- 1Dipartimento di Scienze della Vita e dell'Ambiente, Università Politecnica delle Marche, Ancona, Italy
- 2Istituto Zooprofilattico Sperimentale dell'Umbria e delle Marche “Togo Rosati”, Ancona, Italy
- 3Azienda USL Umbria 1, Perugia, Italy
Microplastics (MPs) and brominated flame retardants (BFRs) represent a synergic threat for aquatic environments and organisms' health status, with an additional concern over food quality and food security for species of commercial interest. In this study, the ingestion of MPs, levels of polybrominated diphenyl ethers (PBDEs), and hexabromocyclododecane (HBCDs) were assessed in organisms from two lakes of Central Italy, with the aims of exploring the bioavailability of these pollutants in freshwater environments, the possible translocation of MPs from digestive to edible tissues, and the relationship between MPs ingestion and bioaccumulation of BFRs. The fish Perca fluviatilis, Anguilla anguilla, Carassus auratus, and the crayfish Procambarus clarkii, all species commercialized for human consumption, were caught in Trasimeno Lake; moreover, P. fluviatilis and Rutilus rutilus were also sampled in Piediluco, a lake strongly influenced by industrial and anthropogenic activities, where fishing for commercial purpose is forbidden. With the exception of C. auratus which showed the highest frequency of MPs ingestion (75%), species from Piediluco Lake exhibited a more elevated percentage of organisms positive to MPs ingestion (45%) and higher levels of PBDEs and HBCDs (mean values of 343 and 792 pg/g, respectively, in P. fluviatilis; 445 and 677 pg/g, respectively, in R. rutilus) than Trasimeno species (25% frequency of MPs ingestion, mean values between 6 and 163 pg/g for PBDEs and 5-107 pg/g for HBCDs). Polyester fibers dominated among MP typologies, and a high occurrence of man-made natural fibers was recorded. The number of MPs extracted in the gastrointestinal tracts of fish and soft bodies of crayfishes positive to MPs ingestion ranged between 1 and 2, whereas no MPs were found in fish filets. Given these results, the risk related to human consumption of Trasimeno organisms appears very low, whereas further investigations are required to better elucidate the possible role of MPs pollution in modulating chemical bioaccumulation in edible tissues. This study contributed to assess both environmental quality and food safety, reinforcing the use of bioindicator species for monitoring plans, in accordance with European recommendations.
Introduction
Microplastics (MPs) and brominated flame retardants (BFRs) are deliberated as contaminants of emerging concern (ECs), often associated considering the wide use of BFRs as plastic additives. Monitoring MPs and BFRs in aquatic environments has become a priority for scientists and policy, due to their persistence in natural environments, bioavailability, and potential toxicity to organisms and to human beings (Lin et al., 2018).
Microplastics are extensively found in sediments, waters, and aquatic biota (Du et al., 2021), existing in a mixture of heterogenous particles, as they encompass a broad range of sizes (<5 mm), vary in colors and shapes (e.g., fiber, fragment, sphere, and film), originate from a large diversity of products (e.g., tires, textiles, packaging, and fishing gears), and are composed by a complex chemical cocktail of polymers and additives (e.g., plasticizers, colorants, and flame retardants), and of adsorbed co-occurring contaminants (Rochman et al., 2019). Ingestion is the major route of MPs exposure for biota and biological effects include a wide spectrum of responses spanning from physical injuries to gene expression alterations, inflammation, immune responses, oxidative stress, microbiota imbalance, and disorders of physiological functions (Avio et al., 2015a; Pittura et al., 2018). Depending on their size, ingested MPs can enter the cells of the digestive system and be translocated to different tissues through circulatory systems (Du et al., 2021). Their permanence in the digestive tract could result in the leakage of adsorbed chemicals but the magnitude of this phenomenon and its contribution to bioaccumulation and toxicological effects are yet to be fully elucidated (Barboza et al., 2018).
Brominated flame retardants are incorporated in electronics, textiles, and plastics to inhibit the risk of fire (Iqbal et al., 2017), and the massive growth of plastic production has led to a parallel increase in the use of flame retardants (Alaee et al., 2003), particularly polybrominated diphenyl ethers (PBDEs) and hexabromocyclododecanes (HBCDs). PBDEs are a family of 209 congeners produced in three main commercial formulations (penta-, octa-, and deca-BDEs), whereas technical products of HBCDs contain mainly three isomers (α-, β-, and γ-HBCD) (Law et al., 2005). Since PBDEs and HBCDs are incorporated into plastic or textiles and not chemically bound, they can gradually leach into the environment during production, usage, and disposal of products. Due to their high lipophilicity and persistence, they can be accumulated in fat of living organisms and biomagnified through the food web (EFSA, 2011; EFSA Panel on Contaminants in the Food Chain (CONTAM), 2021). Since 2009, PBDEs and HBCDs have been progressively listed as POPs under the Stockholm Convention and restricted in many fields (UNEP, 2010, 2013, 2014, 2018) due to their toxic effects, mainly related to endocrine alterations, neurobehavioral disorders, and mutagenicity (Mikula and Svobodova, 2006; Smolarz and Berger, 2009).
Human exposure to PBDEs and HBCDs occurs mainly via diet and, particularly, through fish and fish oil-based products (EFSA, 2011; EFSA Panel on Contaminants in the Food Chain (CONTAM), 2021). The European Food Safety Authority (EFSA) delivered to the European Commission, two scientific opinions on PBDEs and HBCDs, assessing their occurrence in food, including “fish and other seafood,” and the associated risk for human exposure, highlighting the need to obtain more data, since available information is still insufficient to fix limits of BFRs in food and to derive a toxicological reference level (EFSA, 2011; EFSA Panel on Contaminants in the Food Chain (CONTAM), 2021). In 2014, the European Commission issued the first Recommendation aiming to collect data on BFRs in food (European Commission, 2014). More recently, also, the evidence of MPs in commercially important fish and shellfish species raised concerns about possible implications for human consumers (Barboza et al., 2018). Although the presence of MPs in these species was mainly investigated in the gastrointestinal tracts, which are generally not consumed, there is proof that the evisceration does not necessarily eliminate the risk of microplastics intake by humans (Karami et al., 2017). This possibility is certainly greater when organisms are eaten whole (e.g., some mollusks, crustaceans, and small fish; UNEP, 2021) or when MPs are detected in muscle (Abbasi et al., 2018; Akhbarizadeh et al., 2018; Haave et al., 2021). To date, no legislation exists for MPs as contaminants in food (Myszograj, 2020) and the risk for human health related to consumption of seafood products is still unclear; since 2016, EFSA identified the need to generate data on levels of MPs in food and on their potential effects on human health (EFSA, 2016).
The majority of data on BFRs and MPs in aquatic organisms are referred to marine species, whereas our knowledge is more limited for freshwater fish and shellfish despite their relevance as both bioindicators and vehicles of chemicals and MPs for humans. In this respect, this study aimed to assess the presence of MPs, PBDEs, and HBCDs in various species sampled in two Italian lakes Piediluco and Trasimeno (Umbria region, Central Italy), representing important touristic areas where fishing activities are particularly relevant for the local economy, although there are differences in hydrography and anthropic pressure. Fish species of Trasimeno Lake are a local and tourist food resource (Carosi et al., 2019), whereas fishing for human consumption is forbidden in Piediluco Lake where only catch-and-release recreational fishing is allowed. The target species of the study were chosen based on their commercial value and/or ecological relevance within each basin. Both non-edible and edible tissues were analyzed with the specific aims to: (i) define the background levels of MPs in fish species of Trasimeno and Piediluco lakes, to date unexplored, focusing also on sizes, shapes, and polymers of ingested particles; (ii) compare the environmental status of the study areas in relation to MPs and BFRs pollution validating the use of bioindicators for monitoring surveys; (iii) generate data on the presence of MPs and BFRs in edible freshwater species for food safety evaluations; (iv) highlight a possible link between MPs ingestion and bioaccumulation of BFRs as plastic-derived chemicals.
Materials and Methods
Study Areas
Location of the two lakes is shown in Figure 1. Trasimeno Lake is the largest one in Central Italy (Umbria Region) with a surface of 126 km2 and an average depth of 4.7 m. It is a closed laminar lake without natural tributaries, and its hydrological regime depends on local rainfall, typically maximum in autumn and minimum in summer (Tavoloni et al., 2021). In addition to these peculiar features, the Trasimeno Lake is exposed to a limited anthropogenic pressure (Carosi et al., 2019): settlements are not very high in terms of equivalent inhabitants, the civil discharges are subjected to an efficient purification, and most of them are collected outside the lake basin. Even the isolated uncollected drains are not relevant and, to a large extent, are self-purified from the drainage network before they reach the lake (PSR Umbria, 2013). Among the economic activities, this lacustrine area is characterized by an ancient tradition of fishery, with several species of great commercial value (Carosi et al., 2019). Being a natural resource of great interest, Trasimeno Lake is considered as a Special Area of Conservation (IT5210018; Habitat Directive, 1992/43/EEC), Special Protection Area (IT5210070; Bird Directive, 2009/147/EC), and it is also a regional park (Rocchi et al., 2017).
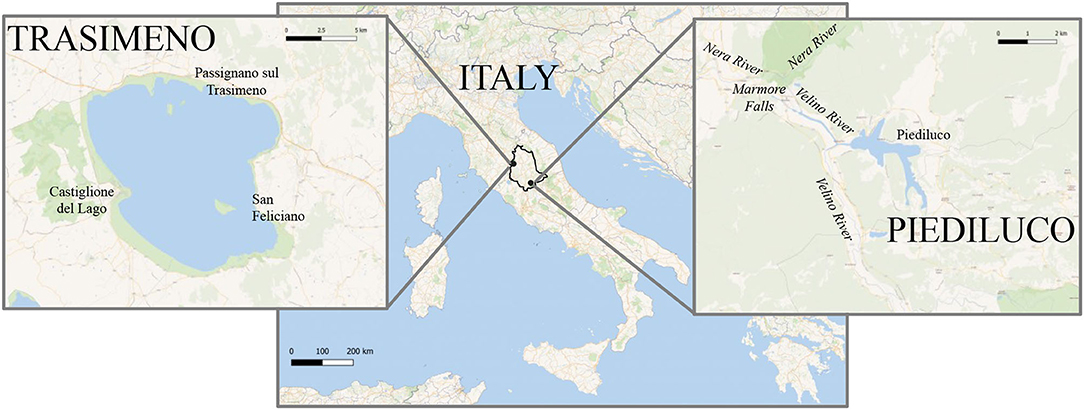
Figure 1. Map of the sampling areas: Trasimeno and Piediluco lakes, located in Central Italy (Umbria region).
Piediluco Lake is the second largest lake in Umbria Region, with a surface of 1.7 km2 and an average depth of 20 m. It receives continuous water inflow from the Nera River and the Velino River, and it is used as a daily water-storage basin for a hydroelectric plant. It was classified as hypereutrophic lake due to increased inflow of nutrients from its drainage basin and tributaries, with a notable contribution from the fish farms located upstream, persistent and intense algal blooms and a poor water quality. Furthermore, it is located within a densely populated area, characterized by the strong presence of industrial settlements in the steel, chemical, electrical, and textile sectors. These conditions, along with the introduction of some non-native species in the past, led to a decay of valuable fish species and to prohibition of commercial fish activities (La Porta et al., 2010).
Sampling and Tissue Collection
Sampling was carried out in 2019 by local fishermen using fyke nets. A total of 136 individuals (n = 20 from Piediluco Lake and n = 116 from Trasimeno Lake) were collected and five species were investigated: roach (Rutilus rutilus, n = 10), European perch (Perca fluviatilis, n = 10 from Piediluco and n = 16 from Trasimeno), goldfish (Carassus auratus, n = 12), European eel (Anguilla anguilla, n = 9), and the red swamp crayfish (Procambarus clarkii, n = 79; Supplementary Table 1).
