- 1Department of Anesthesiology and Perioperative Medicine, General Hospital of Ningxia Medical University, Yinchuan, China
- 2Department of Anesthesiology, People’s Hospital of Ningxia Hui Autonomous Region, Yinchuan, China
Background: Inverse ratio ventilation theoretically increases oxygenation in obese patients. However, it is unknown whether the use of inverse ratio ventilation prolongs the safe apnea time during the induction of anesthesia. The primary objective of our study was to compare the safe apnea time between obese surgical patients receiving inverse ratio ventilation and conventional ratio ventilation during the induction of anesthesia.
Methods: This study is a prospective, randomized controlled trial. Forty obese patients who underwent elective operation under general anesthesia with endotracheal intubation were randomly allocated into the conventional ratio ventilation (CRV) group (n = 20) and inverse ratio ventilation (IRV) group (n = 20). After the patients were preoxygenated through a face mask for 3 min, anesthesia induction was performed. When the patients lost consciousness and spontaneous breathing disappeared, non-invasive positive pressure ventilation was performed for 5 min, and the inspiratory-to-expiratory (I:E) ratio was set as 1:2 in the CRV group and 2:1 in the IRV group. Heart rate, systolic blood pressure, diastolic blood pressure, and pulse oxygen saturation were recorded at four time points: (i) before pre-oxygenation (T0), (ii) pre-oxygenation for 3 min (T1), (iii) non-invasive positive pressure ventilation for 3 min (T2), and (iv) non-invasive positive pressure ventilation for 5 min (T3). Arterial blood was collected at T0, T1, and T3 for arterial blood gas analysis, and arterial oxygen partial pressure and carbon dioxide partial pressure were recorded. The patient’s expiratory oxygen fraction at T1, T2, and T3 were recorded. Peak airway pressure, plateau pressure and mean airway pressure were record at T2 and T3. The safe apnea time was recorded in both groups.
Results: Forty patients completed the study. Baseline parameters were comparable between the two groups. Safe apnea time was significantly longer (210.40 ± 47.47 vs. 153.80 ± 41.54 s, mean difference [95% CI], 56.55 [28.00–85.10], p = 0.0003) and the expired O2 fraction was higher (87.60 ± 2.39 vs. 91.60 ± 1.79, mean difference [95% CI], 4.00 [2.65–5.35], p < 0.0001) at T3 in the IRV group compared to the CRV group.
Conclusion: Volume-controlled inverse ratio ventilation at an I:E ratio of 2:1, compared to conventional ratio ventilation, provided a longer safe apnea time and higher expired O2 fraction in obese patients during the induction of anesthesia.
1 Introduction
Obesity has become one of the major public health problems Its prevalence is alarming (1) and it is associated with numerous comorbidities when obese patients undergo general anesthesia, which include obesity hypoventilation, difficult intubation, obstructive sleep apnea, and other comorbidities (2, 3). Therefore, this raises many concerns and challenges for anesthesiologists. Obese patients are prone to hypoxemia during the induction of general anesthesia due to their altered respiratory mechanics and physiology and changes in respiratory function after anesthesia (4). Their safe apnea time is generally less than 3 min (5, 6), which is significantly shorter than the 8.9 min in healthy people (7). Furthermore, data suggests that there is a negative linear correlation between safe apnea time and increasing obesity (6). The shorter safe apnea time may be related to the decreased functional residual capacity (FRC) and increased oxygen consumption in obese patients (8). Several measures, including head-up (9) or beach-chair positioning (10), non-invasive ventilatory support (5), and high-flow nasal oxygen (11) can improve safe apnea time to varying degrees at present.
In some clinical cases, the anesthesiologist may be required to complete the induction of general anesthesia in an obese patient alone, and both hands have to be used to control the obese patient’s airway. At this time, using mechanical ventilation via mask is feasible and effective to provide respiratory support after the breath disappears. Inverse ratio ventilation (IRV) typically increases oxygenation by increasing the mean airway pressure (MAP), which happens due to more time spent on the higher pressure portion of the cycle (12). Increased MAP can elevate mean alveolar pressure and thus transpulmonary pressure, which can prevent the reduction of FRC, improve alveolar ventilation/blood flow ratio, and finally improve the patient’s oxygenation status (13). Studies have evaluated the effect of IRV in improving oxygenation and gas exchange in obese adult patients (14) and obese children (15) undergoing laparoscopic surgery under general anesthesia and it was demonstrated that IRV can improve oxygenation status significantly compared to the control groups without adverse respiratory and hemodynamic effects. However, it is unclear whether IRV can improve safe apnea time in obese patients during induction of general anesthesia.
