- 1The Second Hospital of Lanzhou University, Lanzhou, China
- 2The Department of General Surgery, The Second Hospital of Lanzhou University, Lanzhou, China
- 3The Laboratory of Hepatic-Biliary-Pancreatic, The Second Hospital of Lanzhou University, Lanzhou, China
Liver fibrosis is a complex process driven by various factors and is a key feature of chronic liver diseases. Its essence is liver tissue remodeling caused by excessive accumulation of collagen and other extracellular matrix. Activation of hepatic stellate cells (HSCs), which are responsible for collagen production, plays a crucial role in promoting the progression of liver fibrosis. Abnormal expression of signaling pathways, such as the TGF-β/Smads pathway, contributes to HSCs activation. Recent studies have shed light on these pathways, providing valuable insights into the development of liver fibrosis. Here, we will review six signaling pathways such as TGF-β/Smads that have been studied more in recent years.
1 Introduction
Liver fibrosis is a condition caused by various factors, leading to an excessive buildup of proteins such as collagen in the liver tissue. It is commonly associated with chronic liver diseases and can progress insidiously. Serious disorders like cirrhosis, portal hypertension, and liver failure can be brought on by advanced liver fibrosis and frequently require liver transplantation (1). Regrettably, there are still no effective drugs approved in clinical practice to delay the progression of liver fibrosis, and treatment options are limited to addressing specific causes.
More and more studies have shown a strong correlation between the activation of HSCs and the occurrence and progression of liver fibrosis (2). The essence of liver fibrosis is extracellular matrix (ECM) overproduction which is primarily product by fibroblast myofibroblasts. Myofibroblasts are uncommon in healthy tissues, and HSCs are the primary precursors of myofibroblasts. They originate from mesothelial cells during embryonic development and are located in the subendothelial space of Disse. In a healthy liver, HSCs are inactive. However, when stimulated by profibrotic factors, HSCs become activated and transform into myofibroblast-like cells, playing an active role in tissue repair. Activated HSCs exhibit functions that inactive HSCs do not possess, such as contractility, proliferation, and enhanced ECM synthesis (3). Multiple signaling pathways, play a crucial role in HSCs activation (4). Once activated, HSCs undergo proliferation, enhance contractility, and transform into myofibroblast-like cells that secrete a large amount of ECM, driving liver tissue remodeling and advancing liver fibrosis.
In recent years, a large number of studies have focused on the activation of HSCs in liver fibrosis by TGF-β/Smad, MAPK, PI3K/AKT, Wnt, NF-κB, and AMPK signaling pathways. Therefore, this article places emphasis on the connection between above six signaling pathways and HSCs activation, delving into the mechanism of hepatic stellate cell activation and hepatic fibrosis from a molecular standpoint.
2 The independent role of six signaling pathways in HSCs activation and hepatic fibrosis
As mentioned above, six signaling pathways play a very important role in the occurrence and development of liver fibrosis through the activation of HSCs. Next, we will focus on the role of each of the six signaling pathways in liver fibrosis in recent years, especially their respective relationships with HSCs activation. More interestingly, we reviewed how these signals changed during HSCs quiescence, activation, aging or apoptosis.
2.1 TGF-β/Smad signal path
Transforming growth factor (TGF)-β is a pivotal cytokine with diverse biological functions and a critical role in driving fibrosis. Recent research has underscored the potential of TGF-β as a key target for combatting liver fibrosis (5). It exerts potent effects in activating HSCs (6). Studies have demonstrated that oxoglaucine, when administered as a preconditioning agent, mitigates the TGF-β-induced the upregulation of α-smooth muscle actin (α-SMA) and Collagen Type I (Col I) in Hepa1c1c7 cells. This underscores the promising potential of oxoglaucine as a therapeutic intervention in the context of fibrosis (7). In Figure 1, TGF-β is activated to form a dimer, which binds to and phosphorylates the TGF-β type II receptor on the cell surface, and then interacts with and activates the TGF-β type I receptor. The latter recruits and phosphorylates Smad2/3. This complex then further recruits Smad4, forming the SMAD2/3/4 complex. Finally, this complex is transported to the nucleus to regulate transcription (8).
In the TGF-β/Smad signaling pathway, Smad2/3 is responsible for promoting fibrosis, while Smad7 acts to prevent disease progression (9). Upon TGF-β activation of HSCs, the levels of α-SMA and Col I are significantly elevated compared to the control group. Notably, there is a parallel increase in the Smad3 levels and phosphorylated Smad3. However, the expression of phosphorylated Smad3 is reduced following transfection with the plasmid of left-right determination factor, indicative of the profibrotic effect of Smad3 activation. It is evident that inhibiting its activation can effectively delay the progression of liver fibrosis (9). The TGF-β/Smad signaling pathway activation leads to the localization of the Smad2/3 complex in the nucleus. However, recent studies have shown that when HSCs are co-cultured with adiposederived stem cells or hepatocyte growth factor, the complex is instead distributed throughout the entire cell. Additionally, the study revealed a significant reduction in the levels of α-SMA and Col I expression at both the gene and protein levels compared to activated HSCs. These findings suggest a promising potential for down-regulating this signaling pathway as an effective treatment for liver fibrosis (10). In a groundbreaking study, it was discovered that when human HSCs LX-2 cells were treated with TGF-β and incubated with lactic acid bacteria, there was a remarkable restoration of normal levels of α-SMA, Col I and phosphorylation of Smad2/3 compared to when lactic acid bacteria were absent. Additionally, the expression level of Smad7 increased, suggesting that lactic acid bacteria have the potential to effectively inhibit fibrosis and reduce proinflammatory markers by suppressing the activation of HSCs in vitro (11).
Additionally, as a member of the GTPase family, Ras-related protein 31 (Rab31) is the most important factor in the regulation of endocytosis and cell membrane transport. When HSCs are stimulated by TGF-β, Rab31 elimination can inhibit the activation process, leading to p-Smad2’s expression level decreasing. As previously mentioned, the binding of TGF-βRII to TGF-βRI and its subsequent endocytosis are necessary for the TGF-β signaling pathway. When HSCs were stimulated with TGF-β1, in particular, the cell membrane and cytoplasm showed a significant increase in the coexpression of TGF-βRII and EEA-1 proteins. However, Rab31 knockdown reduced the co-expression of both proteins, indicating that Rab31 promotes the endocytosis of the TGF-βRII complex. Rab31’s involvement in activating the TGF-β/Smad signaling pathway can be traced back to the promotion of endocytosis of the TGF-βRII complex, which ultimately leads to the activation of HSCs. The activation of HSCs is regulated by the TGF-β/Smad signaling pathway, as suggested by these findings, which contributes to the progression of liver fibrosis (12).
2.2 MAPK signal path
In the world of mammalian biology, one enzyme stands out for its significance: mitogen-activated protein kinase (MAPK). It is worth noting that all eukaryotic cells express MAPK (13). Initially known for its involvement in cell mitosis, MAPK is a protein that binds to serine-threonine. As research has progressed, it has been discovered that MAPK not only plays a crucial role in the growth and differentiation of cells, but also in pathophysiological processes such as inflammation, regulation of lipid metabolism and liver fibrosis (14, 15). MAPK is a family of proteins and currently includes subtypes like ERK, JNK, and p38 (16).
ERK, a crucial member of the MAPK family, plays a significant role in the development of liver fibrosis. The production of type I collagen is controlled by the ERK pathway through the phosphorylation of its downstream molecules, as demonstrated by numerous studies, thereby exacerbating liver fibrosis. Conversely, inhibiting the phosphorylation of the ERK pathway can decrease the expression of Col I (17–19). Recently, a team utilized U0126 to block the phosphorylation of the ERK pathway, which led to a decrease in the expression of paxillin-induced Col I. This study further illustrates the involvement of the ERK pathway in liver fibrosis and highlights the potential of therapeutic drugs that target this pathway (20). When studying the role of ANGPTL8 (also known as lipasin) in the progression of liver fibrosis, it was found that ANGPTL8 is a secreted protein that is highly expressed in the liver during eating and lowly expressed during fasting. It was observed that ANGPTL8 reached the surface of HSCs and bound to its receptor, further activating HSCs activation mediated by the ERK signaling pathway. This eventually leads to liver fibrosis, as shown in Figure 2 (21).
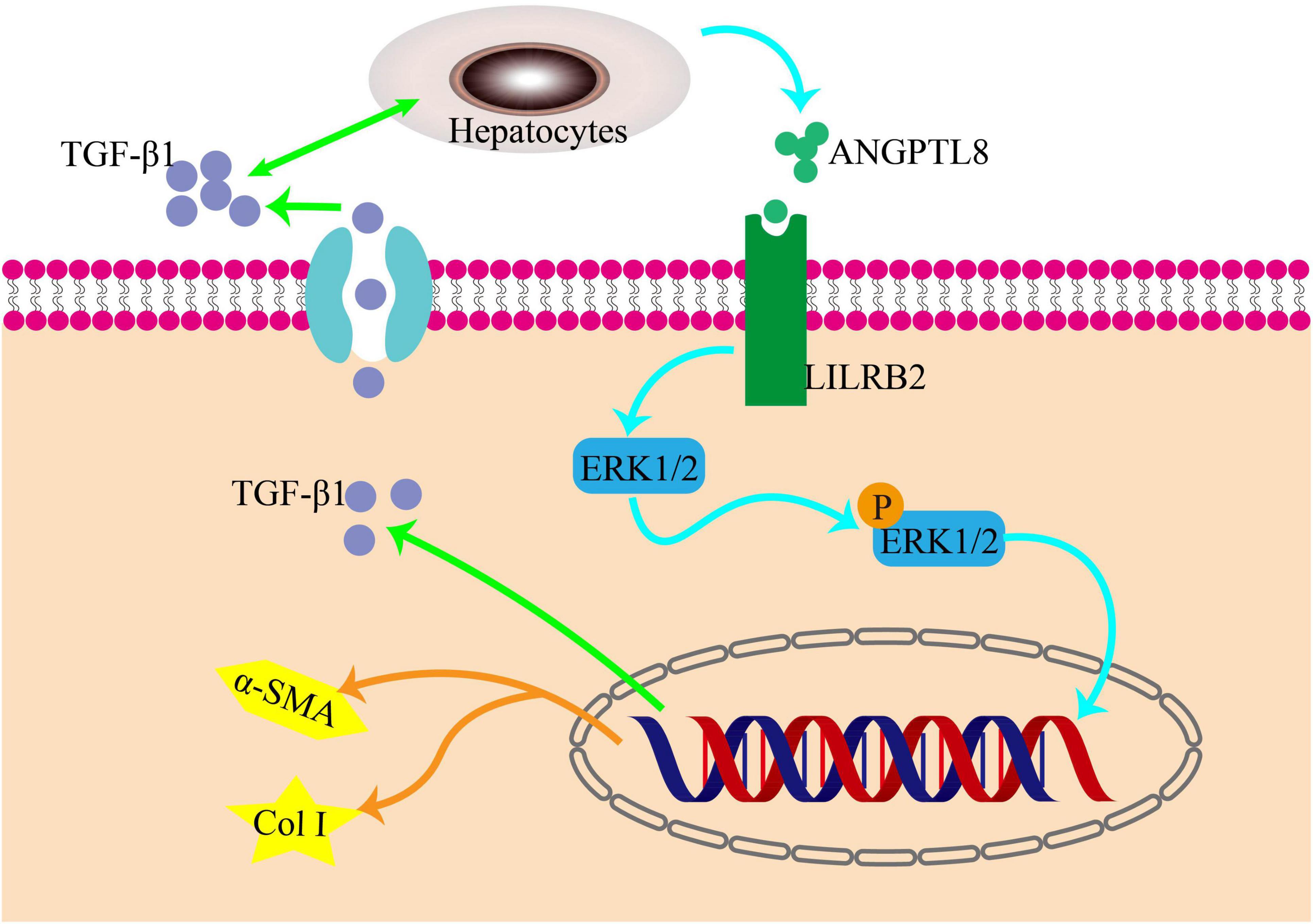
Figure 2. The pro-inflammatory factor ANGPTL8 activates liver stellate cells through the LILRB2/ERK signaling pathway to promote fibrosis. Following a long-term highfat diet, AngPTL8 expression in the liver was increased. The secretion of the inflammatory cytokines ANGPTL8 and TGF-β1 mutually promote each other. ANGPTL8 binds to the LILRB2 receptor on the cell membrane of HSCs, activates HSCs by triggering downstream ERK signals, and subsequently induces the expression of fibrosis factors α-SMA and Col I.
