- China Volleyball College, Beijing Sport University, Beijing, China
Background: Older adults are experiencing a gradual decline in physical function as they age. Previous studies have shown that blood flow restriction (BFR) combined with low-intensity training can improve muscle strength and maintain cardiovascular fitness in older adults. However, it remains unclear which training regimen is the most effective. This study aims to investigate the effects of different BFR regimens combined with low-intensity training on muscle strength and cardiovascular safety in older adults.
Method: PubMed, Web of Science, Embase, the Cochrane Library, Scopus, EBSCOhost, and CNKI were searched up to March 2025 to select eligible studies. The randomized controlled trials that explored the effects of BFR training on muscle strength and cardiovascular safety in older adults were included.
Results: A total of 18 studies with 626 participants were included. The results of this network meta-analysis showed that: 1) in terms of improving muscle strength: compared to the controls, low-frequency, low-pressure, and low-intensity BFR training regimen was significantly related to one-repetition maximum (1RM) strength [weighted mean difference (WMD) = 0.58, 95% CI: 0.81–1.08 P < 0.05]. Moreover, high-frequency, high-pressure, and low-intensity BFR training was associated with increased muscle cross-sectional area [WMD = 0.50,95% CI (−0.10,1.11), P > 0.05] and isometric muscle strength [WMD = 1.44,95% CI (0.75,2.12), P < 0.05]; 2) in terms of cardiovascular health: compared to the controls, BFR training regimens at different pressures and frequencies were not linked to changes in heart rate in older adults (P > 0.05). Moreover, low-frequency, low-pressure, and low-intensity BFR training regimen was associated with increased systolic blood pressure [WMD = 3.40, 95%CI (0.61,6.19), P < 0.05] and diastolic blood pressure [WMD = 13.40, 95%CI (8.96,17.84), P < 0.05] in older adults.
Conclusion: Based on the results, high-frequency, high-pressure, and low-intensity BFR training may serve as the optimal regimen to improve muscle strength and maintain cardiovascular fitness in older adults.Clinical Trial Registration: https://www.crd.york.ac.uk/PROSPERO/, Registration and protocol CRD42024534387.
1 Introduction
In 2024, the World Health Organisation (WHO) stated that the global ageing population continued to rise, with individuals aged 60 and over expected to reach one-sixth of the world’s population by 2030. By 2050, people aged 60 and older will double worldwide (2.1 billion). Ageing mainly impacts the muscular and cardiovascular systems. In terms of the muscular system, ageing leads to a loss of skeletal muscle mass, strength, and fibre size (Grevendonk et al., 2021). Regarding the cardiovascular system, ageing causes a reduction in the ejection fraction and the elasticity of blood vessels (Vakka et al., 2023). The loss of muscle strength and decline in cardiovascular function increase the risk of developing chronic disease in older adults (Zuo et al., 2023). Studies have demonstrated that the prevalence of chronic diseases in older people is significantly higher than in adults, with a prevalence of 81.1 percent in people aged 60 and over (Xiang et al., 2024). Failure to prevent these diseases in older adults will impact their health and cause higher healthcare costs. Training interventions stand as one of the main options to lower the risk of development of these chronic diseases (Vikberg et al., 2019; Zhu et al., 2019). Among the training interventions, traditional resistance training, aerobic exercise (Hughes et al., 2018), and balance exercise (Posadzki et al., 2020) have all been shown to have positive effects on muscle growth. However, these training modalities often require participants to perform high-intensity training with a load value of 70%–80% of 1RM strength for a prolonged period of time (Sharp et al., 2022). Since older adults have lower training tolerance and a higher risk of injury and developing diseases compared to younger adults, their ability to do high-intensity training is limited (Xu et al., 2024). Therefore, the exploration of a training modality that is effective in increasing muscle strength and volume, as well as suitable for the older population is required in medical research.
Blood flow restriction (BFR), also known as KAASTU, is a method of restricting blood flow to proximal muscles by applying a pressure band to the distal limb (Wilkinson et al., 2019). A study has shown that BFR techniques contribute to creating an exercise stimulus. By combining multiple exercise methods, these techniques can enhance body adaptation (Bielitzki et al., 2021). The combination of BFR training with low-intensity muscle contraction exercise (20%–30% of 1RM) (Li et al., 2023) can achieve the effects of high-intensity training (Chua et al., 2022). Therefore, the combination of BFR training and low-intensity exercise can be used as an exercise intervention for older adults to improve their physical functions. BFR training combined with low-intensity exercise enhances muscle hypertrophy and strength gains by elevating metabolic stress (such as lactate accumulation) and hormonal responses (such as growth hormone secretion) (Takarada et al., 2000; Karabulut et al., 2010).
Training programs for older adults need to be carefully designed and managed (Agostini et al., 2023). A study has revealed that BFR pressure levels and exercise frequency are key influencing factors for the effectiveness of muscle growth (Yang et al., 2024). BFR pressure not only affects anabolic and metabolic pathways but also impacts cardiovascular health in older adults. Specifically, appropriate BFR pressure should maximise metabolic stress without completely occluding arterial blood flow or compromising the individual’s safety (McEwen et al., 2019). Furthermore, BFR pressure simultaneously exacerbates the burden on the cardiovascular system. Different levels of pressure have varying effects on physiological functions. Higher pressure during BFR training significantly impacts hemodynamics (Mouser et al., 2019), the autonomic nervous system (Bane et al., 2024), and hormonal responses (Thompson et al., 2024). Moreover, under high-pressure BFR training, metabolic stress is maximized by notably restricted venous return and arterial blood flow, but this may also increase the risk of developing vascular disease (Vervloet et al., 2024). In contrast, low-pressure BFR training partially restricts venous return while preserving arterial blood flow, thus ensuring an adequate supply of oxygen and nutrients during exercise. (Angelopoulos et al., 2021).
Training frequency and duration must be carefully selected to ensure the safety and effectiveness of different pressurised BFR training combined with low-intensity exercise for older adults (Yuan et al., 2023). However, the studies on how these factors influence muscle strength and cardiovascular health in older populations were insufficient and lacked definitive conclusions. Therefore, studies that focus on evaluating the safety and effects of BFR training in older adults, particularly in improving their cardiovascular health, are required. The findings of this study provide effective exercise plans for seniors, thus contributing to improving their training results and reducing health risks. This network meta-analysis compared the effects of different pressures and frequencies of BFR training combined with low-intensity exercise on muscular strength and cardiovascular health in older adults to find the optimal training program, thereby offering insights for clinical decision-making.
2 Materials and methods
This study strictly adhered to the guidelines outlined in the Preferred Reporting Items for Systematic Reviews and Meta-Analyses-Network Meta-Analyses (PRISMA-NMA) (Page et al., 2021) and was registered in PROSPERO (CRD42024534387).
2.1 Criteria for inclusion and exclusion
The inclusion criteria were as follows: 1) study type: randomized controlled trial and randomized crossover trial; 2) individual age: 55 ≤ age <80 years old (de Jong et al., 2006); 3) intervention: the interventions were categorised as experimental and control groups, with the experimental group performing BFR with low-intensity training (BFR-LI) and the control group (CG) performing low-intensity training; the intensity of the training (low intensity: 20%–30%1RM) (Li et al., 2023); the magnitude of pressurization [high pressure (HP) ≥120 mmHg; low pressure (LP) < 100 mmHg](Mattocks et al., 2019); the frequency [high frequency (HF): ≥3 days/week; low frequency (LF): <3 days/week](Scott et al., 2016); the experimental group used one of the following training regimens: low-frequency, low-pressure, and low-intensity BFR training (the LFLP regimen), low-frequency, high-pressure, and low-intensity BFR training (the LFHP regimen), high-frequency, low-pressure, and low-intensity BFR training (the HFLP regimen), and high-frequency, high-pressure, and low-intensity BFR training (the HFHP regimen), while the CG only performed low-intensity training without additional pressurized training; (d) outcome indices: one-repetition maximal strength (1RM), muscle cross-sectional area (CSA), isometric muscle strength (IMS), heart rate (HR), systolic blood pressure (SBP), diastolic blood pressure (DBP).
