- 1Department of Neuroscience, Central Clinical School, Monash University, Melbourne, VIC, Australia
- 2Division of Infectious Disease and Immune Defense, Walter and Eliza Hall Institute of Medical Research, Parkville, VIC, Australia
- 3Monash Health Translation Precinct, Monash University, Melbourne, VIC, Australia
- 4Health Sciences, Vancouver Island University, Nanaimo, BC, Canada
Introduction: Post-traumatic epilepsy (PTE) is a debilitating chronic outcome of traumatic brain injury (TBI), and neuroinflammation is implicated in increased seizure susceptibility and epileptogenesis. However, how common clinical factors, such as infection, may modify neuroinflammation and PTE development has been understudied. The neurotropic parasite, Toxoplasma gondii (T. gondii) incurably infects one-third of the world’s population. Thus, many TBI patients have a pre-existing T. gondii infection at the time of injury. T. gondii infection results in chronic low-grade inflammation and altered signaling pathways within the brain, and preliminary clinical evidence suggest that it may be a risk factor for epilepsy. Despite this, no studies have considered how a pre-existing T. gondii infection may alter the development of PTE.
Methods: This study aimed to provide insight into this knowledge gap by assessing how a pre-existing T. gondii infection alters susceptibility to, and severity of, pentylenetetrazol (PTZ)-induced seizures (i.e., a surrogate marker of epileptogenesis/PTE) at a chronic stage of TBI recovery. We hypothesized that T. gondii will increase the likelihood and severity of seizures following PTZ administration, and that this would occur in the presence of intensified neuroinflammation. To test this, 6-week old male and female C57BL/6 Jax mice were intraperitoneally injected with 50,000 T. gondii tachyzoites or with the PBS vehicle only. At 12-weeks old, mice either received a severe TBI via controlled cortical impact or sham injury. At 18-weeks post-injury, mice were administered 40 mg/kg PTZ and video-recorded for evaluation of seizure susceptibility. Fresh cortical tissue was then collected for gene expression analyses.
Results: Although no synergistic effects were evident between infection and TBI, chronic T. gondii infection alone had robust effects on the PTZ-seizure response and gene expression of markers related to inflammatory, oxidative stress, and glutamatergic pathways. In addition to this, females were more susceptible to PTZ-induced seizures than males. While TBI did not impact PTZ responses, injury effects were evident at the molecular level.
Discussion: Our data suggests that a pre-existing T. gondii infection is an important modifier of seizure susceptibility independent of brain injury, and considerable attention should be directed toward delineating the mechanisms underlying this pro-epileptogenic factor.
1. Introduction
Traumatic brain injury (TBI) greatly contributes to morbidity and mortality worldwide, and one of the most debilitating long-term consequences of TBI is the development of post-traumatic epilepsy (PTE) (Christensen et al., 2009; GBD 2016 Neurology Collaborators, 2019). PTE is defined as one or more unprovoked seizures that occur at least one week after TBI (Verellen and Cavazos, 2010). PTE accounts for 10–20% of acquired epilepsy in the general population and it is reported that between 4 and 50% of individuals with TBI develop PTE (Degrauw et al., 2018; Tubi et al., 2019; Pease et al., 2022). PTE diagnosis is also associated with heightened morbidity and mortality (Burke et al., 2021; Karlander et al., 2022; Ngadimon et al., 2022).
There is evidence that neuroinflammation can increase seizure susceptibility and the likelihood of PTE development (Webster et al., 2017; Sharma et al., 2019). A role for microglia, astrocytes, chemokines, and cytokines have been described in the progression of PTE (Lu et al., 2021; Radpour et al., 2022). In addition, pre-clinical studies indicate that peripheral inflammation and cerebral infection can alter susceptibility to seizures via either a primed immune environment or changes to brain pathophysiology (Ho et al., 2015; Grauncke et al., 2016; Ssentongo et al., 2017; Huang et al., 2018, 2022). However, in the context of PTE, infection has only been considered as a “second-hit” on the back of the neuroinflammatory processes of TBI, rather than as a pre-existing insult or concurrent factor that can modify the neuroinflammatory environment, neural connectivity, and gross anatomy (Sharma et al., 2021; Wang et al., 2021).
Toxoplasma gondii (T. gondii), which belongs to the Apicomplexa phylum, is estimated to chronically and incurably infect one-third of the population worldwide (Montoya and Liesenfeld, 2004). As such, a significant proportion of TBI patients may have a pre-existing infection at the time of injury. As an obligate intracellular parasite, T. gondii invades host cells to survive and replicate, and once in the central nervous system, T. gondii can differentiate into an encysted and slow replicating form that is resistant to immune clearance. A large majority of clinical cases of chronic T. gondii infection occur with type II strains such as Prugniaud (Pru) or ME49, and chronic infection is characterized by low-grade persistent neuroinflammation (Howe and Sibley, 1995; Bergersen et al., 2021; Egorov et al., 2021). T. gondii infection has also been linked to changes in glutamate excitotoxicity and spontaneous seizures in rodent models, and some preliminary clinical findings suggest it as a risk factor for epilepsy (Brooks et al., 2015; David et al., 2016; Sadeghi et al., 2019; Alizadeh Khatir et al., 2021). Yet, no studies to date have investigated T. gondii as a modifier of epileptogenesis in the context of TBI.
Therefore, this study aimed to assess how a pre-existing T. gondii infection alters susceptibility to, and severity of, pentylenetetrazol (PTZ)-induced seizures (i.e., a surrogate indicator of epileptogenesis/PTE) at a chronic stage of TBI recovery. We hypothesized that combined, T. gondii infection and TBI would result in exacerbated seizure susceptibility and severity, and that this would be coupled with an amplified neuroinflammatory environment.
2. Materials and methods
2.1. Animals
A total of 96 C57BL/6J (Jax) (49 male, 47 female) mice were obtained from the Alfred Medical Research and Education Precinct (AMREP) Animal Services (Melbourne, Australia) for use in this study. Mice were group-housed in ventilated Optimice® cages (3–6 mice/cage/sex) under a 12-h light/dark cycle and were given access to food and water ad libitum for the duration of the experiment. All procedures were approved by the AMREP Animal Ethics Committee (#E/2005/2020/M) and performed in accordance with the guidelines of the Australian Code of Practice for the Care and Use of Animals for Scientific Purposes by the Australian National Health and Medical Research Council.
2.2. Experimental design
At 6-weeks of age, mice were randomly allocated to receive either a single intraperitoneal (i.p.) injection of T. gondii tachyzoites or vehicle only (Figure 1A). All mice received 100 μg/mL sulfadiazine sodium (Sigma Aldrich, Burlington, MA, USA) in their drinking water on days 5 to 10 after injection to aid recovery from the acute stage of infection. This treatment is commonly used to control tachyzoite proliferation during active toxoplasmosis and reduce animal death throughout the acute stage of infection (Ochiai et al., 2015; Zhou et al., 2019). Of 48 mice (25 male, 23 female) infected with T. gondii, 2 (1 male, 1 female) did not recover from the acute stage of infection (4.17% total mortality). Mice were allowed 6-weeks after injection for a chronic T. gondii infection to establish, and at this point, received either a TBI that was induced via the controlled cortical impact (CCI) model or a sham injury. Therefore, this study was comprised of four experimental groups per sex: Vehicle + Sham; T. gondii + Sham; Vehicle + CCI; and T. gondii + CCI. Some mice (2 T. gondii + CCI males, 1 T. gondii + Sham female) died prematurely to the 18-week post-injury endpoint. As such, 91 mice underwent PTZ injection and endpoint at 18-weeks post-injury. Investigators were blinded to group allocations immediately following injury throughout all experimentation and data analyses. Body weights were monitored throughout the study (Figure 1B). Males weighed more than females throughout the study. By 6-weeks post-injection, T. gondii mice weighed significantly less than vehicle groups, and this difference was sustained until PTZ administration/endpoint.
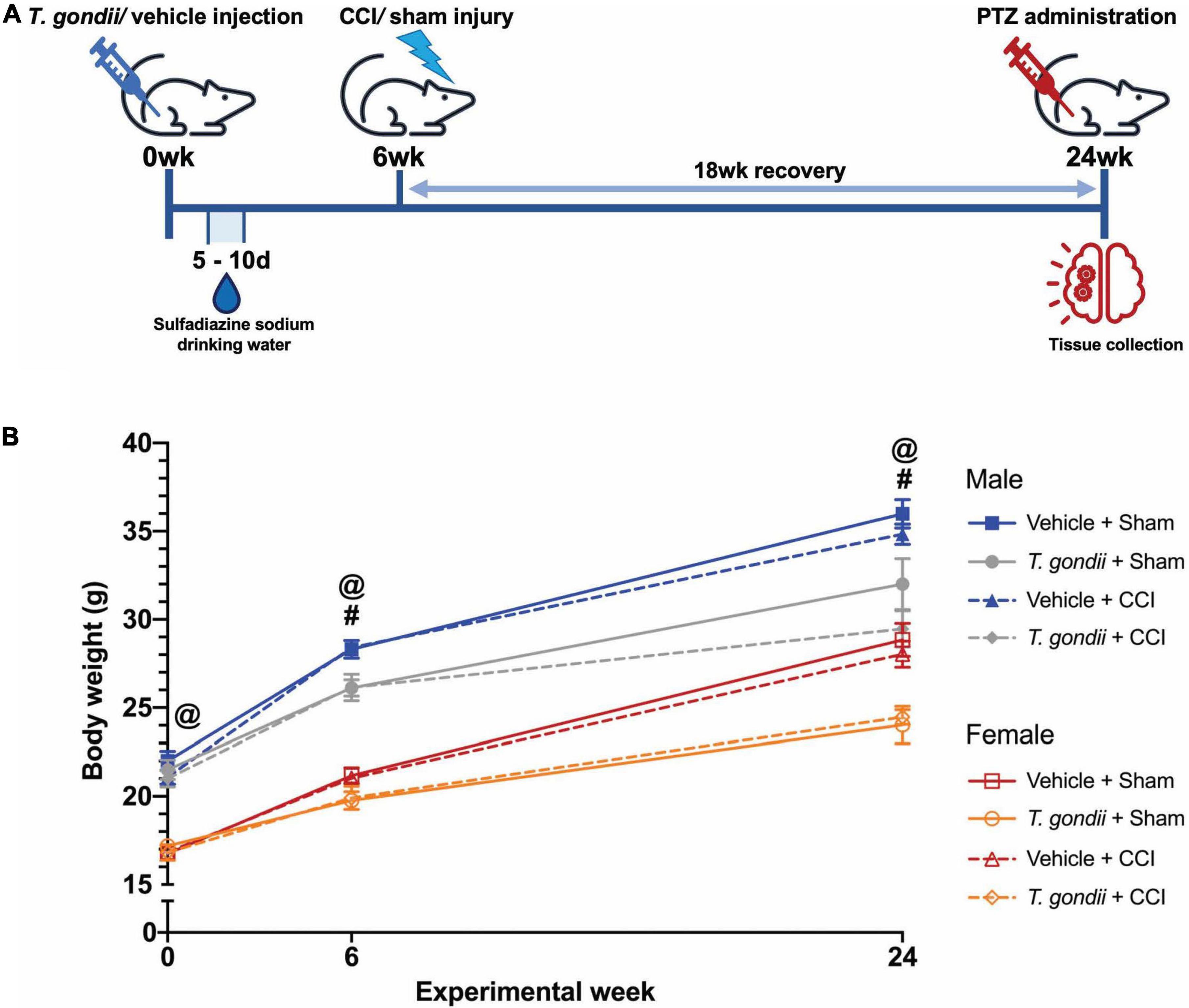
Figure 1. Study timeline. (A) All mice were injected with either Toxoplasma gondii or vehicle only. Six-weeks were allowed to pass to establish a chronic infection. At this point, mice received either a controlled cortical impact (CCI) or sham injury. After an 18-week recovery (i.e., at 24-weeks from the initial T. gondii or vehicle injection), mice underwent pentylenetetrazol (PTZ) administration for assessment of seizure susceptibility immediately prior to euthanasia and tissue collection. (B) Body weight significantly differed between male and female mice throughout the study, with males weighing more than females. By 6-weeks post-injection, T. gondii mice weighed significantly less than vehicle groups, and this difference was sustained until PTZ administration/endpoint. #T. gondii-infected mice significantly differ from vehicle mice, @female mice significantly differ from male mice, p < 0.05. n = 10–12/group/sex. Data shown is mean ± SEM.