We hypothesized that volume-controlled inverse ratio ventilation with an I:E ratio of 2:1 for pre-oxygenation after the breath disappears would result in longer safe apnea time than conventional ratio ventilation with an I:E ratio of 1:2 in obese patients during induction of general anesthesia.
2 Methods
2.1 Study design and randomization
This randomized, double-blind, controlled study was approved by the Institutional Ethics Committee of the General Hospital of Ningxia Medical University (2020-718) in August 2020 and was also conducted at General Hospital of Ningxia Medical University. The study was also registered at ClinicalTrials.gov (NCT04627883) in August 2020. Written informed consent was obtained from all subjects participating in the trial before the surgery. This study was reported in accordance with the CONSORT statement. Forty-eight patients with American Society of Anesthesiologists (ASA) physical status II–III, aged between 18 and 65 years, a body mass index (BMI) ≥ 28 kg · m−2 (Chinese standards for diagnosis of obesity), and a clinical need for endotracheal intubation who were scheduled for elective surgery under general anesthesia were enrolled in this study from August 17, 2020 to January 1, 2022. The exclusion criteria included requiring rapid sequence induction, anticipated or history of difficult airway, uncontrolled gastric reflux disease, moderate to severe comorbidity (respiratory or renal disease, ischemic heart or uncontrolled hypertension or pulmonary hypertension disease, cerebrovascular disease), abnormal basal metabolic rate, and abnormal hemoglobin or hematocrit.
Before patient recruitment, group randomization was performed using an online web-based randomization tool1 with block sizes of six and an allocation ratio of 1:1 by an investigator not involved in the study. Forty-eight eligible participants who underwent elective operation under general anesthesia with endotracheal intubation were randomly allocated into conventional ratio ventilation group (CRV group, n = 24) and inverse ratio ventilation group (IRV group, n = 24) based on the allocation sequence. An anesthetist prepared concealed group assignments in consecutively numbered, sealed, opaque envelopes together with a detailed information of the study ventilation preparation, and then sets ventilation parameters to be adjusted and masked them by a piece of paper. Thereafter, he was no longer involved in the study. All study parameters were observed by another investigator. The randomization was not disclosed to any of the personnel who recorded the study parameters or the patient throughout the study.
2.2 Preparation and anesthesia
All the patients were instructed to fast for at least 8 h and no premedication drugs were given before the induction of anesthesia. After entering the operation room, intravenous access was established in all the patients and they were monitored with an electrocardiogram, non-invasive blood pressure, and pulse oximeter at 20° head-up inclination position. Radial artery was punctured and cannulated under local anesthesia for collecting the arterial blood gases. The inspired O2 concentration was set at 100% and the breathing circuit system was flushed with the O2 bypass for 30 s before being connected to the patients. Pre-oxygenation was performed through a well-fitted facemask firmly applied by four headbands and connected to the anesthesia machine (Fabius GS; Dräger Medical SAS, Antony, France) delivering 10 L · min−1 of 100% oxygen. Both groups adopted the volume-controlled ventilation mode. The ventilator parameters were set as follows: RR represents respiration rate, 12 times · min−1, tidal volume, 8 mL × ideal body weight (IBW), IBW = [50 (men)/45.5 (women) + 0.91 × (height in cm − 152.4 cm)], and the I:E ratio was set 1:2 in CRV group and 2:1 in the IRV group. After pre-oxygenation (breath normally) for 3 min, general anesthesia was induced using midazolam (0.05–0.1 mg · kg−1), propofol (1–2 mg · kg−1), sufentanil (0.3–0.5 ug · kg−1), and rocuronium (0.9 mg · kg−1). A nasopharyngeal airway (the nasal cavity was inducted with topical anesthesia and lubricated before anesthesia) was inserted in all patients, and jaw-thrust was applied when the patient lost consciousness and spontaneous breathing disappeared to ensure patent airway, and mechanical ventilation was initiated via facemask based on the pre-designed ventilation patterns immediately. After 5 min of ventilation, facemask was removed, and tracheal intubation was performed by using a video laryngoscope. Successful intubation was confirmed with capnography (to ventilate approximately 300 mL by using breathing bag). Removing the breathing tube and keeping it disconnected, the safe apnea time, which was defined as the time required for SpO2 to desaturate to 93% measured after the facemask was removed, was recorded, and ventilation was performed after the breathing tube was connected to restore oxygen saturation immediately.
