- 1Deraya Center for Scientific Research, Deraya University, New Minia, Egypt
- 2Department of Pharmacognosy, Faculty of Pharmacy, Minia University, Minia, Egypt
- 3Department of Pharmacognosy, Faculty of Pharmacy, Beni-Suef University, Beni-Suef, Egypt
Essential oils (EOs) have a long-standing tradition and remain widely used in therapeutic applications. Their biological activities are primarily driven by complex interactions among their diverse components which exhibit synergistic or antagonistic effects, resulting in a broad spectrum of pharmacological and biological properties. These components are characterized by distinct molecular formulas, specific molecular weights, and unique physicochemical properties. The composition, content, and bioactivity of EOs are influenced by a variety of internal and external factors. Internal factors include genetic aspects (species, ecotype, chemotype, cultivar, and variety), plant developmental stage, and the specific plant part used. External factors encompass environmental and cultivation conditions (climate, radiation, diurnal and annual rhythms, harvesting practices, latitude, planting dates, irrigation, fertilization, salinity, and growth retardants), postharvest techniques (drying methods and storage conditions), and extraction and distillation processes (methods, moisture, acidity, distillation time, and gas chromatography analysis conditions). Additionally, oil degradation during storage (due to light, temperature, oxygen, chemical composition, and metal contaminants) and factors affecting bioactivity (plant part treated, solvent/detergent used, composition–activity relationships, techniques, biological material, dose/concentration selection, reference compounds, and comparison with literature data) play critical roles in shaping EO properties. This review provides a comprehensive examination of these factors, highlighting their impact on the content, composition, and bioactivity of EOs. By addressing these parameters in detail, this work aims to enhance the understanding of EOs and their therapeutic potential.
1 Introduction
One of the key goods produced by agriculture-based business is EOs of plant origin. They are frequently used as flavoring ingredients in foods, beverages, perfumes, and cosmetics (Burt, 2004). At least 2,000 plant species have been used to make over 3,000 EOs, of which 300 are significant from a commercial standpoint. The estimated market worth of 700 million US dollars and annual production of 40,000–60,000 tonnes of EOs show that both production and consumption are rising globally (Shuaib et al., 2021; Bahl et al., 2018; Ali et al., 2019; Rajabi et al., 2020; Riaz et al., 2021). Like other secondary physiologically active compounds, EOs are unique to some smaller or larger systematic groups of plants, such as families, genera, and even species. The following can be included in the most know EO-producing groups: Lamiaceae (genera: Mentha, Thymus, Salvia, Rosmarinus, Lavandula, Ocimum, Origanum, Hyssopus, Melissa, and Satureja), Asteraceae (genera: Matricaria, Artemisia, Anthemis, Arnica, Tanacetum, and Achillea), Cupressaceae (genus: Juniperus), Piperaceae, Lauraceae (genera: Laurus and Cinnamomum), Zingiberaceae (genera: Zingiber, Curcuma, Alpinia, and Elettaria), Araceae (genus: Acorus), Apiaceae (genera: Pimpinella, Carum, Foeniculum, Anethum, Angelica, Coriandrum, and Levisticum), Myrtaceae (genera: Eugenia and Eucalyptus), Pinaceae (genera: Abies, Pinus, Larix, and Picea), Rutaceae (genera: Citrus and Ruta), and Poaceae (genus: Cymbopogon) (Nurzynska-Wierdak, 2013; Zahran et al., 2020).
Distillation or extraction are the first two steps in the analytical processes used to isolate EOs from medicinal plants. Hydrodistillation, steam distillation, microwave hydrodiffusion, microwave-assisted distillation, expression, enfleurage, organic solvent extraction, gravity, supercritical carbon dioxide (CO2) extraction, solvent-free microwave extraction, high-pressure solvent extraction, ultrasonic extraction, and the phytonic process are some of the different modern extraction techniques available for EOs (Farhat et al., 2010; Raynie, 2006; Hanif et al., 2019; Freitas et al., 2023; Ghasemy-Piranloo et al., 2022). Steam distillation is the method of choice for the commercial extraction of EOs (Masango, 2005; Abdelmohsen et al., 2022; Hesham Abdelhafez et al., 2021). Distillation is frequently carried out by employing a Clevenger, Dean–Stark, or Likens Nikerson apparatus with extended heating and stirring in water or a solvent. More than 70% of the process’s energy and time is used in distillation, which also uses a lot of solvent (Chemat et al., 2009). There are various drawbacks to steam distillation. Water and high temperatures both have the potential to chemically alter EOs. Steam distillation results in the loss of some water-soluble and highly volatile substances. However, it is quite impossible to obtain solvent-free results when performing solvent extraction (Guan et al., 2007). Monoterpenes are infamously prone to chemical changes and losses of more volatile elements after the removal of the solvent under steam distillation conditions and even after ordinary solvent extraction (Lo et al., 2005; Elmaidomy et al., 2017; Mohammad et al., 2019).
To lessen the sample preparation process and the drawbacks of steam distillation, other procedures should be devised. Furthermore, it has been discovered that the method utilized affects the characteristics of the EOs extracted. Using a combination technique of an organic solvent with a low boiling point and steam distillation could replace this procedure because the residues present in the extracts obtained by traditional solvents, which can taint foods and fragrances, make this approach ineffective (Faborode and Favier, 1996). Many of the issues that conventional solvents create during the extraction of oleoresins, such as the challenging separation of the product from the extraction solvent and the significant solvent remains, could be resolved by using organic solvents with low boiling ranges (Debet and Gidley, 2006).
Supercritical fluid extraction using CO2 has recently emerged as a viable alternative to traditional solvent extraction techniques. Due to the specialized and costly high-pressure equipment and technology required, this approach has had trouble finding commercial application (Jarvis and Morgan, 1997). Since it is possible to control the pressure and temperature of the extraction environment, CO2 is typically the most ideal solvent for supercritical fluid extraction (Carvalho et al., 2005). For the extraction of heat-sensitive components, CO2 is appealing due to its adequate critical temperature. Its low surface tension, low viscosity, and high diffusivity make it an intriguing choice for a supercritical solvent. It is a nonflammable, affordable, tasteless, colorless, and pure solvent that does not leave any solvent residue in the finished product. Additionally, due to its nontoxicity, the pharmaceutical and food industries generally consider it a harmless chemical (Varona et al., 2008).
Utilising microwave energy is another extraction technique (Chemat et al., 2003). The use of microwave dielectric heating for the extraction of EOs has received a lot of attention. Examples include microwave-accelerated steam distillation, vacuum microwave hydrodistillation, compressed air microwave distillation, microwave hydrodiffusion, microwave hydrodistillation, solvent-free microwave extraction, microwave steam distillation, and gravity (Farhat et al., 2010).
Low microwave heating and distillation that occur at atmospheric pressure are the foundation of solvent-free microwave extraction. The high yield of EO, low energy needs, and excellent purity of the extracted oil are some of the benefits of this process. It also quickly reaches the extraction temperature of 100 °C for the first EO droplet (Lucchesi et al., 2004). On the other hand, a future extraction procedure that can provide excellent repeatability in less time, streamlined manipulation, decreased solvent consumption, and lower energy input is the extraction of EOs under microwave irradiation without including any organic solvent or water.
Using gravity and microwave hydrodiffusion is a productive, affordable, and environmentally beneficial strategy (Bousbia et al., 2009). This technique was developed to extract volatile chemicals from freshly harvested plant materials that have at least 60% initial moisture (Bousbia et al., 2009). When microwave energy is applied, the natural water within the plant tissue heats up, distorting the plant cells and causing them to burst and fall through gravity without the use of any solvent or water. A further example of a green microwave design is microwave steam diffusion (Farhat et al., 2010). The speed and energy savings of microwave hydrodiffusion, microwave steam diffusion, and gravity processes are their most notable advantages. However, for the EOs to be released from the plant materials, both methods require the presence of water. On the other hand, EOs can be extracted from dried plant materials without the use of any solvent or water (Farhat et al., 2010).
Gas chromatography (GC) (quantitative analysis) and GC–mass spectrometry (GC/MS) (qualitative analysis) are the most used technologies for the chemical analysis of EOs (Keravis et al., 1997). As previously stated, the key components are established by comparing the GC retention periods and mass spectrometry (MS) data to those of known reference standards (Lahlou et al., 2001a). The conditions and procedures used for the analysis should be thoroughly described. They included the oil analysis apparatus, column type and size, carrier gas flow rate, and temperature programming parameters such as injector temperature, detector temperature, and column temperature, in addition to mass spectra.
The compound retention index measured against a reference standard mixture—commonly alkanes—overcomes many of the column variable shortcomings. Most often, the isothermal retention index must specify the phase and should indicate the temperature of analysis when quoting the index value (Bizzo et al., 2023). Just as retention time is not unique, so the equivalence of the retention index is a necessary but insufficient condition for identification. While Kováts’ indices refer to GC performed under isothermal conditions, temperature-programmed GC analysis is more often conducted. An extension of the Kováts indices system to non-isothermal conditions was proposed by Van Den Dool and Kratz (1963). Thus, a retention index should specify whether it applies to isothermal or temperature programmed analysis and then the actual temperature conditions. Apparently, there should be little scope for the incorrect application of retention index values; however, the way reference data are sourced and applied to the “identification” process somewhat uncertain (Bizzo et al., 2023). Retention indices (Kováts’ indices) on two columns of opposite polarity or on the same column but at a different temperature (Lahlou, 2003) must sometimes be employed to validate identification by GC/MS (Denayer and Tilquin, 1993). Co-injection with authentic compounds should also be used to substantiate claims for the identification of new constituents. As a result, data on EO optical rotation, density, and refractive index (Lahlou et al., 2001a) should be included. Moreover, isothermal Kováts’ retention indices are determined according to a formula reported by Van Den Dool and Kratz (1963), Babushok et al. (2011), and Kovats (1958).
Carbon-13 nuclear magnetic resonance (13C NMR), on the other hand, is utilized to analyse chemicals that are difficult to separate using GC as well as molecules with equivalent structural features, such as the stereo-isomeric components of EOs (Tomi et al., 1997). This method is also used to investigate chemical intraspecific variation and may be used to assess EO quality.
High-performance liquid chromatography (HPLC), on the other hand, has been shown to be adaptable and sensitive for detecting EO volatiles (Turek and Stintzing, 2011a). The application of HPLC in the field of volatile chemicals was primarily limited to sample cleansing (Schieberle et al., 1987) or prefractionating processes prior to GC analysis (Jones et al., 1979; Morin et al., 1986; Nilsson et al., 1996). Schwanbeck and Kubeczka (1979) reported on the effective separation of many volatile hydrocarbons in compound combinations, while specific aroma terpenoids in EOs were detected by HPLC in rare situations (Solinas et al., 1981; Newbery et al., 1983; Mohammed, 2009; Wang and Liu, 2012). Ultraviolet–visible spectroscopy (UV-Vis) or even diode array detectors (DADs), which provide spectral information simultaneously for each chromatographic peak, were previously almost exclusively used for the detection of EO volatiles due to their unpredictability and selectivity. Fixed wavelengths, ranging from 195 nm (Ogawa et al., 2002) to 275 nm (Kovar and Friess, 1980), were generally tailored to the compounds’ particular spectrum absorption behavior. The simultaneous selection of multiple characteristic wavelengths has been reported to ensure the identification of a wide range of chemicals generated from distinct chemical classes, even when structurally comparable components such as α- and γ-terpinene were too similar to be chromatographically distinguished (Turek and Stintzing, 2011a). Liquid chromatography combined with mass spectrometry (LC/MS) is a strong analytical method for compound identification. Turek and Stintzing (2011a) created a previously unknown approach to fully examine chemicals in diverse EOs using HPLC/DAD coupled in series with tandem MS working with atmospheric pressure chemical ionization in the positive mode. They concluded that the inferior separation capacity of HPLC compared to GC due to a lower number of theoretical plates could partially be compensated by the benefit of several detection modes in series such as ultraviolet (UV) absorption combined with MS and the ability to simultaneously monitor at multiple wavelengths when using a DAD (Turek and Stintzing, 2011a; Turek et al., 2012).
EOs are concoctions of various volatile chemicals. These oils generally have a solid to liquid consistency, lipophilicity, optical activity, and a distinct odor. In choosing the oil for the formulation of perfumes and eau de toilettes, as well as for pharmaceutical preparations or food ingredients, this last characteristic is crucial. The most significant factor, however, is the activity of the substances present in the volatile aromatic combination. Monoterpene, sesquiterpene, diterpene, and derivatives of phenylpropane make up the multi-component combinations that comprise EOs. More than 1,500 molecules, including hydrocarbons, alcohols, aldehydes, ketones, and esters, have been found in the chemical makeup of various EOs. Sulfur and nitrogen (N) compounds, acetylene derivatives, coumarins, and organic acids are also present (Alizadeh et al., 2010; Baj et al., 2010; Tajuddin and Yusoff, 2010; Nurzyńska-Wierdak, 2012). Natural oils are extremely diverse chemical substances, and so various mechanisms in plants are likely used during their manufacture (Dubey et al., 2003; Lattoo et al., 2006). Many pharmacognostic materials termed “EOs raw material” have EOs as their primary active ingredient. This is one of the largest classes of medicinal raw materials with a long history and widespread therapeutic use (Şahin et al., 2004; Chung et al., 2010). The principal biological activities of EOs include antifungal, antiviral, antibacterial, antimutagenic, anticancer, antidiabetic, anti-inflammatory, and antiprotozoal properties (Raut and Karuppayil, 2014).
Because precise understanding of the chemical constitution and qualities of EOs is required for effective usage, this study combines and discusses the disparate evidence on aspects that influence EO stability, including internal factors (genetics: species, ecotype, chemotypes, cultivars, varieties; plant development; part of the plant used), external factors (environmental and cultivation conditions: climatic and radiation conditions, diurnal and annual rhythm; harvesting-related factors: latitude of cultivation, planting and sowing dates, irrigation, fertilizer variations, salinity, growth retardants; postharvest techniques: drying methods, plant storage; extraction, distillation time, and conditions of analysis: extraction methods, moisture and acidity of distillation water, distillation time, and conditions of GC analysis), oil degradation during storage (light, temperature, oxygen availability, compound structure, chemical composition, and metal contaminants), and factors affecting the bioactivity of essential oils (effect of the treated plant part, effect of the solvent/detergent, the correlation composition-activity, technique used, biological material, choice of the doses/concentrations, reference compounds, and comparison with literature data).
2 Materials and methods
This review conducted a bibliographic investigation from 1950 to 2023 to analyse classic textbooks and peer-reviewed works and access globally recognised scientific databases. The NISCAIR, INFLIBNET, SCIELO, SCOPUS, PUBMED, and Google Scholar databases were used to find peer-reviewed papers. Only relevant papers published in English were considered. Following verification from published literature and a database, the botanically correct names are given.
3 Results of factors affecting essential oil composition, and therapeutic potential
Numerous factors affect EOs’ composition pattern, component concentrations, and yield.
3.1 Internal factors
3.1.1 Genetics
Genetics (species, ecotype, chemotype, cultivars, varieties; plant development; part of plant used) have an impact on the type and quantity of EOs.
3.1.1.1 Species
Species name is the basic unit of classification of plants. It describes one kind of plant within a genus. The chemical makeup of the EO extracted from three wild species of Moroccan thyme (T. satureioides Coss., T. willdenowii Boiss., and T. zygis L.) was studied and compared. Carvacrol (44.95%) and p-cymene (23.36%) were found to be the most prevalent components in T. zygis L. EO. Thymol, carvacrol, and p-cymene were discovered to be the main volatile components in T. willdenowii Boiss. EO. Carvacrol (27.57%) and borneol (21.56%) make up the majority of T. satureioides Coss. EO. On the other hand, the two main ingredients in T. zygis L. were carvacrol (72.33%) and thymol (10.70%). Thymol (65.01%) and carvacrol (21.74%) predominated in T. willdenowii Boiss. Carvacrol (47.35%), borneol (25.04%), β- and ɣ-terpineol were all present in considerable concentrations in T. satureioides Coss (Moukhles et al., 2022). Additionally, Limam et al. (2020) examined the leaf EO diversity of 13 Eucalyptus species, finding that the majority of these species were abundant in the key component 1,8-cineole (82.64% in E. microcarpa) (Limam et al., 2020). A combination of chromatographic and spectroscopic methods has been used to analyze the fruit oil of five species of kumquat (Fortunella japonica, F. margarita, F. crassifolia, F. obovata, and F. hindsii) cultivated in the same pedoclimatic circumstances. Limonene (84.2%–96.3%) had a substantial dominance over the compositions of the five fruit oils. Myrcene (1.3%–12.9%) and germacrene D (0.3%–2.4%) were additional substances that were detected in significant amounts (Sutour et al., 2016). EO distillation of Salvia dugesii and S. gesneriiflora yielded 0.08% and 0.07%, and their chemical compositions revealed the presence of 27 and 25 compounds as main components, respectively. Caryophyllene is highlighted as the most abundant component in essential oils from both studied plants. Bornyl acetate, valeranone, monocyclic geranyl-α-terpinene, and hedycaryol (Lo et al., 2005) showed relative concentrations over 5% in S. gesneriiflora, which is a native species in Mexico. Bicyclic compounds, including spathulenol, caryophyllene oxide, and γ-muurolene, are predominant in S. dugesii (Calderón-Oropeza et al., 2021).
3.1.1.2 Ecotype
Ecotypes are plants which belong to the same species but possess different phenotypical features because of environmental factors such as elevation, climate, and predation. Ecotypes can be seen in wide geographical distributions and may eventually lead to speciation (Mayr, 1947). Kaškonienė et al. (2016) examined the impact of ecotypes on the yield and composition of EOs in seven samples of the Lithuanian plant Chamerion angustifolium (L.) Holub, which was gathered in six different locations; anethole and caryophyllenes (α- and β-) were discovered in all samples. Only three samples had a high concentration of caryophyllenes (α- and β-), making up 54.0–78.5% of the EOs. Two of the samples, collected in Panara, had an anethole concentration of more than 51%, while the sample (from Aleksotas) stood out for having a rather high level of cis-arbusculone (8.5%) (Kaškonienė et al., 2016). Four ecotypes of Citrus deliciosa var. tangarina (Fina Clementine, Nour Clementine, Spinosa Clementine, and Thornless Clementine) were examined in another study for the EOs of their peel and leaf. Three oil samples under evaluation contained limonene as their primary element, and the Nour Clementine leaves contained sabinene as their primary constituent. Generally, total hydrocarbons outnumbered total oxygenated compounds in percentage, and total monoterpenes outnumbered total sesquiterpenes (El-hawary et al., 2013). Citrus sinensis Osbeck (forma Salustiana, forma Valencia, and forma Washington navel) were grown in Kenya. The volatile components of their peel EOs were isolated by cold pressing and were identified by GC and GC-MS. Salustiana, Valencia, and Washington navel peel oils have totals of 56, 73, and 72 components, respectively. The detected elements made up, respectively, 98.7%, 97.8%, and 97.4% of the total volatiles in each oil. The volatile percentages of the Salustiana (96.9%), Valencia (94.5%), and Washington navel (92.7%) oils were predominantly dominated by monoterpene hydrocarbons. The main constituents in each oil were limonene, α-pinene, sabinene, and α-terpinene. Valencia and Washington navel oils had 0.1% of the total volatiles as sesquiterpene hydrocarbons, with (E,E)-farnesene as the predominant component. Salustiana, Valencia, and Washington navel volatiles contained 1.7%, 3.4%, and 4.5% of the total oxygenated compounds, respectively. The key ingredients were linalool, decanal, (Z)-carvone, (Z)-carveol, (E)-carveol, nootkatone, and sabina ketone. The presence of β-phellandrene and α-terpinene in Salustiana, α-phellandrene (Z)-nerolidol, and aromadendrene in Valencia, and p-cymene, α-sinensal, and dodecanoic acid in Washington navel oils can distinguish the three sweet Citrus kinds (Njoroge et al., 2005).