The exclusion criteria were as follows: 1) conference papers; 2) studies with unavailable full text or incomplete data; 3) duplicate publications;4) studies without a clear description of training intensity; 5) studies using intensity indicators that cannot be converted into standard metrics (such as percentage of 1RM, percentage of maximum heart rate, or ratings of perceived exertion).
2.2 Retrieval strategies
Searches were conducted separately by two researchers. PubMed, Web of Science, Embase, the Cochrane Library, Scopus, EBSCOhost, CNKI, Proquest, and medRxiv were searched up to March 2025. The search terms included “BFR training,” “Elder,” and “randomized controlled trial.” Specific search strategies are illustrated in Supplementary Table S1.
2.3 Literature selection and data extraction
Two researchers screened the retrieved literature by reviewing titles and abstracts. Based on inclusion and exclusion criteria, the eligible studies were selected after the full-text review. Any disagreements were addressed by a third reviewer. Data were extracted using an Excel sheet, including the authors, year, sample size, age, intervention program (frequency, intensity, period), and outcome indicators.
2.4 Risk of bias evaluation
The risk of bias was assessed by two researchers using the Physiotherapy Evidence Database (PEDro) scale, and any disagreements were addressed by a third reviewer. 11 items were scored by the PEDro scale. Since the evaluation of item 1 was excluded, the total score was 10. When the score was ≥6, the study was considered to have a low risk of bias.
2.5 Statistical methods
Data preprocessing and analysis were performed independently by two researchers. The extracted data were pre-processed using Excel 2016 and converted into mean differences or standard deviations between pre- and post-intervention values. The network meta-analysis was conducted using Stata 17.0. For outcomes with consistent units, weighted mean difference (WMD) were used; for outcomes with different units, standardized mean difference (SMD) were used, along with 95% confidence intervals (CI). A network evidence diagram was plotted for direct comparisons among different combinations of intervention intensity and frequency. In this diagram, the size of each node represented the sample size, while the thickness of the connecting lines reflected the amount of direct evidence between interventions. Two methods were employed to assess inconsistency: 1) loop inconsistency testing, where a 95% CI of 0 for the inconsistency factor indicated good consistency; 2) the node-splitting method, which separated each node (intervention) into direct and indirect comparisons to evaluate the differences between them. When a significant difference indicated inconsistency, an inconsistency model was applied. When no significant difference was suggested in consistency, a consistency model was used. The optimal combination of intervention intensity and frequency was identified by the cumulative ranking probability curve (SUCRA). To evaluate the robustness of the results, sensitivity analysis was conducted by sequentially excluding individual studies and re-analyzing the data to assess the stability of the findings. Additionally, adjusted funnel plots were utilized to evaluate potential small-sample effects or publication bias. To further examine the impact of publication bias on the results, Egger’s regression test was employed to assess the asymmetry of the funnel plots.
3 Result
3.1 Literature search results
A total of 1350 studies were selected. 84 studies remained after the initial screening. Ultimately, 18 studies were included in this net meta-analysis after a full-text review. The literature screening process is shown in Figure 1.
3.2 Basic characteristics of included studies
A total of 18 studies involving 626 participants, all published in English, were included. In the intervention group, two studies used LFLP interventions (Ferraz et al., 2018; Scott et al., 2018); six studies employed LFHP interventions (Vieira et al., 2013; Yasuda et al., 2013; Yasuda et al., 2014; Yasuda et al., 2015; Cook et al., 2017; Parkington et al., 2022); four studies applied HFLP interventions (Patterson and Ferguson, 2011; Letieri et al., 2018; Lopes et al., 2022); and eight studies employed HFHP interventions (Abe et al., 2010; Ozaki et al., 2010; Ozaki et al., 2011; Segal N. et al., 2015; Segal N. A. et al., 2015; Shimizu et al., 2016; Bigdeli et al., 2020). The muscle strength indices included 1RM (n = 4), CSA (n = 3), IMS (n = 3). These muscle strength indices covered various muscle groups. The cardiovascular indices included HR (n = 3), SBP (n = 4), and DBP (n = 4). The basic characteristics of the included studies are shown in Table 1.
3.3 Risk of bias evaluation results
The PEDro scores of the included studies ranged from 4 to 10, with a median score of 7. Out of the 18 studies, 12 met the predetermined threshold (≥6 points). The included studies were randomized controlled trials and clearly defined their inclusion criteria. Six studies provided detailed descriptions of allocation concealment; 13 studies reported comparable baseline characteristics; two studies implemented blinding for participants; none blinded the investigators; three studies blinded outcome assessors; 15 studies had a dropout rate exceeding 15%; and one study did not perform an intention-to-treat analysis. All studies reported between-group statistics, point estimates, and differences (as shown in Table 2).
3.4 Net meta-analysis
3.4.1 Network evidence diagram
The reticulation graphs showed that the LFHP regimen, HFHP regimen, and controls had larger sample sizes, while the LFLP regimen and the HFLP regimen had smaller sample sizes. Only the LFHP regimen, HFHP regimen, and the controls included the HR metrics. Direct comparisons between other BFR-LI intervention programs were lacking (as shown in Figure 2).
3.4.2 Inconsistency analysis
To assess the consistency in our network meta-analysis, the Inconsistency Factor for each closed loop was calculated. For the IMS, the Inconsistency Factor was 0.32 (95% CI: 0–2.51), with the 95% confidence interval including 0, indicating no significant inconsistency between direct and indirect comparisons (Figure 3). This result was further validated using the node-splitting method. It yielded a p-value >0.05 for IMS (Supplementary Table S2), thus confirming the good local consistency. Based on these findings, a consistency model was applied, as both the IF 95% CI included 0 and the node-splitting p-value >0.05 (Tu et al., 2014).
3.4.3 Meta-analysis results
For the 1RM outcome, compared to the CG, the LFLP intervention significantly improved 1RM (WMD = 0.58, 95% CI: 0.81–1.08, P < 0.05). The LFHP intervention also significantly increased 1RM (WMD = 0.38, 95% CI: 0.01–0.75, P < 0.05). Moreover, LFLP intervention outperformed other BFR-LI training regimens, though the difference was not statistically significant. These results indicated that the low-frequency BFR-LI regimen, whether high or low pressure, can improve 1RM (Figure 4A).