2.3 Data collection
The primary outcome of the study was the safe apnea time in seconds measured after removal of the facemask from patients to the decrease of SpO2 to 93%. The following data were also recorded and analyzed as secondary endpoints: Expired O2 fraction (FeO2) at preoxygenation for 3 min (T1), non-invasive positive pressure ventilation for 3 min (T2) and 5 min (T3); PaO2 and PaCO2 at before preoxygenation(T0), T1 and T3; Ppeak, Pplat, and MAP (MAP = 0.5 (PIP – PEEP) × (Ti/Ttot) + PEEP) at T2 and T3; heart rate (HR), systolic blood pressure (SBP) and diastolic blood pressure (DBP) at T0, T1, T2, and T3.
2.4 Statistical analysis
Sample size estimation was performed using NCSSPASS software (version 11.0.7, update time January 22, 2013). As there was no similar study published, a pilot study was conducted in 12 patients to calculate the sample size. Considering the safe apnea time as the primary outcome in the CRV group (134 ± 37 s) and IRV group (195 ± 16 s) with a confidence interval of 95 and 99%, the minimum required sample size to obtain statistically significant result was calculated to be 20 patients in each group. Finally, we recruited 48 new patients who were equally allocated to the CRV and IRV groups.
Statistical analysis was performed by SPSS (IBM SPSS Statistics, Version 25, IBM Germany, Ehningen, Germany). Data are presented as the mean ± standard deviation (SD) or median (interquartile range, IQR) as required or numbers (n). The normality of quantitative data was tested using the Kolmogorov and Smirnov test. The equality of variance was tested by the Levene test if required. For intergroup comparisons of the parametric continuous variables, Student’s t-test was used. Continuous variables that did not meet the criteria for parametric testing were evaluated using the Mann–Whitney U test. Repeated-measures data were analyzed by repeated-measures analysis of variance. To verify whether categorical variables differed significantly between the groups, the chi-square test was used for comparison. A p value < 0.05 was considered to be statistically significant in a two-sided test.
3 Results
3.1 Flow diagram and patient characteristics
The CONSORT study flow diagram is presented in Figure 1. Enrolment took place between August 17, 2020, and January 1, 2022. This prospective, randomized, and controlled study originally considered 62 eligible patients, of whom 48 were enrolled and randomly assigned to the CRV group (n = 24) and IRV group (n = 24). There was no statistically significant difference between the four groups with regard to age, sex, BMI, hemoglobin (Hb), and ASA physical status, as shown in Table 1.
3.2 Safe apnea time
For the primary outcome, the safe apnea time for patients in the CRV group was 153.80 ± 41.54 s, and that in the IRV group was 210.40 ± 47.47 s. The mean difference [95% CI] between them was 56.55 [28.00–85.10]. Compared with the CRV group, the safe apnea time in the IRV group was longer, and the difference was statistically significant (p = 0.0003), as shown in Figure 2.
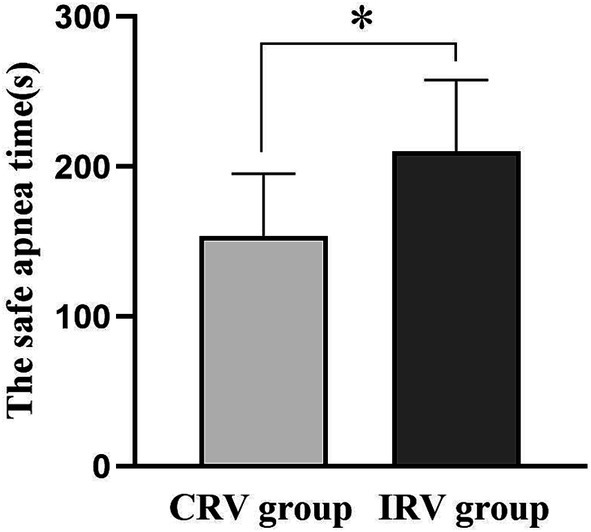
Figure 2. The safe apnea time in both groups. CRV, conventional ratio ventilation; IRV, inverse ratio ventilation. Data are presented as the mean ± SD. *p < 0.05 compared with the CRV group.