2.3. Chronic Toxoplasma gondii infection
Toxoplasma gondii (Pru:tdTomato) tachyzoites were maintained by passage on human foreskin fibroblasts and resuspended in Dulbecco’s phosphate buffered saline (DPBS) to a concentration of 50,000 T. gondii tachyzoites per 200 μL DPBS (Tyebji et al., 2019). Mice allocated to T. gondii groups subsequently received a single i.p., injection of 50,000 tachyzoites and were monitored across a 6-week period for sickness behaviors as previously described (Tyebji et al., 2019). Mice allocated to vehicle groups received 200 μL DPBS only and were similarly monitored across a 6-week period.
2.4. Controlled cortical impact
The CCI model in mice reproduces several pathophysiological and functional features commonly seen in TBI patients (Xiong et al., 2013). CCI was performed at 6-weeks post-injection (12-weeks old) as previously described (Webster et al., 2019). Anesthesia was induced by 4% isoflurane in 1 L/min oxygen and maintained for surgery at 1.5–2% isoflurane via nose cone. Briefly, mice were stabilized in a stereotaxic frame and a midline incision was made to expose the skull. A ∼3 mm diameter craniotomy above the left parietal lobe was performed with a microdrill (0.6 mm drill bit) at a position of 1 mm posterior to Bregma, 1 mm lateral to the midline-sagittal suture, and 1 mm anterior to Lambda. Severe injury parameters were set using an electronic CCI device (Custom Design and Fabrication Inc., Sandston, VA, USA) at 4.5 m/s velocity, 1.71 mm depth, and 150 ms dwell time. Sham injuries were performed as described above, without the delivery of an impact to the parietal lobe. All surgical tools and the impactor tip were cleaned with 80% ethanol between animals and tools were additionally sterilized using a hot bead sterilizer.
2.5. Pentylenetetrazol seizure induction and seizure susceptibility analysis
As a non-competitive γ-aminobutyric acid (GABA)A receptor antagonist, PTZ is able to close chloride channels and prevent hyperpolarization, leading to continuous stimulation of cortical neurons and convulsions (Viswanatha et al., 2020). Prior to euthanasia at 18-weeks post-injury, mice were administered 40 mg/kg PTZ i.p. (Sigma Aldrich) and video-recorded for a period of up to 30 min for evaluation of seizure susceptibility, using a modified seizure severity score to assess behavioral responses (Table 1; Van Erum et al., 2019; Sharma et al., 2021). This dose was chosen based on previous work by Sharma et al. (2019) and Van Erum et al. (2019), which demonstrated a wide range of behavioral responses at 40 mg/kg PTZ i.p., and on average mice reach a score of 4. Briefly, responses assessed ranged from normal behavior (score 0) to tonic extension and death (score 7), with scores of 4–7 being considered as generalized convulsive seizures. As such, seizure latency was considered as the time taken to reach a score greater than or equal to 4. Seizure duration was considered as the total time in which a mouse exhibited scores 4 to 6, and if a score of 7 was reached (i.e., premature death), a maximum value of 1,800 s was assigned.
2.6. Tissue collection
Mice were euthanized at 18-weeks post-surgery immediately following PTZ challenge (i.e., at a maximum of 30 min from PTZ administration), with a single i.p., injection of sodium pentobarbitone (80 mg/kg; Lethabarb; Virbac, Australia). Next, fresh brain tissue from the ipsilateral parietal cortex was collected for gene expression analysis. All samples were frozen on dry ice and stored at −80°C prior to analysis.
In addition, a subset of mice underwent transcardial perfusion with ice-cold sterile saline (0.9% NaCl w/v) followed by 4% paraformaldehyde (PFA) to fix the brain tissue for confirmation of brain damage due to CCI via structural MRI. Brains were post-fixed in 4% PFA overnight at 4°C, washed twice in 1x PBS, then transferred to 1x PBS for storage at 4°C prior to analysis.
2.7. Gene expression analysis
Gene expression analysis was used to examine genes related to immune, oxidative stress, glutamate, and apoptotic pathways. Total RNA was isolated by hand from 20 mg of ipsilateral parietal cortex tissue using a RNeasy® Mini Kit (Qiagen, Germantown, MD, USA). A total of 200 ng of yielded RNA proceeded to cDNA synthesis using Quantabio qScript XLT cDNA SuperMix (Quantabio). Multiplex qPCR was performed with Fluidigm BioMark™ HD. For each sample, 1.25 μL of the resulting cDNA was combined with 3.75 μL of Sample Pre Mix (Life Technologies TaqMan® PreAmp Master Mix and Pooled Taqman assays) and pre-amplified for 14 cycles. The reaction products were diluted 1:5 and loaded onto the Gene expression IFC according to Fluidigm® IFC Standard Taqman Gene expression workflow. 37 TaqMan® gene expression assays related to immune cells, neuroinflammation, oxidative stress, apoptosis, and the glutamate pathway, and 4 housekeeping gene assays were used as detailed in Table 2. Cycle threshold (Ct) values were collected for analysis, using the 2–Δ Δ Ct method.
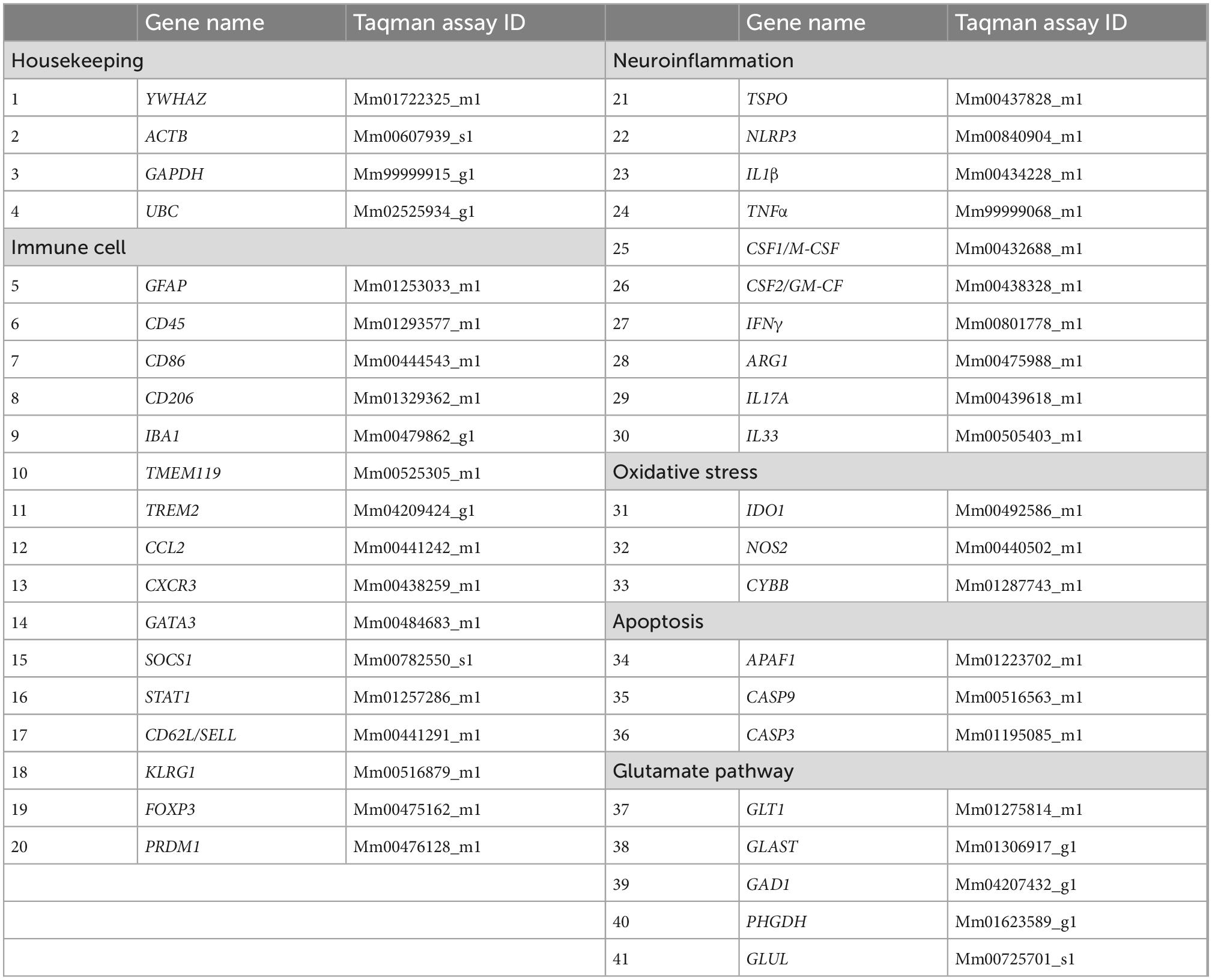
Table 2. Immune cell, neuroinflammatory, glutamate pathway, and housekeeping genes analyzed via RT-PCR using Taqman assays.
2.8. Ex vivo MRI
To verify that the CCI resulted in brain damage, structural MRI data was performed on a subset of brains that were not used for gene expression analysis (Male Vehicle + Sham = 7; Male T. gondii + Sham = 7; Male Vehicle + CCI = 7; Male T. gondii + CCI = 6; Female Vehicle + Sham = 7; Female T. gondii + Sham = 6; Female Vehicle + CCI = 7; Female T. gondii + CCI = 6). Fixed brains were washed in PBS and positioned in fomblin (Solvay Solexis, USA) for imaging with a 9.4T Bruker MRI. A 3D multi-gradient echo image was acquired to confirm injury. Imaging parameters included: repetition time = 75 ms; echo times = 5, 10, 15, …, 50 ms; field of view = 16.32 × 10.88 × 7.14 mm3; matrix size = 192 × 128 × 84; and resolution = 85 × 85 × 85 μm3. Images were reconstructed using in-house code written in MATLAB (r2021a, MathWorks, Natick, MA, USA) and templates constructed for each group using the mean echo image as described previously (Wright et al., 2018, 2019). As shown in Figure 2, the CCI groups had substantial structural brain damage relative to the sham groups.
