- 1Department of Anesthesiology and Perioperative Medicine, The Second Affiliated Hospital of Anhui Medical University, Hefei, Anhui, China
- 2Department of Anesthesiology and Perioperative Medicine, Anhui Provincial Children's Hospital, Hefei, Anhui, China
Objective: This study utilized lung ultrasound to investigate whether lung protective ventilation reduces pulmonary atelectasis and improves intraoperative oxygenation in infants undergoing laparoscopic surgery.
Methods: Eighty young infants (aged 1–6 months) who received general anesthesia for more than 2 h during laparoscopic surgery were randomized into the lung protective ventilation group (LPV group) and the conventional ventilation group (control group). The LPV group received mechanical ventilation starting at 6 mL/kg tidal volume, 5 cmH2O PEEP, 60% inspired oxygen fraction, and half-hourly alveolar recruitment maneuvers. Control group ventilation began with 8–10 mL/kg tidal volume, 0 cmH2O PEEP, and 60% inspired oxygen fraction. Lung ultrasound was conducted five times—T1 (5 min post-intubation), T2 (5 min post-pneumoperitoneum), T3 (at the end of surgery), T4 (post-extubation), and T5 (prior to discharge from the PACU)—for each infant. Simultaneous arterial blood gas analysis was performed at T1, T2, T3, and T4.
Results: Statistically significant differences were observed in pulmonary atelectasis incidence, lung ultrasound scores, and the PaO2, PaCO2, PaO2/FiO2 ratios at T2, T3, and T4. However, at T5, no statistically significant differences were noted in terms of lung ultrasound scores (4.30 ± 1.87 vs. 5.00 ± 2.43, 95% CI: −1.67 to 0.27, p = 0.153) or the incidence of pulmonary atelectasis (32.5% vs. 47.5%, p = 0.171).
Conclusion: In infants aged 1–6 months, lung protective ventilation during laparoscopy under general anesthesia significantly reduced the incidence of pulmonary atelectasis and enhanced intraoperative oxygenation and dynamic lung compliance compared to conventional ventilation. However, these benefits did not persist; no differences were observed in lung ultrasound scores or the incidence of pulmonary atelectasis at PACU discharge.
Clinical trial registration:
1 Introduction
Laparoscopic surgery in infants and toddlers is becoming more widespread because of its minimal invasiveness. During laparoscopic surgery, an increase in intra-abdominal pressure elevates the diaphragm and reduces both chest wall compliance and functional residual capacity (FRC), leading to further atelectasis formation in the dependent lung bases (1). Pulmonary atelectasis contributes to perioperative lung dysfunction and potential injury (2). Neonates, infants and small children have low functional residual capacity, high pulmonary closing capacity and high oxygen consumption, making them particularly susceptible to atelectasis and hypoxemia during laparoscopic procedures (2, 3). Studies have shown that lung protective ventilation (LPV), such as small tidal volumes (4), positive end-expiratory pressure (PEEP) (5, 6) combined with lung recruitment maneuvers (RMs) (7) can be effective in preventing atelectasis in children. However, the LPV remains a subject of debate and is not well studied (8), particularly in infants and neonates. Lung ultrasound (LUS) is a reliable and accurate noninvasive imaging technique that is effective for detecting anesthesia-induced atelectasis in children (9). Considering the potential benefits of lung protective ventilation in adult and pediatrics patients and the limited research on its use in laparoscopic surgery for infants, a randomized controlled trial was conducted to compare lung protective ventilation with conventional ventilation in these surgeries. The aim of this study was to evaluate the effectiveness of lung protective ventilation (LPV) in reducing the incidence of pulmonary atelectasis and improving oxygenation and dynamic lung compliance during surgery in young infants, as assessed by ultrasound, compared to conventional ventilation. We hypothesized that LPV would lead to lower pulmonary atelectasis and higher intraoperative oxygenation and dynamic lung compliance. Additionally, we anticipated that LPV would reduce the risk of postoperative pulmonary complications in young infants.
2 Methods
2.1 Ethical approval
This study was approved by the Medical Ethics Committee of Anhui Provincial Children’s Hospital, China, on March 23, 2022 (approval number: EYLL-2022-026) and was registered at http://www.chictr.org.cn/ (trialnumber: ChiCTR2200058653; April 13, 2022). For infants in this study, voluntary informed consent was obtained and signed by their parents or legal guardians. This single-center prospective randomized controlled trial was conducted from April 2022 to December 2023 at Anhui Provincial Children’s Hospital in China.
2.2 Participants
Infants aged 1–6 months, classified as American Society of Anesthesiologists (ASA) physical status I-II, and scheduled for laparoscopic abdominal surgery under general anesthesia (>2 h) between April 2022 and December 2023 at Anhui Provincial Children’s Hospital were recruited for this study.
The exclusion criteria were those who were preterm infants, had recent pulmonary inflammation (within one month), had preoperative conditions increasing the risks of severe infections, sepsis, or regurgitation and aspiration, or contraindications to radial artery cannulation, classified as ASA III or higher, lacked family consent, or were needed to taken to the postoperative intensive care unit (ICU) after surgery.
2.3 Randomization
Patients were randomized via computer-generated sequence, and their group assignment was sealed in envelopes. Patients were divided into two groups: the lung protective ventilation group (LPV group: small tidal volume, positive end-expiratory pressure, and recruitment maneuvers) and the conventional ventilation group (control group: zero PEEP and no recruitment maneuvers) at a 1:1 ratio. A single investigator opened the envelopes and implemented the respective mechanical ventilation protocols. The assessor responsible for conducting the lung ultrasound evaluations was blinded to the group assignments.
2.4 Anesthesia protocols and ventilator settings
An intravenous cannula was placed prior to transferring the infant to the operating room. Upon the patient’s arrival in the operating room, routine monitoring of blood pressure (BP), electrocardiogram (ECG), oxygen saturation (SpO2), and body temperature was initiated. Following anesthesia induction, invasive arterial pressure monitoring was commenced. Anesthesia induction involved a sequential intravenous slow injection protocol comprising midazolam (0.05 mg/kg), sufentanil (0.3 μg/kg), propofol (3 mg/kg), and cisatracurium (0.1 mg/kg). Following this, a 3.5–4.5 uncuffed endotracheal tube (10) was inserted, and pressure-controlled ventilation was initiated after successful intubation. Prior to intubation, all patients underwent preoxygenation with 60% (11) oxygen. Anesthesia maintenance involved administering a single caudal block of 0.25% ropivacaine (0.6 mL/kg) combined with an intravenous infusion of remifentanil (0.1 to 0.3 μg/kg/min) and inhalation of sevoflurane (2 to 3%) during surgery. The pneumoperitoneum pressure was consistently maintained at 5–7 mmHg.