3.3 Secondary endpoints
As shown in Figure 3, FeO2 was comparable in both the groups at T0. However, at T2 and T3, IRV group (89.45 ± 2.54, 91.60 ± 1.79) had significantly higher FeO2 as compared to CRV group (87.20 ± 2.17, 87.60 ± 2.39) (p = 0.0046, p < 0.0001).
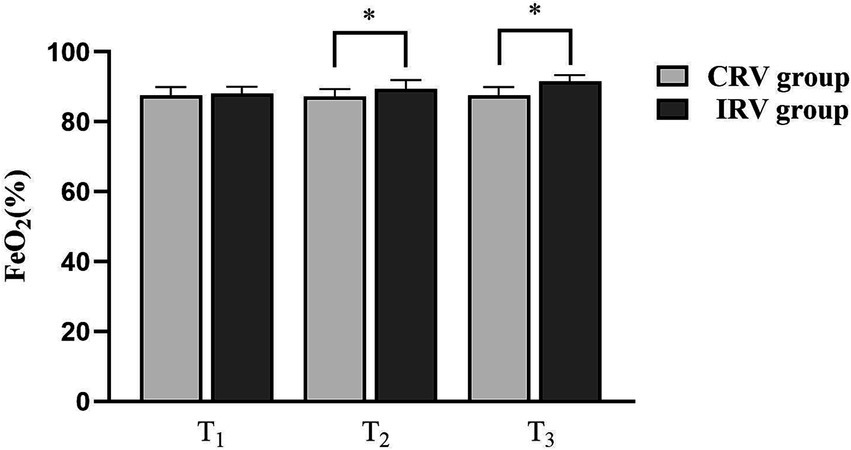
Figure 3. Changes of FeO2 in both groups. CRV, conventional ratio ventilation; IRV, inverse ratio ventilation. Data are presented as the median (IQR). *p < 0.05 compared with the CRV Group.
According to the results of arterial blood gas analysis, the indexes were compared between the two groups. There was no statistically significant difference in PaO₂ and PaCO2 between the two groups of patients at T0, T1, and T3 (p > 0.05). Compared to T0, the PaO₂ of the two groups of patients increased significantly at T1 and T3, and the PaCO2 of the two groups of patients increased at T3 and only increased at T1 in the CRV group, and the differences were statistically significant (p < 0.05), as shown in Table 2.
Compared with the CRV group, the Ppeak and Pplat of the IRV group decreased at both T2 and T3, and the differences were statistically significant (all p < 0.05), as shown in Table 3. MAP was significantly higher in the IRV group compared with the CRV group at both T2 and T3 (p < 0.0001), as shown in Table 3.
As shown in Table 4, there was no statistically significant difference in the HR, systolic blood pressure (SBP), and diastolic blood pressure (DBP) between the two groups of patients at T0, T1, T2, and T3 (p > 0.05). Compared with T0, the HR of patients in the two groups decreased at T1, T2, and T3, the SBP and DBP decreased at T2 and T3, and the difference was statistically significant (p < 0.05).
4 Discussion
The main finding of our study was that volume-controlled inverse ratio ventilation with an I:E ratio of 2:1 prolonged safe apnea time by 57 s and significantly increased FeO2 levels in the obese surgical patients compared with conventional proportional ventilation with an I:E ratio of 1:2 during induction of general anesthesia. In addition, compared with conventional ventilation, inverse ratio ventilation with a 2:1of I:E ratio did not result in carbon dioxide retention or hemodynamic changes.
The main purpose of pre-oxygenation during the induction period of general anesthesia is to increase the oxygen/nitrogen ratio in the lung resting volume (functional residual capacity), so as to prolong the safe apnea time when breathing disappears and maintain SpO2 within a safe range. Clinically, obese patients have a significantly shorter safe apnea time than normal patients and are more likely to lapse into hypoxemia (6, 16). Even if obese patients are pre-oxygenated for 5 min, the SpO2 of patients may still drop rapidly to 90% within 2–3 min after induction without ventilation (5, 6).