P. fluviatilis is the only species caught from both Trasimeno and Piediluco Lake. It is a benthic ichthyophagous middle water predator, one of the most appreciated commercial and game freshwater fish, thus very important for local economies and tourism (Galafassi et al., 2021). In Piediluco Lake, P. fluviatilis population has declined drastically over the years, due to both the environmental deterioration and the competitive interaction with other exotic species (Dezfuli et al., 2013), such as R. rutilus. A. anguilla is a carnivorous bottom fish, representing one of the most important commercial fish species in Trasimeno Lake and the only native among those investigated. C. auratus is an allochthones species highly spread in the Trasimeno Lake for its invasive capacity, elevated tolerance to environmental conditions, high fertility, and wide alimentary regimen. It has a low commercial value, and its meat is used as food only after undergoing specific processing (Dal Bosco et al., 2012). P. clarkii, also known as Louisiana crayfish, was recorded for the first time in Trasimeno Lake in 1999, and after a rapid expansion, it is nowadays well-established, becoming an economical important resource for the local fish market (Dörr et al., 2006).
After the sampling, organisms were immediately transported in laboratory, where total length and total weight of organisms were recorded before tissues dissection. Fishes were measured from the mouth to the caudal fin, while crayfish from the tip of the rostrum to the posterior margin of the cephalothorax. MPs were analyzed in the gastrointestinal tracts of all fish species, in whole soft tissues of crayfish and in the dorsal muscles of only P. fluviatilis and A. anguilla sampled in Trasimeno Lake. BFRs were measured in muscles of all fish species and in whole tissues of crayfish. Once collected, tissues were immediately stored at −20°C until processing. Samples were analyzed as single specimens, excepting for PBDEs and HBCDs determination in P. clarkii and in P. fluviatilis collected from Trasimeno Lake: tissues were pooled putting together individuals of similar size as the material of the single specimen was not sufficient (Supplementary Table 1).
Extraction and Characterization of MPs
To obtain the best extraction yield, different methods were applied depending on organisms and tissues to be processed. Soft tissues of crayfish were processed through an alkaline digestion using KOH (potassium hydroxide), that is, the most applied method to extract microplastics from biota and particularly fast for digestion of small invertebrates (Bessa et al., 2019). Digestion was carried out in 100-ml glass beakers with 10% KOH solution (1:5 w/v) prepared with ultra-pure water: samples were left in an oven at 50°C for the time necessary to complete dissolution of tissues and subsequently filtered under vacuum using mixed cellulose ester (MCE) membrane filters (Ø 47 mm, pore size 8 μm, ClearLine). Filters were recovered in glass Petri dishes and left to dry at 50°C after the addition of a 15% H2O2 solution to ensure the removal of any residual organic matter (Avio et al., 2020).
Given the fatty content, the KOH digestion was not suitable for the extraction of microplastics from fish, because of the formation of oil droplets in the solution that hampers the subsequent steps (Bessa et al., 2019); therefore, in fish species, MPs extraction was carried out according to the method validated by Avio et al. (2015b, 2020) and already applied on a range of marine species (Avio et al., 2017; Bour et al., 2018; Bessa et al., 2019; Cau et al., 2019, 2020; Vital et al., 2021): for that method, a recovery yield higher than 90%, for particles smaller than 100 μm, and 95%, for greater ones, was demonstrated, with no alteration on particle characteristics such as shape, size, and color. Briefly, samples were dried, gently triturated using mortar and pestle, and mixed with a saturated solution of NaCl salt (1.2 gr cm−3) for a density separation of microplastics. The supernatants, obtained after settling of mixed solution, were filtered under vacuum; obtained dried filters were visually examined using a stereomicroscope (GZ 808 Optech with Optech IS 4K-8 digital camera), and a manual sorting was performed through tweezers, to isolate the potential microplastics, i.e., particles holding their shape or stretching and resistance to easy breakage when poked with tweezers, bright and unnaturally colored particles, fragments with sharp geometrical shapes, and sheet-like and thread-like particles (Primpke et al., 2020). Collected items were transferred onto a clean cellulose acetate membrane (Sartorius Stedim Biotech, Ø 47 mm, 0.45 μm pore size), located on a microscope slide for the subsequent polymer characterization, and classified based on shape according to definitions of fibers, fragments, films, and pellet (Avio et al., 2020). Items were additionally measured using an image analysis software (Image View): length was recorded for fibers, whereas other typologies of items (e.g., fragments, films, and pellet) were measured on the basis of the largest dimension following the most widespread criterion (Avio et al., 2020). Fibers longer than 5 mm were not considered as microfibers and excluded from results (Supplementary Figure 1).
The chemical identification was performed through μFTIR spectroscopy, using a Spotlight 200i FT-IR microscope system (Perkin Elmer) equipped with Spectrum Two and driven by Spectrum 10 software. Measurements were taken in the Mid-IR region (wavenumber range: 4,000–600 cm−1) by attenuated total reflection (μATR-FTIR), with the resolution set at 4 cm−1 and the optical aperture's dimension of 100 × 100 μm. IR spectra were acquired after 32 scans per sample, and several backgrounds were performed through the working session. For their interpretation, comparison was carried out with commercial spectra libraries (PerkinElmer®) that were implemented with personal-created ones, resulting from characterization of microplastics extracted during previous studies, and with that compiled within the framework of the JPI-OCEANS project BASEMAN: polymers matching with reference spectra for a Hit Quality Index (HQI) ≥ 0.7 were validated, after, however, a careful examination of peaks characteristics (Avio et al., 2020).
Determination of PBDEs and HBCDs
Chemicals
Standard solutions containing α-HBCD, β-HBCD, γ-HBCD, the respective labeled isotopes α-HBCD-13C12, β-HBCD-13C12, γHBCD-13C12, PBDE 49, 66, 77, 85, 138, 197, and 206 at 50.0 ng/μl and the mass-labeled PBDE recovery standard solution (BDE-77-13C12 and BDE-138-13C12) at 2.0 ng/μl (purity >98%) were supplied by Wellington Laboratories (Guelph, Ontario, Canada). The Method 1614 labeled surrogate stock solution (BDE-28-,−47-,−99-,−100-,−153-,−154-, and−183-13C12 at 1.0 and 10 ng/μl for BDE-209-13C12, purity >98%,) and Method 1614 native par stock solution (BDE-28,−47,−99,−100,−153,−154, and−183, at and 10 ng/μl for BDE-209, purity >98%) were purchased by Cambridge Isotope Laboratories (Andover, MA, USA). The PBDE solutions are in nonane, while HBCDs in toluene. Methanol for LC-MS was supplied by Carlo Erba Reagents (Rodano, Milano, Italy). Cyclohexane (c-Hex), dichloromethane (DCM), ethyl acetate (EtOAc) n-hexane (Hex), and isooctane were pesticide grade (Merck Co., Darmstadt, Germany). Magnesium sulfate, sodium chloride, Extrelut-NT3 columns, and sulfuric acid 98% were delivered from Merck Co. (Darmstadt, Germany). Milli-Q water grade was produced by a Millipore purification system (Bedford, MA, USA). BioBeads S-X3 200–400 mesh was purchased from Bio-Rad Laboratories (Switzerland). Solid Phase Extraction (SPE) Isolute silica 1 g/6ml columns were obtained from Biotage (Uppsala, Sweden).
Analytical Method
The analytical method was reported from Tavoloni et al. (2020). About 20 g of samples was weighed in a polypropylene centrifuge tube, spiked at 1 ng/g (10 ng/g BDE-209-13C12) of labeled BDEs and HBCDs internal standard (IS) and submitted to QuEChERS extraction. About 10 ml of the upper organic layer was transferred in a clean glass tube and reduced in volume at 35°C using the Genevac EZ-2 concentrator (SP Scientific, Ipswich, Suffolk, UK). The residue was purified on H2SO4 acidified Extrelut NT3/SPE Si 1g/6 ml tandem columns assembly and then by gel permeation chromatography (Gilson GPC system equipped with ASPEC XL auto sampler, 307 HPLC pump and UV–VIS detector, Gilson Wisconsin, USA). Each purified extract was divided into two fractions and carefully reduced to dryness. Before GC–MS/MS analysis, PBDEs fraction was resuspended in 125 μl of the syringe standard solution (BDE-77-13C12 and BDE-138-13C12 at 2.0 ng/ml in isooctane). The HBCDs fraction was dissolved in 125 μl of β-HBCD-13C12 solution at 2.0 ng/ml in MeOH: CH3COONH4 2 mm (50:50) (HBCDs syringe standard) prior to LC-MS/ MS injection. The instrumental conditions were reported in Supplementary Tables 2, 3. A total of fifteen PBDE congeners (28, 47, 49, 66, 77, 85, 99, 100, 138, 153, 154, 183, 197, 206, and 209) and three HBCD isomers (α, β, and γ) were measured. PBDEs analysis was performed on a 7890A GC — 7000B triple-quadrupole mass analyzer in EI ionization mode (Agilent Technologies, Palo Alto, CA, USA). The system was equipped with a programmable temperature vaporization (PTV) inlet and7693 auto sampler on aRTX1614 column (15 m × 250 μm × 0.10 μm— Restek) using helium as carrier gas (1 mL/min). HBCD isomer analysis was performed on a hybrid triple-quadrupole/linear ion trap 3200 Q TRAP mass spectrometer (AB Sciex, Darmstadt, Germany) equipped with a Turbo V source and an electrospray ionization (ESI) probe, operated in ESI-negative mode. Mass spectrometer was coupled to a 1200 HPLC, Agilent (Palo Alto, CA, USA), and the chromatographic separation was achieved using a Kinetex XB-C18 column (2.6 μm 100 Å, 100 × 2.10 mm — Phenomenex, Torrance, CA, USA) in gradient conditions. Limits of quantification (LOQs) were set to 5 pg/g for PBDEs (100 pg/g only for BDE−206 and−209) and 10 pg/g for HBCDs. LOQs were assessed on fortified samples (repeatability/reproducibility was compliant to Horwitz theoretical values) and limits of detection (LOD) fixed as one-third LOQs.
QA/QC
MPs Analysis
Several practices were applied to prevent and check microplastics and microfibers contamination by external sources during the whole study, starting from dissection. Procedures were carried out in a clean room, limiting the access to a maximum of two operators at the same time. Cotton laboratory coats and nitrile gloves were worn, and work benches were cleaned with ethanol pure grade.
Glass and metal equipment were used whenever possible and, before use, it was rinsed with ultra-pure water, additionally cleaned with compressed air, and covered with aluminum foils, which were also kept during density separation and filtration operations. All the solutions were prepared in ultra-pure water and additionally filtered with nitrate acetate membrane with pore size of 0.45 μm before use (Avio et al., 2020).
To avoid over-estimation of MPs, procedural blanks (i.e., control samples) were run along with field samples to account for exogenous MPs possibly derived from background contamination, represented by air deposition, release from laboratory coats and clothing of operators, and from procedures used for tissues processing, despite the applied precautions (Hung et al., 2020; O'Connor et al., 2020; Prata et al., 2021).
They consist of 100-ml glass beakers containing pre-filtered 10% KOH solution or distilled water left open on the workbenches during dissection of organisms and subsequently processed as the samples. A total of 14 procedural blanks were performed, one for each battery of around 10 samples. Only fibers were found on procedural blanks filters, with an average of 3.1 ± 1.8 fibers/filter. The 81% of fibers was of natural origin, cellulose- and animal-based, and the remaining 19% was made of polyester (PES). The number of fibers found in a procedural blank was subtracted to the number of fibers found in the biological samples processed in the corresponding battery. Operation was carried out considering both the physical characteristics of fibers (shape and color) and the polymeric nature resulting by the μFTIR characterization.
PBDEs and HBCDs Analysis
The QA/QC procedures are those reported by Tavoloni et al. (2020). Background contamination at any stage of the analytical process was carefully monitored and subtracted to the results when it was not negligible (>10%). Glassware was rinsed with acetone and heated in oven at 200°C overnight. To monitor method performances, two procedural blanks, a blank sample and the same blank sample spiked at 0.100 ng/g for PBDEs and 0.050 ng/g for HBCDs, were processed in each analytical batch. The results were reported in X-chart (compiled with spiked sample recoveries) and R-chart (built measuring the labeled IS recovery repeatability in the blank and the spiked samples). Background laboratory contamination was monitored checking BDE-47 levels in procedural blanks. The analysis was conducted using the isotopic dilution methodology, recording the recoveries of labeled internal standards in each sample. The detailed method performance characteristics as well as the batch-to-batch quality controls are described in our previous papers (Piersanti et al., 2018; Tavoloni et al., 2020). External quality assurance was guaranteed by regular participation to inter-calibration exercises organized by the EURL for halogenated POPs in Feed and Food (European Commission, 2000).