The active compound β-citronellol (β-CIT) identified from Kaffirlime leaves seems to inhibit HSCs activation and reduce ECM deposition by the classical TGFβ1/Smad signaling pathway. Further proteomic analysis and molecular docking revealed a potential additional mode of action involving MAPK tyrosine kinase signaling proteins (22). It was found that exosomes of HepG2.2.15 cells play an important role in the activation, proliferation and fibrosis of LX2 cells. Moreover, KEGG pathway analysis highlights MAPK and other signaling pathways (23). Researchers have discovered that the gut microbiota metabolite 3-indolepropionic acid can activate human HSCs in a lab setting. The expression of fibrosis marker genes and the phosphorylation levels of p38 and JNK are increased by this activation. To confirm this discovery, inhibitors of p38 and JNK were used along with IPA to intervene in HSCs. As a result, the expression of fibrosis genes COL1A2, MMP9, and MMP2 decreased (24). In the study of the mechanism of SalB inhibiting HSCs autophagy and activation, researchers screened 11 protein sites with different expression. Among these, ERK, JNK, and p38 in the MAPK protein family were down-regulated (25). In addition,
SPA3014, a newly-synthesized selective histone deacetylase8, significantly downregulates MAPK-Smad2/3 and JAK2-STAT3 pathways to inhibit histone deacetylase8 and thus reduce the activation of HSCs to play an antifibrotic role (26). These evidences strongly suggest that the development of liver fibrosis is closely tied to the MAPK signaling pathway. Activation of this pathway accelerates HSCs activation, thereby exacerbating the disease. Conversely, inhibiting this pathway can effectively delay disease progression.
2.3 PI3K/AKT signal path
The phosphoinositide 3-kinase (PI3K) is a lipid kinase composed of heterodimers. It phosphorylates the phosphatidyl inositol lipid at the D-3 spot of the inositol ring in response to stimuli from growth factors and hormones. This process regulates cell growth, cycle, migration and survival (27). In mammals, the PI3K enzyme is encoded by different genes with distinct structural characteristics and specific phosphoinositol substrates. This categorization divides PI3K into three categories: I, II and III. Two subclasses, IA and IB, are the main divisions of Class I PI3K, which has been extensively studied. Subclass IA comprises the PI3Kα, PI3kβ, and PI3Kδ subtypes, while subclass IB only includes the PI3Kγ subtype (28). Class I PI3K is a complex composed of a regulatory subunit (p84, p85 or p101) and a catalytic subunit (p110α, β, γ, or δ). p110α and p110β are widely distributed in various tissues, whereas all leukocyte subtypes have high levels of p110γ and p110δ. The new study shows that p110δ levels are low but functionally correlated in white blood cells (29–31).
Cytokines, growth factors, and hormones act as ligands for G protein-coupled receptors (GPCRs) and receptor tyrosine kinases (RTKs) to activate them. Once activated, RTKs then act on the regulatory subunits of the three subtypes of class IA PI3K. In addition, GPCRs activate its downstream Gβγ subunit, which in turn activates PI3Kβ and PI3Kγ(32). The catalytic subunit p110 is activated by the regulatory subunits p85, p84 or p101. This activation results in the phosphorylation of the inositol ring at the 3′ position of phosphorylated phosphatidylinositol (4,5)-bisphosphate (PIP2) and leads to the formation of phosphatidylinositol (3,4,5)-trisphosphate (PIP3). The process can be restored to PIP2 by dephosphorylation of PIP3 under the action of Phosphatase and tensin homolog (PTEN) (33).
Protein kinase B (AKT) is a serine/threonine kinase that connects the activation of growth factor receptors with the regulation of cell growth and metabolism. It plays a vital role in cell signaling (34). PIP3 recruits AKT and phosphoinositide-dependent kinase 1 (PDK1), a fellow Ph-domain kinase, and brings them together on the cell membrane’s surface. PDK1 then further causes AKT phosphorylation, which is decisive for AKT activation (35). After receiving biological information from PI3K, AKT transmits these signals to the downstream target mTOR through various cellular transcription factors. The activation of mTOR can inhibit autophagy by eliminating ubiquitin, which occurs through direct or indirect phosphorylation of AKT (36) (Figure 3).
In recent decades, many studies have found intricate connections between the dysregulation of the PI3K/AKT/mTOR signaling pathway and the occurrence and progression of various human diseases. Additionally, intervening in the dysregulation of this pathway has shown therapeutic potential for a variety of diseases (31). In previous studies on liver fibrosis, researchers found that the activation of the PI3K/AKT/mTOR pathway may be one of the mechanisms contributing to the onset and progression of hepatic fibrosis. The results of some studies using western blot analysis exhibited that the degree of P-PI3K, P-AKT, and P-mTOR proteins in the liver tissue of mice increased significantly after 6 weeks of intraperitoneal injection of CCl4, when contrasted with the control group. This confirmed that the PI3K/AKT/mTOR pathway was activated in the mouse model of liver fibrosis (37). To enhance comprehension of alcoholic liver fibrosis, researchers have investigated the PI3K/AKT/mTOR pathway. In contrast to CCl4-induced liver fibrosis, this study found that the degree of p-mTOR protein in the model band did not change significantly, and in some cases even decreased. However, the expressions of p-PI3K and p-AKT align with previous studies, suggesting that this signaling pathway is indeed activated in alcoholic liver fibrosis (38). After administering microRNA-101, interleukin-22 (IL-22) and exosomes derived from human adipose mesenchymal stem cells (hADMSCs-Exo), the expression levels of moderately phosphorylated molecules in the signaling pathways mentioned above were substantially reduced. This led to improved liver function and reduced liver parenchymal injury, as well as a decrease in the accumulation of ECM. These drugs also were found to inhibit the PI3K/AKT/mTOR pathway. As a result, they alleviated and even reversed liver fibrosis. This indicates that these drugs may have potential as a treatment for liver fibrosis (37–39).
In addition, the PI3K/AKT pathway is not limited to the PI3K/AKT/mTOR signal. Research on phosphoenolpyruvate carboxykinase 1 (PCK1) and metabolic-associated fatty liver disease (MAFLD) reveals that PCK1 deficiency increases GTP in cells, activating RhoA. This, in turn, triggers the PI3K/AKT pathway and raises PDGF-AA secretion, promoting HSC activation and increasing ECM deposition and fibrosis in the MAFLD model. Conversely, AKT/RhoA inhibitors have shown promise in delaying liver fibrosis progression, opening new possibilities for MAFLD treatment (40).
2.4 Wnt signaling pathway
The Wnt/beta (β)-catenin signaling pathway plays a crucial role in directing differentiation, proliferation, maintaining embryonic development and homeostasis. Additionally, it is engaged in the development of diverse human sickness (41). Research has demonstrated that trigger of the Wnt/β-catenin pathway is linked to fibrosis in various organ systems such as the liver, lung, kidney and skin. This association is particularly observed in the corresponding models. Inhibiting this pathway has substantial anti-fibrotic effects (42). Trigger of the Wnt channel can stimulate the propagation and activation of HSCs, leading to liver fibrosis by increasing extracellular matrix synthesis, epithelial-mesenchymal transition or interaction with other fibrotic mediators (43).
The Wnt signaling pathway consists of two different pathways, canonical and non-canonical pathways. The non-canonical pathway is participated in inflammation and lipid accumulation, whereas the canonical pathway is anti-inflammatory and antilipid proliferation and plays an antagonistic role against the non-canonical pathway (44). The classic Wnt/β-catenin pathway relies on β-catenin. β-catenin, a component of the cadherin complex found on the cell membrane, serves as a transmitter of intracellular signals in the Wnt channel. Changes in its function are linked to the onset of several liver diseases, such as liver fibrosis (45). It’s important to note that in normal liver tissue, this protein is found on the liver cell membrane. It attaches E-cadherin to the actin cytoskeleton and plays a role in intercellular adhesion. In damaged liver tissue, βcatenin is found in the cytoplasm as a downstream factor of the Wnt signaling pathway. Upon Wnt activation, β-catenin accumulates in the cytoplasm before translocating to the nucleus. Within the nucleus, it governs the transcription of specific genes through its interaction with the T-cell factor/lymphoid enhancer factor (TCF/LEF) family of transcription factors (46). During this path, β-catenin enlists the assistance of the cAMP response element-binding protein (CREB)-binding protein (CBP) or P300, which is akin to CBP, as coactivators in order to stimulate the transcription of various target genes (47).
When the Wnt signaling pathway is turned off, β-catenin is kept at low levels in the cytoplasm and is controlled by a destabilizing complex consisting of axin, adenomatous polyposis coli (APC), glycogen synthase kinase 3β (GSK-3β) and casein kinase 1 alpha (CK1α). CK1 and GSK-3β phosphorylate ß-catenin and mark it for degradation through ubiquitination orchestrated by ß-catenin repeat sequence (ß-TrCP). Eventually, β-catenin levels become insufficient to activate the transcription process, leading to its degradation by the proteasome (48). Trigger of the canonical pathway occurs when the classical Wnt protein binds to the Frizzled transmembrane receptor and the low-density lipoprotein receptor-related protein 5/6 (LRP5/6). Axin relocates to LRP 5/6, leading to the inactivation of GSK-3ß, dissociation of the system, and dephosphorylation of β-catenin. Consequently, when β-catenin is not phosphorylated, it gathers in the cytoplasm and moves to the nucleus to kick-start the transcription of Wnt target genes (49) (Figures 4a, b).