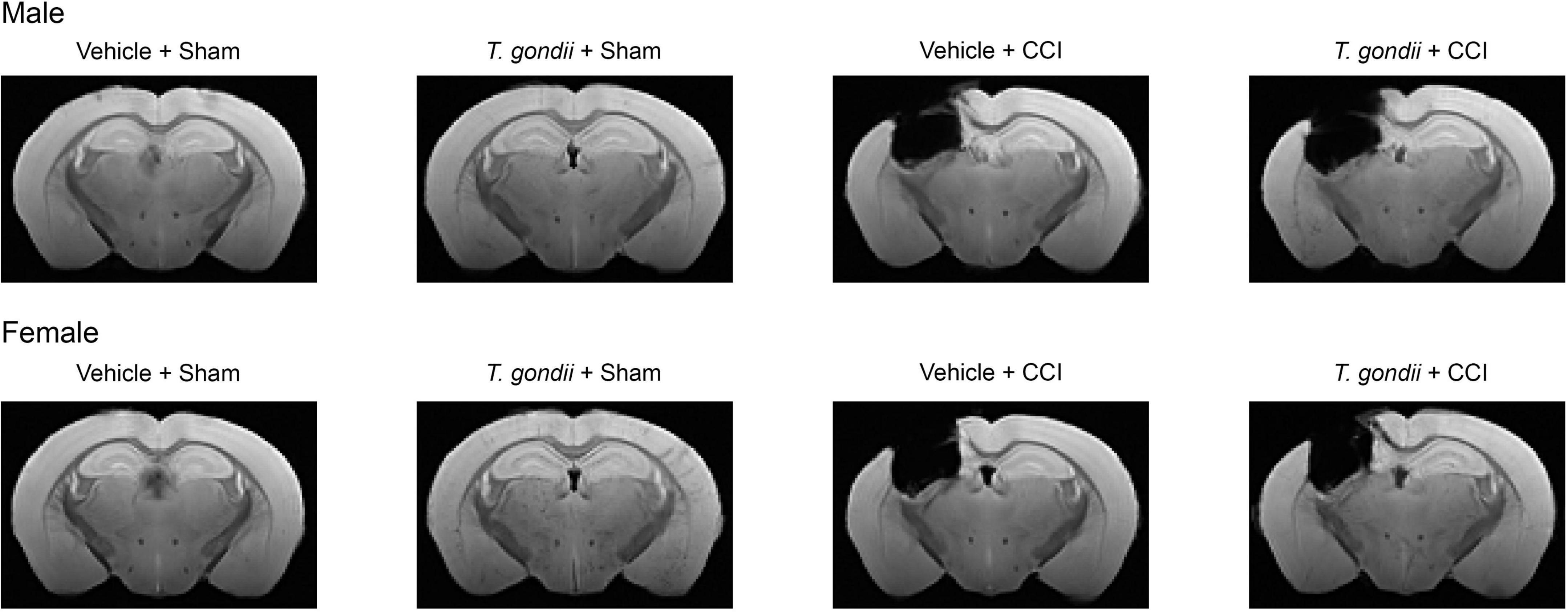
Figure 2. Controlled cortical impact (CCI) results in long-term structural brain damage. Template structural MRI images show chronic brain damage in CCI groups compared to sham groups.
2.9. Statistical analysis
Data was analyzed with SPSS 28.0 software (IBM Corp., Armonk, USA). Responses to PTZ administration and gene expression outcomes were analyzed by 3-way ANOVA, with sex, infection, and injury as between-subject factors, except for the percentage of mice that exhibited a generalized convulsive seizure which was analyzed using Fisher’s exact test. Bonferroni post-hoc comparisons were carried out when appropriate. Statistical significance was set as p < 0.05.
3. Results
3.1. Toxoplasma gondii infection increases chronic susceptibility to, and severity of, PTZ-induced seizures
Following PTZ injection, a main effect of infection [F(1,83) = 22.70, p < 0.001; Figure 3A] and sex [F(1,83) = 9.19, p = 0.003; Figure 3A] was observed on the modified seizure severity score, indicating that both T. gondii-infected and female mice reached higher scores than vehicle and male mice, respectively. Additionally, a higher proportion of mice in T. gondii groups developed generalized convulsive seizures compared to mice in vehicle groups (p < 0.001; Figure 3B). No statistically significant effect of injury or sex was observed on this measure. Of mice that developed a generalized convulsive seizure, a main effect of infection [F(1,52) = 16.98, p < 0.001; Figure 3C] and sex [F(1,52) = 5.71, p = 0.021; Figure 3C] was evident on seizure latency, with T. gondii-infected and female mice found to take less time to exhibit a generalized convulsive seizure compared to vehicle and male mice, respectively. Further to this, T. gondii-infected mice displayed increased seizure duration compared to vehicle mice [F(1,52) = 10.26, p = 0.002; Figure 3D].
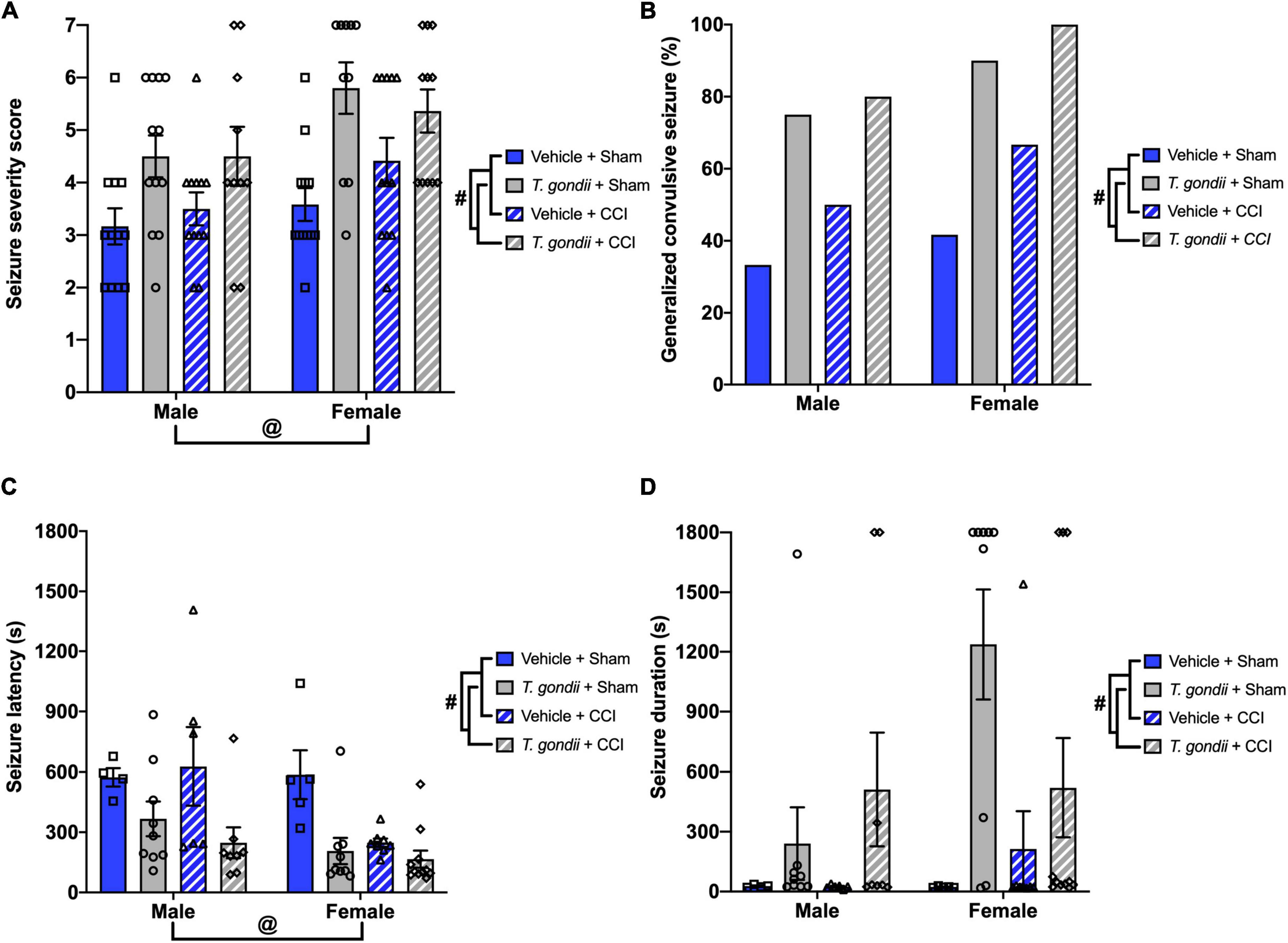
Figure 3. Toxoplasma gondii infection increased chronic susceptibility to pentylenetetrazol (PTZ)-induced seizures irrespective of injury. (A) T. gondii and female mice reached higher seizure severity scores compared to vehicle and male groups, respectively. (B) Overall, a higher percentage of mice in T. gondii groups developed generalized convulsive seizures compared to vehicle groups, yet there was no difference between injured and sham groups. (C) Of the mice that exhibited a generalized convulsive seizure, female and T. gondii groups had decreased seizure latency. (D) In addition, T. gondii-infected mice had increased seizure duration compared to vehicle mice. #T. gondii-infected mice significantly differ from vehicle mice, @female mice significantly differ from male mice, p < 0.05. n = 10–12/group/sex. Data shown is mean ± SEM.
3.2. Toxoplasma gondii infection chronically alters leukocyte and glial gene expression in the ipsilateral cortex
Genes related to leukocytes and glial cells were assessed using Multiplex qPCR. There was a significant effect of infection on the mRNA expression of GFAP [F(1,30) = 19.98, p < 0.001; Figure 4A], CD45 [F(1,30) = 21.44, p < 0.001; Figure 4B], CD86 [F(1,30) = 22.14, p < 0.001; Figure 4C], CD206 [F(1,30) = 20.10, p < 0.001; Figure 4D], IBA1 [F(1,30) = 30.28, p < 0.001; Figure 4E], TMEM119 [F(1,30) = 69.42, p < 0.001; Figure 4F], TREM2 [F(1,30) = 41.52, p < 0.001; Figure 4G], and CCL2 [F(1,30) = 18.06, p < 0.001; Figure 4H], such that expression was increased in T. gondii-infected mice compared to vehicle mice. There was also a main effect of injury on the expression of GFAP [F(1,30) = 4.33, p = 0.046; Figure 4A] and CD206 [F(1,30) = 9.91, p = 0.004; Figure 4D], with expression significantly higher in injured mice compared to sham-injured mice.