Data Expression and Data Analysis
Data on quantification and characterization of MPs for each species were given as (i) an average number of MPs extracted from tissues calculated on organisms positive to ingestion and reported as MPs per individual; (ii) frequency of ingestion, i.e., the percentage of specimens containing at least one MPs on the total number of analyzed organisms; (iii) mean size of MPs extracted from tissues; and (iv) relative percentage distribution (frequency) of each shape and polymer typology to the total number of MPs extracted from samples.
For P. fluviatilis, the only species sampled from both Piediluco and Trasimeno lakes, differences in the average number of MPs ingested per individual were analyzed using a Kruskal–Wallis nonparametric test since data did not comply with the assumptions of normality and homogeneity of variance (checked by Shapiro–Wilk test and Fisher's F-test, respectively); level of significance for the rejection of the null hypothesis was set at p < 0.05. To evaluate whether the ingestion of MPs may depend more on biological factors than on different levels of MPs pollution in the two lakes, the relation between size of organisms (total length and total weight) and both frequencies of ingestion and average number of ingested MPs was assessed applying Pearson correlation coefficient.
The raw results of BDEs and HBCDs were elaborated to obtain the sums using the “lower bound” approach,” namely, summing up zero when the single congener/isomer concentrations were below the limit of quantification. For PBDEs, sums were reported both as sum of the all 15 congeners analyzed and of the “10 indicator congeners,” considered to be of primary interest and included in Commission Recommendation 2014/118/EU (BDE-28,-47,-49,-99,-100,-138,-153,-154,-183,-209).
In addition, a multivariate principal component analysis (PCA) was applied to highlight the differences between the study areas and among species based on the frequency of MPs ingestion and on detected levels of ∑10PBDEs and ∑3HBCDs in organisms. A Pearson correlation matrix was used to check a correlation between MPs ingestion and the bioaccumulation of PBDEs and HBCDs for each species; a statistical significance of 95% was considered to assess the association between variables (i.e., number of MPs ingested per individual and levels of ∑10PBDEs and ∑3HBCDs). Statistical analyses were performed using the statistical R-software (R-Cran project, http://cran.r-project.org/).
Results
MPs Analysis
Microplastic particles were never found in any of the 25 analyzed fish muscles, whereas at least one particle was extracted in 21 of the 57 analyzed gastrointestinal tracts (GITs, 36.8% positive to MPs) and in seven out of 38 P. clarkii specimens (18%). Overall, 42 MPs were detected, of which 32 from GIT and 10 from tissues of crayfish (Supplementary Table 4).
The average number of MPs extracted from organisms ranged between 1 ± 0 MP/individual (R. rutilus of Piediluco and P. fluviatilis of Trasimeno) and 2.5 ± 1.9 MPs/individual (P. fluviatilis of Piediluco) (Figure 2A and Supplementary Table 4). One MP was found in the 79% of organisms, 2 and 4 MPs in the 7%, and 3 and 5 MPs in the 3.5% of analyzed specimens.
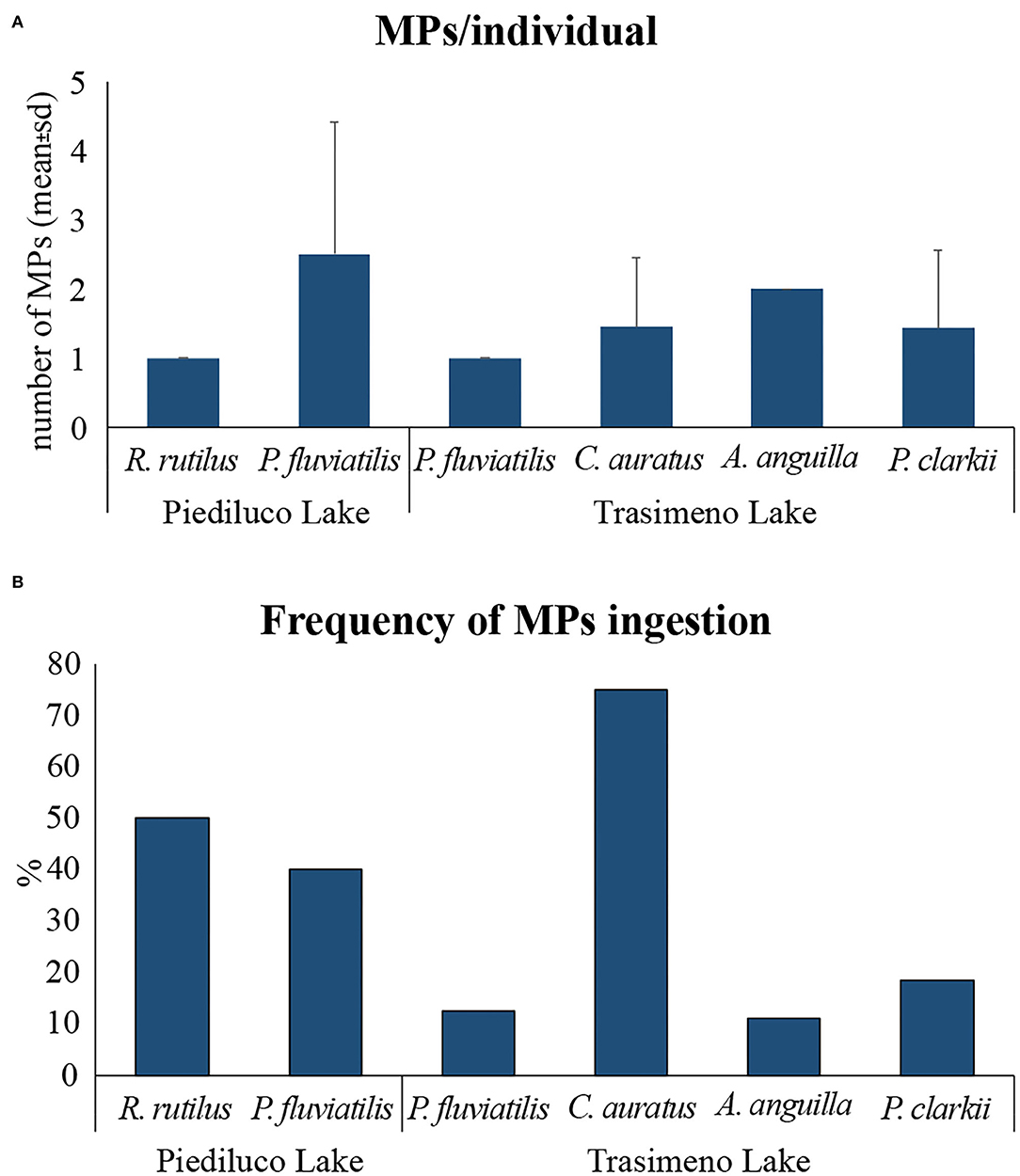
Figure 2. MPs extracted from the gastrointestinal tract of fishes and from the whole soft body of crayfishes sampled in Piediluco and Trasimeno lakes: (A) number of ingested MPs per individual (mean ± sd), (B) frequency of MPs ingestion (%).
The percentage of organisms positive to MPs ingestion was 45% in Piediluco Lake and 25% in Trasimeno Lake where C. auratus was the species showing the highest frequency of ingestion (75%), whereas A. anguilla the lowest (11%). P. fluviatilis caught in Piediluco showed a higher percentage of individuals with MPs in the GITs (40%) compared to those collected in Trasimeno Lake (13%) (Figure 2B and Supplementary Table 4), although the number of ingested MPs did not significantly differ between specimens collected in the different lakes (Kruskal–Wallis test, p > 0.05).
Fiber-shaped MPs were dominant, representing the 93 and 81% of the overall MPs extracted from fish of Piediluco and Trasimeno Lake, respectively, whereas the remaining particles were fragments. P. fluviatilis caught in Piediluco ingested exclusively fiber-shaped MPs, whereas fibers and fragments contributed equally to the total MPs extracted from P. fluviatilis sampled in Trasimeno (Figure 3A and Supplementary Table 4).
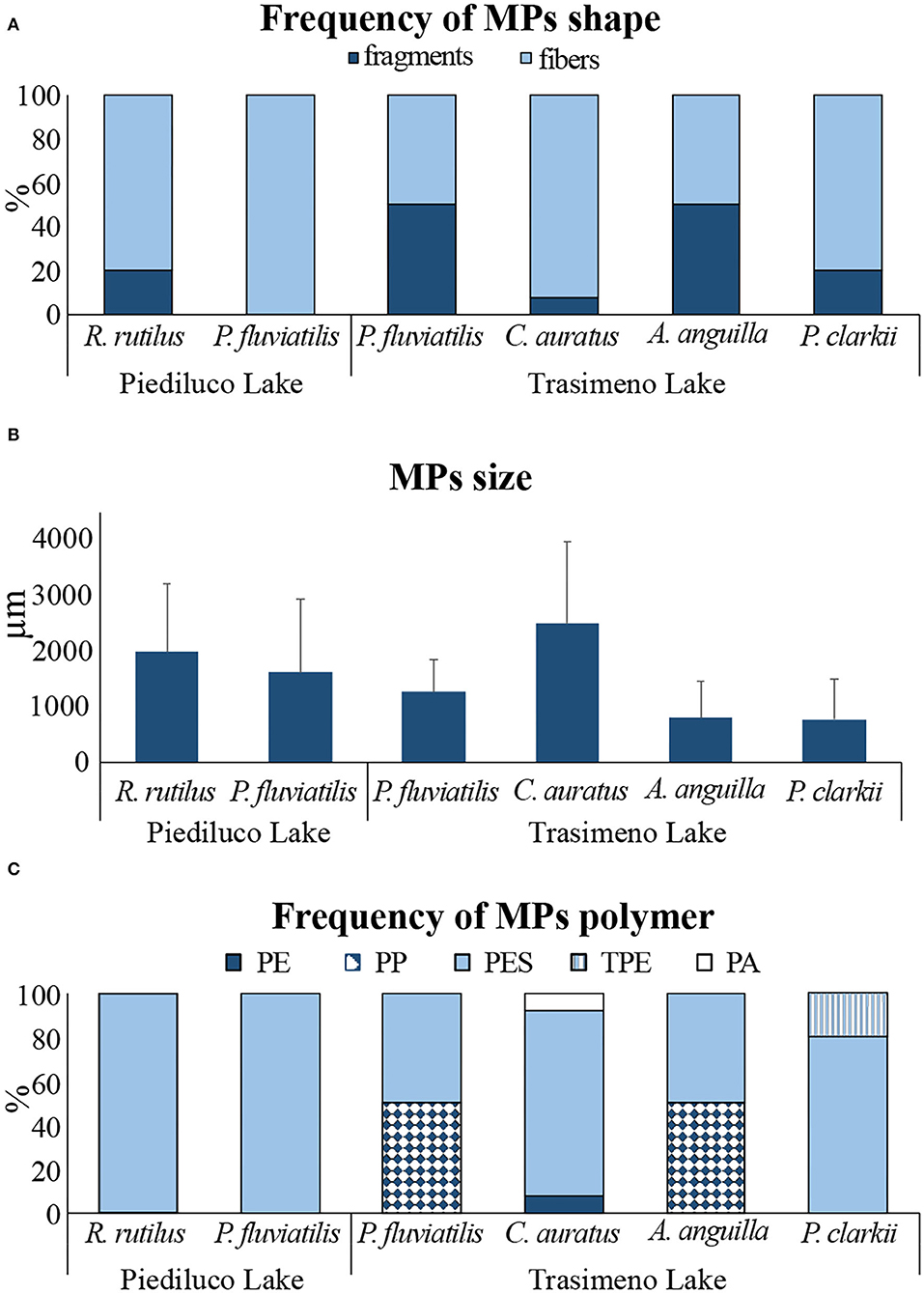
Figure 3. Characterization of MPs extracted from the gastrointestinal tract of fish and from the whole soft body of crayfish sampled in Piediluco and Trasimeno lakes: (A) relative contribution of shapes (%), (B) size of ingested MPs per individual (mean ± sd), (C) relative contribution of polymers (%).
