- 1Winogradsky Institute of Microbiology, Research Centre of Biotechnology, Russian Academy of Sciences, Moscow, Russia
- 2Department of Biotechnology, Delft University of Technology, Delft, Netherlands
- 3NIOZ Royal Netherlands Institute for Sea Research, Texel, Netherlands
Beta-mannans are insoluble plant polysaccharides with beta-1,4-linked mannose as the backbone. We used three forms of this polysaccharide, namely, pure mannan, glucomannan, and galactomannan, to enrich haloarchaea, which have the ability to utilize mannans for growth. Four mannan-utilizing strains obtained in pure cultures were closely related to each other on the level of the same species. Furthermore, another strain selected from the same habitats with a soluble beta-1,4-glucan (xyloglucan) was also able to grow with mannan. The phylogenomic analysis placed the isolates into a separate lineage of the new genus level within the family Natrialbaceae of the class Halobacteria. The strains are moderate alkaliphiles, extremely halophilic, and aerobic saccharolytics. In addition to the three beta-mannan forms, they can also grow with cellulose, xylan, and xyloglucan. Functional genome analysis of two representative strains demonstrated the presence of several genes coding for extracellular endo-beta-1,4-mannanase from the GH5_7 and 5_8 subfamilies and the GH26 family of glycosyl hydrolases. Furthermore, a large spectrum of genes encoding other glycoside hydrolases that were potentially involved in the hydrolysis of cellulose and xylan were also identified in the genomes. A comparative genomics analysis also showed the presence of similar endo-beta-1,4-mannanase homologs in the cellulotrophic genera Natronobiforma and Halococcoides. Based on the unique physiological properties and the results of phylogenomic analysis, the novel mannan-utilizing halolarchaea are proposed to be classified into a new genus and species Natronoglomus mannanivorans gen. nov., sp. nov. with the type strain AArc-m2/3/4 (=JCM 34861=UQM 41565).
Introduction
Recent extensive studies on the functional diversity of extremely halophilic archaea belonging to the class Halobacteria resulted in a significant shift in a longstanding perception of this unique group of extremophiles as copiotrophic dissipotrophs utilizing either rich amino acid mixtures or simple soluble monomeric compounds, such as sugars or organic acids. In particular, it is becoming evidently clear that many haloarchaeal species possess extensive hydrolytic potential against various groups of recalcitrant insoluble polysaccharides, such as chitin, cellulose, and a range of other neutral glucans (Sorokin et al., 2015, 2018, 2019a,b, 2022a, 2023). In our previous study, we used selective enrichments with a range of soluble and insoluble glucans to obtain pure cultures of haloarchaea utilizing various glucans with different monomers and back-bond structures as growth substrates (Sorokin et al., 2022a). One of the selected groups included five isolates of alkaliphilic haloarchaea that have the ability to grow with beta-mannans, including pure beta-1,4-mannan and its two modifications, glucomannan (with glucose and mannose in the backbone) and galactomannan (with galactose in the side chains). To date, the beta-mannans with backbone have been proven to serve as a growth substrate for three characterized species of haloarchaea: Natronoarchaeum mannanilyticum (Shimane et al., 2010) and Haloarcula mannanilytica (Enomoto et al., 2020) use galactomannan as a substrate and cellulotrophic Natronobiforma cellulositropha uses pure beta-1,4-mannan as a substrate (Sorokin et al., 2018). The latter and the heteropolymeric glucomannan have never been tested before to enrich mannan-utilizing haloarchaea. According to the CAZy database (http://www.cazy.org), the only enzymatically characterized mannan-specific glycoside hydrolases (several subfamilies of the GH family 5 and the GH family 26) are known in bacteria and eukarya, mostly in fungi. This finding certainly indicates a substantial gap in the knowledge on the potential presence of functionally active archaeal beta-mannanases and on the identity of archaea that are able to utilize such polymers as growth substrates.
In this study, we provide taxonomic evaluation and functional genome analysis focusing on glycosyl hydrolase families for several strains of haloarchaea, which were previously enriched from neutral and alkaline hypersaline lakes in southwestern Siberia on insoluble beta-1,4-mannans. All mannan-utilizing isolates are closely related, forming a separate lineage within the family Natrialbaceae, and are proposed to be classified as Natronoglomus mannanivorans gen. nov., sp. nov.
Materials and methods
Media and cultivation conditions
Mix surface sediments and brines collected from several hypersaline salt and soda lakes in the Kulunda Steppe (Altai, Russia) were used as inoculum for enrichment cultures. The brine pH (measured with the field pH meter, WWR) in neutral lakes of the chloride-sulfate type ranged from 7.5 to 8.2, and in soda lakes, the pH values ranged from 9.8 to 11. The total salt concentration (measured in the field with a refractometer and verified by the gravimetry in the laboratory) was found to be ranging from 200 to 400 g l−1. Before cultivation, the sediment suspension (one part of sediment:nine parts of brines, v:v) was preincubated for 3 days at 28°C on a rotary shaker with the addition of 200 mg l−1 each of ampicillin and streptomycin to suppress the growth of bacteria. The suspended solids were precipitated by centrifugation and resuspended into two types of base mineral medium containing either 4 M total NaCl at pH 7 or a 3:1 (v:v) mix of 4 M NaCl and sodium-carbonate base with 4 M total Na+ (the final pH was 9.5). The cultures were supplemented with 1 g l−1 each of three types of beta-1,4-mannan (Megazyme, Ireland): pure mannan; glucomannan (beta-1,4 heteropolymer of mannose and glucose with a Gl:Man ratio of 4:1); and galactomannan (beta-1,4-mannan with a side chain decoration of alpha-1,6-linked galactose). The polymers were prepared as 10% suspensions in sterile distilled water and sterilized at 110°C for 30 min, and the enrichments were incubated on a rotary shaker in closed bottles at 37°C until visible substrate degradation (accessed by light microscopy) and the appearance of pink turbidity in the liquid phase occurs. Pure culture isolation was achieved after the plating of final positive serial dilution from single colonies forming the clearance zones on the mannan plates (the mannan suspension was sonicated to reduce the particle size) and showing stable growth in the liquid medium with the beta-1,4-mannan as the sole carbon and energy source.
Phenotypic characterization
Phase contrast microscopy was performed using Zeiss Axioplane Imaging 2 (Germany). The growth in liquid cultures was monitored OD600 after 30 min of gravity sedimentation of insoluble particles or directly in the case of soluble substrates. Thin-section electron microscopy was performed for the cells of type strain AArc-m2/3/4 grown either with beta-mannan or xylan, using a JEOL100 instrument (Japan) as described previously (Sorokin et al., 2018). The ability for anaerobic growth was tested in serum bottles sealed with butyl rubber stoppers after the removal of dissolved oxygen and subjected first to “cold boiling” under a vacuum followed by flashing three times with sterile argon gas. Aerobic substrate utilization, pH-salt profiling, and other standard phenotypic testing were performed as described by Sorokin et al. (2022a). Membrane polar lipids and respiratory menaquinones were extracted from freeze-dried biomass of strains AArc-m2/3/4 and AArc-xg1-1 that were grown with cellobiose at 37°C, 4 M total Na+, and pH 9.5 until the late exponential growth phase and were resolved by Ultra High Pressure Liquid Chromatography-High Resolution Mass Spectrometry (UHPLCHRMS) using an Agilent 1290 Infinity I UHPLC (ThermoFisher Scientific), as described previously (Bale et al., 2021; Sorokin et al., 2022b).
Growth tests were performed in a basal alkaline medium containing 4 M total Na+ (1 M as sodium carbonates and 3 M as NaCl) at pH 9.5. Substrates were added at a final concentration of 1 g l−1 after sterilization from 10% concentrated stocks. In total, 10 ml cultures in 30 ml bottles with screw-capped rubber septum (to prevent evaporation) were incubated on a rotary shaker at 37°C. The growth was monitored by measuring OD600 against a control without substrate, directly in the case of soluble substrate, and after vigorous homogenization and 10 min stasis to allow particles to sediment in the case of insoluble polysaccharides.
Genome sequencing and phylogenomic and functional genomic analyses
The genomes of AArc-m2/3/4 and AArc-xg1-1 were sequenced using the MiSeq Illumina platform and assembled as described previously (Sorokin et al., 2022a). Their draft assemblies were deposited in the GenBank under the numbers GCA_025517485 and GCA_025517495, respectively.
For phylogenomic analysis, 122 conserved single copy archaeal protein markers (Rinke et al., 2021) from the in silico translated genomes of two mannan-utilizing natronoarchaea, the closest related strains KZCA124 and TS33, and all described species of the family Natrialbaceae, were identified and aligned using the GTDB-tk v.1.7.0 with reference data v.202 (Chaumeil et al., 2019). The resulting alignment was treated using trimAL v1.4.1 with –gt 1 option for complete gap elimination (Capella-Gutiérrez et al., 2009). The phylogenomic tree was constructed and drawn using the RAxML v.8.2.12 (the PROTGAMMAILG model, 1,000 rapid bootstrap replications) and iTOL v.6.5.2, respectively (Stamatakis, 2014; Letunic and Bork, 2019). The whole genome comparisons (ANI, AAI, and POPC) were calculated as described earlier (Sorokin et al., 2023).