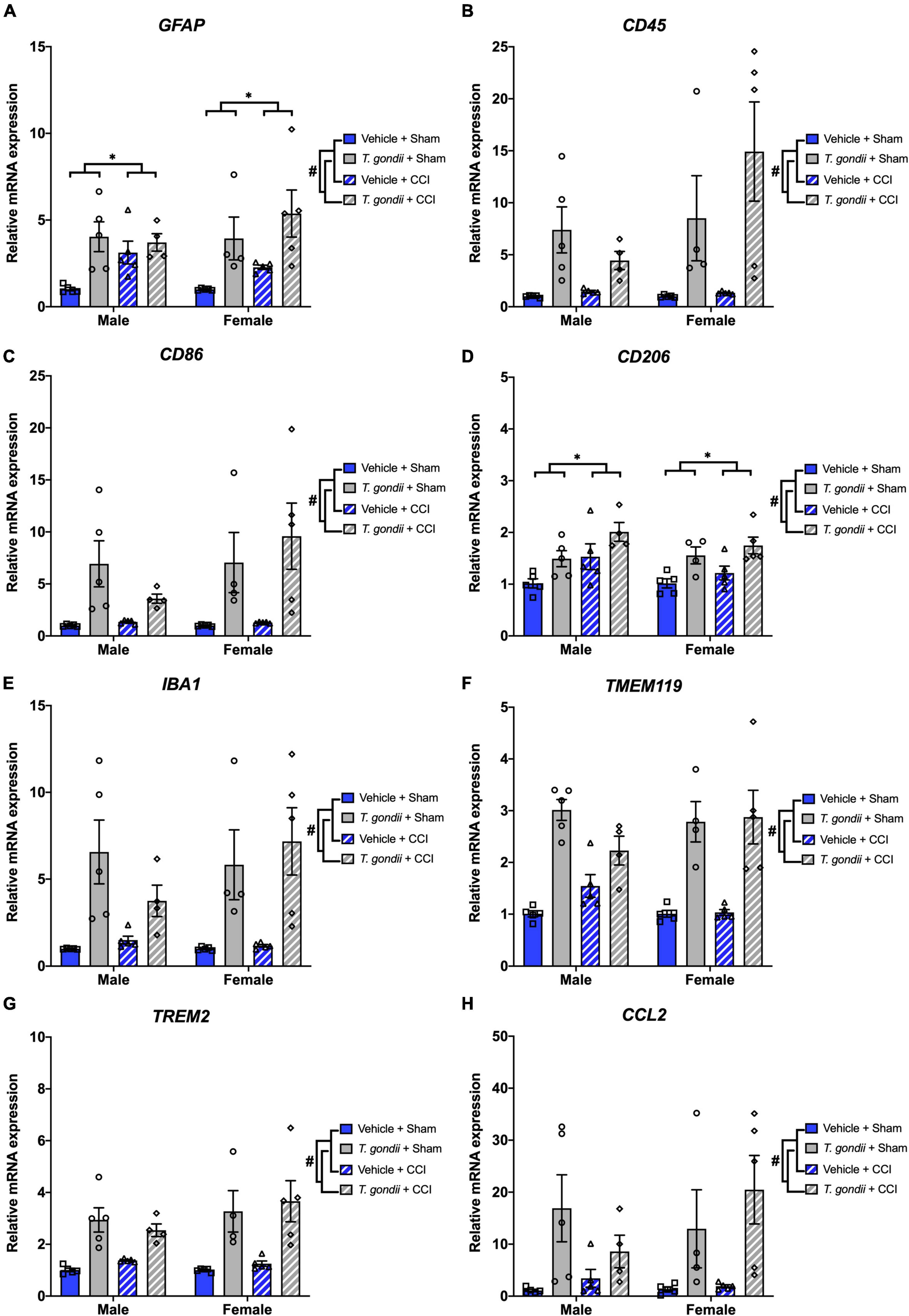
Figure 4. Toxoplasma gondii infection increased leukocyte and glial cell gene expression in the ipsilateral cortex of mice chronically post-controlled cortical impact (CCI). Gene expression of GFAP (A), CD45 (B), CD86 (C), CD206 (D), IBA1 (E), TMEM119 (F), TREM2 (G), and CCL2 (H) was increased in T. gondii infected mice compared to vehicle mice. Gene expression of GFAP (A) and CD206 (D) was also increased in mice that received a CCI compared to sham-injured mice. #T. gondii-infected mice significantly differ from vehicle mice, *CCI mice significantly differ from sham-injured mice, p < 0.05. n = 4–5/group/sex. Data shown is mean ± SEM.
Gene expression of various CD4+ and CD8+ T-cell markers were also assessed using Multiplex qPCR. A main effect of infection was evident on the expression of CXCR3 [F(1,30) = 21.90, p < 0.001; Figure 5A], GATA3 [F(1,30) = 17.52, p < 0.001; Figure 5B], SOCS1 [F(1,30) = 16.84, p < 0.001; Figure 5C], STAT1 [F(1,30) = 17.72, p < 0.001; Figure 5D], CD62L/SELL [F(1,30) = 11.80, p = 0.002; Figure 5E], KLRG1 [F(1,30) = 8.53, p = 0.007; Figure 5F], and PRDM1 [F(1,30) = 16.35, p < 0.001; Figure 5G], by which all were increased in T. gondii-infected mice compared to vehicle mice. Main effects of sex and infection, as well as a sex by infection interaction, were noted for the expression of FOXP3 [F(1,30) = 5.69, p = 0.024, F(1,30) = 13.69, p < 0.001, and F(1,30) = 5.01, p = 0.033, respectively; Figure 5H], with T. gondii-infected females having significantly increased FOXP3 expression compared to males (p = 0.003) and compared to vehicle females (p < 0.001).
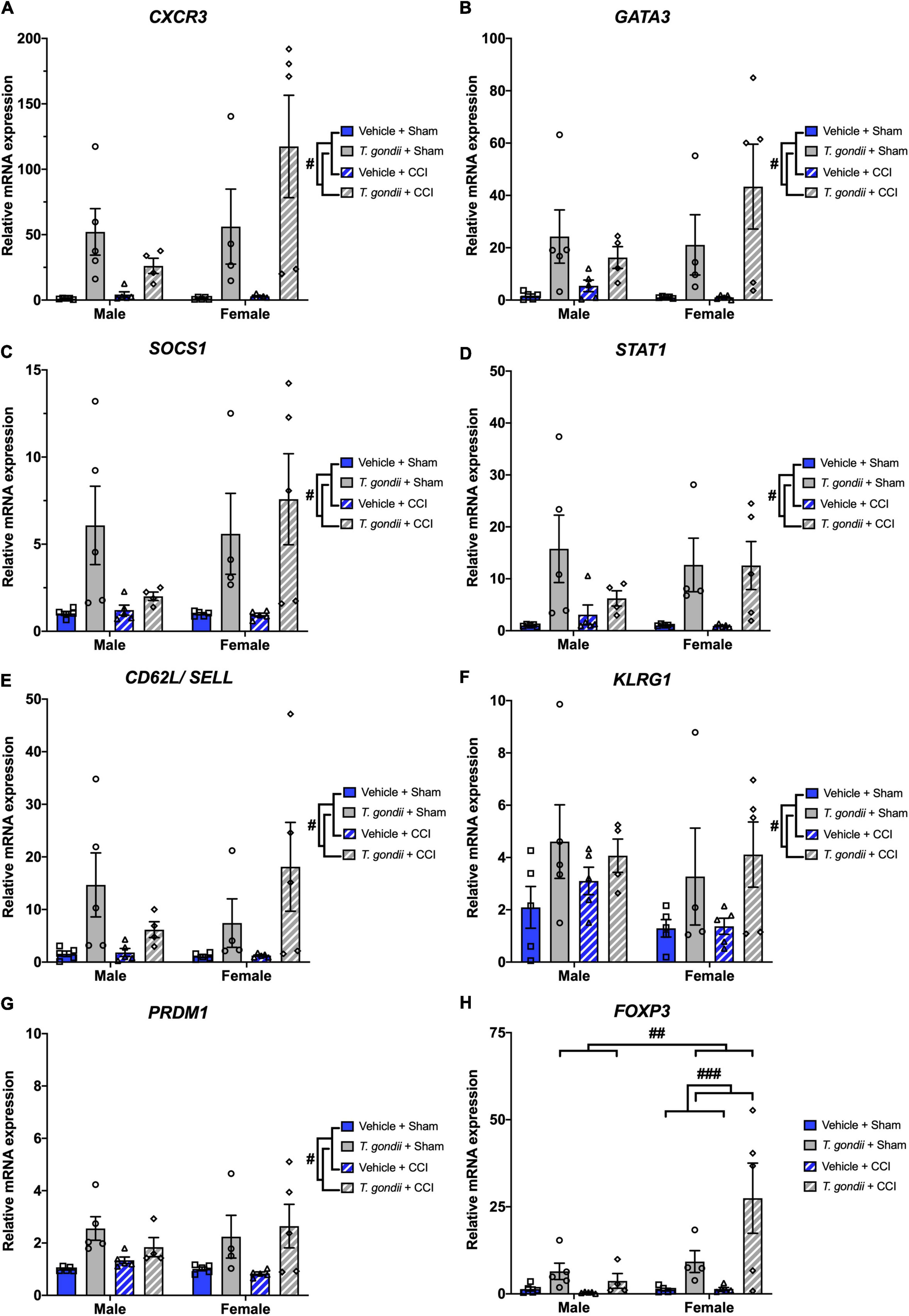
Figure 5. Toxoplasma gondii infection increases gene expression of CD4+ and CD8+ T-cell markers within the ipsilateral cortex chronically post-controlled cortical impact (CCI). T. gondii-infected mice had increased CXCR3 (A), GATA3 (B), SOCS1 (C), STAT1 (D), CD62L/SELL (E), KLRG1 (F), PRDM1 (G), and FOXP3 (H). On top of this, T. gondii-infected females had increased expression of FOXP3 compared to both T. gondii-infected males and vehicle females. #T. gondii-infected mice significantly differ from vehicle mice, ##T. gondii-infected females significantly differ from T. gondii-infected males, ###T. gondii-infected females significantly differ from vehicle females, p < 0.05. n = 4–5/group/sex. Data shown is mean ± SEM.
3.3. Toxoplasma gondii infection chronically amplifies neuroinflammatory and oxidative stress mediators
Next, gene expression of neuroinflammatory and oxidative stress mediators was investigated. A main effect of infection was found on the expression of TSPO [F(1,30) = 11.88, p = 0.002; Figure 6A], NLRP3 [F(1,30) = 22.37, p < 0.001; Figure 6B], IL1β [F(1,30) = 6.94, p = 0.013; Figure 6C], TNFα [F(1,30) = 12.88, p = 0.001; Figure 6D], CSF1/M-CSF [F(1,30) = 36.43, p < 0.001; Figure 6E], CSF2/GM-CSF [F(1,30) = 14.66, p < 0.001; Figure 6F], and IFNγ [F(1,30) = 11.36, p = 0.002; Figure 6G], where for all these genes T. gondii-infected mice exhibited increased expression compared to vehicle mice. A sex by infection by injury interaction was detected for the expression of CSF2/GM-CSF [F(1,30) = 6.03, p = 0.020]. Post-hoc analyses revealed that T. gondii + CCI females had increased expression compared to T. gondii + CCI males (p = 0.010), T. gondii + sham females (p = 0.014), and vehicle + CCI females (p < 0.001), and T. gondii + sham males had increased expression compared to vehicle + sham males (p = 0.013).