The high percentage of fibers influenced both the average size of MPs, ranging from 765 ± 722 μm in P. clarkii to 2,490 ± 1,475 μm in C. auratus (Figure 3B and Supplementary Figure 1), and the high incidence of PES (Figure 3C and Supplementary Table 4). The smallest microplastic found was a fragment of 14 μm extracted from a specimen of C. auratus. Fish from Piediluco ingested exclusively PES-MPs, whereas species sampled in Trasimeno ingested also other polymers: polypropylene (PP) was identified as constituent of 50% of the MPs extracted from both P. fluviatilis and A. anguilla, thermoplastic elastomer (TPE) represented the 20% of MPs found in P. clarkii, and polyamide (PA) and polyethylene (PE) were extracted from specimens of C. auratus, each one contributing to the 7.7% of total characterized MPs (Figure 3C and Supplementary Table 4).
A noteworthy result is the high percentage of cellulosic (e.g., cotton, linen, and kapok) and animal (e.g., wool, cashmere, and angora) fibers that was found in organisms in respect to those of synthetic nature: of the overall fibers extracted from tissues (n = 218), only the 17% (n = 37) were identified as synthetic after the μFTIR characterization, therefore classified as microplastics.
PBDEs and HBCDs Analysis
Polybrominated diphenyl ethers were detected at non-negligible levels in both species sampled in Piediluco Lake and, on average, the measured concentrations were one or two orders of magnitude higher than those found in species from Trasimeno (Figures 4A,B).
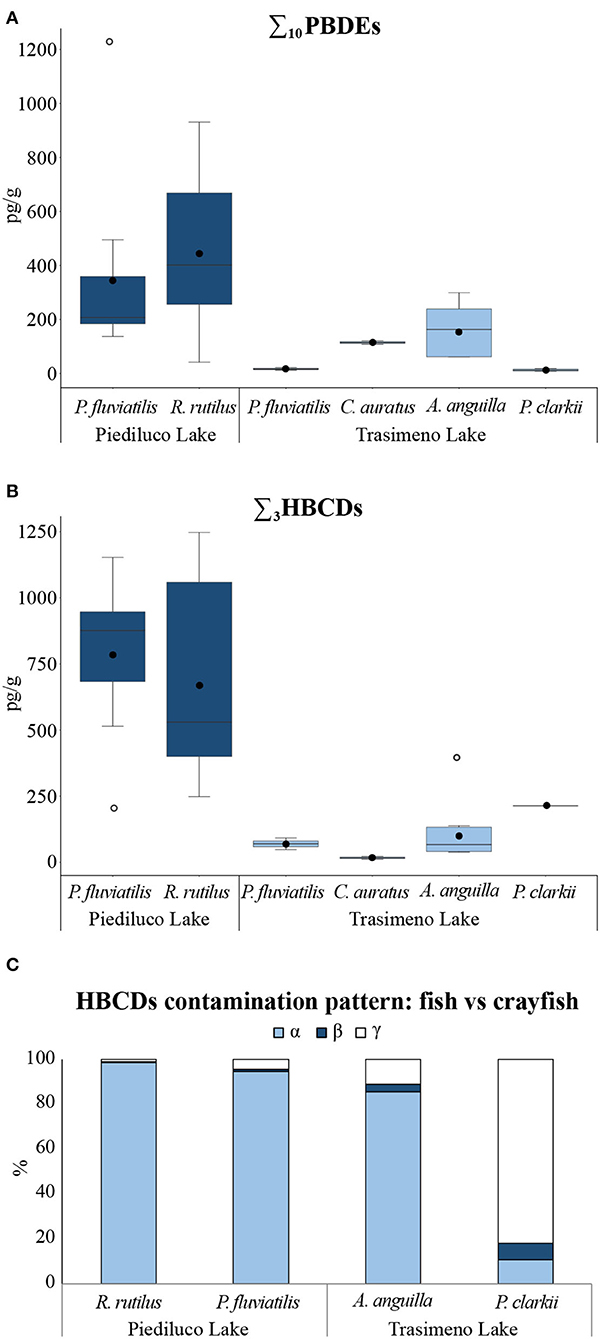
Figure 4. (A) Box plots of (A) ∑10PBDEs concentrations (pg/g) and (B) ∑3HBCDs concentrations (pg/g), in muscle of fishes and in whole soft body of crustaceans sampled in Piediluco and Trasimeno lakes (Italy): the plots depict the lower, median, upper quartile observation, any outliers (empty circles), and mean values (full circles) in the data set; the extensions (“whiskers”) at either ends of the box indicate the minimum and maximum values; (C) HBCDs contamination patterns (abundance ratio for the α-, β-, γ-HBCD isomers) between fishes and crayfishes sampled in Piediluco and Trasimeno lakes (Italy).
In R. rutilus, the ∑10PBDEs ranged from a minimum concentration of 42 pg/g to a maximum of 931 pg/g (mean = 445 pg/g). BDE 47 was the most relevant accumulated congener, and it was measured in all the analyzed specimens along with BDE100 > 154 > 49> 28; only in one sample, BDE209 was measured >LOQ (151 pg/g) (Figure 5A and Supplementary Table 5).
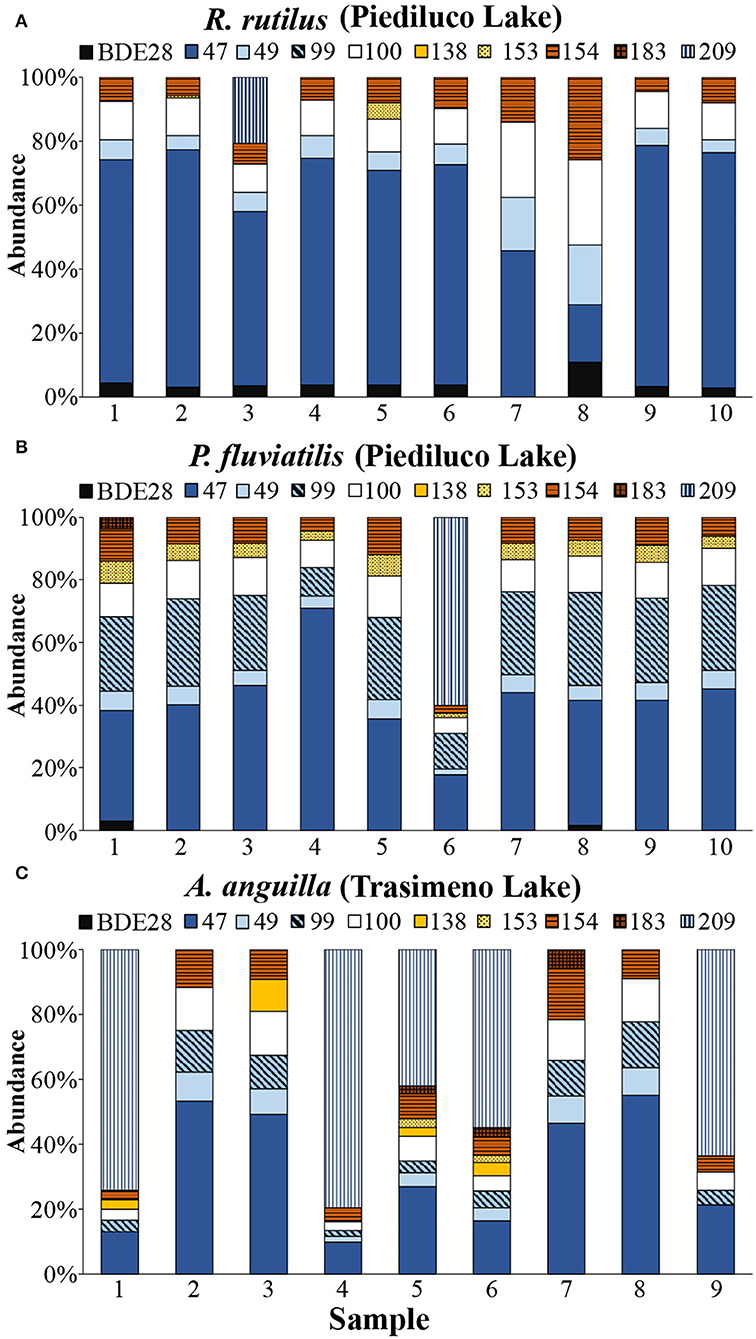
Figure 5. PBDEs contamination patterns in muscle of (A) Rutilus rutilus and (B) Perca fluviatilis sampled in Piediluco Lake and in (C) Anguilla anguilla sampled in Trasimeno Lake.
Compared to R. rutilus, P. fluviatilis caught in the same lake exhibited a slightly lower mean concentration of ∑10PBDEs (343 pg/g), but both the minimum and maximum values detected for the 10-PBDEs sum were higher (137 and 1,218 pg/g, respectively) (Supplementary Table 5). BDE-47,−100,−154, and−49 congeners were measured in all samples as already observed for R. rutilus; in addition, BDE99 and BDE153 were also detected in each specimen of P. fluviatilis. The latter species showed a contamination pattern characterized by BDE 47> 99> 100> 154> 153> 49; BDE209 was quantified in only one sample (298 pg/g) (Figure 5B and Supplementary Table 5).
Among the species sampled in Trasimeno Lake, the higher levels of PBDEs were measured in A. anguilla: the mean concentration of ∑10PBDEs was 163 pg/g with a minimum of 62 pg/g and a maximum of 299 pg/g (Figure 4A). BDE47, 100, and 99 were above LOQs in all analyzed samples, and BDE49 was quantified in seven out of nine specimens, and in 5 of them, also BDE209 was found at concentrations between 103 and 238 pg/g (Figure 5C and Supplementary Table 5). In P. fluviatilis, C. auratus, and P. clarkii, PBDEs were often below LOQs for almost all the congeners (Figure 4A and Supplementary Table 5).
The bioaccumulation of HBCDs showed the same trend observed for the PBDEs: significantly higher levels of ∑3HBCDs were measured in both species caught in Piediluco Lake compared to those of Trasimeno Lake, and among these, only A. anguilla showed measurable levels of HBCDs in all the analyzed specimens (Figure 4B and Supplementary Table 5).
Specifically, in R. rutilus, the ∑3HBCDs ranged from a minimum concentration of 248 pg/g to a maximum of 1,247 pg/g (mean = 677 pg/g); comparable levels were measured in P. fluviatilis from the same lake (minimum ∑3HBCDs value = 215 pg/g, maximum = 1,153 pg/g, mean =792 pg/g; Figure 4B). In both R. rutilus and A. anguilla, the contamination was almost exclusively imputable to α-HBCD (Figures 4C, 6A,B). In A. anguilla from Trasimeno, the HBCDs sum was on average of 107 pg/g with a minimum level of 39 pg/g and a maximum of 396 pg/g (Figure 4B and Supplementary Table 5); the α-isomer was predominant, accounting overall for the 85.5% of contamination (Figures 4C, 6C). Concerning the other species collected in Trasimeno Lake, measurable levels of HBCDs were found only in few samples mostly slightly above the LOQ (Supplementary Table 5). The only exception was found in one pool of P. clarkii with measurable levels of HBCDs, which showed a concentration of γ-HBCD equal to 175 pg/g (Supplementary Table 5), accounting for the 82% of the total contamination (∑3HBCDs = 214 pg/g). This result highlighted a different HBCDs contamination pattern in crayfishes compared to fishes, being γ > α > β and α > γ > β, respectively (Figure 4C).