Functional genome analysis of the glycoside hydrolases (GH) with particular focus on the mannan-hydrolyzing families including the GH5_7, GH5_8, and GH26 families in AArc-m2/3/4 and AArc-xg1-1 (as well as the genomes of related strains KZCA124 and TS33) was performed using the dbCAN v.4 (Zhang et al., 2018) with HMMER (Mistry et al., 2013) and Diamond tools (Buchfink et al., 2015) to detect the target GH domains. Additional domains were searched using the InterPro database (Paysan-Lafosse et al., 2023). Gene clusters of AArc-m2/3/4, AArc-xg1-1, and several other species containing genes encoding endo-beta-mannanases were visualized using the gggenes package in R (https://cran.r-project.org/web/packages/gggenes). The GH5 and GH26 enzymes of strains AArc-m2/3/4 and AArc-xg1-1 and related proteins found by the NCBI BLAST (the organism list was limited to Archaea; the e-value threshold were 1e-10 and 1e-5 for GH5 and GH26, respectively) were used for the phylogenetic reconstruction of the haloarchaeal beta-mannanases. Furthermore, all proteins were analyzed using dbCAN v.4 with HMMER tool to detect target domains; only proteins with target catalytic domains (minimal coverage is 0.4) and some additional carbohydrate-binding domains (minimal coverage is 0.5) were kept in the final protein set. Both sets of sequences (the GH5 and GH26 families) were aligned using MAFFT v.7 with the E-INS-i method (Katoh et al., 2017). The alignments obtained were trimmed using trimAL v1.4.1 with -gt 0.75 (Capella-Gutiérrez et al., 2009). The phylogenetic trees for GH5 and GH26 glycosidases were constructed and decorated as described above for the “ar122”-based phylogenomic tree with a decreased number of bootstrap replications (500).
Results and discussion
Enrichment and isolation of pure cultures of mannan-utilizing haloarchaea
Five enrichment cultures (one from neutral salt lakes and four from soda lakes) showed stable growth with four different polymers. The neutral and soda lake enrichments with beta-1,4-mannan yielded strains HArc-m1 and AArc-m2/3/4T; the soda lake enrichments with galactomannan yielded strain AArc-glctm5 and with glucomannan yielded strain AArc-gm3/4/5-2. An additional enrichment with xyloglucan provided an additional beta-1,4-mannan-utilizing isolate AArc-xg1-1. It was confirmed that the first four isolates selected with different mannan variations could grow with beta-1,4-mannan. The xyloglucan-yielded isolate AArc-xg1-1 was first identified as a potential mannan utilizer based on its phylogenomic proximity to the mannan-enriched strains and functional genome analysis, and it was later confirmed by a growth test. An interesting fact to note is that the neutral salt lake isolate, HArc-m1, turned out to be identical to its counterpart mannan-selected soda lake natronarchaeon, AArc-m2/3/4, and was confirmed to be able to grow optimally at moderately alkaline conditions used for the enrichment of the soda lake isolates, which is a rare example (at least in our experience). Evidently, two factors might have played a role in such a coincidence: the first is the close proximity of the chloride-sulfate and soda lakes in the southern Kulunda steppe (a few kilometers range) and the second is the unique substrate specialization overruling the significance of the alkali-related adaptation.
Phenotypic properties
The cells of all mannan-utilizing strains were mostly true non-motile cocci that were ~1 μm in diameter, becoming especially refractive when grown with mannan and cellulose (Figure 1). Thin sectioning electron microscopy revealed a major difference in the cell ultrastructure between the mannan- and xylan-grown cells: the former had a much thicker cell wall consisting of several layers and an extended pseudoperiplasmic space (Figure 2), which resembled the cellulose-growing cells of Natronobiforma (Sorokin et al., 2018). It might be due to the necessity of these haloarchaea to interact physically with the insoluble glucans.
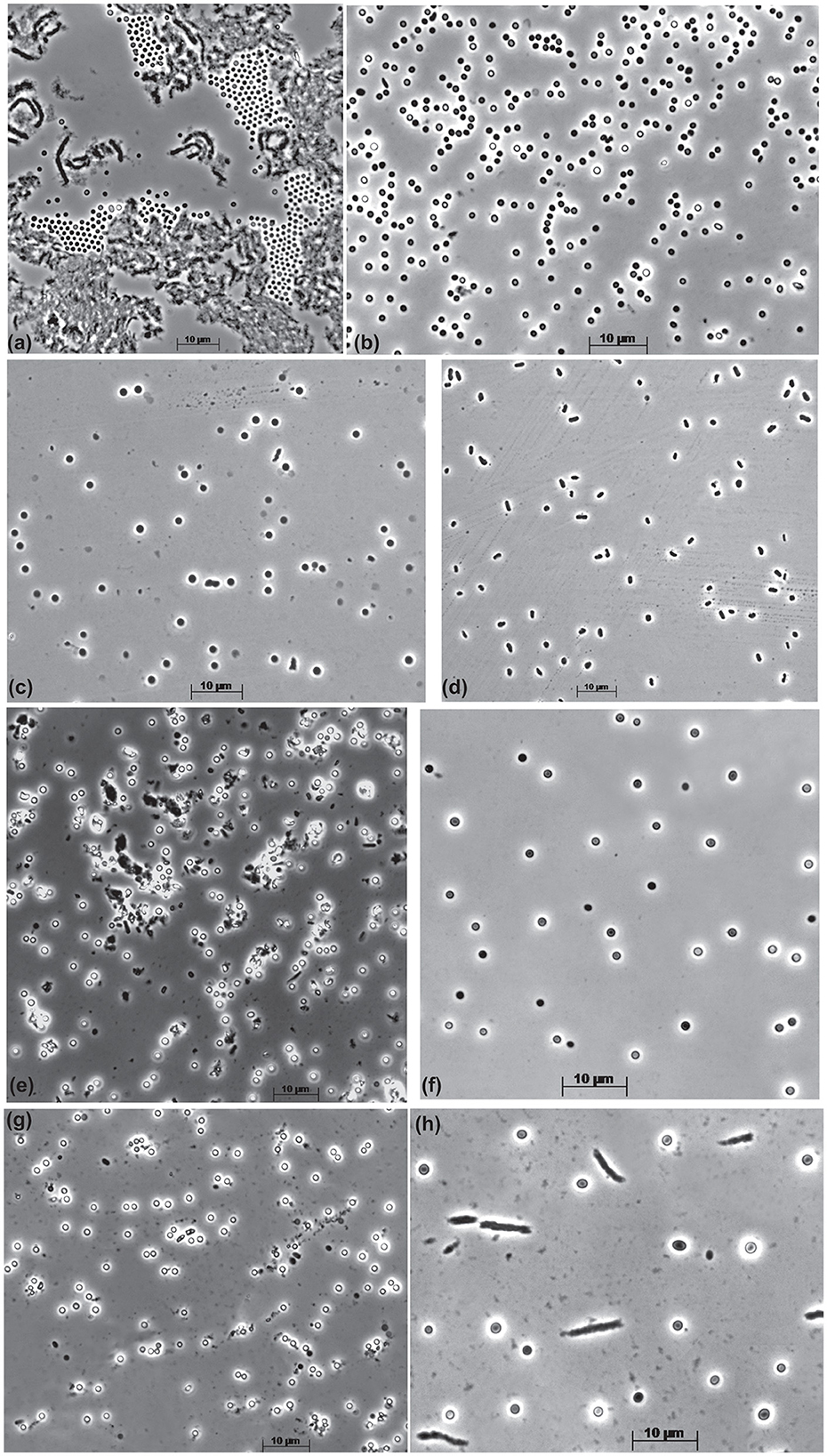
Figure 1. Cell morphology of the beta-1,4-mannan-utilizing haloarchaea growing at 4 M Na+, pH 9.5, and 37oC. (a, b) Strain AArc-m2/3/4 on mannan [(a) aggregated cells in the solid phase with polymer, (b) free cells]; (c, d) strain AArc-xg1-1 [(c) on mannan, (d) on xyloglucan]; (e) strain HArc-m1 on mannan; (f) strain AArc-glctm5 on mannan; (g, h) strain AArc-gm3/4/5-2 [(g) on mannan, (h) on glucomannan].
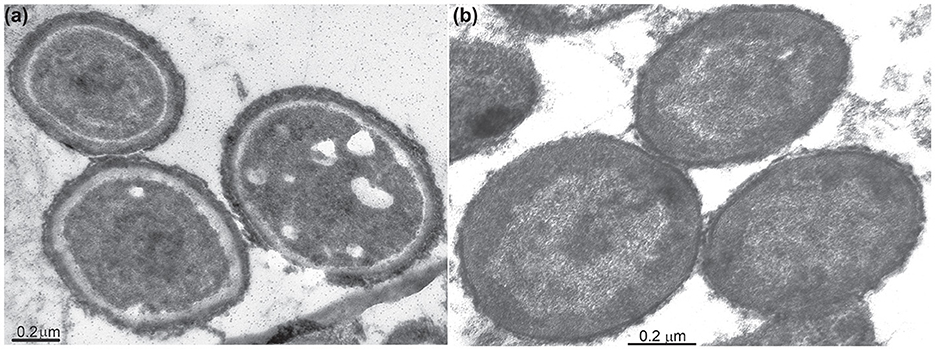
Figure 2. Thin-section electron microscopy microphotographs showing an ultrastructural difference of the AArc-m2/3/4 cells grown with beta-1,4-mannan (a) and xylan (b). The mannan-grown cells have an extra external cell layer and extended pseudoperiplasm in comparison to the cells grown on xylan.
Membrane phospholipid profiling of strains AArc-m2/3/4 and AArc-xg1-1 indicated domination of C20-C20 archaeol and C20-C25 extended archaeol as the core, with phosphatidylglycerolphosphate methyl ester (PGP-Me) and phosphatidylglycerol (PG) as the polar heads in equal proportion (Supplementary Table S1). The glyco- and sulfo-lipids were not detected. The majority of respiratory lipoquinones were represented by the saturated MK-8:8 (75 and 95% in AArc-m2/3/4 and AArc-xg1-1, respectively), and the rest was monounsaturated MK-8:7.
The range of polysaccharides supporting the growth of all isolates included beta-1,4 mannan, glucomannan, amorphous cellulose, xyloglucan, xylan, and arabinoxylan, while galactomannan supported only a weak growth with incomplete degradation. Alpha-glucans from the starch family, beta-glucans with the 1,3- and 1,6-backbone, and the beta-fructans did not support the growth of isolates. Active hydrolysis of the beta-mannan and amorphous cellulose can be observed as the formation of clearance zones around the colonies (Supplementary Figure S1). The range of utilized sugars investigated in the strain AArc-m2/3/4T included galactose, lactose, trehalose, raffinose, rhamnose, sucrose, cellobiose, maltose, melezitose, and melibiose. Interestingly, mannose and glucose, the monomers of two groups of growth-supporting beta-1,4-glucans, were not among the utilized sugars. Most likely, only oligomers were transported inside the cells.
