- 1School of Medicine, Jiangsu University, Zhenjiang, China
- 2Faculty of Civil Engineering and Mechanics, Jiangsu University, Zhenjiang, China
Systemic lupus erythematosus (SLE) is a systemic autoimmune disease, which is mainly caused by the imbalance of immune cells. Current treatment regimens predominately rely on corticosteroids and immunosuppressive agents, accompanied by various side effects. Interleukin-2 (IL-2) is deemed an important cytokine for innate immune cells and adaptive immune cells, especially for the promotion of Treg cells. By combining IL-2/IL-2R system with engineered T cell-based immunotherapies to enhance the therapeutic efficacy of engineered T cells shows its potential in autoimmune diseases. But the pleiotropy of IL-2 may cause simultaneous stimulation and systemic toxicity, limiting its therapeutic use. There is a growing focus on using IL-2 in combination strategies for synergistic immune enhancement. In this article, we review the IL-2/IL-2R signaling, including IL-2 dependent signaling and IL-2 independent signaling, and discuss its functions in regulation of different immune cells. In addition, we summarize major clinical application of low-dose IL-2 treatment in SLE with or without other agents, such as rapamycin, tocilizumab and rituximab, present the IL-2 variants and fusion proteins designed for SLE, and highlight the future trends for research on these cytokine-based immunotherapies. It will help to design further optimized IL-2-based therapy for SLE.
1 Introduction
Systemic lupus erythematosus (SLE) is a systemic autoimmune disease characterized by red speckles on the skin and multiple organ damage. The pathophysiology of SLE is complex that remains incompletely understood. Studies showed that the decreases of regulatory T (Treg) cells as well as the imbalance of effector T (Teff) cell subsets and Treg cell subsets may contribute to the pathogenesis of SLE. Current treatment regimens mainly rely on corticosteroids and immunosuppressive agents. These traditional treatments are likely to result in substantial side effects, such as various infections. Some cases thus become refractory or severe SLE. Sustained remission is achieved in only a small fraction of patients. So it is urgent to develop an effective treatment for SLE.
Interleukin-2 (IL-2) is considered as an important cytokine for the inflammatory immune responses against pathogens and tumors. It was previously introduced in a high dose setting to treat malignant diseases. During 1980s, the clinical application of high-dose IL-2 treatment showed the regression of metastatic renal carcinomas and melanomas (1). In 1992, the therapeutic application of IL-2 (Aldesleukin, trade name Proleukin) in treating the renal cancer was approved by the US Food and Drug Administration (FDA) (2, 3). It was subsequently approved for patients with metastatic melanoma in 1998. Later studies show that IL-2 is key to immune tolerance (4). It is essential to the differentiation and proliferation of Treg cells. Currently, clinical studies have shown the safety and efficacy of low-dose IL-2 in the treatment of SLE (5). Compared to the traditional treatment, low-dose IL-2 treatment is effective without increased infection incidence. However, the optimal dosage of IL-2 therapy remains to be determined. This review takes combination therapies into consideration. By comparing the curative effect of using IL-2 as single agent or as a component of combination therapies to further explore the optimized clinical application of IL-2 in SLE treatment, such as drug dosage adjustment, selection of combination drugs, and patient management.
2 IL-2/IL-2R signaling
2.1 IL-2 dependent IL-2R signaling
IL-2 is a 4-bundle α-helical protein of 15 kDa, produced by antigen-activated CD4+ T cells through the T cell receptor (TCR) and CD28 co-stimulation. CD8+ T cells, natural killer (NK) cells, natural killer T (NKT) cells and dendritic cells (DCs) also secrete IL-2, but in lower quantities. IL-2 regulates immune responses via binding to IL-2 receptor (IL-2R). IL-2R has three subunits, including IL-2Rα (CD25), IL-2Rβ (CD122) and γc (CD132), which can form high-affinity, intermediate-affinity and low-affinity IL-2R by different combinations (6). High-affinity IL-2R (Kd∼10–11M) has three subunits IL-2Rα, IL-2Rβ and γc. Intermediate-affinity IL-2R (Kd ∼10–9M) comprises of two subunits IL-2Rβ and γc. Low-affinity IL-2R (Kd ∼10–8M) contains only IL-2Rα. When IL-2Rα and IL-2Rβ are co-expressed without γc, there is “pseudo-high affinity” binding (Kd ∼ 10–10M), but no signaling occurs (7, 8). The functional receptors are intermediate-affinity IL-2R and high-affinity IL-2R. Intermediate-affinity receptors are expressed by resting T and NK cells, whereas high-affinity receptors are present on activated lymphocytes (7). After IL-2 bind to intermediate-affinity IL-2R, high-affinity receptors are formed by the rapid induction of IL-2Rα, increasing responsiveness to IL-2. Major downstream signaling pathways of IL-2 receptor include STAT5, PI3K and MAPK pathways (9), as shown in Figure 1. The combination of IL-2 and IL-2R can recruit JAK1 and JAK3 to the receptor complex, leading to the phosphorylation and activation of STAT5. Activated STAT5 can migrate to the nucleus and promote the transcription of vital genes such as Foxp3 (10), Blimp1 (11, 12) and Bcl-6 (11, 13) to regulate immune cell differentiation. After stimulated by IL-2, the PI3K-Akt pathway can facilitate cell growth and survival by suppressing exhaustion and increasing metabolism (14, 15). The MAPK pathway mainly plays an important role in the process of cell proliferation and function (16).
