- 1Henan University of Chinese Medicine, Henan Luoyang Orthopedic Hospital (Henan Provincial Orthopedic Hospital), Zhengzhou, China
- 2Department of Osteology, The Second Affiliated Hospital of Luohe Medical College, Luohe, China
- 3Graduate School, Hunan University of Chinese Medicine, Changsha, China
The most common degenerative condition affecting the musculoskeletal system, and the leading cause of persistent low back pain, is intervertebral disc degeneration (IDD). IDD is increasingly common with age and has a variety of etiologic factors including inflammation, oxidative stress, extracellular matrix (ECM) degradation, and apoptosis that interact with each other to cause IDD. Because it is difficult to determine the exact pathogenesis of IDD, there is a lack of effective therapeutic agents. Melatonin has been intensively studied for its strong anti-inflammatory, antioxidant, and anti-apoptotic properties. Melatonin is a pleiotropic indole-stimulating hormone produced by the pineal gland, which can be used to treat a wide range of degenerative diseases. Therefore, melatonin supplementation may be a viable treatment for IDD. This article reviews the current mechanisms of IDD and the multiple roles regarding melatonin’s anti-inflammatory, antioxidant, anti-apoptotic, and mitigating ECM degradation in IDD, incorporating new current research perspectives, as well as recent studies on drug delivery systems.
1 Introduction
One of the main causes of disability and a significant socioeconomic burden is low back pain (LBP), which is acknowledged worldwide. It is well known that 80% of people will experience low back pain at some point in their lives. In the past 20 years, the prevalence of LBP has increased by 50% worldwide, and it is one of the main causes of disability (Violante et al., 2015; Diwan and Melrose, 2023; Hartvigsen et al., 2018). Moreover, one of the main causes of LBP, intervertebral disc degeneration (IDD), accounts for about 40% of all cases of LBP (Cheung et al., 2009). Currently, conservative and surgical approaches are the primary methods used to treat IDD. These two treatment modalities have formed a more mature system and have achieved good results in the clinic (Wu et al., 2020; Xin et al., 2022). Analgesics, anti-inflammatory medications, and physical therapy are the mainstays of conservative treatment, nevertheless, these approaches are only effective in treating symptoms, which merely conceal or postpone the progression of IDD (Xin et al., 2022; Kamali et al., 2021). As a last resort, surgery is used for procedures like spinal fusion or total disc replacement. Modern surgical methods are highly advanced and appropriate for the great majority of individuals with IDD. However, there are dangers associated with surgical treatment as well, such as operative site infection or problems from neighboring segment disease (Xin et al., 2022; Phan et al., 2021; Takahashi et al., 2006). To make up for the limitations of the current clinical therapeutic approaches, a novel therapeutic technique for suppressing IDD must be developed. Small molecules, which are organic substances with a molecular weight of less than 900 Da, have been shown to have regenerative effect on IDD recently. In order to treat discogenic pain and repair damaged intervertebral disks (IVD) by reestablishing tissue homeostasis, several small molecules have demonstrated encouraging outcomes in both in vivo and in vitro studies (Kamali et al., 2021; Pan et al., 2018; Liu MY. et al., 2022; Krupkova et al., 2014), which provided ideas for follow-up research.
As a small molecule drug, melatonin has been proved to promote osteochondral development, repair (Zhang et al., 2010; Gao et al., 2014). Except that melatonin has anti-inflammatory, antioxidant and anti-apoptosis effects, this is consistent with the direction of IDD treatment research. Melatonin has great potential in treating IDD (Tian et al., 2021; Zhang Y. et al., 2019; He et al., 2018; Chen et al., 2020a). Thus, the purpose of this review was to give a thorough theoretical foundation for future pertinent research while concentrating on the possible therapeutic principles of melatonin in IDD (Table 1).
2 Anatomy and mechanisms of IDD
The annulus fibrosus (AF), endplate cartilage (EP), and nucleus pulposus (NP) make up IVD (Zhang et al., 2010; Gao et al., 2014). Nucleus pulposus cells (NPCs) and ECM rich in collagen II and proteoglycans make up NP (Zhang et al., 2010; Gao et al., 2014). The presence of negatively charged side chains in proteoglycans causes the NP to become very hydrated at high osmotic pressures, enabling the IVD to withstand compressive stresses and experience reversible deformation (Zhang et al., 2010; Gao et al., 2014). The passage of fluid and solutes into and out of the disc is intimately linked to the CEP, a layer of hyaline cartilage covering the caudal and cephalad ends of the disc (Zhang et al., 2010; Gao et al., 2014). AF surrounding the outer region of the IVD, is a highly fibrous and well-organized tissue. It consisted of multiple layers of concentric lamellae, angularly laminated in the fiber direction, which restrict the mobility of the IVD and contain the internal NP (Torre et al., 2019). AF is significant in biomechanical characterization of IVDs, because its structural integrity is vital for limiting NP and maintaining physiologic intradiscal stress under load, IDD is the result of degenerative changes in the biomechanical and structural features of the IVD, such as AF cracking and NP volume loss (Chu et al., 2018).
Extracellular matrix (ECM) breakdown, oxidative inflammation, and apoptosis are the most common pathogenic alterations linked to IDD (Clouet et al., 2009; Chen et al., 2024). According to the current study, NPCs (IVD Cells in the central region) keep the ECM in a balanced state. The anabolism and catabolism of ECM are in dynamic balance in a healthy IVD. The maintenance of ECM integrity depends on type I and type II collagens, which give tensile strength, and water-bound proteoglycans, such as aggregated proteoglycans. However, dysregulation of NPC metabolism results in a reduction in their capacity to synthesize ECM components and an increase in the secretion of molecules that promote ECM degradation, such as matrix metalloproteinases (MMP) and metalloproteases with platelet-responsive protein motifs (ADAMTS). IDD typically happens when the ECM’s balance is upset (Vo et al., 2013; Roberts et al., 2000; Liang et al., 2022; Le Maitre et al., 2007).
The inflammatory response also has a significant impact. IVD degeneration is characterized by increased levels of the pro-inflammatory cytokines tumor necrosis factor (TNF), interleukin (IL)-1α, IL-1β, IL-6, and IL-17 secreted by IVD cells, which promote ECM degradation, chemokine production, and IVD cells cellular phenotypic change. Concurrently, chemokines released by degenerative discs encourage immune cell infiltration and activation, so intensifying the inflammatory cascade response (Xin et al., 2022; Risbud and Shapiro, 2014).
This process is also influenced by oxidative stress, which interacts with the inflammatory response. Excess reactive oxygen species (ROS) trigger the nuclear factor kappa-B (NF-κB) and mitogen-activated protein kinase (MAPK) pathways, causing an imbalance in the synthesis and breakdown of ECM in IVD cells as well as an increase in the secretion of pro-inflammatory factors. These alterations eventually cause NPCs to undergo apoptosis and maintain an inflammatory microenvironment, which exacerbates IVD disruption and ROS production (Zhou et al., 2010; Zhu et al., 2019). Cellular senescence is an irreversible cell cycle arrest caused by various external stimuli or telomere unraveling. Senescent cells exhibit a variety of morphological changes as they aggregate into clusters, increase in size, and become flattened and vacuolated. In addition, these cells will also fail to replicate in response to mitotic stimuli and abnormally secrete pro-inflammatory cytokines and matrix-degrading proteases (Acosta et al., 2008). Senescent cells alter the balance of catabolic and anabolic pathways produced by the ECM, a potential cause of IDD (Gruber et al., 2009). Increased ROS in the IDD is linked to NPCs senescence, and it has been demonstrated that raised surface ROS and activated NF-κB cause NPC senescence (Wang J. et al., 2022; Li F. et al., 2021). IVD cells experience senescence, irreversible growth arrest, the synthesis of MMPs and pro-inflammatory cytokines, and overstress, which culminates in programmed cell death, as they age and degenerate (Yurube et al., 2023).
In conclusion, although there is no clear standard explanation for the mechanism of IDD, current studies have demonstrated that multiple factors such as ECM degradation, inflammation, oxidative stress, and apoptosis are interconnected and interact with each other, and work together to promote the progression of IDD (Figure 1).
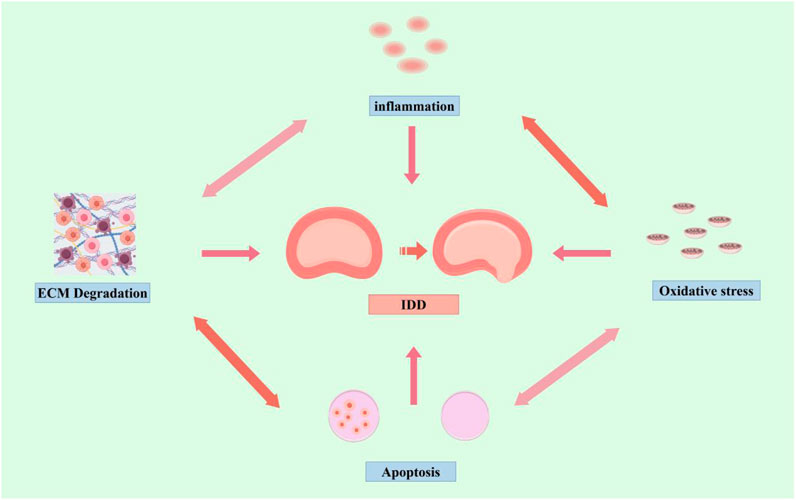
Figure 1. Mechanisms of IDD (by Figdraw). Inflammation, oxidative stress, apoptosis, and ECM degradation, promote the progression of IDD.