Anaerobic growth with cellobiose as a substrate was not observed both at fermentative conditions and in the presence of various electron acceptors, including nitrate, nitrite, N2O, sulfur, thiosulfate, DMSO, and fumarate. Ammonium and urea, but not nitrate, served as the nitrogen source. The cellobiose-dependent growth at pH 9.5 was not inhibited by streptomycin, ampicillin, kanamycin, vancomycin, and tetracycline at concentrations of up to 100 mg l−1.
AArc-m2/3/4T grew optimally (with cellobiose at pH 9) at 3.5 M total Na+ and with the range from 2.0 to 4.5 M. The pH profiling (with cellobiose at 4 M total Na+) was performed for the soda lake isolate, AArc-m2/3/4T, and for the salt lake isolate, HArc-m1. The former showed a weak growth starting from pH 7.2 and grew up to pH 9.7, while the latter had a lower growth pH limit starting from a minimum of pH 6.8 and was able to grow up to pH 9.5. Both strains grew optimally around pH 9. Thus, they can be qualified as facultatively alkaliphilic extreme halophiles.
Phylogenetic analysis
The genomes of AArc-m2/3/4 and AArc-xg1-1 contain a single rrn operon with the 16S rRNA gene most closely related to the species of the genera Halovivax and Saliphagus (~95% sequence identity). However, according to the results of the phylogenomic analysis based on 122 archaeal conserved single-copy protein markers, mannan-utilizing isolates formed a distinct clade within the Natrialbaceae family neighboring the cellulotrophic genus Natronobiforma (Figure 3, Supplementary Figure S2). Furthermore, the whole-genome-based comparative analyses indicated that the novel isolates form an independent lineage of a new genus level within the Natrialbaceae (Supplementary Table S2). Average nucleotide identities (ANI) between the AArc-m2/3/4T and AArc-xg1-1 strains was 98.5%, which means that they belong to the same species; ANI values between novel isolates and the representatives of the closest genera, Natronobiforma and Saliphagus, were 77.9 and 77.1%, respectively, and the range for other type species of the Natrialbaceae varied from 74.1 to 78.6%. Average amino acid identity (AAI) values between the AArc-m2/3/4T and AArc-xg1-1 strains and the type species of the Natrialbaceae ranged from 58.9 to 70.3%, with the highest value being N. cellulositropha. These values are significantly below the threshold for the demarcation of genera within the Natrialbaceae (76%; de la Haba et al., 2021). The percentage of conserved proteins (POCP) between novel strains and the type species of the Natrialbaceae varied from 49.4 to 66.2% with the highest values being N. cellulositropha and Saliphagus infecundisoli. The highest POCP values were slightly higher than the proposed genus-level threshold of 50% (Qin et al., 2014). However, many authors often indicated that a strict boundary of 50% is not suitable for the demarcation of genera within some taxa (Aliyu et al., 2016; Orata et al., 2018; Wirth and Whitman, 2018). Thus, it is likely that, in the case of the Natrialbaceae, the POCP threshold needs to be revised.
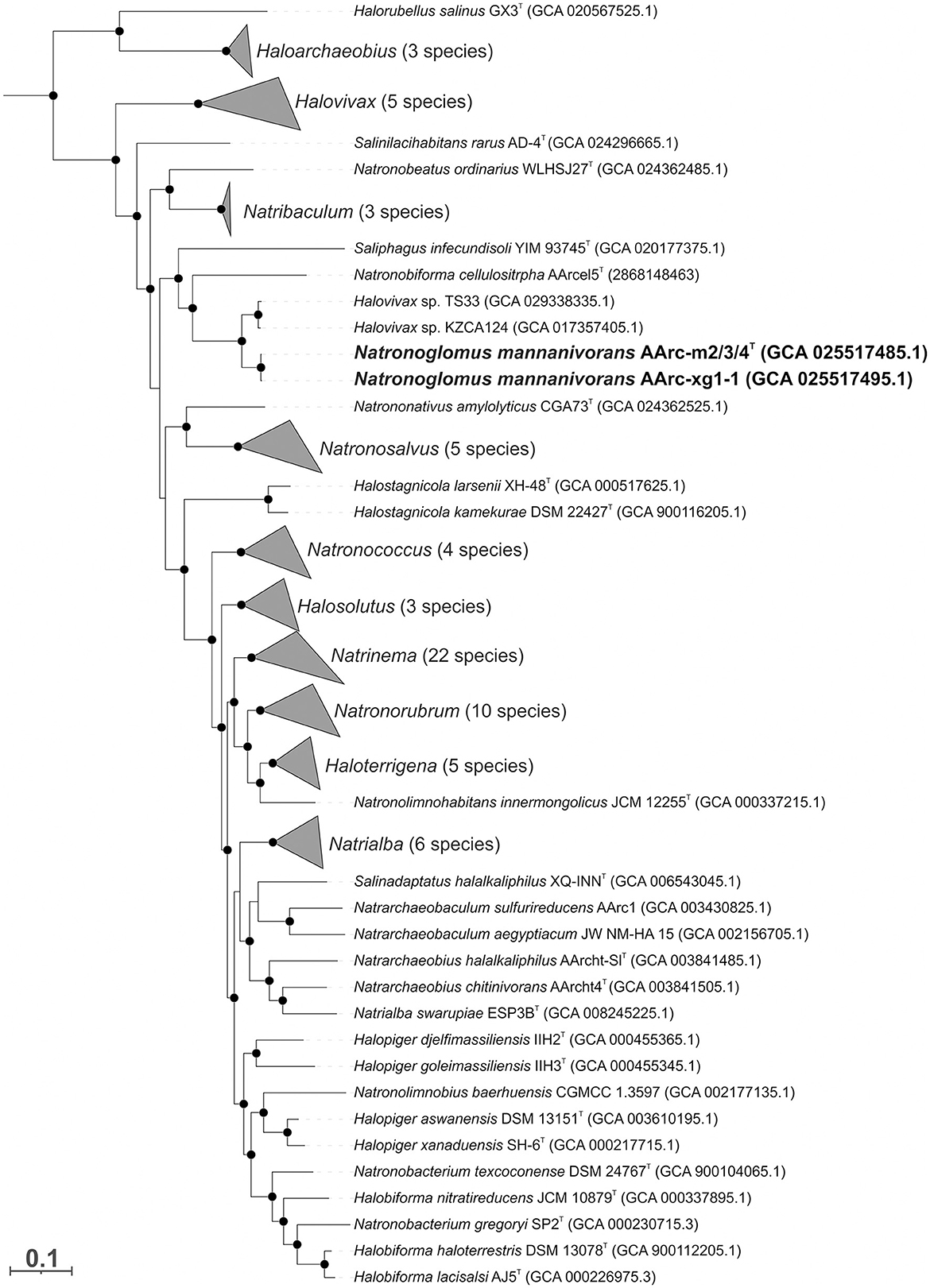
Figure 3. The maximum likelihood phylogenetic tree based on 122 conserved archaeal proteins demonstrating the position of two mannan-utilizing natronoarchaea (in bold) within the family Natrialbaceae. The branch lengths correspond to the number of substitutions per site with corrections associated with the models. The black circles at nodes indicate that the percentage of corresponding support values was above 50. The species of some genera are collapsed, and the numbers of species are given in brackets. Archaeoglobus fulgidus VC-16T, Methanocella paludicola SANAET, and Methanothermobacter thermautotrophicus Delta HT were used as an outgroup (not shown).
Functional genome analysis
Beta-mannan utilization potential
The main functional property of the new isolates is the potential to utilize insoluble beta-1,4-mannans and beta-1,4-glucans (cellulose) as a growth substrate. The two sequenced genomes contained a very similar array of genes encoding the key glycoside hydrolases that were potentially involved in the unique substrate specificity of the novel natronoarchaea. A most interesting group of such enzymes included beta-1,4-mannanases belonging to the families GH26 (one enzyme), GH5_7 (four enzymes, two of them with a carbohydrate binding domain, CBM), and GH5_8 (one enzyme with a CBM) (Table 1). All six proteins have TAT translocation signals (i.e., are extracellular) and are co-located in two large genomic islands together with the genes coding for multiple endo-beta-1,4-glucanases from the GH5 superfamily, which is most likely responsible for cellulose degradation and/or endo-beta-1,4-xylanases from the GH10 family.