Throughout the surgery, haemodynamic stability was maintained, and vasopressors were used as needed. Lactated Ringer’s solution was administered at a rate of 10–15 mL/kg/h during surgery. Additionally, suspended red blood cells, plasma, and albumin were administered as needed. At the end of surgery, the administration of sevoflurane via inhalation and the infusion of remifentanil were ceased. In the LPV group, during surgery, the following ventilation settings were used: tidal volume (TV) of 6 mL/kg, respiratory rate (RR) of 24–28 breaths/min, inspiratory/expiratory (I: E) ratio of 1:1.5, PEEP of 5 cmH2O, fractional inspired oxygen tension (FiO2) of 60% (12) and a flow rate of 2 L/min, with a maximum pressure limit of 30 cmH2O. Lung recruitment maneuvers were performed every 30 min (13). Blood pressure and respiratory parameters were assessed prior to lung recruitment maneuvers to ensure the child was ready for the procedure (14). Recruitment maneuvers were performed, in pressure-controlled mode, with a constant driving pressure of 15 cmH2O. PEEP was increased in steps of 5 cmH2O, from 5 to 15 cmH2O, every three breaths. The target recruitment pressure of 30 cmH2O was maintained for 10 breaths (15). For the control group, a tidal volume (TV) of 8–10 mL/kg, a respiratory rate (RR) of 24–28 breaths/min, an I:E ratio of 1:1.5, a PEEP of 0 cmH2O (16), a FiO2 of 60%, and a flow rate of 2 L/min were used, with a maximum pressure limit of 30 cmH2O, and lung recruitment maneuvers were not performed. When mechanical ventilation was performed after induction in both groups, the presence or absence of autoPEEP (17) was observed and recorded. Postoperatively, upon awakening from anesthesia, 0.02 mg/kg atropine and 0.05 mg/kg neostigmine were administered after the infant recovered spontaneous respiration (18). The tracheal tube was removed upon observing indications like a conjugate gaze, purposeful movements, the eye opening, a tidal volume > 5 mL/kg, and facial ghosting (19). All infants were transferred to the postanesthesia care unit (PACU) and received supplemental oxygen at a rate of 2 L/min through a simple mask equipped with an oxygen reservoir. Gradually reduced to room air when the SpO2 level exceeded 95% and remained stable. After meeting the criteria for discharge from the PACU (20), the infants were returned to their wards.
2.5 Lung ultrasound
Patients were placed in the supine position and scanned using ultrasound (SONIMAGE HS2-KONICA MINOLTA, Shanghai, China) according to the lung ultrasound score examination method described by Acosta et al. (9, 21). Each half of the chest was divided into three regions (anterior, posterior, and lateral) by the anterior and posterior axillary lines and further divided into six regions by an axial line 1 cm above the nipples. The 12 regions in the lung were scanned sequentially from right to left, from cranial to caudal and anterior to posterior ends. Lung ultrasound scores were assessed using ultrasound at T1 (5 min post-intubation), T2 (5 min post-pneumoperitoneum), T3 (at the end of surgery), T4 (post-extubation), and T5 (prior to discharge from the PACU). The 12 quadrants were assigned a score of 0 to 3 based on the following scoring system: 0, 0 to 2 B lines; 1, at least three B lines or one or multiple small subpleural consolidations separated by a normal pleural line; 2, multiple coalescent B lines or multiple small subpleural consolidations separated by a thickened or irregular pleural line; and 3, consolidation or small subpleural consolidation of more than 1 cm × 2 cm (Figure 1). The consolidation scores were recorded at each time point. Significant atelectasis was determined if any region had a consolidation score of 2 (22). The lung ultrasound score (0–36) was then calculated by adding up the 12 individual quadrant scores, with higher scores indicating more severe aeration loss.
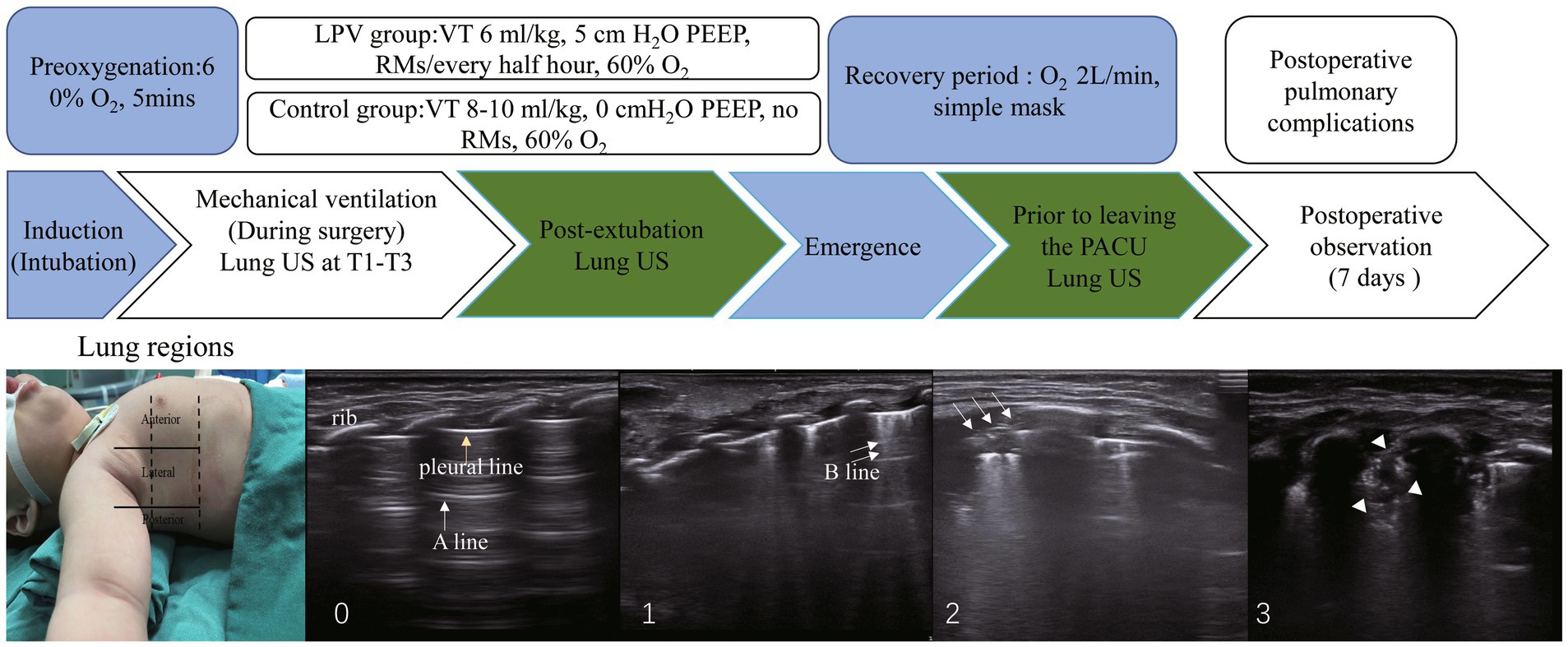
Figure 1. The study protocol and ultrasound lung examination region is shown. From left to right are scores: 0, 0 to 2 B lines; 1, at least three B lines or one or multiple small subpleural consolidations separated by a normal pleural line; 2, multiple coalescent B lines or multiple small subpleural consolidations separated by a thickened or irregular pleural line; 3, consolidation or small subpleural consolidation of more than 1 cm x 2 cm. One yellow arrow, pleural line; one white arrow, A line; two white arrows, B line; three white arrows, subpleural consolidations; white arrowheads, consolidation. Significant atelectasis is determined if any region had a consolidation score of 2. RMs, recruitment maneuvers, PEEP, positive end-expiratory pressure. T1, 5 min post-intubation, T2, 5 min post-pneumoperitoneum, T3, at the end of surgery.