3 Pharmacologic effects of melatonin
A naturally occurring hormone, melatonin is mostly secreted by the pineal gland (Wang SY. et al., 2020). Melatonin, also known as N-acetyl-5-methoxytryptamine, is a neurohormone that maintains circadian rhythms (Pévet, 2016). It is well known that melatonin is widely used for its ability to improve circadian rhythms (Cajochen et al., 2003; Vasey et al., 2021; Zisapel, 2018; Hirayama et al., 2023). Meanwhile, melatonin is safe and widely used in various diseases (Vine et al., 2022; Esposito et al., 2019; Reiter et al., 2002) such as cancers, cardiovascular disease, gastrointestinal disorders, mood disorders (Talib, 2018; Bhattacharya et al., 2019; Ahmad et al., 2023; Favero et al., 2018). Moreover, melatonin has been extensively documented to have a role in the prevention of a number of degenerative illnesses, such as Parkinson’s, Alzheimer’s, osteoporosis, osteoarthritis, IDD, and others (Shen et al., 2022; Sumsuzzman et al., 2021; Tamtaji et al., 2020; Li et al., 2019; Hardeland et al., 2015; Zhang et al., 2022; Li et al., 2022).
Melatonin works in a variety of ways. From an anti-inflammatory standpoint, it inhibits inflammation-promoting processes. TNF-α, IL-6, and IL-1β are among the inflammatory factors whose release is inhibited by melatonin (Zhang Y. et al., 2019). Furthermore, it initiates the anti-inflammatory network through SIRT1 activation, nuclear factor erythroid 2 related factor (Nrf2) upregulation and NF-κB downregulation, as well as the production of IL-4 and IL-10, two anti-inflammatory cytokines (Hardeland, 2019; Arioz et al., 2019). In addition, melatonin acts as an antioxidant with powerful antioxidant properties. Unlike certain classical antioxidants, melatonin reacts with free radicals in a cascade and can be generated under mild oxidative stress. These characteristics of melatonin shield organisms from harmful oxidative damage (Tan et al., 2015; Reiter et al., 2018). Apart from its anti-inflammatory and antioxidant properties, research suggests that melatonin may also have anti-apoptotic effects, it had also been shown that melatonin inhibited apoptosis through activation of the Sirt1/Nrf2 pathway (Zhang W. et al., 2023). All in all, the potent anti-inflammatory, antioxidant, and anti-apoptotic properties of melatonin have been acknowledged in a variety of fields.
The safety of exogenous melatonin is also well established; in a 1967 study, mice survived the administration of a dose of 800 mg/kg of melatonin without experiencing any notable side effects, and its lethal dose is currently unknown in clinical practice (Author anonymous, 2004). However, there is no proof of ophthalmotoxicity, hepatotoxicity, nephrotoxicity, or myelotoxicity. In fact, “melatonin has been given to humans at relatively high doses (1 g per day orally) for 1 month” and researchers have noted high plasma concentrations of melatonin, headaches, dizziness, and drowsiness (Schomerus and Korf, 2005). In summary, melatonin has a good safety record and is being studied extensively in the areas of anti-inflammatory, antioxidant, and anti-apoptotic properties. Therefore, melatonin offers potential as a drug for the treatment of IDD because these effects align with the path of research on IDD treatment.
4 Melatonin and IDD
4.1 Melatonin regulates inflammation
IDD is largely caused by an inflammatory response, and inflammatory mediators are essential to IDD (Li Z. et al., 2023). According to the study, several pro-inflammatory cytokines such as TNF-α, IL-1β, IL-17, IL-1α, and IL-8 are increased significantly in IDD (Li Z. et al., 2023; Li H. et al., 2023; Shao et al., 2021). These cytokines encouraged IVD cell phenotypic alterations and ECM degradation. Furthermore, the deteriorated disc’s production of chemokines encourages immune cell infiltration and activation, which intensifies the inflammatory cascade response. Inflammatory processes exacerbated by cytokines IL-1β and TNF-α are considered to be key mediators of IDD, and therefore, IL-1β and TNF-α are the most important pro-inflammatory cytokines (Wang Y. et al., 2020). According to recent research, the development of IDD is substantially correlated with the activation of NOD-like receptor thermal protein domain associated protein 3 (NLRP3) inflammatory vesicles (Chao-Yang et al., 2021). It has been reported that NLRP3 inflammatory vesicles promote the release of IL-1β, and in addition, IL-1β upregulated the initiation and activation of NLRP3 inflammatory vesicles by enhancing NF-κB signaling and mitochondrial reactive oxygen species (mtROS) production, which constitutes the NLRP3-IL-1β inflammatory loop (Chen et al., 2020b; Chao-Yang et al., 2021; Huang et al., 2021; Fu and Wu, 2023). Melatonin was found by Chen et al. to downregulate NF-κB signaling and mtROS generation, which in turn reduced the activation of NLRP3, IL-1β, and prevented the IL-1β-NLRP3 positive feedback loop in NPCs (Chen et al., 2020b). Nicotinamide phosphoribosyl transferase (NAMPT) is an intracellular enzyme with pro-inflammatory properties (Martínez-Morcillo et al., 2021; Audrito et al., 2020). According to previous studies, NAMPT was involved in the process of IDD, it could contribute to the pathogenesis of IDD (Shi et al., 2022; Yi et al., 2023). Huang et al. revealed that melatonin inhibits the activity of NLRP3 and NAMPT inflammasomes, which may mitigate the matrix degradation caused by TNF-α. Furthermore, by blocking NLRP3 inflammasome activity via MAPK and NF-κB signaling in NPCs, NAMPT downregulation inhibited TNF-α-induced matrix degradation (Huang et al., 2020). Macrophage (Mφ) was rapidly polarized to M1-type in the inflammatory state, and degenerating IVD cells could also secrete inflammatory mediators to activate Mφ, accompanied by a rise in pro-inflammatory factors (Nakazawa et al., 2018; Yang et al., 2019; Takada et al., 2004). Dou et al. showed that lipopolysaccharide (LPS) induced M1-type Mφ polarization with pro-inflammatory properties. Melatonin could suppress M1-type Mφ polarization and alleviate inflammation at the same time. Melatonin inhibited M1-type Mφ polarization and ameliorates inflammation-induced nasopharyngeal carcinoma injury (Dou et al., 2023b). Furthermore, Wu et al. discovered that lipopolysaccharide (LPS) stimulation caused damage to EP cartilaginous cells (EPCs). However, melatonin reduced inflammation and ECM degradation of EPCs via the nuclear factor Kappa-B pathway, lessening the detrimental effects of LPS on EP (Wu et al., 2021). Apart from NPCs and EPCs, Li et al. discovered that melatonin significantly decreased the ROS content and NF-κB pathway activity in TNF-α-treated AF cells. Additionally, the protein expression of p16 and p53, which indicate cellular senescence, was also decreased. These findings imply that melatonin inhibits AF cellular senescence by controlling the ROS/NF-κB pathway in an inflammatory setting (Li et al., 2021b). According to a clinical trial by Tian et al., melatonin supplementation raised plasma melatonin, which may have major therapeutic effects by reducing inflammation. Higher plasma melatonin was also linked to lower levels of IL-6 and TNF-α (Tian et al., 2021). Taken together, these effects attenuated inflammatory damage and prevented inflammation-mediated IDD by interfering with the production of pro-inflammatory cytokines, specifically IL-1β and TNF-α, and suppressing M1-type Mφ polarization.
4.2 Melatonin relieves oxidative stress
Oxidative stress has a high correlation with inflammation (Reuter et al., 2010). Inflammation results from oxidative stress, which is an imbalance between the generation of ROS and their removal by defense mechanisms. Numerous transcription factors can be activated by oxidative stress, which results in the differential expression of several genes involved in inflammatory pathways (Hussain et al., 2016).
ROS as a major performer of oxidative stress, is a class of unstable, highly reactive molecule (Feng et al., 2017; Nasto et al., 2013). It has been found that the progression of IDD is closely related to ROS accumulation and oxidative stress. Oxidative stress promoted apoptosis, triggered inflammatory response, and exacerbated ECM degradation, thereby exacerbated the IDD process (Wang Y. et al., 2023). Melatonin, a potent antioxidant, can reduce oxidative stress damage (Tan et al., 2015; Morvaridzadeh et al., 2020). ROS levels and malondialdehyde (MDA) as important indicators for assessing oxidative stress (Hu et al., 2021). He et al. revealed that melatonin maintains cell viability of NPCs under oxidative stress, leading to decrease in apoptosis rate, ROS levels and MDA, reducing damage from oxidative stress (He et al., 2018). Mesenchymal stem cells (MSCs) have become a novel treatment option in recent years because of their paracrine actions, capacity to develop into intervertebral disc cells, and ability to restore degraded cells (Bhujel et al., 2022; Vadalà et al., 2016). Mesenchymal stem cells from the nucleus pulposus were identified and grown by Risbud et al. (2007), offering a novel strategy for endogenous healing of degenerative disc degeneration. Nucleus pulposus mesenchymal stem cells (NPSC) were present in normal and degenerative NP tissues. NPSCs were better adapted to the hypoxic hypertonic microenvironment of degenerative discs than exogenous stem cells (Huang et al., 2019; Liu et al., 2019). Huang et al. revealed that accumulation of ROS in the intervertebral disc would cause to senescence and apoptosis of NPSCs, ultimately leading to irreversible disc degeneration. Melatonin could effectively alleviate oxidative stress-induced excessive apoptosis of NPSCs and mitochondrial dysfunction by activating the PI3K/Akt pathway (Huang et al., 2023). In conclusion, it can be said that melatonin prevented inflammation-mediated IDD by interfering with the production of pro-inflammatory cytokines like TNF-α and IL-1β. Additionally, melatonin is able to lower ROS levels and reduce oxidative stress damage. These findings suggest that melatonin may be a promising treatment for IDD.
