through the host’s expression of interferons, chemokines, and cytokines such as tumor necrosis factor (TNF), interleukin-1β (IL-1β), and interleukin-6 (IL-6) (21, 22). As the virus continues to replicate, cytokine levels continue to rise, leading to severe tissue damage and cytokine storms in patients (23), which can cause enormous damage to the kidneys (24). As the virus replicates, mutations in the spike protein’s receptor-binding domain (RBD) may occur, resulting in the emergence of new viral variants. These mutations increase the RBD’s affinity for the ACE2 complex, boosting the virus’s infectivity and transmission.
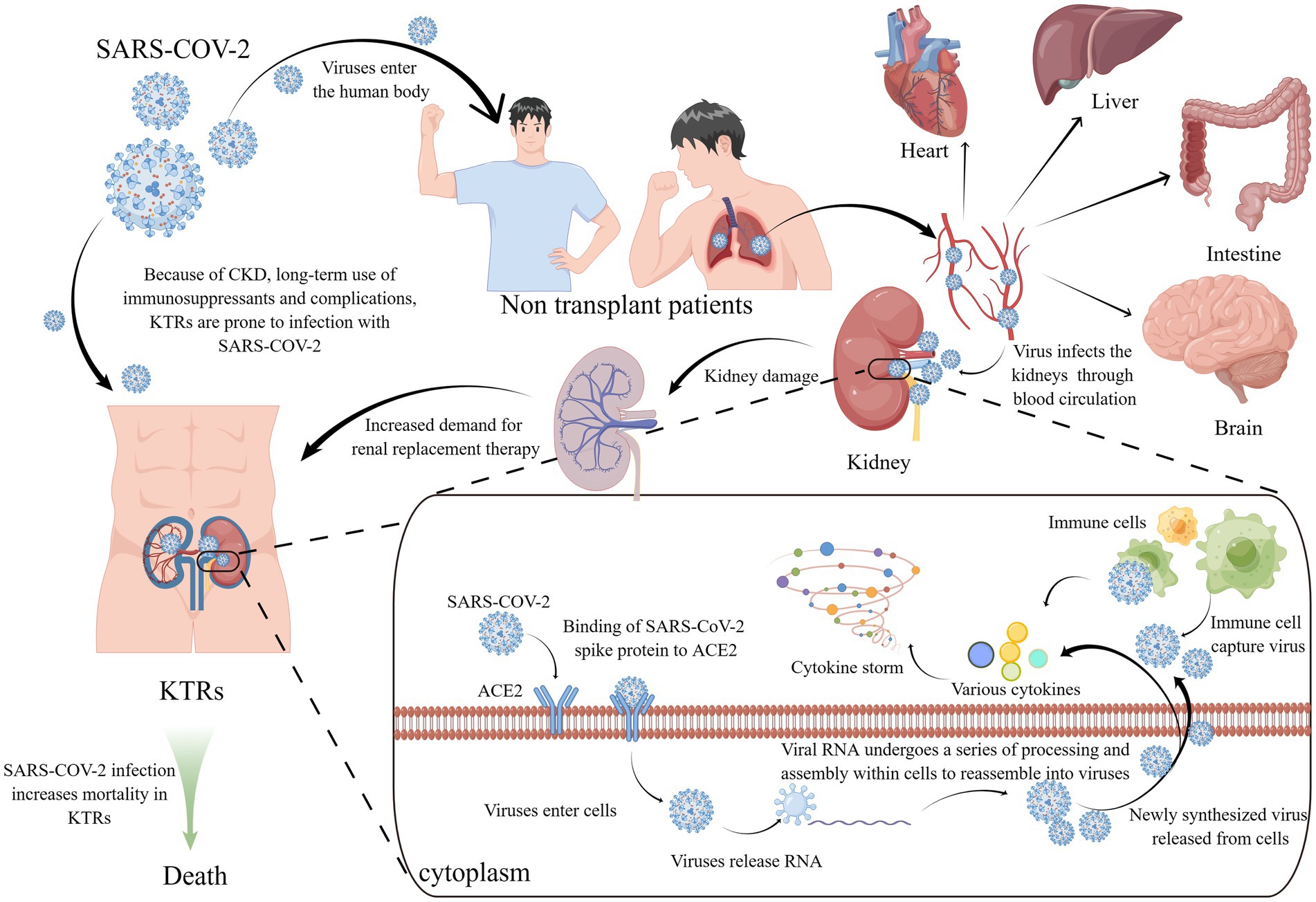
Figure 1. KTRs and SARS-COV-2 and its infection mechanism. KTRs are susceptible to SARS COV-2 due to CKD and many complications. After infection with SARS-COV-2, the mortality of KTR has increased significantly. SARS-COV-2 enters the human body through the respiratory tract, gathers in the lungs, and then spreads to various organs (including the heart, liver, brain, kidney, etc.) through the blood. The viral spike protein of SARS-COV-2 combines with the ACE2 receptor of renal epithelial cells, and then enters the cells, releasing viral RNA. The viral RNA combines with the ribosome, translates, replicates, and other operations to assemble new viruses. The virus is released from the cells through exocytosis, At the same time, cytokines are also released, and subsequently immune cells aggregate and capture the virus, while also releasing a large number of cytokines. The cell storm formed by numerous cytokines and the virus itself damage cells, causing damage to the patient’s kidney and increasing the demand for kidney transplant treatment. KTRs, kidney transplant recipients; SARS-COV-2, Severe acute respiratory syndrome coronavirus 2; CKD, Chronic kidney disease; ACE2, angiotensin-converting enzyme 2.
Since the advent of COVID-19, the World Health Organization (WHO) has identified five distinct variants of SARS-CoV-2, namely, Alpha (B.1.1.7), Beta (B.1.351), Gamma (P.1), Delta (B.1.617), and Omicron (B.1.1.529) (25). The B.1.1.529 variant initially surfaced in South Africa on November 9, 2021, and was later designated as the fifth variant by the WHO and given the name Omicron. By December 22, 2021, the Omicron variant had been identified in 110 countries and regions across the globe (26). Omicron then swiftly surpassed the Delta variant as the prevailing strain on a global scale. The former has been noted for its significantly enhanced transmissibility, although it exhibits a lower case fatality rate than both the Delta variant and the original SARS-CoV-2 virus (27). Despite the decrease in the case fatality rate associated with the Omicron variant when contrasted with earlier strains, a retrospective study conducted on KTRs in a specific region of Spain revealed that following infection with the Omicron variant, the occurrence of pneumonia and the mortality rate continued to surpass those observed in the general population (28). Furthermore, the Omicron variant increased the hospitalization rate of KTRs, possibly due to the increased renal burden caused by inflammation and tissue damage after infection with SARS-CoV-2 (29). Therefore, during the Omicron variant outbreak, KTRs, a vulnerable population, need enhanced focus on infection prevention and suitable treatments for those diagnosed with the variant. In summary, it is essential to conduct more research and create tailored prevention and treatment strategies for KTRs during the Omicron pandemic.
3 Application of monoclonal antibodies for treating KTRs infected with Omicron
SARS-CoV-2, a beta coronavirus, is an RNA virus. Similar to other viruses in this group, certain points in its lifecycle can be identified and blocked using monoclonal antibodies (mAbs), making them a promising option for preventing and treating COVID-19 (30). During the SARS-CoV-2 pandemic, mAbs have been approved for vulnerable populations, including KTRs (31). Nevertheless, with the Omicron variant’s emergence, there is uncertainty about the effectiveness of mAbs. Takashita et al. (32) assessed mAb reactivity via enzyme-linked immunosorbent assays. Results showed bamlanivimab lost its ability to neutralize Omicron, while imdevimab had high neutralization against Beta and Gamma variants but not Omicron. In contrast, caplacizumab, tixagevimab (COV2-2196), cilgavimab (COV2-2130), and sotrovimab precursor (S309) maintained neutralization against Beta, Gamma, and Omicron. However, their FRNT50 values for Omicron were 3.7 to 198.2 times higher than those for Beta or Gamma.
3.1 Sotrovimab
Sotrovimab is a recombinant human mAb designed to target SARS-CoV-2. Its mechanism of action involves neutralizing the virus by binding to a highly conserved epitope located on the RBD of the spike protein (33). Sotrovimab has demonstrated its effectiveness in treating COVID-19 in solid organ transplant recipients (SOTRs) (34). However, its efficacy against Omicron variants in KTRs remains to be validated.
A single-center study revealed favorable tolerability and efficacy characteristics of sotrovimab in transplant patients and preserved activity against the Omicron variant (35). Furthermore, individuals with the Omicron variant who received sotrovimab treatment demonstrated a reduced probability of hospitalization or clinic visits (36). Radcliffe et al. (37) analyzed 122 patients diagnosed with Omicron, including 24 who received sotrovimab treatment, 48 who did not receive outpatient treatment, and the remaining patients who received antiviral therapy. The patients were followed up for more than 30 days, and the hospitalization rate of patients who received sotrovimab treatment within 30 days was 8% (2/24), while that of patients who did not receive outpatient treatment was 27% (13/48), implying that sotrovimab has activity against the Omicron variant. Additionally, a single-center retrospective study conducted by Fernandes et al. (38) revealed that sotrovimab administration was linked to favorable outcomes among KTRs who contracted mild COVID in 2019, including those infected with the Omicron variant.
Although sotrovimab has been approved for adolescents and adults, its effectiveness and safety in KTRs under 12 years of age are unclear. Chiara et al. (39) described the case of an 11-year-old male KTR who got COVID-19 after a kidney transplant. Due to the patient’s kidney function, sotrovimab was administered, and the treatment was deemed successful without adverse effects. This case adds to the body of evidence on sotrovimab’s efficacy and safety in pediatric patients. However, it should be noted that the susceptibility of BA.2 subvariant of the Omicron variant to sotrovimab was diminished (40).
3.2 Bebtelovimab
In February 2022, bebtelovimab received authorization from the United States Food and Drug Administration (FDA) to treat COVID-19 (32). Recent studies have demonstrated that bebtelovimab maintains its effectiveness against the Omicron variant, including its subvariants BA.1.2, BA.4, and BA.5 (41). McCreary et al. (42) assessed the real-world effectiveness of bebtelovimab in outpatients with mild-to-moderate COVID-19 during the Omicron era. The authors concluded that using bebtelovimab for outpatient COVID-19 cases may reduce hospitalization and mortality risks, especially for the elderly and immunocompromised patients. However, it is unclear whether bebtelovimab is effective in immunocompromised groups such as KTRs.
Nonetheless, Shertel et al. (43) study addressed the above point and demonstrated that bebtelovimab can treat SOTRs with COVID-19 caused by the Omicron BA.1 or BA.25 subvariants. Their experimental results suggest that bebtelovimab could be a safe and effective treatment for transplant recipients with mild-to-moderate Omicron infections. However, this study was retrospective and lacked a control group; thus, the results may be less conclusive. Yetmar et al. (44) also explored the use of bebtelovimab in KTRs and found that, despite vaccination rates and viral subvariant differences as potential confounding factors, COVID-19 hospitalization rates were similar in patients treated with bebtelovimab and those treated with sotrovimab.