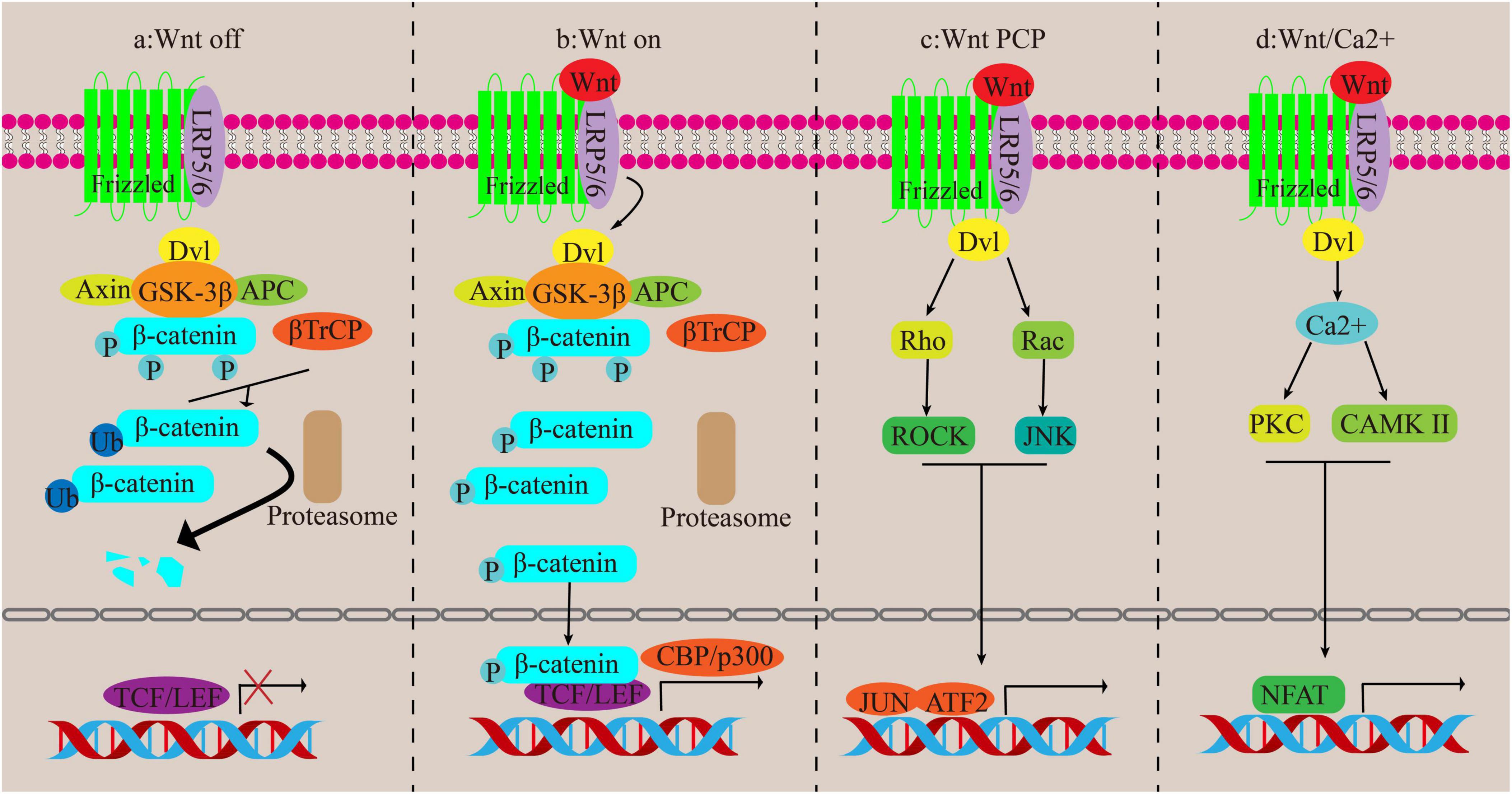
Figure 4. Canonical and non-canonical pathways of Wnt. (a,b) Canonical pathways. (c,d) Non-canonical pathways.
The non-traditional Wnt signaling routes encompass the Wnt/Ca2 + channel and the PCP channel, both devoid of β-catenin participation. In the Wnt/PCP channel, the Wnt protein ties to its recipient and activates the disheveled (Dvl) gene, which then triggers the Rho/ROCK and Rac/JNK pathways, regulating cell functions. In the Wnt/Ca2 + channel, activation of the FZ receptor raises G proteins that actuate Dvl, leading to an increase in cytoplasmic Ca2 + concentration. This then triggers the activation of CAMK II and PKC, followed by the activation of nuclear factors involved in T cell-dependent transcription (43, 50) (Figures 4c, d).
OP-724, previously known as PRI-724, selectively inhibits CBP/beta-catenin. It inhibits the action of β-catenin with CBP through its active metabolite C-82. In mouse models of liver fibrosis, levels of cytokine and chemokine expression, as well as proteins associated with liver fibrosis, are elevated. Treatment with OP-724 can reduce this elevation and activate mitochondrial function, thereby improving liver function and showing anti-fibrotic properties (51, 52). Moreover, clinical studies have revealed that patients with cirrhosis of hepatitis B and C exhibit favorable tolerance to a dose of 280 mg/m2/4 h of PRI-724. After a 12-week treatment period, significant improvements in liver hardness, serum albumin levels and end-stage liver disease model scores were observed, with the patient’s Child-Pugh (CP) grade of liver function improving from class B to CP class A, showcasing the remarkable potential of OP-724 in clinical applications (53).
Indole alkaloids have been found to decrease organ fibrosis. Yohimbine hydrochloride (YHC), an indole alkaloid, has been studied for its impact on TGF-β induced Wnt/β-catenin ligand and receptor expression. When treated with TGF-β in comparison to the control group, the levels of classic Wnt components, Wnt frizzled receptors, and β-catenin were notably increased, while the addition of YHC significantly diminished the levels of these proteins in a dose-dependent manner with YHC (54). Similarly, 20(S)-protopanaxadiol (PPD), a major component of ginseng, weakened the Wnt/β-catenin pathway and depressed collagen deposition by decreasing TGF activity and increasing the levels of P-β-catenin and GSK-3β. Therefore, the degree of liver fibrosis was reduced in the therapy group (55). Furthermore, there is substantial evidence supporting the targeting of the Wnt pathway for liver fibrosis treatment. For example, CD73 regulates HSCs actuation and propagation through the Wnt/β-catenin pathway (56), gandouling alleviates liver fibrosis in Wilson’s disease through the Wnt-/β-catenin pathway (57), phillygenin restrains inflammation and the Wnt/β-catenin pathway improves liver fibrosis in mice treated with CCl4 (58), and so on.
2.5 NF-κB signaling pathway
The nuclear factor-κB (NF-κB) signaling pathway is a highly conserved evolutionary pathway involved in regulating of immunity, inflammatory response and cell function. The NF-κB family contains five subunits that bind to cellular DNA: p50, p52, cRel, p65 (also known as RelA) and RelB, encoded by NF-κB1, NF-κB2, REL, RELA, and RELB, respectively. The heterodimer p50/p65 stands out as the prevalent variant of NF-B and serves as a vital catalyst in the progression of liver cancer (59). Studies of the pathophysiological role of NF-κB in the liver have found that NF-κB is a central link between liver inflammation, fibrosis and hepatocellular carcinoma, suggesting that the NF-κB signaling pathway is a potential aim for preventing or even delay the advance of liver fibrosis (60). However, NF-κB has a dual role, and weakening the NF-κB signaling pathway can have beneficial effects while also having adverse effects on hepatocytes, especially when NF-κB is significantly inhibited (61).
Currently, it is increasingly recognized that inflammation and oxidative stress are intricately intertwined with the onset and advancement of liver fibrosis. When liver cells are exposed to harmful substances, they release reactive oxygen species (ROS) and damage-associated molecular patterns (DAMPs). These DAMPs activate Toll-like receptors (TLRs), tumor necrosis factor receptor, and IL receptor 1. The binding of DAMPs to their ligands triggers the TLR4/myeloid differentiation 88(MYD88) pathway, which then activates NF-κB. This leads to an inflammatory response by promoting the transcription of the NOD-, LRR- and pyrin domain-containing protein 3(NLRP3), similar to the nucleotide-binding oligomerization domain (NOD), procaspase-1, pro-IL-18, and pro-IL-1β. ROS also triggers the assembly of NLRP3 and the adapter apoptosis-related speckle, such as the apoptosis-associated speck-like protein containing a CARD (ASC), and recruits pro-caspase1, leading to the trigger of the inflammasome. Activated inflammasomes promote the growth of IL-1β and IL-18, which in turn activates HSCs, ultimately contributing to the development of liver fibrosis (62–64) (Figure 5).
A recent study demonstrated that ingestion of dibutyl phthalate (a plasticizer, considered a novel chemical pollutant) in mice resulted in NLRP3-mediated pyrodeath of hepatocytes via the NF-κB signaling pathway, activation of LX-2 cells, considerable ECM deposition and elevated hepatic fibrosis index (65). In contrast, fibroblast growth factor 21 (FGF21) inhibits HSC activation by blocking the NF-κB pathway, slowing the course of liver fibrosis (66).
In the past, antiviral drugs were agreed to have a hepatoprotective effect on rat liver fibrosis. Recently, it has been shown that the antiviral drugs Sofosbuvir and Velpatasvir exert their antifibrotic effects through the NF-κB pathway, and their antiviral activity does not appear to be involved in this process. Compared to the model group induced by CTC alone, Sofosbuvir and Velpatasvir alone or in combination could reduce the expression of TNF-α, thus downregulating the NF-κB pathway, and minimize the number of activated HSCs. Reducing or degrading the ECM produced showed an antifibrotic effect (67). In addition, there is increasing evidence for the antifibrotic effect of polysaccharides. For example, Gardenia jasminoides can reduce liver damage and fight fibrosis, but the specific component that plays a role is unclear. Based on its structure and biological activity in plant cells, pectin is an integral part of polysaccharides (68). Therefore, some scholars assumed that the polysaccharides in Gardenia jasminoides had this effect and extracted the homogeneous polysaccharide GJE0.2-2 rich in pectin
from Gardenia Jasminoides. GJE0.2-2 substantially suppressed HSCs actuation in mice with CCl4-induced hepatic fibrosis and reduced collagen deposition. When TGF-β1 is added to LX-2 cells, GJE0.2-2 can directly tie to TLR4 and dephosphorylate the downriver inhibitory protein IκB, resulting in NF-κB binding of NF-B to IκB and retention in the cytoplasm, ultimately leading to the inhibition of NF-κB nuclear translocation in LX-2 cells. Therefore, blocking the activation of HSCs by blocking the NF-κB pathway, indicating the beneficial effect of GJE0.2-2 on the settlement of liver fibrosis. In addition, GJE0.2-2 increased TGF-β induced ROS (69). There are many examples of reducing the degree of liver fibrosis by inhibiting NF-κB signaling pathway, such as salvianolic acid B (70), empagliflozin (71), etc.
2.6 AMPK signaling pathway
Adenosine 5′-monophosphate-activated protein kinase (AMPK) is a heterotrimer made up of two regulatory subunits (β and γ) and a catalytic component (α). As a part of the Serine/threonine kinase family, it is located in varieties of organs (heart, brain, lung, liver, kidney, etc.) (72). AMPK is a key player in the regulation of energy balance. It does this by limiting anabolic pathways which lower ATP consumption, and stimulating catabolic pathways which raise ATP synthesis (73). There is growing evidence that AMPK can prevent fibrosis of the heart (74), liver (75), lungs (76), etc.
In mammals, the two main AMPK-activated cyclic phosphorylated kinases are Ca2 + /calmodulin-dependent protein kinase β (CaMKK2) and tumor suppressor liver kinase B1 (LKB-1) in the Strad-MO25 complex (77). AMPK’s phosphorylation and activation are influenced by LKB1, an AMPK upstream kinase. In the liver, deletion of the LKB1 gene results in phosphorylated AMPK being downregulated and AMPK signaling events not being sensed (78). When intracellular Ca2 + concentration is elevated, CaMKK2 directly phosphorylates AMPK on Thr172 to increase its activity (79).
In a CCL4-induced mouse liver fibrosis model, water extract of earthworms (WEE) increased liver LKB1, AMPK, and GSK3β phosphorylation levels. Similarly, Comparable outcomes were observed in LX-2 cells. After activation of LKB1/AMPK/GSK3β, Nrf2 downstream of LKB1 was induced to enter the liver nucleus and the antioxidant factor level of Nrf2 downstream increased. In AML-12 hepatocytes and LX-2 HSCs, treatment with WEE increased intracellular Nrf2 levels, facilitated its movement into the nucleus and hindered the accumulation of ROS induced by TGF-β1. After knockout of LKB1, the effect of WEE on the AMPK/GSK3β/Nrf2 cascade was also eradicated, and its defensive effect anti-TGF-β1 was eliminated (80). Other researchers evaluated the expression of autophagy-related proteins and AMPK pathway-related proteins in mouse liver tissue, verified that ACE2 overexpression can regulate HSCs autophagy through the AMPK/mTOR pathway, thus alleviating liver fibrosis and liver sinus remodeling by inhibiting HSCs activation and promoting apoptosis (81). In contrast, AMPK pathway agonists can significantly reduce the expression levels of fibrosis marks such as a-SMA, TGF-β and collagen1 in both in vivo and in vitro researches, thus reducing the degree of liver fibrosis (82). In addition, theaflavine was found to up-regulate AMPK activity in HSC LX-2 and mouse livers. Phosphorylation levels of GSK3β, a typical substrate of AMPK, were correspondingly elevated. On the contrary, AMPK inhibitors significantly promoted theaflavine-induced HSCs activation in vitro (82). In clinical studies, AMPK phosphorylation is reduced in the liver of hepatitis C patients, while the level of fibrosis markers is significantly upregulated, and activation of the AMPK pathway can eliminate fibrosis caused by hepatitis C infection (83).