3.1.1.3 Chemotypes
A “chemotype” is plants which belong to the same species that have the same morphological characteristics (relating to form and structure) but produce different quantities of chemical components in their EOs (Clarke, 2008). Rosmarinus officinalis EO chemotypes from eight sites in north Tunisia were studied. According to analyses, the principal constituents of the oils include 1,8-cineole (44.5–57.88%), (+)-camphor (9.27–18.99%), α-pinene (9.18–3.66%), borneol (13.21%), and camphene (3.15–4.69%). Three chemotypes were found, with the first one predominating: 1,8-cineole/camphor/α-pinene, 1,8-cineole/camphor, and camphor/1,8-cineole (Abada et al., 2020). The content and composition of EOs from Juniperus oxycedrus L. sensu lato collected at 20 populations (interpopulations) across Bulgaria were examined. The leaf EOs yield ranged from 0.03% to 0.20% (interpopulation) and from 0.04% to 0.18% (intrapopulation), but the galbuli EOs ranged from 0.83% to 2.6% (interpopulation) and from 1.2% to 1.9% (intrapopulation), respectively. Galbuli EO’s major chemical class ranged from 53.4% to 69.5% (intrapopulation) and was monoterpenes, whereas leaf EO’s dominant chemical class ranged from 46.2% to 67.4% (intrapopulation) in sesquiterpenes. To categorise the populations into eight chemotypes, the major constituents of leaf EOs (α-pinene, limonene, α-curcumene, γ-cadinene, δ-cadinene, manoyl oxide, germacrene D, β-caryophyllene, α-caryophyllene, caryophyllene oxide) were considered. Semerdjieva et al. (2020) established 27 chemical groups for their interpopulation study. Due to its antibacterial properties, the triketone chemotype of manuka, Leptospermum scoparium, has significant commercial value. On the East Cape of New Zealand, oils from 36 different plants all had high triketone concentrations (>20% total triketones), with no seasonal change. The high triketone chemotype was localised to the East Cape, according to analyses of oils from 261 individual manuka plants gathered from 87 sites across New Zealand, while oils with triketone levels as high as 20% were discovered in the Marlborough Sounds region of the South Island. Ten further chemotypes were listed: high geranyl acetate, sesquiterpene-rich with high c-ylangene, α-copaene, and elevated triketones, sesquiterpene-rich with no distinguishing components, sesquiterpene-rich with high trans-methyl cinnamate, high linalol, and sesquiterpene-rich with elevated elemene and selinene. One of the most noticeable chemotypes, with a high concentration of geranyl acetate in the oil, contained aroma components at rather high levels (Douglas et al., 2004). Variations in EO chemotype chemical compositions impact their biological activity (Abada et al., 2020; Melo et al., 2018; Melo et al., 2021; Peixoto et al., 2015; Salem et al., 2015; Blanco et al., 2013). An investigation of European Origanum vulgare focused on the qualitative and quantitative composition of essential oil compounds isolated from 502 individual O. vulgare plants from 17 countries. The content of EO compounds of O. vulgare ranged between 0.03% and 4.6%. The monoterpenes were primarily made up of sabinene, myrcene, p-cymene, 1,8-cineole, β-ocimene, ɣ-terpinene, sabinene hydrate, linalool, α-terpineol, carvacrol methyl ether, linalyl acetate, thymol, and carvacrol. Among the sesquiterpenes, β-caryophyllene, germacrene D, germacrene D-4-ol, spathulenol, caryophyllene oxide, and oplopanone were often present in higher amounts. According to the proportions of cymyl-compounds, sabinyl-compounds, and acyclic linalool/linalyl acetate, three different main monoterpene chemotypes were defined (Lukas et al., 2015).
The yields of Origanum majorana essential oils from Kathmandu and Bhaktapur were found to be 0.5% v/w (50 compounds—91.2%) and 0.8% v/w (41 compounds—98.8%), respectively (Paudel et al., 2022). For O. majorana oil Kathmandu, terpinen-4-ol (32.1%), linalool (13.8%) and γ-terpinene (9.5%) were the most common compounds, followed by linalyl acetate (5.9%), α-terpinene (5%), cis-sabinene hydrate (4.4%), α-terpineol (3.7%), terpinolene (2.5%), bornyl acetate (2.4%), β-caryophyllene (2.4%), cis-p-menth-2-en-1-ol (1.8%) and p-cymene (1.8%) in smaller amounts. Similarly, in O. majorana essential oils from Bhaktapur, terpinen-4-ol (33.35%), linalool (15.37%), p-cymene (6.90%), and linalyl acetate (6.67%) were the most common compounds, followed by cis-sabinene hydrate (3.48%), 1,4-hydroxy cineole (3.35%), bornyl acetate (2.83%), α-terpineol (2.63%), and caryophyllene oxide (2.54%) in smaller amounts. Terpinen-4-ol is the major oxygenated monoterpene found in the EO of the two samples, in which dextrorotatory (+) enantiomer is the most predominant chiral compound. In this study, the essential oil of O. majorana essential oils from Bhaktapur has nearly the racemic mixture of terpinen-4-ol, (+) 52.6% and (−) 47.4%, whereas terpinen-4-ol, (+) 58.31%, is dominant over terpinen-4-ol, (−) 47.69% for O. majorana EOs from Kathmandu. In both EO samples, (−) β-caryophyllene, (−) bornyl acetate, and (−) linalyl acetate were detected as enantiomerically pure, with 100% in levorotatory form. Camphene is the enantiomerically most dominant component that exists in levorotatory form with slight variations, including (−) 96.8% to (−) 97.87% in both EO samples, which is followed by (+) sabinene and (+) cis-sabinene hydrate, with the variation in the enantiomeric distribution in this study. The antioxidant activity for O. majorana EOs from Bhaktapur was found to be relatively moderate, with an IC50 value of 225.61 ± 0.05 μg/mL and an EC50 value of 372.72 ± 0.84 μg/mL, as compared to the standard used. Furthermore, with an MIC value of 78.1 μg/mL, O. majorana EOs from Bhaktapur demonstrated effective antifungal activity against Aspergillus niger and Candida albicans. Moreover, both samples displayed considerable antimicrobial activity (Paudel et al., 2022).
3.1.1.4 Cultivars
A cultivar is a plant that is propagated through human intervention to develop a desirable characteristic and to ensure it retains the characteristics of its parent plant. Cultivars are created from stem cutting, grafting, tissue cultures, or cross-pollination (Merckx et al., 2013). Verma et al. (2013) investigated the effect of cultivars on the production and makeup of Pelargonium graveolens—the rose-scented geranium. In India’s Western Himalayan region, three cultivars—Bourbon, CIM-Pawan, and Kelkar—were grown. It was discovered that Kelkar’s chemical makeup differed from those of the other two cultivars (Verma et al., 2013). A similar investigation was conducted on the EOs of 17 cultivars of fresh Indian Zingiber officinale (Ravi Kiran et al., 2013). The Assam fiberless cultivar stood out among the other cultivars investigated for having the highest EO production (4.17%) and the highest monoterpene hydrocarbon content (38.65%) compared to sesquiterpene hydrocarbon (25.38%). Assam Tinsukia had the greatest citral content (23.66%), and Meghalaya Mahima had the highest zingiberene content (29.89%) of all these cultivars.
3.1.1.5 Varieties
A plant “variety” is found growing and reproducing naturally in the plant kingdom. It varies from its standard species in some way because of natural evolution. Plant varieties are most often found in groupings that have cross-pollinated as adaptations to differences in habitat. Plants grown from the seeds of a species variety are often exact copies of the parent plant (Clarke, 2008). The EO concentration of Daucus carota variety “Cubic” roots was higher than that of D. carota variety “Nanco F1” roots, and the same was true for the leaves of both kinds (Habegger and Schnitzler, 2000). The composition of D. carota leaf EOs varied greatly between types (Kainulainen et al., 1998). Carum carvi EO content is reduced from the “Rekord,” “Prochan,” and “Kepron” varieties to the “Konczewicki” kind (Sedlakova et al., 2001). Additionally, research found that several C. carvi types had 1–9% (m/m) of the EOs that give C. carvi its distinctive flavor and aroma. Compared to the varieties “Dulce” and “Selma,” the sweet Foeniculum vulgare variety “Zefa Fino” recorded the highest levels of EO content in the leaves and bulbs (Semiz et al., 2012). F. vulgare varieties “Sweet Fennel” and “Shumen” had the lowest and greatest EO contents, respectively (Bowes and Zheljazkov, 2004). Variation significantly changed the ratio of the following compounds in Petroselinum crispum’s EOs: β-phellandrene, 1,3,8-p-menthatriene, α-p-dimethylstyrene, myristicin, α-myrcene, and apiole (Petropoulos et al., 2004). The leaves of diverse D. carota kinds (“Flakker 2,” “Nantura,” “Parano,” “Napoli”, “Panther,” “Splendid,” “Nantes 3 Express”) have considerably diverse oil compositions (Kainulainen et al., 2002). The oil output and content of the Coriandrum sativum varieties “Jantar” and “Alekseevski” were different (Zheljazkov et al., 2008).
3.1.2 Plant development
“Plant development” includes all the changes during a plant’s life cycle (Lyndon, 2012).
The age of the plant being harvested is also very significant since it determines not only how much EO is produced overall but also how much of each component is generated in what quantities. Some instances of these ontogenetic differences are Mentha piperita oil, which demonstrated that while immature plants had relatively high proportions of pulegone, menthone and menthol took their place as the leaves matured. Mentha spicata oil has shown a progression from young plants dominating with carvone to older plants dominating with dihydrocarvone. Monoterpenes prevail in oils from the leaves and flowers of Achillea millefolium during flowering, while sesquiterpenes make up the majority of the oil harvested during the vegetative season. Pimpinella anisum plants were gathered at ten stages of development (Oezel, 2009). The fourth step of harvesting generated the maximum output of EOs. The highest-quality EOs with a high (E)-anethole content were produced during the first and second stages of harvesting. Green seeds (green color), mature seeds (yellow), and dry seeds (straw yellow) were the three fenological stadia of F. vulgare harvest evaluated by Stefanini et al. (2006a). Their findings indicated that seeds should be plucked when they are still green to obtain more EOs. Pimpinella anisum seeds picked during the waxy stage had more oil than those harvested earlier or later (Zehtab-Salmasi et al., 2001). Sweet F. vulgare seeds were gathered at the stages of full bloom, waxy seeds, and seed maturing. Plants picked at waxy seed maturity stage produced the most oil (Marotti et al., 1992). According to Osman and El-Wahab (2009), harvesting P. crispum or Anethum graveolens only once or twice to acquire fresh herb reduced plant weight at the flowering stage, which resulted in fewer umbels, seeds, and plants with more oil per plant than plants that were not harvested.
The EO concentration in the vegetative organs of C. sativum (Olle et al., 2010), turnip-rooted Anthriscus cerefolium (Mamedova and Akhmedova, 1991), and other plants gradually rises with plant development and reaches a maximum in the overground sections at the flowering stage. The vegetative organs gradually dry out during the fruit ripening period; as the oil canals flatten, their EO content decreases. The organs’ oil content peaks early in the roots’ growth, during the flowering stage with the stems, and at plant maturity with the leaves. C. carvi, D. carota, and C. sativum all had lower total oil contents in samples taken before full ripening than in samples taken at full ripeness (Gonny et al., 2004). The ratio of the compounds β-phellandrene, 1,3,8-p-menthatriene, α-,p-dimethylstyrene, myristicin, β-myrcene, and apiole was altered significantly by the P. crispum growing stage (Petropoulos et al., 2004). When fruits first formed, a rapid oil accumulation began, and it persisted until the fruits were fully mature (Msaada et al., 2009a). C. sativum’s EO makeup altered dramatically as it matured through the various stages (Msaada et al., 2009b). At the first stage of maturity (immature fruits), the primary chemicals were geranyl acetate (46.27%), linalool (10.96%), nerol (1.53%), and neral (1.42%). Linalool (76.33%), cis-dihydrocarvone (3.21%), and geranyl acetate (2.85%) were noted as the primary ingredients at the middle stage. Linalool (87.54%) and cis-dihydrocarvone (2.36%) make up the majority of the EOs in mature fruits at the peak of their development (Msaada et al., 2007). Sweet F. vulgare’s EO content was assessed at four stages of maturation: immature, premature, mature, and fully mature. Results showed that as fruits grew older, their EO concentration decreased (Telci et al., 2009). The parallel optimal curves in the vegetative and generative organs of C. carvi and F. vulgare, with maximum points at various times and different levels, can be used to identify the accumulation propensity of EOs in these plants (Németh, 2003).
3.1.3 Part of the plant used
In comparison to the overall leaf and stem, A. graveolens EO content was highest in the leaf blade and lowest in the petiole (Lisiewska et al., 2007). Compared to older leaves, young D. carota leaves had higher concentrations of α-asarone, methylisoeugenol, and propenylbenzenes. Additionally, they discovered that the EO makeup of leaflets and petioles differed significantly (Kainulainen et al., 1998). Compared to the roots, D. carota leaves had a much higher quantity of EOs (Habegger and Schnitzler, 2000). The majority (72–84%) of oils extracted from various wild D. carota plant sections were monoterpene hydrocarbons (Staniszewska et al., 2005). According to a multivariate analysis, the basal leaves, roots, and stems of C. sativum and its higher leaves, flowers, and other parts share a significant degree of similarity in EO composition (Msaada et al., 2007). The leaf oil of Laserpitium gallicum L. exhibited a similar chemical make-up to fruit oil (Chizzola, 2007). The EO components of the stems, umbels, fruits, and roots of Astrodaucus orientalis (L.) were examined by Nazemiyeh et al. (2009). Sabinene (23.1%), α-pinene (16.4%), and fenchyl acetate (7.5%) comprised the majority of the stem’s constituents. The three principal components of the EOs of the fruits were sabinene (25.6%), β-pinene (22.3%), and α-copaene (16.1%). While the EOs of the umbels were primarily made up of α-copaene (26.1%), β-pinene (15.3%), and sabinene (13.7%), those of the roots were primarily made up of anisole (37.9%), bornyl acetate (36.9%), and geranyl tilgate (11.4%). F. vulgare dry seeds had the largest trans-anethole content (78.25%) throughout the summer, limonene (42.30%) in spring stems/leaves, and fenchone (16.98%) in green seeds during the autumn (Abd El-Kareem et al., 2024). EOs from the many parts of Eryngium glaciale, including its inflorescences, stems, leaves, and roots, were examined by Olle et al. (2010). Inflorescence oil contained (E)-caryophyllene (15.2%), phyllocladene (43.5%), and valencene (11.5%), while phylloclaene isomer (41.3%) from stems and leaves was their primary oil. Linalool (19.1%) and the phyllocladene isomer (49.4%) were the two main components of the root oil.
3.2 External factors
3.2.1 Environmental and cultivation conditions
Different environmental and cultivation conditions have an impact on the growth and development of plants that produce EOs as well as the type and quantity of EOs.
3.2.1.1 Climatic and radiation conditions
For medicinal plants, there are different relationships between climatic conditions and EO content (Moghaddam et al., 2023). C. sativum oil yields are highest in the coolest, wettest seasons (Olle et al., 2010). In addition, the ratios of the following chemicals varied significantly depending on the climate, which had a substantial impact on the EO composition of P. crispum: 1,3,8-p-menthatriene, β-phellandrene, α-,p-dimethylstyrene, β-myrcene, myristicin, and apiole (Petropoulos et al., 2004).
Higher temperatures often promote the creation of EOs, while particularly hot days could result in an excessive physical loss of oil. Leaching caused by persistent rain can cause the loss of EOs in leaves and roots (WC, 2002). Menthone, menthol, and small amounts of menthofuran have been found in M. piperita L. leaves grown under long-day settings; menthofuran is a prominent component of the EOs in plants cultivated under short-day conditions. Additionally, the reduction pathway is activated with the conversion of menthone to menthol when immature leaves have a long photoperiod. A significant increase (approximately ten-fold) in aromatic compounds, including benzyl alcohol, was found at night in studies on the day–night variations in the relative concentrations of volatiles from flowers of Nicotiana sylvestris and other species, but no such increase was seen in other EO constituents (such as linalool or caryophyllene) (Loughrin et al., 1990). It is also crucial to consider the type of radiation that plants receive. Johnson et al. (1999) discovered that with Ocimum basiclicum, more UV-B radiation enhances levels of both phenylpropanoids and terpenoids in the leaves in comparison to herbs grown under glass and receiving no UV-B radiation. Karousou et al. (1998) raised two chemotypes of M. spicata that were exposed to elevated UV-B radiation, which is comparable to the l5% ozone depletion over Patras, Greece, in regards to aromatic plants. The therapy increased the production of EOs in one chemotype, while the other showed a comparable but unimportant trend.
3.2.1.2 Diurnal and annual rhythm and harvesting-related factors
The amount of EOs varied depending on the time of day and night. C. sativum EO concentration variations during the day were investigated by Ramezani et al. (2009). EO yields (w/w-%) at various times were in the range of 6 (0.432%), 12 (0.436%), 18 (0.404%), and 24 (0.319%) treatments because EO concentration at midday was higher than at other times.
The time of year that the aromatic plant is gathered is typically of great significance. Karamanos and Sotiropoulou (2013) examined Greek O. vulgare oil harvested in two successive seasons to determine the effect of harvesting season on EO output and composition. From approximately 1.5% to 2.0% in the dry warm season to approximately 5.5% in the following wet cold season, the concentration of EOs per plant increased dramatically. Inflorescences had a higher concentration of EOs than leaves. Carvacrol, the primary component of O. vulgare EOs, was more abundant in both leaves and inflorescences during the drier and warmer seasons (70.75–84.88%), while other compounds tended to accumulate at higher levels during the wetter and colder season with only slightly higher levels of carvacrol (56.46–75.12%) (Karamanos and Sotiropoulou, 2013). Another crucial factor in harvesting is the time of day. In Kiyombe (Rwanda), a study on the rose-scented P. graveolens investigated how the time of day affected the yield of EOs. Before EOs were extracted by steam distillation, the P. graveolens plants were manually gathered at 10:00 a.m., 12:00 noon, 2:00 p.m., and 4:00 p.m. They were then allowed to dry for 17 h in the shade. The maximum EO concentration (0.22%) was found in the P. graveolens plants that were collected in the early afternoon (2:00 p.m.) (Malatova et al., 2011).
3.2.1.3 Latitude of cultivation
Medicinal plants have different relationships between latitude and EO content (Moghaddam et al., 2023). C. sativum grown in Lithuania had more EO concentration than that grown in southern Russia (Olle et al., 2010). Johreniopsis seseloides from Teheran and Kurdistan were collected by Fouladi et al. (2006). Monoterpenes (24.05%) and sesquiterpenes (43.19%) were present in the EOs of J. seseloides from Teheran, while those from Kurdistan province had a high monoterpene content (69.67%) and a low sesquiterpene content (26.69%). Between the two growing zones under study, the EO content of C. sativum saw a substantial alteration (Msaada et al., 2007).