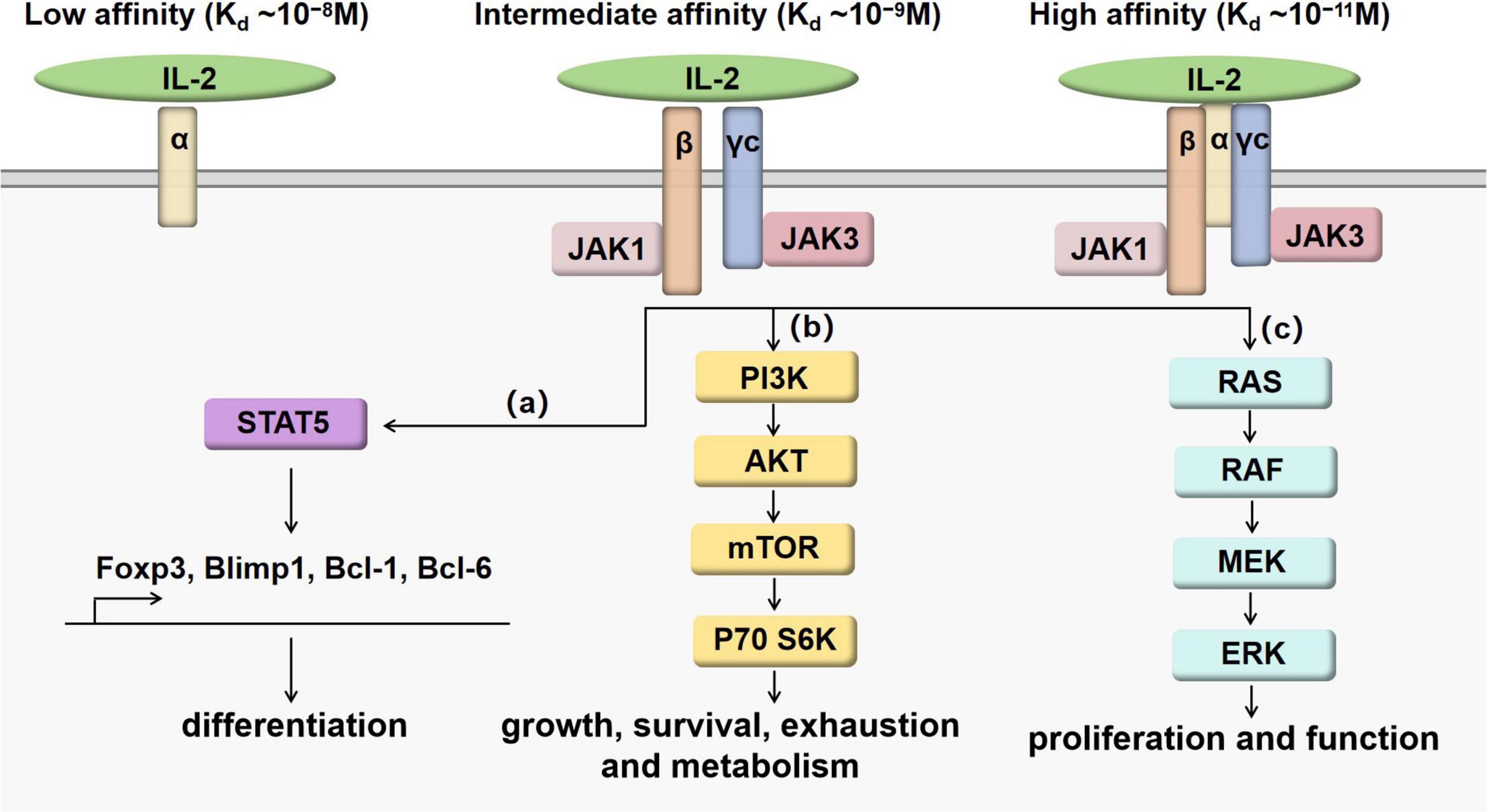
Figure 1. IL-2/IL-2R signaling pathways. When IL-2 binds with intermediate-affinity IL-2R or high-affinity IL-2R, the formation of cytokine-receptor complex leads to the activation of JAK1 next to IL-2Rβ and JAK3 next to γc. Although the binding affinity can be increased by IL-2Rα, IL-2Rα does not work as the signaling subunit. Downstream signaling pathways are presented as (a–c). (a) IL- 2 promotes the phosphorylation of STAT5 affecting the expression of Foxp3, Blimp1, Bcl-1 and Bcl-6 to regulate the differentiation and maturation of T cell subsets. (b) IL- 2 promotes the activation of PI3K-AKT-mTOR pathway to regulate T cell growth, survival, exhaustion and metabolism. (c) IL- 2 promotes the activation of MAPK pathways to regulate T cell proliferation and function.
2.2 IL-2 independent IL-2R signaling
IL-15 belongs to the IL-2 cytokine family, mainly produced by DCs and monocytes/macrophages. IL-15R is a heterotrimeric receptor, including γc, IL-2Rβ and IL-15Rα. IL-15Rα is predominantly expressed on monocytes and DCs, which binds with IL-15 with high affinity (Kd∼10−11 M). It can trigger intracellular signaling pathways following binding with IL-15 (17, 18). IL-15 shares many biological activities with IL-2, including the stimulation of activated T cell proliferation and the generation of cytotoxic effector T cells. Despite these similarities, IL-15 and IL-2 have distinct functions, IL-15 is required for the activation and maintenance of NK cells, the persistence of memory CD8+ T cell, the promotion of immunoglobulin synthesis by B cells, as well as the regulation of lymphoid homeostasis (19, 20). By contrast, IL-2 is the major force for the development and maintenance of Treg cells (21).
3 Regulation of immune cells
3.1 Regulation of innate immune cells
Activated monocytes and macrophages constitutively express IL-2Rβ, which is regulated by IL-2 and IFN-γ (22, 23). IL-2 can increase the cytotoxicity of macrophages and induce activated macrophage to be resistant to infection (24, 25). It is speculated that IL-2R can absorb IL-2 during inflammation to influence macrophage differentiation and restrain T cell expansion and function through this competitive binding (26). DCs can express all three subunits of IL-2R. IL-2 can stimulate DC formation and substantially expand type 1 DCs which improve anti-cancer immunity (27, 28). NK cells do not express IL-2Rα, but constitutively express IL-2Rβ. The majority of IL-2R presented on NK cell surface is low-affinity dimerized complex (27). It is found that IL-2 promotes the cytotoxicity of NK cells in a dose dependent manner (29). Innate lymphoid cells (ILCs) are a population of lymphocytes that distribute in all organs serving as frontline immune guard. IL-2 can efficiently promote the proliferation and survival of group 2 innate lymphoid cells (ILC2s) (30). IL-2 enhances the effector function of ILC2s by stimulating the cytokine production such as IL-5, IL-10 and IL-13 (27).
3.2 Regulation of adaptive immune cells
IL-2 can promote the differentiation of Th1, Th2, Th9, and Treg cells (31–36), but suppress the generation of Th17 (37, 38) and Tfh cells (39–41). During Th1 cell differentiation, IL-2 can induce IFN-γ production and increase expression of T-bet (42). Enhanced mTORC1 signaling by IL-2 can positively influence the differentiation of Th1 cells (40). IL-2 can control Th2 cell fate via the transcription factor STAT5 by regulating the Th2 cytokine gene cluster and stabilizing the accessibility of Il4ra, the gene encoding the IL-4 receptor α-chain (31–33). Th9 cell differentiation is controlled by the competition between IL-2 and IL-21, by influencing STAT5 binding site at the Il9 gene. IL-2 can cut down the expression of Bcl-6, while the increased Bcl-6 expression can inhibit IL-9 production. IL-21 has opposing actions (43). IL-2 can stabilize Foxp3 expression to maintain lineage identity of Treg (34). It is required for the suppressive function and the thymic development of Treg (35, 36). IL-2 can inhibit CD4+ T cells from polarization into Th17 and Tfh cells by reciprocal actions of STAT3 and STAT5, partly contributing to enhanced differentiation of Th1 cells (37, 41). Intriguingly, after Th17 cells establish their identity, IL-2 will promote the proliferation of these cells (44). In addition, IL-2 is shown to inhibit germinal center (GC) formation. There are impaired influenza-specific GCs, long-lived IgG responses with IL-2 administration (39).
Apart from regulating the differentiation of CD4+T cells, IL-2 is also important for CD8+ T cells to regulate their effector function and memory formation, depending on their IL-2Rα expression. After viral infection, CD8+ T cells with high levels of IL-2Rα tend to become terminally differentiated, short-lived effector cells and express cytolytic molecules and cytokines, mediated by Blimp1 induction. While those with low levels of IL-2Rα prefer to increase Bcl-6, IL-7Rα and CD62L expression and preferentially turn to memory cells (41, 45). Besides, exhausted CD8+ T cells express higher levels of IL-2Rβ. Downregulation of IL-2Rβ leads to the reverse of exhausted phenotype, suggesting its the ability to rescue the exhausted state (46).