4.3 Melatonin inhibits IVD cell death
IDD has a very complicated pathophysiology, and one of the main causes of the disease is the death of IVD cells, such as NPCs or EPCs, as a result of the apoptotic pathway being activated (Zhang XB. et al., 2021). Therefore, a viable therapeutic strategy for the therapy of IDD is the suppression of IVD cell death.
According to previous studies, melatonin had several antioxidant and anti-inflammatory effects. In addition to this, melatonin regulated apoptosis and autophagy (Fernández et al., 2015; Zhi et al., 2020; Wang L. et al., 2023). Chen et al. found that melatonin reversed the expression of apoptosis-associated proteins, such as cleaved caspase 3, cytochrome c, Bax, and Bcl-2, and prevented tert-butyl hydroperoxide (TBHP)-induced apoptosis in NPCs in a dose-dependent manner. Subsequent investigation revealed that melatonin prevented NPCs from dying by causing and reducing IDD via mitochondrial autophagy, offering a possible treatment for IDD (Chen et al., 2019). Extracellular regulatory protein kinases (ERK) signaling has been linked to apoptosis, senescence, migration, differentiation, and proliferation of cells (Sun et al., 2015). Ge et al. found that discovered that ERK1/2 activity was considerably elevated by melatonin above a 1 μM concentration, and furthermore, the addition of an ERK inhibitor nearly entirely reversed the effects of melatonin therapy, which prevented NPC apoptosis by cleaving cystatin-3, reducing Bax expression, and boosting Bcl-2. In addition, melatonin (<5 μM) preserved cell cycle arrest in NP cells while increasing the proportion of S-phase cells and lowering the G0/G1 population (Ge et al., 2019). While Zhang et al. was examining the function and mechanism of lncRNA MEG3 in melatonin-mediated NPC action, they discovered that the melatonin-regulated MEG3-miR-15a-5p-PGC-1α/SIRT1 pathway may prevent IL-1β-induced inflammation and NPC apoptosis (Zhang C. et al., 2023).
Previous studies have suggested that diabetes-related hyperglycemia may be one of the risk factors for IDD (Jin et al., 2023; Cannata et al., 2020; Alpantaki et al., 2019). Li et al. observed an increased apoptosis rate of NPCs in a high glucose culture environment compared to the normal environment. This increase was mainly characterized by increased expression of apoptosis marker proteins such as cleaved Caspase-3 and cleaved PARP. In addition, melatonin was found to inhibit apoptosis of NPCs in high glucose environment. Notably, the researchers looked at the protein expression of p-Akt to explore the potential involvement of the PI3K/Akt pathway in IDD. The findings revealed that, in comparison to control NPCs, high-sugar culture significantly decreased the protein expression of p-Akt, but that melatonin addition partially promoted the expression of p-Akt protein in NPCs cultured in medium supplemented with high sugar concentrations. Furthermore, the PI3K/Akt pathway inhibitor LY294002 was added to melatonin-treated NPCs to prevent the activation of this signaling pathway. The findings demonstrated a large rise in the mRNA expression of autophagy-related genes (Beclin-1, Atg3, and Atg5) and a significant drop in the mRNA expression of anti-apoptotic genes (Bcl-2). Pro-apoptotic genes (Bax and cystatin-3) showed the lowest level of mRNA expression. In conclusion, melatonin reduced apoptosis in NPCs by blocking excessive autophagy in high glucose cells via the PI3K/Akt pathway (Li et al., 2021a). Zhang et al. fond that melatonin therapy decreased the frequency of calcification and apoptosis in EPCs. Notably, melatonin increased autophagy and Sirt1 expression and activity in EPCs. The protective effects of melatonin on apoptosis and calcification were reversed when 3-methyladenine blocked autophagy. Conversely, the Sirt1 inhibitor EX-527 decreased both melatonin-induced autophagy and the protective effects of melatonin on calcification and cell death. This indicated that the Sirt1 autophagy pathway mediated the favorable effects of melatonin on EPCs (Zhang Z. et al., 2019). In conclusion, the above studies suggested that melatonin had protective effect on IVD cells, including NPCs, EPCs. Cysteine asparaginase activation and inflammatory vesicles cause cellular pyroptosis, a form of lytic programmed cell death.
In contrast to apoptosis, cellular pyroptosis is characterized by the rupture of the plasma membrane and the release of inflammatory mediators, which speeds up the ECM’s decomposition. According to recent research, as IDD worsens, NLRP3 inflammatory vesicle-mediated pyroptosis is triggered. Targeting cellular pyroptosis in IDD also revealed the ECM’s amazing remodeling capabilities and anti-inflammatory qualities, indicating that cellular pyroptosis plays a role in the IDD process (Luo et al., 2022). Zhao et al. discovered that melatonin protects cells from cellular death and ECM degradation by reducing EGR1-induced overproduction of DDX3X and activation of NLRP3 inflammatory vesicles (Zhao et al., 2024). Xie et al. discovered that while melatonin dramatically inhibited the activity of NLRP3 inflammatory vesicles and decreased pain behavior in a rat model of radiculopathy, NLRP3 inflammatory vesicles were raised in both a dorsal root ganglion model and a rat model of radiculopathy (Xie et al., 2021). In conclusion, there is some study potential for melatonin’s ability to prevent cellular pyroptosis.
According to previous studies, apoptosis and pyroptosis are one of the ways of cell death (Yan et al., 2021; Newton et al., 2024). Apart from apoptosis, ferroptosis has also been linked to IDD (Ohnishi et al., 2022). Ferroptosis is a recently discovered novel form of regulatory cell death (RCD) characterized by iron-dependent mechanisms and accumulation of lipid ROS. Ferroptosis has a high correlation with numerous degenerative illnesses, and its function in the pathogenesis of IDD has drawn more attention (Yan et al., 2021; Fan et al., 2023). In addition, SLC7A11, GPX4, ACSL4, and LPCAT3 are altered during ferroptosis and can be used as marker proteins to detect ferroptosis (Seibt et al., 2019; Chen et al., 2021; Cui et al., 2021; Liu J. et al., 2022). It was shown that in a model of oxidative stress NPCs induced by TBHP, the levels of ferroptosis marker proteins were altered, ferroptosis was observed in this model of NPCs, as evidenced by changes in the expression of PTGS2 and ACSL4 and a decrease in GPX4 and FTH, but the addition of ferroptosis inhibitors reversed these manifestations (Fan et al., 2023; Zhu et al., 2023). This suggested that ferroptosis may be involved in the process of IDD, therefore, studying the relationship between ferroptosis and IDD had become a new research topic. Dou et al. revealed that LPS-stimulated macrophages' conditioned medium (CM) produced a ferroptosis-like environment with alterations in ferroptosis marker proteins, such as downregulation of GPX4 and SLC7A11 levels but upregulation of ACSL4 and LPCAT3 levels, which encouraged damage to NPCs. Subsequently, melatonin subsequently attenuated the damage caused by CM to NPCs, in part because it blocked ferroptosis, as evidenced by the overexpression of GPX4 and SLC7A11 levels and the downregulation of ACSL4 and LPCAT3 levels. The ferroptosis-inducing agent erastin further reduced the protective effect of melatonin in NPCs, while ferritin inhibitor-1 (Fer-1) increased it. This finding highlights the role of ferroptosis in the pathophysiology of IDD and raises the possibility that melatonin could be used as a medication for the clinical treatment of IDD. Despite the fact that a number of ferroptosis-related molecules, including GPX4, SLC7A11, ACSL4, and LPCAT3, contribute to the reduction of NPCS injury through the use of melatonin, the study was restricted to cellular experiments and lacked precise and in-depth research on the mechanism of ferroptosis. Additionally, there were no studies involving higher concentrations of melatonin (Dou et al., 2023a). But research on melatonin and ferroptosis is still in its early stages, and many questions remain unanswered. The present research on ferroptosis in melatonin has just touched the surface of the findings; the precise mechanisms, target molecules, and related signaling pathways remain unknown. More research is needed to determine how melatonin, ferroptosis, and IDD are related (Figure 2).
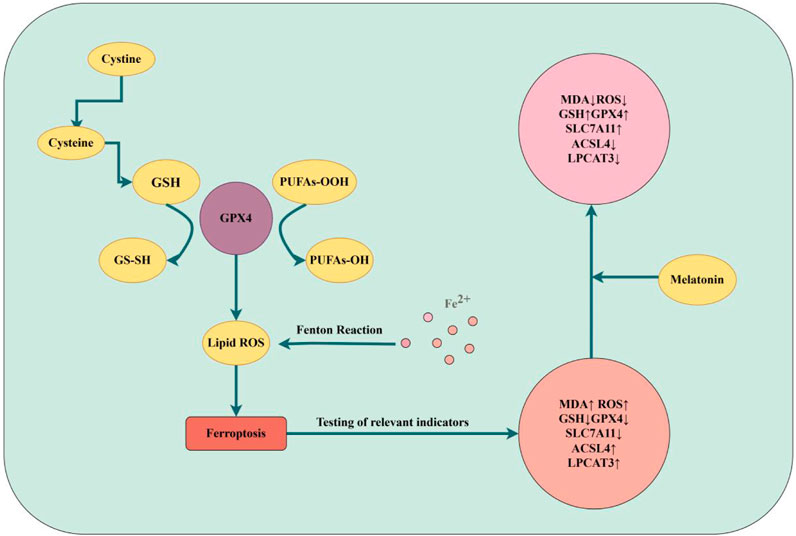
Figure 2. Ferroptosis of IDD (by Figdraw). Mechanisms of iron death and changes in the effects of melatonin on indicators of iron death are described.
4.4 Melatonin regulates ECM remodeling
The ECM’s both catabolism and anabolism are in a state of dynamic equilibrium during normal IVD. Disc degeneration typically happened when multiple stressors upset the balance (Liang et al., 2022; Le Maitre et al., 2007). Collagen II and aggregated proteoglycans are the primary constituents of ECM that preserve its elasticity and volume (Craddock et al., 2018). ECM-degrading enzymes, such as MMP-3, MMP-13, and ADAMTS-4, are upregulated in degenerative IVD specimens in proportion to the degree of degenerative alterations (Vo et al., 2013). Therefore, one of the most important aspects of treating IDD is to keep the ECM stable and avoid its disintegration.