3.2.1.4 Planting and sowing dates, and irrigation
Planting and sowing dates were reported to influence the composition of EOs (Delibaltova, 2020). The planting date of P. crispum significantly changed the content of β-phellandrene, 1,3,8-pmenthatriene, α-,p dimethylstyrene, myristicin, β-myrcene, and apiole (Petropoulos et al., 2004). Belgium, and Rafieiolhossaini et al., 2009 investigated the impact of planting date and seedling age on Matricaria chamomilla according to agro-morphological parameters, EO content, and composition. Four distinct planting dates (15 April, 1 May, 15 May, and 30 May) and five different seedling ages (30, 45, 60, 75, and 90 days) were used in the treatments, which were reproduced three times in a randomized full block design. All the agro-morphological parameters were significantly influenced by the planting date. Early planting dates yielded the highest values for them, whereas later planting dates saw values decline. Planting date had a considerable impact and negative relationship with days after sowing (DAS) and days after transplanting (DAT) to flowering. The chemicals that were significantly influenced by planting date for seedlings that were 30 days old were (E)-farnesene, α-bisabolol oxide A, α-bisabolone oxide, and spathulenol; the highest levels were obtained for the latest planting date. The only agro-morphological traits that were significantly impacted by seedling age at transplanting were plant height and spread. DAS to flowering was significantly enhanced by seedling age, although the effect varied with age. The only compound whose first planting date was significantly impacted by seedling age at transplanting was spathulenol, with 90-day-old seedlings having the maximum yield (Mohammad et al., 2010). Reduced watering frequency caused water stress, which raised P. crispum and F. vulgare’s EO percentages (Mohamed and Abdu, 2004). With watering, F. vulgare’s output of EOs also increased (Mohamed and Abdu, 2004). C. sativum seeds’ EO content increased due to irrigation, although the percentage of linalool in the EOs decreased (Arganosa et al., 1998). P. anisum oil production was decreased by a water shortage during stem elongation and umbel development (Zehtab-Salmasi et al., 2001).
3.2.1.5 Fertilizer variations
Fertilisers are utilized to maintain soil fertility and enhance plant growth and development. Less N applied to D. carota produced more EOs (Schaller and Schnitzler, 2000). C. sativum produced the most oil when treated with ammonium nitrate, but A. graveolens responded better to urea. Lower nutrient concentrations (1.2, 2.4, and 3.6 mS/cm) led to an increase in EO content in A. graveolens leaf (Udagawa, 1994). In curl leaf P. crispum, the content of EOs declined with increasing N rate (3.2, 16.4, 32.4, and 48.6 g m−2) but was unaffected by N treatment in the roots and leaves of both turnip-rooted and plain leaf P. crispum (Petropoulos et al., 2009). In two successive crops, the growth, foliar EO composition, and yield of A. graveolens L. “Ducat” were examined in relation to N fertilization, cultivation season, and harvest stage. For the autumn–winter crops, seeds were seeded in October, and for the spring crop, in January. In a randomised design, nitrogen (NH4NO3) was supplied at four different amounts (50, 150, 300, and 450 ppm). After sowing, the plants were harvested after 158 days (for the autumn–winter crop) and 83 days (for the spring crop). Weighing the plant leaf allowed the separation and analysis of the EOs. The maximum fresh weight of leaf per plant was reached at 300 ppm of N in the spring, but it gradually grew with increasing N up to 450 ppm in the autumn and winter. In the autumn and winter, N treatment had no effect on the amount of EOs present in the leaf; however, in the spring, the concentration of EOs was much higher at 300 ppm N than at other N levels. The primary ingredients in foliar EOs were phellandrene, A. graveolens ether, phellandrene, phellandrene, thujene, myrcene, and cymene. Phellandrene was the main component in both crops. In the autumn–winter crop, the concentrations of phellandrene, β-phellandrene, and A. graveolens ether were also higher at 300 ppm N, but the other constituents were unaffected by higher N. In spring, the concentration of all the EO constituents identified (aside from π-cymene) was highest at 300 ppm N. Consequently, for the autumn–winter crop, 450 ppm N was ideal for biomass and EO yield, whereas 300 ppm N was advised in the spring (Tsamaidi et al., 2010). The effects of four phosphorus levels (0, 20, 40, and 60 kg ha−1) on the production and content of EOs in Cuminum cyminum were examined by Tuncturk and Tuncturk (2006). Their findings indicated that the treatment of 40 kg ha−1 P2O5 produced the maximum oil content and EO yield. Using solutions of NiSO4 in varying concentrations, P. crispum plant soil was enriched with 0, 25, or 100 mg of Ni per kilogram of soil. The findings of this experiment demonstrated that P. crispum oil yield was significantly increased by modest amounts of Ni fertilization, notably 50 mg kg−1 in clay soil (Atta-Aly, 1999). Organic fertilizers (2.5 kg m−2) considerably increased oil yield in F. vulgare (Mohamed and Abdu, 2004).
3.2.1.6 Salinity
With an increase in salt, the oil content of sweet F. vulgare seeds gradually reduced (Ashraf and Akhtar, 2004). Based on dry matter weight, C. sativum EO yield was 0.06% in the control and did not vary at low concentrations (25 mM), but it rose considerably with increasing sodium chloride (NaCl) concentrations to reach 0.12% and 0.21% at 50 and 75 mM NaCl, respectively (Neffati and Marzouk, 2009). When F. vulgare plants were irrigated with saline water (3,355 ppm), the oil output per plant decreased and the anethole content of the oil decreased to 23.2% (Abd El-Wahab, 2006). With 25 and 50 mM NaCl, respectively, the C. sativum EO output significantly increased to 18% and 43%, and it significantly decreased with high salinity (Neffati and Marzouk, 2008).
3.2.1.7 Growth retardants
C. carvi and F. vulgare plants were sprayed with the growth inhibitor (B-9) in concentrations ranging from 500 to 4,000 ppm, which increased the EO content in the seeds. By spraying the plants with 4,000 ppm B-9, maximum oil output was attained (Abou-Zied, 1974).
3.2.2 Postharvest techniques
Drying methods and storage have an impact on the growth and development of plants that produce EOs and frequently on the type and quantity of EOs.
3.2.2.1 Drying methods
One of the most popular preservation techniques is drying, which can increase the shelf life of plants by lowering their moisture content. This means that, depending on their nature and environmental factors, drying procedures may cause the degradation of heat-sensitive plant metabolites and a drop in quality. On Citrus sp. peel, the effects of seven drying techniques—sun, shade, oven, hoover oven, microwave, and freeze drying—were examined. Results revealed that the freeze-dried sample had the greatest EO extraction yield (6.90%) (Farahmandfar et al., 2020). The EOs of M. piperita L. leaf were quantitatively and qualitatively analyzed using various drying techniques. The leaves were dried in thin layers in a hot-air dryer at 50, 60, and 70 °C as well as in a microwave oven at 200, 400, and 800 W. Hydrodistillation was used to extract EOs from the fresh and dried samples, which were then analysed using GC/MS. The hot air-dried leaf at 50 °C and the microwave-dried leaf at 800 W yielded the highest (22.24 g/kg dry matter) and lowest (1.33 g/kg dry matter) oil yields, respectively. In general, the amount of EOs reduced as drying temperature rose. According to the GC/MS analysis of the EOs, the chemical components primarily belonged to the class of oxygenated monoterpenes (72.34%–86.41%). Microwave drying at 200 and 400 W power levels considerably (p < 0.01) reduced the chemical compounds group. The principal components of the M. piperita L. leaf EOs, including menthol, menthone, menthofuran, 1,8-cineole, and menthyl acetate, varied significantly (p < 0.05 and/or p < 0.01) depending on the drying techniques evaluated. The primary component of the oil, menthol, was found to have minimum (35.01%) and maximum (47.50%) concentrations in hot air-dried leaves at 50 °C and microwave-dried leaves at 400 W, respectively. Under microwave drying, the percentage of menthone, the EO’s second ingredient, considerably decreased (p < 0.01) (Beigi et al., 2018). The quantity and chemical makeup of the EOs from Laurus nobilis L. leaves were examined after being subject to six different drying techniques. Fresh and dried samples were hydrodistilled in Clevenger equipment to separate the EOs, which were then examined using GC-MS. The EO content was greatly increased by infrared drying at 45 °C and air drying at room temperature. It was discovered that there were 47 components, the majority of which were oxygenated monoterpenes. Except for air drying at room temperature, the drying technique has no discernible impact on this class of chemicals. With different drying techniques, the primary constituents—1,8-cineole, methyl eugenol, terpinen-4-ol, linalool, and eugenol—showed notable changes. When these compounds were dried by air at room temperature, their concentrations dramatically rose. These outcomes made it possible to conclude that this drying technique generated the greatest outcomes in terms of EOs and bioactive component levels (Sellami et al., 2011).
3.2.2.2 Plant storage
EO content drops with longer storage times (Sedlakova et al., 2001). Storage losses of EOs from dried C. sativum fruit kept in piles or cotton bags over a 2-year period were just 3–5% (Olle et al., 2010).
3.2.3 Extraction, distillation time, and conditions of analysis
Extraction, distillation time, and conditions of analysis have an impact on the type and quantity of EOs.
3.2.3.1 Extraction methods
Different techniques were used for kumquat peel extraction, including hydrodistillation, solvent, and supercritical CO2 (SC-CO2) extractions at densities of 281 g/dm3 (80 bar, 40 °C) and 875 g/dm3 (250 bar, 40 °C). The hydrodistillate of Citrus japonica was a colorless oil that smelled like fruit. The pentane extract was an intensely scented scarlet oil. Red oils from the supercritical extracts precipitated a white substance. Eight monoterpene hydrocarbons, six terpene alcohols, one terpene aldehyde, one terpene ketone, four terpene esters, and four sesquiterpene hydrocarbons were all quantified—24 substances in total. The quantity of the EO’s main constituent, was limonene. In comparison to EOs derived through steam distillation and solvent extraction, those obtained through SC-CO2 extraction had higher levels of linalyl acetate, neryl acetate, and geranyl acetate (Sicari and Poiana, 2017). Cold pressing (CP) and SC-CO2 extraction techniques were used to obtain the peel extract from Turkish-grown clementines (Citrus clementina Hort. Ex. Tan.). At total of 69 components were found, accounting for 99.8% of the total volatiles in both samples. Compared to SC-CO2 extraction, CP extraction produced less oxygenated compounds (3.7%); most of them were carbonyls (2.09%–2.10%), followed by alcohols (1.32%–1.60%) and esters (0.12%–0.40%). Myrcene (4.64%–3.77%) was second to limonene (88.12%–89.28%) as the primary constituent. Linalool (1.02%–1.24%) and decanal (0.71%–0.72%) were the oxygenated substances that were more abundant (İsmail Kirbaşlar et al., 2012).
3.2.3.2 Moisture and acidity of distillation water
Moisture has been suggested as a potential cause of EO degradation (Guenther and Appendix, 1948). For instance, it has been demonstrated that compound spectra are distorted during water distillation processes at approximately 100 °C. It is known that citral can react with acids to form methylacetophenone, p-cymene-8-ol, p-cymene, α-p-dimethylstyrene, and cresols in aqueous solutions (Schieberle et al., 1988). In contrast, Rao et al. (2011) found that even at a water source concentration of 20% (v/v), various oils held in the presence of water did not significantly alter. This concentration is greater than what, under ideal conditions, might be released in EOs during hydrodistillation. Kaul PN. et al. (1997) suggested drying EOs after distillation by adding water-binding materials because their study revealed some notable alterations, such as reduced contents of linalool and geraniol as well as an increase in citronellol and geranyl formate in P. graveolens oil that had been kept at room temperature for 1 year and which contained an undefined amount of water. However, the observed chemical alterations could have also been brought on by the container having 50% air space.
The monoterpene hydrocarbon sabinene is a key component of Juniperus sabina L. oil. When oil samples from different distillation durations were analysed, it seemed that sabinene levels continuously fell while the proportion of an oxygenated molecule, terpinen-4-ol, gradually rose. In the first decade of this century, it was already documented how diluted mineral acids may cause sabinene to convert into terpinen-4-ol (Wallach, 1907). Cooper et al. (1973) and Norin and Smedman (1971) validated the rearrangement of sabinene and reported that, in addition to terpinen-4-ol, other reaction products included α-terpinene, ɣ-terpinene, and terpinolene. Given that the levels of these chemicals increased concurrently with the reduction in sabinene, it was presumed that some conversion had taken place because of the acidity of the distillation water. Therefore, it appeared worthwhile to investigate how the pH of the distillation water affected the separation of the oil. For this, plant material soaked in buffer solutions with a pH range of 2.2–8 was used in distillations. Over the tested pH range, the amount of sabinene in the oil varied significantly. The percentage of sabinene in the distillate was roughly 7.5% at low pH levels. However, when the solution was made alkaline, this percentage rapidly rose to approximately 40% at pH 8 (Wallach, 1908).
3.2.3.3 Distillation time
Zheljazkov et al. (2013a) postulated that the distillation time (DT) can be adjusted for the best P. anisum EOs output and composition. They examined how nine steam DTs—5, 15, 30, 60, 120, 180, 240, 360, and 480 minutes—affected the yield and content of P. anisum seed EOs. To forecast the production of EOs, the concentration of certain constituents, and their yield as a function of DT, researchers created regression models. At 360-min DT, the highest EO output (2.0 g/100 g seed, 2%) was attained. The primary P. anisum oil element trans-anethole’s concentration ranged from 93.5% to 96.2% (as a percentage of the overall oil) and was typically highest at 15- to 60-min DT and lowest at 240- to 480-min DT. However, the yield of trans-anethole rose with increasing DT to reach its maximum at 360-min DT (derived from the EO’s output and the concentration of trans-anethole in the oil). Other oil ingredient concentrations also varied significantly with respect to the DT, with some of them being higher at the shorter DT than at the longer. However, lengthier DT (either 360 or 480 min) produced the maximum yields of these components. P. anisum EOs with variable composition can be produced using DT, which is advantageous for the EO sector (Zheljazkov et al., 2013a). The EO yield and oil compositions of M. piperita L., Cymbopogon citratus, and Cymbopogon martinii oils were modeled through experiments as a function of the steam DT. At a DT of 20 min, the highest EO yields of M. piperita L., C. citratus, and C. martinii were attained; subsequent increases in DT had no further effect on oil yields. In tests with C. citratus and C. martinii, DTs of 240 min resulted in 25–40% lower oil yields than yields at 20–160 min. This study showed that DT can be utilized as a method for extracting EOs from M. piperita L., C. citratus, and C. martinii that have a specific desired composition. Second, Zheljazkov et al. (2013a) discovered that the ideal duration of the DT for maximising the yields of M. piperita L., C. citratus, and C. martinii EOs was significantly shorter than that often employed by researchers and processors. Reduced DT could help producers and processors save money on energy and other resources (Cannon et al., 2013). When Lavandula angustifolia EOs were extracted from dried flowers, the impact of DT on the production and content of the oil was studied. In this experiment, the following DTs were tested: 1.5, 3.75, 3.75, 7.5, 15.5, 30.1, 60.2, 90.2, 120.2, 150.2, 180.2, and 240 min. At 60 min DT, EO output (0.5%–6.8%) peaked. The levels of fenchol (range 1.7–2.9%) and cineole (range 6.4–35%) were highest at the 1.5 min DT and declined with longer DTs. At 7.5–15 min DT the concentration of camphor (range 6.6–9.2%) peaked, while at 30 min DT the concentration of linalool acetate (range 1%–38%) peaked. The findings imply that the output of L. angustifolia EOs might not increase after 60 min DT. The asymptotic nonlinear regression model did a very good job of simulating the change in EO yield as well as the concentrations of cineole, fenchol, and linalool acetate as DT changes. DT can be used to alter the chemical composition of L. angustifolia oil and produce oils from the same L. angustifolia flowers with different chemical compositions. When citing or comparing reports on the production and composition of L. angustifolia EOs, DT must be considered (Zheljazkov et al., 2013b).
3.2.3.4 Conditions of GC analysis
In simulated experimental settings like pyrolysis-GC or thermal heating in the presence of oxygen or an inert environment, the thermolability of several EOs and their constituent aroma compounds, primarily monoterpenoids, was studied by Jakab et al. (2018). Citrus aurantiifolia oil underwent pyrolysis, increasing the amount of total monocyclic monoterpenes (limonene, ɣ-terpinene, p-cymene, β-phellandrene, and p-cymenene) from 55.74% to 66.21%. However, total bridge bicyclic monoterpenes, such as α- and β-pinene, sabinene, and α-thujene, showed a drop in abundance (22.98–12.41%). The pyrolysate of Bergamot orange oil showed the same trend (monocyclic 44.26–48.57% and bicyclic 9.48–6.50%). Additionally, linalyl acetate-dominated total linear alkyl esters (30.60–15.31%) were found to significantly decrease in level. This was due to acetic acid being eliminated, causing their breakdown (about 60%) into linear monoterpene hydrocarbons (1.13%–18.60%) such as β-myrcene and β-ocimene. Elettaria cardamomum oil’s main component α-terpinyl acetate followed a comparable process to breakdown by 48% into monocyclic monoterpenes, primarily limonene.
Additionally, some monoterpenes’ thermos-sensitivity has been examined in their pure form (McGraw et al., 1999). For instance, when heated separately at 90–130 °C with air present, α- and β-pinene demonstrated degradation rates of 23–37% and 22%, respectively. Verbenone, myrtenol, pinocamphone, α-pinene oxide, β-pinene, α-pinene oxide, and α-campholene aldehyde were the degradation products obtained from α-pinene, while myrtenol was the primary product of β-pinene. In a closed system that simulated the conditions of a wood dryer, the thermo-oxidative degradation of limonene, α-terpinene, camphene, and 3-carene was investigated at 120 °C in the presence of oxygen (1.00:1.24). The observed degradation was 50% (5, 24 h), 100% (8, 4 h), 38% (9, 72 h), and 36% (10, 72 h), respectively. Dehydrogenation, elimination, hydrolysis, C–C double bond cleavage, epoxidation, allylic oxidation, and thermal rearrangement all produced a variety of oxidised compounds; the former degradation pathways have been discussed in detail (Stolle et al., 2009; Vandewiele et al., 2011; Mahanta et al., 2021; Turek and Stintzing, 2013) (Table 1).