3.3 Regulation of engineered T cells
Adoptive transfer of engineered T cells has emerged as a potential therapeutic strategy for autoimmune diseases. These genetically modified autologous T cells were initially designed for hematological malignancies. Honaker et al. used homology-directed repair (HDR)-based gene editing to permit thymic T cells to express Foxp3 stably and robustly. These edited thymic Tregs can mediated suppressive activities in the models of inflammatory disease (47). Cook et Al. developed a gene-editing strategy to transform peripheral blood CD4+ T cells into Treg-like cells through the site-specific integration of a strong promoter upstream of the Foxp3 gene. These edited cells also showed immuno-suppressive function (48). It is suggested that engineered Treg cells may exhibit therapeutic potential in a variety of autoimmune disorders (47, 49). However, the deficiency of appropriate cytokine support can lead to poor engraftment of the transferred T cells. It is reported that orthogonal IL-2 and IL-2Rβ system can increase the expansion of engineered T cells. This expansion is selective towards orthogonal IL-2Rβ expressing cells with no appreciable signaling on wild-type T cells. Orthogonal IL-2 can induce IL-2R signaling and support the proliferation of both an IL-2-dependent cell line and primary T cells transduced to express orthogonal IL-2Rβ. These data highlight the potential of combining an orthogonal cytokine approach with T cell-based immunotherapies to enhance the therapeutic efficacy of engineered T cells (50). Besides, the orthogonal IL-2/IL-2Rβ system may be able to eliminate the need for lympho-depleting chemotherapy that is usually used as preconditioning before engineered T cell therapy (51). These proofs showed the potential utility of IL-2/IL-2Rβ system for selective expansion of engineered T cells in autoimmune disorders (52).
4 High-dose or low-dose IL-2
Most clinical studies use the human recombinant IL-2 analog aldesleukin, which has a similar biological activity and a nearly identical biochemical structure compared to the native human IL-2 protein. High-dose IL-2 often uses over 100 million IU per day, while low-dose IL-2 therapy uses 0.3–3 million IU per day. High-dose IL-2 is an FDA approved drug for the treatment of metastatic melanoma and is associated with durable responses in a subset of patients, making it an option for the patients who do not respond to other therapeutic strategies (53). It is reported that high-dose IL-2 can make the stimulatory effects on Treg cells, which induce effector CD8+ T cell differentiation and increase the pool of self-reactive CD8+ T cells (54). A high dose of IL-2 therapy can cause Treg expansion in mice, which dampens immune responses against self-antigens including certain tumor antigens and renders mice susceptible to CD8+ T cell-mediated experimental autoimmune diabetes. In addition, high-dose IL-2 has considerable side effects such as vascular leak syndrome, and usually shows significant toxicity, which limit its efficacy. So high-dose IL-2 should be used with careful patient screening and treatment in malignant conditions, which is not justifiable and effective to applied in autoimmune diseases.
Low-dose IL-2 is more suitable for autoimmune diseases (55). Low-dose IL-2 therapy mainly activates Treg cells that express extensive level of high-affinity IL-2 receptor subunit IL-2Rα. It is reported that low-dose IL-2 treatment promotes Treg cells as well as inhibits Th17 cells and Tfh cells, accompanied by marked reduction of disease activity in patients with SLE (56). Moreover, low-dose IL-2 also sustains cellular immunity with enhanced NK cells (5). The benefit of low-dose IL-2 therapy is seen to be dependent on the expansion of immune tolerance-inducing Treg cells as well as the suppression of Teff cells, including Th17 cells and Tfh cells, contributing to the restoration of immune homeostasis and improvement of autoimmune inflammatory conditions.
5 Low-dose IL-2 and combination therapy in SLE
By searching the ClinicalTrials.gov database for the terms “IL-2” and “systemic lupus erythematosus,” we retrieved 18 clinical trials fulfilled our inclusion criteria. We summarize the available clinical results from early-stage to phase 3 clinical trials in Table 1. In order to avoid the side effects of IL-2, there are some engineered variants of IL-2-based therapeutics, such as IL-2 muteins and fusion proteins (57). Mutated forms of IL-2 are mainly receptor-biased IL-2, to make IL-2 prefer to bind to CD25 or CD122. CD25-biased IL-2 can selectively stimulate Treg cells, which is more suitable for SLE therapy. There are some approaches to selectively increase Treg cell expansion and induce the toxicity of IL-2, including PEGylated IL-2 and IL-2 fusion protein. PEGylated IL-2, including NKTR-358 (58, 59), STK-012 (60), and Dual-31/51-20K (61), is a mutated IL-2 which is permanently linked to PEG. It can obstruct the CD122 binding site and thus induce a CD25 bias. In addition, the linked PEG carrier protein can make IL-2 slowly released in vivo, which increases the half-life of IL-2. IL-2 fusion protein, including BAY 50-4798 (62), NHS-IL2 (63, 64), IL233 (65), AMG592 (66), and PT101 (67), is IL-2 mutein with reducing affinity for CD122. BAY 50-4798 has N88R mutation. NHS-IL2 is IL-2 coupled to anti-DNA-histone complex antibody. IL233 is formed by the fusion of the C-terminus (aa109-aa266) of IL-2 and IL-33 molecules. AMG592 is the fusion of IL-2 and Fc to enhance selectivity towards CD25 and extend the half-life of IL-2. PT101 is also Fc fused IL-2 mutein. Currently, NKTR-358, Dual-31/51-20K, AMG592, PT101 and IL233 are in the clinical trial of SLE.
5.1 Low-dose IL-2 alone
Compared to health controls, SLE patients have decreased Treg and Tfr cells as well as increased Tfh cells, while Th17 cells show no significantly difference between two groups, suggesting that Treg cells play a predominate role in the pathology of SLE. Active patients have a higher ratio of activated Tfh/Tfr cells compared to inactive patients. Furthermore, serum IL-2R level is associated with SLEDAI-2K, anti-dsDNA, C3, ferritin level, CRP, urine sediments and leukocyturia, suggesting its promising potential for SLE activity evaluation (68). After low-dose IL-2 therapy, there is a fourfold increase in circulating Treg cells, whereas Th17 cells are increased slightly in SLE patients (69). In refractory SLE, there are increased Th17 and Treg cells and decreased Th17/Treg ratio after IL-2 administration with markedly reduced clinical symptoms. The urine of discharged patients with lupus nephritis was negative after 3 weeks (70). The loci of Foxp3 at STAT binding sites are marked by bivalent histone modifications. After low-dose IL-2 stimulation, STAT3 and STAT5 selectively bind on Foxp3 and Bcl-6 gene loci accompanied by suppressing H3K27me3, leading to the conversation of memory Tfh to functional Tfr cells and the decrease of plasmablasts (71, 72). It is suggested that low-dose IL-2 is conducive to the formation of immune tolerance, the recovery of immune function and the relieve of clinical symptoms without increasing side effects, worthy of clinical application.
5.2 Low-dose IL-2 combined with rapamycin
Rapamycin acting as a cell cycle inhibitor works on mTOR, which is a downstream target of the PI3K pathway. The use of rapamycin can inhibit the proliferation of contaminating conventional effector cells, facilitate the growth of Treg cells in vitro (73), and promote engraftment and survival of engineered Treg cells in vivo (48). Recent studies have shown that rapamycin is effective in reducing disease activity of SLE with limited side effects (74). Low- dose IL- 2 can selectively enhance Treg cells function and rapamycin can promote the proliferation of Treg cells, the combination of the low- dose IL- 2 and rapamycin has been considered to be a good pair of partner. It is reported that low- dose IL- 2 combined with rapamycin results in an increase in the absolute counts of Treg cells in refractory SLE patients, 26.2% patients with refractory SLE ware improved (75, 76). Addition of rapamycin to cultures containing IL-2 further increases the frequency and absolute number of functional Treg. This increase is not due to selective proliferation of Foxp3+ cells. Instead, it is resulted from an increase in the frequency of Foxp3+ cells induced in G0 phase through delayed activation, while the presence of IL-2 promotes survival and proliferation of the Foxp3+ population (77). Thus, low-dose IL-2 combined with rapamycin may provide a better curative effect for SLE patients by promoting induction, survival and expansion of functional Treg. Besides, the combination of low-dose IL-2 and rapamycin could reduce the dosage of prednison (76). It is beneficial for the SLE patients with serious adverse reactions to the use of corticosteroids.