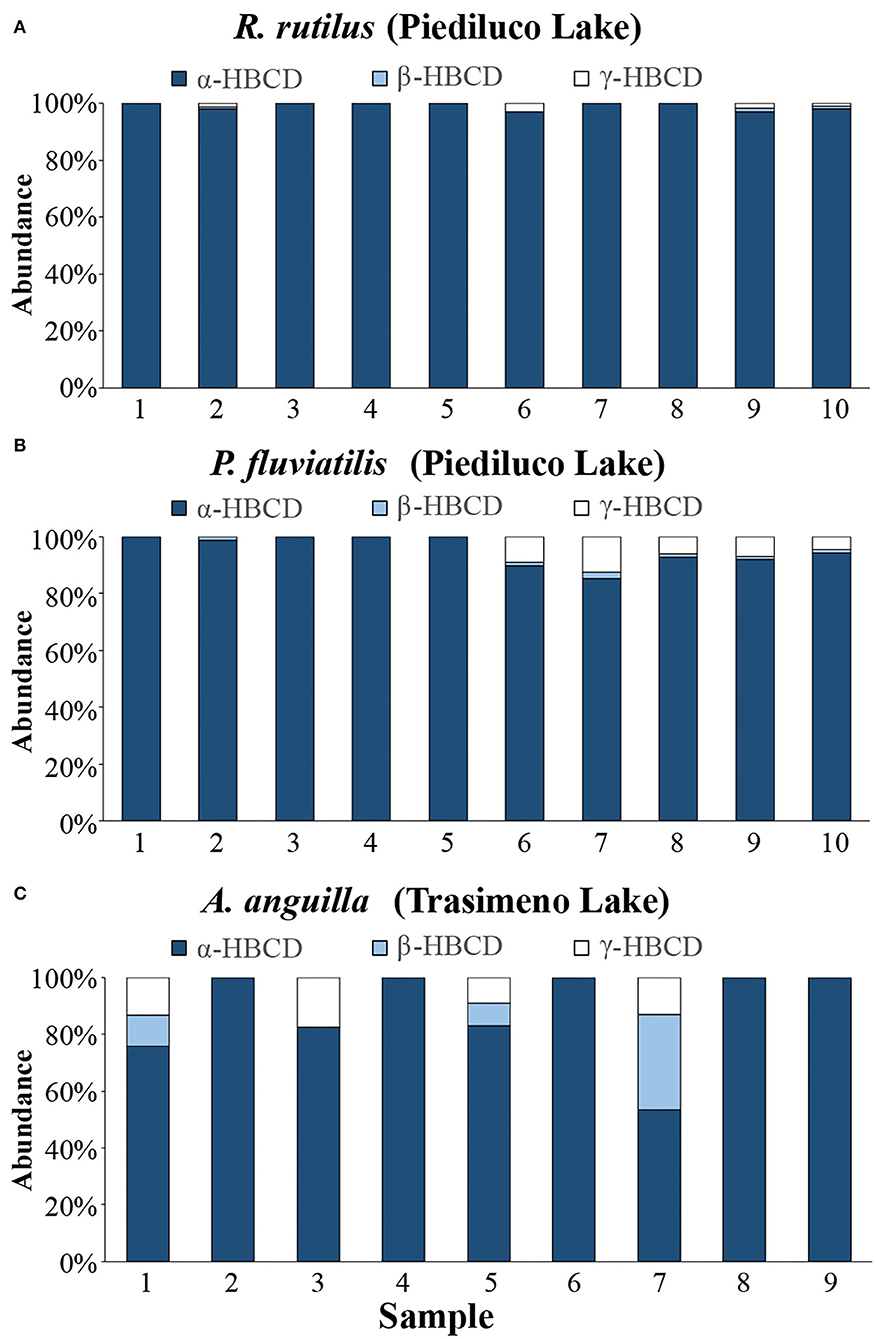
Figure 6. HBCDs contamination patterns (abundance ratio for the α-, β-, γ-HBCD isomers) in muscle of (A) Rutilus rutilus and (B) Perca fluviatilis sampled in Piediluco Lake and in (C) Anguilla anguilla sampled in Trasimeno Lake.
PCA Analysis
The PCA results showed a clear separation between species sampled in Piediluco and Trasimeno Lake along the PC1 axis, which accounted for the 69.6% of the total variance: all the three variables determined this separation; however, the major contribution is given by the levels of PBDEs and HBCDs at the same degree. A further discrimination occurred along the PC2 axis (28.3% of total variance) between C. auratus and the other species sampled in Trasimeno Lake that was exclusively driven by the frequency of MPs ingestion (Figure 7).
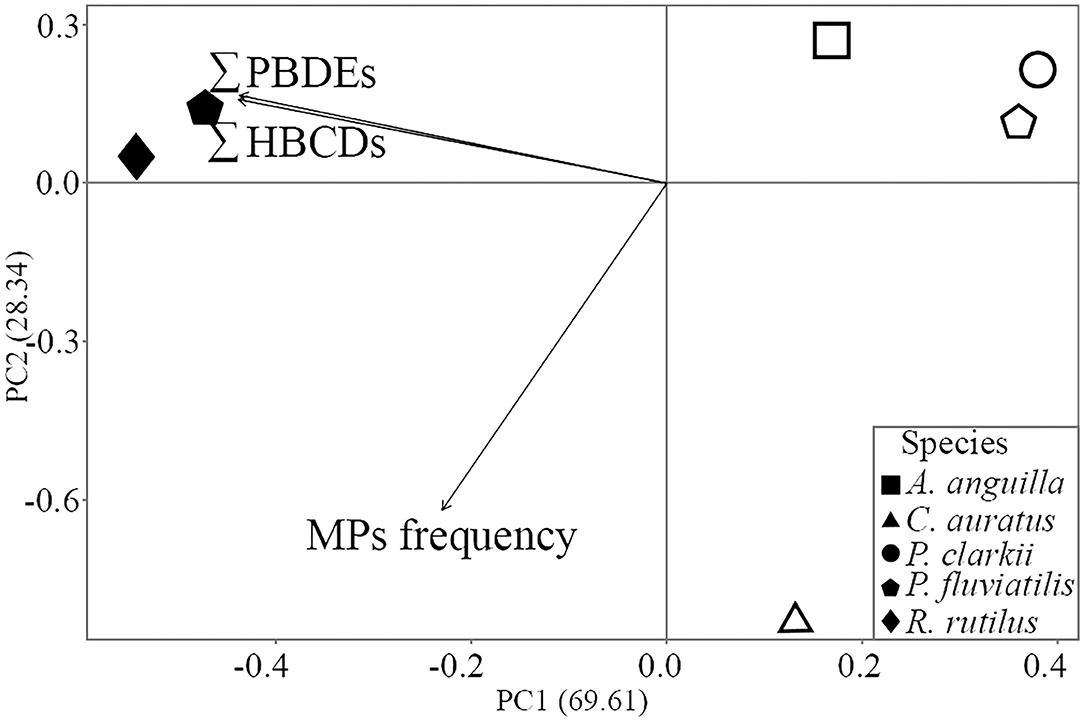
Figure 7. Principal Component Analysis (PCA) ordination based on frequency of MPs ingestion, ∑10 PBDEs and ∑3 HBCDs; Piediluco Lake, filled shapes; Trasimeno Lake, empty shapes; contribution to the separation of each variable is represented by arrows.
Discussion
The MPs and BFRs are considered the two classes of contaminants of emerging concerns in aquatic environments, not only due to the impacts for organisms and ecosystems, but also due to their potential role in food security and food safety, with a direct impact on human health (Barboza et al., 2018).
Despite several investigations which have been carried out in marine and coastal environments, our knowledge is more limited for freshwater species, both in terms of the presence of such contaminants and of potential risk for human health through food consumption.
In this respect, this study provided the first data on the occurrence of MPs and BFRs (i.e., PBDEs and HBCDs) in tissues of fish and crayfish collected from two lakes of Central Italy, with the aim of assessing environmental contamination, food security, and the relationship between MPs ingestion and BFR concentrations.
Analysis of fish GITs and of crayfish whole soft tissues revealed that organisms from both Piediluco and Trasimeno Lakes are exposed to MPs pollution. The presence of MPs in surface waters of Trasimeno was ascertained by a 4-year survey, highlighting an increasing concentration from 8,974 MPs/km2 in 2016 to 25,075 MPs/km2 in 2019 (Goletta dei Laghi campaign, Legambiente-Italy, unpublished data; https://www.legambiente.it/sites/default/files/docs/microplastiche_nei_laghi_2016-2017_legambiente-enea.pdf). No information is available on MPs in waters of Piediluco Lake.
In this study, one particle was found in most individuals positive to ingestion, regardless species and site of collection, consistent with the evidence reported for many other freshwater and marine organisms worldwide, confirming the low number of MPs usually found in aquatic biota (1–4 MPs/organism; Roch et al., 2019). The presence of MPs in GITs is directly dependent not only on their ingestion but also on the retention time of particles in digestive systems (Bour et al., 2020), which is relatively short and influenced by the physical characteristics of MPs, such as size and shape, the presence of food, the gut length, and digestion period (Jabeen et al., 2018; Rochman et al., 2019). Experimental and field studies demonstrated that fiber-shaped microplastics, ingested by amphipods, require longer clearance times than spheres (Ma et al., 2020), and MPs fibers ingested by crabs and lobster can result in the formation of fibrous aggregates within the gut and a reduction in egestion efficiency (Au et al., 2017; Carosi et al., 2019; Cau et al., 2019). Other biological factors, such as size of organisms, may affect the likelihood of microplastic ingestion: larger individuals consume more; in general, due to increased energy demands, therefore, the potential for ingestion of plastic particles may increase as well (Horton et al., 2018). In this study, the size of analyzed organisms did not influence either the number of ingested MPs (Pearson correlation: r = 0.052 and p = 0.92 for correlation with length; r = 0.37 and p = 0.47 for correlation with weight) or the frequency of ingestion (Pearson correlation: r = 0.77 and p = 0.23 for correlation with length; r = 0.7 and p = 0.3 for correlation with weight). Previous studies relating fish size to microplastic ingestion varied in results, showing both a positive correlation (Alomar et al., 2017; Horton et al., 2018) and any significant correlation (Vendel et al., 2017; Avio et al., 2020). The great majority of microplastics extracted in organisms sampled in Piediluco and Trasimeno lakes were fibers, in accordance with other environmental surveys and higher frequency of fibers ingestion compared to any other typology of microplastics, observed in a variety of both freshwater (O'Connor et al., 2020) and marine organisms (Barboza et al., 2018).
The prevalence of polyester fibers and the occurrence of polyamide fibers in C. auratus suggest a textile source in the two lakes more than fragmentation of other plastic products. Although fishing and agriculture are relevant activities both in Piediluco and in Trasimeno lakes, the utilized nets and ropes are mainly made of polypropylene and polyethylene (Coyle et al., 2020), which in our study have been identified only for fragment shaped MPs. In addition, several natural fibers, cellulose- and animal-based, were also found in fishes and crayfishes analyzed in this study (83% of the total extracted).
Also, based on their appearance (mostly were dyed fibers), it can be assumed a man-made textiles derivation for these natural fibers like it was suggested for synthetic ones; therefore, common pathways of release in the environment are plausible, i.e., related to sewage treatment plants, industrial effluents, stormwater, and wind transport. MPs may represent a potential threat to organisms when ingested, because of the physical effects and the possibility to adsorb and release chemical compounds (Kwak et al., 2022). Previous studies indicated that the ingestion of MPs can cause direct effect on marine organism, including histopathological alterations, inflammation, gut blockage, and false satiation, preventing assimilation of ingested foods and resulting in starvation (Bour et al., 2020), with a consequent indirect effect on food quality, reducing the nutritional value of filets or affecting color and texture of fish (A9Amonaite et al., 2018). The potential effects of MPs may have implications on food safety and reduce the fish stocks available for humans in the long period (McIlwraith et al., 2021).