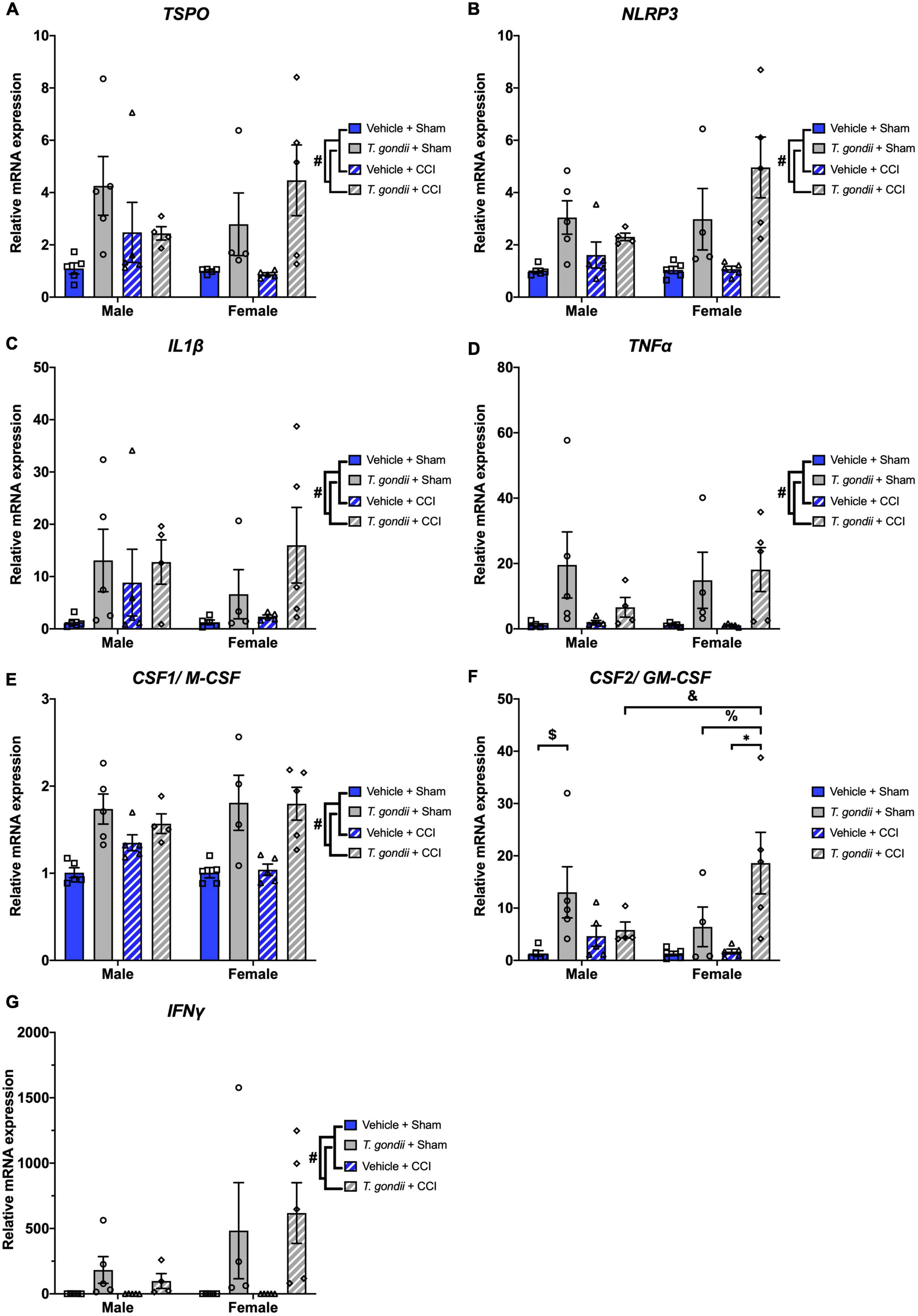
Figure 6. Chronic Toxoplasma gondii infection increases gene expression of neuroinflammatory mediators in both sexes. Increased expression of TSPO (A), NLRP3 (B), IL1β (C), TNFα (D), CSF1/M-CSF (E), CSF2/GM-CSF (F), and IFNγ (G) was increased in T. gondii-infected mice compared to vehicle mice. Further to this, T. gondii + controlled cortical impact (CCI) females also had increased expression of CSF2/GM-CSF compared to T. gondii + CCI males, T. gondii + sham females, and vehicle + CCI females. T. gondii + sham males also had increased expression of CSF2/GM-CSF compared to vehicle + sham males. #T. gondii-infected mice significantly differ from vehicle mice, $T. gondii + sham males significantly differ from vehicle + sham males, &T. gondii + CCI males significantly differ from T. gondii + CCI females, % T. gondii + sham females significantly differ from T. gondii + CCI females, *vehicle + CCI females significantly differ from T. gondii + CCI females, p < 0.05. n = 4–5/group/sex. Data shown is mean ± SEM.
A main effect of infection was also found on the expression of IDO1 [F(1,30) = 5.86, p = 0.022; Figure 7A], CYBB [F(1,30) = 15.67, p < 0.001; Figure 7B], and NOS2 [F(1,30) = 6.16, p = 0.019; Figure 7C], where T. gondii-infected mice had increased expression compared to vehicle mice. A sex by infection interaction was detected for the expression of APAF1 [F(1,30) = 4.24, p = 0.048; Figure 7D] although post-hoc analyses failed to reach significance.
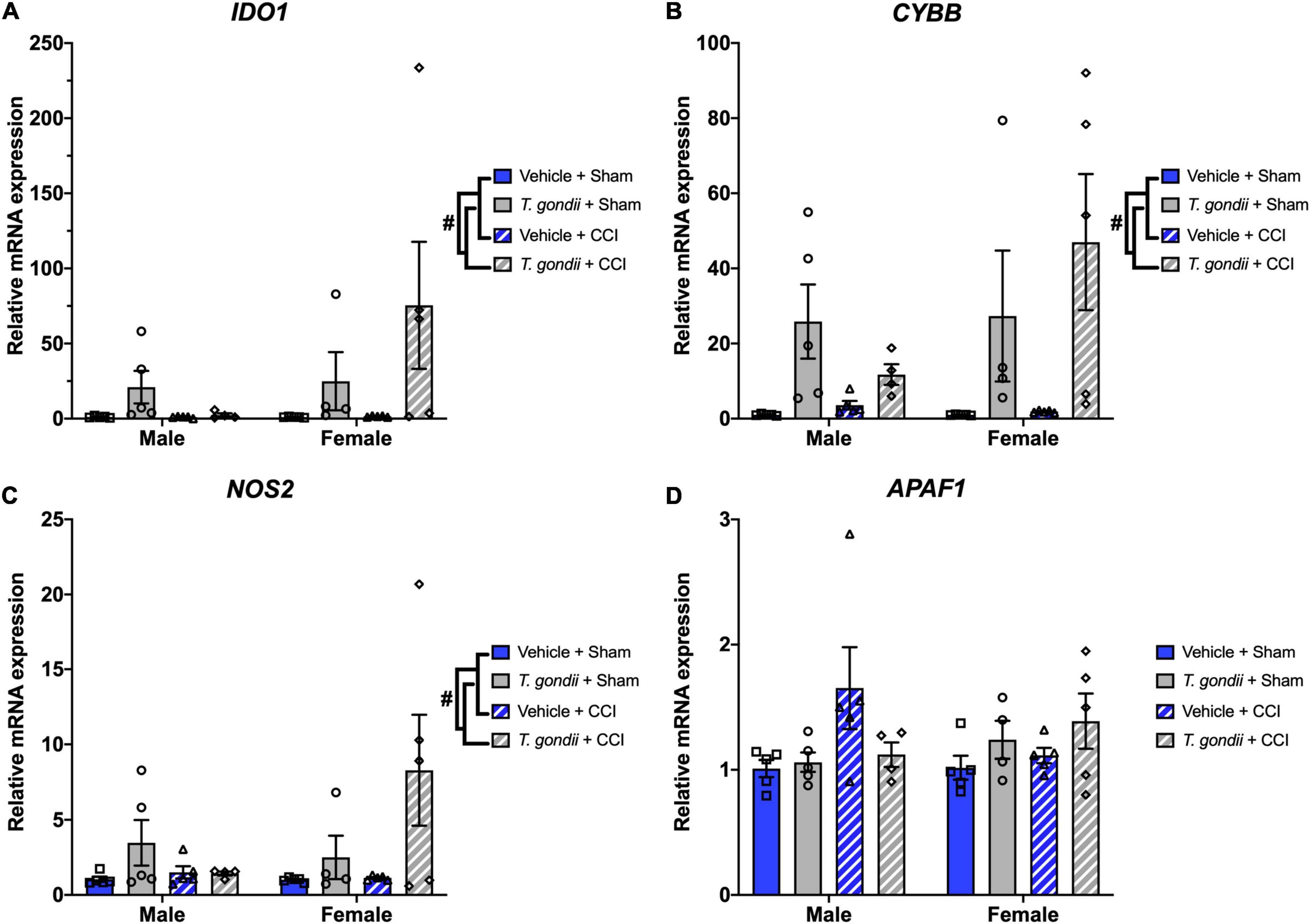
Figure 7. Chronic Toxoplasma gondii infection increases gene expression of oxidative stress mediators. Gene expression of IDO1 (A), CYBB (B), and NOS2 (C) was increased in T. gondii-infected mice compared to vehicle mice. A sex by infection interaction was detected for the expression of APAF1 (D), however, post-hoc analyses failed to reach significance. #T. gondii-infected mice significantly differ from vehicle mice, p < 0.05. n = 4–5/group/sex. Data shown is mean ± SEM.
3.4. Toxoplasma gondii infection, CCI, and sex chronically alter expression of glutamate pathway genes in the ipsilateral cortex
Main effects of infection and sex, as well as a sex by injury interaction, was evident on expression levels of GLT1 [F(1,30) = 12.55, p = 0.001, F(1,30) = 6.90, p = 0.013, and F(1,30) = 7.68, p = 0.010, respectively; Figure 8A] and GAD1 [F(1,30) = 7.35, p = 0.011, F(1,30) = 7.23, p = 0.012, and F(1,30) = 4.32, p = 0.046, respectively; Figure 8B], with post-hoc analyses revealing CCI females had lower expression levels of GLT1 (p < 0.001) and GAD1 (p = 0.002) compared to CCI males. Main effects of infection and injury were seen on GLAST expression [F(1,30) = 4.35, p = 0.046 and F(1,30) = 4.20, p = 0.049, respectively; Figure 8C] in which T. gondii and CCI groups had elevated expression levels compared to vehicle and sham groups, respectively. A main effect of sex was found on expression of PHGDH [F(1,30) = 5.84, p = 0.022; Figure 8D] such that females had lower expression of PHGDH compared to males.