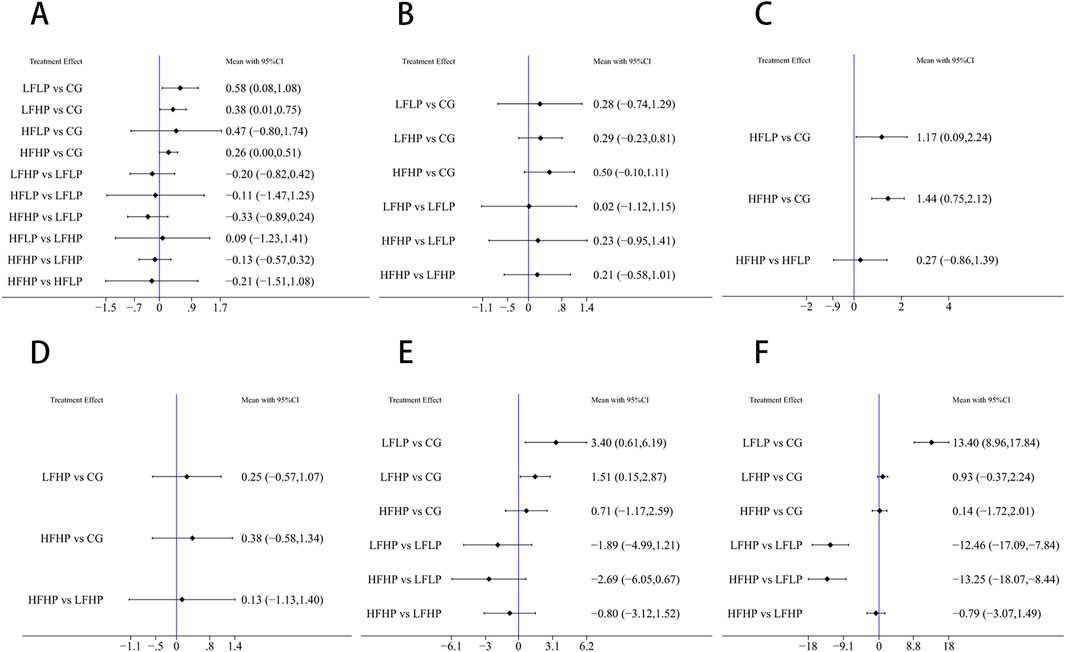
Figure 4. Results of reticulated meta-analysis (A): 1RM (B): CSA (C): IMS (D): HR (E): SBP (F): DBP.
For the CSA outcome, the HFHP intervention showed greater improvement than the CG (WMD = 0.50, 95% CI: −0.10–1.11, P > 0.05), and HFHP intervention also outperformed other BFR-LI training regimens, though differences were not statistically significant. This suggested that HFHP intervention may be the most effective for improving CSA (Figure 4B).
For the IMS outcome, pairwise comparisons showed that HFHP intervention significantly improved IMS compared to the CG (WMD = 1.44, 95% CI: 0.75–2.12, P < 0.05). Similarly, HFLP intervention also significantly improved IMS (WMD = 1.17, 95% CI: 0.09–2.24, P < 0.05). Thus, HFHP intervention can serve as the most effective for enhancing IMS (Figure 4C).
Pairwise comparisons across 1RM, CSA, and IMS indicated that HFHP intervention was more effective in boosting muscle strength than other BFR-LI training regimens with different pressures and frequencies.
For HR, although LFHP and HFHP interventions greatly increased HR compared to controls, the differences were not statistically significant (P > 0.05) (Figure 4D).
For SBP, the LFLP intervention significantly increased SBP compared to controls (WMD = 3.40, 95% CI: 0.61–6.19, P < 0.05). LFHP intervention also significantly increased SBP compared to controls (WMD = 1.51, 95% CI: 0.15–2.87, P < 0.05). Other pairwise comparisons showed no significant differences (Figure 4E).
For DBP, the LFLP regimen significantly increased DBP compared to controls (WMD = 13.40, 95% CI: 8.96–17.84, P < 0.05). In addition, the LFLP regimen increased more DBP compared to both LFHP (WMD = −12.46, 95% CI: −17.09 to −7.84, P < 0.05) and HFHP regimens (WMD = −13.25, 95% CI: −18.07 to −8.44, P < 0.05). Other comparisons were not statistically significant (Figure 4F).
3.4.4 Cumulative probability ranking results
For 1RM, CSA, and IMS outcomes, a higher cumulative area under the SUCRA curve indicated that the intervention was more effective in enhancing muscle strength in older adults. Conversely, for HR, SBP, and DBP, a higher SUCRA value suggested a more detrimental effect on cardiovascular health.
The results indicated that among muscle strength measures, HFHP intervention showed the best performance for both CSA and IMS, while LFLP intervention performed best for 1RM. In cardiovascular measures, the LFLP intervention caused the greatest increases in both SBP and DBP, while the HFHP intervention led to the highest increase in HR. These findings suggested that high-pressure training performed more than three times per week may be optimal for improving muscle strength in older adults. However, older adults should limit LPLF training to maintain cardiovascular health. (Figure 5).
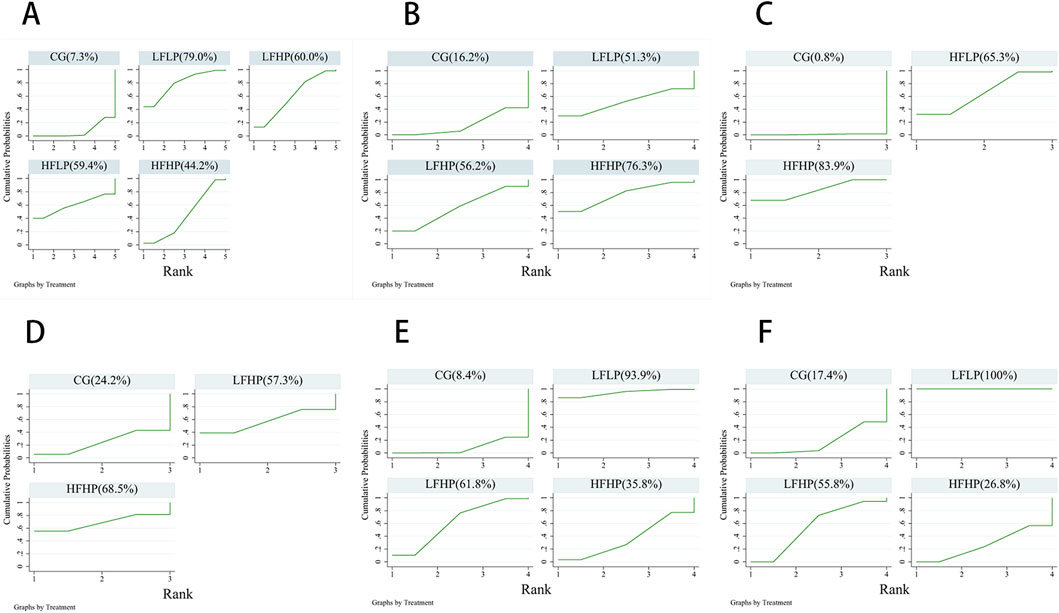
Figure 5. Cumulative ranked probability plots of the effects of each intervention programme on muscle strength and cardiovascular effects. (A): 1RM (B): CSA (C): IMS (D): HR (E): SBP (F): DBP.
3.4.5 Publication bias test
The funnel plot for each indicator was symmetrical. Most of the points were evenly distributed in the funnel plot, with only a small number of points falling on the outside of it. It suggested that the results were generally even. Since publication bias may exist, the results should be interpreted with caution (Figure 6). In the assessment of publication bias, we evaluated the results using Egger’s test. Egger’s test indicated no significant publication bias for metrics such as 1RM, CSA, IMS, HR, and SBP (p > 0.05). However, for DBP, Egger’s test showed significant publication bias (p < 0.05). Given the small sample size for DBP (n < 10), the reliability of Egger’s test results is limited, and we did not proceed with the trim-and-fill method. We recommend future studies with larger sample sizes further to validate the impact of publication bias on DBP. (Supplementary Figure S1).