This phenomenon is mainly related to the disorder of ventilation/perfusion ratio and the reduction of FRC in obese patients, and the inhalation of 100% oxygen during the anesthesia induction period. In obese patients, the redistribution of ventilation toward the lung apices with the bulk of perfusion delivered to the bases has been observed during spontaneous breathing at the end of exhalation, particularly in patients with a reduction in expiratory reserve of less than 300 mL (17). On the other hand, the cephalic displacement of the diaphragm by the abdominal fat in obese patients affects the lung capacity, producing a restrictive ventilatory disorder whose hallmark is the reduction in the FRC and decrease in the expiratory reserve volume (18). Furthermore, Jones RL et al. shown that with an increase in body mass index (between 25 and 35), the decreases in FRC and expiratory reserve volumes are more significant (19). In addition, inhalation of highly concentrated oxygen can lead the formation of reabsorption atelectasis during the intubation time, which will further reduce FRC (20). Finally, with the reduction of oxygen storage in the lungs, obese patients are more likely to develop hypoxemia during induction of general anesthesia.
At present, there are several methods to improve the safe apnea time in obese patients without ventilation, such as choosing an appropriate body position like head-up (9) or beach-chair positioning (10), non-invasive positive pressure ventilatory support (5), high-flow nasal oxygen (11), and selecting an appropriate oxygen concentration during pre-oxygenation (20). The objective of these methods is to increase the lung oxygen reserve of the patients by reducing atelectasis and improving lung volume (2). A study of induction positions in obese patients found that pre-oxygenation in the head-up position prolonged the safe apnea time by an average of 50–60 s compared with the supine position (9). A previous study also has found that the use of high-flow nasal oxygen techniques during intubation can extend the safe apnea time by more than 100 s (11). The combined use of CPAP Continuous Positive Airway Pressure and PEEP Positive End-Expiratory Pressure during induction in morbidly obese patients can extend the safe apnea time by more than 1 min (5). Compared with the above methods, inverse ratio ventilation can also be used as an effective and feasible method to prolong the safe apnea time in obese patients.
In the clinic, during mechanical ventilation under general anesthesia, the I:E ratio is usually set at 1:2 or 1:1.5 to approximate the normal inspiratory and expiratory physiology. However, sometimes we need to use the inverse ratio ventilation to improve oxygenation in patients with reduced FRC, such as neonates and ARDS Acute Respiratory Distress Syndrome patients (21, 22). In our study, we found that the I:E ratio 2:1 of volume-controlled ventilation not only prolongs safe apnea time by an average of 57 s but also increases FeO2 in obese surgical patients during the induction of general anesthesia. The main reason for this is that inverse ratio ventilation increases the mean airway pressure and dynamic compliance by lengthening the inspiratory time and shortening expiratory time, which avoids alveolar collapse and lung atelectasis, increases oxygen reserve, and improves the patient’s oxygenation status (12). Jo et al. shown that inverse ratio ventilation can improve intraoperative arterial oxygenation in morbidly obese patients undergoing bariatric surgery, which may be related to the fact that it can cause an increase in mean airway pressure and dynamic compliance, and finally improve alveolar collapse (23). In addition, intrinsic PEEP produced due to the relatively shortened expiration time of inverse ratio ventilation also helps to improve the oxygen saturation of the patient (24). In clinical practice, FeO2 is usually used to measure the effect of pre-oxygenation, and the ideal endpoint of pre-oxygenation and denitrification is defined as FeO2 of 90% or expired N2 concentration of 5%. Our research results suggest that the use of inverse ratio ventilation can improve FeO2 at a ventilation for 3 and 5 min, and this may also be the objective reason why inverse ratio ventilation prolongs safe apnea time.
We compared the PaO2 of the two groups at different time points and found that there was no statistical difference. Several studies found that the oxygen reserve mainly in the respiratory system, and not in the arterial blood, when inhaling air instead of inhaling 100% O2 (25, 26). Jense et al. found that the safe apnea time was negatively correlated with BMI, but without any correlation with the PaO2 in obese patients (6). In the Mir et al. study, it was also found that compared with mask pre-oxygenation, the rapid nasal oxygen inhalation can prolong the safe apnea time in patients undergoing emergency surgery, but there was no difference in PaO2 between the two groups (27). Our results are consistent with all these studies.
We also fund I:E ratio 2:1 volume-controlled inverse ratio ventilation did not cause carbon dioxide retention or hemodynamic changes. Consistent with our research results, Youn Yi Jo et al. found that there was no significant difference in PaCO2 between different I:E ratios in obese patients undergoing bariatric surgery, and inverse ratio ventilation had no effect on CO2 excretion (23). Previous studies found that patients did not experience serious adverse cardiovascular events when the I:E ratio was chosen to be 2:1 (28). This is also the basis for our study to choose 2:1 as the I:E ratio for non-invasive ventilation.