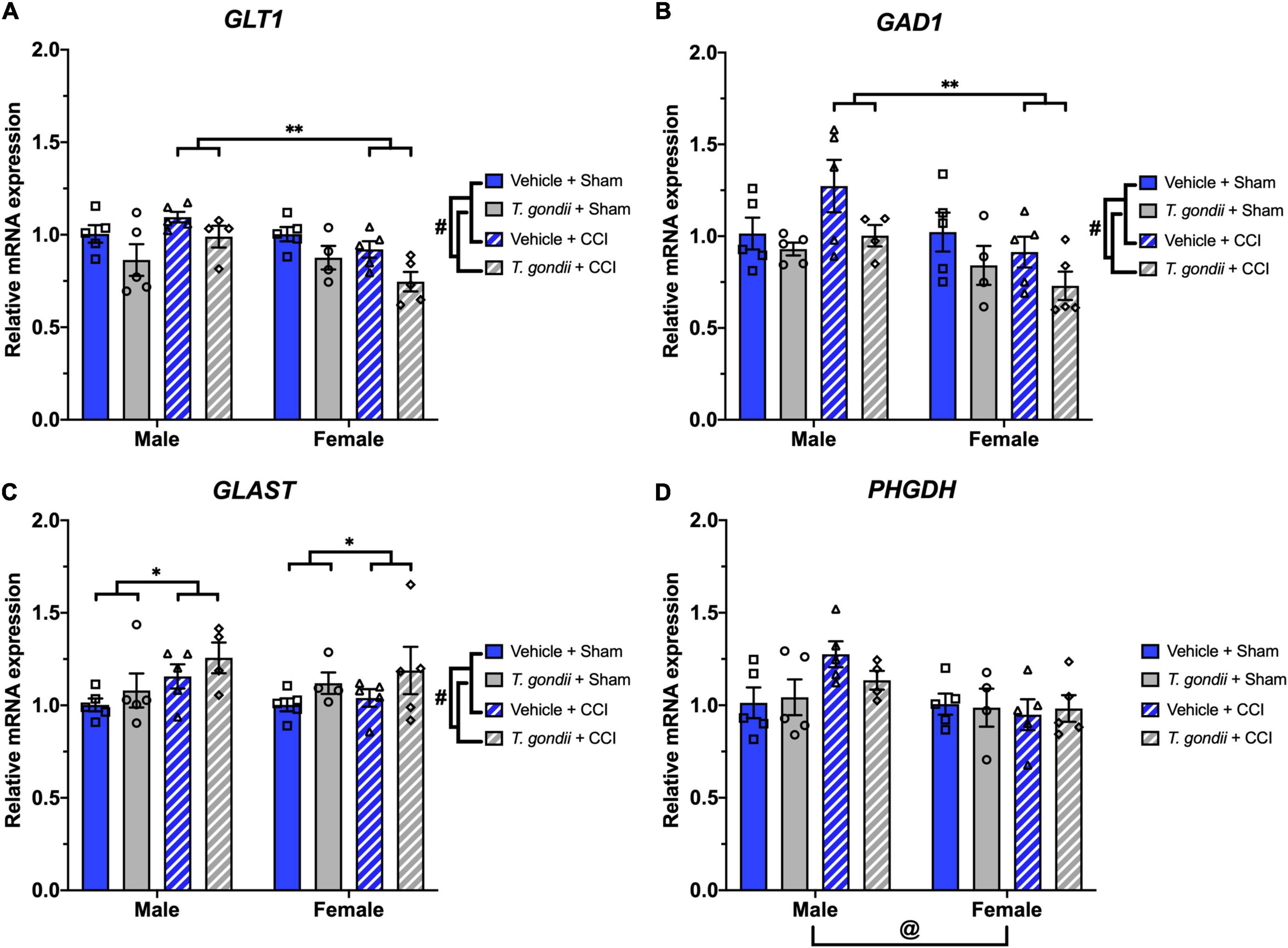
Figure 8. Toxoplasma gondii, controlled cortical impact (CCI), and sex alter the expression of glutamate pathway components. Expression of GLT1 (A) and GAD1 (B) were decreased in T. gondii-infected and female mice compared to vehicle and male mice, respectively. CCI females had lower expression of GLT1 and GAD1 compared to CCI males. In contrast, T. gondii-infected and CCI mice had increased expression of GLAST (C) compared to vehicle and sham mice, respectively. Expression levels of PHGDH (D) were lower in female mice compared to males. #T. gondii-infected mice significantly differ from vehicle mice, *sham mice significantly differ from CCI mice, @male mice significantly differ from female mice, **male CCI mice significantly differ from female CCI mice, p < 0.05. n = 4–5/group/sex. Data shown is mean ± SEM.
No significant effects of sex, infection or injury were found on expression of ARG1, IL17A, IL33, CASP9, CASP3, GLUL, YWHAZ, ACTB, GAPDH, and UBC (data not shown).
4. Discussion
This study examined the effects of a pre-existing T. gondii infection on susceptibility to, and severity of, PTZ-induced seizures at the chronic stage of TBI recovery. We hypothesized that in a combined chronic T. gondii infection and TBI setting, seizure severity, and susceptibility would be exacerbated, and T. gondii + CCI mice would have an exacerbated neuroinflammatory environment compared to other groups. Contrary to our hypotheses, no synergistic effects between infection and TBI were found. However, chronic T. gondii infection alone had robust effects on the PTZ-seizure response and gene expression of markers related to inflammatory, oxidative stress, and glutamatergic pathways. In addition to this, females were more susceptible than males to PTZ administration. Although, TBI did not influence PTZ outcomes, injury effects were evident at the structural and molecular level.
Through assessment of susceptibility to PTZ as a surrogate indicator of epileptogenesis, we found that seizure severity and duration, as well as the percentage of each group that developed a generalized convulsive seizure, were exacerbated for T. gondii-infected mice compared to the non-infected mice, irrespective of injury type. In addition, T. gondii-infected mice had decreased seizure latency in contrast to non-infected mice. These findings align with a previous study that reported increased seizure scores and decreased seizure latency after a PTZ challenge in C57BL/6 mice infected with a type II T. gondii strain (ME49) (Brooks et al., 2015). However, the current study is the first to our knowledge to examine not only seizure susceptibility/severity in T. gondii-infected male and female mice, but also to assess these measures at a chronic timepoint after injection, and in conjunction with quantification of a large range of genes associated with several neuroimmune pathways.
Interestingly, female mice took less time to develop a generalized convulsive seizure and had a higher seizure severity when compared to males, contrary to previous reports that found no difference between sexes (Yuskaitis et al., 2021). This may be due to the use of different strains, age and physiologic stressors between studies. Although no statistically significant sex by infection interactions were found on PTZ outcomes, it should be noted that the T. gondii infection paradigm did not control for body weight (i.e., all mice received a single i.p., injection of 50,000 tachyzoites). Therefore, because the females weighed less than males they were exposed to a higher T. gondii dose based on body weight. In contrast to previous findings (Semple et al., 2017; Sharma et al., 2021), we found no effect of TBI on response to PTZ at this time point. This may be explained by differences in the age at time of injury (i.e., pediatric compared to adult) as pediatric populations respond differently to TBI, have a higher incidence of PTE, and are more likely to develop post-traumatic seizures compared to older groups (Hwang et al., 2019; Webster et al., 2019; Elsamadicy et al., 2021; Mariajoseph et al., 2022). It may also be explained by differences in strain and methodologies used, as murine strains present diverse responses to PTZ, responses can vary between injury models, lower PTZ dosage can result in a greater proportion of subconvulsive events, epileptogenic sequelae vary with the stage of recovery being assessed, and physiologic stressors including EEG implantation and single-housing can increase the number of PTZ-induced seizures (Bolkvadze and Pitkänen, 2012; Mukherjee et al., 2013; Smith et al., 2018; Yuskaitis et al., 2021). Furthermore, although no effect of TBI on response to PTZ was evident at this timepoint, it is possible that the CCI was still pro-epileptogenic in terms of the development of spontaneous seizures. Related to this, although this study demonstrated differences in seizure susceptibility in T. gondii-infected mice, interpretation of generalized convulsive seizure responses to a PTZ challenge is limited in its translation to PTE development, and future studies should incorporate EEG-based analysis to accurately assess chronic spontaneous seizures without the use of PTZ.
At the molecular level, T. gondii infection was found to alter the expression of a myriad of leukocyte, glial, neuroinflammatory, oxidative stress, and glutamate related genes in the ipsilateral cortex in both males and females. These findings are in line with the current understanding of how a chronic T. gondii infection is maintained via activation of the host immune system (Wang et al., 2019; Bergersen et al., 2021), although no study to date has depicted a holistic picture of gene expression changes regarding the murine neuroinflammatory environment beyond 8-weeks post-infection. Nonetheless, the overall amplified neuroinflammation in T. gondii-infected mice compared to vehicle mice may explain the increased seizure susceptibility in these groups (Deng et al., 2021; Radpour et al., 2022). Expression of FOXP3 and CSF2/GM-CSF was particularly amplified in T. gondii + CCI females compared to other groups. The roles of both FOXP3 and CSF2/GM-CSF in epileptogenesis are largely unknown; however, in situ work has demonstrated a role for CSF2/GM-CSF in disrupted neuronal networks, and patient studies indicate increased frequency of FOXP3+ cells are positively correlated with age at onset of temporal lobe epilepsy (Vieira et al., 2016; Dikmen et al., 2020). These may therefore contribute to 100% of the T. gondii + CCI females developing a generalized convulsive seizure after PTZ injection, although more work will need to be conducted to delineate the mechanistic processes involved. Pertinent to this point, a thorough histopathological investigation for T. gondii tachyzoite replication (i.e., a reactivated chronic infection) and resultant structural damage should be conducted given that T. gondii tachyzoite replication in the clinical setting can result in encephalitis, ventriculitis, lesions, and seizures (Pradhan et al., 2007; Derouin and Pelloux, 2008). Some murine strains such as C57BL/6 mice, are more susceptible to toxoplasmic encephalitis (Suzuki et al., 1991), and changes including ventricular dilatation have also been reported in the more resistant Swiss-Webster mice after one-year of infection with a type II T. gondii strain (Hermes et al., 2008). However, as reactivation of a chronic T. gondii infection is also associated with downregulated IFNγ and TNFα expression, and simultaneous depletion of CD4+ and CDD8+ T lymphocytes (Gazzinelli et al., 1992, 1993), among other mechanisms, gene expression analyses in this study do not indicate reactivation of the chronic T. gondii infection yet this cannot be confirmed without histopathological investigation. CCI effects were also evident at the molecular level including increased expression of GFAP, CD206, and GLAST in CCI mice compared to sham-injured mice, and increased expression of GLT1 and GAD1 in CCI males compared to CCI females. Although a sex-specific finding for GLT1 and GAD1 in the context of TBI has not previously been reported, this decrease in CCI females compared to CCI males may further explain the increased susceptibility of females to PTZ injection in this study, given that GLT1 translation has previously been reported to be neuroprotective in the context of pilocarpine-induced temporal lobe epilepsy (Kong et al., 2014).