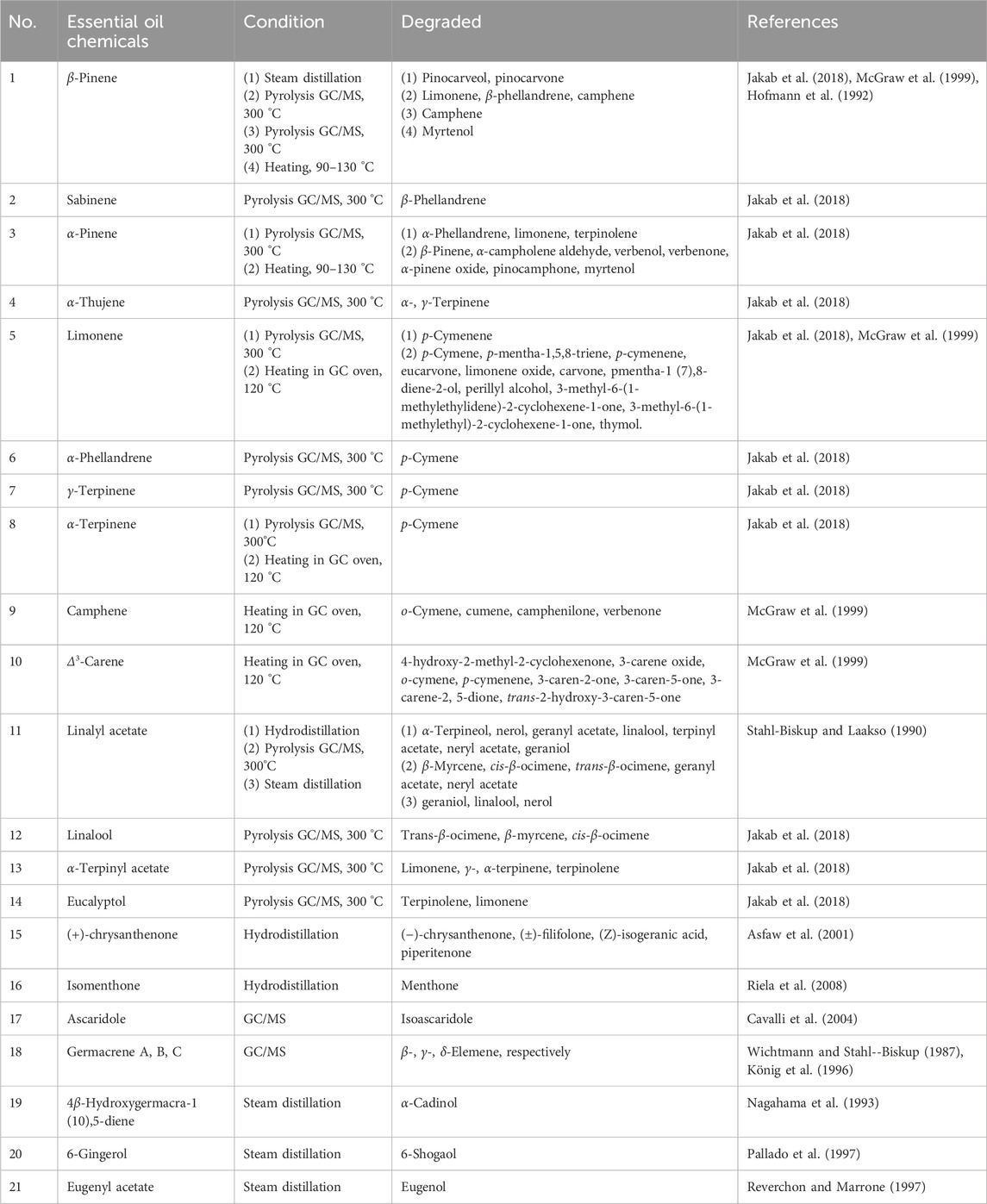
Table 1. Reported thermolabile essential oil components, degradation promoting conditions, and degraded products.
During GC-based EO investigations, sesquiterpenoids with a 1,5-diene system tend to rearrange themselves. GC connected to a flame ionisation or mass detector (FID or MS) is regularly used to investigate EOs due to its high sensitivity (nano to femtogram scale) and ability to resolve a complex mixture of EOs. However, the analytes are subjected to high temperatures (typically up to 250–300 °C) in the column and injector during the run, which may result in the degradation of thermosensitive phytochemicals. Numerous investigations have been conducted to demonstrate the heat conversion of the elemene derivatives of their germacrene counterparts. A high level of elemenes was detected using GC-MS while studying EOs from C. carvi roots and plants (Wichtmann and Stahl--Biskup, 1987). Germacrene A and B were completely rearranged to create the corresponding α- and β-elemene. The prediction was confirmed by heating oil fractions rich in germacrene A and B at 180 °C and 200 °C for 1 and 2 h, respectively. Many EOs, including black C. carvi, Smyrnium olusatrum, Hyptis alata, Achillea millefolium, black C. cyminum, Stachys sp., Ocotea sp., and Eugenia sp., were discovered to coexist with equivalent elemene derivatives during GC-MS analysis (Faraldos et al., 2007). GC-MS revealed that the major component of a chemotype of the German liverwort Preissia quadrata known as “germacrene C” undergoes full rearrangement to racemic β-elemene (König et al., 1996). At higher intake temperatures (280 °C), the thermal rearrangement was reported to be significant. During preparative GC at 390 °C injector temperature, EOs of the liverwort Scapania undulata converted helminthogermacrene to cis-elemene (Adio et al., 2004). Saccogyna viticulosa EOs from liverwort also included isogermacrene A rearrangement to iso β-elemene (Hackl et al., 2004). At a GC injector temperature of 300 °C, bicycloelemene was discovered to be a completely rearranged product of bicyclogermacrene in the EOs of Ribes nigrum buds (Le Quere and Latrasse, 1990). Bicycloelemene and bicyclogermacrene were found together in the EOs of Chloranthus spicatus and S. viticulosa (Hackl et al., 2004). GC-MS investigation of Dicranolejeunea yoshinagana revealed the production of bicycloelemene from (−)-ent-bicyclogermacrene (pure) (Toyota et al., 1996). The chemical profile of oil from the roots of Pimpinella saxifraga subsp. Alpestris was discovered to be dominated by C-12 hydrocarbons, with geijerene predicted to be the thermally rearranged product of pregeijerene (Tabanca et al., 2006). Furanosesquiterpenoids such isofuranodienone, furanodiene, and furanodiene were also found to undergo a similar rearrangement. The EOs of Eugenia uniflora underwent rearrangement from furanodiene (1.2%) to curzerene (85.1%) as a result of conventional GC-MS analysis (Chang et al., 2011). Similarly, furanodienone and isofuranodienone undergo similar rearrangement to become curzerenone and epicurzerenone, respectively (Baldovini et al., 2001). It has also been reported that the oxygenated sesquiterpene linderalactone, which was isolated from the roots of Lindera strychnifolia, underwent radical rearrangement at 160 °C to produce isolinderalactone (Setzer, 2008). Two oxygenated sesquiterpenoids, hedycaryol and germacra-1-(10,4-diene-6-ol), were found to have thermolability when Thymus praecox ssp. Arcticus EOs were analyzed (Stahl-Biskup and Laakso, 1990). At a high GC working temperature, both compounds underwent structural rearrangement to yield the corresponding rearranged products elemol and shyobunol, respectively. By heating two different fractions rich in 31 and 32 at 180 °C for 1 hour and 200 °C for 2 hours, respectively, the researchers were able to confirm their prediction. Elemol was also discovered in the oil of Cryptomeria japonica as the rearranged form of hedycaryol (Nagahama et al., 1993). Additionally, 4-hydroxygermane-1,5-diene would isomerize to ɣ-cadinol (Nagahama et al., 1993). Three separate species of Curcuma (C. wenyujin, C. phaeocaulis, and C. kwangsiensis) were the subject of a study that looked into the EO composition (Yang et al., 2007). When injected singly in their pure form during a GC-MS run, six of the eleven sesquiterpenoids (furanodiene, furanodienone, germacrone, curdione, neocurdione, and curcumol) were found to be thermolabile and visibly transformed into their respective rearrangement product(s). Notably, the temperature of the injector and the kind of injection (split vs. splitless) both affected the creation of artefacts. No studied conditions, however, could stop the GC-MS rearrangement of furanodiene and furanodienone. EOs from Acorus calamus and Melia dubia Cav underwent GC-MS analysis, and shyobunone was discovered to be an artefact created by the rearrangement of bacchascandon (acoragermacrone) (Nagalakshmi et al., 2001). The expected thermal transformation of neolinderalactone to isolinderalactone in L. strychnifolia Vill root oil was confirmed by heating 37 at 300 °C for 1–2 min (Setzer, 2008). It has also been observed that during the distillation of Saussurea lappa EOs, costunolide and dihydrocostunolide thermally changed into dehydrosaussurea lactone and saussurea lactone, respectively (Setzer, 2008), (Table 1).
3.3 Oil degradation during storage
External conditions such as temperature, light, and availability of air oxygen must be carefully considered. Additionally, stability may be governed by the composition of EOs, complex structures, and the presence of contaminants.
3.3.1 Light
It is believed that ultraviolet (UV) and visible (Vis) light speed up the autoxidation process by causing hydrogen abstraction, which generates alkyl radicals (Choe and Min, 2006). Accordingly, when laurel and F. vulgare oils were stored in the dark (Misharina and Polshkov, 2005), the same changes that occurred when they were stored in the light also occurred. Eugenyl acetate, trans-anethole, estragol, and other monoterpenes were decreased, whereas eugenol, p-cymene, and anisaldehyde were increased. Similar results were shown in Citrus sp., oil, where p-cymene increased while geranial, terpinolene, and ɣ-terpinene decreased (Al-Harrasi et al., 2022). It has been demonstrated that monoterpenes in particular breakdown quickly when exposed to light (Misharina et al., 2003).
Turek and Stintzing (2012) recently demonstrated that modifications in various EOs were facilitated by light but that reactions varied among oils from different plant species. While Salvia rosmarinus oil was found be particularly vulnerable to artificial sunlight that easily led to altered chemical composition, EOs from Thymus vulgaris did not change substantially. p-Cymene, camphor, and caryophyllene oxide all increased significantly along with the breakdown of β-caryophyllene and the monoterpenes α-terpinene, β-myrcene, as well as α-phellandrene. Another unnamed ingredient in L. angustifolia oil built up somewhat after the first month of storage under light but degraded again following advanced storage (Turek and Stintzing, 2012), while one tiny chemical in L. angustifolia oil that has not yet been further identified completely broke down when lit.
UV radiation or sunlight with a particular trans-anethole spectral signature causes such photo-artifacts. Cis-anethole anisaldehyde isomerization and oxidation products of trans-anethole reported in F. vulgare oil distribution were found in preserved EOs from P. anisum (photoanethole) and Citrus sp. (photocitral) (Miething et al., 1990). For the former, it was proposed that the light-induced photoanethole, also known as 4,4′-dimethoxystilbene, is caused by photocycloaddition between anethole and anisaldehyde (Miething et al., 1990). Furthermore, trans-anethole in sweet F. vulgare oil completely oxidised to anisaldehyde or isomerized to cis-anethole after 2 months of storage at ambient temperature with light (Misharina and Polshkov, 2005). Trans-anethole, the main component of both sweet and bitter F. vulgare oil, has been reported to transform into cis-anethole—10–12 times more dangerous than the former—when exposed to UV radiation or high temperatures (Braun and Franz, 1999).
3.3.2 Temperature
Ambient temperature has a significant impact on the stability of EOs (Atkins and De Paula, 2013). Lower temperatures, on the other hand, encourage oxygen (O2) solubility in liquids, which may significantly impact the stability of EOs (Bernhard and Marr, 1960). Studies have highlighted the unique reactions of EOs to various storage temperatures (Turek and Stintzing, 2012). When refrigerated for a 3-month test period, S. rosmarinus oil showed considerable stability at lower temperatures. On the other hand, degradation in pine EOs accelerated at 23 °C and even occurred at 5 °C. The stability of volatile plant extracts and EOs often deteriorates with extended storage time as well as temperature increases from 0 to 28 °C (Gopalakrishnan, 1994), 4 to 25 °C (Braun and Franz, 1999), and 23 to 38 °C (Turek and Stintzing, 2013). A rise in p-cymene (Gopalakrishnan, 1994) and a decrease in terpenic hydrocarbons such β-myrcene, β-caryophyllene, sabinene, β-pinene, or γ-terpinene were the most notable changes in EOs from Pinus sp., Syzygium aromaticum bud, E. cardamomum, L. angustifolia, and S. rosmarinus as the temperature increased. Additionally, Braun and Franz (1999) noted that the quick development of 4-anisaldehyde, which has been shown to be a good quality marker, was the most notable change that occurred after storage of F. vulgare oil at 25 °C compared to 4 °C. Additionally, it was noted that an increase in temperature caused the number of many unexplained polymerization products made from myrcene to increase (Dieckmann and Palamand, 1974). It is well known that terpenoids, particularly simple terpenes and aldehydes, are thermolabile and subject to chemical reactions at high temperatures. Both isolated compounds (McGraw et al., 1999) and EOs (Tomaino et al., 2005) have been observed to undergo terpenic conversion reactions after heating. However, terpenoids do not always undergo the same modifications at temperatures above 100 °C as they do under actual storage circumstances. Double bond breaking, epoxidation, dehydrogenation into aromatic systems, and allylic oxidation into alcohols, ketones, and aldehydes are the four main groups into which McGraw et al. (1999) subdivided the oxidative reactions that follow the thermal breakdown of terpenes.
3.3.3 Oxygen availability, compound structure, and chemical composition
It is clear that oxygen access is crucial to the stability of EOs because oxidation reactions are one of the primary causes of EO deterioration (Widmark et al., 1957). Numerous monoterpenes have been demonstrated to consume oxygen while being stored (El-Nikeety et al., 2000). The amount of dissolved oxygen grows with the rate of oil oxidation and is mostly influenced by two factors: the surrounding temperature and the partial pressure of oxygen in the headspace. Without stirring, the sample’s oxygen diffusion occurs gradually over time (Choe and Min, 2006). Henry’s law states that oxygen solubility is highest at low temperatures and dramatically drops as the temperature rises. Since edible oils are oxidised at lower temperatures, peroxyl radicals and hydroperoxides have been reported to be the most prevalent molecules. On the other hand, alkyl or hydroxyl radicals and their reactions became more significant since oxygen was limited at high temperatures (Velasco and Dobarganes, 2002). According to a recent study, EOs are unique in that they are susceptible to oxidation. While peroxide formed in EOs from S. rosmarinus and Pinus sp., when kept at room temperature, it appeared to be significantly more affected by oxygen solubility than the level of peroxide present in L. angustifolia oil and T. vulgaris oil when held at 5 °C. The outcomes for cold-pressed lemon oil held in nearly full containers are equivalent to those for samples maintained under N as a protective gas. After 50 °C storage, it was found that limonene oxide, p-cymene, ɣ-terpineol, and geranic acid increased and limonene, α-terpinene, β-caryophyllene, β-phellandrene, and citral decreased (Nguyen et al., 2009). Therefore, it appeared that the dissolved ambient oxygen in the oils was adequate, at least for small oxidation activities. Additionally, as previously mentioned, EOs can still experience additional degrading processes, such as unreported rearrangements, if oxygen is insufficient (Geier, 2006). These findings suggest that avoiding container headspace may not always prevent oxidation, depending on the EOs and the surrounding temperature. As demonstrated by Geier (2006), EOs should be treated with an inert gas, such as argon, and then thoroughly flushed to eliminate any lingering air. This will successfully prevent the production of peroxides.
The degree of oxidation is significantly influenced by compound structure, which has an impact on the susceptibility of EOs to degradation (Treibs et al., 1960). Since resonance-stabilized radicals with lower activation energies are produced when hydrogen atoms are abstracted, compounds with a large concentration of allylic hydrogen atoms are the most likely candidates for autoxidation (Bäcktorp et al., 2006). Because of their structural predisposition to the formation of multiple radicals stabilised by conjugated double bonds or isomerization to tertiary radicals, polyunsaturated terpenic hydrocarbons are particularly vulnerable to oxidative degradation (Guenther and Appendix, 1948). In line with this, EOs that are mostly composed of unsaturated mono- and sesquiterpenes, such as those derived from Pinus sp., and Cyprus turpentine, are easily changed when stored (Turek and Stintzing, 2011b). Additionally, through a hyper-conjugative effect, electron-donating groups and rising alkyl substitution support a stronger carbon peroxide bond, resulting in more stable and subsequently built-up hydroperoxides (Pratt and Porter, 2003), whereas others are insufficiently stabilised and quickly break down. An illustration of the latter (Sköld et al., 2006) are caryophyllene hydroperoxides that rapidly produce the equivalent epoxide state. Additionally, modifications to each constituent may potentially affect the stability of other molecules (Gopalakrishnan, 1994): easily oxidized chemicals can start the autoxidation of other molecules that are less likely to decompose by transferring reactive oxygen. On the other hand, antioxidant elements may also stop other substances from deteriorating. Autoxidative chain reactions are known to be disrupted, or rather delayed, by phenols such eugenol, thymol, and carvacrol (Treibs et al., 1960). Their ability to quickly remove the phenolic hydrogen atom allows them to produce free, relatively long-lasting radicals that are stabilized by isomerization to an alkyl substituted tertiary position or electron-releasing groups. The stability of the product containing these so-called main antioxidants, which scavenge free reactive alkyl, alkoxy, or peroxyl radicals, is increased (Gopalakrishnan, 1994). In fact, EOs from Thymus sp., which can contain up to 80% phenols, have shown generally high storage stability (Turek and Stintzing, 2011b). Although Hagvall et al. (2008) concluded that the Lavandula sp., oil matrix had no protecting effects on the primary components linalyl acetate, linalool, and β-caryophyllene when comparing autoxidation rates in the oil with those from isolated substances, it is possible that individual EO matrices may affect the stability of compounds therein (Guenther and Appendix, 1948). To obtain detectable hydroperoxides, which were the subject of Hagvall et al. (2008), oxidation was done under many difficult circumstances. Changes in EOs or isolated chemicals may be the result of several interrelated variables acting together rather than just one impact. This may be partially caused by the absence of systematic methods for excluding the factors that control the stability of EOs. As a result, conflicting claims about the significance of specific intrinsic and extrinsic characteristics as a cause of deterioration are frequently made.
Additionally, Wagner–Meerwein rearrangements, a class of carbocation 1,2-rearrangement reactions in which a hydrogen, alkyl, or aryl group migrates from one carbon to a neighbouring carbon, are cationic [1,2]-sigmatropic rearrangements, proceeding suprafacially and with stereochemical retention (Zhuolian et al., 1988; Hanson, 1991). The reaction was initially examined in terms of the rearrangement of α-pinene to bornyl chloride and of camphene via camphene hydrochloride to isobronyl chloride.
3.3.4 Metal contaminants
EOs may become contaminated with metal during the distillation process in ancient stills or when it is stored in metallic containers (Treibs et al., 1960). Heavy metals, particularly copper and ferrous ions, are thought to increase autoxidation on a par with light and heat (Choe and Min, 2006). Cu+ or Fe2+, Fe3+, and Cu2+ catalyze the breakdown of hydroperoxide to produce peroxyl and alkoxy radicals, which in turn facilitate radical oxidation processes. Additionally, it has also been noted that ferrous ions can produce singlet oxygen, which starts the photooxidation process (Choe and Min, 2006).
3.4 Factors affecting the bioactivity of essential oils
The following variables and factors may be considered in studies of the pharmacological effects of EOs.
3.4.1 Effect of the treated plant part
Numerous experiments have been conducted to show a difference in activity between two different parts of the same plant. For molluscicidal testing, oil extracted from the leaf of Citrus aurantium var. Amara Link was used to act against Bulinus truncatus snails. Although oil from the fruit of the same plant had considerable molluscicidal activity at lower concentrations, the LC50 was 1.46 (1.36–1.56) ppm. In truth, little is known about how biological activity is distributed among different plant parts, which is largely because active principles, which are found in some plant parts more frequently than others, are distributed differently (Lahlou and Berrada, 2001).