3.4.6 Subgroup analysis
This study conducted subgroup analyses based on gender. The participants were divided into male, female, and mixed groups to analyse the effects of different pressurization training regimens on 1RM.
In the female group, the LFLP regimen showed statistical significance compared to the control group [WMD = 0.58, 95% CI (0.08, 1.08), P < 0.05] (Supplementary Figure S2B). The results indicated that the LFLP regimen ranked highest in the cumulative probability ranking for 1RM values (Supplementary Figure S2C). The funnel plots for the indicators were largely symmetrical, with most points evenly distributed (Supplementary Figure S2D).
In the male group, the HFHP regimen indicated statistical significance compared to the control group [WMD = 0.46, 95% CI (0.06, 0.86), P < 0.05] (Supplementary Figure S3B). The results suggested that the HFHP intervention ranked highest in the cumulative probability ranking for 1RM values (Supplementary Figure S3C). The funnel plots for the indicators were largely symmetrical, with most points evenly distributed (Supplementary Figure S3D).
For the mixed group, pairwise comparisons revealed no significant differences among the various regimens. No statistical significance was observed when comparing each BFR regimen with the control group (Supplementary Figure S4B). The results indicated that the LFLP intervention ranked highest in the cumulative probability ranking for 1RM values (Supplementary Figure S4C). The funnel plots for the indicators were largely symmetrical, with most points evenly distributed (Supplementary Figure S4D).
3.4.7 Sensitivity analysis
Since the age of the included participants mainly ranged between 60 and 70 years, a sensitivity analysis was conducted by excluding studies with higher age ranges. The results showed that the differences in the outcomes for the effects of different BFR-LI training on 1RM (Supplementary Figure S5) and IMS (Supplementary Figure S6) among older adults were minimal before and after the exclusion. Therefore, the results of this study are relatively reliable and stable.
4 Discussion
This net meta-analysis aims to investigate the effects of various BFR-LI training regimens combined with low-intensity exercise on muscle strength and cardiovascular function in older adults. We integrated four exercise regimens based on different pressure levels (high-pressure BFR ≥120 mmHg; low-pressure BFR <100 mmHg) and exercise frequencies (low frequency <3 sessions per week; high frequency ≥3 sessions per week) to identify the optimal BFR-LI training strategy. This net meta-analysis revealed that under high-pressure conditions, BFR-LI exercise performed three or more times per week yielded greater improvements in both CSA and IMS. Conversely, under low-pressure conditions, BFR-LI exercise performed less than three times per week resulted in better improvements in 1RM. However, under low-pressure conditions, there was a significant increase in both systolic and diastolic blood pressures in the BFR-LI training, while HR remained unaffected by changes in pressure or frequency.
Regarding muscle strength outcomes, both the chosen pressure level and training frequency significantly influenced post-training muscle growth in older adults. Our analysis demonstrated that the HFHP regimen was the most effective for increasing muscle strength. Specifically, high-pressure conditions, on average, were more effective in enhancing muscle strength than low-pressure conditions. This finding is consistent with previous studies which suggested that under high cuff pressures (mean > 120 mmHg), low-intensity exercise at high pressures can yield muscle strength improvements comparable to those achieved with high-intensity exercise at high pressures (Mahmoud et al., 2021). When using very low exercise loads, higher BFR pressures may be more conducive to muscle growth (Dankel et al., 2017). The underlying reasons for these effects are twofold. First, from a hemodynamic perspective, high-pressure BFR-LI leads to greater hemodynamic responses, including significant increases in HR, blood pressure, cardiac output, and the rate-pressure product, compared to low-pressure BFR-LI (Mouser et al., 2019). Second, there are differences in muscle activation. High-pressure BFR-LI may enhance muscle activation, whereas low-pressure BFR-LI might reduce it (Hwang and Willoughby, 2019). This difference potentially influences the degree of muscle strength and hypertrophy achieved (Brandner et al., 2015). Under high-pressure conditions, reduced blood flow to active muscles can result in the accumulation of inorganic phosphate, which induces muscle fatigue (Lixandrão et al., 2015) and prompts the recruitment of fast-twitch fibers and additional motor units to preserve force production, ultimately leading to muscle hypertrophy (Schoenfeld et al., 2019). Furthermore, several studies have shown that low-intensity exercise under low-pressure BFR-LI also contributes to muscle strength gains. For example, when performing elbow flexion exercises at 40% and 90% of arterial occlusion pressure in older adults, no significant differences in strength gains were observed between different pressure levels (Counts et al., 2016). This suggests that lower BFR-LI pressures can be effectively used during training. Regarding training frequency, our analysis indicated that high-pressure training required at least three sessions per week to optimally enhance muscle strength. Increased training frequency enhances muscular adaptations by promoting greater muscle fiber recruitment, activating metabolic pathways, and stimulating protein synthesis (Schoenfeld et al., 2019). Under high-pressure BFR-LI, higher training frequencies can elevate metabolic stress, including the accumulation of lactate and other metabolites, which is a key factor in promoting muscle growth (Moesgaard et al., 2022). Although some studies have suggested that as long as the total training volume is sufficient, lower frequencies can also induce muscle hypertrophy (Neves et al., 2022), the relatively low exercise load in BFR-LI exercise makes increasing frequency a more effective strategy to promote muscle activation. Studies have found that there are differences in the responses to pressurization training between genders. In the female group, the LFLP regimen significantly improved 1RM, while in the male group, the HFHP regimen was more effective in improving 1RM. This suggests that men and women may have different requirements for the intensity and frequency of pressurization training in muscle strength exercise. This difference may potentially be due to variations in physiological structure, hormone levels, or types of muscle fiber. In the subgroup analyses, the funnel plots were largely symmetrical with evenly distributed points, indicating minimal bias in the study results and high reliability of the data. This supports the credibility of the study’s conclusions and indicates the rigor of the study design and methodology.