2.6 Primary outcome
Lung ultrasound scores and significant atelectasis incidence rates were assessed at T1 (5 min post-intubation), T2 (5 min post-pneumoperitoneum), T3 (at the end of surgery), T4 (post-extubation), and T5 (prior to discharge from the PACU).
2.7 Secondary outcomes
Measurements of PaO2 (partial pressure of arterial oxygen), PaCO2 (partial pressure of arterial carbon dioxide), the PaO2/FiO2 ratio, HR (heart rate), MAP (mean arterial pressure), and SpO2 (oxygen saturation) were recorded at T1, T2, T3 and T4. Peak airway pressure (Ppeak) and dynamic lung compliance (Cdyn) were directly measured on the anesthesia machine at T1, T2 and T3. The presence or absence of automatic PEEP and its value were recorded at T1. The time from the recovery of spontaneous breathing to extubation, and the total duration of their stay in the PACU, were both recorded. An independent investigator, blinded to the study details, evaluated the incidence of pulmonary complications within 7 days after surgery. These complications were scored based on the operational definitions of postoperative pulmonary complications provided by Hulzebos et al. (23) (Figure 2).
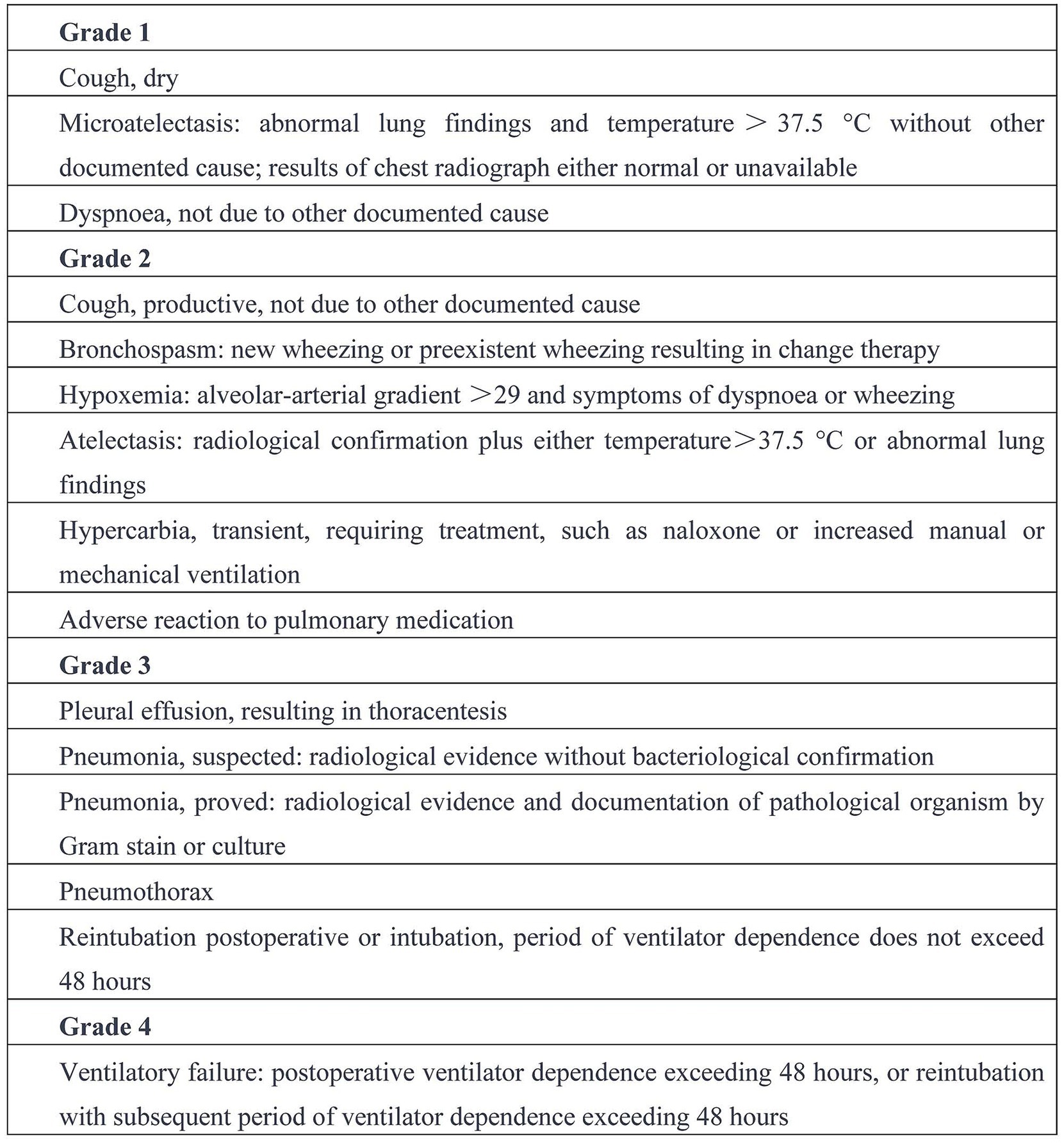
Figure 2. Operational definitions of postoperative pulmonary complications (23).