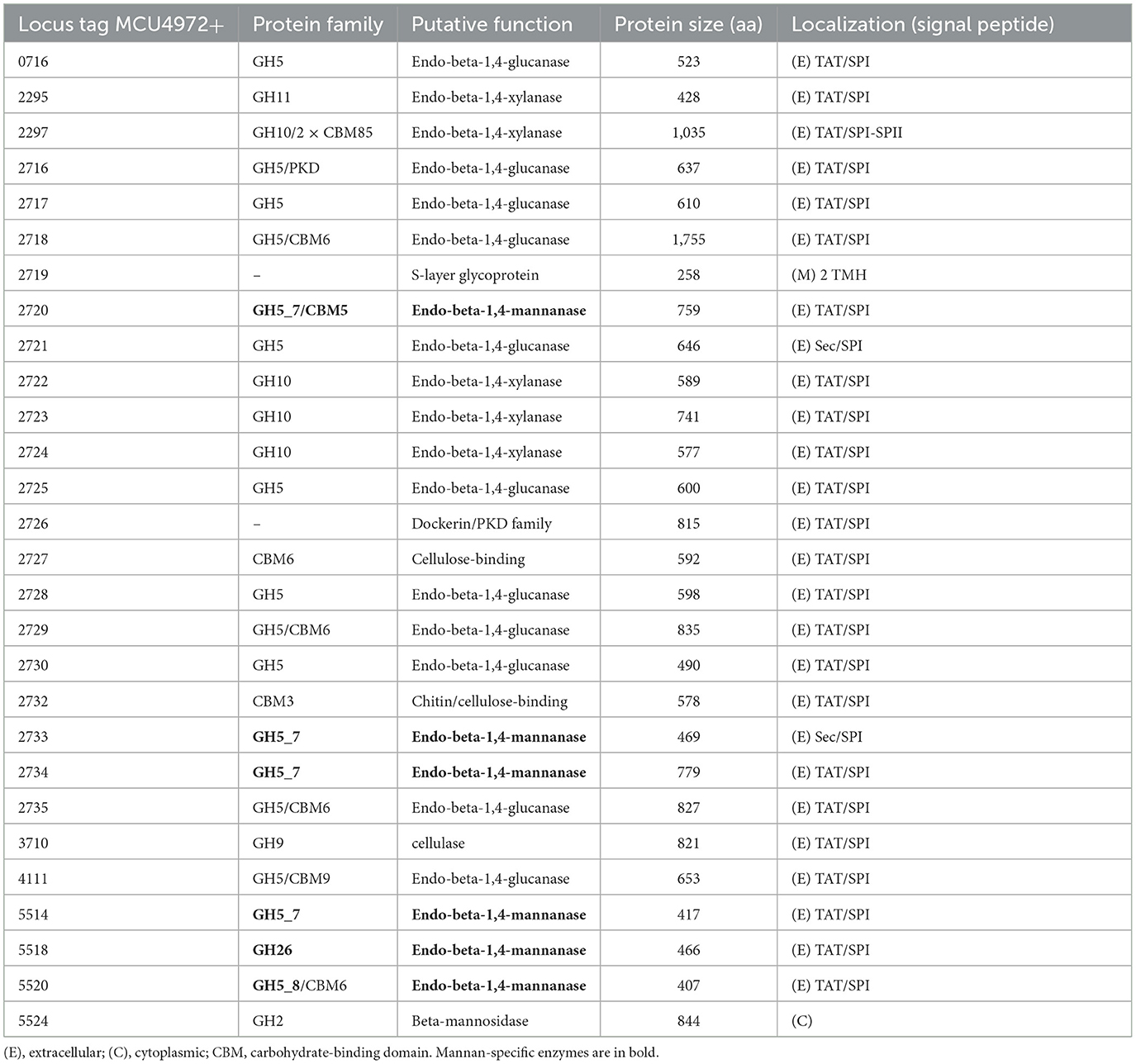
Table 1. Polysaccharide hydrolases potentially involved in the beta-mannan, cellulose, and xylan hydrolysis encoded in the genome of mannan-utilizing strain AArc-m2/3/4.
The first cluster contains genes of three endo-beta-1,4-mannosidases from the GH5_7 and GH5_8 subfamilies and the GH26 family and a beta-mannosidase from the GH2 family (Figure 4A). Moreover, endo-alpha-1,5-L-arabinanase from the GH43 family, a putative enzyme from the GH109 family (homologous to various sugar dehydrogenases), two mannonate dehydratases, putative UDP-glucose 4-epimerase, and a transcriptional regulator of the IclR family were encoded in this cluster. In the genome of AArc-xg1-1, there was an additional CRISPR-associated endonuclease. Very similar clusters were found in the genomes of undescribed strains KZCA124 and TS33, which were closely related to our mannan isolates, but they possessed an additional GH5_7 gene, while genes of GH43 glycosidase were absent. Homologous cluster in N. cellulositropha AArcel5 lacked genes of GH5_8 enzyme and one mannonate dehydratase gene, while Halococcoides cellulosivorans HArcel1 had only a single endo-beta-1,4-mannosidase from GH26.
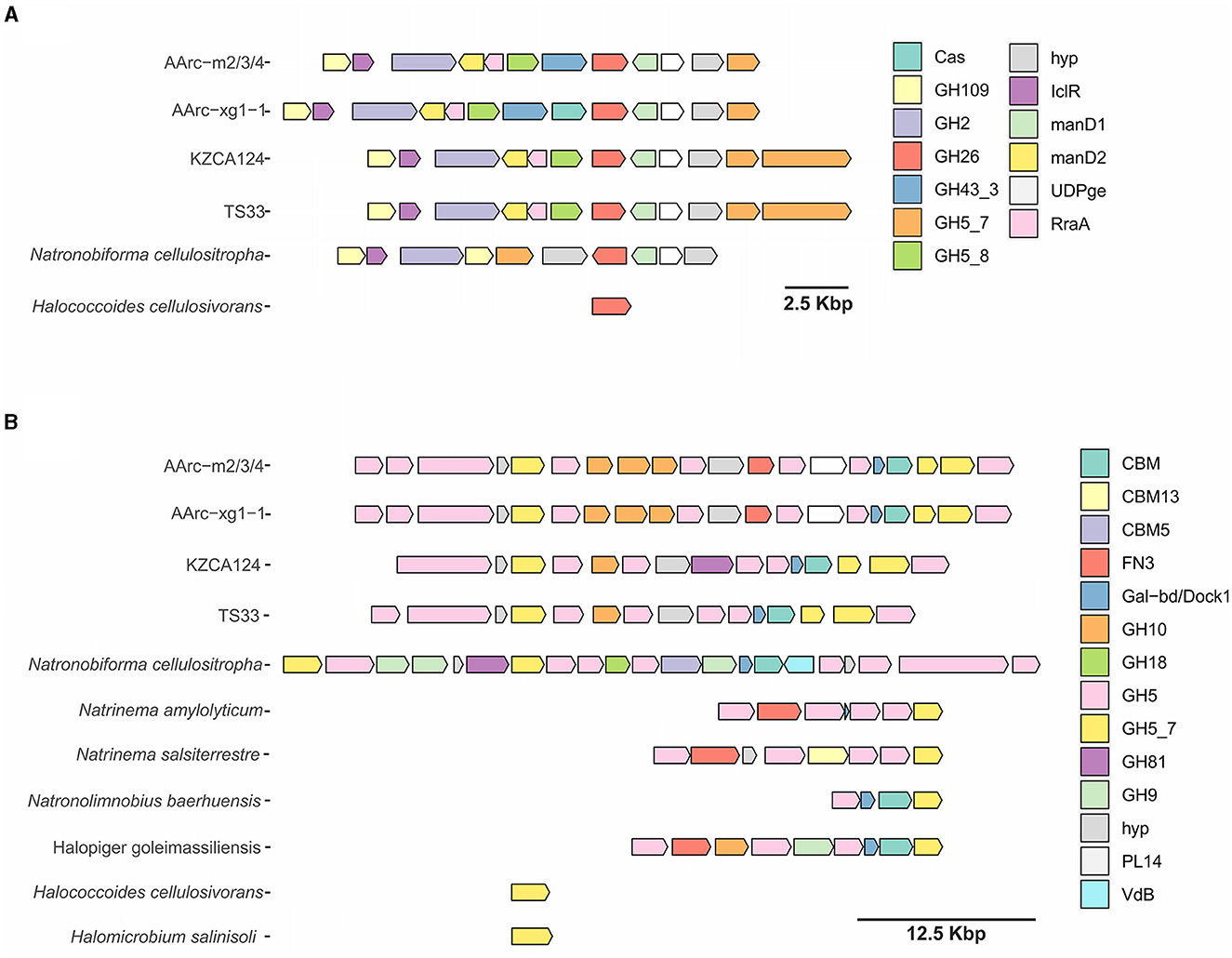
Figure 4. Clusters containing endo-beta-mannanase genes of AArc-m2/3/4T and AArc-xg1-1 strains and similar gene clusters of other haloarchaeae encoding GH26, GH5_7, and GH5_8 families (A) or enzymes of the GH5_7 family only (B): Cas, CRISPR-associated nuclease; GHx, glycoside hydrolase of “x” family; hyp, hypothetical protein; IclR, HTH-type transcriptional regulator of IclR family; manD, mannonate dehydratase; UDPge, UDP-glucose 4-epimerase; RraA, putative oxaloacetate decarboxylase of RraA family; CBM, carbohydrate-binding module; FN3, fibronectin type III domain-containing protein; Gal-db/Dock1, galactose-binding-like domain- and/or type I dockerin domain-containing protein; PL14, polysaccharide lyase of PL14 family; and VbB, vanadium-dependent bromoperoxidase.
The second cluster was much larger and encoded up to 21 proteins (Figure 4B). Identical clusters encoding 11 glycosides from the GH5 family (both endo-beta-1,4-mannanases and endoglucanases), three endo-beta-1,4-xylanases from the GH10 family, putative polysaccharide lyase (PL)14 with an unknown function, and several proteins containing putative CBM domains (galactose-binding-like domain, fibronectin type III, and type I dockerin) were observed in the genomes of AArc-m2/3/4 and AArc-xg1-1. Clusters in the genomes of KZCA124 and TS33 did not encode proteins of the PL14 family and fibronectin type III domain-containing proteins. Moreover, there were fewer genes of endo-glucanases from GH5 and GH10 enzymes. The largest endo-glucanase encoding cluster was found in the genome of N. cellulositropha AArcel5 with 10 x GH5 and GH9 endo-beta-1,4-glucanases, GH81 endo-beta-1,3-1,4-glucanase but lack the GH10 xylanases. Other clusters of the second type consisted of four to nine genes (mostly genes of glycosidases from GH5 and GH5_7). Both H. cellulosivorans and Halomicrobium salinisoli had a single gene encoding endo-beta-1,4-mannanases from the GH5_7 subfamily.