Although mice were euthanized immediately following PTZ challenge to minimize gene expression changes resulting from PTZ administration and seizure activity, the interpretation of these results is limited without the assessment of gene expression in mice that did not receive PTZ. This is important since subtle gene expression changes of inflammatory mediators in the brain have been demonstrated within 3 h of high-dose PTZ (e.g., 70 and 95 mg/kg); however, lower doses of PTZ similar to what was used in this study do not appear to influence gene expression acutely after exposure (e.g., 30 mg/kg; Amada et al., 2013; Karan et al., 2021). Further to this point, it is possible that T. gondii cyst burden and distribution throughout the brain may be meaningful in the explanation of our findings, yet T. gondii burden and distribution was not directly assessed in the current study.
In conclusion, the findings of this study demonstrate a robust effect of chronic T. gondii infection on not only seizure severity and susceptibility at the chronic stage of recovery, but also on the neuroinflammatory factors that may contribute to the epileptogenic process. Although this study is not without limitations, the findings demonstrate that a pre-existing T. gondii infection is a significant modifier of PTZ-induced seizures independent of brain injury, and further attention should be directed toward understanding the mechanisms and potential interventions.
Data availability statement
The original contributions presented in this study are included in the article/supplementary material, further inquiries can be directed to the corresponding author.
Ethics statement
The animal study was reviewed and approved by AMREP Animal Ethics Committee.
Author contributions
SS, MS, and TB conceptualized and designed the study. DW completed MRI analysis. TB and MS completed pentylenetetrazol (PTZ) data collection. TB and SS conducted the data analysis. AV and TW completed gene expression experiments. CT and AU provided the Toxoplasma gondii and assisted in establishing the T. gondii model. BS assisted with the brain injury and PTZ models. All authors contributed to the article and approved the submitted version.
Funding
This study was supported by funding from NHMRC (SS, DW, BS, and RM) and the Brain Foundation (MS and SS).
Acknowledgments
We acknowledge the facilities and scientific and technical assistance of the National Imaging Facility (NIF), a National Collaborative Research Infrastructure Strategy (NCRIS) capability at Monash Biomedical Imaging (MBI), a Technology Research Platform at Monash University. We also acknowledge that the multiplex qPCR was performed by the Medical Genomics Facility at the Monash Health Translation Precinct.
Conflict of interest
The authors declare that the research was conducted in the absence of any commercial or financial relationships that could be construed as a potential conflict of interest.
Publisher’s note
All claims expressed in this article are solely those of the authors and do not necessarily represent those of their affiliated organizations, or those of the publisher, the editors and the reviewers. Any product that may be evaluated in this article, or claim that may be made by its manufacturer, is not guaranteed or endorsed by the publisher.
References
Alizadeh Khatir, A., Moghaddam, S. A., Almukhtar, M., Ghorbani, H., Babazadeh, A., Mehravar, S., et al. (2021). Toxoplasma infection and risk of epilepsy: a case-control study of incident patients. Microb. Pathog. 161:105302. doi: 10.1016/j.micpath.2021.105302
Amada, N., Yamasaki, Y., Williams, C. M., and Whalley, B. J. (2013). Cannabidivarin (CBDV) suppresses pentylenetetrazole (PTZ)-induced increases in epilepsy-related gene expression. PeerJ 1:e214. doi: 10.7717/peerj.214
Bergersen, K. V., Barnes, A., Worth, D., David, C., and Wilson, E. H. (2021). Targeted transcriptomic analysis of C57BL/6 And BALB/c mice during progressive chronic Toxoplasma gondii infection reveals changes in host and parasite gene expression relating to neuropathology and resolution. Front. Cell. Infect. Microbiol. 11:645778. doi: 10.3389/fcimb.2021.645778
Bolkvadze, T., and Pitkänen, A. (2012). Development of post-traumatic epilepsy after controlled cortical impact and lateral fluid-percussion-induced brain injury in the mouse. J. Neurotrauma 29, 789–812. doi: 10.1089/neu.2011.1954
Brooks, J. M., Carrillo, G. L., Su, J., Lindsay, D. S., Fox, M. A., and Blader, I. J. (2015). Toxoplasma gondii infections alter GABAergic synapses and signaling in the central nervous system. mBio 6:e01428-15. doi: 10.1128/mBio.01428-15
Burke, J., Gugger, J., Ding, K., Kim, J. A., Foreman, B., Yue, J. K., et al. (2021). Association of posttraumatic epilepsy with 1-year outcomes after traumatic brain injury. JAMA Netw. Open 4:e2140191. doi: 10.1001/jamanetworkopen.2021.40191
Christensen, J., Pedersen, M. G., Pedersen, C. B., Sidenius, P., Olsen, J., and Vestergaard, M. (2009). Long-term risk of epilepsy after traumatic brain injury in children and young adults: a population-based cohort study. Lancet 373, 1105–1110. doi: 10.1016/S0140-6736(09)60214-2
David, C. N., Frias, E. S., Szu, J. I., Vieira, P. A., Hubbard, J. A., Lovelace, J., et al. (2016). GLT-1-dependent disruption Of Cns glutamate homeostasis and neuronal function by the protozoan parasite Toxoplasma gondii. PLoS Pathog. 12:e1005643. doi: 10.1371/journal.ppat.1005643
Degrauw, X., Thurman, D., Xu, L., Kancherla, V., and Degrauw, T. (2018). Epidemiology of traumatic brain injury-associated epilepsy and early use of anti-epilepsy drugs: an analysis of insurance claims data, 2004-2014. Epilepsy Res. 146, 41–49. doi: 10.1016/j.eplepsyres.2018.07.012
Deng, N., Hu, J., Hong, Y., Ding, Y., Xiong, Y., Wu, Z., et al. (2021). Indoleamine-2,3-dioxygenase 1 deficiency suppresses seizures in epilepsy. Front. Cell. Neurosci. 15:638854. doi: 10.3389/fncel.2021.638854
Derouin, F., and Pelloux, H. (2008). Prevention of toxoplasmosis in transplant patients. Clin. Microbiol. Infect. 14, 1089–1101. doi: 10.1111/j.1469-0691.2008.02091.x
Dikmen, H. O., Hemmerich, M., Lewen, A., Hollnagel, J. O., Chausse, B., and Kann, O. (2020). GM-CSF induces noninflammatory proliferation of microglia and disturbs electrical neuronal network rhythms in situ. J. Neuroinflamm. 17:235. doi: 10.1186/s12974-020-01903-4
Egorov, A. I., Converse, R. R., Griffin, S. M., Styles, J. N., Sams, E., Hudgens, E., et al. (2021). Latent Toxoplasma gondii infections are associated with elevated biomarkers of inflammation and vascular injury. BMC Infect. Dis. 21:188. doi: 10.1186/s12879-021-05882-6
Elsamadicy, A. A., Koo, A. B., David, W. B., Lee, V., Zogg, C. K., Kundishora, A. J., et al. (2021). Post-traumatic seizures following pediatric traumatic brain injury. Clin. Neurol. Neurosurg. 203, 862–866. doi: 10.1016/j.clineuro.2021.106556
Gazzinelli, R., Xu, Y., Hieny, S., Cheever, A., and Sher, A. (1992). Simultaneous depletion of CD4+ and CD8+ T lymphocytes is required to reactivate chronic infection with Toxoplasma gondii. J. Immunol. 149, 175–180.
Gazzinelli, R. T., Eltoum, I., Wynn, T. A., and Sher, A. (1993). Acute cerebral toxoplasmosis is induced by in vivo neutralization of TNF-alpha and correlates with the down-regulated expression of inducible nitric oxide synthase and other markers of macrophage activation. J. Immunol. 151, 3672–3681.