The potential of MPs to be translocated from the GIT to other tissues, to be transferred along the food chain, and to release associate chemicals, will increase with longer retention times, also enhancing the risk for human consumers (Bour et al., 2020). The retention time for synthetic microfibers can vary from a few hours to a couple of days for small invertebrates, such as daphnids, gammarids, sea anemones, and shrimps, and from a few hours to up to 3 weeks for crabs and fish species (Bour et al., 2020); C. auratus has been shown to retain microfibers in the GIT for 6 days after exposure via diet, although internal translocation to other tissues was never observed (Grigorakis et al., 2017). This study never evidenced a MPs transfer from the GIT to the muscle, since neither fragments nor fibers, >10 μm, were found in this tissue for any of analyzed fish species, contrary to what previously observed by other authors (Abbasi et al., 2018; Akhbarizadeh et al., 2018; Haave et al., 2021). These results do not exclude the possible accumulation of smaller microplastics, or even nanoplastics, the assessment of which is actually hampered by technical limitation of the visual sorting and of analytical techniques, such as μFTIR, typically used to characterize polymer typology (Gillibert et al., 2019). Because the gut and stomach contents of fish are not typically eaten by humans, our results demonstrated that the risk of consuming plastic particles is negligible for fish sampled in Trasimeno Lake where commercial fishing is allowed. On the other side, P. clarkii may represent a direct source of MPs intake for consumers, since the whole tissues are eaten; however, the limited number of detected particles and low frequency of ingestion confirm a limited risk of MPs human consumption.
Besides affecting the retention time in the digestive system and egestion, shape of MPs may influence the foraging preferences of organisms. Only microfibers were found in the GIT of C. auratus after exposure via diet to different types of MPs: fish did not ingest food containing fragments and pellets, which were chewed and expelled (Jabeen et al., 2018), suggesting a possible selective behavior in the ingestion of fiber-shaped MPs over others. This may explain the much greater contribution of fibers (82%) than fragments (8%) observed in C. auratus compared to other species collected in Trasimeno Lake. In addition, the feeding behavior of C. auratus may justify the high frequency of specimens with MPs in the gastrointestinal tract (75%): as omnivorous fish with wider range of food sources, which normally swim and forage in different water layers, is more likely to encounter and ingest microplastics than the carnivores P. fluviatilis and A. anguilla, which are, moreover, benthivores such as P. clarkii. The higher susceptibility to MPs ingestion of generalist and benthopelagic species compared to other feeding mode and habit has already been documented (Vendel et al., 2017; Avio et al., 2020).
Given the low frequency of ingestion in the other species of Trasimeno Lake (13–18%) and the high MPs occurrence in C. auratus, demonstrated also in other lakes (Yuan et al., 2019), we can assume that C. auratus is particularly susceptible to ingestion of MPs, even in low impacted environments such as in Trasimeno Lake. Therefore, C. auratus could be suggested as target sentinel species for tracking MPs pollution in inland waters, which fulfills most of the criteria required for bioindicators: it tolerates a range of conditions, accepts different diets, and it is routinely used in ecotoxicology studies (Jabeen et al., 2018). Our results demonstrated that also P. fluviatilis is a suitable bioindicator to monitor the presence of MPs in freshwaters and to discriminate geographical differences at local level (Galafassi et al., 2021). Once the influence of size on MPs ingestion was excluded, we can assume that the higher frequencies of ingestion measured in P. fluviatilis from Piediluco (40%) compared to Trasimeno (13%) may reflect the surrounding environmental contamination, probably related to the greater anthropic pressure and the continuous inflow of Nera and Velino rivers, representing possible sources of MPs pollution in this small lake. To interpret data on MPs accumulation in biota as comprehensive as possible, it is thus necessary considering both level of microplastics in the surrounding environment and biological factors, including size of organisms, feeding habits, living habitat, sex, and different taxonomic groups (fish vs. invertebrates), being all factors that may influence results and discrepancies among species.
Both P. fluviatilis and R. rutilus from Piediluco showed significantly higher accumulation of PBDEs and HBCDs in respect to all the species sampled from the Trasimeno Lake. PBDEs in P. fluviatilis from Piediluco are comparable with the levels measured by Burreau et al. (2004) in Lumparn estuary (Baltic Sea), in rural areas from Finland (Σ17PBDE median = 310 pg/g; from 25 to 2,770 pg/g), or by Fliedner et al. (2018) along the river Danube (660–1,360 pg/g). The levels measured in Piediluco Lake are anyway lower than those measured in P. fluviatilis by Hajšlová et al. (2007) and Pulkrabová et al. (2007) in Czech Republic (mean of 3,900 and of 1,260 pg/g, respectively). Literature data are more limited for HBCDs but our results show lower contamination levels compared to those of Hajšlová et al. (2007) (mean = 3,900 pg/g; min = nq and max = 16,100 pg/g) and Pulkrabová et al. (2007) (mean = 720 pg/g; min = nq and max = 1,590 pg/g) in fish from Elbe river.
All the species caught in the Trasimeno Lake had been previously analyzed for PBDEs and HBCDs (Tavoloni et al., 2021), and the here presented results confirmed that C. auratus, P. fluviatilis, and P. clarkii are mostly not contaminated from these BFRs. In A. Anguilla, lower levels were measured in this study in respect to what previously reported with the ∑10PBDEs, ranging between 62 and 299 pg/g, whereas the ∑15PBDEs measured by Tavoloni et al. (2021) was between 265 and 912 pg/g. As shown in Supplementary Table 5, the sum of the 15 BDE congeners and of the 10 indicators is coincident, confirming that the 10 indicators account for the large part of BDE contamination. The different contamination level found in the two studies may reflect age and/or dimension since the specimens included in this study had a half average length (41 ± 7 cm) compared to previous paper, while similar average weights (154 ± 115 vs. 118 ± 268 g). Otherwise, a second explanation may be found in the possible Trasimeno Lake re-population practice, which foresees the re-introduction of specimens hailing from other freshwater basins, which could justify the significant difference in contamination levels. Anyway, the PBDE and HBCDs contamination patterns were very similar in the two studies. The PBDE congeners abundance was 47 > 100 > 154 > 49 ~ 99 confirming the already highlighted predominance of BDE 154 on the BDE 99 also in case of low contamination levels. The BDE 99 is usually found in other species as the third abundant congener after the BDE 47 and 100. Previously published studies reported similar PBDE profiles in A. anguilla, justifying the high BDE47/BDE99 ratio (>15) as a result of (i) different assimilation efficiencies, (ii) eels metabolic pathways leading to bio-transformation of BDE-99 into−47 or (iii) specific elimination metabolism in eel for the BDE99 congener (Malarvannan et al., 2014; Couderc et al., 2015). Moreover, in this study, A. anguilla samples also exhibited the BDE 209 which was never above LOQ in the paper from Tavoloni et al. (2021).
In P. clarkii, pools belonging to this study BDEs were confirmed as below LOQs for all the congeners in all the pools except for traces of BDE47 measured in two samples. HBCD isomers were all below LOQs in all the samples but one, unlike in Tavoloni et al. (2021) in which high levels were measured in half of the considered pools. In the only pool in which HBCDs were measured, the previous contamination pattern was confirmed with the γ isomer >α>β. At this time, it was not possible to find the literature data on PBDEs in C. auratus from Italian freshwater basins. Comparing our results with those obtained in other European countries, P. fluviatilis from Trasimeno Lake were considerably less contaminated than P. fluviatilis from Baltic Sea, Czech rivers, and German Danube (Burreau et al., 2004; Hajšlová et al., 2007; Pulkrabová et al., 2007; Fliedner et al., 2018), confirming the Trasimeno Lake, an area of low anthropogenic impact.
Besides the importance to monitor BFRs in biota as the indicator of environmental quality of freshwater bodies in the context of the EU Water Framework Directive (WFD, 2000/60/EC amended by 2013/39/EU directive), the assessment of these hydrophobic substances in organisms intended for human consumption is a priority also for implications in food security.
In the absence of regulatory limits in food, BFR levels measured in fish and crustaceans from Trasimeno and Piediluco lakes were compared with threshold values of Environmental Quality Standards (EQSs) fixed by the WFD: EQS for PBDEs in biota (referred to the sum of congeners BDE-28,−47,−99,−100,−153, and−154) has been set at 0.0085 ng/g wet weight (w.w.) while for HBCDs at 167 ng/g w.w.
Mean concentrations for BDEs (∑6PBDEs = sum of 28, 47, 99, 100, 153, and 154 congeners) in most of the species from Trasimeno Lake (P. fluviatilis, C. auratus, and P. clarkii) were below 0.0085 ng/g, except for A. anguilla (mean ∑6PBDEs = 0.070 ng/g), which exceed the EQS about one order of magnitude. The higher levels measured in both the species from Piediluco Lake exceeded the EQSs set for BDEs: ∑6PBDEs reached 1.099 ng/g in P. fluviatilis (mean = 0.291 ng/g) and 0.893 ng/g in R. rutilus (mean = 0.404 ng/g), respectively. It should be noted, however, that it has been expressed criticism toward the EQS reference values for biota contamination assessment (Szlinder-Richert et al., 2014; Ábalos et al., 2019) since in Europe, between 73 and 100% of analyzed fish exceeded these values for BDEs (Eljarrat and Barceló, 2018).
According to EFSA (2011), relevant toxicity data for BDEs are available only for the congeners−47,−99,−153, and−209 characterized by body burdens of 172, 4.2, and 9.6 ng/kg bw/day and 1,700 mg/kg bw/day, respectively. Concerning HBCDs (EFSA Panel on Contaminants in the Food Chain (CONTAM), 2021), a chronic human dietary intake of 2.35 μg/kg bw/day was defined for the sum of –α, -β, and –γ isomers. Freshwater fish consumption was estimated by Roila et al. (2021) in 0.47 g/kg bw/day. The estimated BFRs intakes calculated from the concentration measured in this study were still far from the safety limit defined by EFSA for all the compounds. BDE-47 EDI ranged from 0.0021 (P. fluviatilis from Trasimeno) to 0.177 ng/kg bw/day (R. rutilus from Piediluco), whereas maximum values of 0.036 and 0.008 ng/kg bw/day for BDE-99 and−153 were, respectively, calculated both in P. fluviatilis of Piediluco. In case of BDE-209, the higher EDI (0.038 ng/kg bw/day) was calculated for A. anguilla. As regards HBCDs, the dietary intake for the sum of the three isomers ranged from a minimum of 0.0013 in C. auratus to a maximum of 0.372 ng/kg bw/day in P. fluviatilis caught in Piediluco.
Even though both MPs and BFRs showed higher levels in fish species from Piediluco Lake, a correlation between MPs ingestion and levels of PBDEs and HBCDs was not observed (Supplementary Table 6). In this respect, we can assume that the higher levels of BFRs measured in Piediluco species are a consequence of the local environmental pollution, excluding a direct role of ingested MPs as vectors of these contaminants. However, the consequences of MPs on food security are still largely unknown, due to the lack of accurate estimation of MPs intake via the human food chain, along with uncertain toxicological interactions between MPs and other contaminants such as BFRs. Further studies are thus required to make more realistic human exposure assessments to MPs, to evaluate possible MPs translocation and accumulation in tissues and organs of humans, and the potential toxicological mechanisms of MPs on human health (Li et al., 2021).
Data Availability Statement
The original contributions presented in the study are included in the article/Supplementary Material, further inquiries can be directed to the corresponding author.
Ethics Statement
Ethical review and approval was not required for the animal study because fish species used for the study were obtained from fish market.
Author Contributions
LP was responsible for microplastics analysis, wrote original draft, and reviewed and edited the manuscript. TT was responsible for funding acquisition, conceptualized the study, wrote original draft, and reviewed and edited the manuscript. LV carried out extraction and characterization of microplastics from organisms and elaborated results. AS was responsible for brominated flame retardants analysis, data curation, and reviewed and edited the manuscript. Gd'E carried out statistical elaboration of data. GLV was responsible for fish sampling in Piediluco and Trasimeno lakes and conceptualized the study. FR reviewed and edited the manuscript. AP was responsible for funding acquisition, project administration, conceptualized the study, wrote original draft, and reviewed and edited the manuscript. SG conceptualized the study, wrote original draft, and reviewed and edited the manuscript. All authors contributed to the article and approved the submitted version.
Funding
This work was the part of the project MicroPLASTICs in edible aquatic organisms: ecotoxicological effects, transfer of chemical and biological CONtaminants and susceptibility to bacteria biodegradation (PLASTICON) (RF-2019-12370587), supported by the Italian Ministry of Health, Progetti ordinari di ricerca finalizzata, 2019.
Conflict of Interest
GLV was employed by Azienda USL Umbria 1.
The remaining authors declare that the research was conducted in the absence of any commercial or financial relationships that could be construed as a potential conflict of interest.