3.3 Tixagevimab/cilgavimab
Tixagevimab/cilgavimab, sold under the brand name Evusheld, is a monoclonal antibody drug that has received authorization from both the FDA and the UK Medicines and Healthcare Products Regulatory Agency. It was designed to reduce the risk of COVID-19. This treatment is intended for individuals who are presently uninfected or have not been exposed to the coronavirus and who exhibit a compromised immune response to COVID-19 vaccines. This includes individuals who are not advised to receive vaccinations (45). Consequently, individuals with compromised immune responses to vaccines, including KTRs, may consider tixagevimab/cilgavimab for pre-exposure prophylaxis and treating COVID-19 infections (46).
Tixagevimab/cilgavimab can mimic the natural antibody defense mechanism of the human body by binding to different sites on the spike protein of the novel coronavirus, reducing its ability to enter and infect healthy cells, and helping to clear infected cells (47). Medical practitioners have showcased the ability of this drug to reduce the risk of SARS-CoV-2 infection during the Alpha (B.1.1.7) and Delta (B.1.617.2) waves in unvaccinated individuals (48, 49). Following the emergence of the Omicron variant and its sub-lineages, tixagevimab/cilgavimab was recognized as the sole effective monoclonal combination therapy available (50), with neutralizing activity against the Omicron variant sub-lineages BA.1 and BA.1.1 (51)as well as against sub-lineages BA.2 and BA.5 (49).
Tixagevimab/cilgavimab is suitable for pre-exposure prophylaxis in KTRs because the binding domain of SARS-CoV-2 spike protein IgG titers of the recipient decreases rapidly after the recipient is administered this drug (52). A medical research team compared 222 SOTRs who received tixagevimab/cilgavimab for pre-exposure prophylaxis with 222 who did not. The team found that 5% of those receiving the drug had breakthrough SARS-CoV-2 infections, significantly lower (p < 0.001) compared with 14% in the control group. The results suggested that administering tixagevimab/cilgavimab to SOTRs who were vaccinated during the Omicron surge was safe and mitigated the risk of breakthrough SARS-CoV-2 infections (53).
While tixagevimab/cilgavimab may help prevent KTRs from being infected with the Omicron variant, further experiments are needed to determine the appropriate dosage, as Benotmane et al. (54) suggested that using a dose of 150 mg of each antibody may not provide sufficient protection against Omicron infection. Consequently, it is crucial to establish the required dosage for achieving neutralizing titers against various Omicron sub-lineages. Further research is essential to examine the pharmacological effects and antibody kinetics of the drug to provide valuable insights for the subsequent steps in treatment planning. However, tixagevimab/cilgavimab does not exhibit neutralizing activity against the Omicron sub-variant, BQ.1.1, and additional research is therefore warranted to assess its efficacy against this variant (55).
3.4 Other monoclonal antibodies
Dhand et al. (56) reported using casirivimab/imdevimab for COVID-19 treatment in SOTRs, which could prevent further progression of COVID-19 in KTRs to a certain extent (57). In addition to the results of sotrovimab administration, Fernandes et al. (38) showcased the positive outcomes of casirivimab/imdevimab administration in KTRs infected with the Omicron variant. However, the latest research shows that the inhibitory concentration (IC50) of casirivimab/imdevimab against the Omicron variant is >9000 ng/mL (58). Furthermore, Hoffmann et al. (59) found that Omicron exhibits complete resistance to imdevimab and casirivimab mAbs. Therefore, whether KTRs can benefit from casirivimab/imdevimab still requires further in-depth research, although it can provide at least one treatment option for clinical doctors.
Tocilizumab, a mAb that blocks the IL-6 receptor and acts by reducing inflammation, has been demonstrated to treat infectious complications in KTRs (60). Early administration of tocilizumab during the COVID-19 pandemic was found to decrease the mortality rate among severely ill COVID-19 patients (61). However, no research has explored whether tocilizumab is beneficial for KTRs diagnosed with the Omicron variant.
Takashita et al. (32) in vitro experiments revealed that mAbs such as LY-CoV555 (sold as bamlanivimab), LY-CoV016 (sold as etesevimab), and REGN10987 (sold as imdevimab) that originally acted on other variants lost their neutralizing effect against Omicron. This also limits the selection of mAbs that can be applied to treat KTRs diagnosed with the Omicron variant. In addition, many mAbs, such as BI 767551 (DZIF-10c) and CT-P59 (regdanvimab), have been approved for emergency use in treating COVID-19 (30, 62). However, only a few studies have attempted to ascertain whether these antibodies are suitable for immunocompromised patients.
To sum up, although mAbs have been instrumental in preventing and treating COVID-19, the effectiveness of current mAbs against evolving viruses such as Omicron has waned. Additionally, the distinctive circumstances of KTRs, coupled with limited research on mAb use in this group, have resulted in a shortage of suitable mAbs for this population (Table 1).
Furthermore, the emergence of the latest Omicron sub-variants BQ.1.1 and XBB has made many mAbs that were previously beneficial to KTRs ineffective, such as imdevimab, casirivimab, tixagevimab, cilgavimab, bebtelovimab, and S309 (the precursor of sotrovimab) (55). Therefore, more comprehensive investigations must be conducted to verify the efficacy of existing antibodies or develop new antibodies. The combined use of mAbs is also an important and viable approach (Table 2).
4 Application of antiviral drugs in renal transplant patients diagnosed with Omicron
Antiviral medications play a vital role in helping renal transplant patients fend off SARS-CoV-2 infection. However, due to the ongoing mutations of SARS-CoV-2, the treatment approach for infected renal transplant patients is frequently adapted. Unlike previous variants, Omicron features mutations in both the RNA-dependent RNA polymerase (RdRp) and the primary protease of SARS-CoV-2. These are the specific targets of antiviral drugs such as RdRp inhibitors (such as remdesivir and molnupiravir) and the main protease inhibitor PF-07304814. Consequently, the effectiveness of current drugs against Omicron has been reduced (63). Therefore, it is crucial to identify the antiviral drugs beneficial for renal transplant patients. Current research suggests that antiviral compounds such as remdesivir and molnupiravir are effective against Omicron (32).
4.1 Remdesivir
Remdesivir was initially developed for treating hepatitis C and Ebola virus diseases. During the COVID-19 pandemic, remdesivir exhibited promising therapeutic effects against SARS-CoV-2 (64). Indeed, it is crucial to highlight that remdesivir can interact with cytochrome P450 3A4 substrates, such as tacrolimus and sirolimus, potentially leading to an increase in their blood concentrations. This interaction can have adverse effects on patients, especially in the presence of elevated inflammatory conditions (65), and potential kidney toxicity may also exist (66). Therefore, it is necessary to investigate whether remdesivir is suitable for use in KTRs.
A retrospective cohort study from China demonstrated that remdesivir treatment was safe and clinically feasible in KTRs (67). Similarly, Elec et al. (68) evaluated the effects of remdesivir on overall mortality, ICU mortality, and renal function outcomes in COVID-19 hospitalized patients who underwent kidney transplantation. The authors deduced that remdesivir did not exert any nephrotoxic effects on KTRs.
Remdesivir also exerts therapeutic effects on KTRs diagnosed with the Omicron variant. In Spain, remdesivir has been approved for treating severe pneumonia, respiratory failure, and COVID-19 patients who have had symptoms for less than 8 days. In non-hospitalized patients at high risk of COVID progression, the safety of a three-day course of remdesivir is acceptable, and the risk of hospitalization or death is 87% lower than that of placebo (69). In addition, a cohort study conducted at a clinical hospital in Barcelona, Spain, included all patients with comorbidities (including solid organ transplantation) in the remdesivir treatment cohort. The subsequent cohort study found that in the remdesivir-treated cohort of patients with the Omicron variant, severe illness could only be avoided by taking the drug early (within ≤7 days of symptom onset), and if the drug was administered correctly during the viremia phase of the disease, it was effective. Cacho et al. (70) also noted that remdesivir could be safely administered to KTRs with eGFR <30 mL/min. Therefore, remdesivir is an important and viable treatment option for KTRs diagnosed with the Omicron variant.
4.2 Molnupiravir
Molnupiravir is an orally administered antiviral medication that hinders the replication of SARS-CoV-2 by suppressing the RdRp, thereby exerting antiviral activity (71). Its in vitro effectiveness is approximately on par with that of remdesivir, and it has been proven to lower hospitalization rates compared with a placebo. A large-scale phase III clinical trial involving oral molnupiravir in COVID-19 patients showcased notable clinical advantages.
During the Omicron pandemic, KTRs treated with molnupiravir showed good clinical progress and a lower risk of hospitalization without any adverse effects. At the kidney level, molnupiravir exhibited good tolerance and no evidence of drug-induced nephrotoxicity or interaction with immunosuppressive therapy (72). Poznański et al. demonstrated through their experiments that early treatment with molnupiravir as a standalone therapy was safe and well-tolerated among a cohort of patients with ESKD who were either undergoing hemodialysis or had received a kidney transplant subsequent to SARS-CoV-2 infection (37, 73), and the Omicron variant was highly sensitive to molnupiravir (74, 75). Therefore, molnupiravir is one of the most beneficial drugs for KTRs diagnosed with the Omicron variant.
During the period when the Omicron subvariant BA.2.2 was prevalent in Hong Kong, early treatment with molnupiravir markedly lowered the risk of death in comparison to receiving no antiviral therapy (76). Therefore, we believe that clinical practitioners can consider molnupiravir as a treatment option for KTRs diagnosed with Omicron. The same study also indicated that the early administration of nirmatrelvir/ritonavir had comparable effects, and its use was correlated with a decreased risk of COVID-19-related hospitalization (76).
4.3 Nirmatrelvir/ritonavir
The combination of nirmatrelvir and ritonavir, marketed as Paxlovid by Pfizer, has demonstrated its effectiveness in treating COVID-19 patients and lowering the mortality rate among individuals with severe COVID-19 and those at high risk (77, 78). Nirmatrelvir is an effective inhibitor of the 3-chymotrypsin-like protease (3CLPro), also known as MPRO, in SARS-CoV-2, which plays a crucial role in the process of COVID-19 virus infection (79). Ritonavir, initially developed as an HIV protease inhibitor, slows down the metabolism of nirmatrelvir. It inhibits a specific enzyme, recombinant human CYP3A4 enzyme (CYP3A4), in the intestine, liver, and other body parts (80). In nirmatrelvir/ritonavir, ritonavir functions as an inhibitor of CYP3A4 to slow down the metabolism of nirmatrelvir (81).