To predict the functions of the GH26 and GH5_7/8 subfamilies more reliably, a phylogenetic analysis of these families was performed. This analysis demonstrated that the GH26 encoded in the genomes of the AArc-m2/3/4T and AArc-xg1-1 strains are indeed endo-beta-1,4-mannanases belonging to the clade containing biochemically characterized beta-mannanases from bacteria and fungi (Figure 5). In addition to the catalytic GH26 domain, these enzymes contain the dockerin type I domain participating in the binding of substrate. Enzymes of KZCA124 and TS33, as well as N. cellulositropha and H. cellulosivorans, also belong to this clade (II). It is remarkable that there were more than 100 proteins of the GH26 family from halophilic archaea (and other archaea) belonging to another clade (I), which have a very low level of homology with the confirmed beta-1,4-mannanases. It is likely that these proteins together with the characterized beta-1,3-xylanases should be separated into a different subfamily of the GH26 or even into a separate family. Due to the complexity of the GH5 family, recently including 57 subfamilies (Supplementary Figure S3), analysis of these glycosidases was focused on clades containing endo-beta-mannosidases (GH5_7 and GH5_8 subfamilies). Both AArc-m2/3/4T and AArc-xg1-1 strains possess four enzymes from the GH5_7 subfamily (Figure 6), of which two of them contained additional domains (carbohydrate-binding module family 5/12, fibronectin type III or PKD, and concanavalin A-like lectin domains), which may enhance the hydrolytic activity against insoluble polysaccharides. In addition to the abovementioned enzymes, KZCA124 and TS33 had another protein of GH5_7 containing three fibronectin type III domains. Moreover, one enzyme of the GH5_8 subfamily was encoded in each genome (Figure 7). These proteins consisted of a catalytic domain and the CBM5/12. Both GH5_7 and GH5_8 enzymes from the studied haloarchaea were clustered together with biochemically characterized endo-beta-1,4-mannonases from bacteria, fungi, and plants indicating that haloarcheal proteins should also possess endo-mannonolytic activity.
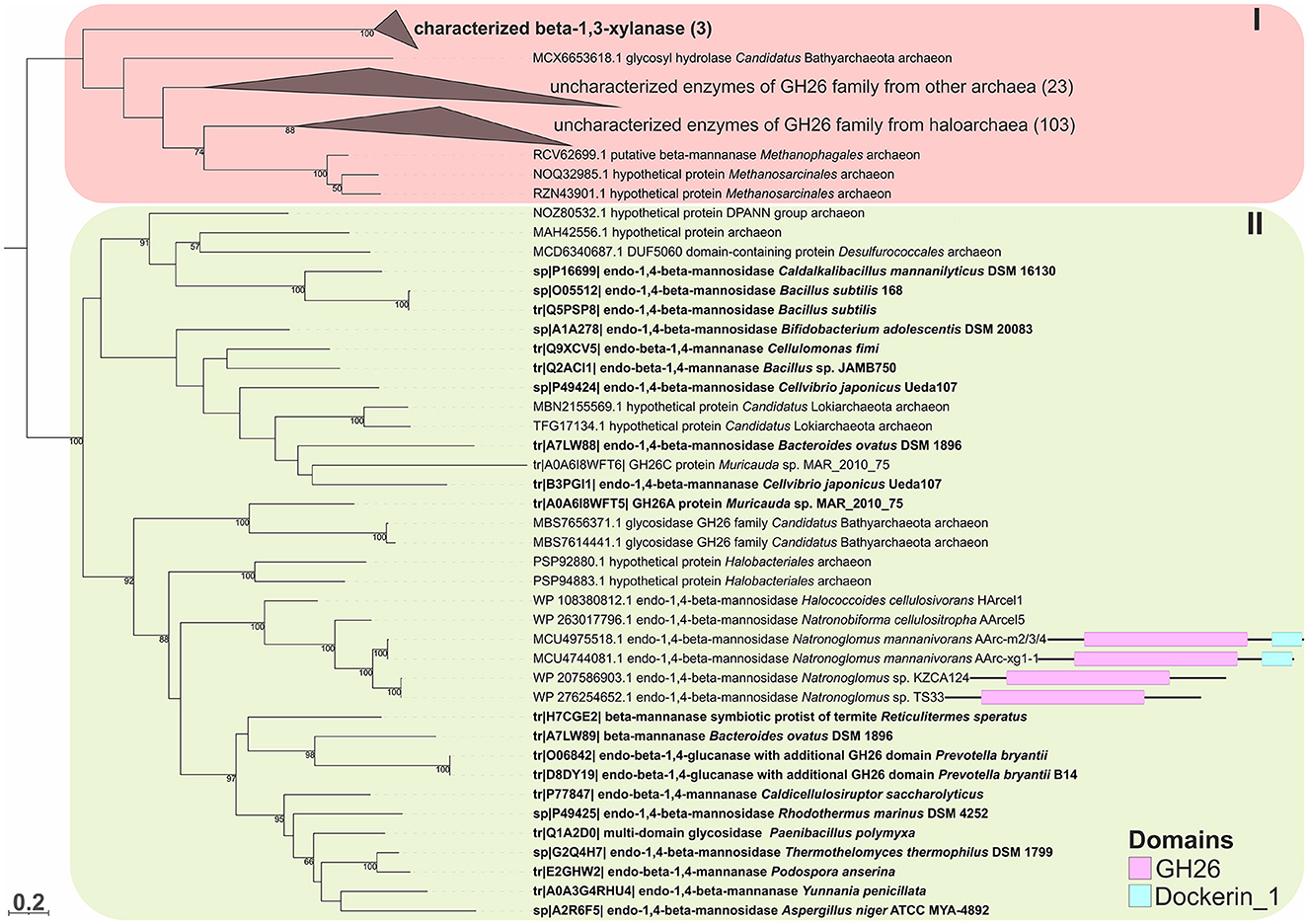
Figure 5. The phylogenetic analysis of the GH26 family endo-beta-1,4-mannanases (with domain architecture) of strains AArc-m2/3/4T and AArc-xg1-1 and closely related strains KZCA124 and TS33. Biochemically characterized enzymes are highlighted in bold. Domain architectures for endo-beta-1,4-mannosidases of AArc-m2/3/4, AArc-xg1-1 strains, and its closest relative are given. The numbers at nodes indicate the percentage of corresponding support values (only values higher than 50% are shown).
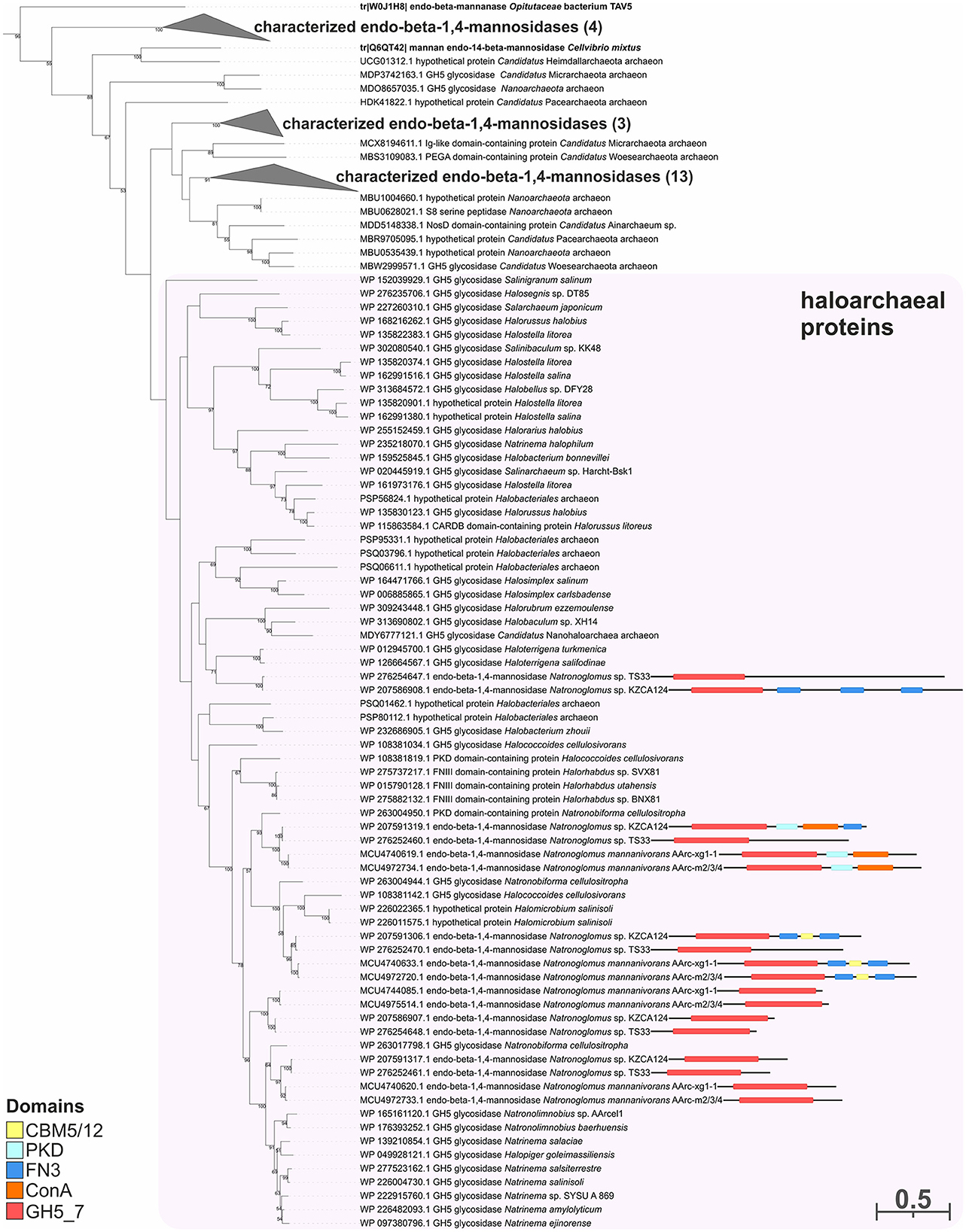
Figure 6. The phylogenetic analysis of enzymes of strains AArc-m2/3/4 and AArc-xg1-1 belonging to the GH5 family (the GH5_7 subfamily). Biochemically characterized enzymes are highlighted in bold. Domain architectures for endo-beta-1,4-mannosidases of AArc-m2/3/4, AArc-xg1-1 strains, and its closest relative (KZCA124 and TS33) are given. The numbers at nodes indicate the percentage of corresponding support values (only values higher than 50% are shown).