3.4.2 Effect of the solvent/detergent
Laboratory solvents are harmful to living things and thus interfere with activity being researched (Lahlou, 2001). The selection of an appropriate solvent (or detergent) is crucial before considering a bioassay involving EOs. To demonstrate the impact of the detergent used for an EO’s dispersion on the results of biological testing, a comparison study of the same “tensioactive” Tween 80 when employed differently in two different pieces of research on B. truncatus snails was conducted by Lahlou and Berrada (2001) and Lahlou et al. (2001b). In this case, two very different sets of results were discovered. To assist in the EOs’ dispersion in the aqueous solution, 1.0% of Tween 80 was added (Lahlou et al., 2001b). The results revealed that at much higher levels (0.20 × 103 ppm ≤ LC50 ≤ 2.85 × 103 ppm for oils and 0.12 × 103 ppm ≤ LC50 ≤ 2.68 × 103 ppm for the main components), these compounds demonstrated a fascinating molluscicidal effect. Milky transparent solutions were created when Tween 80 was applied at a concentration of just 0.2%, and the solutions were then dispersed in water using a 2 kC/s ultrasound frequency for 5 s. In comparison to the latter, the results from this second approach (Lahlou and Berrada, 2001) are sufficient, and at low amounts, the studied oils and their components had an effect (0.28 ppm ≤ LC50 ≤ 2.84 ppm for oils and 0.13 ppm ≤ LC50 ≤ 2.93 ppm for the main components).
3.4.3 The correlation composition-activity
The biological effects of an EO are directly tied to its chemical composition. Therefore, while screening plants utilized in regional folk medicine for biological properties, we noticed a variance in the activity of tested oils and their primary components on numerous biological models (Lahlou and Berrada, 2001; Lahlou, 2001; Lahlou et al., 2001b). EOs generally have a less significant impact than active compounds. To find the physiologically active components, researchers examined the main components of oils that have proved harmful to these biological models. The biological activity of the studied essences from aromatic plants may be attributed to both their major components—such as alcoholic, phenolic, terpenic, or ketonic compounds—as well as to the minor components that are also present in these oils, according to the relationship between composition and activity. It is possible that they will cooperate to make the tested oil more harmful.
3.4.4 Technique used
In our in vitro investigation, the licicidal and niticidal effects of different Moroccan EOs and their main ingredients or plant oil chemotypes on Pediculus humanus capitis (head lice) were investigated using two methods: microatmosphere and direct application (Lahlou and Berrada, 2003). Utilizing the microatmosphere technique, it was shown that some oils, including T. broussonettii, Mentha pulegium, and Chenopodium ambrosioides, were especially effective against lice. Others, primarily Lavandula stoechas, Cedrus atlantica, and Chrysanthemum viscidehirtum, were discovered to be significantly less active utilizing this technique. While EOs from M. pulegium, C. ambrosioides, O. compactum, Thymus broussonettii, and Ruta chalepensis were discovered to be the most potent of those tested (LD100 = 3.10 µL), other oils from Pinus pinea (Pinaceae), Cin A Melaleuca viridiflora, C. sinensis, C. limon, and Myrtus communis EOs were more effective on nits when applied directly (0% of nits hatched), compared to 30% of nits that were exposed to this oil in a micro-atmosphere of 3.10 µL.
3.4.5 Biological material
According to research on the licicidal and niticidal properties of some Moroccan essential oils, T. broussonettii, Mentha pulegium, C. ambrosioides, and R. chalepensis have the most potent effects on head lice, P. humanus capitis. Other oils, such as Origanum compactum, were discovered to be only active on nits up to volumes of 3.10 µL. However, it was found that lice were more vulnerable and died after 15 min of exposure to an application of 0.5 µL of all studied oils when directly applied (Lahlou et al., 2001a).
3.4.6 Choice of dose/concentration
A poison and a cure can be distinguished by their dosages, and this choice must not be dangerous. The study of bioactivity depends on this property. These concentrations should be in accordance with the limitations already mentioned in the literature (other studies with equal activity using the same chemicals or plant types). When an EO or one of its primary components was found to be toxic at concentration, other appropriate lower concentrations (or dilutions of active ones) were prepared and used in all tests on biological organisms to determine the minimal toxic concentration that caused 50% or 90% toxicity, or lethal doses/concentrations LD50-LD90/LC50-LC90. For instance Lahlou et al. (2001a) examined various volumes of the oils (3.10–50 µL) in niticidal and licicidal assays. EOs are rarely used undiluted. The dilution of the low potent volume (3.10 µL) with active niticidal oils obtained from T. broussonettii, M. pulegium, O. compactum, C. ambrosioides, and R. chalepensis was tested at dilution factors of 1:2, 1:4, and 1:10 in alcohol (Lahlou et al., 2001a). With the exception of a few terpenic ketones that are more or less toxic, such as thujone or pulegone, the fragrance concentration in the majority of cosmetic products ranged between 0.5% and 2%, making it impracticable to worry about EO constituents posing a risk of poisoning (Buchbauer, 2000).
3.4.7 Reference compounds
A comparison with a reference standard compound acceptable at the time, carried out in the same laboratory environment, is necessary and unavoidable in this regard for all biological and pharmacological tests using natural products to have adequate controls.
3.4.8 Comparison with literature data
This phase is crucial to conducting a scientific laboratory study with a strict protocol to compare all findings with those published in the literature for other chemicals tested in the same lab settings. It also shows whether the obtained results are intriguing or fall within the recognised ranges (or limits) given in the literature. The activity of oils from the same family of aromatic plants is very comparable. The circumstances of experimentation do present some challenges; therefore, the outcomes can occasionally be viewed as relative. Under the same laboratory circumstances everywhere around the world, using the same plant species oil should produce similar biological or pharmacological activity. Additionally, it is vital to interrogate the literature to determine whether the qualities of the tested plant oils have already been investigated (Lahlou, 2004).
4 Discussion
EOs are made up of lipophilic, highly volatile secondary plant metabolites that have a molecular weight below 300. They are reported to have antiviral, antimicrobial, nematicidal, insecticidal, antifungal, and antioxidant properties (Protzen, 1993), and even activities stimulating the nervous system (Attokaran, 2011). In addition to their widespread use as flavouring components, EOs constitute a “green” alternative in the nutritional, medicinal, and agricultural industries (Turek and Stintzing, 2013).
EOs have wide variability in their composition (Blitzke, 2009). However, the flavour contribution of a single chemical is not only influenced by its concentration; it also depends on the odor threshold, which is influenced by structure and volatility. Therefore, if an ingredient’s aroma value is high enough, even tiny components resulting from oxidation or degradation reactions may have a significant effect on the flavor (Grosch, 2007). Phenylpropanoids, lipophilic terpenoids, or short-chain aliphatic hydrocarbon derivatives (Kubeczka, 1979) of low molecular weight can be classified as the majority of EO constituents, with the first being the most prevalent and distinctive (Treibs et al., 1960). Most are allylic, mono-, bi-, or tricyclic mono- and sesquiterpenoids, which include hydrocarbons, alcohols, ketones, aldehydes, oxides, phenols, and esters. Diterpenes are rarely found in true EOs obtained by distillation due to their low volatility (Treibs et al., 1960).
The components of EOs are known to readily transform into one another by oxidation, isomerization, cyclization, or dehydrogenation reactions that are activated either enzymatically or chemically. This is due to their structural relationship within the same chemical group. When evaluating the stability of EOs, it is important to remember that the starting material’s chemical composition may already be different due to factors such as plant health, development stage, habitat including climate, edaphic conditions, and harvest time (Figueiredo et al., 2008). The structural pattern as a function of harvest time is illustrated by Masotti et al. (2003), who showed a decreasing concentration of a hydrocarbon monoterpene in EOs throughout the year and an increasing amount of an oxidized immediate metabolic successor. Consequently, natural variations in the composition of plant volatiles must be considered when evaluating their quality (Kubeczka, 1993). Terpenoids frequently exhibit both high volatility and thermolability; depending on their specific structural makeup, they may be quickly oxidised or hydrolyzed (Worsfold et al., 2019). It is widely acknowledged that conditions during plant material processing, storage, and distillation, as well as during the treatment of oil afterward, all affect the chemical makeup of EOs (Turek and Stintzing, 2013). When stripped of the compartmentation that protects them in the plant matrix (Treibs, 1956), the constituents of EOs are particularly vulnerable to oxidative harm, chemical changes, or polymerization processes. These aging processes typically result in a more or less noticeable quality decrease (Al-Harrasi et al., 2022). Degrading EOs and certain terpenoids have also been shown to generate a variety of hues, such as yellow staining in C. carvi oil (Preuss, 1964), or changes in consistency up to resinification (Turek and Stintzing, 2013), in addition to the frequent development of unpleasant and frequently pungent odours. For instance, the off-flavor in lemon oil (Sinki et al., 1997) and the fragrant monoterpene p-cymene, which is typically present in aged EOs (McGraw et al., 1999), have both been connected to the oxidative dehydrogenation of single or double unsaturated monocyclic terpenes or acid-catalyzed depletion of citral, respectively.
Some aged EOs and oxidized terpenoids show skin-sensitizing abilities in addition to organoleptic changes and viscosity changes (Turek and Stintzing, 2013), resulting in a hypersensitivity reaction similar to allergic contact dermatitis (Divkovic et al., 2005).
Oxidation is crucial in the breakdown of terpenoids. During this spontaneous, air-induced oxidative process, unsaturated molecules react with aerial oxygen in a free radical chain mechanism to form a variety of primary and secondary oxidation products. The reaction’s course will be influenced by the its chemical composition, oxygen content, energy input, and the impacts of additional reaction partners. Heat, catalytic concentrations of redox-reactive metals, and exposure to light are used to make alkyl radicals, which then quickly react with triplet oxygen to form peroxyl radicals. When oxidation proceeds, primary autoxidation products such hydroperoxides typically gradually degrade based on their unique stability. As a result, they generate a variety of long-lasting secondary oxidized products such as monovalent to polyvalent alcohols, epoxides, ketones, aldehydes, peroxides, or acids as well as extremely viscous, frequently oxygen-containing polymers (Turek and Stintzing, 2013).
Accordingly, it has been found that initially generated oxidation products from terpenoids, referred to as “hydroperoxides”, disintegrate in the presence of light, heat, or rising acidity (Blakeway et al., 1987). Even at ambient temperature, the peroxidic byproducts of the oxidation of the monoterpene terpinolene deteriorate quickly (Borglin et al., 1950). Epoxides, alcohols, and ketones may all be principally formed in the radical chain process that competes with hydroperoxide creation, in addition to their secondary nature (Neuenschwander et al., 2010). The structure of subsequent oxidation products is often still a mystery. Caryophyllene oxide, which results from air oxidation of the sesquiterpene caryophyllene (Sköld et al., 2006) and is usually found in stored EOs (Mockutë et al., 2005), is one well-known stable secondary product. Additionally, it was widely claimed that carveol and the oxidation product carvone were produced by peroxides during limonene autoxidation (Bernhard and Marr, 1960) and limonene oxide (Matura et al., 2005). Many oxygen-bearing terpenoids, such as menthol, 1,8-cineol, and menthone, directly convert into ketones, acids, and aldehydes during oxidation rather than hydroperoxides (Geier, 2006). Disproportionation and cyclization are assumed to be involved in the breakdown of monoterpenes, as evidenced by the development of cyclic isomers such limonene, terpinenes, and p-cymene in oxidative tests with the monoterpene myrcene at high temperatures (Dieckmann and Palamand, 1974).
The aging of EOs has been shown to cause changes that may threaten consumer safety as well as cause sensory and technological impairment. Few studies have examined EO stability over time while taking the influence of various storage circumstances into account, despite the fact that quality monitoring and a complete understanding of EO features in storage are crucial. When studying degradation reactions, single reference compounds or model systems are usually used, and oxidation is frequently accelerated by molecular oxygen, metal catalysts, and photosensitizers (Blumann and Ryder, 1949).
Additionally, it has been noted that degradation processes in mixes, such as EOs, behave differently from those in single compounds. This can be explained by the possibility that changes to one molecule may also have an impact on how that compound oxidizes—for instance by pro- or antioxidative effects (Gopalakrishnan, 1994). Gas chromatography has been a major component of studies on EOs for detecting changes in substance patterns during storage in the presence of air. Even less consideration has been given to storage temperature fluctuation in studies on the composition of EOs (Gopalakrishnan, 1994). Storage times vary from a few days to as long as a year. Significant declines in the levels of unsaturated terpenes such as β-myrcene or γ-terpinene, as well as an increase in p-cymene, were frequently observed under light and elevated temperatures, which was also confirmed in the long-term storage of tea tree oil (Brophy et al., 1989). Furthermore, the augmentation of oxidised chemicals has been documented over time, such as anisaldehyde in F. vulgare oil or caryophyllene oxide and linalool oxide in marjoram oil (Misharina and Polshkov, 2005; Misharina et al., 2003). By using comparative storage, Misharina and Polshkov (2005) were able to highlight the distinctive qualities of EOs. In comparison to EOs from laurel, sweet F. vulgare oil was extremely unstable even in the dark and had nearly totally degraded after 2 months under light, followed by the production of identified oxidation products as well as several unexplored compounds. In an in-depth study, El-Nikeety et al. (2000) evaluated additional physicochemical parameters in addition to GC data. The findings of storing EOs from P. crispum, P. anisum, C. carvi, and C. cyminum under various conditions were found to be inadequately rigorous. Some changes in chemical composition that have been reported may not be the result of oxidative degradation at all but rather the result of evaporation or erroneous analysis. Examples of deviations from the expected outcomes include the decline in caryophyllene oxide in preserved lemon balm oil (Shabby et al., 1995) and the rise in sesquiterpenic hydrocarbon levels (Kaul P. et al., 1997).
Only recently have studies shed light on the effects of certain extrinsic features on up to seven EOs generated from different plant species that systematically encompassed various storage settings. Researchers found that, based on the chemical composition of the EOs, each character responded to light, storage temperature, and oxygen availability differently and to varying degrees. By monitoring changes in chemical patterns as well as clearly specified physicochemical criteria for up to 18 months, each EO’ stability was thus thoroughly assessed (Turek and Stintzing, 2011b). Overall, trustworthy and complete research on EO storage is rare, and definite requirements on optimal storage conditions and EO shelf life have not yet been fully specified (Blitzke, 2009).
5 Conclusion
Economically, it is essential to grow plants that produce essential oils (EOs), such as those utilised in medicine, spices, and cosmetics. Growing conditions affect EO quality and may have a substantial impact on oil yield. Genetic diversity, plant ecotype, chemotype, cultivars or variety, dietary intake, fertilizer application, geographic location, local climate, seasonal variations, stress experienced during development or maturation, and post-harvest drying and storage all have an impact on the chemistry of EOs. Therefore, systematic cultivation strategies for EO-bearing plants based on modern scientific knowledge are necessary to maintain a regular supply of quality plant materials required for EO production.
Additionally, an EO’s yield and composition (constituents), which govern its unique biological properties, are determined by the type of plant material used and the extraction method. As a result, each species of plant has a profile that it will express differently based on these factors. Certain studies on EOs have been published with the intention of elucidating the level of specificity of these compounds’ effects among the many manifestations of their bioactivity. The potential for the thermal degradation of EOs has been studied while working with EO manufacturing facilities. It is essential to improve science in creating and using non-thermal solutions to not only stop degradation but also to achieve sustainability through safety, efficiency, low energy consumption, avoiding the use of organic solvents or firewood, and lowering CO2 emissions. Using freeze-drying, microwave extraction, supercritical fluid extraction, solar distillation, ultrasound-assisted extraction, high pressure processing, electrical heating, or radiation treatments, the sensory attributes of EO plant products have been created and shown in this review. However, the scalability and cost sustainability in comparison to conventional procedures remain a significant hurdle with these cutting-edge technologies.
Author contributions
URA: Investigation, writing – original draft, and writing – review and editing. AHE: writing – original draft, writing – review and editing.
Funding
The author(s) declare that no financial support was received for the research and/or publication of this article.
Conflict of interest
The authors declare that the research was conducted in the absence of any commercial or financial relationships that could be construed as a potential conflict of interest.
The authors declared that they were an editorial board member of Frontiers at the time of submission. This had no impact on the peer review process and the final decision.
Publisher’s note
All claims expressed in this article are solely those of the authors and do not necessarily represent those of their affiliated organizations, or those of the publisher, the editors and the reviewers. Any product that may be evaluated in this article, or claim that may be made by its manufacturer, is not guaranteed or endorsed by the publisher.
References
Abada, M. B., Hamdi, S. H., Masseoud, C., Jroud, H., Bousshih, E., and Jemâa, J. M. B. (2020). Variations in chemotypes patterns of Tunisian Rosmarinus officinalis essential oils and applications for controlling the date moth Ectomyelois ceratoniae (Pyralidae). South Afr. J. Bot. 128, 18–27. doi:10.1016/j.sajb.2019.10.010
Abdelmohsen, U. R., Sayed, A. M., and Elmaidomy, A. H. (2022). Natural products’ extraction and isolation-between conventional and modern techniques. Front. Nat. Prod. 1, 1. doi:10.3389/fntpr.2022.873808
Abd El-Wahab, M. (2006). The efficiency of using saline and fresh water irrigation as alternating methods of irrigation on the productivity of Foeniculum vulgare Mill subsp. vulgare var. vulgare under North Sinai conditions. Res. J. Agr. Biol. Sci. 2 (6), 571–577.
Abd El-Kareem, M. S., Rabbih, M. A., Rashad, A. M., and EL-Hefny, M. (2024). Essential oils from fennel plants as valuable chemical products: gas chromatography–mass spectrometry, FTIR, quantum mechanical investigation, and antifungal activity. Biomass. Conver. Biore. 1–19.
Abou-Zied, E. (1974). Increase in volatile oil and chemical composition in the seeds of caraway and fennel plants induced by succinic acid 2, 2-dimethylhydrazide. Biol. Plant. 16 (2), 123–126. doi:10.1007/bf02920787
Adio, A. M., Paul, C., Kloth, P., and König, W. A. (2004). Sesquiterpenes of the liverwort Scapania undulata. Phytochemistry 65 (2), 199–206. doi:10.1016/j.phytochem.2003.10.018
Al-Harrasi, A., Bhatia, S., Kaushik, D., Ahmed, M. M., Anwer, K., and Sharma, P. (2022). Quality Control of Essential Oils in: Role of essential oils in the management of COVID-19 (CRC Press), 169–177.
Ali, M., Mujib, A., Gulzar, B., and Zafar, N. (2019). Essential oil yield estimation by Gas chromatography–mass spectrometry (GC–MS) after Methyl jasmonate (MeJA) elicitation in in vitro cultivated tissues of Coriandrum sativum L. 3 Biotech. 9, 414–416. doi:10.1007/s13205-019-1936-9
Alizadeh, A., Khoshkhui, M., Javidnia, K., Firuzi, O., Tafazoli, E., and Khalighi, A. (2010). Effects of fertilizer on yield, essential oil composition, total phenolic content and antioxidant activity in Satureja hortensis L.(Lamiaceae) cultivated in Iran. J. Med. Plants Res. 4 (1), 33–40.