The efficacy of nirmatrelvir/ritonavir against the Omicron variant of COVID-19 is a matter of concern. Recent in vitro studies have proven that nirmatrelvir retains its efficacy against all Omicron sub-lineages (82), and there have been numerous reports regarding the clinical effectiveness of nirmatrelvir/ritonavir in patients infected with the Omicron variant (83). Therefore, nirmatrelvir/ritonavir remains an important treatment option for patients diagnosed with the mysterious Omicron variant.
Although KTRs may benefit from nirmatrelvir/ritonavir (84, 85), the potential for this drug to interact with immunosuppressive drugs and harm patients cannot be overlooked (86). The interaction between nirmatrelvir/ritonavir and tacrolimus has been widely reported (87–89). A case report describes a 14-year-old renal transplant recipient maintained on tacrolimus and prednisone postoperatively, who received paxlovid upon infection with omicron, subsequently experiencing supra-therapeutic levels of tacrolimus and elevated serum creatinine (10.1007/s00467-022-05712-0). Concurrently, another report indicated that co-administration of paxlovid significantly reduced the clearance of tacrolimus to approximately 3.9% of the pharmacokinetic values in the normal population, with an inter-compartmental clearance (Q/F) decreased significantly to about 2.7% of the standard pharmacokinetic values. Thus, a significant drug–drug interaction exists between tacrolimus and paxlovid (10.1007/s40261-022-01180-4). Consequently, if paxlovid is administered, tacrolimus should be discontinued, and tacrolimus may be resumed when blood concentrations are within therapeutic range.
Because ritonavir is an inhibitor of CYP3A4, KTRs often use calcineurin inhibitors (CNIs), such as cyclosporine, tacrolimus, and mTOR inhibitors, including sirolimus and everolimus, which are highly dependent on CYP3A4 metabolism. Therefore, when CYP3A4 is exposed to ritonavir inhibition, the patient’s plasma drug levels increase significantly, increasing the risk of drug toxicity. Consequently, KTRs using nirmatrelvir/ritonavir require more frequent monitoring of blood drug concentrations (90). Although patients diagnosed with the Omicron variant may benefit from nirmatrelvir/ritonavir, these inhibitors are critical in preventing rejection reactions in KTRs, and drug interactions must be considered by clinical doctors when prescribing medication (72).
The clinical interaction between nirmatrelvir/ritonavir and immunosuppressive drugs can be effectively managed using standardized dosing schedules. In a single-center retrospective study of 14 KTRs treated with nirmatrelvir/ritonavir, the patients stopped taking tacrolimus 12 h before receiving nirmatrelvir/ritonavir treatment, were administered 20% of the cyclosporine dose, and continued taking the regular dose after completing nirmatrelvir/ritonavir treatment. In the subsequent follow-up, no deaths or viral pneumonia were observed in this study (84). A prior study conducted by Salerno et al. (91) demonstrated that when organ transplant patients adhered to a regimen involving the discontinuation of tacrolimus/mTOR inhibitors or a reduction in cyclosporine doses to 20% of the baseline daily dose over a five-day course of nirmatrelvir/ritonavir treatment, patients were able to prevent an elevation in drug concentration during the nirmatrelvir/ritonavir treatment period. Therefore, it is essential to develop standardized dosing schedules to effectively manage drug interactions and prevent harm caused by such interactions in patients.
If a patient still experiences toxicity symptoms during the use of nirmatrelvir/ritonavir, phenytoin (a CYP3A4 inducer) can be used to relieve these symptoms quickly. In the case of KTRs who develop acute tacrolimus toxicity after using nirmatrelvir/ritonavir, phenytoin can be used to rapidly decrease tacrolimus levels to within the therapeutic range. Moreover, no side effects of phenytoin (such as hypotension and arrhythmia) have been observed in KTRs (92), consistent with the case report results of Sindelar et al. (93).
4.4 Other antiviral drugs
During the COVID-19 pandemic, several antiviral drugs such as azvudine (94), ribavirin (95), and AT-527 (96) demonstrated effectiveness in treating COVID-19. However, their safety and efficacy in KTRs have not yet been characterized. In addition, SARS-CoV-1 3CLPRO and SARS-CoV-2 3CLPRO have approximately 96% amino acid sequence similarity and highly conserved active sites, suggesting that treatment agents developed for SARS-CoV-1 may also exhibit high in vitro efficacy against SARS-CoV-2 (97). Perhaps more antiviral drugs could be sought to help this special population avoid infection with Omicron.
In summary, in Omicron-affected areas, treatment guidelines for KTRs recommend remdesivir, molnupiravir, and nirmatrelvir/ritonavir. When compared with placebo, patients treated with nirmatrelvir/ritonavir exhibited the lowest risk of hospitalization or death (odds ratio [OR], 0.12; 95% confidence interval [CI]: 0.06–0.24), followed by remdesivir (OR, 0.13; 95% CI: 0.03–0.57), and then molnupiravir (OR, 0.67; 95% CI: 0.46–0.99) (98). Therefore, nirmatrelvir/ritonavir should be the first choice for doctors currently. However, due to the special status of KTRs, although nirmatrelvir/ritonavir can reduce the risk of severe COVID-19, its use in conjunction with other immunosuppressants increases drug concentration, and thus, the benefits and risks should be carefully weighed in clinical practice. Patients should use this medication under the guidance of a professional doctor, and healthcare professionals should exercise caution when assessing the prescription of nirmatrelvir/ritonavir for KTRs who are currently taking immunosuppressive drugs.
5 Vaccination for prevention of Omicron infection in KTRs
Since the emergence of the COVID-19 pandemic, researchers worldwide have worked on developing vaccines to boost population immunity and reduce SARS-CoV-2 infection risk. Large-scale vaccination efforts globally are seen as a highly effective strategy for achieving herd immunity against SARS-CoV-2 (99). During the Omicron outbreak, most people received third or fourth vaccine doses. However, it remains unclear whether COVID-19 vaccinations with the Omicron variant can effectively help KTRs, a question that remains both interesting and concerning.
5.1 Vaccination and KTRs
Vaccine administration was found to reduce the mortality rate among SARS-CoV-2-infected KTRs (100, 101). Mosconi et al. (102) also confirmed the efficacy of COVID-19 vaccines in managing the risk of infection and enhancing clinical outcomes in individuals with compromised immune systems.
Although KTRs can benefit from vaccination, previous research has shown that their humoral response to mRNA SARS-CoV-2 vaccines is reduced compared with individuals with normal immune function (103). Tauzin et al. (104) found that immunocompromised SOTRs had suboptimal efficacy following one or two vaccine doses during the COVID-19 pandemic. Moreover, another observational study found that administering the second dose of either the ChAdOx1 nCoV-19 (Oxford-AstraZeneca) or BNT162b2 (Pfizer/BioNTech) vaccine did not reduce the risk of infection (105). However, the third dose improved immunogenicity and reduced the risk of hospitalization after infection with Alpha, Beta, Gamma, and Delta variants (103). Therefore, the primary vaccination plan for transplant recipients is currently recommended to include three doses of the mRNA COVID-19 vaccine. Nevertheless, prior studies have not included the mid-term clinical outcomes of transplant recipients who have been vaccinated against the Omicron variant. This suggests a gap in our understanding of the mid-term effects of vaccination in this specific population (106).
5.2 Effects of the third vaccine on protection against Omicron in KTRs
The limited vaccine options for KTRs due to their unique immunosuppressed status make using a third dose of mRNA vaccine crucial. However, studies have shown that even after receiving three doses of mRNA vaccine, nearly half of the KTRs cannot produce sufficient levels of antibodies, thus failing to provide clinical protection (107). Therefore, it is imperative to thoroughly explore the effectiveness of administering a third vaccine dose in shielding KTRs from the potential threats posed by the Omicron variant.
The third dose of vaccine is beneficial in aiding KTRs to resist Omicron. Benning et al. (108) observed that KTRs significantly reduced levels of anti-S1 IgG, surrogate neutralization, and anti-receptor-binding domain antibodies compared with healthy controls after receiving the third dose of the vaccine. However, their ability to neutralize Omicron is lower than that of SARS-CoV-2 or other variants. Similarly, Panizo et al. (109) examined the impact of a third dose of a matching mRNA vaccine on SARS-CoV-2 RBD total antibody levels in a cohort of 21 KTRs. They detected RBD-specific antibodies in 19 of the 21 KTRs and identified neutralizing antibodies against the SARS-CoV-2, Delta, and Omicron variants, indicating the effectiveness of the third dose. Furthermore, while the neutralizing activity against Delta and Omicron variants in transplant patients remained low after receiving the third vaccine dose, the Fc-mediated effector function in SOTRs reached a level similar to that of a cohort of healthy individuals (104). Stich also indicated that while the standard mRNA vaccine regimen could weaken the humoral immune response in immunocompromised children and adolescents with kidney disease, reducing the vaccine dose could result in effective antibodies against Omicron in approximately 10% of patients (110). In addition, the study revealed that patients who did not develop humoral immune responses after the third vaccine dose either received an immunosuppressive regimen that included MMF or had an inherited T-cell defect disease.
Rodríguez-Cubillo et al. (111) also reported that compared with unvaccinated KTRs, vaccinated KTRs with at least low antibody titers (>20 AU/mL) exhibited improved outcomes in relation to pneumonia and mortality rates. Furthermore, patients with antibody titers >100 AU/mL had even better outcomes compared with those with lower antibody titers. Therefore, further research is required to explore how to improve the vaccine response in patients.
5.3 Optimizing vaccine response in KTRs
Although the third vaccine dose plays a certain role, the humoral and cellular responses of KTRs remain severely impaired after vaccination. Therefore, optimizing the vaccine response in KTRs should be the primary consideration.
5.3.1 Using additional or alternative vaccination strategies is one way to optimize the immune response to vaccination in KTRs
Sanders et al. (112) observed the immune response of 267 KTRs who received the mRNA-1273 vaccine 6 months after transplantation and compared the same with a control group of 181 individuals. The study revealed that 6 months after vaccination, S1-specific antibodies were present in 100% of the control group, 98.7% of CKD G4/5 patients, 95.1% of dialysis patients, and 56.6% of KTRs. Most participants also possessed neutralizing antibodies against SARS-CoV-2 and the Delta variants, although neutralizing antibodies against the Omicron variant were notably scarce. In addition, S-specific T-cell responses were identified in 75.0% of the control group, 69.4% of CKD G4/5 patients, 52.6% of dialysis patients, and 12.9% of KTRs at the six-month mark, with T-cell responses notably lower at 6 months compared with the levels observed 28 days after vaccination. The interplay of low antibody levels, diminished T-cell responses, and the absence of neutralization against emerging variants implies that KTRs might necessitate supplementary or alternative vaccination approaches.