5.3 Low-dose IL-2 combined with tocilizumab
Tocilizumab is a humanized IgG1 monoclonal antibody targeting IL-6. It is reported that two SLE patients with persistent high-grade fever regain normal body temperatures after treatment with tocilizumab, and other symptoms such as arthralgia gradually are relieved as well (78). Although the observed clinical responses are promising, neutropenia may limit the maximum dosage of tocilizumab in patients with SLE (79). It is necessary to explore the combination therapy of tocilizumab to improve its efficacy. It is found that low doses of IL-2 combined with tocilizumab are safe and effective in rheumatoid arthritis (RA) patients (80). The results of this study show that low-dose IL-2 combined with tocilizumab treatment can reduce arthritis symptoms in RA patients, promote the more stable proliferation of Tregs, and suppress the proliferation of Th2, Th17, and Th17/Treg caused by IL-2 treatment alone, suggesting its great value for patients with immune disorders mediated by high levels of Th2 and Th17. Besides, the CRP levels were greatly decreased after the treatment of low-dose IL-2 and tocilizumab, compared to using IL-2 alone. Thus, low-dose IL-2 accompanied with tocilizumab may be an alternative treatment for the patients in the active stage of SLE who fail to respond to initial antibiotics and high-dose glucocorticoids. It is also benefical for the patients who have low Treg cells along with high Th cell. Combined tocilizumab can effectively reverse reduced Treg cells and increased Th cells at the same time contributing to rebuild immune homeostasis.
5.4 Low-dose IL-2 combined with rituximab
Rituximab is a humanized monoclonal antibody targeting CD20 on B cells. The treatment of rituximab can greatly prevent B cells reproduction. The evidences show that rituximab is effective and safe in the treatment of SLE, especially refractory SLE (81, 82). It is indicated that rituximab can improve the symptoms of the SLE patients with neuropsychiatric involvement and reduce the oral steroid dose (83). A recent case report showed that IL-2 plus rituximab can rapidly relieve the symptoms of SLE, such as fever, rash and mucosal ulcer (84). Apart from rituximab, belimumab which also targets B cell has shown its efficacy in the treatment of SLE (85). Belimumab can inhibit the B cell activating factor (BAFF) to decrease the production of autoantibodies. Though there is no clinical trials using IL-2 accompanied with belimumab. It still provides a novel strategy for the IL-2 combination therapy.
The main features of the above therapies are summarized in Table 2.
6 SLE therapy evaluation
To date, there is no universal agreement regarding the optimal tools to assess SLE. The assessment of patients with lupus usually relies on five domains: disease activity, chronic damage resulting from lupus activity or its treatment, unwanted side effects of drugs, health-related quality of life, and economic impact. The primary disease activity instruments are systemic lupus erythematosus disease activity index (SLEDAI), British Isles lupus assessment group index (BILAG), and physician global assessment (PGA) (91). Recently, Diogo Jesus et al. develop a new SLE disease activity score system (SLE-DAS) based on multivariate linear regression analysis with improved sensitivity to the changes in SLE disease activity and higher accuracy of damage prediction (92). As trials are conducted in different individuals with different outcome measures assessed at different time points and different dosing schemes with or without other agents. A conclusive statement on the IL-2 therapy is not possible. But we can assess the efficiency, safety and indication of IL-2 therapy according to the existing evidence.
The results show that compared to the placebo group, SLE patients with active disease improve rapidly and significantly with low-dose IL-2 treatment. Higher proportions of Treg cells, reduced SLEDAI scores and resolution of clinical features are observed, along with decreased serological activities such as reduced autoantibodies and increased serum complements. Different from immunosuppressants and biologics which often increased infection incidence, low-dose IL-2 treatment is effective in SLE without increased infection incidence (5, 93). A common and typical dermatological change at the site of injection is characterized by pain, redness and swelling. This injection site reaction occurred in 33.1% of patients with SLE. A common systemic effect is fever, occurring in 14.4% SLE patients treated with low-dose IL- 2. Transient influenza-like symptoms occurred in 10.3% of patients with SLE. Most of these side effects are mild and recover quickly after discontinuation. There are no serious adverse events in all these studies, including neuropsychiatric manifestations of SLE (NPSLE), herpeszoster and pneumonia (94).
The difficulty of immunotherapy is to find the patients who can benefit from immunotherapy. Selecting appropriate predictive biomarkers is a high priority to distinguish patients who are more likely to respond to IL-2 treatment. We can take advantage of hypothesis-generating data to support clinical trials involving single agents and combinations. Gao et al. develop a mathematical model to find an effective range of IL-2 dosage which is defined by the ratio of CD4+Foxp3– T and CD4+CD25+Foxp3+ Treg cells. They find that SLE patients with lower Treg cells are more likely to benefit from IL-2 treatment (95, 96).
7 Conclusion and perspective
Low-dose IL-2 treatment has great potential as a novel strategy to rebuild the immune homestasis in individuals with SLE. An emerging number of evidence from the clinical trials demonstrated the profound safety and efficacy of low-dose IL-2 in controlling the disease flares in SLE patients. Even refractory symptoms showed improvement. In addition, IL-2 can be utilized with other agents, such as rapamycin, tocilizumab and rituximab, to design a more accurate and personalized treatment plan. However, there are still a few points to be noted. Firstly, current IL-2 treatment is not cell-specific, which may lead to side effects. So it is better to redirect the specificity of IL-2 toward adoptively transferred T cells based on orthogonal IL-2/IL-2Rβ system that can afford precise targeting of IL-2–dependent functions to a specific cell type of interest. For example, targeting cytotoxic CD8+ T and NK cells in anti-cancer treatment, but stimulating Tregs other than effector cells in the treatment of autoimmune diseases. Secondly, due to its short half-life (15–30 min), IL-2 requires frequent applications. The injection frequency is usually once a day, which will increase the pain of patients. It is necessary to modify IL-2 to extend its half-life or change the mode of administration. For example, IL-2 has a molecular weight of 15 kd, which can be absorbed through the skin or nose. It is possible to design transdermal or transmucosal absorption dosage form. Thirdly, future studies need to consider how to find appropriate predictive biomarkers to indicate patients who is susceptible to IL-2 treatment which will improve the efficacy of IL-2 therapy in clinical application. Lastly, it is advisable to conduct more large-scale, multi-center clinical trials to explore its optimal dose and long-term implications, and to study the possibility of combining IL-2 with other novel immunotherapy methods such as engineered T cells.
Author contributions
XX: Writing – original draft, Writing – review & editing. RQ: Writing – review & editing.
Funding
The author(s) declare that financial support was received for the research and/or publication of this article. This work was supported by the National Natural Science Foundation of China (grant no. 12302012).
Conflict of interest
The authors declare that the research was conducted in the absence of any commercial or financial relationships that could be construed as a potential conflict of interest.
Publisher’s note
All claims expressed in this article are solely those of the authors and do not necessarily represent those of their affiliated organizations, or those of the publisher, the editors and the reviewers. Any product that may be evaluated in this article, or claim that may be made by its manufacturer, is not guaranteed or endorsed by the publisher.