There are also some limitations of our study. First, this is a single-center study, and it is necessary to expand the sample in future research to further clarify the effect of volume-controlled inverse ratio ventilation on the safe apnea time in obese patients. Second, all patients were not ventilated for several minutes after the induction of anesthesia, posing a risk of lung injury. Third, we only used PaCO2 to observe whether inverse ratio ventilation would lead to carbon dioxide retention, but neglected to record the changes in end-tidal carbon dioxide, which may be more convenient and valuable. Fourth, we only chose the volume-controlled inverse ratio ventilation mode, and further research is needed on the effect of pressure-controlled inverse ratio ventilation mode on the safe apnea time in obese patients. Fifth, although we did not monitor gastric distention caused by non-invasive positive pressure ventilation, the Ppeak was well below the opening pressure of the upper esophageal sphincter (29), which may indicate that patients are unlikely to experience gastric distension.
In summary, volume-controlled inverse ratio ventilation of I:E ratio 2:1 in obese patients during the anesthesia induction resulted in longer safe apnea time and higher expired O2 fraction without carbon dioxide retention or hemodynamic changes compared to conventional ratio ventilation, which should be considered during the induction of anesthesia in obese surgical patients.
Data availability statement
The raw data supporting the conclusions of this article will be made available by the authors, without undue reservation.
Ethics statement
The studies involving humans were approved by the Institutional Ethics Committee of the General Hospital of Ningxia Medical University. The studies were conducted in accordance with the local legislation and institutional requirements. The participants provided their written informed consent to participate in this study.
Author contributions
YZ: Conceptualization, Data curation, Formal analysis, Funding acquisition, Investigation, Methodology, Project administration, Resources, Software, Writing – original draft, Writing – review & editing. BL: Conceptualization, Data curation, Formal analysis, Investigation, Methodology, Project administration, Software, Writing – original draft, Writing – review & editing. CX: Conceptualization, Data curation, Methodology, Writing – review & editing. YW: Conceptualization, Software, Writing – review & editing. LM: Data curation, Writing – review & editing. FY: Data curation, Writing – review & editing. HM: Conceptualization, Data curation, Formal analysis, Project administration, Resources, Software, Supervision, Writing – review & editing. XN: Conceptualization, Data curation, Formal analysis, Project administration, Resources, Writing – review & editing.
Funding
The author(s) declare that financial support was received for the research and/or publication of this article. This study was supported by the National Natural Science Foundation of China (grant number: 82260228), the Ningxia Natural Science Foundation (grant number: 2022AAC03491) and the Key Research and Development Program of Ningxia (grant number: 2021BEG03073).
Acknowledgments
We gratefully acknowledge the participants and staff members at the General Hospital of Ningxia Medical University.
Conflict of interest
The authors declare that the research was conducted in the absence of any commercial or financial relationships that could be construed as a potential conflict of interest.
Generative AI statement
The authors declare that no Gen AI was used in the creation of this manuscript.
Publisher’s note
All claims expressed in this article are solely those of the authors and do not necessarily represent those of their affiliated organizations, or those of the publisher, the editors and the reviewers. Any product that may be evaluated in this article, or claim that may be made by its manufacturer, is not guaranteed or endorsed by the publisher.