Arganosa, G., Sosulski, F., and Slikard, A. (1998). Seed yields and essential oil of northern-grown coriander (Coriandrum sativum L.). J. herbs, spices and Med. plants 6 (2), 23–32. doi:10.1300/j044v06n02_03
Asfaw, N., Storesund, H. J., Skattebøl, L., and Aasen, A. J. (2001). Coexistence of chrysanthenone, filifolone and (Z)-isogeranic acid in hydrodistillates. Artefacts!. Artefacts! Phytochem. 58 (3), 489–492. doi:10.1016/s0031-9422(01)00254-0
Ashraf, M., and Akhtar, N. (2004). Influence of salt stress on growth, ion accumulation and seed oil content in sweet fennel. Biol. Plant. 48, 461–464. doi:10.1023/b:biop.0000041105.89674.d1
Atta-Aly, M. A. (1999). Effect of nickel addition on the yield and quality of parsley leaves. Sci. Hortic. 82 (1-2), 9–24. doi:10.1016/s0304-4238(99)00032-1
Attokaran, M. (2011). Natural food flavors and colorants. Ames, IA: Blackwell Publishing Ltd and Institute of Food Technologists. chap 68.
Babushok, V., Linstrom, P., and Zenkevich, I. (2011). Retention indices for frequently reported compounds of plant essential oils. J. Phys. Chem. Reference Data 40 (4). doi:10.1063/1.3653552
Bäcktorp, C., Wass, J. T. J., Panas, I., Sköld, M., Börje, A., and Nyman, G. (2006). Theoretical investigation of linalool oxidation. J. Phys. Chem. A 110 (44), 12204–12212. doi:10.1021/jp0603278
Bahl, J., Singh, A., Lal, R., and Gupta, A. (2018). High-yielding improved varieties of medicinal and aromatic crops for enhanced income. New age herbals Resour. Qual. Pharmacogn., 247–265. doi:10.1007/978-981-10-8291-7_12
Baj, T., Kowalski, R., Swiatek, L., Modzelewska, M., and Wolski, T. (2010). Chemical composition and antioxidant activity of the essentials oil of hyssop (Hyssopus officinalis L. ssp. officinalis). Ann. Univ. Mariae Curie-Skłodowska. 23 (3), 55–62.
Baldovini, N., Tomi, F., and Casanova, J. (2001). Identification and quantitative determination of furanodiene, a heat-sensitive compound, in essential oil by 13C-NMR. Phytochemical Analysis Int. J. Plant Chem. Biochem. Tech. 12 (1), 58–63. doi:10.1002/1099-1565(200101/02)12:1<58::aid-pca559>3.0.co;2-9
Beigi, M., Torki-Harchegani, M., and Ghasemi Pirbalouti, A. (2018). Quantity and chemical composition of essential oil of peppermint (Mentha× piperita L.) leaves under different drying methods. Int. J. Food Prop. 21 (1), 267–276. doi:10.1080/10942912.2018.1453839
Bernhard, R. A., and Marr, A. G. (1960). The oxidation of terpenes. I. Mechanism and reaction products of D-limonene autoxidation. J. Food Sci. 25 (4), 517–530. doi:10.1111/j.1365-2621.1960.tb00363.x
Bizzo, H. R., Brilhante, N. S., Nolvachai, Y., and Marriott, P. J. (2023). Use and abuse of retention indices in gas chromatography. J. Chromatogr. A 1708, 464376. doi:10.1016/j.chroma.2023.464376
Blakeway, J., Frey, M., Lacroix, S., and Salerno, M. (1987). Chemical reactions in perfume ageing. Int. J. Cosmet. Sci. 9 (5), 203–214. doi:10.1111/j.1467-2494.1987.tb00475.x
Blanco, M. A., Colareda, G. A., van Baren, C., Bandoni, A. L., Ringuelet, J., and Consolini, A. E. (2013). Antispasmodic effects and composition of the essential oils from two South American chemotypes of Lippia alba. J. Ethnopharmacol. 149 (3), 803–809. doi:10.1016/j.jep.2013.08.007
Blitzke, T. (2009). Ätherische Öle und Aromaextrakte in der Kosmetik, der Aromatherapie und im Lebensmittelbereich. Handb. Des. Arznei-und Gewürzpflanzenbaus 1, 383–394.
Blumann, A., and Ryder, L. (1949). Autoxidation of α-phellandrene. J. Chem. Soc. (Resumed) 436, 2040–2043.
Borglin, J., Lister, D., Lorand, E., and Reese, J. (1950). Oxidation of terpenes with molecular oxygen. I. Oxidation of terpinolene in aqueous Dispersion1. J. Am. Chem. Soc. 72 (10), 4591–4596. doi:10.1021/ja01166a072
Bousbia, N., Vian, M. A., Ferhat, M. A., Meklati, B. Y., and Chemat, F. (2009). A new process for extraction of essential oil from Citrus peels: microwave hydrodiffusion and gravity. J. food Eng. 90 (3), 409–413. doi:10.1016/j.jfoodeng.2008.06.034
Bowes, K. M., and Zheljazkov, V. D. (2004). Essential oil yields and quality of fennel grown in Nova Scotia. HortScience 39 (7), 1640–1643. doi:10.21273/hortsci.39.7.1640
Braun, M., and Franz, G. (1999). Quality criteria of bitter fennel oil in the German pharmacopoeia. Pharm. Pharmacol. Lett. 9, 48–51.
Brophy, J. J., Davies, N. W., Southwell, I. A., Stiff, I. A., and Williams, L. R. (1989). Gas chromatographic quality control for oil of Melaleuca terpinen-4-ol type (Australian tea tree). J. Agric. Food Chem. 37 (5), 1330–1335. doi:10.1021/jf00089a027
Buchbauer, G. (2000). The detailed analysis of essential oils leads to the understanding of their properties. Chem. Weekly-Bombay 45 (49), 163–165.
Burt, S. (2004). Essential oils: their antibacterial properties and potential applications in foods—a review. Int. J. food Microbiol. 94 (3), 223–253. doi:10.1016/j.ijfoodmicro.2004.03.022
Calderón-Oropeza, M. A., Ramírez-Briones, E., Rodríguez-García, G., Salvador-Hernández, J. L., Bedolla-García, B. Y., Zamudio, S., et al. (2021). Metabolic correlations of Salvia dugesii Fernald and Salvia gesneriiflora Lindl. and Paxton with native Salvia plants from four continents using essential oils compositions. Rec. Nat. Prod. 15 (4), 312–323. doi:10.25135/rnp.223-19.121912
Cannon, J. B., Cantrell, C. L., Astatkie, T., and Zheljazkov, V. D. (2013). Modification of yield and composition of essential oils by distillation time. Industrial Crops Prod. 41, 214–220. doi:10.1016/j.indcrop.2012.04.021
Carvalho, R. N., Moura, L. S., Rosa, P. T., and Meireles, M. A. A. (2005). Supercritical fluid extraction from rosemary (Rosmarinus officinalis): kinetic data, extract's global yield, composition, and antioxidant activity. J. Supercrit. Fluids 35 (3), 197–204. doi:10.1016/j.supflu.2005.01.009
Cavalli, J. F., Tomi, F., Bernardini, A. F., and Casanova, J. (2004). Combined analysis of the essential oil of Chenopodium ambrosioides by GC, GC-MS and 13C-NMR spectroscopy: quantitative determination of ascaridole, a heat-sensitive compound. Phytochemical Analysis Int. J. Plant Chem. Biochem. Tech. 15 (5), 275–279. doi:10.1002/pca.761
Chang, R., de Morais, S. A., Napolitano, D. R., Duarte, K. C., Guzman, V. B., and Nascimento, E. (2011). A new approach for quantifying furanodiene and curzerene: a case study on the essential oils of Eugenia uniflora L., Myrtaceae (pitangueira) leaves. Rev. Bras. Farmacogn. 21, 392–396. doi:10.1590/s0102-695x2011005000042
Chemat, F., Abert-Vian, M., and Zill-e-Huma, Y. (2009). “Microwave assisted separations: green chemistry in action,” in Green chemistry research trends (New York, NY: Nova Science Publishers), 33–62.
Chemat, F., Smadja, J., and Lucchesi, M. (2003). Solvent less microwave extraction of natural plants. Eur. Pat. Appl. EP 1 (218), A1.
Chizzola, R. (2007). Composition of the essential oil from laserpitium gallicum. Grown in the wild in southern France. Pharm. Biol. 45 (3), 182–184. doi:10.1080/13880200701213054
Choe, E., and Min, D. B. (2006). Mechanisms and factors for edible oil oxidation. Compr. Rev. food Sci. food Saf. 5 (4), 169–186. doi:10.1111/j.1541-4337.2006.00009.x
Chung, M. J., Cho, S.-Y., Bhuiyan, M. J. H., Kim, K. H., and Lee, S.-J. (2010). Anti-diabetic effects of lemon balm (Melissa officinalis) essential oil on glucose-and lipid-regulating enzymes in type 2 diabetic mice. Br. J. Nutr. 104 (2), 180–188. doi:10.1017/s0007114510001765
Clarke, S. (2008). “Composition of essential oils and other materials,” in Essential chemistry for aromatherapy. Second Edition (Edinburgh: Churchill Livingstone), 123–229.
Cooper, M. A., Holden, C. M., Loftus, P., and Whittaker, D. (1973). The acid catalysed hydration of sabinene and α-thujene. J. Chem. Soc. Perkin Trans. 2 (5), 665–667. doi:10.1039/p29730000665
Debet, M. R., and Gidley, M. J. (2006). Three classes of starch granule swelling: influence of surface proteins and lipids. Carbohydr. Polym. 64 (3), 452–465. doi:10.1016/j.carbpol.2005.12.011
Delibaltova, V. (2020). Effect of sowing period on seed yield and essential oil composition of coriander (Coriandrum sativum L.) in south-east Bulgaria condition. Sci. Pap. Ser. A Agron. 63 (1).
Denayer, R., and Tilquin, B. (1993). Détermination des indices de rétention de composants d'huiles essentielles. J. de Nat. 5 (1), 3–8.
Dieckmann, R. H., and Palamand, S. R. (1974). Autoxidation of some constituents of hops. I. Monoterpene hydrocarbon, myrcene. J. Agric. Food Chem. 22 (3), 498–503. doi:10.1021/jf60193a033
Divkovic, M., Pease, C. K., Gerberick, G. F., and Basketter, D. A. (2005). Hapten–protein binding: from theory to practical application in the in vitro prediction of skin sensitization. Contact Dermat. 53 (4), 189–200. doi:10.1111/j.0105-1873.2005.00683.x
Douglas, M. H., van Klink, J. W., Smallfield, B. M., Perry, N. B., Anderson, R. E., Johnstone, P., et al. (2004). Essential oils from New Zealand manuka: triketone and other chemotypes of Leptospermum scoparium. Phytochemistry 65 (9), 1255–1264. doi:10.1016/j.phytochem.2004.03.019
Dubey, V. S., Bhalla, R., and Luthra, R. (2003). Sucrose mobilization in relation to essential oil biogenesis during palmarosa (Cymbopogon martinii Roxb. Wats. var. motia) inflorescence development. J. Biosci. 28 (4), 479–487. doi:10.1007/bf02705122
El-hawary, S. S., Taha, K. F., Abdel-Monem, A. R., Kirillos, F. N., and Mohamed, A. A. (2013). Chemical composition and biological activities of peels and leaves essential oils of four cultivars of Citrus deliciosa var. tangarina. Am. J. Essent. Oils Nat. Prod. 1 (2), 1–6.
Elmaidomy, A., Hassan, H., Amin, E., Mohamed, W., and Hetta, M. (2017). Premna odorata volatile oil as a new mycobacterium tuberculosis growth inhibitor for the control of tuberculosis disease. Eur. J. Med. Plants 21 (4), 1–11. doi:10.9734/ejmp/2017/38375
El-Nikeety, M., El-Akel, A., El-Hady, M. A., and Badei, A. (2000). Changes in physical properties and chemical constituents of parsley herb volatile oil during storage. Egypt. J. food Sci., 35–49.
Faborode, M., and Favier, J. (1996). Identification and significance of the oil-point in seed-oil expression. J. Agric. Eng. Res. 65 (4), 335–345. doi:10.1006/jaer.1996.0107
Farahmandfar, R., Tirgarian, B., Dehghan, B., and Nemati, A. (2020). Changes in chemical composition and biological activity of essential oil from Thomson navel orange (Citrus sinensis L. Osbeck) peel under freezing, convective, vacuum, and microwave drying methods. Food Sci. and Nutr. 8 (1), 124–138. doi:10.1002/fsn3.1279
Faraldos, J. A., Wu, S., Chappell, J., and Coates, R. M. (2007). Conformational analysis of (+)-germacrene A by variable-temperature NMR and NOE spectroscopy. Tetrahedron 63 (32), 7733–7742. doi:10.1016/j.tet.2007.04.037
Farhat, A., Fabiano-Tixier, A.-S., Visinoni, F., Romdhane, M., and Chemat, F. (2010). A surprising method for green extraction of essential oil from dry spices: microwave dry-diffusion and gravity. J. Chromatogr. A 1217 (47), 7345–7350. doi:10.1016/j.chroma.2010.09.062
Figueiredo, A. C., Barroso, J. G., Pedro, L. G., and Scheffer, J. J. (2008). Factors affecting secondary metabolite production in plants: volatile components and essential oils. Flavour Fragr. J. 23 (4), 213–226. doi:10.1002/ffj.1875
Fouladi, F., Mirtaher, M., and Yasa, N. (2006). Comparison of essential oils composition of Johreniopsis seseloides from Iran, population tehran and Kurdistan. DARU J. Pharm. Sci. 14 (2), 75–77.
Freitas, L. C., Moraes, V. S. V., da Silva, S. B., and de Carvalho Junior, R. N. (2023). Acquisition of essential oils through traditional techniques. Essent. Oils Extr. Methods Appl., 859–869. doi:10.1002/9781119829614.ch36
Ghasemy-Piranloo, F., Kavousi, F., and Kazemi-Abharian, M. (2022). Comparison for the production of essential oil by conventional, novel and biotechnology methods. J. Essent. Oil Res. 34 (5), 455–478. doi:10.1080/10412905.2022.2120557
Gonny, M., Bradesi, P., and Casanova, J. (2004). Identification of the components of the essential oil from wild Corsican Daucus carota L. using 13C-NMR spectroscopy. Flavour Fragr. J. 19 (5), 424–433. doi:10.1002/ffj.1330
Gopalakrishnan, N. (1994). Studies on the storage quality of carbon dioxide-extracted cardamom and clove bud oils. J. Agric. food Chem. 42 (3), 796–798. doi:10.1021/jf00039a039
Grosch, W. (2007). Key odorants of food identified by aroma analysis. Flavourings 2nd ed. Weinheim, Germany: Wiley VCH, 704–743.
Guan, W., Li, S., Yan, R., Tang, S., and Quan, C. (2007). Comparison of essential oils of clove buds extracted with supercritical carbon dioxide and other three traditional extraction methods. Food Chem. 101 (4), 1558–1564. doi:10.1016/j.foodchem.2006.04.009
Habegger, R., and Schnitzler, W. (2000). Aroma compounds in the essential oil of carrots (Daucus carota L. ssp. sativus). 1. Leaves in comparison with roots. J. Appl. Bot. 74 (5/6), 220–223.
Hackl, T., König, W. A., and Muhle, H. (2004). Isogermacrene A, a proposed intermediate in sesquiterpene biosynthesis. Phytochemistry 65 (15), 2261–2275. doi:10.1016/j.phytochem.2004.05.024
Hagvall, L., Sköld, M., Bråred-Christensson, J., Börje, A., and Karlberg, A. T. (2008). Lavender oil lacks natural protection against autoxidation, forming strong contact allergens on air exposure. Contact Dermat. 59 (3), 143–150. doi:10.1111/j.1600-0536.2008.01402.x
Hanif, M. A., Nisar, S., Khan, G. S., Mushtaq, Z., Zubair, M., and Essential, O. (2019). Essential oil research: trends in biosynthesis, analytics. Industrial Appl. Biotechnol. Prod., 3–17.
Hanson, J. R. (1991). Wagner-Meerwein rearrangements. Compr. Org. Synth. 3, 705–719. doi:10.1016/b978-0-08-052349-1.00077-9
Hesham Abdelhafez, O., Refaat Fahim, J., Ramadan Abdelmohsen, U., and Yehia Desoukey, S. (2021). Headspace volatiles of the leaves and flowers of Malvaviscus arboreus Cav.(Malvaceae). J. Mexican Chem. Soc. 65 (1), 141–148. doi:10.29356/jmcs.v65i1.1431
Hofmann, L., Fritz, D., Nitz, S., Kollmannsberger, H., and Drawert, F. (1992). Essential oil composition of three polyploids in the Achillea millefolium ‘complex. Phytochemistry 31 (2), 537–542. doi:10.1016/0031-9422(92)90034-n
İsmail Kirbaşlar, Ş., Gök, A., Gülay Kirbaşlar, F., and Tepe, S. (2012). Volatiles in Turkish clementine (Citrus clementina Hort.) peel. J. Essent. Oil Res. 24 (2), 153–157. doi:10.1080/10412905.2012.659519
Jakab, E., Blazsó, M., Barta-Rajnai, E., Babinszki, B., Sebestyén, Z., Czégény, Z., et al. (2018). Thermo-oxidative decomposition of lime, bergamot and cardamom essential oils. J. Anal. Appl. pyrolysis 134, 552–561. doi:10.1016/j.jaap.2018.08.003
Jarvis, A. P., and Morgan, E. D. (1997). Isolation of plant products by supercritical-fluid extraction. Phytochemical Analysis Int. J. Plant Chem. Biochem. Tech. 8 (5), 217–222. doi:10.1002/(sici)1099-1565(199709/10)8:5<217::aid-pca366>3.3.co;2-a
Johnson, C. B., Kirby, J., Naxakis, G., and Pearson, S. (1999). Substantial UV-B-mediated induction of essential oils in sweet basil (Ocimum basilicum L.). Phytochemistry 51 (4), 507–510. doi:10.1016/s0031-9422(98)00767-5
Jones, B. B., Clark, Jr B. C., and Iacobucci, G. A. (1979). Semipreparative high-performance liquid chromatographic separation of a characterized flavor mixture of monoterpenes. J. Chromatogr. A 178 (2), 575–578. doi:10.1016/s0021-9673(00)92519-6
Kainulainen, P., Nissinen, A., Piirainen, A., Tiilikkala, K., and Holopainen, J. K. (2002). Essential oil composition in leaves of carrot varieties and preference of specialist and generalist sucking insect herbivores. Agric. For. entomology 4 (3), 211–216. doi:10.1046/j.1461-9563.2002.00142.x
Kainulainen, P., Tarhanen, J., Tiilikkala, K., and Holopainen, J. (1998). Foliar and emission composition of essential oil in two carrot varieties. J. Agric. Food Chem. 46 (9), 3780–3784. doi:10.1021/jf980108m
Karamanos, A. J., and Sotiropoulou, D. E. (2013). Field studies of nitrogen application on Greek oregano (Origanum vulgare ssp. hirtum (Link) Ietswaart) essential oil during two cultivation seasons. Industrial Crops Prod. 46, 246–252. doi:10.1016/j.indcrop.2013.01.021
Karousou, R., Grammatikopoulos, G., Lanaras, T., Manetas, Y., and Kokkini, S. (1998). Effects of enhanced UV-B radiation on Mentha spicata essential oils. Phytochemistry 49 (8), 2273–2277. doi:10.1016/s0031-9422(98)00385-9
Kaškonienė, V., Maruška, A., Akuņeca, I., Stankevičius, M., Ragažinskienė, O., Bartkuvienė, V., et al. (2016). Screening of antioxidant activity and volatile compounds composition of Chamerion angustifolium (L.) Holub ecotypes grown in Lithuania. Nat. Prod. Res. 30 (12), 1373–1381. doi:10.1080/14786419.2015.1058792
Kaul, P., Bhattacharya, A., Rajeswara Rao, B., Mallavarapu, G., and Ramesh, S. (1997b). Aetherisches Öl aus Eucalyptus citriodora: zusammensetzung des in Indien erzeugten produkts. Parfümerie Kosmet. 78 (10), 38–40.