Abbreviations
ALT, alanine aminotransferase; AKT, protein kinase B; Anti-VEGF, anti-vascular endothelial growth factor; BAFF, B cell activating factor; Bcl-1, B-cell lymphoma 1 protein; Bcl-6, B-cell lymphoma 6 protein; BILAG, British Isles lupusassessment group index; Blimp1, B lymphocyte-in-duced maturation protein-1; C3, complement protein 3; CD62L, lymphocyte adhesion molecule 1; CRP, C-reactive protein; DC cell, dendritic cell; dsDNA, double-stranded DNA; FDA, Food and Drug Administration; Foxp3, Forkhead box protein P3; GC, Germinal center; IL-2, Interleukin-2; IL-2R, IL-2 receptor; IL-5, Interleukin-5; IL-6, Interleukin-6; IL-10, Interleukin-10; IL-13, Interleukin-13; ILCs, Innate lymphoid cells; ILC2s, Group 2 innate lymphoid cells; JAK1, Janus kinase 1; JAK3, Janus kinase 3; MAPK, Mitogen activated protein kinase; mTOR, Mammalian target of rapamycin; NK cell, Natural killer cell; NKT cell, Natural killer T cell; NPSLE, Neuropsychiatric systemic lupus erythematosus; PBMCs, Peripheral blood mononuclear cells; PGA, Physician global assessment; PI3K, Phosphatidylinositide 3-kinase; RA, Rheumatoid arthritis; SLE, Systemic lupus erythematosus; STAT5, Signal transducer and activator of transcription 5; STAT3, Signal transducer and activator of transcription 3; SLEDAI-2K, Systemiclupus erythematosus disease activity index 2000; TCR, T cell receptor; Teff cell, Effector T cell; Tfh cell, Follicular helper T cell; Tfr cell, Follicular helper regulatory T cell; Th cell, Helper T cell; Treg cell, regulatory T cell.
References
1. Rosenberg S, Lotze M, Muul L, Leitman S, Chang A, Ettinghausen S, et al. Observations on the systemic administration of autologous lymphokine-activated killer cells and recombinant interleukin-2 to patients with metastatic cancer. N Engl J Med. (1985) 313:1485–92. doi: 10.1056/NEJM198512053132327
2. Rosenberg S. IL-2: The first effective immunotherapy for human cancer. J Immunol. (2014) 192:5451–8. doi: 10.4049/jimmunol.1490019
3. Hernandez R, Põder J, LaPorte K, Malek T. Engineering IL-2 for immunotherapy of autoimmunity and cancer. Nat Rev Immunol. (2022) 22:614–28. doi: 10.1038/s41577-022-00680-w
4. Malek T. The biology of interleukin-2. Annu Rev Immunol. (2008) 26:453–79. doi: 10.1146/annurev.immunol.26.021607.090357
5. He J, Zhang R, Shao M, Zhao X, Miao M, Chen J, et al. Efficacy and safety of low-dose IL-2 in the treatment of systemic lupus erythematosus: A randomised, double-blind, placebo-controlled trial. Ann Rheum Dis. (2020) 79:141–9. doi: 10.1136/annrheumdis-2019-215396
6. Malek T, Castro I. Interleukin-2 receptor signaling: At the interface between tolerance and immunity. Immunity. (2010) 33:153–65. doi: 10.1016/j.immuni.2010.08.004
7. Liao W, Lin J, Leonard W. Interleukin-2 at the crossroads of effector responses, tolerance, and immunotherapy. Immunity. (2013) 38:13–25. doi: 10.1016/j.immuni.2013.01.004
8. Arima N, Kamio M, Imada K, Hori T, Hattori T, Tsudo M, et al. Pseudo-high affinity interleukin 2 (IL-2) receptor lacks the third component that is essential for functional IL-2 binding and signaling. J Exp Med. (1992) 176:1265–72. doi: 10.1084/jem.176.5.1265
9. Spolski R, Li P, Leonard W. Biology and regulation of IL-2: From molecular mechanisms to human therapy. Nat Rev Immunol. (2018) 18:648–59. doi: 10.1038/s41577-018-0046-y
10. Yi Z, Lin W, Stunz L, Bishop G. The adaptor TRAF3 restrains the lineage determination of thymic regulatory T cells by modulating signaling via the receptor for IL-2. Nat Immunol. (2014) 15:866–74. doi: 10.1038/ni.2944
11. Sun Q, Cai D, Liu D, Zhao X, Li R, Xu W, et al. BCL6 promotes a stem-like CD8+ T cell program in cancer via antagonizing BLIMP1. Sci Immunol. (2023) 8:eadh1306. doi: 10.1126/sciimmunol.adh1306
12. Bordon Y. STAT5 reins in the follicular helpers. Nat Rev Immunol. (2012) 12:152. doi: 10.1038/nri3170
13. Dubois S, Miljkovic MD, Fleisher T, Hsu J, Waldmann TA, Conlon KC. Transient inhibition of the JAK1/3-stat5 pathway by ruxolitinib is insufficient to produce clinical benefit in patients with indolent adult T-cell leukemia. Blood. (2017) 130(Suppl 1):3840. doi: 10.1182/blood.V130.Suppl_1.3840.3840
14. Fung M, Rohwer F, McGuire KL. IL-2 activation of a PI3K-dependent STAT3 serine phosphorylation pathway in primary human T cells. Cell Signal. (2003) 15:625–36. doi: 10.1016/s0898-6568(03)00003-2
15. Vargas-Hernandez A, Satter LF. Role of Src-PI3K signaling activation in regulation of STAT5b-deficient NK cell function in response to IL-2. Clin. Immunol. (2023) 250(Suppl):109370. doi: 10.1016/j.clim.2023.109370
16. Mu P, Huo J, Li X, Li W, Li X, Ao J, et al. IL-2 signaling couples the MAPK and mTORC1 axes to promote T cell proliferation and differentiation in teleosts. J Immunol. (2022) 208:1616–31. doi: 10.4049/jimmunol.2100764
17. Sim G, Radvanyi L. The IL-2 cytokine family in cancer immunotherapy. Cytokine Growth Factor Rev. (2014) 25:377–90. doi: 10.1016/j.cytogfr.2014.07.018
18. Goleij P, Rahimi M, Pourshahroudi M, Tabari M, Muhammad S, Suteja R, et al. The role of IL-2 cytokine family in asthma. Cytokine. (2024) 180:156638. doi: 10.1016/j.cyto.2024.156638
19. Waldmann T. The biology of interleukin-2 and interleukin-15: Implications for cancer therapy and vaccine design. Nat Rev Immunol. (2006) 6:595–601. doi: 10.1038/nri1901
20. Yang Y, Lundqvist A. Immunomodulatory effects of IL-2 and IL-15; Implications for cancer immunotherapy. Cancers. (2020) 12:3586. doi: 10.3390/cancers12123586
21. Read K, Powell M, McDonald P, Oestreich K. IL-2, IL-7, and IL-15: Multistage regulators of CD4(+) T helper cell differentiation. Exp Hematol. (2016) 44:799–808. doi: 10.1016/j.exphem.2016.06.003
22. Bosco M, Espinoza-Delgado I, Schwabe M, Gusella G, Longo D, Sugamura K, et al. Regulation by interleukin-2 (IL-2) and interferon gamma of IL-2 receptor gamma chain gene expression in human monocytes. Blood. (1994) 83:2995–3002. doi: 10.1182/blood.V83.10.2995.2995
23. Scheibenbogen C, Keilholz U, Richter M, Andreesen R, Hunstein W. The interleukin-2 receptor in human monocytes and macrophages: Regulation of expression and release of the alpha and beta chains (p55 and p75). Res Immunol. (1992) 143:33–7. doi: 10.1016/0923-2494(92)80077-x
24. Belosevic M, Finbloom D, Meltzer M, Nacy C. IL-2. A cofactor for induction of activated macrophage resistance to infection. J Immunol. (1990) 145:831–9.