Footnotes
References
1. Yárnoz-Esquiroz, P, Olazarán, L, Aguas-Ayesa, M, Perdomo, CM, García-Goñi, M, Silva, C, et al. ‘Obesities’: Position statement on a complex disease entity with multifaceted drivers. Eur J Clin Investig. (2022) 52:e13811. doi: 10.1111/eci.13811
2. Shah, U, Wong, J, Wong, DT, and Chung, F. Preoxygenation and intraoperative ventilation strategies in obese patients: a comprehensive review. Curr Opin Anaesthesiol. (2016) 29:109–18. doi: 10.1097/aco.0000000000000267
3. Juvin, P, Lavaut, E, Dupont, H, Lefevre, P, Demetriou, M, Dumoulin, JL, et al. Difficult tracheal intubation is more common in obese than in lean patients. Anesth Analg. (2003) 97:595–600. doi: 10.1213/01.Ane.0000072547.75928.B0
4. Hedenstierna, G, Tokics, L, Scaramuzzo, G, Rothen, HU, Edmark, L, and Öhrvik, J. Oxygenation impairment during anesthesia: influence of age and body weight. Anesthesiology. (2019) 131:46–57. doi: 10.1097/aln.0000000000002693
5. Rajan, S, Joseph, N, Tosh, P, Paul, J, and Kumar, L. Effects of preoxygenation with tidal volume breathing followed by apneic oxygenation with and without continuous positive airway pressure on duration of safe apnea time and arterial blood gases. Anesth Essays Res. (2018) 12:229–33. doi: 10.4103/aer.AER_219_17
6. Jense, HG, Dubin, SA, Silverstein, PI, and O’Leary-Escolas, U. Effect of obesity on safe duration of apnea in anesthetized humans. Anesth Analg. (1991) 72:89–93. doi: 10.1213/00000539-199101000-00016
7. Gambee, AM, Hertzka, RE, and Fisher, DM. Preoxygenation techniques: comparison of three minutes and four breaths. Anesth Analg. (1987) 66:468–70. doi: 10.1213/00000539-198705000-00019
8. Nimmagadda, U, Salem, MR, and Crystal, GJ. Preoxygenation: physiologic basis, benefits, and potential risks. Anesth Analg. (2017) 124:507–17. doi: 10.1213/ane.0000000000001589
9. Dixon, BJ, Dixon, JB, Carden, JR, Burn, AJ, Schachter, LM, Playfair, JM, et al. Preoxygenation is more effective in the 25 degrees head-up position than in the supine position in severely obese patients: a randomized controlled study. Anesthesiology. (2005) 6:1110–5. doi: 10.1097/00000542-200506000-00009
10. Couture, EJ, Provencher, S, Somma, J, Lellouche, F, Marceau, S, and Bussières, JS. Effect of position and positive pressure ventilation on functional residual capacity in morbidly obese patients: a randomized trial. Can J Anaesth. (2018) 65:522–8. doi: 10.1007/s12630-018-1050-1
11. Wong, DT, Dallaire, A, Singh, KP, Madhusudan, P, Jackson, T, Singh, M, et al. High-flow nasal oxygen improves safe apnea time in morbidly obese patients undergoing general anesthesia: a randomized controlled trial. Anesth Analg. (2019) 129:1130–6. doi: 10.1213/ane.0000000000003966
12. Sembroski, E, Sanghavi, DK, and Bhardwaj, A. Inverse ratio ventilation. Treasure Island (FL): StatPearls Publishing LLC (2024).
13. Hirabayashi, G, Yokose, Y, Oshika, H, Saito, M, Maruyama, K, and Andoh, T. Effects of volume-targeted pressure-controlled inverse ratio ventilation on functional residual capacity and dead space in obese patients undergoing robot-assisted laparoscopic radical prostatectomy. BJA Open. (2022) 3:100020. doi: 10.1016/j.bjao.2022.100020
14. Zhang, WP, and Zhu, SM. The effects of inverse ratio ventilation on cardiopulmonary function and inflammatory cytokine of bronchoaveolar lavage in obese patients undergoing gynecological laparoscopy. Acta Anaesthesiol Taiwanica. (2016) 54:1–5. doi: 10.1016/j.aat.2015.11.001
15. Gong, Y, Liu, L, and Zhang, W. Pressure-controlled inverse ratio ventilation improves gas exchange in obese children undergoing laparoscopic surgery: a randomized controlled study. Am J Transl Res. (2023) 11:6551–7.