It has been demonstrated that the PI3K/Akt signaling pathway contributes to IDD and that preventing ECM degradation can be achieved by activating this signaling system (Ouyang et al., 2017). By activating the PI3K/Akt pathway, IGF-1 dramatically reduced MMP-13 production and activity in rat endplate chondrocytes, increasing the amount of type II collagen (Zhang et al., 2009). Furthermore, by increasing Akt activity, IGF-1 and bone morphogenetic protein 7 treatment of bovine NP cells synergistically encouraged the buildup of aggregated proteoglycans (Zhang et al., 2009). To sum up, PI3K/Akt pathway activation is critical for reducing ECM degradation. Melatonin also has a role in the regulation of this signaling pathway, as reported by Li et al., melatonin interacts with the membrane receptor MT1/2, regulates the PI3K-Akt signaling pathway, and enhances the expression of Collagen II and aggregated proteoglycans in NPCs, and downregulates the expression of MMP-3 and MMP-9, all of which lead to remodeling of the extracellular matrix (Li et al., 2017). NF-κB pathways have long been recognized as a causative factor in IDD (Zhang GZ. et al., 2021), Chen et al. found that melatonin treatment of NPCs for 24 h resulted in a significant increase in the expression of type II collagen and aggregated proteoglycans in NPCs, with a corresponding decrease in the levels of ADAMTS-4, ADAMTS-5 and MMP-3, the underlying mechanism for this discovery, which was also confirmed in animal tests, may be that melatonin reduces ECM degradation by triggering autophagy via the NF-κB signaling pathway (Chen et al., 2020a). Furthermore, by decreasing melatonin receptor-mediated activation of the PI3K/AKT and NF-κB pathways, Liang et al. discovered that melatonin decreased NPCs catabolism and death (Liang et al., 2024).
Shen et al. discovered that melatonin facilitated the expression of markers associated with the extracellular matrix, such as COL2A1, ACAN, and SOX9. Meanwhile, cell proliferation in melatonin-treated group increased from day 3 (Shen et al., 2020). Zhang et al. discovered that melatonin downregulates matrix MMP-3 levels and increases the expression of collagen II and aggrecan to modify IL-1β-induced ECM remodeling (Zhang Y. et al., 2019). According to certain research, melatonin’s suppression of ECM disruption may be achieved via reducing oxidative stress, inflammation, and apoptosis as well as by influencing autophagy in IVD cells (Hemati et al., 2022).
When considered collectively, these findings imply that melatonin was a medication that preserved ECM homeostasis in IVD and offer fresh perspectives on treating.
5 New advances in drug delivery systems
Because of their lack of vascularization, IVD have a limited capacity to heal themselves after injury. Therefore, we need to find a new drug delivery system to better utilize the role of drugs in IVDs. Hydrogel injection, a minimally invasive technique, may represent a novel approach to regenerating or restoring the biological function and structure of IVD. In IVD tissue engineering, hydrogels are widely used due to their high biocompatibility, regulated degradation rates, and non-toxic breakdown products. IVD regeneration is a novel and promising strategy, according to Hu et al., who discovered that implantation of melatonin-containing hydrogels improved in situ regeneration of AF tissue and might be utilized to prevent IVD degeneration by maintaining hydration of rat NPs in a rat model of IVD abnormalities (Hu et al., 2023). Even though hydrogel can increase NPCs self-renewal and restore disc height, its poor mechanical qualities could prevent it from being used further (Liu et al., 2021). Wu et al. created the composite hydrogel Mel-MBG/SA, an injectable mesoporous bioactive glass/sodium alginate hydrogel loaded with melatonin. This system was validated in rats and offered a hybrid system with sustained melatonin release to reduce inflammation linked to IDD pathology and attenuate oxidative stress caused by IL-1β. successfully reduced rat tail inflammation.This method preserved their injectability and anti-inflammatory benefits while enhancing their mechanical qualities (Wu et al., 2023). In conclusion, melatonin and biomaterials science can be combined to improve drug delivery, but there aren't many studies on the topic. It can be used in a variety of ways in the future, including combining melatonin with hydrogels, melatonin with nanoparticles, and many more. Additionally, as artificial intelligence (AI) advances, it will be possible to use AI to predict drug-material interactions, optimize formulation design, and significantly improve drug delivery through multidisciplinary crossover.
6 Conclusions and prospects
IDD is the pathologic basis of degenerative spinal diseases and also one of the main causes of LBP. The pathological changes of IDD include ECM degradation, apoptosis of NPCs, and AF rupture. Mechanistically, IDD is associated with increased MMP in IVD, in addition to oxidative stress, involvement of inflammatory mediators, and activation of apoptotic pathways contribute to IDD. Melatonin is widely used for its ability to improve circadian rhythms and its low toxicity. Notably, it also has potent anti-inflammatory, antioxidant, and anti-apoptotic properties. Many studies have shown that melatonin slows the development of IDD through these pathways.
Notably, ferroptosis, as an emerging research hotspot, has been shown to be involved in the progression of IDD based on existing research evidence. It has been shown that melatonin regulates several iron death-related molecules, such as GPX4, SLC7A11, ACSL4 and LPCAT3, and reduces the damage of NPCs, but the exact and detailed mechanisms and pathways of iron death in this process are not fully understood. There are still several aspects of the specific link between melatonin and ferroptosis that need to be investigated in future studies, first, the sensitivity of NPCs to ferroptosis in the presence of different concentrations of melatonin. Second, the specific mechanisms and pathways between ferroptosis, IDD and melatonin. Third, validation by animal experiments is needed.
Although a large number of studies have shown that melatonin has a role in IDD, its specific clinical application still requires the following work. First, large-scale randomized controlled trials are needed to determine the optimal dose and mode of administration for the prevention or treatment of IDD. Second, detailed studies are needed to report the side effects, resistance to long-term administration and therapeutic effects of melatonin. In addition, the specific mechanism of melatonin’s modulating effect on IDD needs further in-depth study.
In the future research on melatonin and IDD, single-cell sequencing and proteomics technology can be combined to clarify the targeting mechanism of melatonin in different cell subpopulations of the intervertebral disc (e.g., NPCs and annulus fibrosus cells), and in the animal modelling, old animals (e.g., 16-month-old mice) are suitable for long-term melatonin intervention due to the natural degeneration close to the pathological process of human beings; moreover, the absence of vascular properties of the disc limits drug penetration and requires the development of a more penetrating nanocarrier, which can be combined with material science. Moreover, due to the absence of blood vessels in the intervertebral disc, it is necessary to develop nanocarriers with stronger penetration, which can be combined with material science. In conclusion, this review contributes to the understanding of the role of melatonin in IDD, describes the advantages of melatonin that may be used as a potential new treatment for IDD as well as the work needed as a conventional therapy, and helps to stimulate further research on melatonin and IDD (Figure 3).
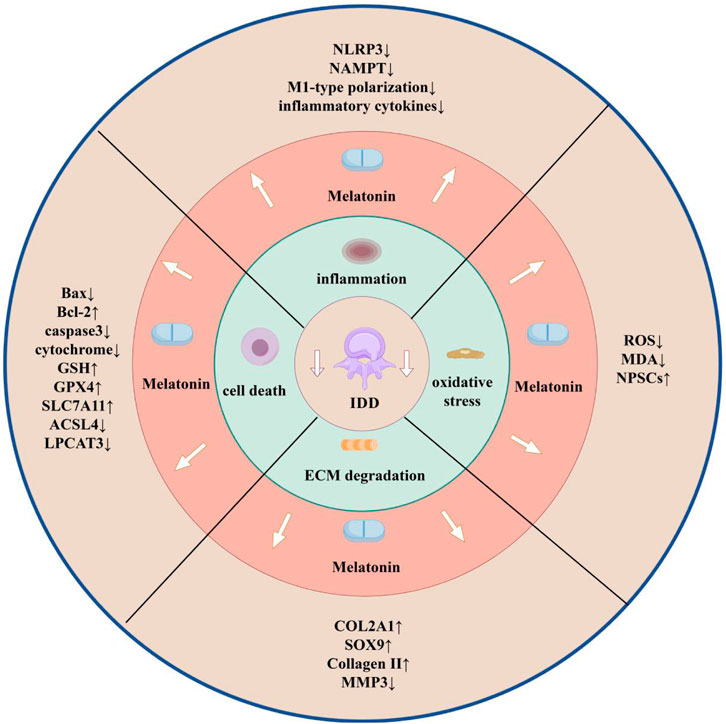
Figure 3. Melatonin and IDD (by Figdraw). Effects of melatonin on IDD through modulation of inflammation, oxidative stress, ECM degradation, and cell death, and changes in each indicator.
Author contributions
JYi: Writing – original draft, Writing – review and editing. LW: Writing – original draft, Writing – review and editing. KZ: Writing – original draft, Writing – review and editing. JYa: Writing – original draft, Writing – review and editing. ML: Writing – original draft, Writing – review and editing. MZ: Writing – original draft, Writing – review and editing. JL: Writing – original draft, Writing – review and editing.
Funding
The author(s) declare that financial support was received for the research and/or publication of this article. Key Research and Development Project in Henan Province (231111310500), Youth Science Award Project of the Provincial-level Joint Fund for Science and Technology Research and Development Project in Henan Province (225200810084), Henan Province Medical Science and Technology Project Joint Construction Project (LHGJ20230490), Scientific Research Project of Henan Zhongyuan Medical Science and Technology Innovation and Development Foundation (ZYYC2023ZD).