25. Hancock W, Muller W, Cotran R. Interleukin 2 receptors are expressed by alveolar macrophages during pulmonary sarcoidosis and are inducible by lymphokine treatment of normal human lung macrophages, blood monocytes, and monocyte cell lines. J Immunol. (1987) 138:185–91. doi: 10.4049/jimmunol.138.1.185
26. Herrmann F, Cannistra S, Levine H, Griffin J. Expression of interleukin 2 receptors and binding of interleukin 2 by gamma interferon-induced human leukemic and normal monocytic cells. J Exp Med. (1985) 162:1111–6. doi: 10.1084/jem.162.3.1111
27. Zhou P. Emerging mechanisms and applications of low-dose IL-2 therapy in autoimmunity. Cytokine Growth Factor Rev. (2022) 67:80–8. doi: 10.1016/j.cytogfr.2022.06.003
28. Raeber M, Rosalia R, Schmid D, Karakus U, Boyman O. Interleukin-2 signals converge in a lymphoid-dendritic cell pathway that promotes anticancer immunity. Sci Transl Med. (2020) 12:eaba5464. doi: 10.1126/scitranslmed.aba5464
29. Domzig W, Stadler B, Herberman R. Interleukin 2 dependence of human natural killer (NK) cell activity. J Immunol. (1983) 130:1970–3.
30. Roediger B, Kyle R, Tay S, Mitchell A, Bolton H, Guy T, et al. IL-2 is a critical regulator of group 2 innate lymphoid cell function during pulmonary inflammation. J Allergy Clin Immunol. (2015) 136:1653–63.e7. doi: 10.1016/j.jaci.2015.03.043
31. Cote-Sierra J, Foucras G, Guo L, Chiodetti L, Young H, Hu-Li J, et al. Interleukin 2 plays a central role in Th2 differentiation. Proc Natl Acad Sci U S A. (2004) 101:3880–5. doi: 10.1073/pnas.0400339101
32. Liao W, Lin J, Wang L, Li P, Leonard W. Modulation of cytokine receptors by IL-2 broadly regulates differentiation into helper T cell lineages. Nat Immunol. (2011) 12:551–9. doi: 10.1038/ni.2030
33. Liao W, Schones D, Oh J, Cui Y, Cui K, Roh T, et al. Priming for T helper type 2 differentiation by interleukin 2-mediated induction of interleukin 4 receptor alpha-chain expression. Nat Immunol. (2008) 9:1288–96. doi: 10.1038/ni.1656
34. Feng Y, Arvey A, Chinen T, van der Veeken J, Gasteiger G, Rudensky A. Control of the inheritance of regulatory T cell identity by a cis element in the Foxp3 locus. Cell. (2014) 158:749–63. doi: 10.1016/j.cell.2014.07.031
35. Bayer A, Yu A, Malek T. Function of the IL-2R for thymic and peripheral CD4+CD25+ Foxp3+ T regulatory cells. J Immunol. (2007) 178:4062–71. doi: 10.4049/jimmunol.178.7.4062
36. Brandenburg S, Takahashi T, de la Rosa M, Janke M, Karsten G, Muzzulini T, et al. IL-2 induces in vivo suppression by CD4(+)CD25(+)Foxp3(+) regulatory T cells. Eur J Immunol. (2008) 38:1643–53. doi: 10.1002/eji.200737791
37. Laurence A, Tato C, Davidson T, Kanno Y, Chen Z, Yao Z, et al. Interleukin-2 signaling via STAT5 constrains T helper 17 cell generation. Immunity. (2007) 26:371–81. doi: 10.1016/j.immuni.2007.02.009
38. Yang X, Ghoreschi K, Steward-Tharp S, Rodriguez-Canales J, Zhu J, Grainger J, et al. Opposing regulation of the locus encoding IL-17 through direct, reciprocal actions of STAT3 and STAT5. Nat Immunol. (2011) 12:247–54. doi: 10.1038/ni.1995
39. Ballesteros-Tato A, León B, Graf B, Moquin A, Adams P, Lund F, et al. Interleukin-2 inhibits germinal center formation by limiting T follicular helper cell differentiation. Immunity. (2012) 36:847–56. doi: 10.1016/j.immuni.2012.02.012
40. Ray J, Staron M, Shyer J, Ho P, Marshall H, Gray S, et al. The Interleukin-2-mTORc1 kinase axis defines the signaling, differentiation, and metabolism of T helper 1 and follicular B helper T cells. Immunity. (2015) 43:690–702. doi: 10.1016/j.immuni.2015.08.017
41. Ross S, Cantrell D. Signaling and function of interleukin-2 in T Lymphocytes. Annu Rev Immunol. (2018) 36:411–33. doi: 10.1146/annurev-immunol-042617-053352
42. Boyman O, Sprent J. The role of interleukin-2 during homeostasis and activation of the immune system. Nat Rev Immunol. (2012) 12:180–90. doi: 10.1038/nri3156
43. Liao W, Spolski R, Li P, Du N, West E, Ren M, et al. Opposing actions of IL-2 and IL-21 on Th9 differentiation correlate with their differential regulation of BCL6 expression. Proc Natl Acad Sci U S A. (2014) 111:3508–13. doi: 10.1073/pnas.1301138111
44. Amadi-Obi A, Yu C, Liu X, Mahdi R, Clarke G, Nussenblatt R, et al. TH17 cells contribute to uveitis and scleritis and are expanded by IL-2 and inhibited by IL-27/STAT1. Nat Med. (2007) 13:711–8. doi: 10.1038/nm1585
45. Kalia V, Sarkar S, Subramaniam S, Haining W, Smith K, Ahmed R. Prolonged interleukin-2Ralpha expression on virus-specific CD8+ T cells favors terminal-effector differentiation in vivo. Immunity. (2010) 32:91–103. doi: 10.1016/j.immuni.2009.11.010
46. Beltra J, Bourbonnais S, Bédard N, Charpentier T, Boulangé M, Michaud E, et al. IL2Rβ-dependent signals drive terminal exhaustion and suppress memory development during chronic viral infection. Proc Natl Acad Sci U S A. (2016) 113:E5444–53. doi: 10.1073/pnas.1604256113
47. Honaker Y, Hubbard N, Xiang Y, Fisher L, Hagin D, Sommer K, et al. Gene editing to induce FOXP3 expression in human CD4+ T cells leads to a stable regulatory phenotype and function. Sci Transl Med. (2020) 12:eaay6422. doi: 10.1126/scitranslmed.aay6422
48. Cook P, Yang S, Uenishi G, Grimm A, West S, Wang L, et al. A chemically inducible IL-2 receptor signaling complex allows for effective in vitro and in vivo selection of engineered CD4+ T cells. Mol Ther. (2023) 31:2472–88. doi: 10.1016/j.ymthe.2023.04.021
49. Yang S, Singh A, Drow T, Tappen T, Honaker Y, Barahmand-Pour-Whitman F, et al. Pancreatic islet-specific engineered Tregs exhibit robust antigen-specific and bystander immune suppression in type 1 diabetes models. Sci Transl Med. (2022) 14:eabn1716. doi: 10.1126/scitranslmed.abn1716
50. Zhang Q, Hresko M, Picton L, Su L, Hollander M, Nunez-Cruz S, et al. A human orthogonal IL-2 and IL-2Rβ system enhances CAR T cell expansion and antitumor activity in a murine model of leukemia. Sci Transl Med. (2021) 13:eabg6986. doi: 10.1126/scitranslmed.abg6986
51. Hirayama A, Gauthier J, Hay K, Voutsinas J, Wu Q, Gooley T, et al. The response to lymphodepletion impacts PFS in patients with aggressive non-Hodgkin lymphoma treated with CD19 CAR T cells. Blood. (2019) 133:1876–87. doi: 10.1182/blood-2018-11-887067