2.8 Safety of the intervention
The study evaluated the likelihood of potential side effects, such as transient hypotension (defined as less than 80% of baseline blood pressure) and oxygen desaturation (SpO2 below 95%), following regular alveolar recruitment maneuvers.
2.9 Statistical analysis
Unless otherwise specified, all the data are presented as the mean ± standard deviation or median (interquartile range). The Shapiro–Wilk test was used to assess the normality of the distribution. Outcomes were assessed using independent t tests, Mann–Whitney U tests and chi–square tests. A two-tailed p value <0.05 was considered to indicate statistical significance. Repeated-measures ANOVA was used to detect differences in the measured parameters between treatment groups and over time via a mixed-model procedure, with Bonferroni’s test for multiple comparisions. p values <0.05 were considered to indicate statistical significance. Data analysis was performed using SPSS software (version 25.0; SPSS, Inc., Chicago, Illinois, USA) and data visualization were carried out with GraphPad Prims 9 (GraphPad Software, SanDiego, CA, USA).
2.10 Sample size calculation
We used PASS 15.0 to calculate the sample size, basing our calculations on data from prior research. One study reported that lung ultrasound had an accuracy of 88% for detecting pulmonary atelectasis (9). According to our pilot study, the incidence of ultrasound-detected pulmonary atelectasis was 70%. We assumed this incidence would be halved by lung recruitment maneuvers and PEEP (15). With an alpha error of 0.05 and a power of 80%, the required sample size was calculated to be 29 patients per group. Accounting for a 20% dropout rate, we determined that a total of 73 patients would be needed, and thus we planned to enroll 80 patients in the study.
3 Results
Patient enrolment started on April 2022. A total of 80 patients were randomly assigned to the LPV (n = 40) or control (n = 40) group (Figure 3). There were no statistically significant differences in age, gender, height, weight, duration of surgery, or duration of anesthesia between the two groups of children (Table 1).
3.1 Primary outcome
There were statistically significant differences between the LPV group and the control group at T2 in terms of ultrasound scores (6.35 ± 2.66 vs. 10.95 ± 4.07, 95% CI (−6.13 to −3.07), p < 0.001) and the incidence of atelectasis (62.5% vs. 87.5%, p = 0.010); at T3 in terms of ultrasound scores (6.65 ± 3.15 vs. 12.53 ± 4.29, 95% CI (−7.55 to −4.20), p < 0.001) and the incidence of atelectasis (55% vs. 90%, p = 0.0005); and at T4 in terms of ultrasound scores (5.50 ± 2.75 vs. 8.00 ± 2.92, 95% CI (−3.76 to −1.24), p < 0.001) and the incidence of atelectasis (35% vs. 72.5%, p = 0.001). At T1, there was no statistically significant difference in lung ultrasound score (7.35 ± 5.06 vs. 5.85 ± 4.14, 95% CI (−0.56 to 3.56), p = 0.151), the incidence of atelectasis (80% vs. 77.5%, p = 0.785). Moreover, there were no statistically significant differences between the LPV group and the control group at T5 in terms of ultrasound score (4.30 ± 1.87 vs. 5.00 ± 2.43, 95% CI (−1.67 to 0.27), p = 0.153) or incidence of atelectasis (32.5% vs. 47.5%, p = 0.171) (Table 2). Ultrasound scores of both groups at various time points are presented in Figure 4.
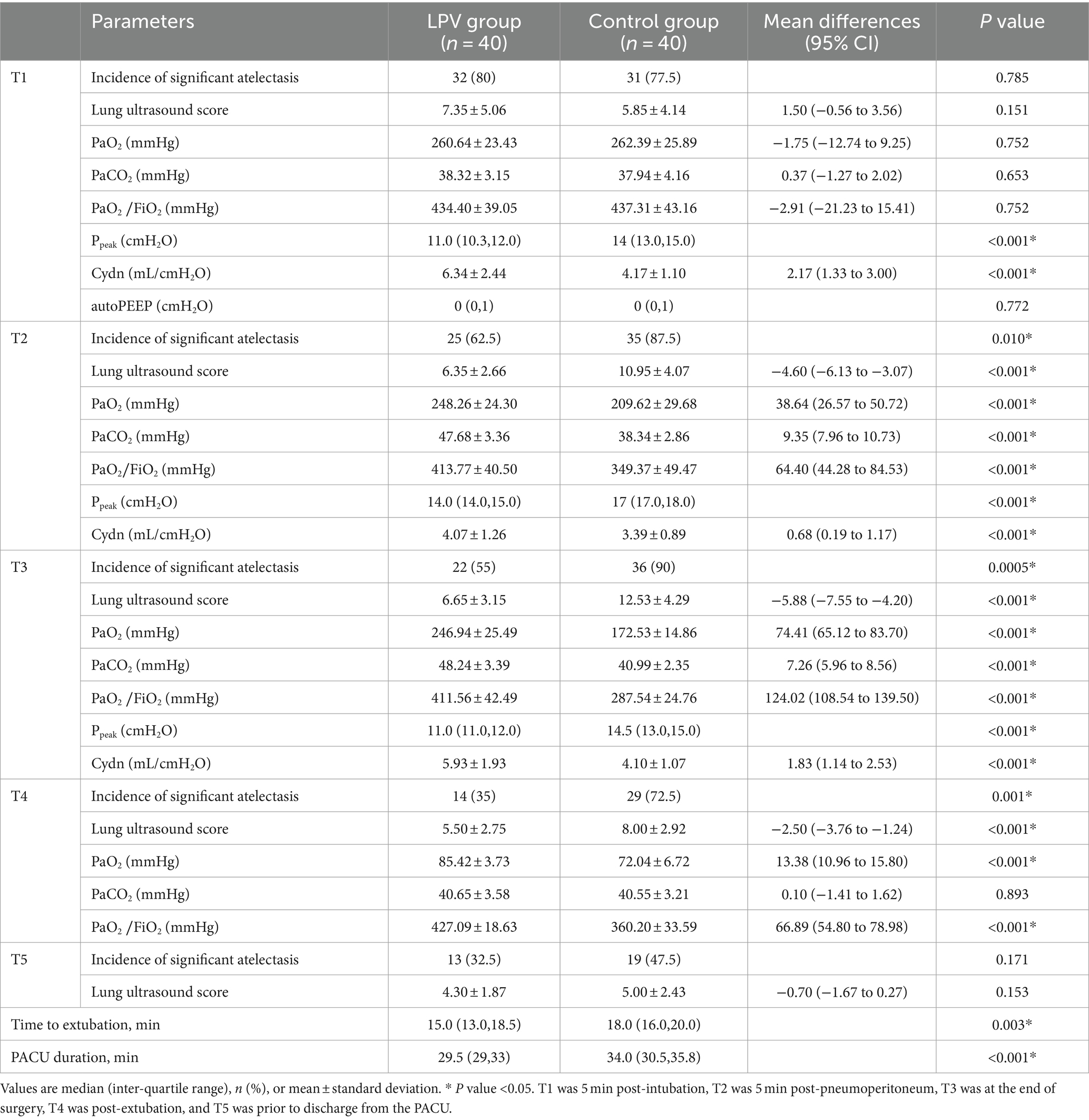
Table 2. Lung ultrasound score and comparison of intra- and postoperative variables between the LPV group and control group.