Conflict of interest
The authors declare that the research was conducted in the absence of any commercial or financial relationships that could be construed as a potential conflict of interest.
Generative AI statement
The authors declare that no Generative AI was used in the creation of this manuscript.
Publisher’s note
All claims expressed in this article are solely those of the authors and do not necessarily represent those of their affiliated organizations, or those of the publisher, the editors and the reviewers. Any product that may be evaluated in this article, or claim that may be made by its manufacturer, is not guaranteed or endorsed by the publisher.
References
Acosta J. C., O'Loghlen A., Banito A., Guijarro M. V., Augert A., Raguz S., et al. (2008). Chemokine signaling via the CXCR2 receptor reinforces senescence. Cell 133, 1006–1018. doi:10.1016/j.cell.2008.03.038
Ahmad S. B., Ali A., Bilal M., Rashid S. M., Wani A. B., Bhat R. R., et al. (2023). Melatonin and health: insights of melatonin action, biological functions, and associated disorders. Cell Mol. Neurobiol. 43 (6), 2437–2458. doi:10.1007/s10571-023-01324-w
Alpantaki K., Kampouroglou A., Koutserimpas C., Effraimidis G., Hadjipavlou A. (2019). Diabetes mellitus as a risk factor for intervertebral disc degeneration: a critical review. Eur. Spine J. 28 (9), 2129–2144. doi:10.1007/s00586-019-06029-7
Arioz B. I., Tastan B., Tarakcioglu E., Tufekci K. U., Olcum M., Ersoy N., et al. (2019). Melatonin attenuates LPS-induced acute depressive-like behaviors and microglial NLRP3 inflammasome activation through the SIRT1/nrf2 pathway. Front. Immunol. 10, 1511. doi:10.3389/fimmu.2019.01511
Audrito V., Messana V. G., Deaglio S. (2020). NAMPT and NAPRT: two metabolic enzymes with key roles in inflammation. Front. Oncol. 10, 358. doi:10.3389/fonc.2020.00358
Bhattacharya S., Patel K. K., Dehari D., Agrawal A. K., Singh S. (2019). Melatonin and its ubiquitous anticancer effects. Mol. Cell Biochem. 462 (1-2), 133–155. doi:10.1007/s11010-019-03617-5
Bhujel B., Shin H. E., Choi D. J., Han I. (2022). Mesenchymal stem cell-derived exosomes and intervertebral disc regeneration: review. Int. J. Mol. Sci. 23 (13), 7306. doi:10.3390/ijms23137306
Cajochen C., Kräuchi K., Wirz-Justice A. (2003). Role of melatonin in the regulation of human circadian rhythms and sleep. J. Neuroendocrinol. 15 (4), 432–437. doi:10.1046/j.1365-2826.2003.00989.x
Cannata F., Vadalà G., Ambrosio L., Fallucca S., Napoli N., Papalia R., et al. (2020). Intervertebral disc degeneration: a focus on obesity and type 2 diabetes. Diabetes Metab. Res. Rev. 36 (1), e3224. doi:10.1002/dmrr.3224
Chao-Yang G., Peng C., Hai-Hong Z. (2021). Roles of NLRP3 inflammasome in intervertebral disc degeneration. Osteoarthr. Cartil. 29 (6), 793–801. doi:10.1016/j.joca.2021.02.204
Chen F., Jiang G., Liu H., Li Z., Pei Y., Wang H., et al. (2020b). Melatonin alleviates intervertebral disc degeneration by disrupting the IL-1β/NF-κB-NLRP3 inflammasome positive feedback loop. Bone Res. 8, 10. doi:10.1038/s41413-020-0087-2
Chen F., Liu H., Wang X., Li Z., Zhang J., Pei Y., et al. (2020a). Melatonin activates autophagy via the NF-κB signaling pathway to prevent extracellular matrix degeneration in intervertebral disc. Osteoarthr. Cartil. 28, 1121–1132. doi:10.1016/j.joca.2020.05.011
Chen X., Li J., Kang R., Klionsky D. J., Tang D. (2021). Ferroptosis: machinery and regulation. Autophagy 17 (9), 2054–2081. doi:10.1080/15548627.2020.1810918
Chen X., Zhang A., Zhao K., Gao H., Shi P., Chen Y., et al. (2024). The role of oxidative stress in intervertebral disc degeneration: mechanisms and therapeutic implications. Ageing Res. Rev. 98, 102323. doi:10.1016/j.arr.2024.102323
Chen Y., Wu Y., Shi H., Wang J., Zheng Z., Chen J., et al. (2019). Melatonin ameliorates intervertebral disc degeneration via the potential mechanisms of mitophagy induction and apoptosis inhibition. J. Cell Mol. Med. 23, 2136–2148. doi:10.1111/jcmm.14125
Cheung K. M., Karppinen J., Chan D., Ho D. W., Song Y. Q., Sham P., et al. (2009). Prevalence and pattern of lumbar magnetic resonance imaging changes in a population study of one thousand forty-three individuals. Spine (Phila Pa 1976) 34, 934–940. doi:10.1097/BRS.0b013e3181a01b3f
Chu G., Shi C., Lin J., Wang S., Wang H., Liu T., et al. (2018). Biomechanics in annulus fibrosus degeneration and regeneration. Adv. Exp. Med. Biol. 1078, 409–420. doi:10.1007/978-981-13-0950-2_21
Clouet J., Vinatier C., Merceron C., Pot-Vaucel M., Hamel O., Weiss P., et al. (2009). The intervertebral disc: from pathophysiology to tissue engineering. Jt. Bone Spine 76, 614–618. doi:10.1016/j.jbspin.2009.07.002
Craddock R. J., Hodson N. W., Ozols M., Shearer T., Hoyland J. A., Sherratt M. J. (2018). Extracellular matrix fragmentation in young, healthy cartilaginous tissues. Eur. Cell Mater 35, 34–53. doi:10.22203/eCM.v035a04
Cui Y., Zhang Y., Zhao X., Shao L., Liu G., Sun C., et al. (2021). ACSL4 exacerbates ischemic stroke by promoting ferroptosis-induced brain injury and neuroinflammation. Brain Behav. Immun. 93, 312–321. doi:10.1016/j.bbi.2021.01.003
Diwan A. D., Melrose J. (2023). Intervertebral disc degeneration and how it leads to low back pain. JOR Spine 6, e1231. doi:10.1002/jsp2.1231
Dou X., Luo Q., Xie L., Zhou X., Song C., Liu M., et al. (2023b). Medical prospect of melatonin in the intervertebral disc degeneration through inhibiting M1-type macrophage polarization via SIRT1/notch signaling pathway. Biomedicines 11, 1615. doi:10.3390/biomedicines11061615
Dou X., Ma Y., Luo Q., Song C., Liu M., Liu X., et al. (2023a). Therapeutic potential of melatonin in the intervertebral disc degeneration through inhibiting the ferroptosis of nucleus pulpous cells. J. Cell Mol. Med. 27, 2340–2353. doi:10.1111/jcmm.17818
Esposito S., Laino D., D'Alonzo R., Mencarelli A., Di Genova L., Fattorusso A., et al. (2019). Pediatric sleep disturbances and treatment with melatonin. J. Transl. Med. 17 (1), 77. doi:10.1186/s12967-019-1835-1
Fan C., Chu G., Yu Z., Ji Z., Kong F., Yao L., et al. (2023). The role of ferroptosis in intervertebral disc degeneration. Front. Cell Dev. Biol. 11, 1219840. doi:10.3389/fcell.2023.1219840
Favero G., Moretti E., Bonomini F., Reiter R. J., Rodella L. F., Rezzani R. (2018). Promising antineoplastic actions of melatonin. Front. Pharmacol. 9, 1086. doi:10.3389/fphar.2018.01086
Feng C., Yang M., Lan M., Liu C., Zhang Y., Huang B., et al. (2017). ROS: crucial Intermediators in the pathogenesis of intervertebral disc degeneration. Oxid. Med. Cell Longev. 2017, 5601593. doi:10.1155/2017/5601593
Fernández A., Ordóñez R., Reiter R. J., González-Gallego J., Mauriz J. L. (2015). Melatonin and endoplasmic reticulum stress: relation to autophagy and apoptosis. J. Pineal Res. 59 (3), 292–307. doi:10.1111/jpi.12264
Fu J., Wu H. (2023). Structural mechanisms of NLRP3 inflammasome assembly and activation. Annu. Rev. Immunol. 41, 301–316. doi:10.1146/annurev-immunol-081022-021207
Gao W., Lin M., Liang A., Zhang L., Chen C., Liang G., et al. (2014). Melatonin enhances chondrogenic differentiation of human mesenchymal stem cells. J. Pineal Res. 56 (1), 62–70. doi:10.1111/jpi.12098
Ge J., Zhou Q., Niu J., Wang Y., Yan Q., Wu C., et al. (2019). Melatonin protects intervertebral disc from degeneration by improving cell survival and function via activation of the ERK1/2 signaling pathway. Oxid. Med. Cell Longev. 2019, 5120275. doi:10.1155/2019/5120275
Gruber H. E., Ingram J. A., Davis D. E., Hanley E. N. (2009). Increased cell senescence is associated with decreased cell proliferation in vivo in the degenerating human annulus. Spine J. 9, 210–215. doi:10.1016/j.spinee.2008.01.012