52. Sockolosky J, Trotta E, Parisi G, Picton L, Su L, Le A, et al. Selective targeting of engineered T cells using orthogonal IL-2 cytokine-receptor complexes. Science. (2018) 359:1037–42. doi: 10.1126/science.aar3246
53. Marabondo S, Kaufman H. High-dose interleukin-2 (IL-2) for the treatment of melanoma: Safety considerations and future directions. Expert Opin Drug Saf. (2017) 16:1347–57. doi: 10.1080/14740338.2017.1382472
54. Tsyklauri O, Chadimova T, Niederlova V, Kovarova J, Michalik J, Malatova I, et al. Regulatory T cells suppress the formation of potent KLRK1 and IL-7R expressing effector CD8 T cells by limiting IL-2. Elife. (2023) 12:e79342. doi: 10.7554/eLife.79342
55. Klatzmann D, Abbas A. The promise of low-dose interleukin-2 therapy for autoimmune and inflammatory diseases. Nat Rev Immunol. (2015) 15:283–94. doi: 10.1038/nri3823
56. He J, Zhang X, Wei Y, Sun X, Chen Y, Deng J, et al. Low-dose interleukin-2 treatment selectively modulates CD4(+) T cell subsets in patients with systemic lupus erythematosus. Nat Med. (2016) 22:991–3. doi: 10.1038/nm.4148
57. Raeber M, Sahin D, Karakus U, Boyman O. A systematic review of interleukin-2-based immunotherapies in clinical trials for cancer and autoimmune diseases. EBioMedicine. (2023) 90:104539. doi: 10.1016/j.ebiom.2023.104539
58. Dixit N, Fanton C, Langowski J, Kirksey Y, Kirk P, Chang T, et al. NKTR-358: A novel regulatory T-cell stimulator that selectively stimulates expansion and suppressive function of regulatory T cells for the treatment of autoimmune and inflammatory diseases. J Transl Autoimmun. (2021) 4:100103. doi: 10.1016/j.jtauto.2021.100103
59. Fanton C, Dixit N, Siddhanti S, Lu L, Dickerson D, Kotzin B, et al. Selective induction of functional regulatory T-cells in healthy volunteers by NKTR-358, a novel IL-2 conjugate treg stimulator, in development for the treatment of autoimmune diseases. Arthritis Rheumatol. (2019) 71:98.
60. Emmerich J, Bauer M, Semana M, Jayaraman B, Vivona S, McCauley S, et al. STK-012, an alpha/beta selective IL-2 mutein for the activation of antigen-activated T cells in solid tumors. Cancer Res. (2021) 81:1744.
61. Zhang B, Sun J, Wang Y, Ji D, Yuan Y, Li S, et al. Site-specific PEGylation of interleukin-2 enhances immunosuppression via the sustained activation of regulatory T cells. Nat Biomed Eng. (2021) 5:1288–305. doi: 10.1038/s41551-021-00797-8
62. Margolin K, Atkins M, Dutcher J, Ernstoff M, Smith J, Clark J, et al. Phase I trial of BAY 50-4798, an interleukin-2-specific agonist in advanced melanoma and renal cancer. Clin Cancer Res. (2007) 13:3312–9. doi: 10.1158/1078-0432.CCR-06-1341
63. Gillessen S, Gnad-Vogt U, Gallerani E, Beck J, Sessa C, Omlin A, et al. A phase I dose-escalation study of the immunocytokine EMD 521873 (Selectikine) in patients with advanced solid tumours. Eur J Cancer. (2013) 49:35–44. doi: 10.1016/j.ejca.2012.07.015
64. van den Heuvel M, Verheij M, Boshuizen R, Belderbos J, Dingemans A, De Ruysscher D, et al. NHS-IL2 combined with radiotherapy: Preclinical rationale and phase Ib trial results in metastatic non-small cell lung cancer following first-line chemotherapy. J Transl Med. (2015) 13:32. doi: 10.1186/s12967-015-0397-0
65. Stremska M, Jose S, Sabapathy V, Huang L, Bajwa A, Kinsey G, et al. IL233, A novel IL-2 and IL-33 hybrid cytokine, ameliorates renal injury. J Am Soc Nephrol. (2017) 28:2681–93. doi: 10.1681/ASN.2016121272
66. Tchao N, Gorski KS, Yuraszeck T, Sohn SJ, Ishida K, Wong H, et al. Amg 592 is an investigational IL-2 mutein that induces highly selective expansion of regulatory T cells. Blood. (2017) 130(Suppl 1):696. doi: 10.1182/blood.V130.Suppl_1.696.696
67. Sundy JS, Otipoby KL, Higginson-Scott N, Visweswaraiah J, Sampson E, Kis-Toth K, et al. AB0282 safety, tolerability and selective expansion of regulatory t cells by a single dose of the novel il-2 mutein Pt101 in a phase 1 study in healthy volunteers. Ann Rheumat Dis. (2021) 80(Suppl 1):1167. doi: 10.1136/annrheumdis-2021-eular.1200
68. Aristova M, Panafidina TA, Gorbunova Y, Avdeeva A, Popkova T. LP-203 The new markers of systemic lupus erythematosus activity: Focus on interleukin (IL)-1b and soluble IL-2 receptor. Lupus Sci Med. (2023) 10(Suppl 1):A101.2–A102 doi: 10.1136/lupus-2023-KCR.147
69. Liu X, Jing X, Wu X, Wang J, Liang Z, Hao M. OP0043 The number of circulating regulatory t cells is reduced and low-dose il-2 selectively stimulates its proliferation in patients with systemic lupus erythematosus. Ann Rheumatol Dis. (2017) 76:69. doi: 10.1136/annrheumdis-2017-eular.4221
70. Liu XQ, Wu XY, Wang CH, Zhang SX, Li XF. Low-dose IL-2: A novel therapeutic strategy for refractory systemic lupus erythematosus. Int J Rheumat Dis. (2016) 19:283. doi: 10.1111/1756-185X.12962
71. Hao H, Nakayamada S, Kaoru Y, Ohkubo N, Iwata S, Tanaka Y, et al. IL-2 drives the conversion of T follicular helper to T follicularregulatory cells through epigenetic modification in systemic lupus erythematosus. Ann Rheumat Dis. (2020) 79:339. doi: 10.1136/annrheumdis-2020-eular.651
72. Hao H, Nakayamada S, Yamagata K, Ohkubo N, Iwata S, Inoue Y, et al. Conversion of T follicular helper cells to T follicular regulatory cells by interleukin-2 through transcriptional regulation in systemic lupus erythematosus. Arthrit Rheumatol. (2021) 73:132–42. doi: 10.1002/art.41457
73. Kang J, Huddleston S, Fraser J, Khoruts A. De novo induction of antigen-specific CD4+CD25+Foxp3+ regulatory T cells in vivo following systemic antigen administration accompanied by blockade of mTOR. J Leukoc Biol. (2008) 83:1230–9. doi: 10.1189/jlb.1207851
74. Oaks Z, Winans T, Huang N, Banki K, Perl A. Activation of the mechanistic target of rapamycin in SLE: Explosion of evidence in the last five years. Curr Rheumatol Rep. (2016) 18:73. doi: 10.1007/s11926-016-0622-8
75. Jing X, Hao M, Gao C, Li X, Chen J. Low-dose IL-2 combined with rapamycin efficiently promoted disease remission and recovered the balance of Th17/regulatory T cells in patients with refractory systemic lupus erythematosus. Arthritis Rheumatol. (2018) 70:973.