Publisher's Note
All claims expressed in this article are solely those of the authors and do not necessarily represent those of their affiliated organizations, or those of the publisher, the editors and the reviewers. Any product that may be evaluated in this article, or claim that may be made by its manufacturer, is not guaranteed or endorsed by the publisher.
Acknowledgments
We are grateful to Paolo Meazzini, Federica Lisi, Lisa Elisei (Azienda USL Umbria 1, Perugia), and Danilo Serva (Azienda USL Umbria 2, Terni) carrying out fish sampling in Piediluco lake.
Supplementary Material
The Supplementary Material for this article can be found online at: https://www.frontiersin.org/articles/10.3389/frwa.2022.902885/full#supplementary-material
References
Ábalos, M., Barceló, D., Parera, J., la Farré, M., Llorca, M., Eljarrat, M., et al. (2019). Levels of regulated POPs in fish samples from the Sava River Basin. Comparison to legislated quality standard values. Sci. Tot. Environ. 647, 20–28. doi: 10.1016/j.scitotenv.2018.07.371
Abbasi, S., Soltani, N., Keshavarzi, B., Moore, F., Turner, A., and Hassanaghaei, M. (2018). Microplastics in different tissues of fish and prawn from the Musa Estuary, Persian Gulf. Chemosphere 205, 80–87. doi: 10.1016/j.chemosphere.2018.04.076
Akhbarizadeh, R., Moore, F., and Keshavarzi, B. (2018). Investigating a probable relationship between microplastics and potentially toxic elements in fish muscles from northeast of Persian Gulf. Environ. Pollut. 232, 154–163. doi: 10.1016/j.envpol.2017.09.028
Alaee, M., Arias, P., Sjödin, A., and Bergman, Å. (2003). An overview of commercially used brominated flame retardants, their applications, their use patterns in different countries/regions and possible modes of release. Environ. Int. 29, 683–689. doi: 10.1016/S0160-4120(03)00121-1
Alomar, C., Sureda, A., Capó, X., Guijarro, B., Tejada, S., and Deudero, S. (2017). Microplastic ingestion by Mullus surmuletus Linnaeus, 1758 fish and its potential for causing oxidative stress. Environ. Res., 159, 135–142. doi: 10.1016/j.envres.2017.07.043
A9Amonaite, G., Larsson, K., Undeland, I., Sturve, and J Carney Almroth, B. (2018). Size matters: ingestion of relatively large microplastics contaminated with environmental pollutants posed little risk for fish health and fillet quality. Environ. Sci. Technol. 52, 14381–14391. doi: 10.1021/acs.est.8b04849
Au, S. Y., Lee, C. M., Weinstein, J. E., van den Hurk, P., and Klaine, S. J. (2017). Trophic transfer of microplastics in aquatic ecosystems: identifying critical research needs. Integr. Environ. Assess. Manag. 13, 505–509. doi: 10.1002/ieam.1907
Avio, C. G., Cardelli, L. R., Gorbi, S., Pellegrini, D., and Regoli, F. (2017). Microplastics pollution after the removal of the Costa Concordia wreck: first evidences from a biomonitoring case study. Env. Poll. 227, 207–214. doi: 10.1016/j.envpol.2017.04.066
Avio, C. G., Gorbi, S., Milan, M., Benedetti, M., Fattorini, D., d'Errico, G., et al. (2015a). Pollutants bioavailability and toxicological risk from microplastics to marine mussels. Env. Poll., 198, 211–222. doi: 10.1016/j.envpol.2014.12.021
Avio, C. G., Gorbi, S., and Regoli, F. (2015b). Experimental development of a new protocol for extraction and characterization of microplastics in fish tissues: first observations in commercial species from Adriatic Sea. Mar. Environ. Res. 111, 18–26. doi: 10.1016/j.marenvres.2015.06.014
Avio, C. G., Pittura, L., d'Errico, G., Abel, S., Amorello, S., Marino, M., et al. (2020). Distribution and characterization of microplastic particles and textile microfibers in Adriatic food webs: general insights for biomonitoring strategies. Environ. Pollut. 258:113766. doi: 10.1016/j.envpol.2019.113766
Barboza, L. G. A., Vethaak, A. D., Lavorante, B. R., Lundebye, A. K., and Guilhermino, L. (2018). Marine microplastic debris: an emerging issue for food security, food safety and human health. Mar. Pollut. Bull. 133, 336–348. doi: 10.1016/j.marpolbul.2018.05.047
Bessa, F., Frias, J., Kögel, T., Lusher, A., Andrade, J. M., Antunes, J., et al. (2019). Harmonized Protocol for Monitoring Microplastics in Biota. European Union Funded Practices. doi: 10.13140/RG.2.2.28588.72321/1
Bour, A., Avio, C. G., Gorbi, S., Regoli, F., and Hylland, K. (2018). Presence of microplastics in benthic and epibenthic organisms: influence of habitat, feeding mode and trophic level. Env. Pollut. 243, 1217–1225. doi: 10.1016/j.envpol.2018.09.115
Bour, A., Hossain, S., Taylor, M., Sumner, M., and Carney Almroth, B. (2020). Synthetic microfiber and microbead exposure and retention time in model aquatic species under different exposure scenarios. Front. Environ. Sci. 2020:83. doi: 10.3389/fenvs.2020.00083
Burreau, S., Zebühr, Y., Broman, D., and Ishaq, R. (2004). Biomagnification of polychlorinated biphenyls (PCBs) and polybrominated diphenyl ethers (PBDEs) studied in pike (Esox lucius), perch (Perca fluviatilis) and roach (Rutilus rutilus) from the Baltic Sea. Chemosphere 55, 1043–1052. doi: 10.1016/j.chemosphere.2003.12.018
Carosi, A., Ghetti, L., Padula, R., and Lorenzoni, M. (2019). Potential effects of global climate change on fisheries in the Trasimeno Lake (Italy), with special reference to the goldfish Carassius auratus invasion and the endemic southern pike Esox cisalpinus decline. Fish. Manag. Ecol. 26, 500–511. doi: 10.1111/fme.12318
Cau, A., Avio, C. G., Dessì, C., Follesa, M. C., Moccia, D., Regoli, F., et al. (2019). Microplastics in the crustaceans Nephrops norvegicus and Aristeus antennatus: flagship species for deep-sea environments? Env. Pollut. 255:113107. doi: 10.1016/j.envpol.2019.113107
Cau, A., Avio, C. G., Dessì, C., Moccia, D., Pusceddu, A., Regoli, F., et al. (2020). Benthic crustacean digestion can modulate the environmental fate of microplastics in the deep sea. Environ. Sci. Technol. 54, 4886–4892. doi: 10.1021/acs.est.9b07705
Couderc, M., Poirier, L., Zalouk-Vergnoux, A., Kamari, A., Blanchet-Letrouvé, I., Marchand, P., et al. (2015). Occurrence of POPs and other persistent organic contaminants in the European eel (Anguilla anguilla) from the Loire estuary, France. Sci. Tot. Environ. 505, 199–215. doi: 10.1016/j.scitotenv.2014.09.053
Coyle, R., Hardiman, G., and O'Driscoll, K. (2020). Microplastics in the marine environment: a review of their sources, distribution processes, uptake and exchange in ecosystems. Case Stud. Therm. Eng. 2:100010. doi: 10.1016/j.cscee.2020.100010
Dal Bosco, A., Mugnai, C., Mourvaki, E., and Castellini, C. (2012). Seasonal changes in the fillet fatty acid profile and nutritional characteristics of wild Trasimeno Lake goldfish (Carassius auratus L.). Food Chem. 132, 830–834. doi: 10.1016/j.foodchem.2011.11.043
Dezfuli, B. S., Lui, A., Giari, L., Pironi, F., Manera, M., Lorenzoni, M., et al. (2013). Piscidins in the intestine of European perch, Perca fluviatilis, naturally infected with an enteric worm. Fish Shellfish Immunol. 35, 1539–1546. doi: 10.1016/j.fsi.2013.08.023
Dörr, A. J. M., La Porta, G., Pedicillo, G., and Lorenzoni, M. (2006). Biology of Procambarus clarkii (Girard, 1852) in lake Trasimeno. Bull. Fr. Pêche Piscic. 380–381, 1155–1168. doi: 10.1051/kmae:2006018
Du, S., Zhu, R., Cai, Y., Xu, N., Yap, P. S., Zhang, Y., et al. (2021). Environmental fate and impacts of microplastics in aquatic ecosystems: a review. RSC Adv. 11, 15762–15784. doi: 10.1039/D1RA00880C
EFSA (2011). Panel on contaminants in the food chain (CONTAM). Scientific opinion on polybrominated diphenyl ethers (PBDEs) in Food. EFSA J. 9:2156. doi: 10.2903/j.efsa.2011.2156
EFSA (2016). Presence of microplastics and nanoplastics in food, with particular focus on seafood. EFSA J. 14:4501. doi: 10.2903/j.efsa.2016.4501
EFSA Panel on Contaminants in the Food Chain (CONTAM) (2021). Update of the risk assessment of hexabromocyclododecanes (HBCDDs) in food. EFSA J. 19:6421. doi: 10.2903/j.efsa.2021.6421
Eljarrat, E., and Barceló, D. (2018). How do measured PBDE and HCBD levels in river fish compare to the European Environmental Quality Standards? Environ. Res. 160, 203–211. doi: 10.1016/j.envres.2017.09.011
European Commission (2000). Directive 2000/60/EC of the European Parliament and of the Council of 23 October 2000 establishing a framework for Community action in the field of water policy. J. Eur. Commun. 327, 1–72. Available online at: http://faolex.fao.org/docs/pdf/eur23005.pdf
European Commission (2014). Commission Recommendation 2014/118/EU of 3 March 2014 on the monitoring of traces of brominated flame retardants in food. Off. J. Eur. Union. 5:2014. Available online at: http://data.europa.eu/eli/reco/2014/118/oj
Fliedner, A., Rüdel, H., Lohmann, N., Buchmeier, G., and Koschorreck, J. (2018). Biota monitoring under the Water Framework Directive: on tissue choice and fish species selection. Environ. Pollut. 235, 129–140. doi: 10.1016/j.envpol.2017.12.052
Galafassi, S., Sighicelli, M., Pusceddu, A., Bettinetti, R., Cau, A., Temperini, M. E., et al. (2021). Microplastic pollution in perch (Perca fluviatilis, Linnaeus 1758) from Italian south-alpine lakes. Environ. Pollut. 288:117782. doi: 10.1016/j.envpol.2021.117782
Gillibert, R., Balakrishnan, G., Deshoules, Q., Tardivel, M., Magazzù, A., Donato, A., et al. (2019). Raman tweezers for small microplastics and nanoplastics identification in seawater. Environ. Sci. Technol. 53, 9003–9013. doi: 10.1021/acs.est.9b03105
Grigorakis, S., Mason, S. A., and Drouillard, K. G. (2017). Determination of the gut retention of plastic microbeads and microfibers in goldfish (Carassius auratus). Chemosphere 169, 233–238. doi: 10.1016/j.chemosphere.2016.11.055
Haave, M., Gomiero, A., Schönheit, J., Nilsen, H., and Olsen, A. B. (2021). Documentation of microplastics in tissues of wild coastal animals. Front. Environ. Sci. 9:31. doi: 10.3389/fenvs.2021.575058
Hajšlová, J., Pulkrabová, J., Poustka, J., Cajka, T., and Randák, T. (2007). Brominated flame retardants and related chlorinated persistent organic pollutants in fish from river Elbe and its main tributary Vltava. Chemosphere 69, 1195–1203. doi: 10.1016/j.chemosphere.2007.06.030
Horton, A. A., Jürgens, M. D., Lahive, E., van Bodegom, P. M., and Vijver, M. G. (2018). The influence of exposure and physiology on microplastic ingestion by the freshwater fish Rutilus rutilus (roach) in the River Thames, UK. Environ. Pollut., 236, 188–194. doi: 10.1016/j.envpol.2018.01.044