This network meta-analysis revealed that a LFLP regimen was associated with more adverse cardiovascular effects. Regarding pressure, cuff pressure is one of the most important factors influencing the safety of BFR-LI training. Our findings are controversial compared to previous studies, which have indicated that following the LFLP regimen, the cardiovascular load is lower than that observed with high-intensity exercise without BFR (Ferreira et al., 2017). However, our study further observed that under low-pressure BFR-LI conditions, the cardiovascular responses during low-intensity exercise were more pronounced. Specifically, during low-pressure BFR-LI exercise, HR, SBP, and DBP increased significantly compared to both pure low-intensity exercise and other BFR exercises. This phenomenon may be related to exercise frequency. BFR-LI training has a dual impact on the autonomic nervous system. In the short term, BFR-LI training may activate the sympathetic nervous system and increase heart rate and blood pressure by partially restricting blood flow, thus leading to local muscle hypoxia and the accumulation of metabolites (such as lactate and hydrogen ions). These metabolites send signals to the central nervous system by stimulating type III and IV afferent fibers in the muscles, thus activating the sympathetic nervous system and increasing heart rate and blood pressure to cope with local hypoxia and metabolic stress, especially during high-pressure training (Igor S, 2021). In the long term, BFR-LI training may increase the release of nitric oxide (NO) and promote vasodilation by enhancing the activity of the parasympathetic nervous (Ferreira Junior et al., 2019) and improving vascular function (Shimizu et al., 2013; Killinger et al., 2020). The enhancement of parasympathetic nervous activity helps balance the overactivation of the sympathetic nervous system, thereby improving heart rate variability and blood pressure regulation, and reducing cardiovascular risk (Ma et al., 2024). The included studies that investigated low-pressure BFR-LI training involved relatively low exercise frequencies and durations and did not cover the impact of the HFLP regimen on cardiovascular outcomes. Therefore, future studies should explore the HFLP regimen to comprehensively evaluate the effects of different frequencies on cardiovascular health in older adults. Some studies have pointed out that due to excessive cuff pressure, high-pressure BFR-LI training can induce vascular occlusion that may trigger excessive muscle reflex activation (such as the activation of type III and IV afferent fibers), thus resulting in overactivation of the sympathetic nervous system, increased HR, elevated blood pressure, and increased vascular resistance (Sacino and Rosenblatt, 2019). However, the HFHP regimen can produce adaptive responses in older adults. Long-term BFR-LI training may reduce the muscle’s sensitivity to accumulated metabolites and signaling molecules, thereby potentially mitigating the training-induced increases in systolic blood pressure (Cho and Lee, 2024) and resulting in less cardiovascular harm. In contrast, LFLP training may provoke a marked acute increase in HR, systolic, and diastolic blood pressures. These responses are similar to those seen in high-intensity exercise (Zhang et al., 2022), which could elevate the risk of cardiovascular adverse events and neural injury (Sacino and Rosenblatt, 2019), causing greater discomfort for participants. This discrepancy may be attributed to age-related changes in the autonomic nervous system and neural aging in individuals who lack long-term regular physical exercise, which affects blood pressure regulation (Liu et al., 2022), thereby significantly increasing the risk of cardiovascular events during and after exercise.
The impact of BFR-LI training on cardiovascular health in older adults is complex. For individuals who cannot tolerate high mechanical stress on joints and suffer from skeletal muscle dysfunction, BFR-LI trainig may offer benefits (Rolnick et al., 2022). However, in patients with chronic conditions such as hypertension (Bezerra Silveira et al., 2022), diabetes (Camm et al., 2018), and chronic inflammation, improper use of BFR may increase the risk of cardiovascular diseases.
In terms of venous thrombosis, the effects of BFR-LI training on older adults are dual-sided: on one hand, it improves blood circulation by increasing the shear stress of blood flow, enhancing endothelial function, and promoting collateral circulation (Pereira-Neto et al., 2021). BFR-LI training also facilitates fibrinolysis by increasing tissue plasminogen activator (t-PA), reducing plasminogen activator inhibitor-1 (PAI-1), and activating the fibrinolytic system, thereby lowering the risk of thrombosis (Landers et al., 2025); on the other hand, it may increase the risk of thrombosis due to blood stasis, endothelial injury, and hypercoagulability (Engbers et al., 2010). Blood stasis occurs because older adults often have slower blood flow (Rosendaal et al., 2007), and BFR-LI training may further exacerbate this stasis. Endothelial injury is due to mechanical damage to vascular endothelial cells resulting from improper use of the cuff or excessive pressure during BFR-LI training (Chulvi-Medrano et al., 2023). Additionally, BFR-LI training may activate the coagulation system and inflammatory responses, causing a hypercoagulable state. Since older adults already have stronger coagulation function, BFR-LI training may further increase the risk of thrombosis. BFR-LI training should be conducted under professional guidance, with comprehensive screening and for thrombosis risks in older adults to ensure safety and effectiveness. (Heitkamp, 2015).
In future studies, it is essential to further investigate the impact of the HFLP regimen and the HFHP- regimen on cardiovascular indices in older populations. Although existing studies have revealed that the LFLP regimen has a pronounced effect on improving 1RM, the potential for LFLP training to trigger substantial cardiovascular responses in older adults must be considered. Therefore, considering the need to enhance muscle strength while preserving cardiovascular health, the HFHP regimen may represent a more suitable training strategy. This regimen aims to optimize the parameters of BFR-LI training to effectively boost muscle strength in older individuals while minimizing potential cardiovascular risks. It is recommended to further investigate the long-term effects of BFR-LI in different populations (such as older adults, and patients with chronic diseases) and clarify its safety and indications.
5 Limitation
1) The daily training habits of participants varied; the BFR-LI application sites were inconsistent; and the lack of blinding may led to uncertain outcomes. 2) Heterogeneity may occur in the cardiovascular effects of different training modalities on older adults. 3) Due to the limited number of studies included, statistical significance may not have been established; however, clinical significance cannot be ruled out. 4) Considering the limited literature and the insufficient data on individual differences and long-term safety, a systematic review of future studies should be conducted to refine our findings and strengthen data support for further research.
6 Conclusion
The HFHP regimen (pressures above 120 mmHg, 3 days per week) effectively enhances muscle strength and maintains cardiovascular health in older adults. In contrast, the LFLP regimen, though enhancing 1RM, may not be suitable for those with cardiovascular issues due to stronger physiological responses. The cardiovascular effects of the HFLP regimen remain unstudied and warrant further investigation.
When conducting research on different BFR-LI regimens, it is also important to consider various factors. In BFR-LI training, while training frequency and pressure magnitude are critical, other factors such as band size, duration, and intervals should also be considered to optimize the regimen. Although the HFHP regimen is beneficial, the cardiovascular risks associated with BFR-LI training remain uncertain. These risks, such as blood stasis and thrombosis, are particularly concerning for high-risk individuals, including those with hypertension or diabetes.
To address these challenges, Tailoring protocols based on gender, goals, and physiological traits can maximize benefits and reduce risks. Further research is needed to enhance BFR-LI training’s safety and effectiveness across diverse populations.
Data availability statement
The original contributions presented in the study are included in the article/Supplementary Material, further inquiries can be directed to the corresponding author.
Author contributions
MR: Conceptualization, Writing – original draft, Data curation, Formal Analysis, Investigation, Methodology, Software, Visualization. GX: Conceptualization, Data curation, Formal Analysis, Investigation, Software, Writing – original draft. XT: Conceptualization, Data curation, Formal Analysis, Investigation, Writing – original draft. SS: Conceptualization, Data curation, Methodology, Software, Writing – original draft. MZ: Writing – review and editing, Conceptualization, Project administration, Resources, Supervision, Validation.
Funding
The author(s) declare that no financial support was received for the research and/or publication of this article.
Acknowledgments
We would like to thank all the participants who have been involved in the manuscript and the authors from the included studies.
Conflict of interest
The authors declare that the research was conducted in the absence of any commercial or financial relationships that could be construed as a potential conflict of interest.
Generative AI statement
The author(s) declare that no Gen AI was used in the creation of this manuscript.
Publisher’s note
All claims expressed in this article are solely those of the authors and do not necessarily represent those of their affiliated organizations, or those of the publisher, the editors and the reviewers. Any product that may be evaluated in this article, or claim that may be made by its manufacturer, is not guaranteed or endorsed by the publisher.