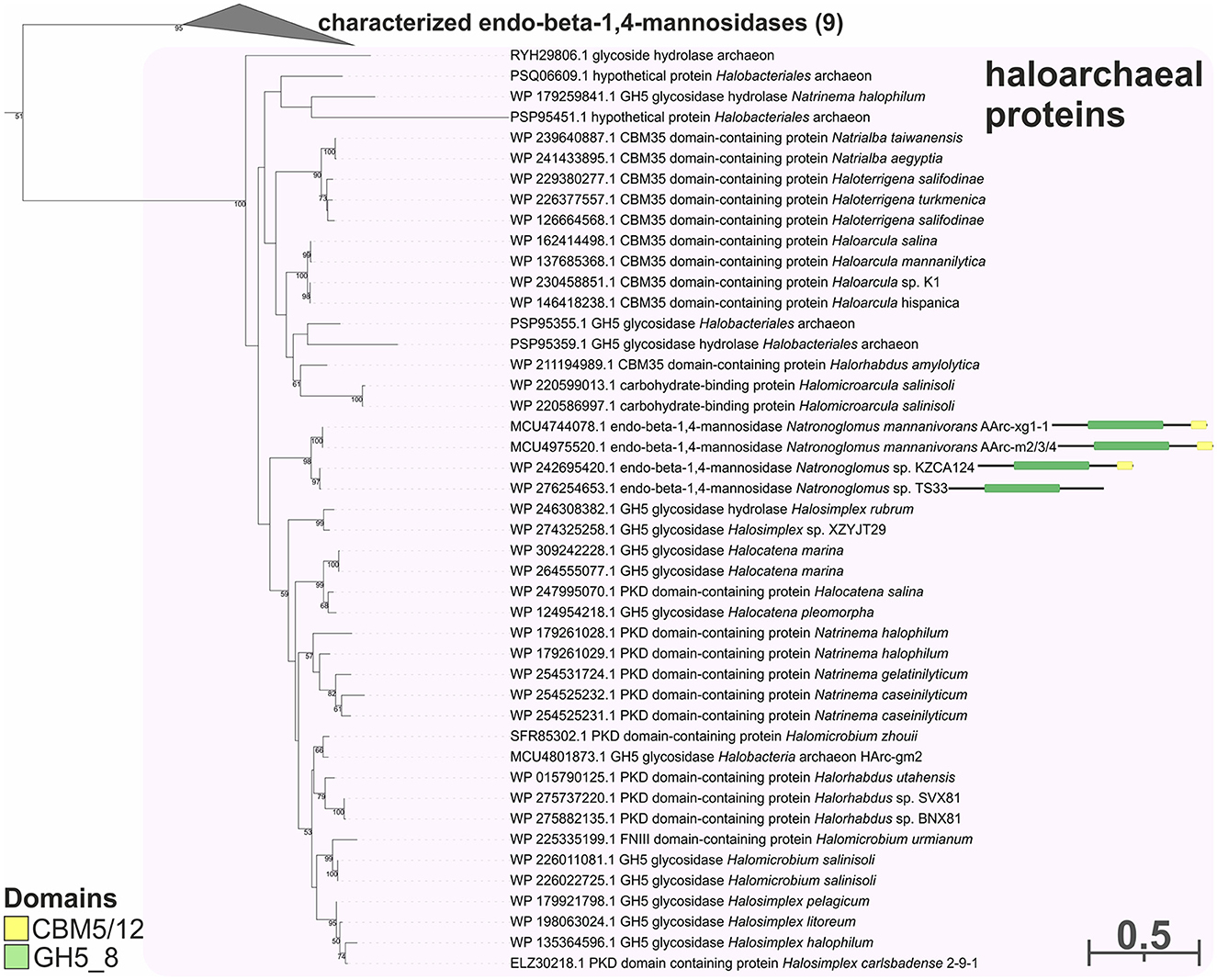
Figure 7. The phylogenetic analysis of enzymes of strains AArc-m2/3/4 and AArc-xg1-1 belonging to the GH5 family (the GH5_8 subfamily). Domain architectures for endo-beta-1,4-mannosidases of AArc-m2/3/4, AArc-xg1-1 strains, and its closest relative (KZCA124 and TS33) are given. The numbers at nodes indicate the percentage of corresponding support values (only values higher than 50% are shown).
Other repertoire of polysaccharide hydrolases encoded in the genomes
In addition to the mannanase-cellulase-xylanase GH families, both sequenced genomes also encoded a large repertoire of other polysaccharide-active enzymes including putative PL families listed in Supplementary Tables S3 and S4. The major difference between these groups of hydrolases is cell localization: the former are generally all extracellular, while many proteins from the latter group are cytoplasmic. The putative substrate for such enzymes includes cellulose/xyloglucan (GH3, GH5, and GH9 families), xylan/arabinoxylan (GH3, GH10, GH11, GH51, GH67, and GH115 families) arabinogalactan (GH2, GH30, GH42, GH43, GH51, and GH154 families), starch (GH13 family), acidic polysaccharides, such as polygalacturonan (PL1, PL22, and GH28), rhamnogalacturonan (PL11, PL26, PL42, GH4, and GH105), and alginate, and their oligomers. However, growth and hydrolytic activity were only observed with arabinoxylan and xyloglucan.
Central sugar metabolism
Taking into account that the GH2 family beta-mannosidases are intracellular and the strains are unable to grow on mannose, it is plausible to assume that mannan is hydrolyzed to mannooligosaccharides outside the cells, and then, the oligomers are imported into the cells. The phosphotransferase system (PTS) transporter genes were not found in the genomes of AArc-m2/3/4T and AArc-xg1-1 similar to cellulotrophic haloarchaea (Elcheninov et al., 2023). On the other hand, there were multiple genes of putative carbohydrate-specific ABC transporters (e.g., MCU497+ 1351–1354; 1378–1381; 3422–3426; 4795.1–4797.1; 4083–4081; and 5056–5061) in AArc-m2/3/4T, some of which likely import mannooligosaccharides into the cells, and furthermore, they are hydrolyzed by the GH2 beta-mannosidases. It is likely that mannose is oxidized during the side activity of glucose dehydrogenase or putative oxidoreductase (MCU4975802) distantly homologous to mannose-specific aldohexose dehydrogenase from Thermoplasma acidophilum (Nishiya et al., 2004). Under the action of mannonate dehydratases ManD (the genes of two of them, 5517 and 5522, were co-located in the gene cluster with mannanases), mannose is converted to 2-dehydro-3-deoxy-D-gluconate. Finally, it enters into the semi-phosphorylative Entner–Doudoroff (ED) pathway with the generation of glyceraldehyde-3-phosphate and pyruvate (Figure 8). Genes encoding enzymes of terminal steps in the ED pathway were also present: 2-dehydro-3-deoxy-D-gluconate kinase (3928 and 5533) and 2-dehydro-deoxy-6-phosphogluconate aldolase (2034).
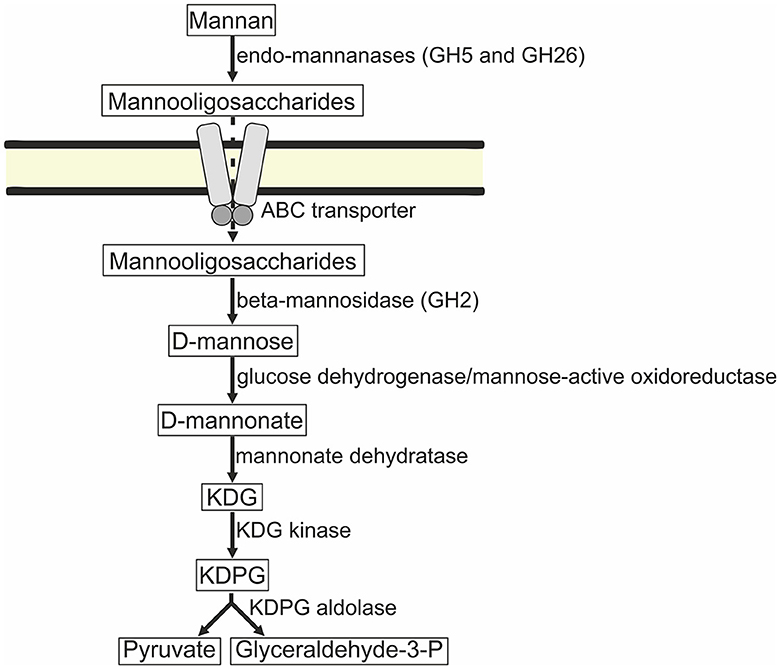
Figure 8. Predicted mannose catabolism pathway in strains AArc-m2/3/4 and AArc-xg1-1. ABC, ATP-binding cassette; KDG, 2-dehydro-3-deoxy-D-gluconate; KDPG, 2-dehydro-deoxy-6-phosphogluconate.
Other metabolically important traits
Other functionally important proteins from the genome of AArc-m2/3/4T are listed in Supplementary Table S5, including the ion-pH homeostasis, energy-related respiratory complexes, and some others. In particular, an incomplete denitrification pathway is encoded, including Cu-nitrite reductase NirK, archaeal type of NO-reductase qNor, and N2O reductase, but lacks nitrate reductase Nar. Despite this, the organisms did not grow anaerobically with either nitrite or N2O as acceptors (with cellobiose as substrate).
Taxonomy conclusion
Comparative properties of the novel isolates in relation to the type species of the most related genera are shown in Table 2. The most similar in properties from the three related genera is obviously N. cellulositropha, but it was unable to grow with glucomannan and galactomannans, and its sugar utilization spectrum is much more restricted. The other two species of the related genera (Natronosalvus and Saliphagus) lack the beta-mannanase genes in their genomes and, thus, most likely would be able to grow with this polysaccharide type. In addition, S. infecundisoli is a neutraphilic haloarchaeon unable to grow at high pH. Furthermore, the mannan-utilizing natronoarchaea nearly lacked glycolipids, while in Natronosalvus and Saliphagus, several glycolipids constituted a large fraction of the membrane polar lipids, and the latter also has sulfolipid PGS not usually present in the soda lake natronoarchaea (Bale et al., 2021).