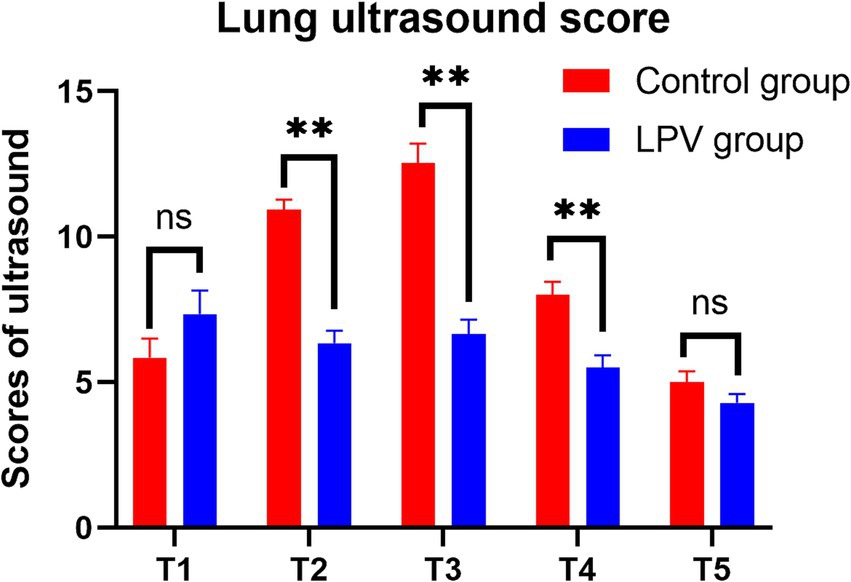
Figure 4. Comparison of lung ultrasound scores at different time points between the two groups. Ns, no significance. ** indicates statistical significance.
3.2 Secondary outcomes
At T1, there was no statistically significant difference in PaO2 (260.64 ± 23.43 vs. 262.39 ± 25.89, 95% CI (−12.74 to 9.25), p = 0.752), PaCO2 (38.32 ± 3.15 vs. 37.94 ± 4.16, 95% CI (−1.27 to 2.02), p = 0.653), or the PaO2/FiO2 ratio (434.40 ± 39.05 vs. 437.31 ± 43.16, 95% CI (−21.23 to 15.41), p = 0.752). There were statistically significant differences between the LPV group and the control group at T2 and T3 in terms of the PaO2, PaCO2, PaO2/FiO2 or Cdyn; at T4, in terms of the PaO2 or PaO2/FiO2. The LPV group demonstrated shorter extubation times and reduced lengths of stay in the PACUcompared to the control group (Table 2). There was no significant difference in autoPEEP between the two groups at T1. The two groups showed no significant difference in HR, MAP or SpO2 at T1, T2, T3, or T4 (Table 3). No significant difference was observed in the incidence of pulmonary complications within 7 days after surgery (p = 0.516) (Table 4). Alveolar recruitment maneuvers did not show transient hypotension and oxygen desaturation.
4 Discussion
In this prospective RCT, the primary outcome indicated that lung protective ventilation reduced the incidence of pulmonary atelectasis in infants during laparoscopy under general anesthesia compared to conventional ventilation, enhanced intraoperative oxygenation and Cdyn, the duration of extubation and the length of stay in the PACU were both reduced. However, this improvement was transient, with no observed differences in lung ultrasound scores or incidence of pulmonary atelectasis at discharge from the PACU. Moreover, no significant differences were observed in the incidence of postoperative pulmonary complications within 7 days after surgery.
Our results align with previous studies (7, 15) demonstrating that laparoscopic surgery under general anesthesia can lead to pulmonary atelectasis in children, we provide data on infants. Acosta et al. (15) discovered that lung collapse resulting from capnoperitoneum can be mitigated through the use of LPV in all children aged 6 months to 7 years undergoing laparoscopic surgery. The incidence of pulmonary atelectasis resulting from pneumoperitoneum can potentially be reduced through the use of LPV. The primary mechanisms involve the application of lung recruitment maneuvers and PEEP, which effectively mitigate diaphragmatic elevation and subsequent intra-abdominal pressure increase caused by intraperitoneal gas accumulation (24). Recruitment maneuvers can re-expand atelectatic lung tissue and improve lung compliance; however, they may also carry risks such as barotrauma, hemodynamic instability, and worsening oxygenation (14), the pressure-volume curve or loop method, the end-expiratory lung volume–static compliance method, and ultrasound can all be used to assess the effectiveness of lung recruitment (7, 25). Other studies have shown that the optimal PEEP under RM can be determined based on the static compliance of the respiratory system (crs) (26). This study revealed that combining lung recruitment maneuvers with PEEP effectively reopened collapsed alveoli and enhanced intraoperative oxygenation and dynamic lung compliance during laparoscopic surgery in small infants. However, one limitation is that the extent of alveolar recruitment remains somewhat unclear.