Hod et al. (113) reported that a fourth dose of BNT162b vaccine could effectively reduce the incidence and severity of Omicron in KTRs. Although Del Bello et al. (107) previously suggested that delaying the fourth dose of the vaccine might enhance vaccine immunogenicity in KTRs, they concluded that timely vaccination-induced antibody concentration increases still appear insufficient to provide clinical protection for KTRs, particularly against Omicron, where breakthrough infections occur even at very high concentrations. Presently, there is conflicting data from current studies regarding the efficacy of a fourth vaccine dose in KTRs. Nevertheless, administering a fourth vaccine dose represents another effort to enhance the immune response of KTRs to vaccination.
Chang et al. (114) found that the three-dose mRNA vaccine had limited effectiveness in eliciting antiviral antibodies against all viral strains in fully vaccinated, COVID-19-naïve SOTRs. Only 34.0% of patients exhibited detectable anti-RBD IgG and neutralizing activity against the Alpha, Beta, and Delta variants, and merely 8.5% of patients demonstrated additional neutralizing capacity against Omicron. In contrast, 79.5% of convalescent SOTRs who received two vaccine doses displayed high levels of anti-RBD IgG and neutralizing activity against Omicron. These findings underscore the substantial influence of prior infection on the evolution of the immune response to SARS-CoV-2 vaccination in SOTRs, emphasizing the necessity for alternative strategies to safeguard vaccine-hyporesponsive populations.
5.3.2 Immunomodulatory strategies as one of the optimization methods for KTRs’ vaccination response
KTRs require immunosuppressants before and after transplantation to reduce the occurrence of immune rejection reactions. Studies have indicated that KTRs can significantly improve their existing vaccine protection efficacy by reducing or temporarily discontinuing the use of immunosuppressants (115). Therefore, the problem of a significant decrease in the serum conversion rate of KTRs after COVID-19 vaccination may be resolved by adjusting adaptive immunomodulatory strategies (116).
5.3.2.1 Mycophenolic acid
Mycophenolic acid (MPA) is currently the most commonly used anti-proliferative drug in clinical practice. Kühn et al. (117) observed that the humoral response to vaccination was significantly stronger in KTRs who stopped MPA 1 month after vaccination. The transplant function remained stable in KTRs who discontinued MPA use during the study period, and no rejection reactions occurred in the patients. Therefore, cessation of MPA use may be related to enhanced allogeneic immune responses. However, this effect lasted only for 3 months because in this study, a general decrease in humoral immunity was observed in all KTRs 3 months after vaccination. Subsequently, Benninng et al. (116) demonstrated that short-term discontinuation of MPA could increase the antibody titers of KTRs after vaccination. These results showed that SARS-CoV-2-specific antibodies in individuals who discontinued MPA use during vaccination increased significantly. Simultaneously, in patients who discontinued MPA for a short time, the transplantation function and the level of free DNA from donor sources remained stable, indicating that the patient’s kidney transplant status was good, and there was no acute rejection reaction during the short-term follow-up period.
Frölke et al. (118) concluded that modifying MMF/MPA therapy prior to vaccination may enhance the vaccine response in KTRs who face a heightened risk of not developing antibodies after receiving the SARS-CoV-2 vaccine. Interestingly, Regele et al. (119) found that discontinuing MPA or azathioprine (AZA) for 2 weeks did not increase the serum conversion rate of KTRs who did not respond to the previous three to four doses of SARS-CoV-2 vaccine. However, the sample size of their study was small, and immunosuppression was discontinued only briefly for 2 weeks; accordingly, no conclusion can be drawn from this trial regarding the effects of MPA/AZA discontinuation on serum conversion rates.
5.3.2.2 Mycophenolate mofetil
MMF, marketed under the brand name Cellcept, is a semi-synthetic derivative of MPA that was isolated from the fermentation broth of Penicillium glaucum. It is primarily used to prevent allograft rejection in organ transplantation (120).
Kantauskaite found that the likelihood of producing anti-SARS-CoV-2 antibodies in KTRs treated with immunosuppressive regimens that did not include MMF was 13-fold higher than that in KTRs treated with MMF-containing regimens. In KTRs treated with MMF, daily doses of MMF equal to or less than 1 g were associated with up to a five-fold increase in humoral immune response to the vaccine compared to KTRs treated with higher doses of MMF (121). Hence, MMF utilization is associated with a weakened immune response to SARS-CoV-2 vaccination in KTRs, and adjusting the MMF dosage is a viable option to enhance the humoral immune response.
In a multicenter observational study, the impact of the third SARS-CoV-2 vaccine on KTRs who were previously seronegative was analyzed. The study focused on temporarily reducing the MMF dose among propensity-matched KTRs. Among 174 previously seronegative KTRs, 56 (32%) became seropositive after the third dose, while only three KTRs developed neutralizing antibodies against the Omicron variant. The researchers concluded that reducing the MMF dose by 33% or more before vaccination is a promising strategy to enhance the effectiveness of SARS-CoV-2 vaccination in KTRs (121). Another study demonstrated that immunosuppressive regimens, excluding MMF, along with lower doses of calcineurin inhibitors and the incorporation of everolimus, were correlated with an increased rate of humoral response in elderly KTRs following COVID-19 vaccination (122). Stumpf et al. (123) also indicated that MMF/MPA is the chief mediator responsible for the delayed humoral response and decreased antibody decline in KTRs after SARS-CoV-2 mRNA vaccination. Although suspension of MMF may increase the immune response to SARS-CoV-2 vaccination, further exploration is required to ensure serum conversion.
5.3.2.3 Belatacept
CNIs can bind to calcineurin and inhibit its activity, thereby exerting immunosuppressive effects. Cyclosporine and tacrolimus are drugs commonly used in KTRs (124). Compared with CNIs, belatacept can significantly reduce the production of DSA during the transplantation process, improve the survival rate of transplants, and maximize the avoidance of CNI nephrotoxicity (125). Therefore, some immunosuppressive regimens gradually favor the use of belatacept.
Amid the COVID-19 pandemic, treating SOTRs with belatacept diminished the immune responses to SARS-CoV-2 mRNA vaccination. It is recommended that the vaccine be administered separately from belatacept infusion to increase immunogenicity (126). In a study by Wiedemann et al., 52 KTRs were observed, with 31 receiving CNIs treatment and 21 receiving belatacept treatment. After two vaccine doses, 46.9% of patients generated anti-S IgG. Among them, 21.4% in the belatacept group produced anti-spike IgG, while 83.3% in the CNIs group did. Moreover, T-cell function was notably weaker in the belatacept group compared with the CNIs group. This indicates that belatacept-based regimens significantly reduce both humoral and cellular vaccine responses in transplant patients. From a clinical standpoint, it is crucial to assess IgG responses in transplant recipients on belatacept-based regimens and devise appropriate preventive or post-exposure strategies (127, 128). Chavarot et al. (129) found that with the emergence of the third dose of the BNT162b2 mRNA COVID-19 vaccine, the antibody response of KTRs receiving belatacept treatment with the three doses of mRNA vaccine was weaker. The study by Morena et al. (130) revealed that factors related to the decrease in anti-SARS-CoV-2 antibody response after mRNA vaccination in belatacept KTRs may include the use of prednisone, age ≥ 60 years, glomerular filtration rate < 45 mL/min/1.73 m2, and no history of SARS-CoV-2 infection.
In summary, the distinctive traits of KTRs result in their limited response to vaccination. Therefore, enhancing vaccine potency is crucial to maximize their vaccine benefits. Modifying vaccine administration strategies during clinical care can boost KTRs’ immune response to SARS-CoV-2 vaccination. Additionally, further research is needed to ascertain the impact of reducing immunosuppressive drugs on post-vaccination immunity while managing the risk of rejection during vaccination-related changes in immunosuppression.
6 Conclusion and perspectives
The COVID-19 pandemic has lasted nearly 4 years, causing significant impacts on global health and the economy. The virus still threatens human health and life, and the current situation remains uncertain as infection rates fluctuate. KTRs are especially vulnerable to COVID-19 due to their extensive immunosuppressive treatment, which weakens their immune system and leads to added complications. Their reduced immunity leaves them at increased risk for COVID-19, particularly in the face of the ongoing transmission of the Omicron variant. In the next phase of the pandemic, it is crucial to prioritize developing and implementing effective preventive and control measures for those with potential immune deficiencies.
People with weakened immune systems have a reduced ability to produce protective antibodies, and mAbs and antiviral drugs can offer added protection against COVID-19. While sotrovimab, bebtelovimab, and tixagevimab/cilgavimab are suitable for KTRs, their effectiveness has waned with further Omicron mutations. Therefore, more research is warranted to develop new mAbs or mAb combinations for COVID-19 prevention and treatment in KTRs, offering safe, long-lasting protection in addition to vaccination. Although antiviral drugs can also benefit KTRs, they may interact with other immunosuppressive drugs, potentially increasing drug levels in the blood. Hence, clinical guidelines should be updated to address this trade-off.
As immunosuppressed individuals, KTRs are at higher risk of severe COVID-19. Thus, timely vaccination is crucial for them despite potentially reduced vaccine response. Vaccination has been proven to significantly decrease COVID-19 severity and mortality. Therefore, it is advisable to urge KTRs in stable post-transplantation phases to get vaccinated against COVID-19.
Additionally, with the ongoing emergence of new virus variantssuch as XBB and its lineage EG.5, which can evade host immune defenses, developing next-generation vaccines and alternative therapeutic approaches, including passive immunization, post-exposure prophylaxis, and cellular therapy, has gained heightened significance to enhance safeguarding against vaccine inadequacy. Additionally, considering the measurement of spike antibody titers and serum neutralization as routine indicators of vaccine response, mAbs, alternative vaccine regimens, or a reduction in immunosuppression could be selectively provided for KTRs. Adjusting immunosuppressive therapy strategies may improve vaccine responsiveness. However, it also exposes the potential risks of acute or chronic rejection. Therefore, reducing or temporarily stopping immunosuppressive treatment policies remains a dilemma when it comes to improving humoral responses.
Currently, the global COVID-19 situation remains uneven, with some regions experiencing low numbers of new cases, whereas others continue to persist. Many countries experienced repeated peaks during the epidemic. In the future, vulnerable populations, such as the elderly and those with weakened immunity or underlying conditions, must be the prime focus of prevention and control. Vaccines, specific drugs, and social distancing are effective measures against Omicron. As a susceptible population, KTRs require greater attention and effort to protect themselves. Further research is crucial to establish appropriate prevention and treatment guidelines.
Author contributions
ZZ: Conceptualization, Data curation, Investigation, Supervision, Validation, Visualization, Writing – original draft, Writing – review & editing. HS: Conceptualization, Funding acquisition, Investigation, Supervision, Writing – original draft. XH: Conceptualization, Validation, Visualization, Writing – review & editing. ZX: Conceptualization, Validation, Writing – review & editing. MF: Writing – review & editing. YB: Visualization, Writing – review & editing. YD: Visualization, Writing – review & editing. BL: Visualization, Writing – review & editing. XS: Validation, Writing – review & editing. JZ: Conceptualization, Project administration, Writing – review & editing. CS: Investigation, Project administration, Supervision, Writing – original draft, Writing – review & editing.