3 The internal crosstalk among the above multiple signal pathways
As mentioned earlier, activation of HSCs is a key step in the development of liver fibrosis. There are multiple signaling pathways involved in the activation of HSCs. However, these signaling pathways are not solely involved in this process.
First, these signaling pathways can be activated simultaneously or sequentially through their respective receptors in response to external stimuli, thus playing a combined role. LPS is a specific ligand for TLR4 on HSCs membranes, it can trigger more than one of signaling pathways to activate HSCs to promote liver fibrosis, including NF-κB and MAPK (84). Instead, an intervention could block multiple signaling pathways simultaneously, thereby preventing HSCs activation and delaying liver fibrosis. Schisantherin A significantly inhibited the proliferation and activation of HSCs by inhibiting the expression of proteins associated with MAPK and NF-κB signaling pathways (85). Taxifolin also inhibits HSCs activation and ECM production by regulating the PI3K/AKT/mTOR and TGF-β1/Smads (86). Helenalin inhibits HSCs activation by inhibiting Mir-200A-mediated PI3K/Akt and NF-κB pathways, and is a potential drug for the treatment of liver fibrosis (87). In liver fibrosis patients with diabetic, blunting p38 MAPKα and ERK1/2 activities by empagliflozin enhances the antifibrotic effect of metformin and augments its AMPK-induced NF-κB inactivation. The metformin/empagliflozin combined therapy could be promising in preventing hepatic inflammation and fibrosis via exhibiting complementary effects (88).
What’s more, there are interactions between these signaling pathways. Activation of one pathway can activate or enhance the action of another pathway. In addition to activating the Smad pathway, TGF-β can also activate non-Smad signaling pathways, such as PI3K/AKT, MAPK, NF-κB, and others. These pathways can regulate the classical Smad pathway and affect TGF-β-mediated biological responses (89–91). Salvia miltiorrhiza and Radix astragali can regulate the expression of CyclinD1, a key factor in Wnt/β-catenin signaling pathway. The expression of CyclinD1 is closely related to the activation of hepatic stellate cells and liver regeneration. Interestingly, the regulatory effect of the herbal extract on β-catenin was much less pronounced than that on the expression of CyclinD1. This suggests that other signaling pathways are involved in regulating the expression of CyclinD1, such as interleukin-8, MAPK, and PI3K (92, 93). In addition, NF-κB and MAPK signal transduction have interactions, and MAPK activation can lead to the phosphorylation of IκB/IκB and the movement of NF-κB p65 to the nucleus (94, 95). Tianhuang formula inhibits the activation of HSCs by significantly inhibiting the activation of p38 MAPK and NF-κB p65, thereby inhibiting liver fibrosis (96).
4 Potential therapeutic effects of various signaling pathways in hepatic fibrosis
So far, the development of anti-fibrosis therapeutics represents an unsolved area with great potential. Of course, previous studies have also explored a large number of treatment methods targeting the above signaling pathways to prevent the activation of HSCs, thereby alleviating liver fibrosis. They include: drugs (Table 1), bioactive molecules in natural products (Table 2), and small molecule compounds (Table 3).
5 Conclusion and outlook
Chronic liver disease of multiple etiologies, such as toxic injury, viral infection, autoimmune diseases, metabolic disorders and genetic ailments, can develop liver fibrosis. Liver fibrosis is regarded an intermediate stage, and effective measures can delay or even reverse its process. Otherwise, it progresses to cirrhosis and the end of liver diseases (96). HSCs activation in liver fibrosis has been shown to is directly connected to the progression of liver fibrosis. Under the action of pro-inflammatory factors, HSCs activation is promoted through various signaling pathways mentioned earlier. The activated HSCs then proliferate, become more contractile and transform into fibroblast-like fibroblasts that secrete a large amount of ECM. It showed fibrosis in vitro. In vivo, it leads to liver tissue remodeling and angiogenesis, and then promotes the progress of liver fibrosis.
The evidence summarized here clearly shows that HSCs activation involves activation of multiple signaling pathways. However, these signaling pathways do not play a role alone, but a variety of signaling pathways often work together to participate in the activation of HSCs. For example, a polysaccharide from the roots of Codonopsis pilosula can promote the activation of HSCs in vivo and in vitro through the combined action of TGF-β1/Smad3 and TLR4/NF-κB signaling pathways, eventually promote liver fibrosis (167). In addition, there are also many non-coding RNAs (including long non-coding RNAs, microRNAs and circular RNAs) through the TGFβ pathway, Wnt/βcatenin pathway,PI3K/AKT pathway and other pathways are engaged in the liver fibrosis (168).
Since the activation of HSCs is crucial in driving the advancement of liver fibrosis, we thought of two ways to delay or even reverse liver fibrosis. On the one hand, inactivation of activated HSCs is one way to induce senescence. However, the persistence of senescent cells can interact with inflammatory cells and reshape the microenvironment to further induce aging-related dysfunction (169). Therefore, this approach needs to be further studied. As mentioned earlier, HSCs activation is inhibited by blocking the signaling pathways mentioned above. In the past, we have conducted extensive studies on the molecular mechanisms of HSCs activation in liver fibrosis. We have also tabulated some interventions that have been found in recent years to prevent liver fibrosis. They include drugs, bioactive molecules in natural products and small molecule compounds that target the above signaling pathways to prevent HSCs activation. However, these methods are still in the basic research stage and have little application in clinical practice. Furthermore, there are still few studies on the potential synergistic mechanisms that may exist in various pathways of HSCs activation. Therefore, there is had no choice but to explore new potential targets by substantial amounts of preclinical and clinical trials, and more attention should be paid to the combined action of multiple signaling pathways that activate HSCs in order to solve the urgent need of liver fibrosis treatment.
Author contributions
YZ: Writing – original draft, Writing – review and editing. LR: Writing – review and editing. YT: Writing – review and editing. XG: Writing – review and editing. FW: Writing – review and editing. YZ: Funding acquisition, Writing – review and editing.
Funding
The author(s) declare financial support was received for the research, authorship, and/or publication of this article. This work was supported by the Cuiying Scientific and Technological Innovation Program of the Second Hospital of Lanzhou University (grant number CY2021-MS-B18 and CY2023-BJ-15).
Conflict of interest
The authors declare that the research was conducted in the absence of any commercial or financial relationships that could be construed as a potential conflict of interest.
Publisher’s note
All claims expressed in this article are solely those of the authors and do not necessarily represent those of their affiliated organizations, or those of the publisher, the editors and the reviewers. Any product that may be evaluated in this article, or claim that may be made by its manufacturer, is not guaranteed or endorsed by the publisher.
References
2. Kamm D, McCommis K. Hepatic stellate cells in physiology and pathology. J Physiol. (2022) 600:1825–37. doi: 10.1113/JP281061
3. Huang Y, Deng X, Liang J. Modulation of hepatic stellate cells and reversibility of hepatic fibrosis. Exp Cell Res. (2017) 352:420–6. doi: 10.1016/j.yexcr.2017.02.038
4. Hwang S, Park S, Yaseen U, Lee H, Cha J. KLF10 Inhibits TGF-β-Mediated Activation of Hepatic Stellate Cells via Suppression of ATF3 Expression. Int J Mol Sci. (2023) 24:12602. doi: 10.3390/ijms241612602
5. Dewidar B, Meyer C, Dooley S, Meindl-Beinker AN. TGF-β in Hepatic Stellate Cell Activation and Liver Fibrogenesis-Updated 2019. Cells. (2019) 8:1419. doi: 10.3390/cells8111419
6. Ding X, Zhu X, Xu D, Li S, Yang Q, Feng X, et al. NPM promotes hepatotoxin-induced fibrosis by inhibiting ROS-induced apoptosis of hepatic stellate cells and upregulating lncMIAT-induced TGF-β2. Cell Death Dis. (2023) 14:575. doi: 10.1038/s41419-023-06043-0
7. Azamov B, Lee K, Hur J, Muradillaeva S, Shim W, Lee C, et al. Oxoglaucine Suppresses Hepatic Fibrosis by Inhibiting TGFβ-Induced Smad2 Phosphorylation and ROS Generation. Molecules. (2023) 28:4971. doi: 10.3390/molecules28134971
8. Hu H, Chen D, Wang Y, Feng Y, Cao G, Vaziri N, et al. New insights into TGF-β/Smad signaling in tissue fibrosis. Chem Biol Interact. (2018) 292:76–83. doi: 10.1016/j.cbi.2018.07.008
9. Chen L, Yang T, Lu D, Zhao H, Feng Y, Chen H, et al. Central role of dysregulation of TGF-β/Smad in CKD progression and potential targets of its treatment. Biomed Pharmacother. (2018) 101:670–81. doi: 10.1016/j.biopha.2018.02.090
10. Yang Y, Bu F, Yang Y, Li H, Huang C, Meng X, et al. LEFTY2 alleviates hepatic stellate cell activation and liver fibrosis by regulating the TGF-β1/Smad3 pathway. Mol Immunol. (2020) 126:31–9. doi: 10.1016/j.molimm.2020.07.012