Kaul, P. N., Rajeswara Rao, B. R., Bhattacharya, A. K., Mallavarapu, G. R., and Ramesh, S. I. (1997a). Changes in chemical composition of rose-scented geranium (Pelargonium sp.) oil during storage. J. Essent. Oil Res. 9 (1), 115–117. doi:10.1080/10412905.1997.9700729
Keravis, G. (1997). “Spectrométrie de masse et chromatographie dans l’analyse des plantes aromatiques et huiles essentielles,” in Proceedings of the intern congr arom medicinal plants. Editors E. Oils, B. Benjilali, M. Ettalibi, M. Ismaili-Alaoui, and S. Zrira (Rabat, Morocco: Actes Editions).
König, W. A., Bülow, N., Fricke, C., Melching, S., Rieck, A., and Muhle, H. (1996). The sesquiterpene constituents of the liverwort Preissia quadrata. Phytochemistry 43 (3), 629–633. doi:10.1016/0031-9422(96)00354-8
Kovar, K., and Friess, D. (1980). Bestimmung von ätherischen Ölen in Mischungen — Vergleich HPLC mit GC. Arch. DER Pharm. 313 (5), 416–428. doi:10.1002/ardp.19803130505
Kovats, vE. (1958). Gas-chromatographische charakterisierung organischer verbindungen. Teil 1: retentionsindices aliphatischer halogenide, alkohole, aldehyde und ketone. Helvetica Chim. Acta 41 (7), 1915–1932. doi:10.1002/hlca.19580410703
Kubeczka, K. (1979). “Possibilities of quality determination of essential oils which are used in medicine,” in Vorkommen und Analytik ätherischer Ole (Stuttgart, Germany: Thieme), 60–71.
Kubeczka, K.-H. (1993). Moeglichkeiten und Grenzen der Qualitaets-beurteilung arzneilich verwendeter aetherischer Oele. Paperb. APV 34, 85.
Lahlou, M. (2001). Contribution à l’étude des activités antiparasitaires et insecticides par les plantes médicinales Marocaines: Doctoral Thesis. Faculty of Sciences Ain Chock, Casablanca.
Lahlou, M. (2003). Composition and molluscicidal properties of essential oils of five Moroccan Pinaceae. Pharm. Biol. 41 (3), 207–210. doi:10.1076/phbi.41.3.207.15097
Lahlou, M. (2004). Methods to study the phytochemistry and bioactivity of essential oils. Phytotherapy Res. Int. J. Devoted Pharmacol. Toxicol. Eval. Nat. Prod. Deriv. 18 (6), 435–448. doi:10.1002/ptr.1465
Lahlou, M., and Berrada, R. (2001). Potential of essential oils in schistosomiasis control in Morocco. Int. J. Aromather. 11 (2), 87–96. doi:10.1016/s0962-4562(01)80023-0
Lahlou, M., and Berrada, R. (2003). Composition and niticidal activity of essential oils of three chemotypes of Rosmarinus officinalis L. acclimatized in Morocco. Flavour Fragr. J. 18 (2), 124–127. doi:10.1002/ffj.1160
Lahlou, M., Berrada, R., Agoumi, A., and Hmamouchi, M. (2001a). The potential effectiveness of essential oils in the control of human head lice in Morocco. Int. J. Aromather. 10 (3-4), 108–123. doi:10.1016/s0962-4562(01)80005-9
Lahlou, M., Berrada, R., and Hmamouchi, M. (2001b). Molluscicidal activity of thirty essential oils on Bulinus truncatus. Thérapie 56 (1), 71–72.
Lattoo, S. K., Dhar, R. S., Dhar, A. K., Sharma, P. R., and Agarwal, S. G. (2006). Dynamics of essential oil biosynthesis in relation to inflorescence and glandular ontogeny in Salvia sclarea. Flavour Fragr. J. 21 (5), 817–821. doi:10.1002/ffj.1733
Le Quere, J. L., and Latrasse, A. (1990). Composition of the essential oils of black currant buds (Ribes nigrum L.). J. Agric. Food Chem. 38 (1), 3–10. doi:10.1021/jf00091a001
Limam, H., Jemaa, M. B., Tammar, S., Ksibi, N., Khammassi, S., Jallouli, S., et al. (2020). Variation in chemical profile of leaves essential oils from thirteen Tunisian Eucalyptus species and evaluation of their antioxidant and antibacterial properties. Industrial Crops Prod. 158, 112964. doi:10.1016/j.indcrop.2020.112964
Lisiewska, Z., Kmiecik, W., and Słupski, J. (2007). Content of basic components and volatile oils in green dill as depending on the height and usable parts of plants. J. food Qual. 30 (3), 281–299. doi:10.1111/j.1745-4557.2007.00121.x
Lo, P. M., Ragusa, S., Trozzi, A., Dugo, P., Visinoni, F., Fazio, A., et al. (2005). A comparison between different techniques for the isolation of rosemary essential oil. J. Sep. Sci. 28 (3), 273–280. doi:10.1002/jssc.200400037
Loughrin, J. N., Hamilton-Kemp, T. R., Andersen, R. A., and Hildebrand, D. F. (1990). Volatiles from flowers of Nicotiana sylvestris, N. otophora and Malus× domestica: headspace components and day/night changes in their relative concentrations. Phytochemistry 29 (8), 2473–2477. doi:10.1016/0031-9422(90)85169-g
Lucchesi, M. E., Chemat, F., and Smadja, J. (2004). Solvent-free microwave extraction of essential oil from aromatic herbs: comparison with conventional hydro-distillation. J. Chromatogr. a 1043 (2), 323–327. doi:10.1016/j.chroma.2004.05.083
Lukas, B., Schmiderer, C., and Novak, J. (2015). Essential oil diversity of European Origanum vulgare L.(Lamiaceae). Phytochemistry 119, 32–40. doi:10.1016/j.phytochem.2015.09.008
Mahanta, B. P., Bora, P. K., Kemprai, P., Borah, G., Lal, M., and Haldar, S. (2021). Thermolabile essential oils, aromas and flavours: degradation pathways, effect of thermal processing and alteration of sensory quality. Food Res. Int. 145, 110404. doi:10.1016/j.foodres.2021.110404
Malatova, K., Hitimana, N., Niyibizi, T., Simon, J. E., and Juliani, H. R. (2011). Optimization of harvest regime and post-harvest handling in geranium production to maximize essential oil yield in Rwanda. Industrial Crops Prod. 34 (2), 1348–1352. doi:10.1016/j.indcrop.2010.12.018
Mamedova, S., and Akhmedova, E. (1991). Essential oil of turnip-root chervil. Chem. Nat. Compd. 27 (2), 248–249. doi:10.1007/bf00629777
Marotti, M., Dellacecca, V., Piccaglia, R., and Giovanelli, E. (1992). “Agronomic and chemical evaluation of three varieties of Foeniculum vulgare Mill,” in WOCMAP I-medicinal and aromatic plants conference: part 3 of 4 331.
Masango, P. (2005). Cleaner production of essential oils by steam distillation. J. Clean. Prod. 13 (8), 833–839. doi:10.1016/j.jclepro.2004.02.039
Masotti, V., Juteau, F., Bessière, J. M., and Viano, J. (2003). Seasonal and phenological variations of the essential oil from the narrow endemic species Artemisia molinieri and its biological activities. J. Agric. food Chem. 51 (24), 7115–7121. doi:10.1021/jf034621y
Matura, M., Sköld, M., Börje, A., Andersen, K. E., Bruze, M., Frosch, P., et al. (2005). Selected oxidized fragrance terpenes are common contact allergens. Contact Dermat. 52 (6), 320–328. doi:10.1111/j.0105-1873.2005.00605.x
Mayr, E. (1947). Ecological factors in speciation. Evolution 1, 263–288. doi:10.1111/j.1558-5646.1947.tb02723.x
McGraw, G. W., Hemingway, R. W., Ingram, L. L., Canady, C. S., and McGraw, W. B. (1999). Thermal degradation of terpenes: camphene, Δ3-carene, limonene, and α-terpinene. Environ. Sci. and Technol. 33 (22), 4029–4033. doi:10.1021/es9810641
Melo, C. R., Blank, A. F., Oliveira, B. M. S., Santos, A. C. C., Cristaldo, P. F., Araújo, A. P. A., et al. (2021). Formicidal activity of essential oils of Myrcia lundiana chemotypes on Acromyrmex balzani. Crop Prot. 139, 105343. doi:10.1016/j.cropro.2020.105343
Melo, C. R., Picanco, M. C., Santos, A. A., Santos, I. B., Pimentel, M. F., Santos, A. C., et al. (2018). Toxicity of essential oils of Lippia gracilis chemotypes and their major compounds on Diaphania hyalinata and non-target species. Crop Prot. 104, 47–51. doi:10.1016/j.cropro.2017.10.013
Merckx, V. S., Freudenstein, J. V., Kissling, J., Christenhusz, M. J., Stotler, R. E., Crandall-Stotler, B., et al. (2013). Taxonomy and classification. Mycoheterotrophy Biol. plants living fungi, 19–101. doi:10.1007/978-1-4614-5209-6_2
Miething, H., Seger, V., and Hänsel, R. (1990). Determination of photoanethole from a stored essential oil of anise fruits as 4, 4′-dimethoxystilbene by high performance liquid chromatography–ultraviolet coupling. Phytotherapy Res. 4 (3), 121–123. doi:10.1002/ptr.2650040311
Misharina, T., and Polshkov, A. (2005). Antioxidant properties of essential oils: autoxidation of essential oils from laurel and fennel and of their mixtures with essential oil from coriander. Appl. Biochem. Microbiol. 41, 610–618. doi:10.1007/s10438-005-0111-8
Misharina, T., Polshkov, A., Ruchkina, E., and Medvedeva, I. (2003). Changes in the composition of the essential oil of marjoram during storage. Appl. Biochem. Microbiol. 39, 311–316.
Mockutë, D., Bernotienë, G., and Judþentienë, A. (2005). Storage-induced changes in essential oil composition of Leonurus cardiaca L. plants growing wild in Vilnius and of commercial herbs. Chemija 16 (2).
Moghaddam, H. H., Jafari, A. A., Sefidkon, F., and Jari, S. K. (2023). Influence of climatic factors on essential oil content and composition of 20 populations of Nepeta binaludensis Jamzad from Iran. Appl. Biol. Chem. 66 (1), 2. doi:10.1186/s13765-022-00750-6
Mohamed, M. A., and Abdu, M. (2004). Growth and oil production of fennel (Foeniculum vulgare Mill): effect of irrigation and organic fertilization. Biol. Agric. and Hortic. 22 (1), 31–39. doi:10.1080/01448765.2004.9754986
Mohammad, R., Hamid, S., An, A., Norbert, D. K., and Patrick, V. D. (2010). Effects of planting date and seedling age on agro-morphological characteristics, essential oil content and composition of German chamomile (Matricaria chamomilla L.) grown in Belgium. Industrial Crops Prod. 31 (1), 145–152. doi:10.1016/j.indcrop.2009.09.019
Mohammad, W. A., Abbas, S. S., Schaalan, M. F., Elmaidomy, A. H., Hassan, H. M., Amin, E., et al. (2019). Immunomodulatory effect of Premna odorata volatile oils in Mycobacterium tuberculosis by inhibiting TLR4/NF-κB pathway. J. Herbmed Pharmacol. 8 (1), 1–7. doi:10.15171/jhp.2019.01
Mohammed, M. J. (2009). Isolation and identification of anethole from Pimpinella anisum L. fruit oil. An antimicrobial study. J. Pharm. Res. 2 (5), 915–919.
Morin, P., Caude, M., Richard, H., and Rosset, R. (1986). Semipreparative separation of terpenoids from essential oils by high-performance liquid chromatography and their subsequent identification by gas chromatography—mass spectrometry. J. Chromatogr. A 363 (1), 37–56. doi:10.1016/s0021-9673(00)88991-8
Moukhles, A., Ellaghdach, A., Driss, A. B., El Amrani, M. A., Aghmiz, A., and Mansour, A. I. (2022). Chemical profile and in vitro antibacterial potential of essential oils and hydrolat extracts from aerial parts of three wild species of Moroccan Thymus. Sci. Afr. 18, e01434. doi:10.1016/j.sciaf.2022.e01434
Msaada, K., Hosni, K., Taarit, M. B., Chahed, T., Kchouk, M. E., and Marzouk, B. (2007). Changes on essential oil composition of coriander (Coriandrum sativum L.) fruits during three stages of maturity. Food Chem. 102 (4), 1131–1134. doi:10.1016/j.foodchem.2006.06.046
Msaada, K., Hosni, K., Taarit, M. B., Hammami, M., and Marzouk, B. (2009a). Effects of growing region and maturity stages on oil yield and fatty acid composition of coriander (Coriandrum sativum L.) fruit. Sci. Hortic. 120 (4), 525–531. doi:10.1016/j.scienta.2008.11.033
Msaada, K., Taarit, M. B., Hosni, K., Hammami, M., and Marzouk, B. (2009b). Regional and maturational effects on essential oils yields and composition of coriander (Coriandrum sativum L.) fruits. Sci. Hortic. 122 (1), 116–124. doi:10.1016/j.scienta.2009.04.008
Nagahama, S., Tazaki, M., Kobayashi, H., and Sumimoto, M. (1993). Sesquiterpene alcohols from Cryptomeria japonica and C. Fortunei leaf oil. Phytochemistry 33 (4), 879–882. doi:10.1016/0031-9422(93)85295-3
Nagalakshmi, M., Thangadurai, D., Anuradha, T., and Pullaiah, T. (2001). Essential oil constituents of Melia dubia, a wild relative of Azadirachta indica growing in the Eastern Ghats of Peninsular India. Flavour Fragr. J. 16 (4), 241–244. doi:10.1002/ffj.986
Nazemiyeh, H., Razavi, S. M., Delazar, A., Asnaashari, S., Khoi, N. S., Daniali, S., et al. (2009). Distribution profile of volatile constituents in different parts of Astrodaucus orientalis (L.) Drude. Rec. Nat. Prod. 3 (3).
Neffati, M., and Marzouk, B. (2008). Changes in essential oil and fatty acid composition in coriander (Coriandrum sativum L.) leaves under saline conditions. Industrial crops Prod. 28 (2), 137–142. doi:10.1016/j.indcrop.2008.02.005
Neffati, M., and Marzouk, B. (2009). Roots volatiles and fatty acids of coriander (Coriandrum sativum L.) grown in saline medium. Acta physiol. plant. 31 (3), 455–461. doi:10.1007/s11738-008-0253-4
Németh, E. (2003). “Changes in essential oil quantity and quality influenced by ontogenetic factors,” in III WOCMAP congress on medicinal and aromatic plants-volume 1 (Bioprospecting and Ethnopharmacology), 675.
Neuenschwander, U., Guignard, F., and Hermans, I. (2010). Mechanism of the aerobic oxidation of α-pinene. ChemSusChem Chem. and Sustain. Energy and Mater. 3 (1), 75–84. doi:10.1002/cssc.200900228
Newbery, J., de Haddad, M. L., and Charlwood, K. (1983). High-performance liquid chromatography of terpenoid alcohols in essential oils. Anal. Chim. Acta 147, 387–391. doi:10.1016/0003-2670(83)80109-3
Nguyen, H., Campi, E. M., Jackson, W. R., and Patti, A. F. (2009). Effect of oxidative deterioration on flavour and aroma components of lemon oil. Food Chem. 112 (2), 388–393. doi:10.1016/j.foodchem.2008.05.090
Nilsson, U., Bergh, M., Shao, L., and Karlberg, A.-T. (1996). Analysis of contact allergenic compounds in oxidized d-limonene. Chromatographia 42 (3-4), 199–205. doi:10.1007/bf02269653
Njoroge, S. M., Koaze, H., Karanja, P. N., and Sawamura, M. (2005). Essential oil constituents of three varieties of Kenyan sweet oranges (Citrus sinensis). Flavour Fragr. J. 20 (1), 80–85. doi:10.1002/ffj.1377
Norin, T., and Smedman, L. (1971). Cyclopropylcarbinyl rearrangements in sabinene and alpha-thujene. Acta Chem. Scand. 25 (6), 2010–and.
Nurzyńska-Wierdak, R. (2012). Sweet basil essential oil composition: relationship between cultivar, foliar feeding with nitrogen and oil content. J. Essent. Oil Res. 24 (3), 217–227. doi:10.1080/10412905.2012.676763
Nurzynska-Wierdak, R. (2013). Does mineral fertilization modify essential oil content and chemical composition in medicinal plants? Acta Sci. Pol. Hortorum Cultus 12 (5).
Oezel, A. (2009). Anise (Pimpinella anisum): changes in yields and component composition on harvesting at different stages of plant maturity. Exp. Agric. 45 (1), 117–126. doi:10.1017/s0014479708006959
Ogawa, C. A., Diagone, C. A., and Lanças, F. M. (2002). Separation of monoterpenes in orange essential oil by capillary liquid chromatography and micellar electrokinetic chromatography. J. Liq. Chromatogr. and Relat. Technol. 25 (10-11), 1651–1659. doi:10.1081/jlc-120005712
Olle, M., Bender, I., and Koppe, R. (2010). The content of oils in umbelliferous crops and its formation. Agron. Res. 8 (3), 687–696.
Osman, Y., and El-Wahab, M. (2009). Economic evaluations for harvesting management of parsley (Petroselinium sativum crispum (Mill.) Nym) and dill (Anithum Graveolens L.) plants under North Sinai conditions. Res. J. Agric. Biol. Sci. 5 (3), 218–222.