76. Zhao C, Chu Y, Liang Z, Zhang B, Wang X, Jing X, et al. Low dose of IL-2 combined with rapamycin restores and maintains the long-term balance of Th17/Treg cells in refractory SLE patients. BMC Immunol. (2019) 20:32. doi: 10.1186/s12865-019-0305-0
77. Long S, Buckner J. Combination of rapamycin and IL-2 increases de novo induction of human CD4(+)CD25(+)FOXP3(+) T cells. J Autoimmun. (2008) 30(4):293–302. doi: 10.1016/j.jaut.2007.12.012
78. Chaoyi M, Shrestha B, Hui L, Qiujin D, Ping F. Tocilizumab therapy for persistent high-grade fever in systemic lupus erythematosus: Two cases and a literature review. J Int Med Res. (2022) 50:3000605221088558. doi: 10.1177/03000605221088558
79. Illei G, Shirota Y, Yarboro C, Daruwalla J, Tackey E, Takada K, et al. Tocilizumab in systemic lupus erythematosus: Data on safety, preliminary efficacy, and impact on circulating plasma cells from an open-label phase I dosage-escalation study. Arthritis Rheum. (2010) 62:542–52. doi: 10.1002/art.27221
80. Zhang S, Chen H, Wang J, Shao H, Cheng T, Pei R, et al. The efficacy and safety of short-term and low-dose IL-2 combined with tocilizumab to treat rheumatoid arthritis. Front Immunol. (2024) 15:1359041. doi: 10.3389/fimmu.2024.1359041
81. van Schaik M, Arends E, Wetzels M, Kraaij T, Verbruggen S, van der Kooij S, et al. Long-term safety and efficacy of the combination of belimumab and rituximab in the treatment of severe and refractory SLE: A preliminary report. Lupus Sci Med. (2025) 12:e001424. doi: 10.1136/lupus-2024-001424
82. Wilson M, Mahmoud K, Vital EM, Brown S, Yusof Y, Hensor EM, et al. O14 Rituximab objective outcome measures trial in SLE (ROOTS): Outcomes of randomised and rescue rituximab therapy in a double-blind randomised placebo-controlled trial. Lupus Sci Med. (2024) 11(Suppl):1. doi: 10.1136/lupus-2024-el.24
83. David T, Hum RM, Sutton E, Parker E, McCarthy E, Bruce IN, et al. O17 Effectiveness of rituximab in the treatment of neuro-psychiatric SLE: Results from the British isles lupus assessment group biologics register. Rheumatology. (2021) 60(Suppl):1. doi: 10.1093/rheumatology/keab246.016
84. Li M, Jin Y, He J. Purtscher-like retinopathy associated with systemic lupus erythematosus treated with rituximab plus low-dose interleukin-2: A case report. Int J Rheum Dis. (2023) 26(7):1373–6. doi: 10.1111/1756-185X.14623
85. Hernandez-Flórez D, Valor L, del Río T, Nieto JC, Martinez J, Ovalles J, et al. AB0520 Evaluating the effect of belimumab on clinical disease activity and b-cell in patients with systemic lupus erythematosus. Ann Rheum Dis. (2015) 74(Suppl 2):1074. doi: 10.1136/annrheumdis-2015-eular.3328
86. Hannon C, McCourt C, Lima H, Chen S, Bennett C. Interventions for cutaneous disease in systemic lupus erythematosus. Cochrane Database Syst Rev. (2021) 3:CD007478. doi: 10.1002/14651858.CD007478.pub2
87. Liang K, He J, Wei Y, Zeng Q, Gong D, Qin J, et al. Sustained low-dose interleukin-2 therapy alleviates pathogenic humoral immunity via elevating the Tfr/Tfh ratio in lupus. Clin Transl Immunol. (2021) 10:e1293. doi: 10.1002/cti2.1293
88. Rosenzwajg M, Lorenzon R, Cacoub P, Pham H, Pitoiset F, El Soufi K, et al. Immunological and clinical effects of low-dose interleukin-2 across 11 autoimmune diseases in a single, open clinical trial. Ann Rheum Dis. (2019) 78:209–17. doi: 10.1136/annrheumdis-2018-214229
89. Fontenot J, Hoover E, Elder J, Montelaro R. Evaluation of feline immunodeficiency virus and feline leukemia virus transmembrane peptides for serological diagnosis. J Clin Microbiol. (1992) 30:1885–90. doi: 10.1128/jcm.30.7.1885-1890.1992
90. Humrich J, Cacoub P, Rosenzwajg M, Pitoiset F, Pham H, Guidoux J, et al. Low-dose interleukin-2 therapy in active systemic lupus erythematosus (LUPIL-2): A multicentre, double-blind, randomised and placebo-controlled phase II trial. Ann Rheum Dis. (2022) 81:1685–94. doi: 10.1136/ard-2022-222501
91. Banjari M, Touma Z, Gladman D. Improving measures of disease activity in systemic lupus erythematosus. Expert Rev Clin Immunol. (2023) 19:193–202. doi: 10.1080/1744666X.2023.2156339
92. Jesus D, Matos A, Henriques C, Zen M, Larosa M, Iaccarino L, et al. Derivation and validation of the SLE Disease Activity Score (SLE-DAS): A new SLE continuous measure with high sensitivity for changes in disease activity. Ann Rheum Dis. (2019) 78:365–71. doi: 10.1136/annrheumdis-2018-214502
93. Farooq A, Trehan S, Singh G, Arora N, Mehta T, Jain P, et al. A comprehensive review of low-dose interleukin-2 (IL-2) therapy for systemic lupus erythematosus: Mechanisms, efficacy, and clinical applications. Cureus. (2024) 16:e68748. doi: 10.7759/cureus.68748
94. Su Q, Wang X, Li Y, Zhang J, Bai C, Wang X, et al. Efficacy, safety and the lymphocyte subset changes of low-dose IL-2 in patients with autoimmune rheumatic diseases: A systematic review and meta-analysis. Rheumatol Ther. (2024) 11:79–96. doi: 10.1007/s40744-023-00620-7
95. Gao X, He J, Sun X, Li F. Dynamically modeling the effective range of IL-2 dosage in the treatment of systemic lupus erythematosus. IScience. (2022) 25:104911. doi: 10.1016/j.isci.2022.104911
Keywords: interleukin-2, SLE, immunotherapy, engineered T cells, IL-2
Citation: Xia X and Qu R (2025) A glimpse into the application of the immunomodulatory effect of IL-2 in systemic lupus erythematosus. Front. Med. 12:1552473. doi: 10.3389/fmed.2025.1552473
Received: 28 December 2024; Accepted: 07 April 2025;
Published: 23 April 2025.
Edited by:
Cong-Qiu Chu, Oregon Health and Science University, United StatesReviewed by:
José Pablo Romero-López, Universidad Nacional Autónoma de México, MexicoHeyong Wang, Chengdu University of Traditional Chinese Medicine, China
Copyright © 2025 Xia and Qu. This is an open-access article distributed under the terms of the Creative Commons Attribution License (CC BY). The use, distribution or reproduction in other forums is permitted, provided the original author(s) and the copyright owner(s) are credited and that the original publication in this journal is cited, in accordance with accepted academic practice. No use, distribution or reproduction is permitted which does not comply with these terms.
*Correspondence: Xin Xia, MTAwMDAwNTA2NkB1anMuZWR1LmNu; Rui Qu, MTAwMDAwNTI4NkB1anMuZWR1LmNu