Hardeland R. (2019). Aging, melatonin, and the pro- and anti-inflammatory networks. Int. J. Mol. Sci. 20 (5), 1223. doi:10.3390/ijms20051223
Hardeland R., Cardinali D. P., Brown G. M., Pandi-Perumal S. R. (2015). Melatonin and brain inflammaging. Prog. Neurobiol. 127-128, 46–63. doi:10.1016/j.pneurobio.2015.02.001
Hartvigsen J., Hancock M. J., Kongsted A., Louw Q., Ferreira M. L., Genevay S., et al. (2018). What low back pain is and why we need to pay attention. Lancet 391, 2356–2367. doi:10.1016/S0140-6736(18)30480-X
He R., Cui M., Lin H., Zhao L., Wang J., Chen S., et al. (2018). Melatonin resists oxidative stress-induced apoptosis in nucleus pulposus cells. Life Sci. 199, 122–130. doi:10.1016/j.lfs.2018.03.020
Hemati K., Pourhanifeh M. H., Fatemi I., Hosseinzadeh A., Mehrzadi S. (2022). Anti-degenerative effect of melatonin on intervertebral disc: protective contribution against inflammation, oxidative stress, apoptosis, and autophagy. Curr. Drug Targets 23 (7), 711–718. doi:10.2174/1389450123666220114151654
Hirayama J., Hattori A., Takahashi A., Furusawa Y., Tabuchi Y., Shibata M., et al. (2023). Physiological consequences of space flight, including abnormal bone metabolism, space radiation injury, and circadian clock dysregulation: implications of melatonin use and regulation as a countermeasure. J. Pineal Res. 74 (1), e12834. doi:10.1111/jpi.12834
Hu S., Zhang C., Qian T., Bai Y., Chen L., Chen J., et al. (2021). Promoting nrf2/sirt3-dependent mitophagy suppresses apoptosis in nucleus pulposus cells and protects against intervertebral disc degeneration. Oxid. Med. Cell Longev. 2021, 6694964. doi:10.1155/2021/6694964
Hu X., Tian X., Yang C., Ling F., Liu H., Zhu X., et al. (2023). Melatonin-loaded self-healing hydrogel targets mitochondrial energy metabolism and promotes annulus fibrosus regeneration. Mater Today Bio 23, 100811. doi:10.1016/j.mtbio.2023.100811
Huang Y., Peng Y., Sun J., Li S., Hong J., Zhou J., et al. (2020). Nicotinamide phosphoribosyl transferase controls NLRP3 inflammasome activity through MAPK and NF-κB signaling in nucleus pulposus cells, as suppressed by melatonin. Inflammation 43 (3), 796–809. doi:10.1007/s10753-019-01166-z
Huang Y., Xu W., Zhou R. (2021). NLRP3 inflammasome activation and cell death. Cell Mol. Immunol. 18 (9), 2114–2127. doi:10.1038/s41423-021-00740-6
Huang Z., Cheng X., Zhao J., Liu Z., Wang J., Feng X., et al. (2019). Influence of simvastatin on the biological behavior of nucleus pulposus-derived mesenchymal stem cells. Iran. J. Basic Med. Sci. 22 (12), 1468–1475. doi:10.22038/IJBMS.2019.14068
Huang Z. N., Wang Z. Y., Cheng X. F., Huang Z. Z., Han Y. L., Cui Y. Z., et al. (2023). Melatonin alleviates oxidative stress-induced injury to nucleus pulposus-derived mesenchymal stem cells through activating PI3K/Akt pathway. J. Orthop. Transl. 43, 66–84. doi:10.1016/j.jot.2023.10.002
Hussain T., Tan B., Yin Y., Blachier F., Tossou M. C., Rahu N. (2016). Oxidative stress and inflammation: what polyphenols can do for us. Oxid. Med. Cell Longev. 2016, 7432797. doi:10.1155/2016/7432797
Jin P., Xing Y., Xiao B., Wei Y., Yan K., Zhao J., et al. (2023). Diabetes and intervertebral disc degeneration: a Mendelian randomization study. Front. Endocrinol. (Lausanne) 14, 1100874. doi:10.3389/fendo.2023.1100874
Kamali A., Ziadlou R., Lang G., Pfannkuche J., Cui S., Li Z., et al. (2021). Small molecule-based treatment approaches for intervertebral disc degeneration: current options and future directions. Theranostics 11, 27–47. doi:10.7150/thno.48987
Krupkova O., Sekiguchi M., Klasen J., Hausmann O., Konno S., Ferguson S. J., et al. (2014). Epigallocatechin 3-gallate suppresses interleukin-1β-induced inflammatory responses in intervertebral disc cells in vitro and reduces radiculopathic pain in rats. Eur. Cell Mater 28, 372–386. doi:10.22203/ecm.v028a26
Le Maitre C. L., Pockert A., Buttle D. J., Freemont A. J., Hoyland J. A. (2007). Matrix synthesis and degradation in human intervertebral disc degeneration. Biochem. Soc. Trans. 35, 652–655. doi:10.1042/BST0350652
Li F., Sun X., Zheng B., Sun K., Zhu J., Ji C., et al. (2021c). Arginase II promotes intervertebral disc degeneration through exacerbating senescence and apoptosis caused by oxidative stress and inflammation via the NF-κB pathway. Front. Cell Dev. Biol. 9, 737809. doi:10.3389/fcell.2021.737809
Li H., Wang X., Pan H., Xiao C., Wang C., Guo S., et al. (2023b). The mechanisms and functions of IL-1β in intervertebral disc degeneration. Exp. Gerontol. 177, 112181. doi:10.1016/j.exger.2023.112181
Li J., Li J., Cao C., Sun J., Wang S., Ruan Z., et al. (2021b). Melatonin inhibits annulus fibrosus cell senescence through regulating the ROS/NF-κB pathway in an inflammatory environment. Biomed. Res. Int. 2021, 3456321. doi:10.1155/2021/3456321
Li J., Wang C., Xue L., Zhang F., Liu J. (2021a). Melatonin suppresses apoptosis of nucleus pulposus cells through inhibiting autophagy via the PI3K/Akt pathway in a high-glucose culture. Biomed. Res. Int. 2021, 4604258. doi:10.1155/2021/4604258
Li S., Si H., Xu J., Liu Y., Shen B. (2022). The therapeutic effect and mechanism of melatonin on osteoarthritis: from the perspective of non-coding RNAs. Front. Genet. 13, 968919. doi:10.3389/fgene.2022.968919
Li T., Jiang S., Lu C., Yang W., Yang Z., Hu W., et al. (2019). Melatonin: another avenue for treating osteoporosis. J. Pineal Res. 66 (2), e12548. doi:10.1111/jpi.12548
Li Z., Li X., Chen C., Chan M., Wu W., Shen J. (2017). Melatonin inhibits nucleus pulposus (NP) cell proliferation and extracellular matrix (ECM) remodeling via the melatonin membrane receptors mediated PI3K-Akt pathway. J. Pineal Res. 63 (3). doi:10.1111/jpi.12435
Li Z., Yang H., Hai Y., Cheng Y. (2023a). Regulatory effect of inflammatory mediators in intervertebral disc degeneration. Mediat. Inflamm. 2023, 6210885. doi:10.1155/2023/6210885
Liang H., Luo R., Li G., Zhang W., Song Y., Yang C. (2022). The Proteolysis of ECM in intervertebral disc degeneration. Int. J. Mol. Sci. 23, 1715. doi:10.3390/ijms23031715
Liang Z. H., Song J., Shangguan W. J., Zhang Q. Q., Shao J., Zhang Y. H. (2024). Melatonin mitigates matrix stiffness-induced intervertebral disk degeneration by inhibiting reactive oxygen species and melatonin receptors mediated PI3K/AKT/NF-κB pathway. Am. J. Physiol. Cell Physiol. 327, C1236–C1248. doi:10.1152/ajpcell.00630.2023
Liu J., Kang R., Tang D. (2022b). Signaling pathways and defense mechanisms of ferroptosis. FEBS J. 289 (22), 7038–7050. doi:10.1111/febs.16059
Liu J., Wang D., Li Y., Zhou Z., Zhang D., Li J., et al. (2021). Overall structure construction of an intervertebral disk based on highly anisotropic wood hydrogel composite materials with mechanical matching and buckling buffering. ACS Appl. Mater Interfaces 13, 15709–15719. doi:10.1021/acsami.1c02487
Liu M. Y., Zhang L., Zang W. D., Zhang K. G., Li H. J., Gao Y. Z. (2022a). Pharmacological effects of resveratrol in intervertebral disc degeneration: a literature review. Orthop. Surg. 14, 3141–3149. doi:10.1111/os.13560
Liu Y., Li Y., Huang Z. N., Wang Z. Y., Nan L. P., Wang F., et al. (2019). The effect of intervertebral disc degenerative change on biological characteristics of nucleus pulposus mesenchymal stem cell: an in vitro study in rats. Connect. Tissue Res. 60 (4), 376–388. doi:10.1080/03008207.2019.1570168
Luo J., Yang Y., Wang X., Chang X., Fu S. (2022). Role of pyroptosis in intervertebral disc degeneration and its therapeutic implications. Biomolecules 12, 1804. doi:10.3390/biom12121804