Funding
The author(s) declare that financial support was received for the research, authorship, and/or publication of this article. This study was supported by the funding of Natural Science Foundation of China (No. 81972373) and the Natural Science Foundation of Fujian (No. 2023D008).
Acknowledgments
We also thank Fiedraw (www.fiedraw.com) for the assistance in creating scheme.
Conflict of interest
The authors declare that the research was conducted in the absence of any commercial or financial relationships that could be construed as a potential conflict of interest.
Publisher’s note
All claims expressed in this article are solely those of the authors and do not necessarily represent those of their affiliated organizations, or those of the publisher, the editors and the reviewers. Any product that may be evaluated in this article, or claim that may be made by its manufacturer, is not guaranteed or endorsed by the publisher.
References
1. Dharia, A, Boulet, J, Sridhar, VS, and Kitchlu, A. Cancer screening in solid organ transplant recipients: a focus on screening liver, lung, and kidney recipients for cancers related to the transplanted organ. Transplantation. (2022) 106:e64–5. doi: 10.1097/tp.0000000000003773
2. Loupy, A, Mengel, M, and Haas, M. Thirty years of the international Banff classification for allograft pathology: the past, present, and future of kidney transplant diagnostics. Kidney Int. (2022) 101:678–91. doi: 10.1016/j.kint.2021.11.028
3. Kalantar-Zadeh, K, Jafar, TH, Nitsch, D, Neuen, BL, and Perkovic, V. Chronic kidney disease. Lancet. (2021) 398:786–802. doi: 10.1016/s0140-6736(21)00519-5
4. Abecassis, M, Bartlett, ST, Collins, AJ, Davis, CL, Delmonico, FL, Friedewald, JJ, et al. Kidney transplantation as primary therapy for end-stage renal disease: a National Kidney Foundation/kidney disease outcomes quality initiative (NKF/KDOQITM) conference. Clin J Am Soc Nephrol. (2008) 3:471–80. doi: 10.2215/cjn.05021107
5. Wolfe, RA, Ashby, VB, Milford, EL, Ojo, AO, Ettenger, RE, Agodoa, LYC, et al. Comparison of mortality in all patients on dialysis, patients on dialysis awaiting transplantation, and recipients of a first cadaveric transplant. N Engl J Med. (1999) 341:1725–30. doi: 10.1056/nejm199912023412303
6. Hu, B, Guo, H, Zhou, P, and Shi, ZL. Characteristics of SARS-CoV-2 and COVID-19. Nat Rev Microbiol. (2021) 19:141–54. doi: 10.1038/s41579-020-00459-7
7. Cravedi, P, Mothi, SS, Azzi, Y, Haverly, M, Farouk, SS, Pérez-Sáez, MJ, et al. COVID-19 and kidney transplantation: results from the TANGO international transplant consortium. Am J Transplant. (2020) 20:3140–8. doi: 10.1111/ajt.16185
8. Fan, Y, Li, X, Zhang, L, Wan, S, Zhang, L, and Zhou, F. SARS-CoV-2 omicron variant: recent progress and future perspectives. Signal Transduct Target Ther. (2022) 7:141. doi: 10.1038/s41392-022-00997-x
9. de Meester, J, de Bacquer, D, Naesens, M, Meijers, B, Couttenye, MM, de Vriese, AS, et al. Incidence, characteristics, and outcome of COVID-19 in adults on kidney replacement therapy: a Regionwide registry study. J Am Soc Nephrol. (2021) 32:385–96. doi: 10.1681/ASN.2020060875
10. Akalin, E, Azzi, Y, Bartash, R, Seethamraju, H, Parides, M, Hemmige, V, et al. Covid-19 and kidney transplantation. N Engl J Med. (2020) 382:2475–7. doi: 10.1056/NEJMc2011117
11. Han, X, and Ye, Q. Kidney involvement in COVID-19 and its treatments. J Med Virol. (2021) 93:1387–95. doi: 10.1002/jmv.26653
12. Sagnelli, C, Sica, A, Gallo, M, Peluso, G, Varlese, F, D’Alessandro, V, et al. Renal involvement in COVID-19: focus on kidney transplant sector. Infection. (2021) 49:1265–75. doi: 10.1007/s15010-021-01706-6
13. Caillard, S, Chavarot, N, Francois, H, Matignon, M, Greze, C, Kamar, N, et al. Is COVID-19 infection more severe in kidney transplant recipients? Am J Transplant. (2021) 21:1295–303. doi: 10.1111/ajt.16424
14. Mahalingasivam, V, Su, G, Iwagami, M, Davids, MR, Wetmore, JB, and Nitsch, D. COVID-19 and kidney disease: insights from epidemiology to inform clinical practice. Nat Rev Nephrol. (2022) 18:485–98. doi: 10.1038/s41581-022-00570-3
15. Fisher, AM, Schlauch, D, Mulloy, M, Dao, A, Reyad, AI, Correll, M, et al. Outcomes of COVID-19 in hospitalized solid organ transplant recipients compared to a matched cohort of non-transplant patients at a national healthcare system in the United States. Clin Transpl. (2021) 35:e14216. doi: 10.1111/ctr.14216
16. Chen, N, Zhou, M, Dong, X, Qu, J, Gong, F, Han, Y, et al. Epidemiological and clinical characteristics of 99 cases of 2019 novel coronavirus pneumonia in Wuhan, China: a descriptive study. Lancet. (2020) 395:507–13. doi: 10.1016/S0140-6736(20)30211-7
17. Diao, B, Wang, C, Wang, R, Feng, Z, Zhang, J, Yang, H, et al. Human kidney is a target for novel severe acute respiratory syndrome coronavirus 2 infection. Nat Commun. (2021) 12:2506. doi: 10.1038/s41467-021-22781-1
18. Ronco, C, Tetta, C, Mariano, F, Wratten, ML, Bonello, M, Bordoni, V, et al. Interpreting the mechanisms of continuous renal replacement therapy in sepsis: the peak concentration hypothesis. Artif Organs. (2003) 27:792–801. doi: 10.1046/j.1525-1594.2003.07289.x
19. Gheblawi, M, Wang, K, Viveiros, A, Nguyen, Q, Zhong, JC, Turner, AJ, et al. Angiotensin-converting enzyme 2: SARS-CoV-2 receptor and regulator of the renin-angiotensin system: celebrating the 20th anniversary of the discovery of ACE2. Circ Res. (2020) 126:1456–74. doi: 10.1161/CIRCRESAHA.120.317015
20. Wang, W, Xu, Y, Gao, R, Lu, R, Han, K, Wu, G, et al. Detection of SARS-CoV-2 in different types of clinical specimens. JAMA. (2020) 323:1843–4. doi: 10.1001/jama.2020.3786
21. Kawai, T, and Akira, S. The role of pattern-recognition receptors in innate immunity: update on toll-like receptors. Nat Immunol. (2010) 11:373–84. doi: 10.1038/ni.1863
22. Stetson, DB, and Medzhitov, R. Type I interferons in host defense. Immunity. (2006) 25:373–81. doi: 10.1016/j.immuni.2006.08.007
23. Huang, C, Wang, Y, Li, X, Ren, L, Zhao, J, Hu, Y, et al. Clinical features of patients infected with 2019 novel coronavirus in Wuhan, China. Lancet. (2020) 395:497–506. doi: 10.1016/s0140-6736(20)30183-5
24. Li, XQ, Liu, H, Meng, Y, Yin, HY, Gao, WY, Yang, X, et al. Critical roles of cytokine storm and secondary bacterial infection in acute kidney injury development in COVID-19: a multi-center retrospective cohort study. J Med Virol. (2021) 93:6641–52. doi: 10.1002/jmv.27234
25. Cosar, B, Karagulleoglu, ZY, Unal, S, Ince, AT, Uncuoglu, DB, Tuncer, G, et al. SARS-CoV-2 mutations and their viral variants. Cytokine Growth Factor Rev. (2022) 63:10–22. doi: 10.1016/j.cytogfr.2021.06.001
26. Thakur, V, and Ratho, RK. OMICRON (B.1.1.529): a new SARS-CoV-2 variant of concern mounting worldwide fear. J Med Virol. (2022) 94:1821–4. doi: 10.1002/jmv.27541
27. Cristelli, MP, Rissoni, RAP, Viana, LA, Tedesco-Silva, H, and Medina-Pestana, J. How did the omicron surge affect kidney transplant recipients compared with a cohort from the general population? Transplantation. (2022) 106:e382–3. doi: 10.1097/tp.0000000000004193
28. Villanego, F, Vigara, LA, Alonso, M, Orellana, C, Gómez, AM, Eady, M, et al. Trends in COVID-19 outcomes in kidney transplant recipients during the period of omicron variant predominance. Transplantation. (2022) 106:e304–5. doi: 10.1097/tp.0000000000004126
29. Anjan, S, Khatri, A, Viotti, JB, Cheung, T, Garcia, LAC, Simkins, J, et al. Is the omicron variant truly less virulent in solid organ transplant recipients? Transpl Infect Dis. (2022) 24:e13923. doi: 10.1111/tid.13923
30. Hwang, YC, Lu, RM, Su, SC, Chiang, PY, Ko, SH, Ke, FY, et al. Monoclonal antibodies for COVID-19 therapy and SARS-CoV-2 detection. J Biomed Sci. (2022) 29:1. doi: 10.1186/s12929-021-00784-w
31. Klein, EJ, Hardesty, A, Vieira, K, and Farmakiotis, D. Use of anti-spike monoclonal antibodies in kidney transplant recipients with COVID-19: efficacy, ethnic and racial disparities. Am J Transplant. (2022) 22:640–5. doi: 10.1111/ajt.16843
32. Takashita, E, Kinoshita, N, Yamayoshi, S, Sakai-Tagawa, Y, Fujisaki, S, Ito, M, et al. Efficacy of antibodies and antiviral drugs against Covid-19 omicron variant. N Engl J Med. (2022) 386:995–8. doi: 10.1056/NEJMc2119407
34. Dhand, A, Okumura, K, Wolfe, K, Lobo, SA, Nog, R, Keller, M, et al. Sotrovimab for treatment of COVID-19 in solid organ transplant recipients. Transplantation. (2022) 106:e336–7. doi: 10.1097/tp.0000000000004136
35. Pinchera, B, Buonomo, AR, Scotto, R, Carrano, R, Salemi, F, Galluccio, F, et al. Sotrovimab in solid organ transplant patients with early, mild/moderate SARS-CoV-2 infection: a single-center experience. Transplantation. (2022) 106:e343–5. doi: 10.1097/tp.0000000000004150