Our findings indicate that recruitment maneuvers, followed by a PEEP of 5 cmH2O, are both effective and safe for reducing the number of atelectatic areas following laparoscopic surgery in young infants, but the specific numerical setting for PEEP remains controversial. J-H Lee’s (4) study posits that a PEEP of 10 cm H₂O is an appropriate level for children in mechanically ventilation. However, other studies (6, 27) have indicated improvements in lung ultrasound scores and reductions in the incidence of atelectasis in children administered a PEEP of 5 cmH₂O. Although the application of PEEP facilitates the reopening of collapsed alveoli and improves oxygenation, the use of high levels of PEEP must be carefully considered due to potential risks such as alveolar overdistension and hemodynamic instability (28). These effects are particularly undesirable in infants undergoing laparoscopic surgery. Due to potential side effects, clinicians must exercise caution when using 10 cmH2O PEEP during laparoscopic surgery for small infants. For safety considerations, this study employed 5 cmH₂O PEEP in the LPV group.
Studies have shown that the detrimental effects of capnoperitoneum could be reversed by a protective ventilation strategy combining lung recruitment and an individualized PEEP titration during laparoscopic surgery in children (5), the individualized lung protective ventilation during laparoscopic surgery in young infantsshould be the matter of future studies.
Avoiding high fractions of oxygen in inspired gas during induction and maintenance of anesthesia may prevent the formation of atelectasis (29). Studies have shown that a lower oxygen concentration during anesthesia induction is associated with a lower risk of atelectasis immediately after anesthesia induction in children, and 60% oxygen should be applied to prevent atelectasis (11). The changes in oxygen concentration were consistent in both groups, with 60% FiO2 used at induction and intraoperatively, potentially reducing the occurrence of pulmonary atelectasis. At extubation, the air oxygen concentration was used, and in the PACU, the infants’ FiO2 was 0.29. To avoid residual effects of anesthetic drugs, incomplete lung re-expansion, reduced chest wall, and diaphragmatic activity caused by surgical injury and pain, and gastrointestinal reactions (30), postoperative oxygen respiratory support was maintained during the awakening period until SpO2 stabilized at 95% or higher. Once the SpO2 level exceeded 95% and stabilized, the FiO2 was gradually reduced to room air levels. The infants in both groups did not develop hypoxemia after breathing air. The potential effects of oxygen concentration changes warrant further exploration.
Our study demonstrated that patients in both groups experienced significant improvements in pulmonary ventilation after extubation, despite variations in PaO2, extubation times, and lengths of stay in the PACU. There were no statistically significant differences in ultrasound scores and incidence of significant pulmonary atelectasis in infants discharged from the PACU, which is consistent with the findings of Zhu’s (18) study. Some patients might have performed uncontrolled recruitment maneuvers by sighing or coughing (31), the sigh is a normal homeostatic reflex that maintains lung compliance and decreases the risk of atelectasis (32). Both groups of infants have different body movements, coughing or crying, which may improve ventilation of the lungs.
Despite hypercapnia occurring during the intraoperative period, it was restored to acceptable levels by the time of extubation (33). The findings of our research indicate that LPV not only enhances ventilation and mitigates intraoperative atelectasis but also preserves hemodynamic stability without inducing fluctuations. Our secondary findings indicated no variation in respiratory complications within 7 days after surgery, which may relate to the normal respiratory physiology of the infants included in the study. In fact, the potential of different tidal volumes to reduce the risk of postoperative pulmonary complications depends on the patient’s respiratory compliance (34). Moreover, large-sample, multicenter studies are necessary to further investigate the clinical significance of lung protective ventilation in small infant laparoscopic surgery.
Our study has several limitations. First, we included only infants with normal respiratory physiology, and those with lung disease or who were critically ill were not evaluated. Second, while all sonographers involved in our study were professionally trained, the observed atelectasis may be explained by the fact that small atelectasis can be hidden within the rib’s acoustic shadows whenever the longitudinally oriented probe placed in the traditional orientation crosses the rib (9), during the second ultrasound examination, factors such as the patient’s positioning, the presence of surgical drapes, the requirement for sterility, and the surgeon’s maneuvers can impede the accessibility and effectiveness of the ultrasound, we conducted a comprehensive examination of pulmonary ultrasound images by adjusting the probe orientation and pausing the surgery to minimize the potential for misinterpretation (35). Third, we were unable to confirm the effect of recruitment maneuvers, although we assessed the potential risks associated with recruitment maneuvers and adhered to the established standards for their implementation, we could not rule out the possibility of overinflation, and we applied a PEEP of 5 cmH2O in this trial, which might not be the optimal PEEP during laparoscopic surgery in infants. Fourth, we did not take into account the effect of autoPEEP when setting up ventilation, we recorded auto PEEP during mechanical ventilation after endotracheal intubation, however, the data of the two groups showed no difference, equalizing the possible influence of auto PEEP on the results. And we looked for the etiology and manage patients with auto PEEP (17), such as the use of increased depth of anesthesia to reduce sputum obstruction and avoid airway spasm, as well as the avoidance of thinner tracheal intubation (36, 37), thus reducing the potential impact that autoPEEP could have on this study. Further exploration of individualized PEEP in small infants via laparoscopy at a later date is warranted.
In conclusion, lung protective ventilation significantly reduced the incidence of pulmonary atelectasis in infants aged 1–6 months during laparoscopy under general anesthesia compared with conventional ventilation and improved intraoperative oxygenation and Cdyn, reduced in both the time to extubation and the length of stay in the PACU, however, pulmonary atelectasis did not seem to last very long, there was no difference in its incidence at discharge from the PACU, and nor in postoperative pulmonary complications within 7 days after surgery.
Data availability statement
The raw data supporting the conclusions of this article will be made available by the authors, without undue reservation.
Ethics statement
The studies involving humans were approved by the Medical Ethics Committee of Anhui Provincial Children’s Hospital. The studies were conducted in accordance with the local legislation and institutional requirements. Written informed consent for participation in this study was provided by the participants’ legal guardians/next of kin.
Author contributions
KY: Data curation, Investigation, Methodology, Validation, Writing – original draft, Writing – review & editing. JW: Data curation, Formal analysis, Investigation, Methodology, Validation, Writing – original draft. HW: Data curation, Writing – original draft. YS: Formal analysis, Methodology, Visualization, Writing – review & editing. YX: Conceptualization, Data curation, Formal analysis, Funding acquisition, Investigation, Methodology, Project administration, Software, Supervision, Validation, Visualization, Writing – original draft, Writing – review & editing. QC: Conceptualization, Data curation, Formal analysis, Funding acquisition, Investigation, Methodology, Project administration, Resources, Software, Supervision, Validation, Visualization, Writing – original draft, Writing – review & editing.