11. Kanmani P, Kim H. Probiotics counteract the expression of hepatic profibrotic genes via the attenuation of TGF-β/SMAD signaling and autophagy in hepatic stellate cells. PLoS One. (2022) 17:e0262767. doi: 10.1371/journal.pone.0262767
12. Qiu C, Liu Y, Huang S, Ning B, He S, Zhong L. Rab31 promotes activation of hepatic stellate cells by accelerating TGF-β receptor II complex endocytosis. Int J Biochem Cell Biol. (2022) 144:106170. doi: 10.1016/j.biocel.2022.106170
13. Chang L, Karin M. Mammalian MAP kinase signalling cascades. Nature. (2001) 410:37–40. doi: 10.1038/35065000
14. Li X, Shi J, Teng Y, Liu Z. The preventative effect of Baihe Gujin Pill on cisplatin-induced acute kidney injury by activating the PI3K/AKT and suppressing the NF-κB/MAPK pathways. J Ethnopharmacol. (2024) 318:117071. doi: 10.1016/j.jep.2023.117071
15. Chen P, Wang R, Liu F, Li S, Gu Y, Wang L, et al. Schizandrin C regulates lipid metabolism and inflammation in liver fibrosis by NF-κB and p38/ERK MAPK signaling pathways. Front Pharmacol. (2023) 14:1092151. doi: 10.3389/fphar.2023.1092151
16. Chen Z, Ding W, Yang X, Lu T, Liu Y. Isoliquiritigenin, a potential therapeutic agent for treatment of inflammation-associated diseases. J Ethnopharmacol. (2024) 318:117059. doi: 10.1016/j.jep.2023.117059
17. Rockey D, Weymouth N, Shi Z. Smooth muscle α actin (Acta2) and myofibroblast function during hepatic wound healing. PLoS One. (2013) 8:e77166. doi: 10.1371/journal.pone.0077166
18. Rockey D, Du Q, Weymouth N, Shi Z. Smooth Muscle α-Actin Deficiency Leads to Decreased Liver Fibrosis via Impaired Cytoskeletal Signaling in Hepatic Stellate Cells. Am J Pathol. (2019) 189:2209–20. doi: 10.1016/j.ajpath.2019.07.019
19. Shi Z, Ren M, Rockey D. Myocardin and myocardin-related transcription factor-A synergistically mediate actin cytoskeletal-dependent inhibition of liver fibrogenesis. Am J Physiol Gastrointest Liver Physiol. (2020) 318:G504–17. doi: 10.1152/ajpgi.00302.2019
20. Hijazi N, Shi Z, Rockey D. Paxillin regulates liver fibrosis via actin polymerization and ERK activation in hepatic stellate cells. J Cell Sci. (2023) 136:jcs261122. doi: 10.1242/jcs.261122
21. Zhang Z, Yuan Y, Hu L, Tang J, Meng Z, Dai L, et al. ANGPTL8 accelerates liver fibrosis mediated by HFD-induced inflammatory activity via LILRB2/ERK signaling pathways. J Adv Res. (2023) 47:41–56. doi: 10.1016/j.jare.2022.08.006
22. Buakaew W, Krobthong S, Yingchutrakul Y, Potup P, Thongsri Y, Daowtak K, et al. Investigating the Antifibrotic Effects of β-Citronellol on a TGF-β1-Stimulated LX-2 Hepatic Stellate Cell Model. Biomolecules. (2024) 14:800. doi: 10.3390/biom14070800
23. Gao Y, Li L, Zhang S, Mang Y, Zhang X, Feng S. HepG2.2.15-derived exosomes facilitate the activation and fibrosis of hepatic stellate cells. World J Gastroenterol. (2024) 30:2553–63. doi: 10.3748/wjg.v30.i19.2553
24. Yuan X, Yang J, Huang Y, Li J, Li Y. Gut Microbiota Metabolite 3-Indolepropionic Acid Directly Activates Hepatic Stellate Cells by ROS/JNK/p38 Signaling Pathways. Biomolecules. (2023) 13:1464. doi: 10.3390/biom13101464
25. Jiang N, Zhang J, Ping J, Xu L. Salvianolic acid B inhibits autophagy and activation of hepatic stellate cells induced by TGF-β1 by downregulating the MAPK pathway. Front Pharmacol. (2022) 13:938856. doi: 10.3389/fphar.2022.938856
26. Lee C, Choi Y, Cho H, Bang I, Hao L, Lee S, et al. Histone deacetylase 8 inhibition alleviates cholestatic liver injury and fibrosis. Biochem Pharmacol. (2021) 183:114312. doi: 10.1016/j.bcp.2020.114312
27. Cantley L. The phosphoinositide 3-kinase pathway. Science. (2002) 296:1655–7. doi: 10.1126/science.296.5573.1655
28. Curigliano G, Shah R. Safety and Tolerability of Phosphatidylinositol-3-Kinase (PI3K) Inhibitors in Oncology. Drug Saf. (2019) 42:247–62. doi: 10.1007/s40264-018-0778-4
29. Alzahrani A. PI3K/Akt/mTOR inhibitors in cancer: At the bench and bedside. Semin Cancer Biol. (2019) 59:125–32. doi: 10.1016/j.semcancer.2019.07.009
30. Vanhaesebroeck B, Perry M, Brown J, André F, Okkenhaug K. PI3K inhibitors are finally coming of age. Nat Rev Drug Discov. (2021) 20:741–69. doi: 10.1038/s41573-021-00209-1
31. Huang J, Chen L, Wu J, Ai D, Zhang J, Chen T, et al. Targeting the PI3K/AKT/mTOR Signaling Pathway in the Treatment of Human Diseases: Current Status. Trends, and Solutions. J Med Chem. (2022) 65:16033–61. doi: 10.1021/acs.jmedchem.2c01070
32. Houslay D, Anderson K, Chessa T, Kulkarni S, Fritsch R, Downward J, et al. Coincident signals from GPCRs and receptor tyrosine kinases are uniquely transduced by PI3Kβ in myeloid cells. Sci Signal. (2016) 9:ra82. doi: 10.1126/scisignal.aae0453
33. Yang J, Nie J, Ma X, Wei Y, Peng Y, Wei X. Targeting PI3K in cancer: mechanisms and advances in clinical trials. Mol Cancer. (2019) 18:26. doi: 10.1186/s12943-019-0954-x
34. Chu N, Viennet T, Bae H, Salguero A, Boeszoermenyi A, Arthanari H, et al. The structural determinants of PH domain-mediated regulation of Akt revealed by segmental labeling. Elife. (2020) 9:e59151. doi: 10.7554/eLife.59151
35. Szymonowicz K, Oeck S, Malewicz N, Jendrossek V. New Insights into Protein Kinase B/Akt Signaling: Role of Localized Akt Activation and Compartment-Specific Target Proteins for the Cellular Radiation Response. Cancers. (2018) 10:78. doi: 10.3390/cancers10030078
36. Liu K, Yang Y, Zhou F, Xiao Y, Shi L. Inhibition of PI3K/AKT/mTOR signaling pathway promotes autophagy and relieves hyperalgesia in diabetic rats. Neuroreport. (2020) 31:644–9. doi: 10.1097/WNR.0000000000001461
37. Lei Y, Wang Q, Shen L, Tao Y, Liu C. MicroRNA-101 suppresses liver fibrosis by downregulating PI3K/Akt/mTOR signaling pathway. Clin Res Hepatol Gastroenterol. (2019) 43:575–84. doi: 10.1016/j.clinre.2019.02.003
38. Meng Y, Zhao R, Huo L. Interleukin-22 alleviates alcohol-associated hepatic fibrosis, inhibits autophagy, and suppresses the PI3K/AKT/mTOR pathway in mice. Alcohol Clin Exp Res. (2023) 47:448–58. doi: 10.1111/acer.15021
39. Zhang Z, Shang J, Yang Q, Dai Z, Liang Y, Lai C, et al. Exosomes derived from human adipose mesenchymal stem cells ameliorate hepatic fibrosis by inhibiting PI3K/Akt/mTOR pathway and remodeling choline metabolism. J Nanobiotechnology. (2023) 21:29. doi: 10.1186/s12951-023-01788-4
40. Ye Q, Liu Y, Zhang G, Deng H, Wang X, Tuo L, et al. Deficiency of gluconeogenic enzyme PCK1 promotes metabolic-associated fatty liver disease through PI3K/AKT/PDGF axis activation in male mice. Nat Commun. (2023) 14:1402. doi: 10.1038/s41467-023-37142-3
41. Li X, Jiang F, Hu Y, Lang Z, Zhan Y, Zhang R, et al. Schisandrin B Promotes Hepatic Stellate Cell Ferroptosis via Wnt Pathway-Mediated Ly6Clo Macrophages. J Agric Food Chem. (2023) doi: 10.1021/acs.jafc.3c03409 [Epub ahead of print].
42. Nishikawa K, Osawa Y, Kimura K. Wnt/β-Catenin Signaling as a Potential Target for the Treatment of Liver Cirrhosis Using Antifibrotic Drugs. Int J Mol Sci. (2018) 19:3103. doi: 10.3390/ijms19103103
43. Hu H, Cao G, Wu X, Vaziri N, Zhao Y. Wnt signaling pathway in aging-related tissue fibrosis and therapies. Ageing Res Rev. (2020) 60:101063. doi: 10.1016/j.arr.2020.101063
44. Ackers I, Malgor R. Interrelationship of canonical and non-canonical Wnt signalling pathways in chronic metabolic diseases. Diab Vasc Dis Res. (2018) 15:3–13. doi: 10.1177/1479164117738442
45. Monga S. β-Catenin Signaling and Roles in Liver Homeostasis, Injury, and Tumorigenesis. Gastroenterology. (2015) 148:1294–310. doi: 10.1053/j.gastro.2015.02.056
46. Zhang F, Wang F, He J, Lian N, Wang Z, Shao J, et al. Regulation of hepatic stellate cell contraction and cirrhotic portal hypertension by Wnt/β-catenin signalling via interaction with Gli1. Br J Pharmacol. (2021) 178:2246–65. doi: 10.1111/bph.15289
47. Kahn M. Can we safely target the WNT pathway? Nat Rev Drug Discov. (2014) 13:513–32. doi: 10.1038/nrd4233
48. Duspara K, Bojanic K, Pejic J, Kuna L, Kolaric T, Nincevic V, et al. Targeting the Wnt Signaling Pathway in Liver Fibrosis for Drug Options: An Update. J Clin Transl Hepatol. (2021) 9:960–71. doi: 10.14218/JCTH.2021.00065
49. Shree Harini K, Ezhilarasan D. Wnt/beta-catenin signaling and its modulators in nonalcoholic fatty liver diseases. Hepatobiliary Pancreat Dis Int. (2023) 22:333–45. doi: 10.1016/j.hbpd.2022.10.003
50. Wang J, Li L, Li L, Yan Q, Li J, Xu T. Emerging role and therapeutic implication of Wnt signaling pathways in liver fibrosis. Gene. (2018) 674:57–69. doi: 10.1016/j.gene.2018.06.053
51. Ouchi H, Mizutani Y, Yoshimura K, Sato Y, Kimura K, Haruyama Y, et al. Anti-inflammatory and antifibrotic effects of CBP/β-catenin inhibitor for hepatocytes: small molecular inhibitor. OP-724 possibly improves liver function. Med Mol Morphol. (2023) 56:94–105. doi: 10.1007/s00795-022-00343-8
52. Kimura M, Nishikawa K, Osawa Y, Imamura J, Yamaji K, Harada K, et al. Inhibition of CBP/β-catenin signaling ameliorated fibrosis in cholestatic liver disease. Hepatol Commun. (2022) 6:2732–47. doi: 10.1002/hep4.2043
53. Kimura K, Kanto T, Shimoda S, Harada K, Kimura M, Nishikawa K, et al. Safety, tolerability, and anti-fibrotic efficacy of the CBP/β-catenin inhibitor PRI-724 in patients with hepatitis C and B virus-induced liver cirrhosis: An investigator-initiated, open-label, non-randomised, multicentre, phase 1/2a study. EBioMedicine. (2022) 80:104069. doi: 10.1016/j.ebiom.2022.104069