GBD 2016 Neurology Collaborators (2019). Global, regional, and national burden of traumatic brain injury and spinal cord injury, 1990–2016: a systematic analysis for the Global Burden Of Disease Study 2016. Lancet Neurol. 18, 56–87. doi: 10.1016/S1474-4422(18)30499-X
Grauncke, A. C., Souza, T. L., Ribeiro, L. R., Brant, F., Machado, F. S., and Oliveira, M. S. (2016). Increased susceptibility to pentylenetetrazol following survival of cerebral malaria in mice. Epilepsia 57, e140–e145. doi: 10.1111/epi.13425
Hermes, G., Ajioka, J. W., Kelly, K. A., Mui, E., Roberts, F., Kasza, K., et al. (2008). Neurological and behavioral abnormalities, ventricular dilatation, altered cellular functions, inflammation, and neuronal injury in brains of mice due to common, persistent, parasitic infection. J. Neuroinflamm. 5:48. doi: 10.1186/1742-2094-5-48
Ho, Y. H., Lin, Y. T., Wu, C. W., Chao, Y. M., Chang, A. Y., and Chan, J. Y. (2015). Peripheral inflammation increases seizure susceptibility via the induction of neuroinflammation and oxidative stress in the hippocampus. J. Biomed. Sci. 22:46. doi: 10.1186/s12929-015-0157-8
Howe, D. K., and Sibley, L. D. (1995). Toxoplasma gondii comprises three clonal lineages: correlation of parasite genotype with human disease. J. Infect. Dis. 172, 1561–1566. doi: 10.1093/infdis/172.6.1561
Huang, W. Y., Lai, Y. L., Liu, K. H., Lin, S., Chen, H. Y., Liang, C. H., et al. (2022). TNFα-mediated necroptosis in brain endothelial cells as a potential mechanism of increased seizure susceptibility in mice following systemic inflammation. J. Neuroinflamm. 19:29. doi: 10.1186/s12974-022-02406-0
Huang, W. Y., Lin, S., Chen, H. Y., Chen, Y. P., Chen, T. Y., Hsu, K. S., et al. (2018). NADPH oxidases as potential pharmacological targets against increased seizure susceptibility after systemic inflammation. J. Neuroinflamm. 15:140. doi: 10.1186/s12974-018-1186-5
Hwang, S. Y., Ong, J. W., Ng, Z. M., Foo, C. Y., Chua, S. Z., Sri, D., et al. (2019). Long-term outcomes in children with moderate to severe traumatic brain injury: a single-centre retrospective study. Brain Injury 33, 1420–1424. doi: 10.1080/02699052.2019.1641625
Karan, A. A., Spivak, Y. S., Gerasimov, K. A., Suleymanova, E. M., Volobueva, M. N., Kvichansky, A. A., et al. (2021). CB2 receptors modulate seizure-induced expression of pro-inflammatory cytokines in the hippocampus but not neocortex. Mol. Neurobiol. 58, 4028–4037. doi: 10.1007/s12035-021-02395-w
Karlander, M., Ljungqvist, J., Sörbo, A., and Zelano, J. (2022). Risk and cause of death in post-traumatic epilepsy: a register-based retrospective cohort study. J. Neurol. 269, 6014–6020. doi: 10.1007/s00415-022-11279-5
Kong, Q., Chang, L. C., Takahashi, K., Liu, Q., Schulte, D. A., Lai, L., et al. (2014). Small-molecule activator of glutamate transporter eaat2 translation provides neuroprotection. J. Clin. Investig. 124, 1255–1267. doi: 10.1172/JCI66163
Lu, D. C., Zador, Z., Yao, J., Fazlollahi, F., and Manley, G. T. (2021). Aquaporin-4 reduces post-traumatic seizure susceptibility by promoting astrocytic glial scar formation in mice. J. Neurotrauma 38, 1193–1201. doi: 10.1089/neu.2011.2114
Mariajoseph, F. P., Chen, Z., Sekhar, P., Rewell, S. S., O’Brien, T. J., Antonic-Baker, A., et al. (2022). Incidence and risk factors of posttraumatic epilepsy following pediatric traumatic brain injury: a systematic review and meta-analysis. Epilepsia 63, 2802–2812. doi: 10.1111/epi.17398
Montoya, J. G., and Liesenfeld, O. (2004). Toxoplasmosis. Lancet 363, 1965–1976. doi: 10.1016/S0140-6736(04)16412-X
Mukherjee, S., Zeitouni, S., Cavarsan, C. F., and Shapiro, L. A. (2013). Increased seizure susceptibility in mice 30 days after fluid percussion injury. Front. Neurol. 4:28. doi: 10.3389/fneur.2013.00028
Ngadimon, I. W., Aledo-Serrano, A., Arulsamy, A., Mohan, D., Khoo, C. S., Cheong, W. L., et al. (2022). An interplay between post-traumatic epilepsy and associated cognitive decline: a systematic review. Front. Neurol. 13:827571. doi: 10.3389/fneur.2022.827571
Ochiai, E., Sa, Q., Brogli, M., Kudo, T., Wang, X., Dubey, J. P., et al. (2015). CXCL9 is important for recruiting immune T cells into the brain and inducing an accumulation of the T cells to the areas of tachyzoite proliferation to prevent reactivation of chronic cerebral infection with Toxoplasma gondii. Am. J. Pathol. 185, 314–324. doi: 10.1016/j.ajpath.2014.10.003
Pease, M., Gonzalez-Martinez, J., Puccio, A., Nwachuku, E., Castellano, J. F., Okonkwo, D. O., et al. (2022). Risk factors and incidence of epilepsy after severe traumatic brain injury. Annu. Neurol. 92, 663–669. doi: 10.1002/ana.26443
Pradhan, S., Yadav, R., and Mishra, V. N. (2007). Toxoplasma meningoencephalitis in HIV-seronegative patients: clinical patterns, imaging features and treatment outcome. Trans. R. Soc. Trop. Med. Hyg. 101, 25–33. doi: 10.1016/j.trstmh.2006.02.021
Radpour, M., Choopani, S., Pourbadie, H. G., and Sayyah, M. (2022). Activating toll-like receptor 4 after traumatic brain injury inhibits neuroinflammation and the accelerated development of seizures in rats. Exp. Neurol. 357:114202. doi: 10.1016/j.expneurol.2022.114202
Sadeghi, M., Riahi, S. M., Mohammadi, M., Saber, V., Aghamolaie, S., Moghaddam, S. A., et al. (2019). An updated meta-analysis of the association between Toxoplasma gondii infection and risk of epilepsy. Trans. R. Soc. Trop. Med. Hyg. 113, 453–462.
Semple, B. D., O’Brien, T. J., Gimlin, K., Wright, D. K., Kim, S. E., Casillas-Espinosa, P. M., et al. (2017). Interleukin-1 receptor in seizure susceptibility after traumatic injury to the pediatric brain. J. Neurosci. 37, 7864–7877. doi: 10.1523/JNEUROSCI.0982-17.2017
Sharma, R., Leung, W. L., Zamani, A., O’Brien, T. J., Casillas Espinosa, P. M., and Semple, B. D. (2019). Neuroinflammation in post-traumatic epilepsy: pathophysiology and tractable therapeutic targets. Brain Sci. 9:318. doi: 10.3390/brainsci9110318
Sharma, R., Zamani, A., Dill, L. K., Sun, M., Chu, E., Robinson, M. J., et al. (2021). A systemic immune challenge to model hospital-acquired infections independently regulates immune responses after pediatric traumatic brain injury. J. Neuroinflamm. 18:72. doi: 10.1186/s12974-021-02114-1
Smith, D., Rau, T., Poulsen, A., MacWilliams, Z., Patterson, D., Kelly, W., et al. (2018). Convulsive seizures and EEG spikes after lateral fluid-percussion injury in the rat. Epilepsy Res. 147, 87–94. doi: 10.1016/j.eplepsyres.2018.09.005
Ssentongo, P., Robuccio, A. E., Thuku, G., Sim, D. G., Nabi, A., Bahari, F., et al. (2017). A murine model to study epilepsy and SUDEP induced by malaria infection. Sci. Rep. 7:43652. doi: 10.1038/srep43652
Suzuki, Y., Joh, K., Orellana, M. A., Conley, F. K., and Remington, J. S. (1991). A gene(s) within the H-2D region determines the development of toxoplasmic encephalitis in mice. Immunology 74, 732–739.
Tubi, M. A., Lutkenhoff, E., Blanco, M. B., McArthur, D., Villablanca, P., Ellingson, B., et al. (2019). Early seizures and temporal lobe trauma predict post-traumatic epilepsy: a longitudinal study. Neurobiol. Dis. 123, 115–121. doi: 10.1016/j.nbd.2018.05.014
Tyebji, S., Seizova, S., Garnham, A. L., Hannan, A. J., and Tonkin, C. J. (2019). Impaired social behaviour and molecular mediators of associated neural circuits during chronic Toxoplasma gondii infection in female mice. Brain Behav. Immun. 80, 88–108. doi: 10.1016/j.bbi.2019.02.028
Van Erum, J., Van Dam, D., and De Deyn, P. P. (2019). PTZ-induced seizures in mice require a revised Racine scale. Epilepsy Behav. 95, 51–55. doi: 10.1016/j.yebeh.2019.02.029
Verellen, R. M., and Cavazos, J. E. (2010). Post-traumatic epilepsy: an overview. Therapy 7, 527–531. doi: 10.2217/THY.10.57
Vieira, ÉL. M., De Oliveira, G. N. M., Lessa, J. M. K., Gonçalves, A. P., Oliveira, A. C. P., Bauer, M. E., et al. (2016). Peripheral leukocyte profile in people with temporal lobe epilepsy reflects the associated proinflammatory state. Brain Behav. Immun. 53, 123–130. doi: 10.1016/j.bbi.2015.11.016
Viswanatha, G. L., Shylaja, H., Kishore, D. V., Venkataranganna, M. V., and Prasad, N. B. L. (2020). Acteoside isolated from colebrookea oppositifolia smith attenuates epilepsy in mice via modulation of gamma-aminobutyric acid pathways. Neurotoxic. Res. 38, 1010–1023. doi: 10.1007/s12640-020-00267-0
Wang, T., Sun, X., Qin, W., Zhang, X., Wu, L., Li, Y., et al. (2019). From inflammatory reactions to neurotransmitter changes: implications for understanding the neurobehavioral changes in mice chronically infected with Toxoplasma gondii. Behav. Brain Res. 359, 737–748. doi: 10.1016/j.bbr.2018.09.011
Wang, Y., Andrade, P., and Pitkänen, A. (2021). Peripheral infection after traumatic brain injury augments excitability in the perilesional cortex and dentate gyrus. Biomedicines 9:1946. doi: 10.3390/biomedicines9121946
Webster, K. M., Sun, M., Crack, P., O’Brien, T. J., Shultz, S. R., and Semple, B. D. (2017). Inflammation in epileptogenesis after traumatic brain injury. J. Neuroinflamm. 14:10. doi: 10.1186/s12974-016-0786-1
Webster, K. M., Sun, M., Crack, P. J., O’Brien, T. J., Shultz, S. R., and Semple, B. D. (2019). Age-dependent release of high-mobility group box protein-1 and cellular neuroinflammation after traumatic brain injury in mice. J. Comp. Neurol. 527, 1102–1117. doi: 10.1002/cne.24589
Wright, D. K., Brady, R. D., Kamnaksh, A., Trezise, J., Sun, M., McDonald, S. J., et al. (2019). ‘Repeated mild traumatic brain injuries induce persistent changes in plasma protein and magnetic resonance imaging biomarkers in the rat. Sci. Rep. 9:14626. doi: 10.1038/s41598-019-51267-w
Wright, D. K., O’Brien, T. J., Mychasiuk, R., and Shultz, S. R. (2018). ‘Telomere length and advanced diffusion MRI as biomarkers for repetitive mild traumatic brain injury in adolescent rats. Neuroimage Clin. 18, 315–324. doi: 10.1016/j.nicl.2018.01.033
Xiong, Y., Mahmood, A., and Chopp, M. (2013). Animal models of traumatic brain injury. Nat. Rev. Neurosci. 14, 128–142. doi: 10.1038/nrn3407
Yuskaitis, C. J., Rossitto, L. A., Groff, K. J., Dhamne, S. C., Zhang, B., Lalani, L. K., et al. (2021). Factors influencing the acute pentylenetetrazole-induced seizure paradigm and a literature review. Ann. Clin. Transl. Neurol. 8, 1388–1397. doi: 10.1002/acn3.51375
Keywords: epileptogenesis, immune response, neuroinflammation, oxidative stress, post-traumatic epilepsy (PTE)
Citation: Baker TL, Uboldi AD, Tonkin CJ, Wright DK, Vo A, Wilson T, Mychasiuk R, McDonald SJ, Semple BD, Sun M and Shultz SR (2023) Pre-existing Toxoplasma gondii infection increases susceptibility to pentylenetetrazol-induced seizures independent of traumatic brain injury in mice. Front. Mol. Neurosci. 15:1079097. doi: 10.3389/fnmol.2022.1079097
Received: 25 October 2022; Accepted: 09 December 2022;
Published: 05 January 2023.
Edited by:
Shaunik Sharma, The University of Iowa, United StatesReviewed by:
Aylin Yasmin Reid, University Health Network (UHN), CanadaChristos Panagiots Lisgaras, New York University, United States
Hebert Luis Hernandez-Montiel, Universidad Autónoma de Querétaro, Mexico
Copyright © 2023 Baker, Uboldi, Tonkin, Wright, Vo, Wilson, Mychasiuk, McDonald, Semple, Sun and Shultz. This is an open-access article distributed under the terms of the Creative Commons Attribution License (CC BY). The use, distribution or reproduction in other forums is permitted, provided the original author(s) and the copyright owner(s) are credited and that the original publication in this journal is cited, in accordance with accepted academic practice. No use, distribution or reproduction is permitted which does not comply with these terms.
*Correspondence: Sandy R. Shultz, ✉ c2FuZHkuc2h1bHR6QG1vbmFzaC5lZHU=
†These authors have contributed equally to this work