Supplementary material
The Supplementary Material for this article can be found online at: https://www.frontiersin.org/articles/10.3389/fphys.2025.1587876/full#supplementary-material
References
Abe T., Sakamaki M., Fujita S., Ozaki H., Sugaya M., Sato Y., et al. (2010). Effects of low-intensity walk training with restricted leg blood flow on muscle strength and aerobic capacity in older adults. J. geriatric Phys. Ther. 33 (1), 34–40. doi:10.1097/JPT.0b013e3181d07a73
Agostini D., Gervasi M., Ferrini F., Bartolacci A., Stranieri A., Piccoli G., et al. (2023). An integrated approach to skeletal muscle health in aging. Nutrients 15 (8), 1802. doi:10.3390/nu15081802
Angelopoulos P., Mylonas K., Tsigkas G., Tsepis E., Billis E., Fousekis K. (2021). Blood flow restriction training in cardiovascular disease patients. doi:10.5772/intechopen.96076
Bane A., Wilson L., Jumper J., Spindler L., Wyatt P., Willoughby D. (2024). Effects of blood flow restriction resistance training on autonomic and endothelial function in persons with Parkinson’s disease. J. Parkinson’s Dis. 14 (4), 761–775. doi:10.3233/JPD-230259
Bezerra Silveira A. L., Monteiro De Carvalho L., Di Masi F., Pio T. W. S., Melibeu Bentes C. (2022). Blood flow restriction training on hypertesive subjects: a systematic review. Arch. Med. del Deporte 39 (2), 101–107. doi:10.18176/archmeddeporte.00080
Bielitzki R., Behrendt T., Behrens M., Schega L. (2021). Current techniques used for practical blood flow restriction training: a systematic review. J. Strength Cond. Res. 35 (10), 2936–2951. doi:10.1519/JSC.0000000000004104
Bigdeli S., Dehghaniyan M. H., Amani-Shalamzari S., Rajabi H., Gahreman D. E. (2020). Functional training with blood occlusion influences muscle quality indices in older adults. Archives Gerontology and Geriatrics 90, N.PAG-N.PAG 90, 104110. doi:10.1016/j.archger.2020.104110
Brandner C. R., Kidgell D. J., Warmington S. A. (2015). Unilateral bicep curl hemodynamics: low-pressure continuous vs high-pressure intermittent blood flow restriction. Scand. J. Med. and Sci. Sports 25 (6), 770–777. doi:10.1111/sms.12297
Camm A. J., Lüscher T. F., Maurer G., Serruys P. W. (2018). Cardiovascular risk assessment in diabetes and pre-diabetes. doi:10.1093/med/9780198784906.003.0216
Cho C., Lee S. (2024). The effects of blood flow restriction aerobic exercise on body composition, muscle strength, blood biomarkers, and cardiovascular function: a narrative review. Int. J. Mol. Sci. 25 (17), 9274. doi:10.3390/ijms25179274
Chua M. T., Sim A., Burns S. F. (2022). Acute and chronic effects of blood flow restricted high-intensity interval training: a systematic review. Sports Med. Open 8 (122). doi:10.1186/s40798-022-00506-y
Chulvi-Medrano I., Cortell-Tormo J. M., Hernández-Sánchez S., Picón-Martínez M., Rolnick N. (2023). Blood flow restriction training in clinical rehabilitation: occlusion pressure methods relative to the limb occlusion pressure. J. Sport Rehabilitation 32 (4), 361–368. doi:10.1123/jsr.2022-0240
Cook S. B., LaRoche D. P., Villa M. R., Barile H., Manini T. M. (2017). Blood flow restricted resistance training in older adults at risk of mobility limitations. Exp. Gerontol. 99, 138–145. doi:10.1016/j.exger.2017.10.004
Counts B. R., Dankel S. J., Barnett B. E., Kim D., Mouser J. G., Allen K. M., et al. (2016). Influence of relative blood flow restriction pressure on muscle activation and muscle adaptation. Muscle and Nerve 53 (3), 438–445. doi:10.1002/mus.24756
Dankel S. J., Jessee M. B., Buckner S. L., Mouser J. G., Mattocks K. T., Loenneke J. P. (2017). Are higher blood flow restriction pressures more beneficial when lower loads are used? Physiol. Int. 104 (3), 247–257. doi:10.1556/2060.104.2017.3.2
de Jong J., Lemmink K. A. P. M., Stevens M., de Greef M. H. G., Rispens P., King A. C., et al. (2006). Six-month effects of the Groningen active living model (GALM) on physical activity, health and fitness outcomes in sedentary and underactive older adults aged 55–65. Patient Educ. Couns. 62 (1), 132–141. doi:10.1016/j.pec.2005.06.017
Engbers M. J., Van Hylckama Vlieg A., Rosendaal F. R. (2010). Venous thrombosis in the elderly: incidence, risk factors and risk groups. J. Thrombosis Haemostasis 8 (10), 2105–2112. doi:10.1111/j.1538-7836.2010.03986.x
Ferraz R. B., Gualano B., Rodrigues R., Kurimori C. O., Fuller R., Lima F. R., et al. (2018). Benefits of resistance training with blood flow restriction in knee osteoarthritis. Med. and Sci. Sports and Exerc. 50 (5), 897–905. doi:10.1249/MSS.0000000000001530
Ferreira M. L. V., Sardeli A. V., Souza G. V., Bonganha V., Santos L. D. C., Castro A., et al. (2017). Cardiac autonomic and haemodynamic recovery after a single session of aerobic exercise with and without blood flow restriction in older adults. J. sports Sci. 35 (24), 2412–2420. doi:10.1080/02640414.2016.1271139
Ferreira Junior A., Schamne J. C., Altimari L. R., Okano A. H., Okuno N. M. (2019). Effect of walk training combined with blood flow restriction on resting heart rate variability and resting blood pressure in middle-aged men. Mot. Rev. Educ. Física 25 (2), e101945. doi:10.1590/s1980-6574201900020005
Grevendonk L., Connell N. J., McCrum C., Fealy C. E., Bilet L., Bruls Y. M. H., et al. (2021). Impact of aging and exercise on skeletal muscle mitochondrial capacity, energy metabolism, and physical function. Nat. Commun. 12 (1), 4773. doi:10.1038/s41467-021-24956-2
Heitkamp H. (2015). Training with blood flow restriction. Mechanisms, gain in strength and safety. J. sports Med. Phys. Fit. 55, 446–456.