54. Sharma N, Sistla R, Andugulapati S. Yohimbine ameliorates liver inflammation and fibrosis by regulating oxidative stress and Wnt/β-catenin pathway. Phytomedicine. (2024) 123:155182. doi: 10.1016/j.phymed.2023.155182
55. Li C, Zhan Y, Zhang R, Tao Q, Lang Z, Zheng J. 20(S)- Protopanaxadiol suppresses hepatic stellate cell activation via WIF1 demethylation-mediated inactivation of the Wnt/β-catenin pathway. J Ginseng Res. (2023) 47:515–23. doi: 10.1016/j.jgr.2022.05.005
56. Jia W, Zhou T, Dai J, Liu Z, Zhang Y, Zang D, et al. CD73 regulates hepatic stellate cells activation and proliferation through Wnt/β-catenin signaling pathway. Eur J Pharmacol. (2021) 890:173667. doi: 10.1016/j.ejphar.2020.173667
57. Cheng C, Wang Q, Huang Y, Xue Q, Wang Y, Wu P, et al. Gandouling inhibits hepatic fibrosis in Wilson’s disease through Wnt-1/β-catenin signaling pathway. J Ethnopharmacol. (2023) 311:116445. doi: 10.1016/j.jep.2023.116445
58. Wang C, Liu Y, Gong L, Xue X, Fu K, Ma C, et al. Phillygenin Ameliorates Carbon Tetrachloride-Induced Liver Fibrosis: Suppression of Inflammation and Wnt/β-Catenin Signaling Pathway. Inflammation. (2023) 46:1543–60. doi: 10.1007/s10753-023-01826-1
59. Li Y, Lu L, Tu J, Zhang J, Xiong T, Fan W, et al. Reciprocal Regulation Between Forkhead Box M1/NF-κB and Methionine Adenosyltransferase 1A Drives Liver Cancer. Hepatology. (2020) 72:1682–700. doi: 10.1002/hep.31196
60. Elsharkawy A, Mann D. Nuclear factor-kappaB and the hepatic inflammation-fibrosis-cancer axis. Hepatology. (2007) 46:590–7. doi: 10.1002/hep.21802
61. Luedde T, Schwabe RF. NF-κB in the liver–linking injury, fibrosis and hepatocellular carcinoma. Nat Rev Gastroenterol Hepatol. (2011) 8:108–18. doi: 10.1038/nrgastro.2010.213
62. Gao J, Liu Q, Li J, Hu C, Zhao W, Ma W, et al. Fibroblast Growth Factor 21 dependent TLR4/MYD88/NF-κB signaling activation is involved in lipopolysaccharide-induced acute lung injury. Int Immunopharmacol. (2020) 80:106219. doi: 10.1016/j.intimp.2020.106219
63. Ramos-Tovar E, Muriel P. Molecular Mechanisms That Link Oxidative Stress, Inflammation, and Fibrosis in the Liver. Antioxidants (Basel). (2020) 9:1279. doi: 10.3390/antiox9121279
64. Knorr J, Wree A, Tacke F, Feldstein A. The NLRP3 Inflammasome in Alcoholic and Nonalcoholic Steatohepatitis. Semin Liver Dis. (2020) 40:298–306. doi: 10.1055/s-0040-1708540
65. Huo S, Li B, Du J, Zhang X, Zhang J, Wang Q, et al. Dibutyl phthalate induces liver fibrosis via p38MAPK/NF-κB/NLRP3-mediated pyroptosis. Sci Total Environ. (2023) 897:165500. doi: 10.1016/j.scitotenv.2023.165500
66. Yang B, Lu L, Zhou D, Fan W, Barbier-Torres L, Steggerda J, et al. Regulatory network and interplay of hepatokines, stellakines, myokines and adipokines in nonalcoholic fatty liver diseases and nonalcoholic steatohepatitis. Front Endocrinol (Lausanne). (2022) 13:1007944. doi: 10.3389/fendo.2022.1007944
67. Yasmeen S, Khan A, Anwar F, Akhtar M, Yasmeen S, Shah S. An insight into the hepatoprotective role of Velpatasvir and Sofosbuvir per se and in combination against carbon tetrachloride-induced hepatic fibrosis in rats. Environ Sci Pollut Res Int. (2023) 30:95660–72. doi: 10.1007/s11356-023-29134-z
68. Bian Y, Zeng H, Tao H, Huang L, Du Z, Wang J, et al. A pectin-like polysaccharide from Polygala tenuifolia inhibits pancreatic cancer cell growth in vitro and in vivo by inducing apoptosis and suppressing autophagy. Int J Biol Macromol. (2020) 162:107–15. doi: 10.1016/j.ijbiomac.2020.06.054
69. Ma X, Zhou W, Nie Y, Jing X, Li S, Jin C, et al. A novel branched galacturonan from Gardenia jasminoides alleviates liver fibrosis linked to TLR4/NF-κB signaling. Int J Biol Macromol. (2023) 245:125540. doi: 10.1016/j.ijbiomac.2023.125540
70. Wang R, Li S, Chen P, Yue X, Wang S, Gu Y, et al. Salvianolic acid B suppresses hepatic stellate cell activation and liver fibrosis by inhibiting the NF-κB signaling pathway via miR-6499-3p/LncRNA-ROR. Phytomedicine. (2022) 107:154435. doi: 10.1016/j.phymed.2022.154435
71. Elseweidy M, Ali A, Hassanin S, Mahmoud Y. Empagliflozin ameliorates liver fibrosis in NASH rat model via targeting hepatic NF-κB/SOX9/OPN signaling and osteocalcin level. Naunyn Schmiedebergs Arch Pharmacol. (2024) 397:3449–59. doi: 10.1007/s00210-023-02826-6
72. Jiang S, Li T, Yang Z, Yi W, Di S, Sun Y, et al. AMPK orchestrates an elaborate cascade protecting tissue from fibrosis and aging. Ageing Res Rev. (2017) 38:18–27. doi: 10.1016/j.arr.2017.07.001
73. Ceni E, Mello T, Galli A. Pathogenesis of alcoholic liver disease: role of oxidative metabolism. World J Gastroenterol. (2014) 20:17756–72. doi: 10.3748/wjg.v20.i47.17756
74. Wang H, Huang M, Bei W, Yang Y, Song L, Zhang D, et al. FTZ attenuates liver steatosis and fibrosis in the minipigs with type 2 diabetes by regulating the AMPK signaling pathway. Biomed Pharmacother. (2021) 138:111532. doi: 10.1016/j.biopha.2021.111532
75. Gu X, Han Y, Yang C, Ji H, Lan Y, Bi Y, et al. Activated AMPK by metformin protects against fibroblast proliferation during pulmonary fibrosis by suppressing FOXM1. Pharmacol Res. (2021) 173:105844. doi: 10.1016/j.phrs.2021.105844
76. Yan Y, Zhou X, Xu H, Melcher K. Structure and Physiological Regulation of AMPK. Int J Mol Sci. (2018) 19:3534. doi: 10.3390/ijms19113534
77. Zhang J, Ma X, Fan D. Ginsenoside CK ameliorates hepatic lipid accumulation via activating the LKB1/AMPK pathway in vitro and in vivo. Food Funct. (2022) 13:1153–67. doi: 10.1039/d1fo03026d
78. Zhao P, Saltiel A. From overnutrition to liver injury: AMP-activated protein kinase in nonalcoholic fatty liver diseases. J Biol Chem. (2020) 295:12279–89. doi: 10.1074/jbc.REV120.011356
79. Zhang T, Wang C, Song A, Lei X, Li G, Sun H, et al. Water extract of earthworms mitigates mouse liver fibrosis by potentiating hepatic LKB1/Nrf2 axis to inhibit HSC activation and hepatocyte death. J Ethnopharmacol. (2024) 321:117495. doi: 10.1016/j.jep.2023.117495
80. Wu Y, Yin A, Sun J, Xu W, Zhang C. Angiotensin-converting enzyme 2 improves liver fibrosis in mice by regulating autophagy of hepatic stellate cells. World J Gastroenterol. (2023) 29:4975–90. doi: 10.3748/wjg.v29.i33.4975
81. Hu L, Su L, Dong Z, Wu Y, Lv Y, George J, et al. AMPK agonist AICAR ameliorates portal hypertension and liver cirrhosis via NO pathway in the BDL rat model. J Mol Med (Berl). (2019) 97:423–34. doi: 10.1007/s00109-019-01746-4
82. Shu G, Sun H, Zhang T, Zhu A, Lei X, Wang C, et al. Theaflavine inhibits hepatic stellate cell activation by modulating the PKA/LKB1/AMPK/GSK3β cascade and subsequently enhancing Nrf2 signaling. Eur J Pharmacol. (2023) 956:175964. doi: 10.1016/j.ejphar.2023.175964
83. Jung G, Jeon J, Choi Y, Jang S, Park S, Kim M, et al. Small heterodimer partner attenuates profibrogenic features of hepatitis C virus-infected cells. Liver Int. (2015) 35:2233–45. doi: 10.1111/liv.12871
84. Zhou J, Zhang X, Wan L, Yu J, Li T, Lu Z, et al. Zi Qi Decoction Alleviates Liver Fibrosis by Inhibiting the Toll-Like Receptor 4 (TLR4)-Related Nuclear Factor kappa b (NF-κB) and Mitogen-Activated Protein Kinase (MAPK) Signaling Pathways. Med Sci Monit. (2021) 27:e929438. doi: 10.12659/MSM.929438
85. Wang H, Che J, Cui K, Zhuang W, Li H, Sun J, et al. Schisantherin A ameliorates liver fibrosis through TGF-β1mediated activation of TAK1/MAPK and NF-κB pathways in vitro and in vivo. Phytomedicine. (2021) 88:153609. doi: 10.1016/j.phymed.2021.153609
86. Liu X, Liu W, Ding C, Zhao Y, Chen X, Ling D, et al. Taxifolin, Extracted from Waste Larix olgensis Roots, Attenuates CCl4-Induced Liver Fibrosis by Regulating the PI3K/AKT/mTOR and TGF-β1/Smads Signaling Pathways. Drug Des Devel Ther. (2021) 15:871–87. doi: 10.2147/DDDT.S281369
87. Fang B, Wen S, Li Y, Bai F, Wei Y, Xiong Y, et al. Prediction and verification of target of helenalin against hepatic stellate cell activation based on miR-200a-mediated PI3K/Akt and NF-κB pathways. Int Immunopharmacol. (2021) 92:107208. doi: 10.1016/j.intimp.2020.107208
88. Abdelhamid A, Youssef M, Abd El-Fattah E, Gobba N, Gaafar A, Girgis S, et al. Blunting p38 MAPKα and ERK1/2 activities by empagliflozin enhances the antifibrotic effect of metformin and augments its AMPK-induced NF-κB inactivation in mice intoxicated with carbon tetrachloride. Life Sci. (2021) 286:120070. doi: 10.1016/j.lfs.2021.120070
89. Lu Z, Shan Q, Hu S, Zhao Y, Zhang G, Zhu M, et al. Discovery of 1,8-naphthalidine derivatives as potent anti-hepatic fibrosis agents via repressing PI3K/AKT/Smad and JAK2/STAT3 pathways. Bioorg Med Chem. (2021) 49:116438. doi: 10.1016/j.bmc.2021.116438
90. Liu X, Wang X, Xu L, Fan J, Yuan Q, Zhang F, et al. Targeting delivery of a novel TGF-β type I receptor-mimicking peptide to activated hepatic stellate cells for liver fibrosis therapy via inhibiting the TGF-β1/Smad and p38 MAPK signaling pathways. Eur J Pharmacol. (2024) 977:176708. doi: 10.1016/j.ejphar.2024.176708
91. Ciceu A, Balta C, Herman H, Gharbia S, Ignat S, Dinescu S, et al. Complexation with Random Methyl-β-Cyclodextrin and (2-Hidroxypropyl)-β-Cyclodextrin Enhances In Vivo Anti-Fibrotic and Anti-Inflammatory Effects of Chrysin via the Inhibition of NF-κB and TGF-β1/Smad Signaling Pathways and Modulation of Hepatic Pro/Anti-Fibrotic miRNA. Int J Mol Sci. (2021) 22:1869. doi: 10.3390/ijms22041869
92. Cao T, Lu Y, Zhu M, Cheng J, Ye B, Fang N, et al. Effects of Salvia miltiorrhiza and Radix astragali on the TGF-β/Smad/Wnt pathway and the pathological process of liver fibrosis in rats. Cell Mol Biol. (2020) 66:46–51.