Martínez-Morcillo F. J., Cantón-Sandoval J., Martínez-Menchón T., Corbalán-Vélez R., Mesa-Del-Castillo P., Pérez-Oliva A. B., et al. (2021). Non-canonical roles of NAMPT and PARP in inflammation. Dev. Comp. Immunol. 115, 103881. doi:10.1016/j.dci.2020.103881
Morvaridzadeh M., Sadeghi E., Agah S., Nachvak S. M., Fazelian S., Moradi F., et al. (2020). Effect of melatonin supplementation on oxidative stress parameters: a systematic review and meta-analysis. Pharmacol. Res. 161, 105210. doi:10.1016/j.phrs.2020.105210
Nakazawa K. R., Walter B. A., Laudier D. M., Krishnamoorthy D., Mosley G. E., Spiller K. L., et al. (2018). Accumulation and localization of macrophage phenotypes with human intervertebral disc degeneration. Spine J. 18 (2), 343–356. doi:10.1016/j.spinee.2017.09.018
Nasto L. A., Robinson A. R., Ngo K., Clauson C. L., Dong Q., St Croix C., et al. (2013). Mitochondrial-derived reactive oxygen species (ROS) play a causal role in aging-related intervertebral disc degeneration. J. Orthop. Res. 31 (7), 1150–1157. doi:10.1002/jor.22320
Newton K., Strasser A., Kayagaki N., Dixit V. M. (2024). Cell death. Cell 187 (2), 235–256. doi:10.1016/j.cell.2023.11.044
Ohnishi T., Iwasaki N., Sudo H. (2022). Causes of and molecular targets for the treatment of intervertebral disc degeneration: a review. Cells 11 (3), 394. doi:10.3390/cells11030394
Ouyang Z. H., Wang W. J., Yan Y. G., Wang B., Lv G. H. (2017). The PI3K/Akt pathway: a critical player in intervertebral disc degeneration. Oncotarget 8, 57870–57881. doi:10.18632/oncotarget.18628
Pan Z., Sun H., Xie B., Xia D., Zhang X., Yu D., et al. (2018). Therapeutic effects of gefitinib-encapsulated thermosensitive injectable hydrogel in intervertebral disc degeneration. Biomaterials 160, 56–68. doi:10.1016/j.biomaterials.2018.01.016
Pévet P. (2016). Melatonin receptors as therapeutic targets in the suprachiasmatic nucleus. Expert Opin. Ther. Targets 20 (10), 1209–1218. doi:10.1080/14728222.2016.1179284
Phan K., Nazareth A., Hussain A. K., Dmytriw A. A., Nambiar M., Nguyen D., et al. (2021). Correction to: relationship between sagittal balance and adjacent segment disease in surgical treatment of degenerative lumbar spine disease: meta-analysis and implications for choice of fusion technique. Eur. Spine J. 30, 3774. doi:10.1007/s00586-021-07000-1
Qiu X., Liang T., Wu Z., Zhu Y., Gao W., Gao B., et al. (2022). Melatonin reverses tumor necrosis factor-alpha-induced metabolic disturbance of human nucleus pulposus cells via MTNR1B/Gαi2/YAP signaling. Int. J. Biol. Sci. 18, 2202–2219. doi:10.7150/ijbs.65973
Reiter R. J., Tan D. X., Rosales-Corral S., Galano A., Zhou X. J., Xu B. (2018). Mitochondria: central organelles for melatonin's antioxidant and anti-aging actions. Molecules 23 (2), 509. doi:10.3390/molecules23020509
Reiter R. J., Tan D. X., Sainz R. M., Mayo J. C., Lopez-Burillo S. (2002). Melatonin: reducing the toxicity and increasing the efficacy of drugs. J. Pharm. Pharmacol. 54 (10), 1299–1321. doi:10.1211/002235702760345374
Reuter S., Gupta S. C., Chaturvedi M. M., Aggarwal B. B. (2010). Oxidative stress, inflammation, and cancer: how are they linked. Free Radic. Biol. Med. 49 (11), 1603–1616. doi:10.1016/j.freeradbiomed.2010.09.006
Risbud M. V., Guttapalli A., Tsai T. T., Lee J. Y., Danielson K. G., Vaccaro A. R., et al. (2007). Evidence for skeletal progenitor cells in the degenerate human intervertebral disc. Spine (Phila Pa 1976) 32 (23), 2537–2544. doi:10.1097/BRS.0b013e318158dea6
Risbud M. V., Shapiro I. M. (2014). Role of cytokines in intervertebral disc degeneration: pain and disc content. Nat. Rev. Rheumatol. 10, 44–56. doi:10.1038/nrrheum.2013.160
Roberts S., Caterson B., Menage J., Evans E. H., Jaffray D. C., Eisenstein S. M. (2000). Matrix metalloproteinases and aggrecanase: their role in disorders of the human intervertebral disc. Spine (Phila Pa 1976) 25, 3005–3013. doi:10.1097/00007632-200012010-00007
Schomerus C., Korf H. W. (2005). Mechanisms regulating melatonin synthesis in the mammalian pineal organ. Ann. N. Y. Acad. Sci. 1057, 372–383. doi:10.1196/annals.1356.028
Seibt T. M., Proneth B., Conrad M. (2019). Role of GPX4 in ferroptosis and its pharmacological implication. Free Radic. Biol. Med. 133, 144–152. doi:10.1016/j.freeradbiomed.2018.09.014
Shao Z., Wang B., Shi Y., Xie C., Huang C., Chen B., et al. (2021). Senolytic agent Quercetin ameliorates intervertebral disc degeneration via the Nrf2/NF-κB axis. Osteoarthr. Cartil. 29 (3), 413–422. doi:10.1016/j.joca.2020.11.006
Shen C., Li Y., Chen Y., Huang L., Zhang F., Wu W. (2020). Melatonin prevents the binding of vascular endothelial growth factor to its receptor and promotes the expression of extracellular matrix-associated genes in nucleus pulposus cells. Exp. Ther. Med. 20 (5), 106. doi:10.3892/etm.2020.9227
Shen S., Liao Q., Wong Y. K., Chen X., Yang C., Xu C., et al. (2022). The role of melatonin in the treatment of type 2 diabetes mellitus and Alzheimer's disease. Int. J. Biol. Sci. 18 (3), 983–994. doi:10.7150/ijbs.66871
Shi Q., Xu L., Zeng X. (2022). Sirtuin 1 participates in intervertebral disc degeneration via the nicotinamide phosphoribosyl transferase/nicotinamide adenine dinucleotide/sirtuin 1 pathway responsible for regulating autophagy of nucleus pulposus cells. Exp. Ther. Med. 23 (4), 267. doi:10.3892/etm.2022.11193
Sumsuzzman D. M., Choi J., Jin Y., Hong Y. (2021). Neurocognitive effects of melatonin treatment in healthy adults and individuals with Alzheimer's disease and insomnia: a systematic review and meta-analysis of randomized controlled trials. Neurosci. Biobehav Rev. 127, 459–473. doi:10.1016/j.neubiorev.2021.04.034
Sun Y., Liu W. Z., Liu T., Feng X., Yang N., Zhou H. F. (2015). Signaling pathway of MAPK/ERK in cell proliferation, differentiation, migration, senescence and apoptosis. J. Recept Signal Transduct. Res. 35 (6), 600–604. doi:10.3109/10799893.2015.1030412
Takada T., Nishida K., Doita M., Miyamoto H., Kurosaka M. (2004). Interleukin-6 production is upregulated by interaction between disc tissue and macrophages. Spine (Phila Pa 1976) 29 (10), 1089–1093. doi:10.1097/00007632-200405150-00007
Takahashi J., Shono Y., Hirabayashi H., Kamimura M., Nakagawa H., Ebara S., et al. (2006). Usefulness of white blood cell differential for early diagnosis of surgical wound infection following spinal instrumentation surgery. Spine (Phila Pa 1976) 31, 1020–1025. doi:10.1097/01.brs.0000214895.67956.60
Talib W. H. (2018). Melatonin and cancer hallmarks. Molecules 23 (3), 518. doi:10.3390/molecules23030518
Tamtaji O. R., Reiter R. J., Alipoor R., Dadgostar E., Kouchaki E., Asemi Z. (2020). Melatonin and Parkinson disease: current status and future perspectives for molecular mechanisms. Cell Mol. Neurobiol. 40 (1), 15–23. doi:10.1007/s10571-019-00720-5
Tan D. X., Manchester L. C., Esteban-Zubero E., Zhou Z., Reiter R. J. (2015). Melatonin as a potent and inducible endogenous antioxidant: synthesis and metabolism. Molecules 20 (10), 18886–18906. doi:10.3390/molecules201018886
Tian Y., Ji Y., Mei X., Pan J., He W., Sun J., et al. (2021). Lower plasma melatonin in the intervertebral disk degeneration patients was associated with increased proinflammatory cytokines. Clin. Interv. Aging 16, 215–224. doi:10.2147/CIA.S290045
Torre O. M., Mroz V., Bartelstein M. K., Huang A. H., Iatridis J. C. (2019). Annulus fibrosus cell phenotypes in homeostasis and injury: implications for regenerative strategies. Ann. N. Y. Acad. Sci. 1442 (1), 61–78. doi:10.1111/nyas.13964
Vadalà G., Russo F., Ambrosio L., Papalia R., Denaro V. (2016). Mesenchymal stem cells for intervertebral disc regeneration. J. Biol. Regul. Homeost. Agents 30 (4 Suppl. 1), 173–179.