Hughes D. C., Ellefsen S., Baar K. (2018). Adaptations to endurance and strength training. Cold Spring Harb. Perspect. Med. 8 (6), a029769. doi:10.1101/cshperspect.a029769
Hwang P. S., Willoughby D. S. (2019). Mechanisms behind blood flow-restricted training and its effect toward muscle growth. J. Strength Cond. Res. 33 (Suppl. 1), S167-S179–S179. doi:10.1519/JSC.0000000000002384
Igor S. B. (2021). Cardiovascular physiological effects generated by exercise with restriction of blood flow: a mini review. Archives Addict. Rehabilitation 3 (1). doi:10.36959/843/429
Karabulut M., Abe T., Sato Y., Bemben M. G. (2010). The effects of low-intensity resistance training with vascular restriction on leg muscle strength in older men. Eur. J. Appl. Physiology 108 (1), 147–155. doi:10.1007/s00421-009-1204-5
Killinger B., Lauver J. D., Donovan L., Goetschius J. (2020). The effects of blood flow restriction on muscle activation and hypoxia in individuals with chronic ankle instability. J. Sport Rehabilitation 29 (5), 633–639. doi:10.1123/jsr.2018-0416
Landers J. B., Allen M., Oladele I., Lowe L., Ali N., Rainey J., et al. (2025). Serum tissue plasminogen activator after cycling with blood flow restriction. Vasc. Biol. 7 (1), e240008. doi:10.1530/VB-24-0008
Letieri R. V., Teixeira A. M., Furtado G. E., Lamboglia C. G., Rees J. L., Gomes B. B. (2018). Effect of 16 weeks of resistance exercise and detraining comparing two methods of blood flow restriction in muscle strength of healthy older women: a randomized controlled trial. Exp. Gerontol. 114, 78–86. doi:10.1016/j.exger.2018.10.017
Li N., Yang J., Liao Y. (2023). The effect of blood flow restriction training combined with electrical muscle stimulation on neuromuscular adaptation: a randomized controlled trial. Front. Physiology 14, 1182249. doi:10.3389/fphys.2023.1182249
Liu W.-L., Lin Y.-Y., Mundel T., Chou C.-C., Liao Y.-H. (2022). Effects of acute interval exercise on arterial stiffness and cardiovascular autonomic regulatory responses: a narrative review of potential impacts of aging. Front. Cardiovasc. Med. 9, 864173. doi:10.3389/fcvm.2022.864173
Lixandrão M. E., Ugrinowitsch C., Laurentino G., Libardi C. A., Aihara A. Y., Cardoso F. N., et al. (2015). Effects of exercise intensity and occlusion pressure after 12 weeks of resistance training with blood-flow restriction. Eur. J. Appl. Physiology 115 (12), 2471–2480. doi:10.1007/s00421-015-3253-2
Lopes K. G., Farinatti P., Bottino D. A., de Souza M., Maranhão P., Bouskela E., et al. (2022). Exercise with blood flow restriction improves muscle strength and mass while preserving the vascular and microvascular function and structure of older adults. Clin. Hemorheol. Microcirc. 82 (1), 13–26. doi:10.3233/CH-221395
Ma X., Lin X., Zhou L., Li W., Yi Q., Lei F., et al. (2024). The effect of blood flow-restrictive resistance training on the risk of atherosclerotic cardiovascular disease in middle-aged patients with type 2 diabetes: a randomized controlled trial. Front. Endocrinol. 15, 1482985. doi:10.3389/fendo.2024.1482985
Mahmoud W. S., Osailan A., Ahmed A. S., Elnaggar R. K., Radwan N. L. (2021). Optimal parameters of blood flow restriction and resistance training on quadriceps strength and cross-sectional area and pain in knee osteoarthritis. Isokinet. Exerc. Sci. 29 (4), 393–402. doi:10.3233/IES-200235
Mattocks K. T., Mouser J. G., Jessee M. B., Dankel S. J., Buckner S. L., Bell Z. W., et al. (2019). High blood flow restriction pressure is necessary to induce vascular adaptations with very low-load training: 2406 board #70 may 31 11:00 AM - 12:30 PM. Med. and Sci. Sports and Exerc. 51 (6S), 659. doi:10.1249/01.mss.0000562468.02160.19
McEwen J. A., Owens J. G., Jeyasurya J. (2019). Why is it crucial to use personalized occlusion pressures in blood flow restriction (BFR) rehabilitation? J. Med. Biol. Eng. 39 (2), 173–177. doi:10.1007/s40846-018-0397-7
Moesgaard L., Beck M. M., Christiansen L., Aagaard P., Lundbye-Jensen J. (2022). Effects of periodization on strength and muscle hypertrophy in volume-equated resistance training programs: a systematic review and meta-analysis. Sports Med. Auckl. N.Z. 52 (7), 1647–1666. doi:10.1007/s40279-021-01636-1
Mouser J. G., Mattocks K. T., Buckner S. L., Dankel S. J., Jessee M. B., Bell Z. W., et al. (2019). High-pressure blood flow restriction with very low load resistance training results in peripheral vascular adaptations similar to heavy resistance training. Physiol. Meas. 40 (3), 035003. doi:10.1088/1361-6579/ab0d2a
Neves R. P., Vechin F. C., Teixeira E. L., Da Silva D. D., Ugrinowitsch C., Roschel H., et al. (2022). Effect of different training frequencies on maximal strength performance and muscle hypertrophy in trained individuals—a within-subject design. PLOS ONE 17 (10), e0276154. doi:10.1371/journal.pone.0276154
Ozaki H., Miyachi M., Nakajima T., Abe T. (2011). Effects of 10 weeks walk training with leg blood flow reduction on carotid arterial compliance and muscle size in the elderly adults. Angiology 62 (1), 81–86. doi:10.1177/0003319710375942
Ozaki H., Sakamaki M., Yasuda T., Fujita S., Ogasawara R., Sugaya M., et al. (2010). Increases in thigh muscle volume and strength by walk training with leg blood flow reduction in older participants. Journals Gerontology Ser. A Biol. Sci. Med. Sci. 66A (3), 257–263. doi:10.1093/gerona/glq182
Page M. J., McKenzie J. E., Bossuyt P. M., Boutron I., Hoffmann T. C., Mulrow C. D., et al. (2021). The PRISMA 2020 statement: an updated guideline for reporting systematic reviews. BMJ 372, n71. doi:10.1136/bmj.n71
Parkington T., Maden-Wilkinson T., Klonizakis M., Broom D. (2022). Comparative perceptual, affective, and cardiovascular responses between resistance exercise with and without blood flow restriction in older adults. Int. J. Environ. Res. Public Health 19 (23), 16000. doi:10.3390/ijerph192316000
Patterson S. D., Ferguson R. A. (2011). Enhancing strength and postocclusive calf blood flow in older people with training with blood-flow restriction. J. Aging Phys. Activity 19 (3), 201–213. doi:10.1123/japa.19.3.201
Pereira-Neto E. A., Lewthwaite H., Boyle T., Johnston K., Bennett H., Williams M. T. (2021). Effects of exercise training with blood flow restriction on vascular function in adults: a systematic review and meta-analysis. PeerJ 9, e11554. doi:10.7717/peerj.11554
Posadzki P., Pieper D., Bajpai R., Makaruk H., Könsgen N., Neuhaus A. L., et al. (2020). Exercise/physical activity and health outcomes: an overview of Cochrane systematic reviews. BMC Public Health 20 (1), 1724. doi:10.1186/s12889-020-09855-3
Rolnick N., Neto I. V. D. S., Fonseca E. F. D., Neves R. V. P., Rosa T. D. S., Nascimento D. D. C. (2022). Potential implications of blood flow restriction exercise on patients with chronic kidney disease: a brief review. J. Exerc. Rehabilitation 18 (2), 81–95. doi:10.12965/jer.2244082.041
Rosendaal F. R., Van Hylckama Vlieg A., Doggen C. J. M. (2007). Venous thrombosis in the elderly. J. Thrombosis Haemostasis 5, 310–317. doi:10.1111/j.1538-7836.2007.02489.x
Sacino A., Rosenblatt K. (2019). Early management of acute spinal cord injury—Part I: initial injury to surgery. J. Neuroanaesth. Crit. care 6 (3), 213–221. doi:10.1055/s-0039-1694688