Pallado, P., Tassinato, G., D'Alpaos, M., and Traldi, P. (1997). Gas chromatography/mass spectrometry in aroma chemistry: a comparison of essential oils and flavours extracted by classical and supercritical techniques. Rapid Commun. mass Spectrom. 11 (12), 1335–1341. doi:10.1002/(sici)1097-0231(199708)11:12<1335::aid-rcm970>3.0.co;2-r
Paudel, P. N., Satyal, P., Satyal, R., Setzer, W. N., and Gyawali, R. (2022). Chemical composition, enantiomeric distribution, antimicrobial and antioxidant activities of Origanum majorana L. essential oil from Nepal. Molecules 27 (18), 6136. doi:10.3390/molecules27186136
Peixoto, M. G., Bacci, L., Blank, A. F., Araújo, A. P. A., Alves, P. B., Silva, J. H. S., et al. (2015). Toxicity and repellency of essential oils of Lippia alba chemotypes and their major monoterpenes against stored grain insects. Industrial Crops Prod. 71, 31–36. doi:10.1016/j.indcrop.2015.03.084
Petropoulos, S., Daferera, D., Akoumianakis, C., Passam, H., and Polissiou, M. (2004). The effect of sowing date and growth stage on the essential oil composition of three types of parsley (Petroselinum crispum). J. Sci. Food Agric. 84 (12), 1606–1610. doi:10.1002/jsfa.1846
Petropoulos, S. A., Daferera, D., Polissiou, M. G., and Passam, H. C. (2009). Effect of nitrogen-application rate on the biomass, concentration, and composition of essential oils in the leaves and roots of three types of parsley. J. Plant Nutr. Soil Sci. 172 (2), 210–215. doi:10.1002/jpln.200700311
Pratt, D. A., and Porter, N. A. (2003). Role of hyperconjugation in determining carbon− oxygen bond dissociation enthalpies in alkylperoxyl radicals. Org. Lett. 5 (4), 387–390. doi:10.1021/ol027094x
Preuss, F. R. (1964). Uber photomechanische Veränderungen von Bestandteilen des Kümmelöles. Dtsch. Apoth. Ztg 104, 1797–1803.
Rajabi, H., Mosleh, M. H., Mandal, P., Lea-Langton, A., and Sedighi, M. (2020). Emissions of volatile organic compounds from crude oil processing–Global emission inventory and environmental release. Sci. Total Environ. 727, 138654. doi:10.1016/j.scitotenv.2020.138654
Ramezani, S., Rahmanian, M., Jahanbin, R., Mohajeri, F., Rezaei, M. R., and Solaimani, B. (2009). Diurnal changes in essential oil content of Coriander (Coriandrum sativum L.) aerial parts from Iran. Res. J. Biol. Sci. 4 (3), 277–281.
Rao, B. R., Rajput, D., and Patel, R. (2011). Storage of essential oils: influence of presence of water for short periods on the composition of major constituents of the essential oils of four economically important aromatic crops. J. Essent. Oil Bear. Plants 14 (6), 673–678. doi:10.1080/0972060x.2011.10643988
Raut, J. S., and Karuppayil, S. M. (2014). A status review on the medicinal properties of essential oils. Industrial crops Prod. 62, 250–264. doi:10.1016/j.indcrop.2014.05.055
Ravi Kiran, C., Chakka, A. K., Padmakumari Amma, K., Nirmala Menon, A., Sree Kumar, M., and Venugopalan, V. (2013). Essential oil composition of fresh ginger cultivars from North-East India. J. Essent. oil Res. 25 (5), 380–387. doi:10.1080/10412905.2013.796496
Raynie, D. E. (2006). Modern extraction techniques. Anal. Chem. 78 (12), 3997–4004. doi:10.1021/ac060641y
Reverchon, E., and Marrone, C. (1997). Supercritical extraction of clove bud essential oil: isolation and mathematical modeling. Chem. Eng. Sci. 52 (20), 3421–3428. doi:10.1016/s0009-2509(97)00172-3
Riaz, U., Iqbal, S., Sohail, M. I., Samreen, T., Ashraf, M., Akmal, F., et al. (2021). A comprehensive review on emerging importance and economical potential of medicinal and aromatic plants (MAPs) in current scenario. Pak. J. Agric. Res. 34, doi:10.17582/journal.pjar/2021/34.2.381.392
Riela, S., Bruno, M., Formisano, C., Rigano, D., Rosselli, S., Saladino, M. L., et al. (2008). Effects of solvent-free microwave extraction on the chemical composition of essential oil of Calamintha nepeta (L.) Savi compared with the conventional production method. J. Sep. Sci. 31 (6-7), 1110–1117. doi:10.1002/jssc.200700425
Şahin, F., Güllüce, M., Daferera, D., Sökmen, A., Sökmen, M., Polissiou, M., et al. (2004). Biological activities of the essential oils and methanol extract of Origanum vulgare ssp. vulgare in the Eastern Anatolia region of Turkey. Food control. 15 (7), 549–557. doi:10.1016/j.foodcont.2003.08.009
Salem, M. Z., Ashmawy, N. A., Elansary, H. O., and El-Settawy, A. A. (2015). Chemotyping of diverse Eucalyptus species grown in Egypt and antioxidant and antibacterial activities of its respective essential oils. Nat. Prod. Res. 29 (7), 681–685. doi:10.1080/14786419.2014.981539
Schaller, R. G., and Schnitzler, W. H. (2000). Nitrogen nutrition and flavour compounds of carrots (Daucus carota L) cultivated in Mitscherlich pots. J. Sci. Food Agric. 80 (1), 49–56. doi:10.1002/(sici)1097-0010(20000101)80:1<49::aid-jsfa498>3.0.co;2-9
Schieberle, P., Ehrmeier, H., and Grosch, W. (1988). Aroma compounds resulting from the acid catalysed breakdown of citral. Z. für Lebensm. Forsch. 187, 35–39. doi:10.1007/bf01454320
Schieberle, P., Maier, W., Firl, J., and Grosch, W. (1987). HRGC separation of hydroperoxides formed during the photosensitized oxidation of (R)—(+)-Limonene. J. High Resolut. Chromatogr. 10 (11), 588–593. doi:10.1002/jhrc.1240101102
Schwanbeck, J., and Kubeczka, K. (1979). Application of HPLC for separation of volatile terpene hydrocarbons. Vorkommen Anal. ätherischer Öle, 72–76.
Sedlakova, J., Kocourkova, B., and Kuban, V. (2001). Determination of essential oils content and composition in caraway (Carum carvi L.). Czech J. Food Sci. 19 (1), 31–36. doi:10.17221/6571-cjfs
Sellami, I. H., Wannes, W. A., Bettaieb, I., Berrima, S., Chahed, T., Marzouk, B., et al. (2011). Qualitative and quantitative changes in the essential oil of Laurus nobilis L. leaves as affected by different drying methods. Food Chem. 126 (2), 691–697. doi:10.1016/j.foodchem.2010.11.022
Semiz, G., Ünlükara, A., Yurtseven, E., Suarez, D., Telci, I., et al. (2012). Salinity impact on yield, water use, mineral and essential oil content of fennel (Foeniculum vulgare Mill). J. Agricul. Sci. 18 (3), 177–186.
Semerdjieva, I. B., Zheljazkov, V. D., Dincheva, I., Astatkie, T., and Kačániová, M. (2020). Chemotypes of Juniperus oxycedrus in Bulgaria and the antimicrobial activity of galbuli essential oils. Industrial Crops Prod. 158, 113005. doi:10.1016/j.indcrop.2020.113005
Setzer, W. N. (2008). Ab initio analysis of the Cope rearrangement of germacrane sesquiterpenoids. J. Mol. Model. 14, 335–342. doi:10.1007/s00894-008-0274-3
Shabby, A., El-Gengaihi, S., and Khattab, M. (1995). Oil of Melissa officinalis L., as affected by storage and herb drying. J. Essent. oil Res. 7 (6), 667–669. doi:10.1080/10412905.1995.9700525
Shuaib, K. A., Azeez, O. I., and Kayode, R. A. (2021). Global health: challenges and responses. Natural volatiles and essential oils. Nat. Volatiles Essent. Oils 8 (4), 11351–11361.
Sicari, V., and Poiana, M. (2017). Comparison of the volatile component of the essential oil of kumquat (Fortunella margarita swingle) extracted by supercritical carbon dioxide, hydrodistillation and conventional solvent extraction. J. Essent. Oil Bear. Plants 20 (1), 87–94. doi:10.1080/0972060x.2017.1282841
Sinki, G., Assaf, R., and Lombardo, J. (1997). Flavor changes: a review of the principal causes and reactions. Perfumer and flavorist 22 (4), 23–31.
Sköld, M., Karlberg, A.-T., Matura, M., and Börje, A. (2006). The fragrance chemical β-caryophyllene—air oxidation and skin sensitization. Food Chem. Toxicol. 44 (4), 538–545. doi:10.1016/j.fct.2005.08.028
Solinas, V., Gessa, C., and Delitala, L. F. (1981). High-performance liquid chromatographic analysis of carvacrol and thymol in the essential oil of Thymus capitatus. J. Chromatogr. A 219 (2), 332–337. doi:10.1016/s0021-9673(00)87947-9
Stahl-Biskup, E., and Laakso, I. (1990). Essential oil polymorphism in Finnish Thymus species. Planta medica. 56 (05), 464–468. doi:10.1055/s-2006-961012
Staniszewska, M., Kula, J., Wieczorkiewicz, M., and Kusewicz, D. (2005). Essential oils of wild and cultivated carrots—the chemical composition and antimicrobial activity. J. Essent. Oil Res. 17 (5), 579–583. doi:10.1080/10412905.2005.9699002
Stefanini, M., Ming, L., Marques, M., Meireles, M., Moura, L., and Marchese, J. (2006a). Seed productivity, yield and composition of the essential oil of fennel Foeniculum vulgare var. dulcis in the season of the year. Rev. Bras. Plantas Med. 8, 86–90.
Stolle, A., Ondruschka, B., and Hopf, H. (2009). Thermal rearrangements of monoterpenes and monoterpenoids. Helvetica Chim. Acta 92 (9), 1673–1719. doi:10.1002/hlca.200900041
Sutour, S., Luro, F., Bradesi, P., Casanova, J., and Tomi, F. (2016). Chemical composition of the fruit oils of five Fortunella species grown in the same pedoclimatic conditions in Corsica (France). Nat. Product. Commun. 11 (2), 1934578X1601100231. doi:10.1177/1934578x1601100231
Tabanca, N., Demirci, B., Ozek, T., Kirimer, N., Baser, K. H. C., Bedir, E., et al. (2006). Gas chromatographic–mass spectrometric analysis of essential oils from Pimpinella species gathered from Central and Northern Turkey. J. Chromatogr. A 1117 (2), 194–205. doi:10.1016/j.chroma.2006.03.075
Tajuddin, S. N., and Yusoff, M. M. (2010). Chemical composition of volatile oils of Aquilaria malaccensis (Thymelaeaceae) from Malaysia. Nat. Product. Commun. 5 (12), 1965–1968. doi:10.1177/1934578x1000501229
Telci, I., Demirtas, I., and Sahin, A. (2009). Variation in plant properties and essential oil composition of sweet fennel (Foeniculum vulgare Mill.) fruits during stages of maturity. Industrial Crops Prod. 30 (1), 126–130. doi:10.1016/j.indcrop.2009.02.010
Tomaino, A., Cimino, F., Zimbalatti, V., Venuti, V., Sulfaro, V., De Pasquale, A., et al. (2005). Influence of heating on antioxidant activity and the chemical composition of some spice essential oils. Food Chem. 89 (4), 549–554. doi:10.1016/j.foodchem.2004.03.011
Tomi, F., Bighelli, A., Bradesi, P., and Casanova, J. (1997). “Analyse des huiles essentielles par RMN 13C,” in Proceedings of the intern congr arom med plants. Editors E. Oils, B. Benjilali, M. Ettalibi, M. Ismaili-Alaoui, and S. Zrira (Rabat, Morocco: Actes Editions), 499.
Toyota, M., Koyama, H., Mizutani, M., and Asakawa, Y. (1996). (−)-ent-spathulenol isolated from liverworts is an artefact. Phytochemistry 41 (5), 1347–1350. doi:10.1016/0031-9422(95)00798-9
Treibs, W. (1956). Gewinnung atherischer Ole durch Destillation, Extraktion und Pressung. E Gildemeister/Fr Hoffmann Die ätherischen Ole 1, 307–309.
Treibs, W., Gildemeister, M. D., and Hoffmann, F. (1960). Die ätherischen öle band IIIa. Berlin: Akademie-Verlag.
Tsamaidi, D., Karapanos, I., Passam, H., Daferera, D., and Polissiou, M. (2010). “The yield and composition of dill essential oil in relation to N application, season of cultivation and stage of harvest,” in XXVIII international horticultural congress on science and horticulture for people (IHC2010): international symposium on 936.
Tuncturk, R., and Tuncturk, M. (2006). Effects of different phosphorus levels on the yield and quality components of cumin (Cuminum cyminum L.). Res. J. Agric. Biol. Sci. 2 (6), 336–340.
Turek, C., Kirschmann, N., and Stintzing, F. (2012). Quality monitoring of selected essential oils upon storage at different temperature regimes. Z. für Arznei-& Gewürzpflanzen. 17 (2), 73–79.
Turek, C., and Stintzing, F. C. (2011a). Application of high-performance liquid chromatography diode array detection and mass spectrometry to the analysis of characteristic compounds in various essential oils. Anal. Bioanal. Chem. 400, 3109–3123. doi:10.1007/s00216-011-4976-5
Turek, C., and Stintzing, F. C. (2011b). Evaluation of selected quality parameters to monitor essential oil alteration during storage. J. food Sci. 76 (9), C1365–C1375. doi:10.1111/j.1750-3841.2011.02416.x
Turek, C., and Stintzing, F. C. (2012). Impact of different storage conditions on the quality of selected essential oils. Food Res. Int. 46 (1), 341–353. doi:10.1016/j.foodres.2011.12.028
Turek, C., and Stintzing, F. C. (2013). Stability of essential oils: a review. Compr. Rev. food Sci. food Saf. 12 (1), 40–53.
Udagawa, Y. (1994). Some responses of dill (Anethum graveolens) and thyme (Thymus vulgaris), grown in hydroponic, to the concentration of nutrient solution. Hydroponics Transpl. Prod. 396, 203–210. doi:10.17660/actahortic.1995.396.24
Van Den Dool, H., and Kratz, P. D. (1963). A generalization of the retention index system including linear temperature programmed gas-liquid partition chromatography. J. Chromatogr. 11, 463–471. doi:10.1016/s0021-9673(01)80947-x
Vandewiele, N., Van Geem, K., Reyniers, M.-F., and Marin, G. (2011). “Modeling the thermal rearrangements of bicyclic monoterpenes,” in 7th international conference on chemical kinetics (ICCK 2011).
Varona, S., Martin, A., Cocero, M., and Gamse, T. (2008). Supercritical carbon dioxide fractionation of Lavandin essential oil: experiments and modeling. J. Supercrit. Fluids 45 (2), 181–188. doi:10.1016/j.supflu.2007.07.010
Velasco, J., and Dobarganes, C. (2002). Oxidative stability of virgin olive oil. Eur. J. Lipid Sci. Technol. 104 (9-10), 661–676. doi:10.1002/1438-9312(200210)104:9/10<661::aid-ejlt661>3.0.co;2-d
Verma, R. S., Rahman, L. U., Verma, R. K., Chauhan, A., and Singh, A. (2013). Essential oil composition of Pelargonium graveolens L’Her ex Ait. cultivars harvested in different seasons. J. Essent. Oil Res. 25 (5), 372–379. doi:10.1080/10412905.2013.782476
Wallach, O. (1907). Über Sabinen und dessen Beziehungen zum Terpinen. Berichte Dtsch. Chem. Ges. 40, 585–595. doi:10.1002/cber.19070400188
Wallach, O. (1908). Zur Kenntniss der Terpene und der ätherischen Oele. Justus Liebig's Ann. Chem. 360 (1-2), 82–104. doi:10.1002/jlac.19083600105
Wang, L.-H., and Liu, H.-J. (2012). Determination of fragrance allergens in essential oils and evaluation of their in vitro permeation from essential oil formulations through cultured skin. J. Anal. Chem. 67, 64–71. doi:10.1134/s1061934812010182
Wichtmann, E. M., and Stahl--Biskup, E. (1987). Composition of the essential oils from caraway herb and root. Flavour Fragr. J. 2 (2), 83–89. doi:10.1002/ffj.2730020207
Widmark, G., Blohm, S., Sandberg, R., Schliack, J., and Reio, L. (1957). A Comparative Study of the Autoxidation of delta3-Carene, alpha-Pinene, beta-Pinene, and (+)-Limonene. Acta Chem. Scand. 11, 392–394. doi:10.3891/acta.chem.scand.11-0392
Worsfold, P., Townshend, A., Poole, C. F., and Miró, M. (2019). Encyclopedia of analytical science. Elsevier.
Yang, F., Li, S., Zhao, J., Lao, S., and Wang, Y. (2007). Optimization of GC–MS conditions based on resolution and stability of analytes for simultaneous determination of nine sesquiterpenoids in three species of Curcuma rhizomes. J. Pharm. Biomed. analysis 43 (1), 73–82. doi:10.1016/j.jpba.2006.06.014
Zahran, E. M., Abdelmohsen, U. R., Khalil, H. E., Desoukey, S. Y., Fouad, M. A., and Kamel, M. S. (2020). Diversity, phytochemical and medicinal potential of the genus Ocimum L.(Lamiaceae). Phytochem. Rev. 19, 907–953. doi:10.1007/s11101-020-09690-9
Zehtab-Salmasi, S., Javanshir, A., Omidbaigi, R., Alyari, H., and Ghassemi-Golezani, K. (2001). Effects of water supply and sowing date on performance and essential oil production of anise (Pimpinella anisum L.). Acta Agron. Hung. 49 (1), 75–81. doi:10.1556/aagr.49.2001.1.9
Zheljazkov, V. D., Astatkie, T., O'Brocki, B., and Jeliazkova, E. (2013a). Essential oil composition and yield of anise from different distillation times. HortScience 48 (11), 1393–1396. doi:10.21273/hortsci.48.11.1393
Zheljazkov, V. D., Cantrell, C. L., Astatkie, T., and Jeliazkova, E. (2013b). Distillation time effect on lavender essential oil yield and composition. J. Oleo Sci. 62 (4), 195–199. doi:10.5650/jos.62.195
Zheljazkov, V. D., Pickett, K. M., Caldwell, C. D., Pincock, J. A., Roberts, J. C., and Mapplebeck, L. (2008). Cultivar and sowing date effects on seed yield and oil composition of coriander in Atlantic Canada. Industrial crops Prod. 28 (1), 88–94. doi:10.1016/j.indcrop.2008.01.011
Keywords: essential oils, environmental, cultivation, postharvest, therapeutic potential
Citation: Abdelmohsen UR and Elmaidomy AH (2025) Exploring the therapeutic potential of essential oils: a review of composition and influencing factors. Front. Nat. Prod. 4:1490511. doi: 10.3389/fntpr.2025.1490511
Received: 03 September 2024; Accepted: 11 March 2025;
Published: 17 April 2025.
Edited by:
Luciana Scotti, Federal University of Paraíba, BrazilReviewed by:
Abdelnaser Abdelghany Elzaawely, Tanta University, EgyptMario A. Gómez-Hurtado, Michoacana University of San Nicolás de Hidalgo, Mexico
Copyright © 2025 Abdelmohsen and Elmaidomy. This is an open-access article distributed under the terms of the Creative Commons Attribution License (CC BY). The use, distribution or reproduction in other forums is permitted, provided the original author(s) and the copyright owner(s) are credited and that the original publication in this journal is cited, in accordance with accepted academic practice. No use, distribution or reproduction is permitted which does not comply with these terms.
*Correspondence: Usama Ramadan Abdelmohsen, dXNhbWEucmFtYWRhbkBtdS5lZHUuZWc=; Abeer H. Elmaidomy, YWJlZXIwMTExNTBAcGhhcm0uYnN1LmVkdS5lZw==