Hung, C., Klasios, N., Zhu, X., Sedlak, M., Sutton, R., and Rochman, C. M. (2020). Methods matter: methods for sampling microplastic and otheranthropogenic particles and their implications for monitoringand ecological risk assessment. Environ. Manag. 17, 282–291. doi: 10.1002/ieam.4325
Iqbal, M., Syed, J. H., Katsoyiannis, A., Malik, R. N., Farooqi, A., Butt, A., et al. (2017). Legacy and emerging flame retardants (FRs) in the freshwater ecosystem: a review. Environ. Res. 152, 26–42. doi: 10.1016/j.envres.2016.09.024
Jabeen, K., Li, B., Chen, Q., Su, L., Wu, C., Hollert, H., et al. (2018). Effects of virgin microplastics on goldfish (Carassius auratus). Chemosphere 213, 323–332. doi: 10.1016/j.chemosphere.2018.09.031
Karami, A., Golieskardi, A., Ho, Y. B., Larat, V., and Salamatinia, B. (2017). Microplastics in eviscerated flesh and excised organs of dried fish. Sci. Rep. 7:5473. doi: 10.1038/s41598-017-05828-6
Kwak, J. I., Liu, H., Wang, D., Lee, Y. H., Lee, J. S., and An, Y. J. (2022). Critical review of environmental impacts of microfibers in different environmental matrices. Comp. Biochem. Physiol. C Toxicol. Pharmacol. 251:109196. doi: 10.1016/j.cbpc.2021.109196
La Porta, G., Angeli, V., Bicchi, A., Carosi, A., Pedicillo, G., Viali, P., and Lorenzoni, M. (2010). Variations in the fish community in lake Piediluco (Italy) caused by changes in the lake's trophic status and the introduction of alien species. J. Appl. Ichthyol. 26, 53–59. doi: 10.1111/j.1439-0426.2010.01498.x
Law, R. J., Kohler, M., Heeb, N. V., Gerecke, A. C., Schmid, P., Voorspoels, S., et al. (2005). Hexabromocyclododecane challenges scientists and regulators. Environ. Sci. Technol. 39, 281A-287A. doi: 10.1021/es053302f
Li, Q., Ma, C., Zhang, Q., and Shi, H. (2021). Microplastics in shellfish and implications for food safety. Curr. Opin. Food Sci. 40, 192–197. doi: 10.1016/j.cofs.2021.04.017
Lin, W., Li, X., Yang, M., Lee, K., Chen, B., and Zhang, B. H. (2018). Brominated flame retardants, microplastics, and biocides in the marine environment: recent updates of occurrence, analysis, and impacts. Adv. Mar. Biol. 81, 167–211. doi: 10.1016/bs.amb.2018.09.007
Ma, H., Pu, S., Liu, S., Bai, Y., Mandal, S., and Xing, B. (2020). Microplastics in aquatic environments: toxicity to trigger ecological consequences. Environ. Pollut. 261:114089. doi: 10.1016/j.envpol.2020.114089
Malarvannan, G., Belpaire, C., Geeraerts, C., Eulaers, I., Neels, H., and Covaci, A. (2014). Assessment of persistent brominated and chlorinated organic contaminants in the European eel (Anguilla anguilla) in Flanders, Belgium: Levels, profiles and health risk. Sci. Total Environ. 482–483, 222–233. doi: 10.1016/j.scitotenv.2014.02.127
McIlwraith, H. K., Kim, J., Helm, P., Bhavsar, S. P., Metzger, J. S., and Rochman, C. M. (2021). Evidence of microplastic translocation in wild-caught fish and implications for microplastic accumulation dynamics in food webs. Environ. Sci. Technol. 55, 12372–12382. doi: 10.1021/acs.est.1c02922
Mikula, P., and Svobodova, Z. (2006). Brominated flame retardants in the environment: their sources and effects (a review). Acta Vet. Brno. 75, 587–599. doi: 10.2754/avb200675040587
Myszograj, M. (2020). Microplastic in food and drinking water-environmental monitoring data. Civil Envir. Eng. Rep. 30, 201–209. doi: 10.2478/ceer-2020-0060
O'Connor, J. D., Mahon, A. M., Ramsperger, A. F., Trotter, B., Redondo-Hasselerharm, P. E., Koelmans, A. A., et al. (2020). Microplastics in freshwater biota: a critical review of isolation, characterization, and assessment methods. Glob. Challenges 4:1800118. doi: 10.1002/gch2.201800118
Piersanti, A., Tavoloni, T., Bastari, E., Lestingi, C., Romanelli, S., and Rossi, R. (2018). A GC-EI-MS/MS method for the determination of 15 polybrominated diphenyl ethers (PBDEs) in fish and shellfish tissues. Food Anal. Methods 11, 355–366. doi: 10.1007/s12161-017-1006-z
Pittura, L., Avio, C. G., Giuliani, M. E., d'Errico, G., Keiter, S. H., Cormier, B., et al. (2018). Microplastics as vehicles of environmental PAHs to marine organisms: combined chemical and physical hazards to the Mediterranean mussels, Mytilus galloprovincialis. Front. Mar. Sci. 5:103. doi: 10.3389/fmars.2018.00103
Prata, J. C., Reis, V., da Costa, J. P., Mouneyrac, C., Duarte, A. C., and Rocha-Santos, T. (2021). Contamination issues as a challenge in quality control and quality assurance in microplastics analytics. J. Hazard. Mater. 403:123660. doi: 10.1016/j.jhazmat.2020.123660
Primpke, S., Christiansen, S. H., Cowger, W., De Frond, H., Deshpande, A., Fischer, M., et al. (2020). Critical assessment of analytical methods for the harmonized and cost-efficient analysis of microplastics. Appl. Spectrosc., 74, 1012–1047. doi: 10.1177/0003702820921465
PSR Umbria (2013). Programma di sviluppo rurale per l'Umbria 2007-2013. Available online at: https://www.regione.umbria.it/documents/18/1216477/PSR+2007_2013+PO+15.pdf/3c26c3c5-0364-406b-afce-ead30018aa0c (accessed January 18, 2022).
Pulkrabová, J., Hajšlová, J., Poustka, J., and Kazda, R. (2007). Fish as biomonitors of polybrominated diphenyl ethers and hexabromocyclododecane in Czech aquatic ecosystems: pollution of the elbe river basin. Environ. Health Perspect. 115, 28–34. doi: 10.1289/ehp.9354
Rocchi, L., Paolotti, L., and Fagioli, F. F. (2017). Defining agri-environmental schemes in the buffer areas of a natural regional park: an application of choice experiment using the latent class approach. Land Use Policy 66, 141–150. doi: 10.1016/j.landusepol.2017.04.033
Roch, S., Walter, T., Ittner, L. D., Friedrich, C., and Brinker, A. (2019). A systematic study of the microplastic burden in freshwater fishes of south-western Germany-Are we searching at the right scale?. Sci. Total Environ. 689, 1001–1011. doi: 10.1016/j.scitotenv.2019.06.404
Rochman, C. M., Brookson, C., Bikker, J., Djuric, N., Earn, A., Bucci, K., et al. (2019). Rethinking microplastics as a diverse contaminant suite. Environ. Toxicol. Chem. 38, 703–711. doi: 10.1002/etc.4371
Roila, R., Branciari, R., Ranucci, D., Stramenga, A., Tavoloni, T., Stecconi, T., et al. (2021). Risk characterization and benefit–risk assessment of brominated flame retardant in commercially exploited freshwater fishes and crayfish of Lake Trasimeno, Italy. Int. J. Environ. Res. Public Health 18:8763. doi: 10.3390/ijerph18168763
Smolarz, K., and Berger, A. (2009). Long-term toxicity of hexabromocyclododecane (HBCDD) to the benthic clam Macoma balthica (L.) from the Baltic Sea. Aquat. Toxicol. 95, 239–247. doi: 10.1016/j.aquatox.2009.09.010
Szlinder-Richert, J., Ruczynska, W., Nermer, T., Usydus, Z., and Robak, S. (2014). The occurrence of organic contaminants in European eel (Anguilla anguilla) in Poland: an environmental quality assessment. Chemosphere 114, 282–290. doi: 10.1016/j.chemosphere.2014.04.042
Tavoloni, T., Stecconi, T., Galarini, R., Bacchiocchi, S., Dörr, A. J. M., Elia, A. C., et al. (2021). BFRs (PBDEs and HBCDs) in freshwater species from Lake Trasimeno (Italy): the singular case of HBCDs in red swamp crayfish. Sci. Total Environ. 758:143585. doi: 10.1016/j.scitotenv.2020.143585
Tavoloni, T., Stramenga, A., Stecconi, T., Siracusa, M., Bacchiocchi, S., and Piersanti, A. (2020). Single sample preparation for brominated flame retardants in fish and shellfish with dual detection: GC-MS/MS (PBDEs) and LC-MS/MS (HBCDs). Anal. Bioanal. Chem. 412, 397–411. doi: 10.1007/s00216-019-02250-x
UNEP (2010). Listing of Decabromodiphenyl Ether, Decis. SC-8/10. Available online at: http://chm.pops.int/Convention/ConferenceofthePartiesCOP/COPDecisions/tabid/208/Default.aspx (accessed January 8, 2019).
UNEP (2013). Listing of Hexabromocyclododecane, Decis. SC-6/13. Available online at: http://chm.pops.int/Convention/ConferenceofthePartiesCOP/COPDecisions/tabid/208/Default.aspx (accessed February 6, 2019).
UNEP (2014). Listing of Hexabromodiphenyl Ether and Heptabromodiphenyl Ether, Decis. SC-4/14. Available online at: http://chm.pops.int/Convention/ConferenceofthePartiesCOP/COPDecisions/tabid/208/Default.aspx (accessed February 4, 2019).
UNEP (2018). Listing of Tetrabromodiphenyl Ether and Pentabromodiphenyl Ether, Decis. SC-4/18. Available online at: http://chm.pops.int/Convention/ConferenceofthePartiesCOP/COPDecisions/tabid/208/Default.aspx (accessed February 4, 2019).
UNEP (2021). From Pollution to Solution: A Global Assessment of Marine Litter and Plastic Pollution. Available online at: https://www.unep.org/resources/pollution-solution-global-assessment-marine-litter-and-plastic-pollution (accessed November 1, 2021).
Vendel, A. L., Bessa, F., Alves, V. E. N., Amorim, A. L. A., Patrício, J., and Palma, A. R. T. (2017). Widespread microplastic ingestion by fish assemblages in tropical estuaries subjected to anthropogenic pressures. Mar. Pollut. Bull., 117, 448–455. doi: 10.1016/j.marpolbul.2017.01.081
Vital, S. A., Cardoso, C., Avio, C., Pittura, L., Regoli, F., and Bebianno, M. J. (2021). Do microplastic contaminated seafood consumption pose a potential risk to human health?. Mar. Pollut. Bull. 171:112769. doi: 10.1016/j.marpolbul.2021.112769
Keywords: microplastics (MPs), brominated flame retardants (BFRs), fish, freshwater, bioaccumulation, human health
Citation: Pittura L, Tavoloni T, Ventura L, Stramenga A, d'Errico G, Lo Vaglio G, Regoli F, Piersanti A and Gorbi S (2022) Microplastics and Brominated Flame Retardants in Freshwater Fishes From Italian Lakes: Implication for Human Health. Front. Water 4:902885. doi: 10.3389/frwa.2022.902885
Received: 23 March 2022; Accepted: 26 May 2022;
Published: 29 June 2022.
Edited by:
Susanne Brander, Oregon State University, United StatesReviewed by:
Yongning Wu, China National Center for Food Safety Risk Assessment, ChinaAlice A. Horton, University of Southampton, United Kingdom
Copyright © 2022 Pittura, Tavoloni, Ventura, Stramenga, d'Errico, Lo Vaglio, Regoli, Piersanti and Gorbi. This is an open-access article distributed under the terms of the Creative Commons Attribution License (CC BY). The use, distribution or reproduction in other forums is permitted, provided the original author(s) and the copyright owner(s) are credited and that the original publication in this journal is cited, in accordance with accepted academic practice. No use, distribution or reproduction is permitted which does not comply with these terms.
*Correspondence: Stefania Gorbi, s.gorbi@univpm.it
†These authors have contributed equally to this work and share first authorship