Schoenfeld B. J., Grgic J., Krieger J. (2019). How many times per week should a muscle be trained to maximize muscle hypertrophy? A systematic review and meta-analysis of studies examining the effects of resistance training frequency. J. Sports Sci. 37 (11), 1286–1295. doi:10.1080/02640414.2018.1555906
Scott B., Peiffer J., Goods P. (2016). The effects of supplementary low-load blood flow restriction training on morphological and performance-based adaptations in team sport athletes. J. Strength Cond. Res. 31, 2147–2154. doi:10.1519/JSC.0000000000001671
Scott B. R., Peiffer J. J., Thomas H. J., Marston K. J., Hill K. D. (2018). Hemodynamic responses to low-load blood flow restriction and unrestricted high-load resistance exercise in older women. Front. physiology 9 (OCT), 1324. doi:10.3389/fphys.2018.01324
Segal N., Davis M. D., Mikesky A. E. (2015a). Efficacy of blood flow-restricted low-load resistance training for quadriceps strengthening in men at risk of symptomatic knee osteoarthritis. Geriatric Orthop. Surg. and rehabilitation 6 (3), 160–167. doi:10.1177/2151458515583088
Segal N. A., Williams G. N., Davis M. C., Wallace R. B., Mikesky A. E. (2015b). Efficacy of blood flow–restricted, low-load resistance training in women with risk factors for symptomatic knee osteoarthritis. PM&R 7 (4), 376–384. doi:10.1016/j.pmrj.2014.09.014
Sharp T., Grandou C., Coutts A. J., Wallace L. (2022). The effects of high-intensity multimodal training in apparently healthy populations: a systematic review. Sports Med. - Open 8, 43. doi:10.1186/s40798-022-00434-x
Shimizu R., Hotta K., Yamamoto S., Matsumoto T., Kamiya K., Kato M., et al. (2016). Low-intensity resistance training with blood flow restriction improves vascular endothelial function and peripheral blood circulation in healthy elderly people. Eur. J. Appl. Physiology 116 (4), 749–757. doi:10.1007/s00421-016-3328-8
Shimizu R., Kamekawa D., Akiyama A., Yokoyama M., Kato M., Kamiya K., et al. (2013). The low-intensity resistance training with blood flow restriction improves vascular endothelial function resulting in the reduction of arterial stiffness in the healthy elderly. Eur. Heart J. 34 (Suppl. 1), P5820. doi:10.1093/eurheartj/eht310.P5820
Takarada Y., Takazawa H., Sato Y., Takebayashi S., Tanaka Y., Ishii N. (2000). Effects of resistance exercise combined with moderate vascular occlusion on muscular function in humans. J. Appl. Physiology 88 (6), 2097–2106. doi:10.1152/jappl.2000.88.6.2097
Thompson K. M. A., Gamble A. S. D., Coates A. M., Burr J. F. (2024). Impact of blood flow restriction exercise on central hemodynamics and fluid regulating hormones. Med. and Sci. Sports and Exerc. 56 (2), 362–369. doi:10.1249/MSS.0000000000003307
Tu Y.-K., Salanti G., Del Giovane C., Chaimani A., Caldwell D. M., Higgins J. P. T. (2014). Evaluating the quality of evidence from a network meta-analysis. PLoS ONE 9 (7), e99682. doi:10.1371/journal.pone.0099682
Vakka A., Warren J. S., Drosatos K. (2023). Cardiovascular aging: from cellular and molecular changes to therapeutic interventions. J. Cardiovasc. aging 3 (3), 23. doi:10.20517/jca.2023.09
Vervloet G., Fregosi L., Gauthier A., Grenot P., Balestra C. (2024). Impact of five weeks of strengthening under blood flow restriction (BFR) or supplemental oxygen breathing (normobaric hyperoxia) on the medial gastrocnemius. J. Funct. Morphol. Kinesiol. 9 (4), 258. doi:10.3390/jfmk9040258
Vieira P. J. C., Chiappa G. R., Umpierre D., Stein R., Ribeiro J. P. (2013). Hemodynamic responses to resistance exercise with restricted blood flow in young and older men. J. Strength Cond. Res. 27 (8), 2288–2294. doi:10.1519/JSC.0b013e318278f21f
Vikberg S., Sörlén N., Brandén L., Johansson J., Nordström A., Hult A., et al. (2019). Effects of resistance training on functional strength and muscle mass in 70-year-old individuals with pre-sarcopenia: a randomized controlled trial. J. Am. Med. Dir. Assoc. 20 (1), 28–34. doi:10.1016/j.jamda.2018.09.011
Wilkinson B. G., Donnenwerth J. J., Peterson A. R. (2019). Use of blood flow restriction training for postoperative rehabilitation. Curr. Sport. Med. Rep. 18 (6), 224. doi:10.1249/JSR.0000000000000604
Xiang Z., Wang H., Li H. (2024). Comorbidity risk and distribution characteristics of chronic diseases in the elderly population in China. BMC Public Health 24 (1), 360. doi:10.1186/s12889-024-17855-w
Xu W., Mu D., Wang Y., Wang Y., Wang C., Zhang X. (2024). Association between oxidative balance score and sarcopenia in US adults: NHANES 2011-2018. Front. Nutr. 11, 1342113. doi:10.3389/fnut.2024.1342113
Yang K., Chee C. S., Abdul Kahar J., Tengku Kamalden T. F., Li R., Qian S. (2024). Effects of blood flow restriction training on physical fitness among athletes: a systematic review and meta-analysis. Sci. Rep. 14 (1), 16615. doi:10.1038/s41598-024-67181-9
Yasuda T., Fukumura K., Fukuda T., Uchida Y., Iida H., Meguro M., et al. (2013). Muscle size and arterial stiffness after blood flow-restricted low-intensity resistance training in older adults. Scand. J. Med. and Sci. Sports 24 (5), 799–806. doi:10.1111/sms.12087
Yasuda T., Fukumura K., Sato Y., Yamasoba T., Nakajima T. (2014). Effects of detraining after blood flow-restricted low-intensity training on muscle size and strength in older adults. Aging Clin. Exp. Res. 26 (5), 561–564. doi:10.1007/s40520-014-0208-0
Yasuda T., Fukumura K., Uchida Y., Koshi H., Iida H., Masamune K., et al. (2015). Effects of low-load, elastic band resistance training combined with blood flow restriction on muscle size and arterial stiffness in older adults. Journals Gerontology Ser. A Biol. Sci. Med. Sci. 70 (8), 950–958. doi:10.1093/gerona/glu084
Zhang T., Tian G., Wang X. (2022). Effects of low-load blood flow restriction training on hemodynamic responses and vascular function in older adults: a meta-analysis. Int. J. Environ. Res. Public Health 19 (11), 6750. doi:10.3390/ijerph19116750
Zhu L.-Y., Chan R., Kwok T., Cheng K.C.-C., Ha A., Woo J. (2019). Effects of exercise and nutrition supplementation in community-dwelling older Chinese people with sarcopenia: a randomized controlled trial. Age Ageing 48 (2), 220–228. doi:10.1093/ageing/afy179
Keywords: blood flow restriction training, low-intensity training, older adults, muscle strength, cardiovascular safety
Citation: Ren M, Xian G, Tan X, Sun S and Zhang M (2025) Effect of different blood flow restriction training regimens combined with low-intensity training on muscle strength and cardiovascular safety in older adults: a systematic review and network meta-analysis. Front. Physiol. 16:1587876. doi: 10.3389/fphys.2025.1587876
Received: 05 March 2025; Accepted: 02 April 2025;
Published: 28 April 2025.
Edited by:
Tim Werner, Salisbury University, United StatesReviewed by:
Paolo Capodaglio, University of Milan, ItalyThiago Santos Rosa, Catholic University of Brasilia (UCB), Brazil
Copyright © 2025 Ren, Xian, Tan, Sun and Zhang. This is an open-access article distributed under the terms of the Creative Commons Attribution License (CC BY). The use, distribution or reproduction in other forums is permitted, provided the original author(s) and the copyright owner(s) are credited and that the original publication in this journal is cited, in accordance with accepted academic practice. No use, distribution or reproduction is permitted which does not comply with these terms.
*Correspondence: Ming Zhang, MjE0N0Bic3UuZWR1LmNu