Funding
The author(s) declare that financial support was received for the research, authorship, and/or publication of this article. This study was supported by grants from the Natural Science Foundation of Anhui Children’s Hospital (grant No. 21etyy005 to YX), the major project of Natural Science Research of Anhui Higher Education Institutes (grant No.2023AH040376 to QC).
Conflict of interest
The authors declare that the research was conducted in the absence of any commercial or financial relationships that could be construed as a potential conflict of interest.
Publisher’s note
All claims expressed in this article are solely those of the authors and do not necessarily represent those of their affiliated organizations, or those of the publisher, the editors and the reviewers. Any product that may be evaluated in this article, or claim that may be made by its manufacturer, is not guaranteed or endorsed by the publisher.
References
1. Spadaro, S, Karbing, DS, Mauri, T, Marangoni, E, Mojoli, F, Valpiani, G, et al. Effect of positive end-expiratory pressure on pulmonary shunt and dynamic compliance during abdominal surgery. Br J Anaesth. (2016) 116:855–61. doi: 10.1093/bja/aew123
2. Lagier, D, Zeng, C, Fernandez-Bustamante, A, and Vidal Melo, MF. Perioperative pulmonary atelectasis: part II. Clinical Implications. Anesthesiology. (2022) 136:206–36. doi: 10.1097/aln.0000000000004009
3. Baroncini, S, Gentili, A, Pigna, A, Fae, M, Tonini, C, and Tognù, A. Anaesthesia for laparoscopic surgery in paediatrics. Minerva Anestesiol. (2002) 68:406–13. doi: 10.1016/j.eeus.2006.08.003
4. Lee, JH, Kang, P, Song, IS, Ji, SH, Lee, HC, Jang, YE, et al. Determining optimal positive end-expiratory pressure and tidal volume in children by intratidal compliance: a prospective observational study. Br J Anaesth. (2022) 128:214–21. doi: 10.1016/j.bja.2021.09.024
5. Acosta, CM, Poliotto, S, Abrego, D, Bradley, D, de Esteban, S, Mir, F, et al. Effect of an individualized lung protective ventilation on lung strain and stress in children undergoing laparoscopy: an observational cohort study. Anesthesiology. (2023) 140:430–41. doi: 10.1097/ALN.0000000000004856
6. Lee, HM, Min, JY, Lee, JR, Lee, MH, and Byon, HJ. Effects of positive end-expiratory pressure on pulmonary atelectasis after paediatric laparoscopic surgery as assessed by ultrasound: a randomised controlled study. Anaesth Crit Care Pain Med. (2022) 41:101034. doi: 10.1016/j.accpm.2022.101034
7. Gao, Q, Ji, H, Wu, Z, and Zhao, P. Effect of ultrasound-guided lung recruitment manoeuvre on perioperative atelectasis during laparoscopy in young infants: a randomised controlled trial. J Clin Anesth. (2023) 86:111075. doi: 10.1016/j.jclinane.2023.111075
8. Musch, G, and Vidal Melo, MF. Intraoperative protective mechanical ventilation: fact or fiction? Anesthesiology. (2022) 137:381–3. doi: 10.1097/ALN.0000000000004366
9. Acosta, CM, Maidana, GA, Jacovitti, D, Belaunzaran, A, Cereceda, S, Rae, E, et al. Accuracy of transthoracic lung ultrasound for diagnosing anesthesia-induced atelectasis in children. Anesthesiology. (2014) 120:1370–9. doi: 10.1097/ALN.0000000000000231
10. Isa, M, Holzki, J, Hagemeier, A, Rothschild, MA, and Coté, CJ. Anatomical in vitro investigations of the pediatric larynx: A call for manufacturer redesign of tracheal tube cuff location and perhaps a call to reconsider the use of Uncuffed tracheal tubes. Anesth Analg. (2021) 133:894–902. doi: 10.1213/ANE.0000000000005565
11. Kim, HI, Min, JY, Lee, JR, Kwan Woong, C, Cho, MR, and Byon, HJ. The effect of oxygen concentration on atelectasis formation during induction of general anesthesia in children: a prospective randomized controlled trial. Paediatr Anaesth. (2021) 31:1276–81. doi: 10.1111/pan.14304
12. Abdallah, NM, Elela, AHA, Maghawry, HH, and Alkonaiesy, RM. Effect of different mechanical ventilation modes on cerebral blood flow during thoracoscopic surgery in neonates: a randomised controlled trial. Indian J Anaesth. (2022) 66:651–6. doi: 10.4103/ija.ija_1065_21
13. Jang, Y-E, Ji, S-H, Kim, E-H, Lee, J-H, Kim, J-T, and Kim, H-S. Effect of regular alveolar recruitment on intraoperative atelectasis in paediatric patients ventilated in the prone position: a randomised controlled trial. Br J Anaesth. (2020) 124:648–55. doi: 10.1016/j.bja.2020.01.022
14. Bruins, S, Sommerfield, D, Powers, N, and von Ungern-Sternberg, BS. Atelectasis and lung recruitment in pediatric anesthesia: an educational review. Paediatr Anaesth. (2022) 32:321–9. doi: 10.1111/pan.14335
15. Acosta, CM, Sara, T, Carpinella, M, Volpicelli, G, Ricci, L, Poliotto, S, et al. Lung recruitment prevents collapse during laparoscopy in children: a randomised controlled trial. Eur J Anaesthesiol. (2018) 35:573–80. doi: 10.1097/EJA.0000000000000761
16. Sun, Y, Shen, S, Deng, X, Cai, Y, and Du, Y. Lung protective ventilation in infants undergoing cardiopulmonary bypass surgery for congenital heart disease: a prospective randomized controlled trial. Pediatr Anesth. (2020) 30:814–22. doi: 10.1111/pan.13894
17. Keller, M, Applefeld, W, Acho, M, and Lee, BW. How I Teach Auto-PEEP: Applying the Physiology of Expiration. Sch. (2022) 3:610–24. doi: 10.34197/ats-scholar.2022-0024HT
18. Zhu, C, Zhang, S, Dong, J, and Wei, R. Effects of positive end-expiratory pressure/recruitment manoeuvres compared with zero end-expiratory pressure on atelectasis in children: a randomised clinical trial. Eur J Anaesthesiol. (2021) 38:1026–33. doi: 10.1097/EJA.0000000000001451