93. Xiong Y, Lu H, Xu H. Galangin Reverses Hepatic Fibrosis by Inducing HSCs Apoptosis via the PI3K/Akt, Bax/Bcl-2, and Wnt/β-Catenin Pathway in LX-2 Cells. Biol Pharm Bull. (2020) 43:1634–42. doi: 10.1248/bpb.b20-00258
94. Khalil R, Shata A, Abd El-Kader E, Sharaf H, Abdo W, Amin N, et al. Vildagliptin, a DPP-4 inhibitor, attenuates carbon tetrachloride-induced liver fibrosis by targeting ERK1/2, p38α, and NF-κB signaling. Toxicol Appl Pharmacol. (2020) 407:115246. doi: 10.1016/j.taap.2020.115246
95. Tang Q, Wang Q, Sun Z, Kang S, Fan Y, Hao Z. Bergenin Monohydrate Attenuates Inflammatory Response via MAPK and NF-κB Pathways Against Klebsiella pneumonia Infection. Front Pharmacol. (2021) 12:651664. doi: 10.3389/fphar.2021.651664
96. Lan T, Chen B, Hu X, Cao J, Chen S, Ding X, et al. Tianhuang formula ameliorates liver fibrosis by inhibiting CCL2-CCR2 axis and MAPK/NF-κB signaling pathway. J Ethnopharmacol. (2024) 321:117516. doi: 10.1016/j.jep.2023.117516
97. Liu J, Kong D, Qiu J, Xie Y, Lu Z, Zhou C, et al. Praziquantel ameliorates CCl4 -induced liver fibrosis in mice by inhibiting TGF-β/Smad signalling via up-regulating Smad7 in hepatic stellate cells. Br J Pharmacol. (2019) 176:4666–80. doi: 10.1111/bph.14831
98. Dinarvand N, Afarin R, Shakerian E, Bavarsad S, Mohammadtaghvaei N. The effect of saraglitazar on TGF-β-induced smad3 phosphorylation and expression of genes related to liver fibrosis in LX2 cell line. Mol Biol Rep. (2024) 51:541. doi: 10.1007/s11033-024-09443-3
99. El-Wakeel S, Rahmo R, El-Abhar H. Anti-fibrotic impact of Carvedilol in a CCl-4 model of liver fibrosis via serum microRNA-200a/SMAD7 enhancement to bridle TGF-β1/EMT track. Sci Rep. (2018) 8:14327. doi: 10.1038/s41598-018-32309-1
100. Lee S, Kim S, Hur W, Kang B, Lee H, Nam H, et al. Tenofovir disoproxil fumarate directly ameliorates liver fibrosis by inducing hepatic stellate cell apoptosis via downregulation of PI3K/Akt/mTOR signaling pathway. PLoS One. (2021) 16:e0261067. doi: 10.1371/journal.pone.0261067
101. Li X, Li H, Zhang S, Zhang R, Li J, Wei Y, et al. Protective effect of Idelalisib on carbon tetrachloride-induced liver fibrosis via microRNA-124-3P/phosphatidylinositol-3-hydroxykinase signalling pathway. J Cell Mol Med. (2021) 25:11185–97. doi: 10.1111/jcmm.17039
102. Xiu A, Ding Q, Li Z, Zhang C. Doxazosin Attenuates Liver Fibrosis by Inhibiting Autophagy in Hepatic Stellate Cells via Activation of the PI3K/Akt/mTOR Signaling Pathway. Drug Des Devel Ther. (2021) 15:3643–59. doi: 10.2147/DDDT.S317701
103. Li H, You P, Xia Y, Cai Y, Tu Y, Wang M, et al. Yu Gan Long Ameliorates Hepatic Fibrosis by Inhibiting PI3K/AKT, Ras/ERK and JAK1/STAT3 Signaling Pathways in CCl4-induced Liver Fibrosis Rats. Curr Med Sci. (2020) 40:539–47. doi: 10.1007/s11596-020-2211-3
104. Zhang C, An R, Bao Y, Meng X, Wang T, Sun H, et al. Inhibitory effects of octreotide on the progression of hepatic fibrosis via the regulation of Bcl-2/Bax and PI3K/AKT signaling pathways. Int Immunopharmacol. (2019) 73:515–26. doi: 10.1016/j.intimp.2019.05.055
105. Zhou M, Zhao X, Liao L, Deng Y, Liu M, Wang J, et al. Forsythiaside A Regulates Activation of Hepatic Stellate Cells by Inhibiting NOX4-Dependent ROS. Oxid Med Cell Longev. (2022) 2022:9938392. doi: 10.1155/2022/9938392
106. Rampa D, Feng H, Allur-Subramaniyan S, Shim K, Pekcec A, Lee D, et al. Kinin B1 receptor blockade attenuates hepatic fibrosis and portal hypertension in chronic liver diseases in mice. J Transl Med. (2022) 20:590. doi: 10.1186/s12967-022-03808-7
107. Zaafan M, Abdelhamid A. Dasatinib ameliorates thioacetamide-induced liver fibrosis: modulation of miR-378 and miR-17 and their linked Wnt/β-catenin and TGF-β/smads pathways. J Enzyme Inhib Med Chem. (2022) 37:118–24. doi: 10.1080/14756366.2021.1995379
108. Mandala A, Chen W, Armstrong A, Malhotra M, Chavalmane S, McCommis K, et al. PPAR α agonist fenofibrate attenuates iron-induced liver injury in mice by modulating the Sirt3 and β-catenin signaling. Am J Physiol Gastrointest Liver Physiol. (2021) 321:G262–9. doi: 10.1152/ajpgi.00129.2021
109. Esmail M, Saeed N, Michel H, El-Naga R. The ameliorative effect of niclosamide on bile duct ligation induced liver fibrosis via suppression of NOTCH and Wnt pathways. Toxicol Lett. (2021) 347:23–35. doi: 10.1016/j.toxlet.2021.04.018
110. Huang G, Wei S, Huang Y, Xing W, Wang L, Liang L. Mechanism of combined use of vitamin D and puerarin in anti-hepatic fibrosis by regulating the Wnt/β-catenin signalling pathway. World J Gastroenterol. (2018) 24:4178–85. doi: 10.3748/wjg.v24.i36.4178
111. Zhang C, Liu X, Sun H, Meng X, Bao Y, Zhang H, et al. Octreotide attenuates hepatic fibrosis and hepatic stellate cells proliferation and activation by inhibiting Wnt/β-catenin signaling pathway, c-Myc and cyclin D1. Int Immunopharmacol. (2018) 63:183–90. doi: 10.1016/j.intimp.2018.08.005
112. Akcora B, Storm G, Bansal R. Inhibition of canonical WNT signaling pathway by β-catenin/CBP inhibitor ICG-001 ameliorates liver fibrosis in vivo through suppression of stromal CXCL12. Biochim Biophys Acta Mol Basis Dis. (2018) 1864:804–18. doi: 10.1016/j.bbadis.2017.12.001
113. Hasan H, Abdel-Rafei M, Galal S. Diosmin attenuates radiation-induced hepatic fibrosis by boosting PPAR-γ expression and hampering miR-17-5p-activated canonical Wnt-β-catenin signaling. Biochem Cell Biol. (2017) 95:400–14. doi: 10.1139/bcb-2016-0142
114. Koizumi A, Kaji K, Nishimura N, Asada S, Matsuda T, Tanaka M, et al. Effects of elafibranor on liver fibrosis and gut barrier function in a mouse model of alcohol-associated liver disease. World J Gastroenterol. (2024) 30:3428–46. doi: 10.3748/wjg.v30.i28.3428
115. Lee S, Kim S, Choi I, Song Y, Kim N, Ryu H, et al. Inhibition of 11β-hydroxysteroid dehydrogenase 1 relieves fibrosis through depolarizing of hepatic stellate cell in NASH. Cell Death Dis. (2022) 13:1011. doi: 10.1038/s41419-022-05452-x
116. Zhao R, Zhao H, Guo Q, Mu Y, Zhang J, Su Y, et al. Edaravone protects against liver fibrosis progression via decreasing the IL-1β secretion of macrophages. Chem Biol Interact. (2022) 368:110251. doi: 10.1016/j.cbi.2022.110251
117. Xie Y, Du D, Zhang L, Yang Y, Zou Z, Li Z, et al. TJ-M2010-5, A self-developed MyD88 inhibitor, attenuates liver fibrosis by inhibiting the NF-κB pathway. Chem Biol Interact. (2022) 354:109839. doi: 10.1016/j.cbi.2022.109839
118. Sharawy M, El-Kashef D, Shaaban A, El-Agamy D. Anti-fibrotic activity of sitagliptin against concanavalin A-induced hepatic fibrosis. Role of Nrf2 activation/NF-κB inhibition. Int Immunopharmacol. (2021) 100:108088. doi: 10.1016/j.intimp.2021.108088
119. Hassan H, Nageeb M, Mohammed H, Samy W, Fawzy A, Afifi R, et al. Dapagliflozin dampens liver fibrosis induced by common bile duct ligation in rats associated with the augmentation of the hepatic Sirt1/AMPK/PGC1α/FoxO1 axis. Toxicol Appl Pharmacol. (2024) 489:116991. doi: 10.1016/j.taap.2024.116991
120. Li Z, Ding Q, Ling L-P, Wu Y, Meng D-X, Li X, et al. Metformin attenuates motility, contraction, and fibrogenic response of hepatic stellate cells in vivo and in vitro by activating AMP-activated protein kinase. World Journal of Gastroenterology. (2018) 247:819–32.
121. Lin L, Li R, Cai M, Huang J, Huang W, Guo Y, et al. Andrographolide Ameliorates Liver Fibrosis in Mice: Involvement of TLR4/NF- κ B and TGF- β 1/Smad2 Signaling Pathways. Oxid Med Cell Longev. (2018) 2018:7808656. doi: 10.1155/2018/7808656
122. Wang F, Gan J, Li R, Yang R, Mao X, Liu S, et al. Naringin from Ganshuang granule inhibits inflammatory to relieve liver fibrosis through TGF-β-Smad signaling pathway. PLoS One. (2024) 19:e0304185. doi: 10.1371/journal.pone.0304185
123. Li S, Gan L, Tian Y, Tian Y, Fan R, Huang D, et al. Presegetane diterpenoids from Euphorbia sieboldiana as a new type of anti-liver fibrosis agents that inhibit TGF-β/Smad signaling pathway. Bioorg Chem. (2021) 114:105222. doi: 10.1016/j.bioorg.2021.105222
124. Kang R, Tian W, Cao W, Sun Y, Zhang H, Feng Y, et al. Ligustroflavone ameliorates CCl4-induced liver fibrosis through down-regulating the TGF-β/Smad signaling pathway. Chin J Nat Med. (2021) 19:170–80. doi: 10.1016/S1875-5364(21)60018-3
125. Ma R, Xie N, Shu Y, Wu Y, He P, Xiang Y, et al. Cannabidiol alleviates carbon tetrachloride-induced liver fibrosis in mice by regulating NF-κB and PPAR-α pathways. Exp Biol Med (Maywood). (2024) 249:10141. doi: 10.3389/ebm.2024.10141
126. Cai S, Wu L, Yuan S, Liu G, Wang Y, Fang L, et al. Carvacrol alleviates liver fibrosis by inhibiting TRPM7 and modulating the MAPK signaling pathway. Eur J Pharmacol. (2021) 898:173982. doi: 10.1016/j.ejphar.2021.173982
127. Elfeky M, Mantawy E, Gad A, Fawzy H, El-Demerdash E. Mechanistic aspects of antifibrotic effects of honokiol in Con A-induced liver fibrosis in rats: Emphasis on TGF-β/SMAD/MAPK signaling pathways. Life Sci. (2020) 240:117096. doi: 10.1016/j.lfs.2019.117096
128. Tang Y, Zhu Z, Li M, Gao L, Wu X, Chen J, et al. Lycorine relieves the CCl4-induced liver fibrosis mainly via the JAK2/STAT3 and PI3K/AKT signaling pathways. Toxicol Appl Pharmacol. (2024) 489:117017. doi: 10.1016/j.taap.2024.117017
129. Wei L, Chen Q, Guo A, Fan J, Wang R, Zhang H. Asiatic acid attenuates CCl4-induced liver fibrosis in rats by regulating the PI3K/AKT/mTOR and Bcl-2/Bax signaling pathways. Int Immunopharmacol. (2018) 60:1–8. doi: 10.1016/j.intimp.2018.04.016
130. Wu L, Zhang Q, Mo W, Feng J, Li S, Li J, et al. Quercetin prevents hepatic fibrosis by inhibiting hepatic stellate cell activation and reducing autophagy via the TGF-β1/Smads and PI3K/Akt pathways. Sci Rep. (2017) 7:9289. doi: 10.1038/s41598-017-09673-5
131. Lin I, Chiou Y, Wu L, Tsai C, Chen C, Chuang W, et al. CCM111 prevents hepatic fibrosis via cooperative inhibition of TGF-β. Wnt and STAT3 signaling pathways. J Food Drug Anal. (2019) 27:184–94. doi: 10.1016/j.jfda.2018.09.008