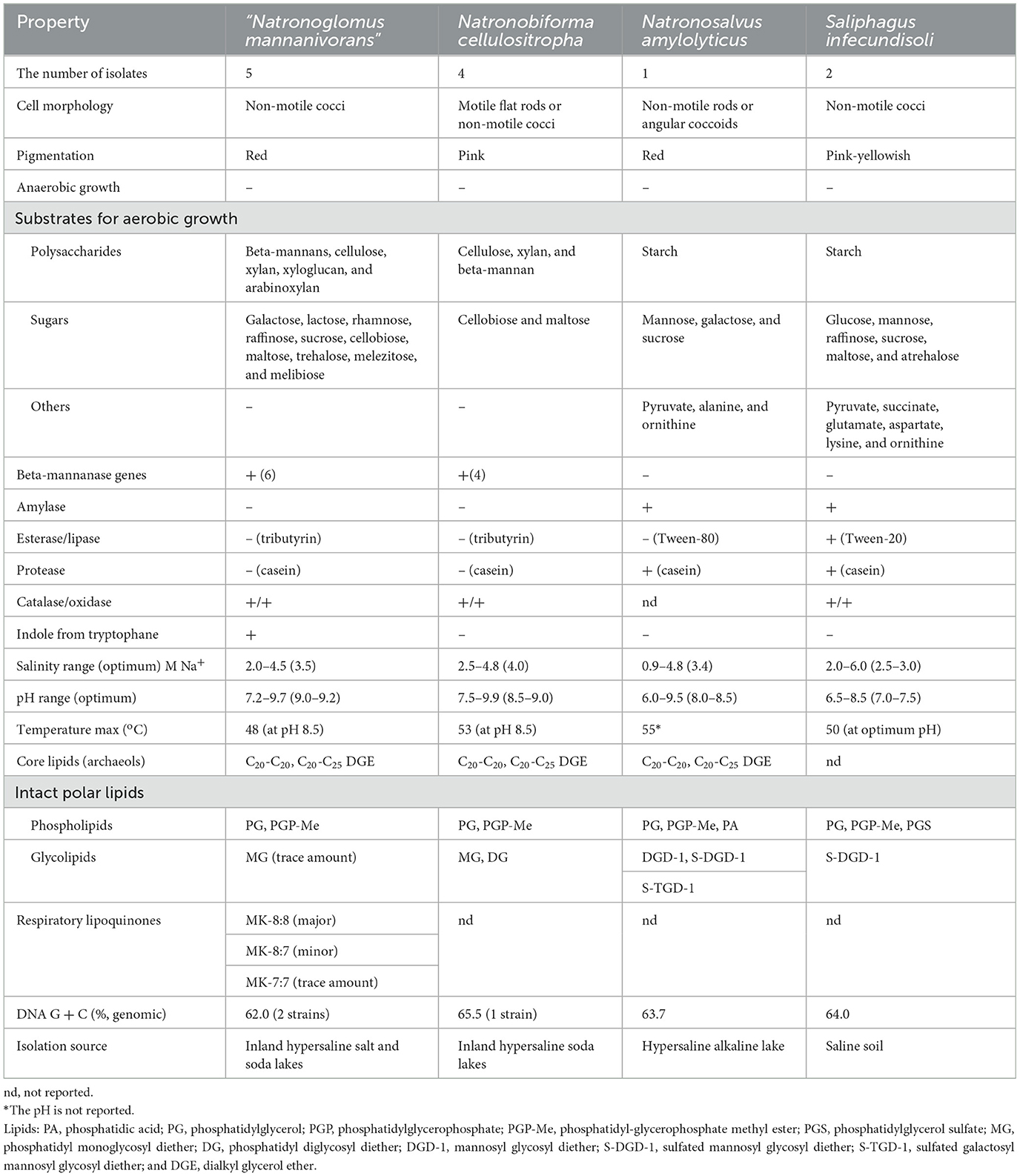
Table 2. Comparative properties of the β-mannan utilizing isolates with the closest related genera from the family Natrialbaceae (Sorokin et al., 2018; Yin et al., 2018; Tan et al., 2023).
In conclusion, taking into account the unique functional specialization and distant phylogeny, the beta-mannan-utilizing natronoarchaea from Siberian hypersaline soda lakes are proposed to form a new genus and species Natronoglomus mannanivorans.
Description of Natronoglomus mannanivorans gen. nov., sp. nov.
Natronoglomus gen. nov.
Na.tro.no.glo'mus. Gr. neut. n. natron arbitrarily derived from Arabic n. natrun or natron, soda; L. neut. n. glomus, a ball; N.L. neut. n. Natronoglomus, a coccoid natronoarchaeon.
Natrononoarchaea from hypersaline lakes with mostly coccoid cells. Aerobic organoheterotrophs with the ability to utilize insoluble beta-mannans and cellulose as growth substrates. Extremely halophilic and moderately alkaliphilic. The dominant polar membrane lipids are phosphatidylglycerophosphate methyl ether (PGP-Me) and phosphatidylglycerol (PG) with C20-C20 and C20-C25 archaeol cores. The genus belongs to the Natrialbaceae family, class Halobacteria. A three-letter abbreviation is Ngm.
Natronoglomus mannanivorans sp. nov.
man.na.ni.vo'rans. N.L. neut. n. mannanum, mannan; L. pres. part. vorans, devouring; N.L. part. adj. mannanivorans, mannan devouring.
The cells are mostly non-motile cocci of 0.8–1.2 μm producing red pigments. The cells grown on mannan have a thick cell wall and extended pseudoperiplasm. The cells lyze in distilled water. The colony morphology varied depending on the substrate. On insoluble polysaccharides, the colonies were mostly pale-pink, thin, spreading, and irregular, up to 4 mm; on soluble sugars, the colonies were pink, more regular, and convex, up to 3 mm. The core membrane diether lipids are dominated by C20-C20 DGE (archaeol) and C20-C25 DGE (extended archaeol). The polar lipid head groups include phosphatidylglycerolphosphate methyl ester (PGP-Me) and phosphatidylglycerol (PG). The dominant respiratory menaquinone is MK-8:8, with the MK-8:7 second in abundance. The species are obligatory aerobic saccharolytic heterotrophs that are able to grow with insoluble beta-1,4-mannan, glucomannan, and cellulose. Sugars supporting the growth include galactose, rhamnose, raffinose, lactose, sucrose, cellobiose, maltose, trehalose, melezitose, and melibiose. Weak growth was observed on glycerol and pyruvate. Organic compounds tested (in the type strain) but not utilized included fructose, arabinose, ribose, xylose, sugar alcohols, acetate, ethanol, lactate, succinate, fumarate, malate, glycine, aspartate, glutamate, arginine, yeast extract, and peptone from casein. Ammonium and urea serve as the nitrogen source. Oxidase and catalase are positive. Mesophilic, with a maximum growth temperature of 48°C, is a low Mg-demanding, extreme halophile, with a range of Na+ for growth from 2.5 to 4.5 M (optimum at 3.5 M), and a facultative alkaliphile, with a pH range from 6.8 to 9.6 for growth (optimum at 9–9.2). The G + C content of the DNA is 62% (two genomes), and the habitat is hypersaline salt lakes. The type strain (AArc-m2/3/4T= JCM 34861 = UQM 41565) was isolated from sediments of hypersaline soda lakes in the Kulunda Steppe (Altai, Russia). The species also includes other four closely related isolates, one of which is deposited in JCM (AArc-xg1-1 = JCM 34866). The draft genome assemblies' accession numbers of strains AArc-m2/3/4T and AArc-xg1-1 in the GenBank are GCA_025517485 and GCA_025517495.
Data availability statement
The datasets presented in this study can be found in online repositories. The names of the repository/repositories and accession number(s) can be found in the article/Supplementary material.
Author contributions
DS: Conceptualization, Investigation, Writing—original draft. AE: Investigation, Writing—original draft. NB: Investigation, Writing—original draft. JS: Writing—original draft. IK: Writing—original draft.
Funding
The author(s) declare financial support was received for the research, authorship, and/or publication of this article. All Russian authors were supported by the Russian Ministry of Higher Education and Science. DS was also partially supported by the Gravitation-SIAM Program of the Dutch Ministry of Education and Sciences (grant no. 24002002). NB and JS-D acknowledge support from the ERC Horizon 2020 Program (grant agreement no. 694569 – MICROLIPIDS).
Conflict of interest
The authors declare that the research was conducted in the absence of any commercial or financial relationships that could be construed as a potential conflict of interest.
The author(s) declared that they were an editorial board member of Frontiers, at the time of submission. This had no impact on the peer review process and the final decision.
Publisher's note
All claims expressed in this article are solely those of the authors and do not necessarily represent those of their affiliated organizations, or those of the publisher, the editors and the reviewers. Any product that may be evaluated in this article, or claim that may be made by its manufacturer, is not guaranteed or endorsed by the publisher.