36. Piccicacco, N, Zeitler, K, Ing, A, Montero, J, Faughn, J, Silbert, S, et al. Real-world effectiveness of early remdesivir and sotrovimab in the highest-risk COVID-19 outpatients during the omicron surge. J Antimicrob Chemother. (2022) 77:2693–700. doi: 10.1093/jac/dkac256
37. Radcliffe, C, Palacios, CF, Azar, MM, Cohen, E, and Malinis, M. Real-world experience with available, outpatient COVID-19 therapies in solid organ transplant recipients during the omicron surge. Am J Transplant. (2022) 22:2458–63. doi: 10.1111/ajt.17098
38. Fernandes, G, Devresse, A, Scohy, A, de Greef, J, Yombi, JC, Belkhir, L, et al. Monoclonal antibody therapy in kidney transplant recipients with Delta and omicron variants of SARS-CoV-2: a single-center Case series. Kidney Med. (2022) 4:100470. doi: 10.1016/j.xkme.2022.100470
39. di Chiara, C, Mengato, D, de Pieri, M, Longo, G, Benetti, E, Venturini, F, et al. Early use of Sotrovimab in children: a Case report of an 11-year-old kidney transplant recipient infected with SARS-CoV-2. Children (Basel). (2022) 9:451. doi: 10.3390/children9040451
40. Tao, K, Tzou, PL, Kosakovsky Pond, SL, Ioannidis, JPA, and Shafer, RW. Susceptibility of SARS-CoV-2 omicron variants to therapeutic monoclonal antibodies: systematic review and Meta-analysis. Microbiol Spectr. (2022) 10:e0092622. doi: 10.1128/spectrum.00926-22
41. Yamasoba, D, Kosugi, Y, Kimura, I, Fujita, S, Uriu, K, Ito, J, et al. Neutralisation sensitivity of SARS-CoV-2 omicron subvariants to therapeutic monoclonal antibodies. Lancet Infect Dis. (2022) 22:942–3. doi: 10.1016/s1473-3099(22)00365-6
42. McCreary, EK, Kip, KE, Collins, K, Minnier, TE, Snyder, GM, Steiner, A, et al. Evaluation of Bebtelovimab for treatment of Covid-19 during the SARS-CoV-2 omicron variant era. Open Forum Infect Dis. (2022) 9:ofac517. doi: 10.1093/ofid/ofac517
43. Shertel, T, Lange, NW, Salerno, DM, Hedvat, J, Jennings, DL, Choe, JY, et al. Bebtelovimab for treatment of COVID-19 in ambulatory solid organ transplant recipients. Transplantation. (2022) 106:e463–4. doi: 10.1097/tp.0000000000004278
44. Yetmar, ZA, Beam, E, O'Horo, JC, Seville, MT, Brumble, L, Ganesh, R, et al. Outcomes of bebtelovimab and sotrovimab treatment of solid organ transplant recipients with mild-to-moderate coronavirus disease 2019 during the omicron epoch. Transpl Infect Dis. (2022) 24:e13901. doi: 10.1111/tid.13901
45. Nguyen, Y, Flahault, A, Chavarot, N, Melenotte, C, Cheminant, M, Deschamps, P, et al. Pre-exposure prophylaxis with tixagevimab and cilgavimab (Evusheld) for COVID-19 among 1112 severely immunocompromised patients. Clin Microbiol Infect. (2022) 28:1654.e1–4. doi: 10.1016/j.cmi.2022.07.015
46. DeWolf, S, Laracy, JC, Perales, MA, Kamboj, M, van den Brink, MRM, and Vardhana, S. SARS-CoV-2 in immunocompromised individuals. Immunity. (2022) 55:1779–98. doi: 10.1016/j.immuni.2022.09.006
47. Levin, MJ, Ustianowski, A, de Wit, S, Launay, O, Avila, M, Templeton, A, et al. Intramuscular AZD7442 (Tixagevimab-Cilgavimab) for prevention of Covid-19. N Engl J Med. (2022) 386:2188–200. doi: 10.1056/NEJMoa2116620
48. Focosi, D, and Casadevall, A. A critical analysis of the use of Cilgavimab plus Tixagevimab monoclonal antibody cocktail (Evusheld™) for COVID-19 prophylaxis and treatment. Viruses. (2022) 14:1999. doi: 10.3390/v14091999
49. Touret, F, Baronti, C, Pastorino, B, Villarroel, PMS, Ninove, L, Nougairède, A, et al. In vitro activity of therapeutic antibodies against SARS-CoV-2 omicron BA.1, BA.2 and BA.5. Sci Rep. (2022) 12:12609. doi: 10.1038/s41598-022-16964-z
50. Ducloux, D, and Courivaud, C. REGEN-Cov antibody combination to prevent COVID-19 infection in kidney transplant recipient without detectable antibody response to optimal vaccine scheme. Kidney Int. (2022) 101:645–6. doi: 10.1016/j.kint.2021.12.015
51. Touret, F, Martin-Blondel, G, de Lamballerie, X, Dupont, A, Izopet, J, Mentré, F, et al. Low to undetectable omicron BQ.1.1 neutralization by patient's sera a month after initiation of AZD7442 600 mg. J Infect. (2023) 86:e126–9. doi: 10.1016/j.jinf.2023.02.002
52. Benotmane, I, Velay, A, Vargas, GG, Olagne, J, Cognard, N, Heibel, F, et al. A rapid decline in the anti-receptor-binding domain of the SARS-CoV-2 spike protein IgG titer in kidney transplant recipients after tixagevimab-cilgavimab administration. Kidney Int. (2022) 102:1188–90. doi: 10.1016/j.kint.2022.07.022
53. Al Jurdi, A, Morena, L, Cote, M, Bethea, E, Azzi, J, and Riella, LV. Tixagevimab/cilgavimab pre-exposure prophylaxis is associated with lower breakthrough infection risk in vaccinated solid organ transplant recipients during the omicron wave. Am J Transplant. (2022) 22:3130–6. doi: 10.1111/ajt.17128
54. Benotmane, I, Velay, A, Gautier-Vargas, G, Olagne, J, Obrecht, A, Cognard, N, et al. Breakthrough COVID-19 cases despite prophylaxis with 150 mg of tixagevimab and 150 mg of cilgavimab in kidney transplant recipients. Am J Transplant. (2022) 22:2675–81. doi: 10.1111/ajt.17121
55. Imai, M, Ito, M, Kiso, M, Yamayoshi, S, Uraki, R, Fukushi, S, et al. Efficacy of antiviral agents against omicron subvariants BQ.1.1 and XBB. N Engl J Med. (2023) 388:89–91. doi: 10.1056/NEJMc2214302
56. Dhand, A, Lobo, SA, Wolfe, K, Feola, N, Lee, L, Nog, R, et al. Casirivimab-imdevimab for treatment of COVID-19 in solid organ transplant recipients: an early experience. Transplantation. (2021) 105:e68–9. doi: 10.1097/tp.0000000000003737
57. Kijima, Y, Shimizu, T, Kato, S, Kano, K, Horiuchi, T, Nozaki, T, et al. Case report of COVID-19 infection after kidney transplant treated with Casirivimab-Imdevimab and mycophenolate Mofetil changed to Everolimus. Transplant Proc. (2022) 54:1561–3. doi: 10.1016/j.transproceed.2021.12.018
58. Planas, D, Saunders, N, Maes, P, Guivel-Benhassine, F, Planchais, C, Buchrieser, J, et al. Considerable escape of SARS-CoV-2 omicron to antibody neutralization. Nature. (2022) 602:671–5. doi: 10.1038/s41586-021-04389-z
59. Hoffmann, M, Krüger, N, Schulz, S, Cossmann, A, Rocha, C, Kempf, A, et al. The omicron variant is highly resistant against antibody-mediated neutralization: implications for control of the COVID-19 pandemic. Cell. (2022) 185:447–456.e11. doi: 10.1016/j.cell.2021.12.032
60. Sethi, S, Peng, A, Najjar, R, Vo, A, Jordan, SC, and Huang, E. Infectious complications in tocilizumab-treated kidney transplant recipients. Transplantation. (2021) 105:1818–24. doi: 10.1097/tp.0000000000003512
61. Laffont-Lozes, P, Laureillard, D, Loubet, P, Stephan, R, Chiaruzzi, M, Clemmer, E, et al. Effect of tocilizumab on mortality in patients with SARS-CoV-2 pneumonia caused by Delta or omicron variants: a propensity-matched analysis in Nimes university hospital, France. Antibiotics (Basel). (2023) 12:88. doi: 10.3390/antibiotics12010088
62. Chen, P, Nirula, A, Heller, B, Gottlieb, RL, Boscia, J, Morris, J, et al. SARS-CoV-2 neutralizing antibody LY-CoV555 in outpatients with Covid-19. N Engl J Med. (2021) 384:229–37. doi: 10.1056/NEJMoa2029849
63. Bansal, K, and Kumar, S. Mutational cascade of SARS-CoV-2 leading to evolution and emergence of omicron variant. Virus Res. (2022) 315:198765. doi: 10.1016/j.virusres.2022.198765
64. Agostini, ML, Andres, EL, Sims, AC, Graham, RL, Sheahan, TP, Lu, X, et al. Coronavirus susceptibility to the antiviral Remdesivir (GS-5734) is mediated by the viral polymerase and the proofreading exoribonuclease. MBio. (2018) 9:e00221-18. doi: 10.1128/mBio.00221-18
65. Hirai, T, Mizuta, A, Sasaki, T, Nishikawa, K, Inoue, T, and Iwamoto, T. Drug-drug interaction between remdesivir and immunosuppressant agents in a kidney transplant recipient. Int J Clin Pharmacol Ther. (2022) 60:439–44. doi: 10.5414/CP204239
66. Gérard, AO, Laurain, A, Fresse, A, Parassol, N, Muzzone, M, Rocher, F, et al. Remdesivir and acute renal failure: a potential safety signal from disproportionality analysis of the WHO safety database. Clin Pharmacol Ther. (2021) 109:1021–4. doi: 10.1002/cpt.2145
67. Meshram, HS, Kute, VB, Patel, H, Banerjee, S, Navadiya, V, Desai, S, et al. Feasibility and safety of remdesivir in SARS-CoV2 infected renal transplant recipients: a retrospective cohort from a developing nation. Transpl Infect Dis. (2021) 23:e13629. doi: 10.1111/tid.13629
68. Elec, F, Magnusson, J, Elec, A, Muntean, A, Antal, O, Moisoiu, T, et al. COVID-19 and kidney transplantation: the impact of remdesivir on renal function and outcome - a retrospective cohort study. Int J Infect Dis. (2022) 118:247–53. doi: 10.1016/j.ijid.2022.03.015
69. Gottlieb, RL, Vaca, CE, Paredes, R, Mera, J, Webb, BJ, Perez, G, et al. Early Remdesivir to prevent progression to severe Covid-19 in outpatients. N Engl J Med. (2022) 386:305–15. doi: 10.1056/NEJMoa2116846