132. Perumal N, Perumal M, Halagowder D, Sivasithamparam N. Morin attenuates diethylnitrosamine-induced rat liver fibrosis and hepatic stellate cell activation by co-ordinated regulation of Hippo/Yap and TGF-β1/Smad signaling. Biochimie. (2017) 140:10–9. doi: 10.1016/j.biochi.2017.05.017
133. Cao J, Sun Y, Ding X, Li S, Chen B, Lan T. [Arbutin ameliorates liver fibrosis in mice by inhibiting macrophage recruitment and regulating the Akt/NF-κB and Smad signaling pathways]. Nan Fang Yi Ke Da Xue Xue Bao. (2024) 44:652–9. doi: 10.12122/j.issn.1673-4254.2024.04.05
134. Zhang L, Liu C, Yin L, Huang C, Fan S. Mangiferin relieves CCl4-induced liver fibrosis in mice. Sci Rep. (2023) 13:4172. doi: 10.1038/s41598-023-30582-3
135. Qiu J, Chai Y, Duan F, Zhang H, Han X, Chen L, et al. 6-Shogaol alleviates CCl4-induced liver fibrosis by attenuating inflammatory response in mice through the NF-κB pathway. Acta Biochim Pol. (2022) 69:363–70. doi: 10.18388/abp.2020_5802
136. Zaghloul R, Zaghloul A, El-Kashef D. Hepatoprotective effect of Baicalin against thioacetamide-induced cirrhosis in rats: Targeting NOX4/NF-κB/NLRP3 inflammasome signaling pathways. Life Sci. (2022) 295:120410. doi: 10.1016/j.lfs.2022.120410
137. Ma Z, Sheng L, Li J, Qian J, Wu G, Wang Z, et al. Resveratrol Alleviates Hepatic Fibrosis in Associated with Decreased Endoplasmic Reticulum Stress-Mediated Apoptosis and Inflammation. Inflammation. (2022) 45:812–23. doi: 10.1007/s10753-021-01586-w
138. Ren Y, Li H, Zhou L, Lu Z, Shi J, Geng Y, et al. Protective Effect of Spore Powder of Antrodia camphorata ATCC 200183 on CCl4-Induced Liver Fibrosis in Mice. Nutrients. (2020) 12:2778. doi: 10.3390/nu12092778
139. Hu N, Guo C, Dai X, Wang C, Gong L, Yu L, et al. Forsythiae Fructuse water extract attenuates liver fibrosis via TLR4/MyD88/NF-κB and TGF-β/smads signaling pathways. J Ethnopharmacol. (2020) 262:113275. doi: 10.1016/j.jep.2020.113275
140. Lv M, Chen S, Shan M, Si Y, Huang C, Chen J, et al. Arctigenin induces activated HSCs quiescence via AMPK-PPARγ pathway to ameliorate liver fibrosis in mice. Eur J Pharmacol. (2024) 974:176629. doi: 10.1016/j.ejphar.2024.176629
141. Lan T, Yu Y, Zhang J, Li H, Weng Q, Jiang S, et al. Cordycepin Ameliorates Nonalcoholic Steatohepatitis by Activation of the AMP-Activated Protein Kinase Signaling Pathway. Hepatology. (2021) 74:686–703. doi: 10.1002/hep.31749
142. Zheng G, Liu J, Guo F, Zhang Z, Jiang Y, Lin Y, et al. The in vitro and in vivo study of a pyrazole derivative, J-1063, as a novel anti-liver fibrosis agent: Synthesis, biological evaluation, and mechanistic analysis. Bioorg Chem. (2022) 122:105715. doi: 10.1016/j.bioorg.2022.105715
143. Shen D, Cheng H, Cai B, Cai W, Wang B, Zhu Q, et al. N-n-Butyl haloperidol iodide ameliorates liver fibrosis and hepatic stellate cell activation in mice. Acta Pharmacol Sin. (2022) 43:133–45. doi: 10.1038/s41401-021-00630-7
144. Zou G, Zuo S, Lu S, Hu R, Lu Y, Yang J, et al. Bone morphogenetic protein-7 represses hepatic stellate cell activation and liver fibrosis via regulation of TGF-β/Smad signaling pathway. World J Gastroenterol. (2019) 25:4222–34. doi: 10.3748/wjg.v25.i30.4222
145. Liu L, Wang P, Wang Y, Zhang Y, Li C, Yang Z, et al. MiR-130a-3p Alleviates Liver Fibrosis by Suppressing HSCs Activation and Skewing Macrophage to Ly6Clo Phenotype. Front Immunol. (2021) 12:696069. doi: 10.3389/fimmu.2021.696069
146. Liao J, Zhang Z, Yuan Q, Luo L, Hu X. The mouse Anxa6/miR-9-5p/Anxa2 axis modulates TGF-β1-induced mouse hepatic stellate cell (mHSC) activation and CCl4-caused liver fibrosis. Toxicol Lett. (2022) 362:38–49. doi: 10.1016/j.toxlet.2022.04.004
147. Yang L, Fu W, Zhu Y, Wang XGT. β4 suppresses lincRNA-p21-mediated hepatic apoptosis and fibrosis by inhibiting PI3K-AKT-NF-κB pathway. Gene. (2020) 758:144946. doi: 10.1016/j.gene.2020.144946
148. Dong Z, Li S, Wang X, Si L, Ma R, Bao L, et al. lncRNA GAS5 restrains CCl4-induced hepatic fibrosis by targeting miR-23a through the PTEN/PI3K/Akt signaling pathway. Am J Physiol Gastrointest Liver Physiol. (2019) 316:G539–50. doi: 10.1152/ajpgi.00249.2018
149. Mi X, Hou J, Jiang S, Liu Z, Tang S, Liu X, et al. Maltol Mitigates Thioacetamide-induced Liver Fibrosis through TGF-β1-mediated Activation of PI3K/Akt Signaling Pathway. J Agric Food Chem. (2019) 67:1392–401. doi: 10.1021/acs.jafc.8b05943
150. Takashima M, Parsons C, Ikejima K, Watanabe S, White E, Rippe R. The tumor suppressor protein PTEN inhibits rat hepatic stellate cell activation. J Gastroenterol. (2009) 44:847–55. doi: 10.1007/s00535-009-0073-3
151. Kumar P, Raeman R, Chopyk D, Smith T, Verma K, Liu Y, et al. Adiponectin inhibits hepatic stellate cell activation by targeting the PTEN/AKT pathway. Biochim Biophys Acta Mol Basis Dis. (2018) 1864:3537–45. doi: 10.1016/j.bbadis.2018.08.012
152. Shi W, Ju D, Li H, Yuan L, Cui J, Luo D, et al. CD147 Promotes CXCL1 Expression and Modulates Liver Fibrogenesis. Int J Mol Sci. (2018) 19:1145. doi: 10.3390/ijms19041145
153. Liu Q, Chen J, Ma T, Huang W, Lu C. DCDC2 inhibits hepatic stellate cell activation and ameliorates CCl4-induced liver fibrosis by suppressing Wnt/β-catenin signaling. Sci Rep. (2024) 14:9425. doi: 10.1038/s41598-024-59698-w
154. Fu R, Zu S, Liu Y, Li J, Dang W, Liao L, et al. Selective bromodomain and extra-terminal bromodomain inhibitor inactivates macrophages and hepatic stellate cells to inhibit liver inflammation and fibrosis. Bioengineered. (2022) 13:10914–30. doi: 10.1080/21655979.2022.2066756
155. Zhou Y, Chen Y, Zhang X, Xu Q, Wu Z, Cao X, et al. Brahma-Related Gene 1 Inhibition Prevents Liver Fibrosis and Cholangiocarcinoma by Attenuating Progenitor Expansion. Hepatology. (2021) 74:797–815. doi: 10.1002/hep.31780
156. Pan Q, Guo C, Xu Q, Wang J, Li H, Fang C. miR-16 integrates signal pathways in myofibroblasts: determinant of cell fate necessary for fibrosis resolution. Cell Death Dis. (2020) 11:639. doi: 10.1038/s41419-020-02832-z
157. Chen Y, Chen X, Ji Y, Zhu S, Bu F, Du X, et al. PLK1 regulates hepatic stellate cell activation and liver fibrosis through Wnt/β-catenin signalling pathway. J Cell Mol Med. (2020) 24:7405–16. doi: 10.1111/jcmm.15356
158. Du J, Ren W, Zhang Q, Fu N, Han F, Cui P, et al. Heme Oxygenase-1 Suppresses Wnt Signaling Pathway in Nonalcoholic Steatohepatitis-Related Liver Fibrosis. Biomed Res Int. (2020) 2020):4910601. doi: 10.1155/2020/4910601
159. Rong X, Liu J, Yao X, Jiang T, Wang Y, Xie F. Human bone marrow mesenchymal stem cells-derived exosomes alleviate liver fibrosis through the Wnt/β-catenin pathway. Stem Cell Res Ther. (2019) 10:98. doi: 10.1186/s13287-019-1204-2
160. Li W, Yu X, Zhu C, Wang Z, Zhao Z, Li Y, et al. Notum attenuates HBV-related liver fibrosis through inhibiting Wnt 5a mediated non-canonical pathways. Biol Res. (2019) 52:10. doi: 10.1186/s40659-019-0217-8
161. Yu H, Yao Y, Bu F, Chen Y, Wu Y, Yang Y, et al. Blockade of YAP alleviates hepatic fibrosis through accelerating apoptosis and reversion of activated hepatic stellate cells. Mol Immunol. (2019) 107:29–40. doi: 10.1016/j.molimm.2019.01.004
162. Li G, Lu J, Wang C, Chang X, Qu Z, Zhang W, et al. Discovery of Sophoridine α-Aryl Propionamide Derivative ZM600 as a Novel Antihepatic Fibrosis Agent. J Med Chem. (2024) 67:11389–400. doi: 10.1021/acs.jmedchem.4c01010
163. Aoyama Y, Naiki-Ito A, Xiaochen K, Komura M, Kato H, Nagayasu Y, et al. Lactoferrin Prevents Hepatic Injury and Fibrosis via the Inhibition of NF-κB Signaling in a Rat Non-Alcoholic Steatohepatitis Model. Nutrients. (2021) 14:42. doi: 10.3390/nu14010042
164. Yang J, Xu C, Wu M, Wu Y, Jia X, Zhou C, et al. MicroRNA-124 inhibits hepatic stellate cells inflammatory cytokines secretion by targeting IQGAP1 through NF-κB pathway. Int Immunopharmacol. (2021) 95:107520. doi: 10.1016/j.intimp.2021.107520
165. Li J, Jiang H, Wang A, Bu F, Jia P, Zhu S, et al. Hesperetin derivative-16 attenuates CCl4-induced inflammation and liver fibrosis by activating AMPK/SIRT3 pathway. Eur J Pharmacol. (2022) 915:174530. doi: 10.1016/j.ejphar.2021.174530
166. Zhou B, Ling L, Zhang F, Liu T, Zhou H, Qi X, et al. Fibronectin Type III Domain-Containing 5 Attenuates Liver Fibrosis Via Inhibition of Hepatic Stellate Cell Activation. Cell Physiol Biochem. (2018) 48:227–36. doi: 10.1159/000491722
167. Meng X, Kuang H, Wang Q, Zhang H, Wang D, Kang TA. polysaccharide from Codonopsis pilosula roots attenuates carbon tetrachloride-induced liver fibrosis via modulation of TLR4/NF-κB and TGF-β1/Smad3 signaling pathway. Int Immunopharmacol. (2023) 119:110180. doi: 10.1016/j.intimp.2023.110180
168. Li Q, Gong T, Huang Y, Kang L, Warner C, Xie H, et al. Role of noncoding RNAs in liver fibrosis. World J Gastroenterol. (2023) 29:1446–59. doi: 10.3748/wjg.v29.i9.1446
Keywords: liver fibrosis, HSCs, activation, signaling pathways, review
Citation: Zhang Y, Ren L, Tian Y, Guo X, Wei F and Zhang Y (2024) Signaling pathways that activate hepatic stellate cells during liver fibrosis. Front. Med. 11:1454980. doi: 10.3389/fmed.2024.1454980
Received: 26 June 2024; Accepted: 26 August 2024;
Published: 18 September 2024.
Edited by:
Paula Vieira Teixeira Vidigal, Federal University of Minas Gerais, BrazilReviewed by:
Tuo Shao, Harvard Medical School, United StatesWei Chen, Capital Medical University, China
Copyright © 2024 Zhang, Ren, Tian, Guo, Wei and Zhang. This is an open-access article distributed under the terms of the Creative Commons Attribution License (CC BY). The use, distribution or reproduction in other forums is permitted, provided the original author(s) and the copyright owner(s) are credited and that the original publication in this journal is cited, in accordance with accepted academic practice. No use, distribution or reproduction is permitted which does not comply with these terms.
*Correspondence: Yawu Zhang, MTM2MDkzMjA1MDdAMTYzLmNvbQ==