Supplementary material
The Supplementary Material for this article can be found online at: https://www.frontiersin.org/articles/10.3389/fmicb.2024.1364606/full#supplementary-material
References
Aliyu, H., Lebre, P., Blom, J., Cowan, D., and De Maayer, P. (2016). Phylogenomic re-assessment of the thermophilic genus Geobacillus. Syst. Appl. Microbiol. 39, 527–533. doi: 10.1016/j.syapm.2016.09.004
Bale, N. J., Ding, S., Hopmans, E. C., Villanueva, L., and Boschman, R. C. (2021). Lipidomics of environmental microbial communities. I: visualization of specific niches using untargeted analysis of high-resolution mass spectrometry data. Front. Microbiol. 12:659302. doi: 10.3389/fmicb.2021.659302
Buchfink, B., Xie, C., and Huson, D. H. (2015). Fast and sensitive protein alignment using DIAMOND. Nat. Methods 12, 59–60. doi: 10.1038/nmeth.3176
Capella-Gutiérrez, S., Silla-Martínez, J. M., and Gabaldón, T. (2009). trimAl: a tool for automated alignment trimming in large-scale phylogenetic analyses. Bioinformatics 25, 1972–1973. doi: 10.1093/bioinformatics/btp348
Chaumeil, P. A., Mussig, A. J., Hugenholtz, P., and Parks, D. H. (2019). GTDB-Tk: a toolkit to classify genomes with the genome taxonomy. Bioinformatics 36, 1925–1927. doi: 10.1093/bioinformatics/btz848
de la Haba, R.R., Minegishi, H., Kamekura, M., Shimane, Y., and Ventosa, A. (2021). Phylogenomics of Haloarchaea: the controversy of the genera Natrinema-Haloterrigena. Front. Microbiol. 12:740909. doi: 10.3389/fmicb.2021.740909
Elcheninov, A. G., Ugolkov, Y. A., Elizarov, I. M., Klyukina, A. A., Kublanov, I. V., Sorokin, D. Y., et al. (2023). Cellulose metabolism in halo(natrono)archaea: a comparative genomics study. Front. Microbiol. 14:1112247. doi: 10.3389/fmicb.2023.1112247
Enomoto, S., Shimane, Y., Ihara, K., Kamekura, M., Itoh, T., Ohkuma, M., et al. (2020). Haloarcula mannanilytica sp. nov., a galactomannan-degrading haloarchaeon isolated from commercial salt. Int. J. Syst. Evol. Microbiol. 70, 6331–6337. doi: 10.1099/ijsem.0.004535
Katoh, K., Rozewicki, J., and Yamada, K. D. (2017). MAFFT online service: multiple sequence alignment, interactive sequence choice and visualization. Brief. Bioinform. 20, 1160–1166. doi: 10.1093/bib/bbx108
Letunic, I., and Bork, P. (2019). Interactive Tree Of Life (iTOL) v4: recent updates and new developments. Nucleic Acids Res. 47, W256–W259. doi: 10.1093/nar/gkz239
Mistry, J., Finn, R. D., Eddy, S. R., Bateman, A., and Punta, M. (2013). Challenges in homology search: HMMER3 and convergent evolution of coiled-coil regions. Nucleic Acids Res. 41:e121. doi: 10.1093/nar/gkt263
Nishiya, Y., Tamura, N., and Tamura, T. (2004). Analysis of bacterial glucose dehydrogenase homologs from thermoacidophilic archaeon Thermoplasma acidophilum: finding and characterization of aldohexose dehydrogenase. Biosci. Biotechnol. Biochem. 68, 2451–2456. doi: 10.1271/bbb.68.2451
Orata, F. D., Meier-Kolthoff, J. P., Sauvageau, D., and Stein, L. Y. (2018). Phylogenomic analysis of the gammaproteobacterial methanotrophs (order Methylococcales) calls for the reclassification of members at the genus and species levels. Front. Microbiol. 9:3162. doi: 10.3389/fmicb.2018.03162
Paysan-Lafosse, T., Blum, M., Chuguransky, S., Grego, T., Pinto, B. L., Salazar, G. A., et al. (2023). InterPro in 2022. Nucleic Acids Res. 51, D418–D427. doi: 10.1093/nar/gkac993
Qin, Q.-L., Xie, B.-B., Zhang, X.-Y., Chen, X.-L., Zhou, B.-C., Zhou, J., et al. (2014). A proposed genus boundary for the prokaryotes based on genomic insights. J. Bacteriol. 196, 2210–2215. doi: 10.1128/JB.01688-14
Rinke, C., Chuvochina, M., Mussig, A. J., Chaumeil, P.-A., Davín, A. A., Waite, D. W., et al. (2021). A standardized archaeal taxonomy for the Genome Taxonomy Database. Nat. Microbiol. 6, 946–959. doi: 10.1038/s41564-021-00918-8
Shimane, Y., Hatada, Y., Minegishi, H., Mizuki, T., Echigo, A., Miyazaki, M., et al. (2010). Natronoarchaeum mannanilyticum gen. nov., sp. nov., an aerobic, extremely halophilic archaeon isolated from commercial salt. Int. J. Syst. Evol. Microbiol. 60, 2529–2534. doi: 10.1099/ijs.0.016600-0
Sorokin, D. Y., Elcheninov, A. G., Khijniak, T. V., Kolganova, T. V., and Kublanov, I. V. (2022a). Selective enrichment on a wide polylsaccharide spectrum allowed isolation of novel metabolic and taxonomic groups of haloarchaea from hypersaline lakes. Front. Microbiol. 13:1059347. doi: 10.3389/fmicb.2022.1059347
Sorokin, D. Y., Elcheninov, A. G., Toshchakov, S. V., Bale, N. J., Sinninghe Damsté, J. S., Khijniak, T. V., et al. (2019b). Natrarchaeobius chitinivorans gen. nov., sp. nov., Natrarchaeobius halalkaliphilus sp. nov., alkaliphilic, chitin-utilizing haloarchaea from hypersaline alkaline lakes. Syst. Appl. Microbiol. 42, 309–318. doi: 10.1016/j.syapm.2019.01.001
Sorokin, D. Y., Elcheninov, A. S., Merkel, A. Y., Bale, N. J., Sinninghe Damsté, J. S., Kublanov, I. V., et al. (2023). Halapricum hydrolyticum, a beta-1,3-glucan utilizing haloarchaeon from hypersaline lakes. Syst. Appl. Microbiol. 46:126471. doi: 10.1016/j.syapm.2023.126471
Sorokin, D. Y., Khijniak, T. V., Kostrikina, N. A., Elcheninov, A. G., Toshchakov, S. V., Bale, N. J., et al. (2018). Natronobiforma cellulositropha gen. nov., sp. nov., a novel haloalkaliphilic member of the family Natrialbaceae (class Halobacteria) from hypersaline alkaline lakes. Syst. Appl. Microbiol. 41, 355–362. doi: 10.1016/j.syapm.2018.04.002
Sorokin, D. Y., Khijniak, T. V., Kostrikina, N. A., Elcheninov, A. G., Toshchakov, S. V., Bale, N. J., et al. (2019a). Halococcoides cellulosivorans gen. nov., sp. nov., an extremely halophilic cellulose-utilizing haloarchaeon from hypersaline lakes. Int. J. Syst. Evol. Microbiol. 69, 1327–1335. doi: 10.1099/ijsem.0.003312
Sorokin, D. Y., Toshchakov, S. V., Kolganova, T. V., and Kublanov, I. V. (2015). Halo(natrono)archaea isolated from hypersaline lakes utilize cellulose and chitin as growth substrates. Front. Microbiol. 6:942. doi: 10.3389/fmicb.2015.00942
Sorokin, D. Y., Yakimov, M. M., Messina, E., Merkel, A. Y., Koenen, M., Bale, N. J., et al. (2022b). Natranaeroarchaeum sulfidigenes gen. nov., sp. nov., carbohydrate-utilizing sulfur-respiring haloarchaeon from hypersaline soda lakes, a member of a new family Natronoarchaeaceae fam. nov. in the order Halobacteriales. Syst. Appl. Microbiol. 45:126356. doi: 10.1016/j.syapm.2022.126356
Stamatakis, A. (2014). RAxML version 8: a tool for phylogenetic analysis and post-analysis of large phylogenies. Bioinformatics 30, 1312–1313. doi: 10.1093/bioinformatics/btu033
Tan, S., Cheng, M., Li, X.-X., Hu, Y., Ma, X., Hou, J., et al. (2023). Natronosalvus halobius gen. nov., sp. nov., Natronosalvus caseinilyticus sp. nov., Natronosalvus vescus sp. nov., Natronosalvus rutilus sp. nov., Natronosalvus amylolyticus sp. nov., halophilic archaea isolated from salt lakes and soda lakes. Int. J. Syst. Evol. Microbiol. 73:006036. doi: 10.1099/ijsem.0.006036
Wirth, J. S., and Whitman, W. B. (2018). Phylogenomic analyses of a clade within the Roseobacter group suggest taxonomic reassignments of species of the genera Aestuariivita, Citreicella, Loktanella, Nautella, Pelagibaca, Ruegeria, Thalassobius, Thiobacimonas and Tropicibacter, and the proposal. Int. J. Syst. Evol. Microbiol. 68, 2393–2411. doi: 10.1099/ijsem.0.002833
Yin, X.-Q., Liu, B.-B., Chu, X., Salam, N., Li, X., Wang, Z.-W., et al. (2018). Saliphagus infecundisoli gen. nov., sp. nov., an extremely halophilic archaeon isolated from a saline soil. Int. J. Syst. Evol. Microbiol. 67, 4154–4160. doi: 10.1099/ijsem.0.002270
Keywords: hypersaline lakes, haloarchaea, glucomannan, galactomannan, beta-1,4-mannan
Citation: Sorokin DY, Elcheninov AG, Bale NJ, Sinninghe Damsté JS and Kublanov IV (2024) Natronoglomus mannanivorans gen. nov., sp. nov., beta-1,4-mannan utilizing natronoarchaea from hypersaline soda lakes. Front. Microbiol. 15:1364606. doi: 10.3389/fmicb.2024.1364606
Received: 02 January 2024; Accepted: 14 February 2024;
Published: 12 March 2024.
Edited by:
Mohammad Ali Amoozegar, University of Tehran, IranReviewed by:
Heng-Lin Cui, Jiangsu University, ChinaDoug Bartlett, University of California, San Diego, United States
Copyright © 2024 Sorokin, Elcheninov, Bale, Sinninghe Damsté and Kublanov. This is an open-access article distributed under the terms of the Creative Commons Attribution License (CC BY). The use, distribution or reproduction in other forums is permitted, provided the original author(s) and the copyright owner(s) are credited and that the original publication in this journal is cited, in accordance with accepted academic practice. No use, distribution or reproduction is permitted which does not comply with these terms.
*Correspondence: Dimitry Y. Sorokin, soroc@inmi.ru; d.sorokin@tudelft.nl