70. Cacho, J, Nicolás, D, Bodro, M, Cuadrado-Payán, E, Torres-Jaramillo, V, Gonzalez-Rojas, Á, et al. Use of remdesivir in kidney transplant recipients with SARS-CoV-2 omicron infection. Kidney Int. (2022) 102:917–21. doi: 10.1016/j.kint.2022.08.001
71. Bai, Y, Shen, M, and Zhang, L. Antiviral efficacy of Molnupiravir for COVID-19 treatment. Viruses. (2022) 14:36–45. doi: 10.3390/v14040763
72. Villamarín, M, Márquez-Algaba, E, Esperalba, J, Perelló, M, Los Arcos, I, Campany, D, et al. Preliminary clinical experience of Molnupiravir to prevent progression of COVID-19 in kidney transplant recipients. Transplantation. (2022) 106:2200–4. doi: 10.1097/tp.0000000000004306
73. Poznański, P, Augustyniak-Bartosik, H, Magiera-Żak, A, Skalec, K, Jakuszko, K, Mazanowska, O, et al. Molnupiravir when used alone seems to be safe and effective as outpatient COVID-19 therapy for Hemodialyzed patients and kidney transplant recipients. Viruses. (2022) 14:2224. doi: 10.3390/v14102224
74. Li, P, Wang, Y, Lavrijsen, M, Lamers, MM, de Vries, AC, Rottier, RJ, et al. SARS-CoV-2 omicron variant is highly sensitive to molnupiravir, nirmatrelvir, and the combination. Cell Res. (2022) 32:322–4. doi: 10.1038/s41422-022-00618-w
75. Fiaschi, L, Dragoni, F, Schiaroli, E, Bergna, A, Rossetti, B, Giammarino, F, et al. Efficacy of licensed monoclonal antibodies and antiviral agents against the SARS-CoV-2 omicron sublineages BA.1 and BA.2. Viruses. (2022) 14:1374. doi: 10.3390/v14071374
76. Wong, CKH, Au, ICH, Lau, KTK, Lau, EHY, Cowling, BJ, and Leung, GM. Real-world effectiveness of molnupiravir and nirmatrelvir plus ritonavir against mortality, hospitalisation, and in-hospital outcomes among community-dwelling, ambulatory patients with confirmed SARS-CoV-2 infection during the omicron wave in Hong Kong: an observational study. Lancet. (2022) 400:1213–22. doi: 10.1016/s0140-6736(22)01586-0
77. Zheng, Q, Ma, P, Wang, M, Cheng, Y, Zhou, M, Ye, L, et al. Efficacy and safety of Paxlovid for COVID-19:a meta-analysis. J Infect. (2023) 86:66–117. doi: 10.1016/j.jinf.2022.09.027
78. Najjar-Debbiny, R, Gronich, N, Weber, G, Khoury, J, Amar, M, Stein, N, et al. Effectiveness of Paxlovid in reducing severe coronavirus disease 2019 and mortality in high-risk patients. Clin Infect Dis. (2023) 76:e342–9. doi: 10.1093/cid/ciac443
79. Ionescu, MI. An overview of the crystallized structures of the SARS-CoV-2. Protein J. (2020) 39:600–18. doi: 10.1007/s10930-020-09933-w
80. Hammond, J, Leister-Tebbe, H, Gardner, A, Abreu, P, Bao, W, Wisemandle, W, et al. Oral Nirmatrelvir for high-risk, nonhospitalized adults with Covid-19. N Engl J Med. (2022) 386:1397–408. doi: 10.1056/NEJMoa2118542
81. Marzolini, C, Kuritzkes, DR, Marra, F, Boyle, A, Gibbons, S, Flexner, C, et al. Recommendations for the Management of Drug-Drug Interactions between the COVID-19 antiviral Nirmatrelvir/ritonavir (Paxlovid) and Comedications. Clin Pharmacol Ther. (2022) 112:1191–200. doi: 10.1002/cpt.2646
82. Focosi, D, McConnell, S, Shoham, S, Casadevall, A, Maggi, F, and Antonelli, G. Nirmatrelvir and COVID-19: development, pharmacokinetics, clinical efficacy, resistance, relapse, and pharmacoeconomics. Int J Antimicrob Agents. (2023) 61:106708. doi: 10.1016/j.ijantimicag.2022.106708
83. Zhong, W, Jiang, X, Yang, X, Feng, T, Duan, Z, Wang, W, et al. The efficacy of paxlovid in elderly patients infected with SARS-CoV-2 omicron variants: results of a non-randomized clinical trial. Front Med (Lausanne). (2022) 9:980002. doi: 10.3389/fmed.2022.980002
84. Devresse, A, Briol, S, de Greef, J, Lemaitre, F, Boland, L, Haufroid, V, et al. Safety, efficacy, and relapse of Nirmatrelvir-ritonavir in kidney transplant recipients infected with SARS-CoV-2. Kidney Int Rep. (2022) 7:2356–63. doi: 10.1016/j.ekir.2022.08.026
85. Belden, KA, Yeager, S, Schulte, J, Cantarin, MPM, Moss, S, Royer, T, et al. Saving lives with nirmatrelvir/ritonavir one transplant patient at a time. Transpl Infect Dis. (2023) 25:e14037. doi: 10.1111/tid.14037
86. Lemaitre, F, Grégoire, M, Monchaud, C, Bouchet, S, Saint-Salvi, B, and Polard, E. Management of drug-drug interactions with nirmatrelvir/ritonavir in patients treated for Covid-19: guidelines from the French Society of Pharmacology and Therapeutics (SFPT). Therapie. (2022) 77:509–21. doi: 10.1016/j.therap.2022.03.005
87. Berar Yanay, N, Bogner, I, Saker, K, and Tannous, E. Paxlovid-tacrolimus drug-drug interaction in a 23-year-old female kidney transplant patient with COVID-19. Clin Drug Investig. (2022) 42:693–5. doi: 10.1007/s40261-022-01180-4
88. Young, C, Papiro, T, and Greenberg, JH. Elevated tacrolimus levels after treatment with nirmatrelvir/ritonavir (Paxlovid) for COVID-19 infection in a child with a kidney transplant. Pediatr Nephrol. (2023) 38:1387–8. doi: 10.1007/s00467-022-05712-0
89. Prikis, M, and Cameron, A. Paxlovid (Nirmatelvir/ritonavir) and tacrolimus drug-drug interaction in a kidney transplant patient with SARS-2-CoV infection: a Case report. Transplant Proc. (2022) 54:1557–60. doi: 10.1016/j.transproceed.2022.04.015
90. Hsu, A, Granneman, GR, and Bertz, RJ. Ritonavir. Clinical pharmacokinetics and interactions with other anti-HIV agents. Clin Pharmacokinet. (1998) 35:275–91. doi: 10.2165/00003088-199835040-00002
91. Salerno, DM, Jennings, DL, Lange, NW, Kovac, D(B), Shertel, T, Chen, JK, et al. Early clinical experience with nirmatrelvir/ritonavir for the treatment of COVID-19 in solid organ transplant recipients. Am J Transplant. (2022) 22:2083–8. doi: 10.1111/ajt.17027
92. Kwon, EJ, Yun, GA, Park, S, Kim, S, Chae, DW, Park, HS, et al. Treatment of acute tacrolimus toxicity with phenytoin after Paxlovid (nirmatrelvir/ritonavir) administration in a kidney transplant recipient. Kidney Res Clin Pract. (2022) 41:768–70. doi: 10.23876/j.krcp.22.218
93. Sindelar, M, McCabe, D, and Carroll, E. Tacrolimus drug-drug interaction with Nirmatrelvir/ritonavir (Paxlovid™) managed with phenytoin. J Med Toxicol. (2023) 19:45–8. doi: 10.1007/s13181-022-00922-2
94. Zhang, JL, Li, YH, Wang, LL, Liu, HQ, Lu, SY, Liu, Y, et al. Azvudine is a thymus-homing anti-SARS-CoV-2 drug effective in treating COVID-19 patients. Signal Transduct Target Ther. (2021) 6:414. doi: 10.1038/s41392-021-00835-6
95. Drożdżal, S, Rosik, J, Lechowicz, K, Machaj, F, Szostak, B, Przybyciński, J, et al. An update on drugs with therapeutic potential for SARS-CoV-2 (COVID-19) treatment. Drug Resist Updat. (2021) 59:100794. doi: 10.1016/j.drup.2021.100794
96. Good, SS, Westover, J, Jung, KH, Zhou, XJ, Moussa, A, la Colla, P, et al. AT-527, a double prodrug of a guanosine nucleotide analog, is a potent inhibitor of SARS-CoV-2 in vitro and a promising Oral antiviral for treatment of COVID-19. Antimicrob Agents Chemother. (2021) 65:e02479-20. doi: 10.1128/aac.02479-20
97. Lata, S, and Akif, M. Comparative protein structure network analysis on 3CL(pro) from SARS-CoV-1 and SARS-CoV-2. Proteins. (2021) 89:1216–25. doi: 10.1002/prot.26143
98. Lai, CC, Wang, YH, Chen, KH, Chen, CH, and Wang, CY. The clinical efficacy and safety of anti-viral agents for non-hospitalized patients with COVID-19: a systematic review and network Meta-analysis of randomized controlled trials. Viruses. (2022) 14:1706. doi: 10.3390/v14081706
99. Araf, Y, Akter, F, Tang, YD, Fatemi, R, Parvez, MSA, Zheng, C, et al. Omicron variant of SARS-CoV-2: genomics, transmissibility, and responses to current COVID-19 vaccines. J Med Virol. (2022) 94:1825–32. doi: 10.1002/jmv.27588
100. Rivera, J, Mendoza, MS, Covarrubias, LG, Ramírez, ALQ, Reyes, LC, Hernández, MB, et al. Mortality due to COVID-19 in renal transplant recipients, related to variants of SARS-CoV-2 and vaccination in Mexico. Transplant Proc. (2022) 54:1434–8. doi: 10.1016/j.transproceed.2022.05.010
101. Kodali, L, Budhiraja, P, and Gea-Banacloche, J. COVID-19 in kidney transplantation-implications for immunosuppression and vaccination. Front Med (Lausanne). (2022) 9:1060265. doi: 10.3389/fmed.2022.1060265
102. Mosconi, G, Fantini, M, Righini, M, Flachi, M, Semprini, S, Hu, L, et al. Efficacy of SARS-CoV-2 vaccination in dialysis patients: epidemiological analysis and evaluation of the clinical progress. J Clin Med. (2022) 11:4723. doi: 10.3390/jcm11164723
103. Kamar, N, Abravanel, F, Marion, O, Couat, C, Izopet, J, and Del Bello, A. Three doses of an mRNA Covid-19 vaccine in solid-organ transplant recipients. N Engl J Med. (2021) 385:661–2. doi: 10.1056/NEJMc2108861
104. Tauzin, A, Beaudoin-Bussières, G, Gong, SY, Chatterjee, D, Gendron-Lepage, G, Bourassa, C, et al. Humoral immune responses against SARS-CoV-2 spike variants after mRNA vaccination in solid organ transplant recipients. iScience. (2022) 25:104990. doi: 10.1016/j.isci.2022.104990