Vasey C., McBride J., Penta K. (2021). Circadian rhythm dysregulation and restoration: the role of melatonin. Nutrients 13 (10), 3480. doi:10.3390/nu13103480
Vine T., Brown G. M., Frey B. N. (2022). Melatonin use during pregnancy and lactation: a scoping review of human studies. Braz J. Psychiatry 44 (3), 342–348. doi:10.1590/1516-4446-2021-2156
Violante F. S., Mattioli S., Bonfiglioli R. (2015). Low-back pain. Handb. Clin. Neurol. 131, 397–410. doi:10.1016/B978-0-444-62627-1.00020-2
Vo N. V., Hartman R. A., Yurube T., Jacobs L. J., Sowa G. A., Kang J. D. (2013). Expression and regulation of metalloproteinases and their inhibitors in intervertebral disc aging and degeneration. Spine J. 13, 331–341. doi:10.1016/j.spinee.2012.02.027
Wang D., Peng P., Dudek M., Hu X., Xu X., Shang Q., et al. (2022a). Restoring the dampened expression of the core clock molecule BMAL1 protects against compression-induced intervertebral disc degeneration. Bone Res. 10, 20. doi:10.1038/s41413-022-00187-z
Wang J., Xia D., Lin Y., Xu W., Wu Y., Chen J., et al. (2022b). Oxidative stress-induced circKIF18A downregulation impairs MCM7-mediated anti-senescence in intervertebral disc degeneration. Exp. Mol. Med. 54, 285–297. doi:10.1038/s12276-022-00732-0
Wang L., Wang C., Li X., Tao Z., Zhu W., Su Y., et al. (2023b). Melatonin and erastin emerge synergistic anti-tumor effects on oral squamous cell carcinoma by inducing apoptosis, ferroptosis, and inhibiting autophagy through promoting ROS. Cell Mol. Biol. Lett. 28 (1), 36. doi:10.1186/s11658-023-00449-6
Wang S. Y., Shi X. C., Laborda P. (2020a). Indole-based melatonin analogues: Synthetic approaches and biological activity. Eur. J. Med. Chem. 185, 111847. doi:10.1016/j.ejmech.2019.111847
Wang Y., Che M., Xin J., Zheng Z., Li J., Zhang S. (2020b). The role of IL-1β and TNF-α in intervertebral disc degeneration. Biomed. Pharmacother. 131, 110660. doi:10.1016/j.biopha.2020.110660
Wang Y., Cheng H., Wang T., Zhang K., Zhang Y., Kang X. (2023a). Oxidative stress in intervertebral disc degeneration: molecular mechanisms, pathogenesis and treatment. Cell Prolif. 56 (9), e13448. doi:10.1111/cpr.13448
Wu P. H., Kim H. S., Jang I. T. (2020). Intervertebral disc diseases part 2: a review of the current diagnostic and treatment strategies for intervertebral disc disease. Int. J. Mol. Sci. 21, 2135. doi:10.3390/ijms21062135
Wu R., Huang L., Xia Q., Liu Z., Huang Y., Jiang Y., et al. (2023). Injectable mesoporous bioactive glass/sodium alginate hydrogel loaded with melatonin for intervertebral disc regeneration. Mater Today Bio 22, 100731. doi:10.1016/j.mtbio.2023.100731
Wu X., Liu Y., Du J., Li X., Lin J., Ni L., et al. (2021). Melatonin attenuates intervertebral disk degeneration via maintaining cartilaginous endplate integrity in rats. Front. Physiol. 12, 672572. doi:10.3389/fphys.2021.672572
Xie L., Zhao Z., Chen Z., Ma X., Xia X., Wang H., et al. (2021). Melatonin alleviates radiculopathy against apoptosis and NLRP3 inflammasomes via the parkin-mediated mitophagy pathway. Spine (Phila Pa 1976) 46, E859–E868. doi:10.1097/BRS.0000000000003942
Xin J., Wang Y., Zheng Z., Wang S., Na S., Zhang S. (2022). Treatment of intervertebral disc degeneration. Orthop. Surg. 14, 1271–1280. doi:10.1111/os.13254
Yan H. F., Zou T., Tuo Q. Z., Xu S., Li H., Belaidi A. A., et al. (2021). Ferroptosis: mechanisms and links with diseases. Signal Transduct. Target Ther. 6 (1), 49. doi:10.1038/s41392-020-00428-9
Yang H., Liu B., Liu Y., He D., Xing Y., An Y., et al. (2019). Secreted factors from intervertebral disc cells and infiltrating macrophages promote degenerated intervertebral disc catabolism. Spine (Phila Pa 1976) 44 (9), E520–E529. doi:10.1097/BRS.0000000000002953
Yi J., Zhou Q., Huang J., Niu S., Ji G., Zheng T. (2023). Lipid metabolism disorder promotes the development of intervertebral disc degeneration. Biomed. Pharmacother. 166, 115401. doi:10.1016/j.biopha.2023.115401
Yurube T., Takeoka Y., Kanda Y., Kuroda R., Kakutani K. (2023). Intervertebral disc cell fate during aging and degeneration: apoptosis, senescence, and autophagy. N. Am. Spine Soc. J. 14, 100210. doi:10.1016/j.xnsj.2023.100210
Zhang C., Qiu Y., Yuan F. (2023b). The long non-coding RNA maternally expressed 3-micorRNA-15a-5p axis is modulated by melatonin and prevents nucleus pulposus cell inflammation and apoptosis. Basic Clin. Pharmacol. Toxicol. 133, 603–619. doi:10.1111/bcpt.13939
Zhang G. Z., Liu M. Q., Chen H. W., Wu Z. L., Gao Y. C., Ma Z. J., et al. (2021b). NF-κB signalling pathways in nucleus pulposus cell function and intervertebral disc degeneration. Cell Prolif. 54, e13057. doi:10.1111/cpr.13057
Zhang K., Wang H., Mo L., Huang X., Yuan C., Liu C. (2024). Melatonin attenuates degenerative disc degression by downregulating DLX5 via the TGF/Smad2/3 pathway in nucleus pulposus cells. JOR Spine 7, e70014. doi:10.1002/jsp2.70014
Zhang L., Su P., Xu C., Chen C., Liang A., Du K., et al. (2010). Melatonin inhibits adipogenesis and enhances osteogenesis of human mesenchymal stem cells by suppressing PPARγ expression and enhancing Runx2 expression. J. Pineal Res. 49 (4), 364–372. doi:10.1111/j.1600-079X.2010.00803.x
Zhang M., Zhou Q., Liang Q. Q., Li C. G., Holz J. D., Tang D., et al. (2009). IGF-1 regulation of type II collagen and MMP-13 expression in rat endplate chondrocytes via distinct signaling pathways. Osteoarthr. Cartil. 17, 100–106. doi:10.1016/j.joca.2008.05.007
Zhang W., Wang X., Tang Y., Huang C. (2023a). Melatonin alleviates doxorubicin-induced cardiotoxicity via inhibiting oxidative stress, pyroptosis and apoptosis by activating Sirt1/Nrf2 pathway. Biomed. Pharmacother. 162, 114591. doi:10.1016/j.biopha.2023.114591
Zhang X. B., Hu Y. C., Cheng P., Zhou H. Y., Chen X. Y., Wu D., et al. (2021a). Targeted therapy for intervertebral disc degeneration: inhibiting apoptosis is a promising treatment strategy. Int. J. Med. Sci. 18 (13), 2799–2813. doi:10.7150/ijms.59171
Zhang Y., He F., Chen Z., Su Q., Yan M., Zhang Q., et al. (2019a). Melatonin modulates IL-1β-induced extracellular matrix remodeling in human nucleus pulposus cells and attenuates rat intervertebral disc degeneration and inflammation. Aging (Albany NY) 11, 10499–10512. doi:10.18632/aging.102472
Zhang Y., Liu T., Yang H., He F., Zhu X. (2022). Melatonin: a novel candidate for the treatment of osteoarthritis. Ageing Res. Rev. 78, 101635. doi:10.1016/j.arr.2022.101635
Zhang Z., Lin J., Tian N., Wu Y., Zhou Y., Wang C., et al. (2019b). Melatonin protects vertebral endplate chondrocytes against apoptosis and calcification via the Sirt1-autophagy pathway. J. Cell Mol. Med. 23, 177–193. doi:10.1111/jcmm.13903
Zhao K., Zhang Y., Liao Z., Zhang W., Li G., Shi P., et al. (2024). Melatonin mitigates intervertebral disc degeneration by suppressing NLRP3 inflammasome activation via the EGR1/DDX3X pathway. FASEB J. 38, e70143. doi:10.1096/fj.202302453RRR
Zhi S. M., Fang G. X., Xie X. M., Liu L. H., Yan J., Liu D. B., et al. (2020). Melatonin reduces OGD/R-induced neuron injury by regulating redox/inflammation/apoptosis signaling. Eur. Rev. Med. Pharmacol. Sci. 24 (3), 1524–1536. doi:10.26355/eurrev_202002_20211
Zhou R., Tardivel A., Thorens B., Choi I., Tschopp J. (2010). Thioredoxin-interacting protein links oxidative stress to inflammasome activation. Nat. Immunol. 11, 136–140. doi:10.1038/ni.1831
Zhu H., Sun B., Shen Q. (2019). TNF-α induces apoptosis of human nucleus pulposus cells via activating the TRIM14/NF-κB signalling pathway. Artif. Cells Nanomed Biotechnol. 47, 3004–3012. doi:10.1080/21691401.2019.1643733
Zhu J., Sun R., Sun K., Yan C., Jiang J., Kong F., et al. (2023). The deubiquitinase USP11 ameliorates intervertebral disc degeneration by regulating oxidative stress-induced ferroptosis via deubiquitinating and stabilizing Sirt3. Redox Biol. 62, 102707. doi:10.1016/j.redox.2023.102707
Keywords: intervertebral disc degeneration, melatonin, oxidative stress, low back pain, inflammation, cell death
Citation: Yin J, Wan L, Zhang K, Yang J, Liu M, Zhao M and Li J (2025) Progress of melatonin in the treatment of intervertebral disc degeneration. Front. Physiol. 16:1529315. doi: 10.3389/fphys.2025.1529315
Received: 08 February 2025; Accepted: 23 April 2025;
Published: 14 May 2025.
Edited by:
Liang Kang, First Affiliated Hospital of Anhui Medical University, ChinaReviewed by:
Miaoheng Yan, First Affiliated Hospital of Zhengzhou University, ChinaHuiwen Pang, University of Queensland, Australia
Copyright © 2025 Yin, Wan, Zhang, Yang, Liu, Zhao and Li. This is an open-access article distributed under the terms of the Creative Commons Attribution License (CC BY). The use, distribution or reproduction in other forums is permitted, provided the original author(s) and the copyright owner(s) are credited and that the original publication in this journal is cited, in accordance with accepted academic practice. No use, distribution or reproduction is permitted which does not comply with these terms.
*Correspondence: Mingyu Zhao, em15eW0yMDAzQDE2My5jb20=; Jitian Li, aml0aWFubGVlQGhvdG1haWwuY29t
†These authors have contributed equally to this work and share first authorship