16. Berthoud, MC, Peacock, JE, and Reilly, CS. Effectiveness of preoxygenation in morbidly obese patients. Br J Anaesth. (1991) 67:464–6. doi: 10.1093/bja/67.4.464
17. Holley, HS, Milic-Emili, J, Becklake, MR, and Bates, DV. Regional distribution of pulmonary ventilation and perfusion in obesity. J Clin Invest. (1967) 46:475–81. doi: 10.1172/jci105549
18. Steier, J, Lunt, A, Hart, N, Polkey, MI, and Moxham, J. Observational study of the effect of obesity on lung volumes. Thorax. (2014) 69:752–9. doi: 10.1136/thoraxjnl-2014-205148
19. Jones, RL, and Nzekwu, MM. The effects of body mass index on lung volumes. Chest. (2006) 130:827–33. doi: 10.1378/chest.130.3.827
20. Edmark, L, Kostova-Aherdan, K, Enlund, M, and Hedenstierna, G. Optimal oxygen concentration during induction of general anesthesia. Anesthesiology. (2003) 98:28–33. doi: 10.1097/00000542-200301000-00008
21. Ludwigs, U, Klingstedt, C, Baehrendtz, S, Wegenius, G, and Hedenstierna, G. Volume-controlled inverse ratio ventilation in oleic acid induced lung injury. Effects on gas exchange, hemodynamics, and computed tomographic lung density. Chest. (1995) 108:804–9. doi: 10.1378/chest.108.3.804
22. Zavala, E, Ferrer, M, Polese, G, Masclans, JR, Planas, M, Milic-Emili, J, et al. Effect of inverse I:E ratio ventilation on pulmonary gas exchange in acute respiratory distress syndrome. Anesthesiology. (1998) 1:35–42. doi: 10.1097/00000542-199801000-00008
23. Jo, YY, Kim, JY, Park, CK, Chang, YJ, and Kwak, HJ. The effect of ventilation strategy on arterial and cerebral oxygenation during laparoscopic bariatric surgery. Obes Surg. (2016) 26:339–44. doi: 10.1007/s11695-015-1766-8
24. Ludwigs, U, Klingstedt, C, Baehrendtz, S, and Hedenstierna, G. A comparison of pressure- and volume-controlled ventilation at different inspiratory to expiratory ratios. Acta Anaesthesiol Scand. (1997) 41:71–7. doi: 10.1111/j.1399-6576.1997.tb04615.x
25. Nunn, JF, Bergman, NA, and Coleman, AJ. Factors influencing the arterial oxygen tension during anaesthesia with artificial ventilation. Br J Anaesth. (1965) 37:898–914. doi: 10.1093/bja/37.12.898
26. Campbell, IT, and Beatty, PC. Monitoring preoxygenation. Br J Anaesth. (1994) 72:3–4. doi: 10.1093/bja/72.1.3
27. Mir, F, Patel, A, Iqbal, R, Cecconi, M, and Nouraei, SA. A randomised controlled trial comparing transnasal humidified rapid insufflation ventilatory exchange (THRIVE) pre-oxygenation with facemask pre-oxygenation in patients undergoing rapid sequence induction of anaesthesia. Anaesthesia. (2017) 72:439–43. doi: 10.1111/anae.13799
28. Pinsky, MR. The effects of mechanical ventilation on the cardiovascular system. Crit Care Clin. (1990) 6:663–78. doi: 10.1016/S0749-0704(18)30360-9
29. Cajander, P, Edmark, L, Ahlstrand, R, Magnuson, A, and de Leon, A. Effect of positive end-expiratory pressure on gastric insufflation during induction of anaesthesia when using pressure-controlled ventilation via a face mask: a randomised controlled trial. Eur J Anaesthesiol. (2019) 36:625–32. doi: 10.1097/EJA.0000000000001016
Keywords: safe apnea time, pre-oxygenation, inverse ratio ventilation, functional residual capacity, obesity, oxygen saturation, expiratory oxygen fraction
Citation: Zhang Y, Li B, Xu C, Wu Y, Ma L, Yang F, Ma H and Ni X (2025) Volume-controlled inverse ratio ventilation improves safe apnea time in obese patients during the induction of general anesthesia: a randomized controlled trial. Front. Med. 12:1574634. doi: 10.3389/fmed.2025.1574634
Edited by:
Luigi Vetrugno, University of Studies G. d’Annunzio Chieti and Pescara, ItalyReviewed by:
Domenico Junior Brunetti, Policlinico Tor Vergata, ItalyMarco Tiseo, Ospedale dei Castelli, Italy
Roberto Polidoro, Policlinico Tor Vergata, Italy
Copyright © 2025 Zhang, Li, Xu, Wu, Ma, Yang, Ma and Ni. This is an open-access article distributed under the terms of the Creative Commons Attribution License (CC BY). The use, distribution or reproduction in other forums is permitted, provided the original author(s) and the copyright owner(s) are credited and that the original publication in this journal is cited, in accordance with accepted academic practice. No use, distribution or reproduction is permitted which does not comply with these terms.
*Correspondence: Hanxiang Ma, bWFoYW54aWFuZ0Bob3RtYWlsLmNvbQ==; Xinli Ni, eGlubGluaTZAbnlmeS5jb20uY24=
†These authors share first authorship