19. Templeton, TW, Goenaga-Diaz, EJ, Downard, MG, McLouth, CJ, Smith, TE, Templeton, LB, et al. Assessment of common criteria for awake Extubation in infants and young children. Anesthesiology. (2019) 131:801–8. doi: 10.1097/ALN.0000000000002870
20. Bizuneh, YB, Berhe, YW, Ashagrie, HE, Lema, GF, and Fentie, DY. Current practice of discharging patients from post anesthesia care unit after surgical operations. Res Square. (2020) 2018. doi: 10.21203/rs.3.rs-110088/v1
21. Monastesse, A, Girard, F, Massicotte, N, Chartrand-Lefebvre, C, and Girard, M. Lung ultrasonography for the assessment of perioperative atelectasis: a pilot feasibility study. Anesth Analg. (2017) 124:494–504. doi: 10.1213/ane.0000000000001603
22. Song, IK, Kim, EH, Lee, JH, Ro, S, Kim, HS, and Kim, JT. Effects of an alveolar recruitment manoeuvre guided by lung ultrasound on anaesthesia-induced atelectasis in infants: a randomised, controlled trial. Anaesthesia. (2017) 72:214–22. doi: 10.1111/anae.13713
23. Hulzebos, EHJ, Helders, PJM, Favié, NJ, De Bie, RA, Brutel de la Riviere, A, and Van Meeteren, NLU. Preoperative intensive inspiratory muscle training to prevent postoperative pulmonary complications in high-risk patients undergoing CABG surgery: a randomized clinical trial. JAMA. (2006) 296:1851–7. doi: 10.1001/jama.296.15.1851
24. Zeng, C, Lagier, D, Lee, J-W, and Vidal Melo, MF. Perioperative pulmonary atelectasis: part I. Biology and Mechanisms. Anesthesiology. (2022) 136:181–205. doi: 10.1097/ALN.0000000000003943
25. Zheng, M. Respiratory mechanics: revisiting the appraisement of lung recruitment. Respir Care. (2023) 68:1262–70. doi: 10.4187/respcare.10601
26. D’Antini, D, Rauseo, M, Grasso, S, Mirabella, L, Camporota, L, Cotoia, A, et al. Physiological effects of the open lung approach during laparoscopic cholecystectomy: focus on driving pressure. Minerva Anestesiol. (2018) 84:159–67. doi: 10.23736/S0375-9393.17.12042-0
27. Zhu, C, Zhang, R, Jiang, Y, et al. (2020). Protective Lung Ventilation in Children at Low Risk: Is it Necessary?. SSRN Electronic Journal. doi: 10.2139/ssrn.3605344
28. Serpa Neto, A, and Schultz, MJ. Optimizing the settings on the ventilator: high PEEP for all? JAMA. (2017) 317:1413–4. doi: 10.1001/jama.2017.2570
29. Grandville, B, Petak, F, Albu, G, Bayat, S, Pichon, I, and Habre, W. High inspired oxygen fraction impairs lung volume and ventilation heterogeneity in healthy children: a double-blind randomised controlled trial. Br J Anaesth. (2019) 122:682–91. doi: 10.1016/j.bja.2019.01.036
30. Suzuki, S. Oxygen administration for postoperative surgical patients: a narrative review. J Intensive Care. (2020) 8:79. doi: 10.1186/s40560-020-00498-5
31. Pereira, SM, Tucci, MR, Morais, CCA, Simoes, CM, Tonelotto, BFF, Pompeo, MS, et al. Individual positive end-expiratory pressure settings optimize intraoperative mechanical ventilation and reduce postoperative atelectasis. Anesthesiology. (2018) 129:1070–81. doi: 10.1097/ALN.0000000000002435
32. Hartland, BL, Newell, TJ, and Damico, N. Alveolar recruitment maneuvers under general anesthesia: a systematic review of the literature. Respir Care. (2015) 60:609–20. doi: 10.4187/respcare.03488
33. Wong, SK, Chim, M, Allen, J, Butler, A, Tyrrell, J, Hurley, T, et al. Carbon dioxide levels in neonates: what are safe parameters? Pediatr Res. (2022) 91:1049–56. doi: 10.1038/s41390-021-01473-y
34. Suleiman, A, Costa, E, Santer, P, Tartler, TM, Wachtendorf, LJ, Teja, B, et al. Association between intraoperative tidal volume and postoperative respiratory complications is dependent on respiratory elastance: a retrospective, multicentre cohort study. Br J Anaesth. (2022) 129:263–72. doi: 10.1016/j.bja.2022.05.005
35. Cammarota, G, Simonte, R, Longhini, F, Spadaro, S, Vetrugno, L, and De Robertis, E. Advanced point-of-care bedside monitoring for acute respiratory failure. Anesthesiology. (2023) 138:317–34. doi: 10.1097/ALN.0000000000004480
36. Coppola, S, Caccioppola, A, Froio, S, Ferrari, E, Gotti, M, Formenti, P, et al. Dynamic hyperinflation and intrinsic positive end-expiratory pressure in ARDS patients. Crit Care Lond Engl. (2019) 23:375. doi: 10.1186/s13054-019-2611-6
Keywords: pulmonary atelectasis, lung protective ventilation, positive end-expiratory pressure, recruitment maneuvers, infant, laparoscopy, ultrasonography
Citation: Yue K, Wang J, Wu H, Sun Y, Xia Y and Chen Q (2024) A comparison of the effects of lung protective ventilation and conventional ventilation on the occurrence of atelectasis during laparoscopic surgery in young infants: a randomized controlled trial. Front. Med. 11:1486236. doi: 10.3389/fmed.2024.1486236
Edited by:
Luigi Vetrugno, University of Studies G. d’Annunzio Chieti and Pescara, ItalyReviewed by:
Savino Spadaro, University of Ferrara, ItalyAnna Camporesi, Ospedale dei Bambini Vittore Buzzi, Italy
Copyright © 2024 Yue, Wang, Wu, Sun, Xia and Chen. This is an open-access article distributed under the terms of the Creative Commons Attribution License (CC BY). The use, distribution or reproduction in other forums is permitted, provided the original author(s) and the copyright owner(s) are credited and that the original publication in this journal is cited, in accordance with accepted academic practice. No use, distribution or reproduction is permitted which does not comply with these terms.
*Correspondence: Yin Xia, eGlheWluMjIwMUBvdXRsb29rLmNvbQ==; Qi Chen, Y3FfOTExQDEyNi5jb20=
†These authors have contributed equally to this work and share first authorship