105. Boyarsky, BJ, Werbel, WA, Avery, RK, Tobian, AAR, Massie, AB, Segev, DL, et al. Antibody response to 2-dose SARS-CoV-2 mRNA vaccine series in solid organ transplant recipients. JAMA. (2021) 325:2204–6. doi: 10.1001/jama.2021.7489
106. Wong, G, Rowlandson, M, Sabanayagam, D, Ginn, AN, Kable, K, Sciberras, F, et al. COVID-19 infection with the omicron SARS-CoV-2 variant in a cohort of kidney and kidney pancreas transplant recipients: clinical features, risk factors, and outcomes. Transplantation. (2022) 106:1860–6. doi: 10.1097/tp.0000000000004203
107. Del Bello, A, Kamar, N, Marion, O, Izopet, J, and Abravanel, F. Humoral and cellular responses to a delayed fourth SARS-CoV-2 mRNA-based vaccine in weak responders to 3 doses kidney transplant recipients. Vaccines (Basel). (2022) 10:1439. doi: 10.3390/vaccines10091439
108. Benning, L, Morath, C, Bartenschlager, M, Kim, H, Reineke, M, Beimler, J, et al. Neutralizing antibody response against the B.1.617.2 (delta) and the B.1.1.529 (omicron) variants after a third mRNA SARS-CoV-2 vaccine dose in kidney transplant recipients. Am J Transplant. (2022) 22:1873–83. doi: 10.1111/ajt.17054
109. Panizo, N, Giménez, E, Albert, E, Zulaica, J, Rodríguez-Moreno, A, Rusu, L, et al. SARS-CoV-2-spike antibody and T-cell responses elicited by a homologous third mRNA COVID-19 dose in hemodialysis and kidney transplant recipients. Microorganisms. (2022) 10:2275. doi: 10.3390/microorganisms10112275
110. Stich, M, di Cristanziano, V, Tönshoff, B, Weber, LT, Dötsch, J, Rammer, MT, et al. Humoral immune response and live-virus neutralization of the SARS-CoV-2 omicron (BA.1) variant after COVID-19 mRNA vaccination in children and young adults with chronic kidney disease. Pediatr Nephrol. (2022) 38:1935–48. doi: 10.1007/s00467-022-05806-9
111. Rodríguez-Cubillo, B, Moreno de la Higuera, MA, Pérez-Flores, I, Calvo Romero, N, Aiffil, AS, Arribi Vilela, A, et al. Clinical effectiveness of SARS-CoV-2 vaccination in renal transplant recipients. Antibody levels impact in pneumonia and death. Transplantation. (2022) 106:e476–87. doi: 10.1097/tp.0000000000004261
112. Sanders, JF, Messchendorp, AL, de Vries, RD, Baan, CC, van Baarle, D, van Binnendijk, R, et al. Antibody and T-cell responses 6 months after coronavirus disease 2019 messenger RNA-1273 vaccination in patients with chronic kidney disease, on Dialysis, or living with a kidney transplant. Clin Infect Dis. (2023) 76:e188–99. doi: 10.1093/cid/ciac557
113. Hod, T, Ben-David, A, Mor, E, Olmer, L, Halperin, R, Indenbaum, V, et al. Humoral response to the fourth BNT162b2 vaccination and link between the fourth dose, omicron infection, and disease severity in renal transplant recipients. Transplantation. (2023) 107:192–203. doi: 10.1097/tp.0000000000004383
114. Chang, CC, Vlad, G, Vasilescu, ER, Li, P, Husain, SA, Silvia, EA, et al. Previous SARS-CoV-2 infection or a third dose of vaccine elicited cross-variant neutralising antibodies in vaccinated solid-organ transplant recipients. Clin Transl Immunology. (2022) 11:e1411. doi: 10.1002/cti2.1411
115. Rincon-Arevalo, H, Choi, M, Stefanski, AL, Halleck, F, Weber, U, Szelinski, F, et al. Impaired humoral immunity to SARS-CoV-2 BNT162b2 vaccine in kidney transplant recipients and dialysis patients. Sci Immunol. (2021) 6:eabj1031. doi: 10.1126/sciimmunol.abj1031
116. Benning, L, Morath, C, Kühn, T, Bartenschlager, M, Kim, H, Beimler, J, et al. Humoral response to SARS-CoV-2 mRNA vaccination in previous non-responder kidney transplant recipients after short-term withdrawal of mycophenolic acid. Front Med (Lausanne). (2022) 9:958293. doi: 10.3389/fmed.2022.958293
117. Kühn, T, Speer, C, Morath, C, Bartenschlager, M, Kim, H, Beimler, J, et al. Immune response to COVID-19 mRNA vaccination in previous nonresponder kidney transplant recipients after short-term withdrawal of mycophenolic acid 1 and 3 months after an additional vaccine dose. Transplantation. (2023) 107:1139–50. doi: 10.1097/tp.0000000000004516
118. Frölke, SC, Bouwmans, P, Messchendorp, AL, Geerlings, SE, Hemmelder, MH, Gansevoort, RT, et al. Predictors of nonseroconversion to SARS-CoV-2 vaccination in kidney transplant recipients. Transplant Direct. (2022) 8:e1397. doi: 10.1097/txd.0000000000001397
119. Regele, F, Heinzel, A, Hu, K, Raab, L, Eskandary, F, Faé, I, et al. Stopping of mycophenolic acid in kidney transplant recipients for 2 weeks Peri-vaccination does not increase response to SARS-CoV-2 vaccination-a non-randomized, controlled pilot study. Front Med (Lausanne). (2022) 9:914424. doi: 10.3389/fmed.2022.914424
120. Sievers, TM, Rossi, SJ, Ghobrial, RM, Arriola, E, Nishimura, P, Kawano, M, et al. Mycophenolate mofetil. Pharmacotherapy. (1997) 17:1178–97. doi: 10.1002/j.1875-9114.1997.tb03082.x
121. Kantauskaite, M, Müller, L, Kolb, T, Fischer, S, Hillebrandt, J, Ivens, K, et al. Intensity of mycophenolate mofetil treatment is associated with an impaired immune response to SARS-CoV-2 vaccination in kidney transplant recipients. Am J Transplant. (2022) 22:634–9. doi: 10.1111/ajt.16851
122. de Boer, SE, Berger, SP, van Leer-Buter, CC, Kroesen, BJ, van Baarle, D, and Sanders, JF. Enhanced humoral immune response after COVID-19 vaccination in elderly kidney transplant recipients on Everolimus versus mycophenolate Mofetil-containing immunosuppressive regimens. Transplantation. (2022) 106:1615–21. doi: 10.1097/tp.0000000000004177
123. Stumpf, J, Siepmann, T, Schwöbel, J, Glombig, G, Paliege, A, Steglich, A, et al. MMF/MPA is the Main mediator of a delayed humoral response with reduced antibody decline in kidney transplant recipients after SARS-CoV-2 mRNA vaccination. Front Med (Lausanne). (2022) 9:928542. doi: 10.3389/fmed.2022.928542
124. Farouk, SS, and Rein, JL. The many faces of Calcineurin inhibitor toxicity-what the FK? Adv Chronic Kidney Dis. (2020) 27:56–66. doi: 10.1053/j.ackd.2019.08.006
125. Noble, J, Jouve, T, Janbon, B, Rostaing, L, and Malvezzi, P. Belatacept in kidney transplantation and its limitations. Expert Rev Clin Immunol. (2019) 15:359–67. doi: 10.1080/1744666x.2019.1574570
126. Abravanel, F, Marion, O, del Bello, A, Beunon, T, Romieu-Mourez, R, Couat, C, et al. Humoral and cellular immune responses of solid organ transplant patients on Belatacept to three doses of mRNA-based anti-SARS-CoV-2 vaccine. Vaccines (Basel). (2022) 10:354. doi: 10.3390/vaccines10030354
127. Wiedemann, A, Pellaton, C, Dekeyser, M, Guillaumat, L, Déchenaud, M, Krief, C, et al. Longitudinal evaluation of the impact of immunosuppressive regimen on immune responses to COVID-19 vaccination in kidney transplant recipients. Front Med (Lausanne). (2022) 9:978764. doi: 10.3389/fmed.2022.978764
128. Terrec, F, Jouve, T, Malvezzi, P, Janbon, B, Naciri Bennani, H, Rostaing, L, et al. Belatacept use after kidney transplantation and its effects on risk of infection and COVID-19 vaccine response. J Clin Med. (2021) 10:5159. doi: 10.3390/jcm10215159
129. Chavarot, N, Morel, A, Leruez-Ville, M, Vilain, E, Divard, G, Burger, C, et al. Weak antibody response to three doses of mRNA vaccine in kidney transplant recipients treated with belatacept. Am J Transplant. (2021) 21:4043–51. doi: 10.1111/ajt.16814
130. Morena, L, Al Jurdi, A, Azzi, J, Fishman, J, and Riella, LV. Factors associated with reduced anti-SARS-CoV-2 antibody responses after mRNA vaccination in kidney transplant recipients on Belatacept. Kidney Int Rep. (2022) 7:2513–6. doi: 10.1016/j.ekir.2022.08.001
Glossary
Keywords: Omicron, kidney transplant recipients, drugs, vaccines, treatment
Citation: Zheng Z, Sun H, Hu X, Xuan Z, Fu M, Bai Y, Du Y, Liu B, Sui X, Zheng J and Shao C (2024) Prevention and treatment strategies for kidney transplant recipients in the context of long-term existence of COVID-19. Front. Med. 11:1287836. doi: 10.3389/fmed.2024.1287836
Edited by:
Csaba Kopitkó, Uzsoki Street Hospital, HungaryReviewed by:
Endrit Shahini, National Institute of Gastroenterology S. de Bellis Research Hospital (IRCCS), ItalyNianqiao Gong, Huazhong University of Science and Technology, China
Copyright © 2024 Zheng, Sun, Hu, Xuan, Fu, Bai, Du, Liu, Sui, Zheng and Shao. This is an open-access article distributed under the terms of the Creative Commons Attribution License (CC BY). The use, distribution or reproduction in other forums is permitted, provided the original author(s) and the copyright owner(s) are credited and that the original publication in this journal is cited, in accordance with accepted academic practice. No use, distribution or reproduction is permitted which does not comply with these terms.
*Correspondence: Chen Shao, Y3NoYW9AeGFoLnhtdS5lZHUuY24=; Jianzhong Zheng, anpoemhlbmdkb2NAMTYzLmNvbQ==
†These authors have contributed equally to this work